- 1Zhengzhou Research Base, State Key Laboratory of Cotton Biology, Zhengzhou University, Zhengzhou, China
- 2State Key Laboratory of Cotton Biology, Institute of Cotton Research, Chinese Academy of Agricultural Sciences, Anyang, China
- 3Saint John Paul the Great Catholic High School, Dumfries, VA, United States
Brassinosteroids (BRs), an efficient plant endogenous hormone, significantly promotes plant nutrient growth adapting to biological and abiotic adversities. BRs mainly promote plant cell elongation by regulating gene expression patterns. EXORDIUM (EXO) genes have been characterized as the indicators of BR response genes. Cotton, an ancient crop, is of great economic value and its fibers can be made into all kinds of fabrics. However, EXO gene family genes have not been full identified in cotton. 175 EXO genes were identified in nine plant species, of which 39 GhEXO genes in Gossypium hirsutum in our study. A phylogenetic analysis grouped all of the proteins encoded by the EXO genes into five major clades. Sequence identification of conserved amino acid residues among monocotyledonous and dicotyledonous species showed a high level of conservation across the N and C terminal regions. Only 25% the GhEXO genes contain introns besides conserved gene structure and protein motifs distribution. The 39 GhEXO genes were unevenly distributed on the 18 At and Dt sub-genome chromosomes. Most of the GhEXO genes were derived from gene duplication events, while only three genes showed evidence of tandem duplication. Homologous locus relationships showed that 15 GhEXO genes are located on collinear blocks and that all orthologous/paralogous gene pairs had Ka > Ks values, indicating purifying selection pressure. The GhEXO genes showed ubiquitous expression in all eight tested cotton tissues and following exposure to three phytohormones, IAA, GA, and BL. Furthermore, GhEXO7_At was mainly expressed in response to BL treatment, and was predominantly expressed in the fibers. GhEXO7_At was found to be a plasma membrane protein, and its ectopic expression in Arabidopsis mediated BR-regulated plant growth and development with altered expression of DWF4, CPD, KCS1, and EXP5. Additionally, the functions of GhEXO7_At were confirmed by virus-induced gene silencing (VIGS) in cotton. This study will provide important genetic resources for future cotton breeding programs.
Introduction
Brassinosteroids (BRs), known as the sixth hormone, regulate a host of developmental process of plants including cell elongation and division and cell wall biosynthesis in plants (Yang et al., 2011; Saini et al., 2015). Provides a new way for crop improvement, the endogenous BR level has a significant impact on plant growth and development (Tanaka et al., 2005; Wei and Li, 2020). BR-deficient mutants show extreme dwarfing, delayed senescence, and decreased male fertility (Choe, 2006). BR mainly affects plant traits by promoting cell expansion and show a dose-specific effect in stimulating plant growth. Excessive application of bioactive BR will cause down-regulation of BR biosynthesis genes and up-regulation of BR inactivating genes, thereby hindering normal plant development (Tanaka et al., 2005).
Brassinosteroids in plant growth and development also play a crucial role by regulating gene expression patterns. cDNA and oligonucleotide microarrays have been used to identify BR-regulated genes (Müssig and Altmann, 2003). Some BR-response mediators (such as BEE1, BEE2, BEE3, and BRH1) are regulated at the transcription level by BR (Friedrichsen et al., 2002; Molnar et al., 2002). EXORDIUM (AtEXO) was initially identified as an indicator of BR response genes (Coll-Garcia et al., 2004). EXO was first found in Arabidopsis using a promoter trap insertional mutagenesis system (Farrar et al., 2003). AtEM201 is a transgenic line with a T-DNA inserted into the EXO gene promoter. The relative transcription of EXO was significantly decreased in AtEM201, although the plant phenotype was normal (Farrar et al., 2003). The EXO-GUS fusion protein activity was high in apical meristems and young leaves (Farrar et al., 2003). Similarly, plants that specifically degraded EXO gene mRNA through RNA interference (RNAi) exhibited a normal phenotype (Coll-Garcia et al., 2004). However, exo knockout mutants exhibit a dwarf phenotype due to reduced cell expansion (Schroder et al., 2009). EXO-overexpression plants show enhanced vegetative growth and increased mRNA levels of BR-up-regulated genes, which is similar to the growth phenotype of BR-treated plants (Coll-Garcia et al., 2004). BR-deficient mutants exhibit weak EXO expression, while BR treatment of plants resulted in increased EXO transcript levels (Coll-Garcia et al., 2004). A BR hypersensitive mutant, bes1-D, shows constitutive EXO expression (Yin et al., 2002).
EXO and EXO-Like (EXO/EXL) genes encode eight homologous proteins in Arabidopsis (Schroder et al., 2009). EXO genes are phosphate-induced-1-Like (PHI-1-Like) genes, because the 300 amino acid conserved region of the EXO protein constitutes a phi-1 domain (PFAM entry PF04674) (Quan et al., 2019). The PHI-1 genes were originally reported to be associated with tobacco cell cultures (Sano et al., 1999). Many plants contain PHI-1 gene family members; there are 19 PHI-1 genes in potato (Solanum tuberosum L.), 12 in rice (Oryza sativa L.), and 19 in maize (Zea mays L.) (Quan et al., 2019). erg1 is a potato PHI-1-Like gene involved in ethylene and salicylic acid pathways (Dellagi et al., 2000). Overexpression of EgPHI-1 significantly increased tolerance to NaCl, PEG, and mannitol treatments (Sousa et al., 2014). At present, there are very few papers reporting studies on EXO genes, and the functions of EXO proteins are unclear, particularly in cotton.
Cotton (Gossypium spp.) has gained immense attention for its natural fiber, and is the basis of a worldwide textile industry. In Arabidopsis, EXO family genes have been identified, but EXO genes have not been comprehensive and systematical analysis in cotton. With the advancement of genome sequencing technology, identified many different gene families in cotton become possible (Du et al., 2018; Ma et al., 2018; Yang et al., 2020).
In this study, the EXO gene family in four cotton species; Gossypium hirsutum, Gossypium arboreum, Gossypium raimondii, and Gossypium barbadense has been comprehensively and systematically analyzed. We then performed a phylogenetic analysis, and analyzed the sequences for conserved amino acids, gene structure, conserved protein motifs, chromosomal location, and genetic synteny. Furthermore, we preliminarily investigated tissue-specific expression profiles of GhEXO family members and examined their reacts to phytohormone stimuli. We then overexpressed GhEXO7_At in Arabidopsis, the subcellular localization of GhEXO7 has been determined in leaf epidermal cells of Nicotiana benthamiana, and used virus-induced gene silencing (VIGS) in cotton to explore the functions of the GhEXO7_At gene. The results of our study may help to elucidate the functions of the GhEXO genes in the growth and development of cotton.
Materials and Methods
EXO Gene Family Protein Sequence Identification
First, we downloaded the Arabidopsis EXO protein sequences from the TAIR database1 and used them as search queries to identify EXO protein-encoding genes in the genomes of different plant species including G. hirsutum (ZM24_ICR), G. arboreum (ICR, version 1.0), G. raimondii (JGI, version 2.0), G. barbadense (HAU, version 1.0), Sorghum bicolor (version 2.1), Selaginella moellendorffii (version 1.0), Physcomitrella patens (version 3.3), and O. sativa (version 7.0). The latest genome data for all species except Arabidopsis and cotton has been obtained from Phytozome (version 11)2. The G. arboreum, G. hirsutum, and G. barbadense genomic data were downloaded from the Gossypium Resource and Network Database3. We downloaded G. raimondii databases from the Cotton Functional Genomics Database4. The Arabidopsis database was downloaded from TAIR 105. Next, all presumptive EXO protein sequences were verified by Interproscan 63.06 (Jones et al., 2014) and SMART7 (Letunic et al., 2015). We then used ExPASy ProtParam8 to estimate the physicochemical properties of GhEXO family members.
Conserved Sequence Logos and Phylogenetic Tree Construction
The WEBLOG was used for conservative amino acid residue analysis (Crooks et al., 2004). We use the maximum likelihood (ML) method which configured with default parameters by poisson model with MEGA X to construct the evolutionary tree (Kumar et al., 2012). We then used Evolview9 to visualize the phylogenetic tree (Subramanian et al., 2019).
Gene Structure and Protein Conserved Motif Analysis
To determine the types of protein motifs, present and to perform gene structure analysis, the predicted GhEXO protein sequences were aligned with ClustalW, and a phylogenetic tree was produced with the NJ method as implemented in MEGA X. We analyzed GhEXO gene intron/exon structures using the Gene Structure Display Server 2.0 (Hu et al., 2015). In addition, using MEME online tool to forecast GhEXO protein domains (Bailey et al., 2006). TBtools10 software was used to visualize the distribution pattern of the conserved protein motifs (Chen et al., 2018).
Chromosomal Location, Synteny, and Ka/Ks Analysis
First, we obtained the chromosomal position information for the GhEXO genes from the gff3 file of the cotton genome annotation data, and the genes were located on the chromosomes by MapInspect (He et al., 2012). We then used the GhEXO protein sequences seek queries against the whole genomes. The results were analyzed by MCScanX to generate collinearity pairs, which were visualized using Circos (version 0.69) software with the default parameters (Krzywinski et al., 2009). Finally, we use the PAML package to calculate the replacement rates of synonymous (Ks) and non-synonymous (Ka) (Yang, 2007).
Cotton Tissue Expression Profile and Phytohormone Induction Treatment Assay
In our study, for the sake of confirm the expression pattern, we selected eight different tissues of G. hirsutum breed CRI24. Before phytohormone treatment, cotton seedlings at the cotyledon stage were transferred to a liquid medium and continued to grow the three-four leaf stage (Yang et al., 2014). Then we added the final concentration of 100 micromoles per liter of IAA, 100 micromoles per liter of GA, 10 micromoles per liter of BL to treat cotton seedlings for 1, 3, and 5 h. An equal volume of the corresponding solvent was added to the deionized water as a blank control.
Toteal RNA extraction used th TrelifefTM RNAprep Pure Plant Plus Kit (Tsingke, Beijing, China). Reverse transcription of 1000 ng RNA is performed with the EasyScript® All-in-One First-Strand cDNA Synthesis SuperMix (TransGen, Beijing, China) following the operating manuals. We used GhHistone3 (GenBank accession no. AF024716) and AtActin2 (AT3G18780.1) as internal controls for gene expression normalization. AceQ qPCR SYBR Green Master Mix (Low ROX Premixed) (Vazyme, Nanjing, China) was used for efficient amplification as a qRT-PCR on a Light-Cycler 480 (Roche Diagnostics, Germany). The data processing was used 2–ΔΔCt method (Livak and Schmittgen, 2001). Details regarding all of the primers used in this study are provided in Supporting Information (Supplementary Table 1).
Vector Construction, Subcellular Localization, and Creation of Transgenic Lines
To examine the function of GhEXO7_At, Arabidopsis (Col-0) plants were used to creation transgenic overexpressed GhEXO7_At lines. We amplified the full-length coding sequence (CDS) of GhEXO7_At gene from cDNA template. The full-length CDS was cloned into pCAMBIA-2301 driven by the 35S promoter. Next, we used the flower dip method to transform wild-type (Col-0) plants with Agrobacterium tumefaciens GV3101 (Clough and Bent, 1998). The transgenic lines were screened on 1/2 MS medium containing kanamycin (0.05 mg/mL) in a plant incubator at 22°C with a photoperiod set to 16 h/8 h. For VIGS, a 300 bp unique region of the GhEXO7_At CDS was cloned into the pTRV2 before transferred into A. tumefaciens (strain GV3101). Infiltration into upland cotton CRI24 was carried out as mentioned in previous study (Gao et al., 2011). The selected tissue was immersed in FAA fixation solution before dehydrate, transparency, tissues embedding in paraffin, and slices. Then, the sections were stained with toluidine blue, observed with Leica M165FC epifluorescence stereomicroscope (Leica Instruments GmbH).
To confirm the expression position of the GhEXO7_At-GFP fusion protein in cells, we cloned the full-length GhEXO7_At CDS into a vector to produce an in-frame GFP protein fusion. N. benthamiana leaves were co-infiltrated with the Agrobacterium strains together with the tombusvirus p19 suppressor of silencing (Witte et al., 2004). We left the plants in the dark for 24 h after co-infiltration. At 72 h after infiltration, stained with fluorescent membrane marker MM 4-64 [N-(3-Triethylammoniumpropyl)-4-(6-(4-(diethylamino) phenyl)hexatrienyl)pyridiniumdibromide] (AAT Bioquest, United States) for 8 min, GhEXO7_At was co-localized with MM 4-64 in N. benthamiana epidermal cells under fluorescence microscopy (Vida and Emr, 1995; Chen et al., 2015). Arabidopsis mesophyll protoplasts were isolated and transfected using the Arabidopsis Protoplast Preparation and Transformation Kit (Coolaber, Beijing, China) as described previously (Yoo et al., 2007; Ren et al., 2021). After 6–18 h of culture, Arabidopsis mesophyll protoplasts were observed with a fluorescence microscope.
Results
Identification of EXO Gene Family Members
We confirmed 21 nucleotide sequences in G. arboreum, 39 in G. hirsutum, 19 in G. raimondii, and 40 in G. barbadense as EXO genes. Additionally, we also identified the EXO genes in another dicotyledonous plant species (eight in Arabidopsis), two monocotyledonous species (12 in O. sativa and 12 in S. bicolor), moss (17 in P. patens), and seven in the lycophyte S. moellendorffii. We were surprised to discover that the number of EXO family members in diploid cotton (G. arboretum and G. raimondii) have almost half as tetraploid cotton (G. hirsutum and G. barbadense). In our study, we focused on the EXO genes in G. hirsutum. We first collected basic information on the GhEXO genes including start and end points, gene ID, chromosomal location of genes, the number of nucleotides in CDS, quantities of amino acid, and predicted molecular weight (MW) for the encoded proteins, isoelectric point (pI) is the pH of a protein solution when its net charge is zero, and Grand average of hydropathicity (GRVAY) values indicates the hydrophilicity and hydrophobicity of the protein (Table 1). The lengths of the predicted EXO proteins ranged from 246 (GhEXO17_At) to 384 (GhEXO5_At) amino acids, with MWs ranging from 27,297.26 Da (GhEXO17_At) to 41,826.73 Da (GhEXO5_At). The calculated pI values varied from 5.50 (GhEXO13_At) to 9.51 (GhEXO19_Dt). In addition, the minimum (−0.324) and maximum (0.091) GRAVY values were discovered for the GhEXO17_At protein and GhEXO15_At protein, respectively.
Phylogenetic Analysis of Proteins Encoded by the EXO Family Genes
In order to clarify the evolution of EXO genes among various species, an evolutionary tree containing nine representative species was constructed. The tree showed that all 175 predicted EXO proteins from the nine different plant species could be grouped into five major clades (Clades I-V) (Figure 1). The results of 175 EXO proteins analysis indicated that 8 of them were from Arabidopsis thaliana (At), 21 from G. arboretum (Ga), 39 from G. hirsutum (Gh), 19 from G. raimondii (Gr), 40 from G. barbadense (Gb), 12 from O. sativa (Os), 12 from S. bicolor (Sobic), 7 from S. moellendorffii (Sm), and 17 from P. patens (Pp).
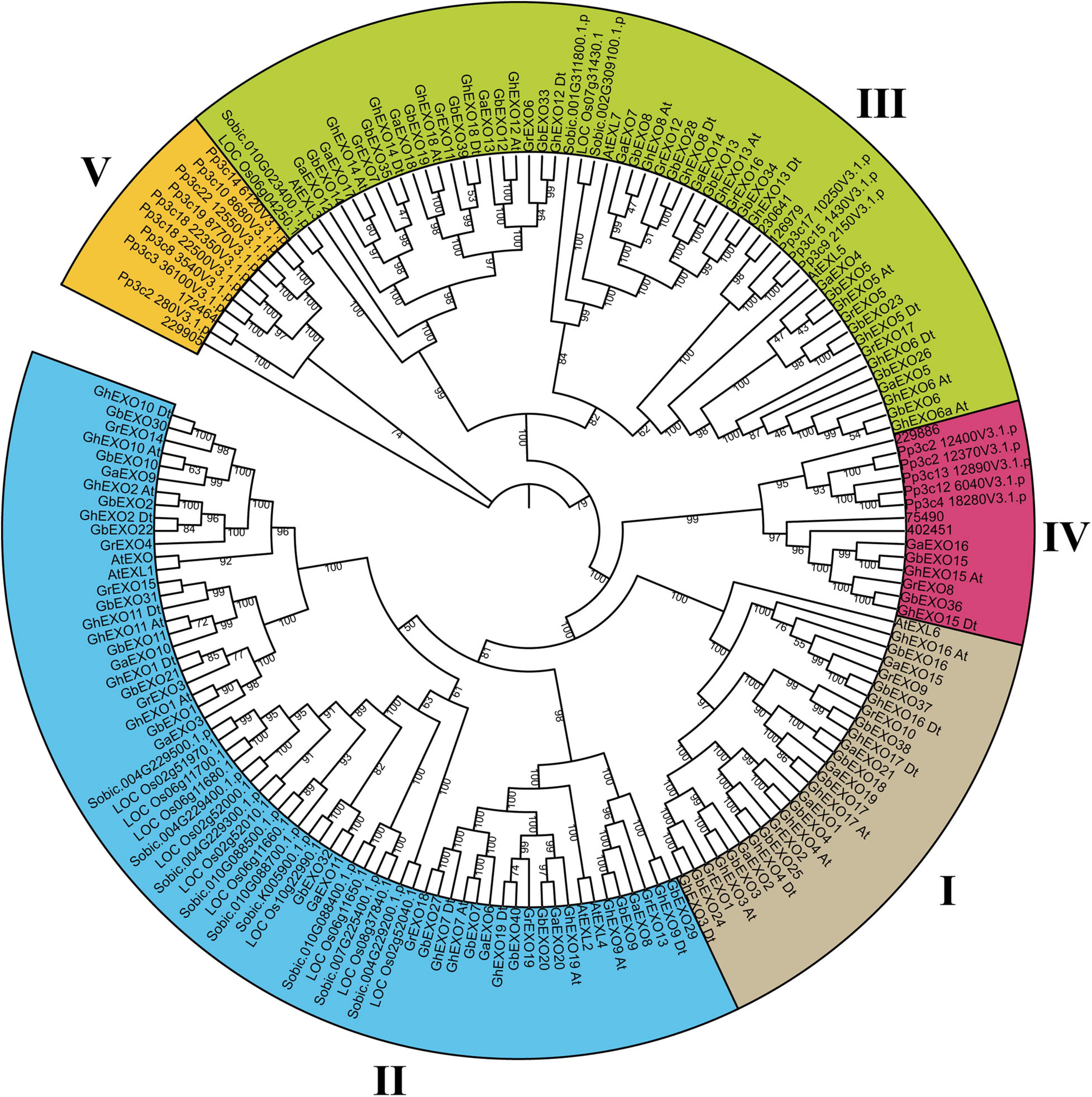
Figure 1. Phylogenetic analysis of predicted proteins encoded by the EXO genes from nine plant species. Phylogenetic analysis grouped all 175 EXO proteins into five clades in a tree calculated using the ML (maximum likelihood) method. Clades I–IV are shown in different colors. The prefixes At, Ga, Gh, Gr, Os, Zm, Sobic, Sm, and Pp are used to identify EXO family proteins from A. thaliana, G. arboreum, G. hirsutum, G. raimondii, O. sativa, Z. mays, S. bicolor, S. moellendorffii, and P. patens, respectively. In addition, At and Dt refer to the A and D sub-genomes of upland cotton (G. hirsutum), respectively.
In our analysis, Clade II included the most EXO proteins (66) followed by Clade III (57), Clade I (27), Clade IV (14), and Clade V (11). Although Clade II contained the most EXO proteins, with most of them coming from seven different species, the Clade III members come from all nine species included in the analysis. Most importantly, Clade I and Clade II contain only proteins from dicots and monocots, with no EXO proteins from either S. moellendorffii or P. patens, suggesting that these two groups may be specific to angiosperms. On the contrary, all 11 proteins in Clade V originate from the moss P. patens (9) and from S. moellendorffii (2). Interestingly, no algae homologs were identified, implying that the evolution of the EXO gene was accompanied by the emergence of bryophytes, and that the EXO genes present in higher plants may be derived from moss. Alternatively, the EXO genes could have been lost from the algal lineage. Clade IV contains proteins from the four cotton species, P. patens, and S. moellendorffii, while no monocot EXO proteins were present. For the purpose of further study in the evolution of EXO proteins among the cotton, phylogenetic analysis was performed in four cotton species (G. hirsutum, G. arboreum, G. raimondii, and G. barbadense) using the NJ method (Supplementary Figure 1). The whole EXO proteins in this tree were grouped into four clades (Clades I–IV).
Although the EXO proteins from dicots and monocots clustered close together, G. hirsutum and G. barbadense experienced a significant increase in EXO gene number, because the number of EXO proteins from these two cotton species was almost twice that from all other species in the phylogenetic analysis. Furthermore, a host of cheek by jowl clustering homologous genes were occurred in every species. This suggests that gene replication event is the dominant reason for the increase of EXO gene in plants.
Conserved Amino Acid Residue Analysis
In order to identify the homeodomain sequence and study the highly uniform amino acid sites in the GhEXO region, proteins from At, Os, and Gh were identified by multiple sequence alignments. The consequences showed that the distribution of amino acid residues in proteins from these three species was very similar at most positions. Specifically, it is highly conserved certain amino acid sites that covering E [2], C [7], G [9], G [12], G [14], G [22], D [27], G [31], Y [34], N [35], G [38], L [45], and C [57] were discovered. No specific conservative amino acid residue composition bias was observed in arbitrary position, indicating that in all observed species, no matter the N-terminal or C-terminal of the domain has an ultra-conservative distribution pattern (Figure 2).
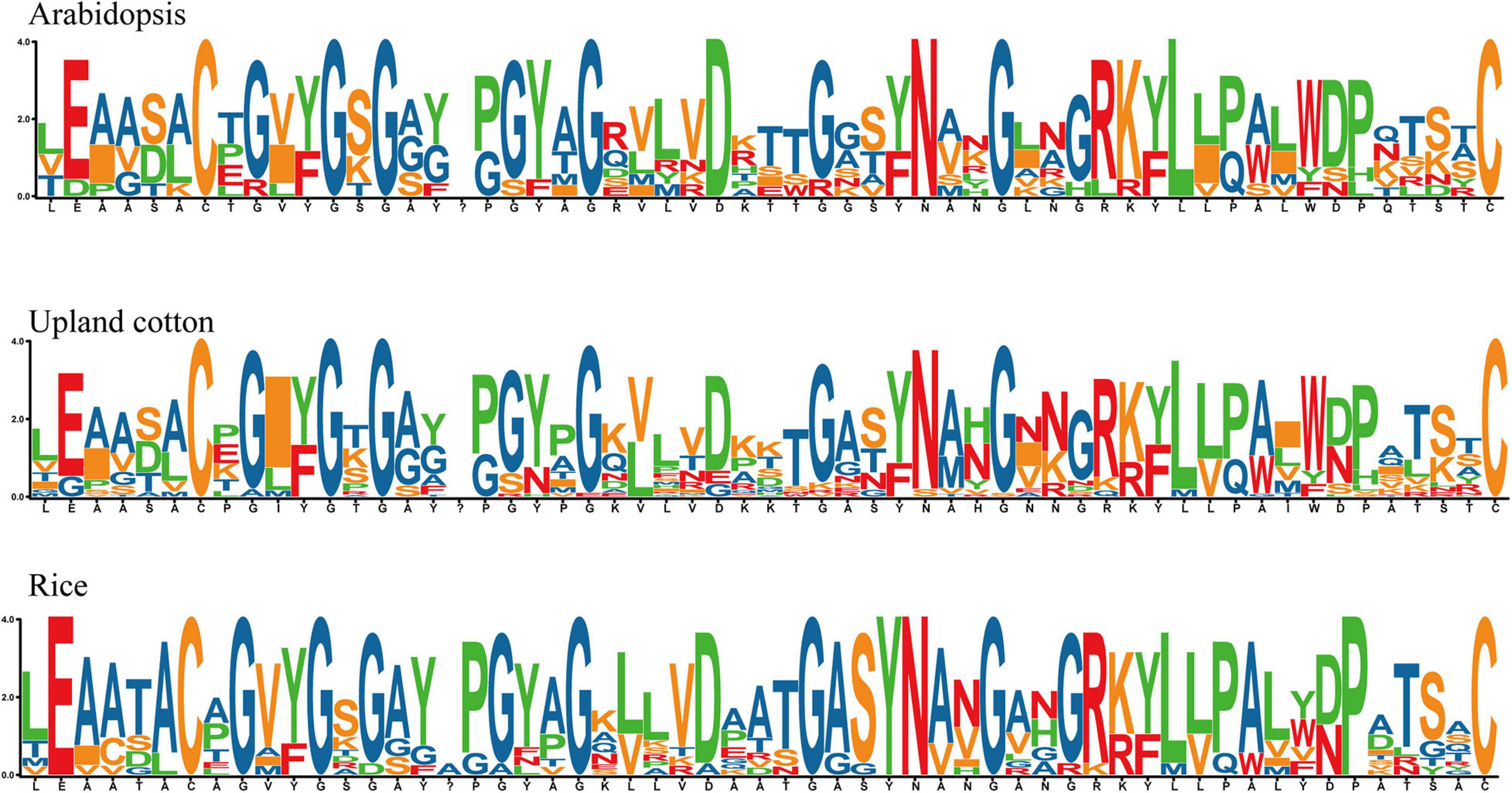
Figure 2. Sequence logo analysis of conserved amino acid residues in predicted EXO proteins. Sequence logos of conserved amino acid residues in the three plant species Arabidopsis thaliana, Upland cotton (G. hirsutum), and rice (O. sativa) showed highly conserved amino acids in EXO proteins during the evolution of dicot and monocot species.
Chromosomal Distribution, Gene Duplication, and Gene Synteny Analysis
The position of the GhEXO gene on the cotton chromosomes can be predicted, through the analysis of the GhEXO genome sequence (GFF3 profiles). A total of 39 GhEXO genes were inhomogeneous distribution on 18 chromosomes, 20 and 19 of them distributed on the At and Dt sub-genome chromosomes, severally (Supplementary Figure 2). The most genes (four genes each) were located on homologous chromosomes A05 and D05. Seven chromosomes out of 18 contained only one GhEXO gene. Among the 39 GhEXO genes, only the orthologs of GhEXO3, GhEXO4, and GhEXO5 are not located on the homologous sub-genome chromosomes of At and Dt. Previous study has shown that translocation events occur on chromosomes A02 and A03 explaining the fact of GhEXO3_At, GhEXO4_At, and GhEXO5_At, happen to be on the A02 and A03 chromosomes, are distributed on non-homologous chromosomes compared with its orthologs on the sub-genome chromosomes of Dt (Zhang et al., 2015).
An MCSCAN analysis can be used to detect various types of gene duplication (Yang et al., 2018). Tandem duplication, segmental duplication, and whole-genome duplication contribute to gene family expansion (Xu et al., 2012). A typical allotetraploid derived from two diploid species, G. hirsutum, is an indispensable material for analyzing polyploid effects (Paterson et al., 2012). Most GhEXO genes were identified as resulting from whole genome duplication (WGD) or segmental duplication (Supplementary Table 2). Three genes might have undergone tandem duplication (Supplementary Table 2). To identify the homologous locus relationships on different sub-genomes, we proceeded a collinearity analysis. Our results indicate that 15 pairs of genes are present in colinear blocks (Figure 3). Among the duplicated genes, GhEXO3_At formed eight pairs of genes with significant collinearity and GhEXO1_At formed seven pairs of genes with significant collinearity, indicating that they were active during evolution (Figure 3). There were no singleton and proximal duplications detected in the chromosomal regions containing the GhEXO genes (Supplementary Table 2).
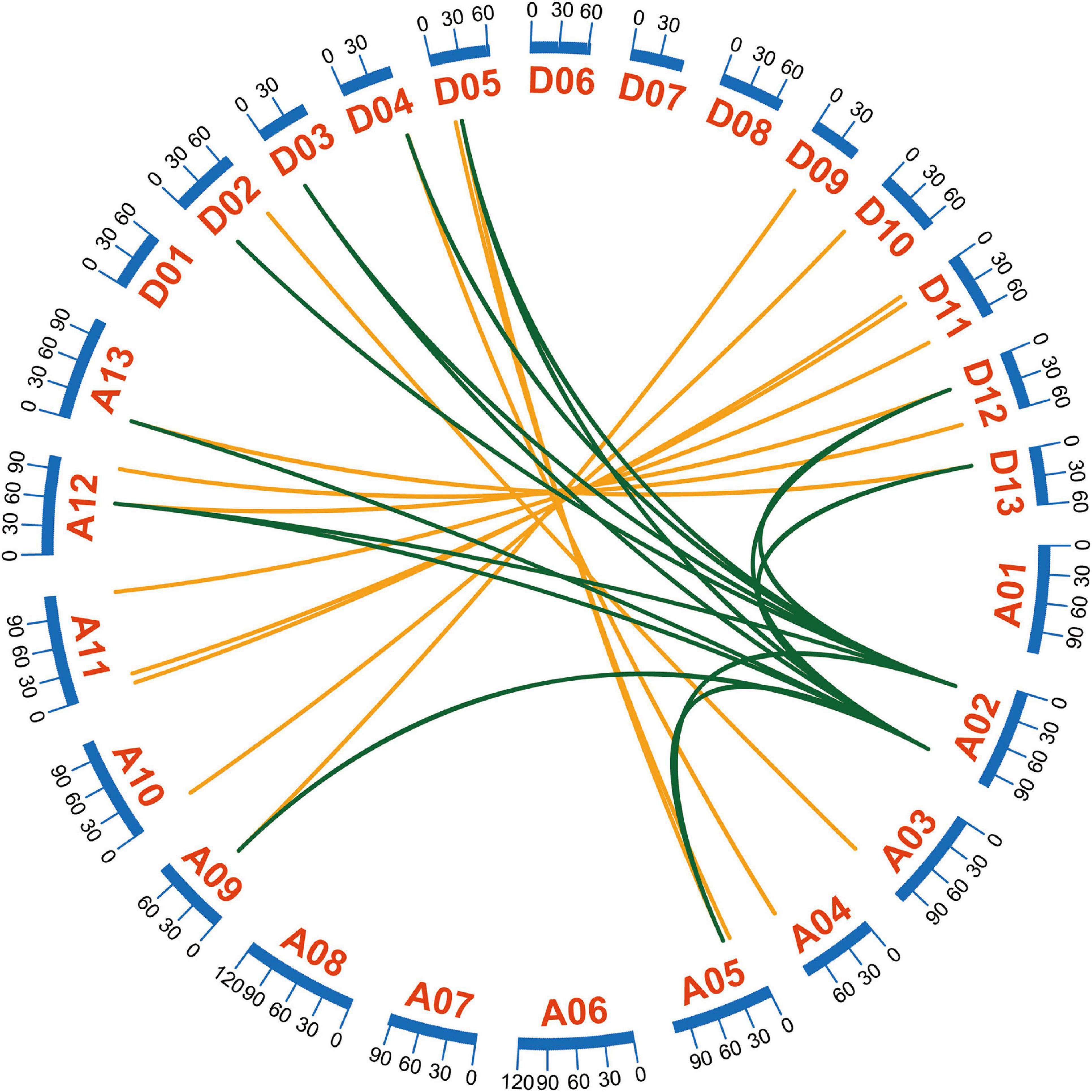
Figure 3. Collinearity analyses of G. hirsutum EXO genes. Collinearity analyses of the GhEXO genes on the 18 chromosomes of G. hirsutum. A01–A13 represent chromosomes of the At sub-genome and D01-D13 correspond to chromosomes of the Dt sub-genome. Gene pairs formed by segmental duplication within the At and Dt sub-genomes are linked by green lines. The orange lines connect gene pairs identified on homologous chromosome pairs from the At and Dt sub-genomes.
Cotton species have experienced genome expansion during evolution, and the number of genes continues to increase. Duplicated genes may have undergone functional differentiation, including partial or complete loss of the previous functions, gaining new functions or maintaining previous functions (Vandepoele et al., 2003). The non-synonymous (Ka) and synonymous substitution (Ks) ratios (Ka/Ks) of GhEXO genes allowed us to determine which kind of selection pressure (positive selection, negative selection, or purifying selection) acted on it. When Ka < Ks, the genes were selected for purification, indicating that there was no natural selection pressure; when Ka = Ks, the genes neutral evolution; and Ka > Ks, genes are strongly subject to positive selection show that rapid recent genetic evolution. The Ka/Ks ratios of 22 homologous pairs, including three paralogous pairs that arose from WGD or segmental duplication and 19 orthologous pairs were calculated. All the values were found to be <1 (Table 2), indicating that the GhEXO genes experienced purifying selection pressure, and that the protein functions may be conserved after gene family expansion.
Exon/Intron Organization and Conserved Motif Analysis
According to previous studies, gene structure can reflect evolutionary relationships between different plant species and the exon/intron distribution patterns of genes is associated with their biological functions (Roy and Gilbert, 2006). To further explore the phylogenetic relationships and potential protein functions amongst the GhEXO genes, we performed exon/intron structure and conserved motif analyses (Supplementary Figure 3). Our results indicate that most of the GhEXO genes with similar exon/intron numbers and distribution patterns belong to the same subfamilies. Most of the GhEXO genes have no introns. Ten of the 39 GhEXO genes analyzed in this study contain introns, accounting for 25% of the total GhEXO genes (Supplementary Figure 3B). To investigate the conserved motif distribution pattern in the GhEXO proteins, we constructed a phylogenetic tree with the neighbor joining method to couple with the domain positions (Supplementary Figure 3A). The results showed that most GhEXO proteins have similar motif distribution patterns; for example, motifs 3, 4, and 7 were present in all proteins (Supplementary Figure 3A). Motif 8 was only detected in the 15 proteins of Clade III, while motif 10 was absent. Furthermore, motif 10 was present in all GhEXO proteins of Clades I and II except in GhEXO2_At/Dt and GhEXO10_At/Dt. The two members of Clade IV lacked both motif 5 and motif 8. In general, the motif distribution patterns of the same sub-family GhEXO proteins in phylogenetic trees were similar.
Responses of GhEXO Members Under Phytohormonal Treatments and Its Expression Profile Analysis in Disparate Tissues
The expression level of functional genes in various tissues is closely related to plant phenotype. Therefore, we investigated the expression profiles of GhEXO genes in eight different cotton tissues (root, stem, leaf, flower, and 0, 5, 10, and 20 DPA fiber) using qRT-PCR (Figure 4). Based on the expression patterns, GhEXO12 (the gene on At and Dt sub-genomes) and GhEXO13 were found to be preferentially expressed in all tested fiber tissues. However, GhEXO10 was expressed at 5, 10, and 20 DPA (days post-anthesis), GhEXO5 was expressed at 10 and 20 DPA, while GhEXO1, GhEXO6, GhEXO9, and GhEXO15 were expressed only at 20 DPA. Similarly, GhEXO2, GhEXO4, GhEXO11, and GhEXO12 were expressed only at 10 DPA. GhEXO3 was mainly expressed at 5 DPA, while GhEXO7 was preferentially expressed in 0 DPA fiber tissue. GhEXO8, GhEXO14, GhEXO16, GhEXO17, GhEXO18, and GhEXO19 were advantageous expressed in the roots.
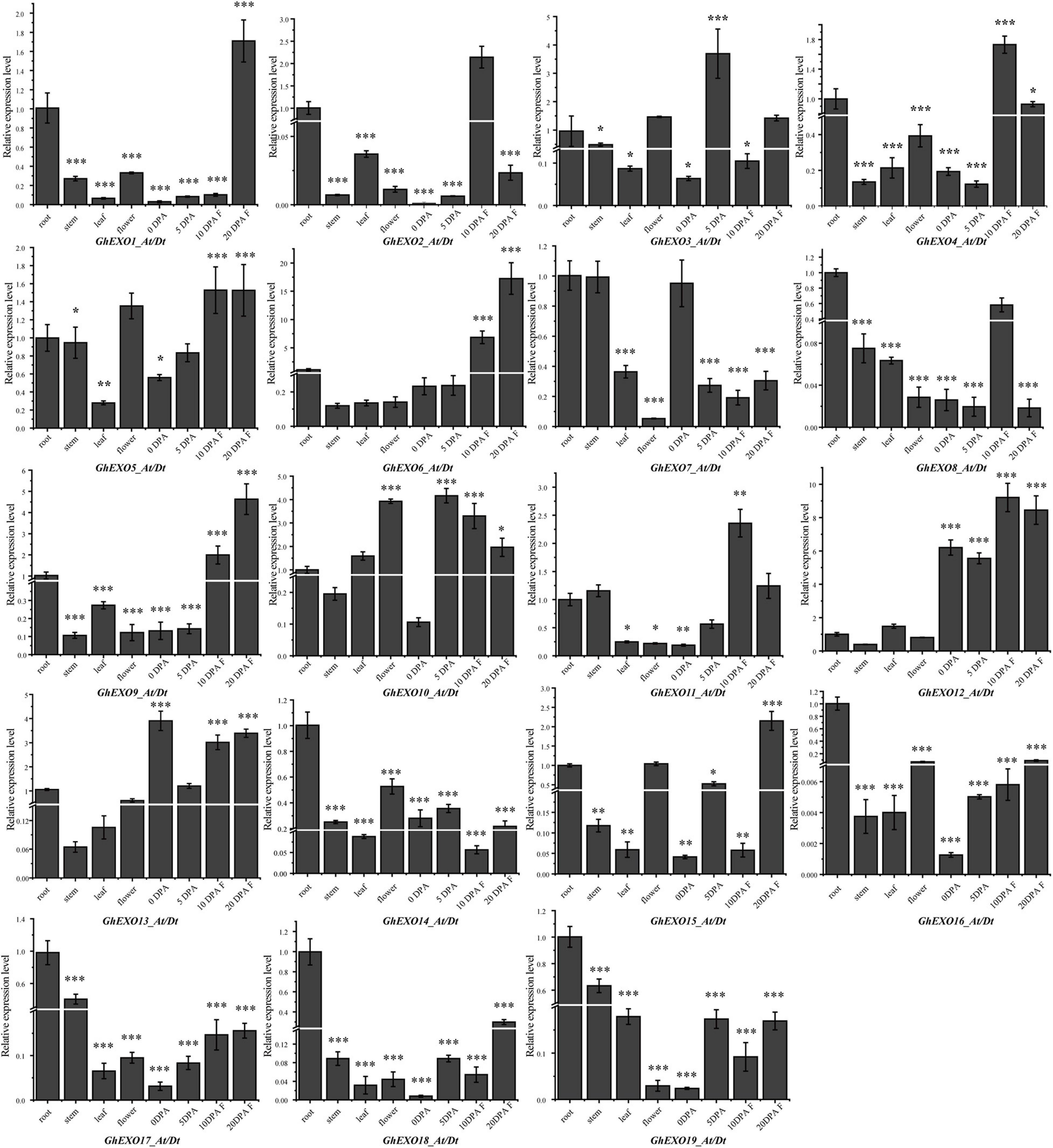
Figure 4. Tissue specific expression pattern analysis of GhEXO genes. Expression of 19 GhEXO genes in four different tissues and in fibers at 0, 5, 10, and 20 days after anthesis (DPA) as determined by qRT-PCR. Error bars represent the standard deviations of three independent experiments. Significant differences were determined by Student’s t-test: ∗p < 0.05, ∗∗p < 0.01, ∗∗∗p < 0.001.
Furthermore, exposure to different phytohormones (Auxin: IAA, Gibberellins: GA3, and Brassinosteroids: BL) revealed variable expression patterns in GhEXO family members. As shown in Figure 5, GhEXO3, GhEXO4, GhEXO7, and GhEXO17 were upregulated while GhEXO1, GhEXO9, GhEXO10, GhEXO11, GhEXO12, and GhEXO14 were completely downregulated in response to all tested hormones at all sampled time points. However, GhEXO5 and GhEXO18 showed up-regulation at all points after IAA treatment. GhEXO19 was significantly induced by GA, especially at the 3 h time point. Interestingly, GhEXO7 and GhEXO15 responded significantly to BL, especially at the 1 h time point. GhEXO7 was predominantly expressed at 0 DPA. Moreover, GhEXO7, gene on At and Dt sub-genomes, and AtEXO are extreme resemblance and may play similar functions in plants. Therefore, we selected the GhEXO7 gene for further functional characterization.
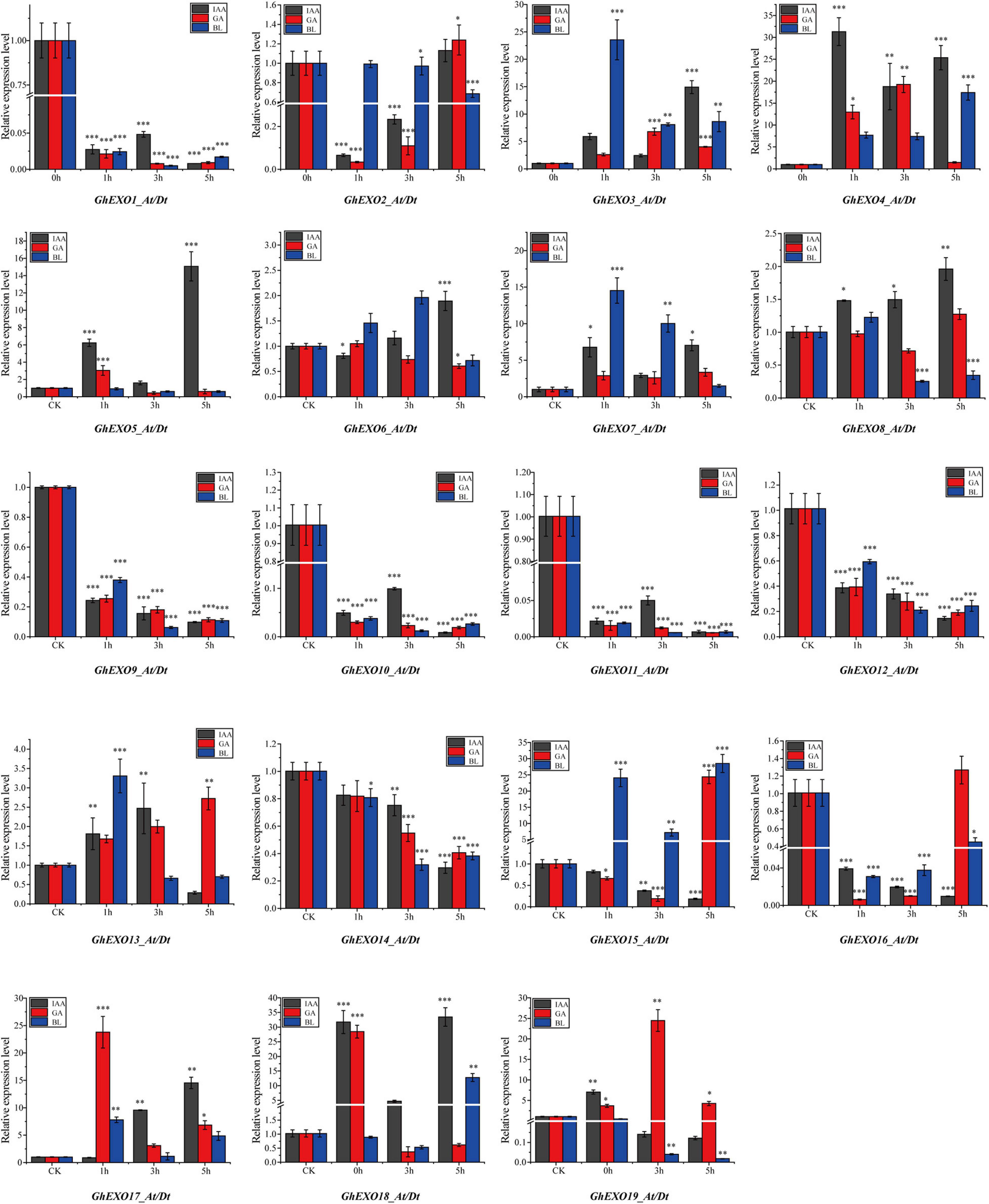
Figure 5. Expression pattern analysis of GhEXO genes under hormone treatment. Expression patterns of 19 GhEXO genes in response to three hormone treatments (IAA, GA, and BL) as determined by qRT-PCR analysis. The error bars show the standard deviations of three independent repeats. Student’s t-test: ∗p < 0.05, ∗∗p < 0.01, ∗∗∗p < 0.001.
Subcellular Localization of the GhEXO7_At, Ectopic Expression, and VIGS of GhEXOs
Above all, we determined the localization of the GhEXO7_At protein in N. benthamiana leaf epidermal cells and in Arabidopsis mesophyll protoplasts in order to further investigate the roles of GhEXO gene family members. The epidermal cells of N. benthamiana leaves were stained with an amphiphilic small molecule styrene dye MM 4-64 (Nguyen et al., 2018; Lichius and Zeilinger, 2019). The results of confocal laser scanning microscopy showed that the green fluorescence excited by EXO7_At-GFP protein was basically consistent with the red fluorescence of MM 4-64, membrane marker, indicated that GhEXO7_At is localized to the plasma membrane as compared to the MM 4-64 (Figure 6A). We also found that GhEXO7_At is localized to the cell membranes in Arabidopsis protoplasts (Figure 6B). From these results, we conclude that GhEXO7_At is a plasma membrane-localized protein.
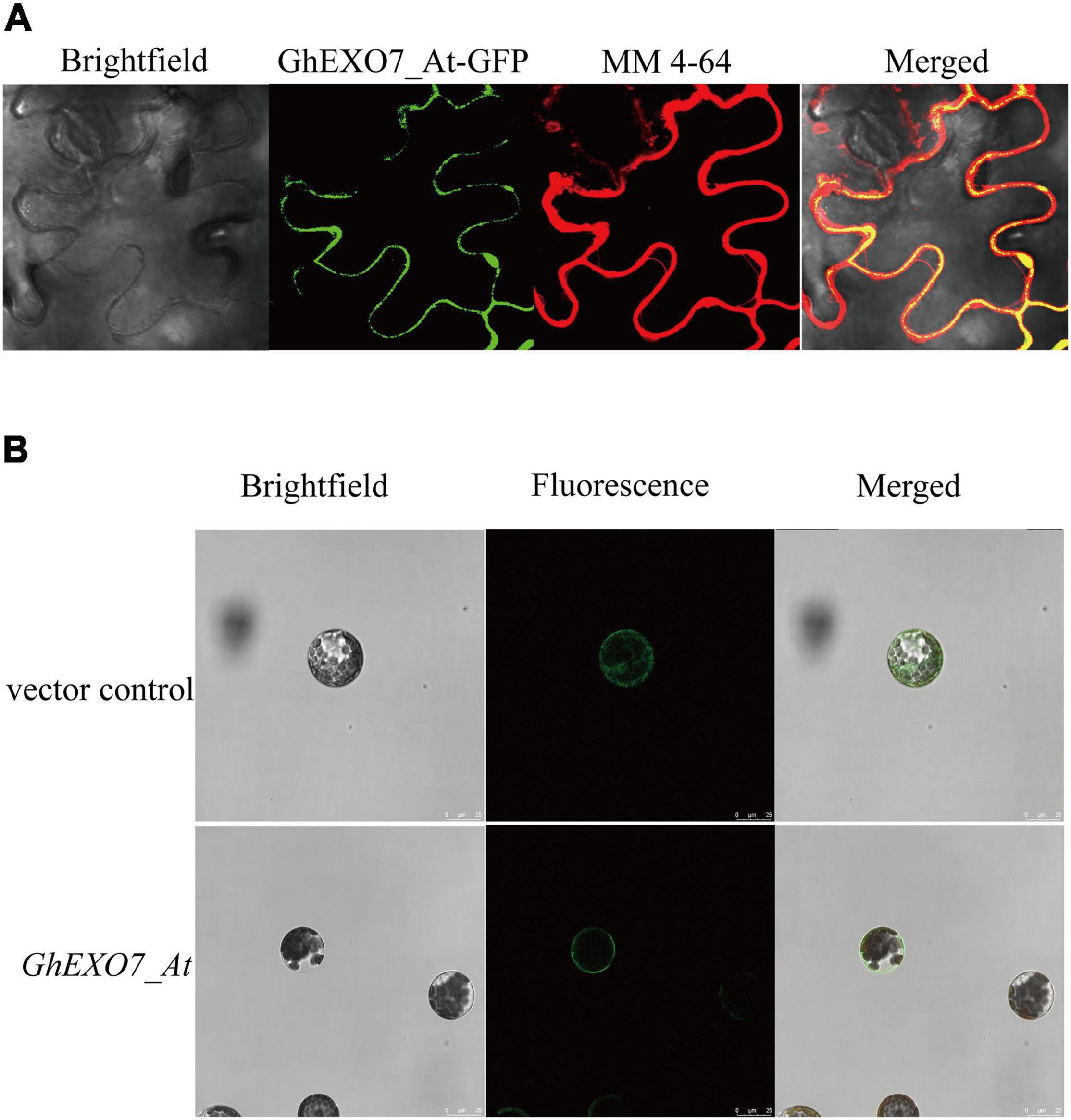
Figure 6. Subcellular localization of GhEXO7_At. (A) The Brightfield, GhEXO7_At-GFP Fluorescence, MM 4-64, and Merged images of infiltrated N. benthamiana leaf epidermal cells are shown. (B) The empty vector (control) and the GhEXO7_At-GFP vector were introduced into Arabidopsis mesophyll protoplasts. The Brightfield, Fluorescence (GFP), and Merged images are shown.
To confirm the role of the GhEXO7_At gene in plant growth and development, the GhEXO7_At CDS was transformed in Arabidopsis Col-0 plants under the control of the CaMV 35S promoter. The transgenic lines over expressing GhEXO7_At showed enhanced growth compared to wild-type (WT) plants (Figure 7A). We also determined the relative expression level of GhEXO7_At estimate the relative degree of overexpression. The qRT-PCR results confirmed that the GhEXO7_At gene expression level was many folds higher in the overexpression lines compared to the WT control (Figure 7D). The lengths and widths of the fifth rosette leaves were increased in the GhEXO7_At-overexpression lines compared to the control (Figures 7E,F). Leaf lengths in lines L1, L2, and L3 were 34, 25, and 23% longer and were 20, 16, and 17% wider compared to WT, respectively. In addition, according to the statistics, its roots length was 27% longer on average than WT, and the fresh weight was significantly increased by 52.2% when it was grown on MS medium for 14 days (Figures 7B,G,H). The transgenic lines showed enhanced growth was also verified in the leaf cells histological observations experiment (Figure 7C). According to report, BR promotes plant growth and cell elongation; therefore, we performed expression analyses of BR biosynthesis genes (DWF4 and CPD), the fatty acid elongase gene KCS1, and the cell elongation gene EXP5 in the transgenic Arabidopsis lines. The results showed that DWF4 and CPD expression was downregulated while the expression of KCS1 and EXP5 was upregulated in the transgenic GhEXO7_At-overexpressing Arabidopsis lines (Figure 7I).
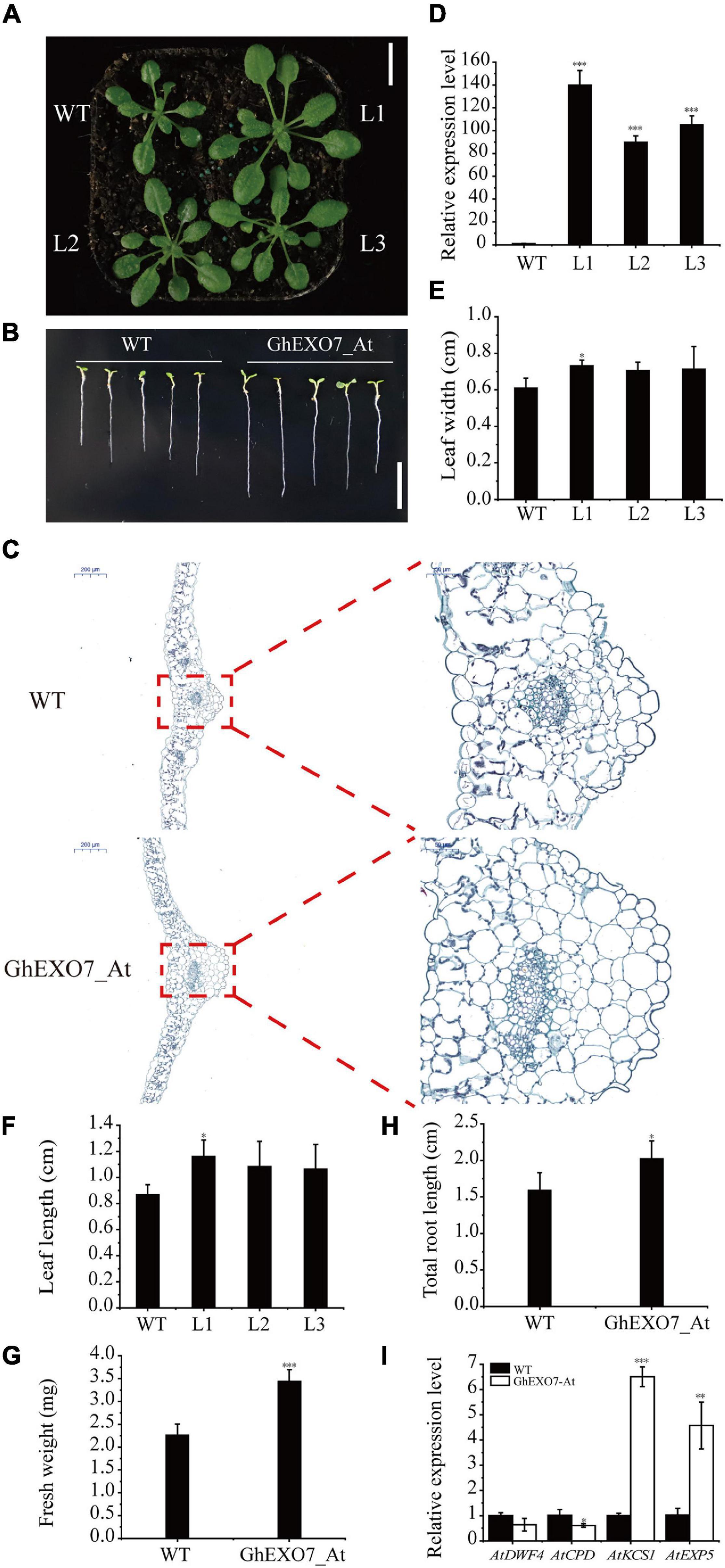
Figure 7. Phenotypes of plants from Arabidopsis lines overexpressing GhEXO7_At. (A) Phenotypic comparison of three GhEXO7_At lines and Col-0 (WT). Scale bars = 1 cm. (B) The root phenotypes of WT and GhEXO7_At-OE plants grown 14 days in the 1/2 MS. Scale bars = 1 cm. (C) Comparison of cells size from the fifth leaf of GhEXO7_At-OE and WT. (D) Relative GhEXO7_At expression levels in Col-0 and GhEXO7_At transgenic plants. Width (E) and length (F) of the fifth rosette leaf in WT and GhEXO7_At overexpression plants, Student’s t-test: ∗p < 0.05. Comparison of plant fresh weight (G) and total root length (H) of GhEXO7_At-OE and WT. (I) Relative expression levels of BR-repressed genes (CPD and DWF4) and BR-induced genes (EXP5 and KCS1) in Col-0 and the GhEXO7_At transgenic plants. Significant differences in expression were determined by Student’s t-test: ∗p < 0.05, ∗∗p < 0.01, ∗∗∗p < 0.001.
To further explore the function of GhEXO7_At, a VIGS vector carrying a region of the GhEXO7_At gene and the empty vector control were individually inoculated into cotton seedlings at the 2-leaf stage. The GhEXO7_At gene was silenced, which was verified by quantifying the relative expression levels in the infected cotton plants. We found that GhEXO7_At gene expression was decreased by 70–80% in the silenced plants (two randomly hosen lines VIGS-GhEXO7_At-1 and VIGS-GhEXO7_At-2) as compared to the control plants (Figure 8F). We also examined the expression of other GhEXO genes from the same phylogenetic clade as GhEXO7_At. The expression levels were reduced to varying degrees in the silenced plants (Supplementary Figure 4). The role of GhEXO7_At and its subfamily genes in plant growth and development was confirmed by results showing that leaf length, width (Figure 8A and Supplementary Figure 6A,B) decreased to varying degrees compared with vector control plants, leaf cells histological observations showed that the cells in the silenced plants were small and cell expansion was limited (Figure 8D). Furthermore, plant height (Figure 8B and Supplementary Figure 6C), fiber length (Figure 8C and Supplementary Figure 6D), root length, and root fresh weight (Figure 8E and Supplementary Figure 6E,F) were reduced compared to the VIGS vector control plants. The expression of GhEXO7_At/Dt was most significantly down-regulated, so GhEXO7 serves the main function.
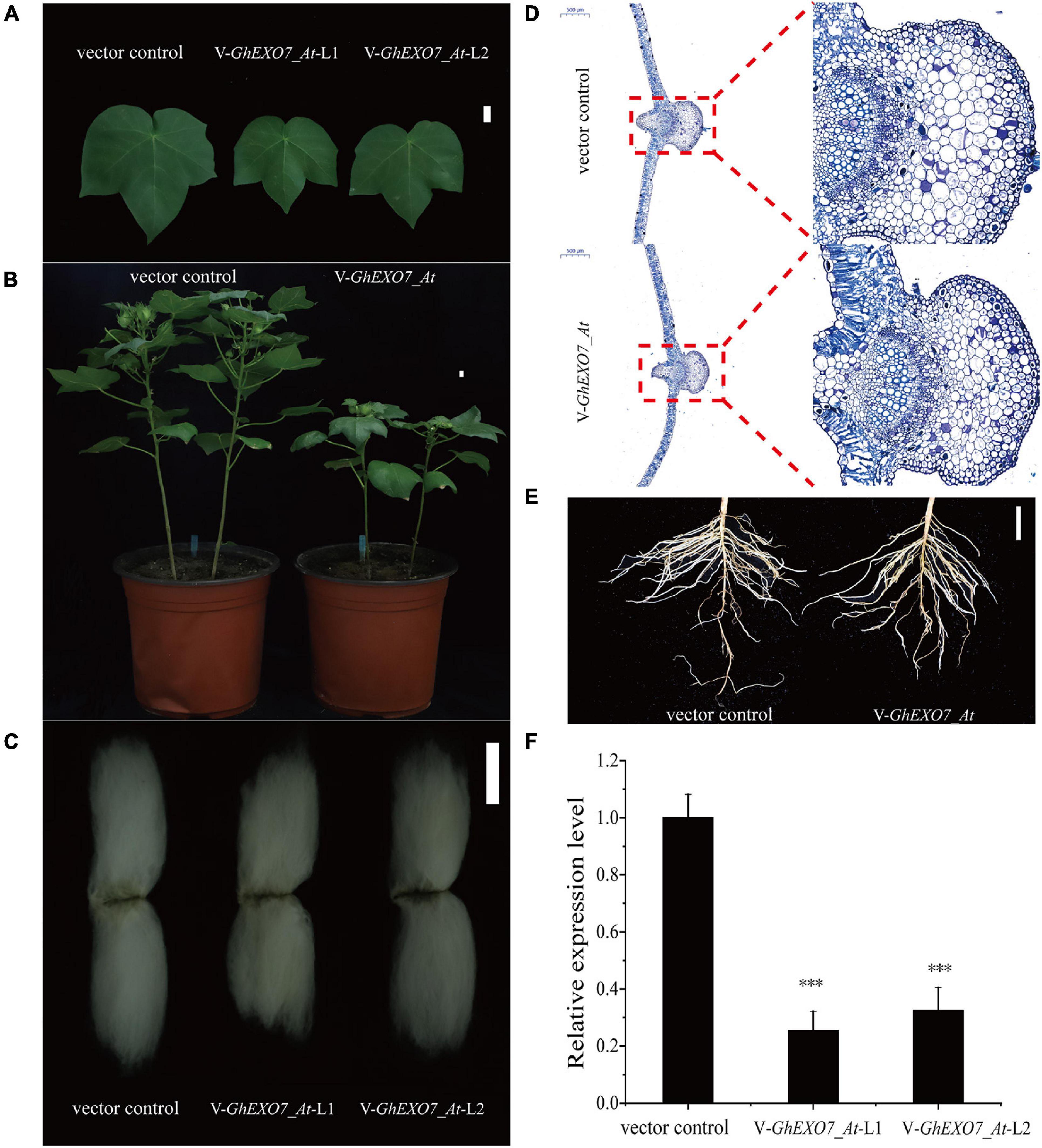
Figure 8. Virus-induced gene silencing (VIGS) of GhEXO7_At in cotton. (A) Phenotypes of leaves of the vector control and VIGS: GhEXO7_At plants. Scale bars = 1 cm. (B) Plant architecture of the vector control and VIGS: GhEXO7_At plants. Scale bars = 1 cm. (C) Fibers produced by the vector control and VIGS: GhEXO7_At plants. Scale bar = 1 cm. (D) Comparison of cells size from the fourth leaf of vector control and VIGS: GhEXO7_At plants. (E) The root phenotypes of vector control and VIGS: GhEXO7_At plants grown 30 days in the greenhouse. (F) Relative expression levels of the GhEXO7_At gene in the vector control and VIGS: GhEXO7_At plants. Student’s t-test: ∗∗∗p < 0.001.
Discussion
As an important economic crop, cotton (G. hirsutum) is cultivated around the globe, providing an important source of raw fiber for the textile industry. When grown in the field, cotton plants are exposed to a variety of biotic and abiotic stresses. In recent years, considerable progress has been made in cotton genomics research, which has resulted in reference genome sequences for many diploid (Wang et al., 2012; Li et al., 2014) and all allotetraploid cotton species (Paterson et al., 2012; Li et al., 2015; Zhang et al., 2015; Hu et al., 2019; Wang et al., 2019; Chen et al., 2020; Huang et al., 2020). In addition, re-sequencing studies have included the wide range of cotton verities, cultivars, and land races (Du et al., 2018; Ma et al., 2018). Many gene families in cotton, including GhPERK (Qanmber et al., 2019b), GhGGPPS (Ali et al., 2020), GhHH3 (Qanmber et al., 2019a), GhAAI (Qanmber et al., 2019c), GhBES1 (Liu et al., 2018), GhSnRK2 (Liu et al., 2017), GhRING-H2 (Qanmber et al., 2018), GhIDD (Ali et al., 2019), MIKC-type MADS-Box (Ren et al., 2017), GhKLCR (Li et al., 2019), GhGSK (Wang et al., 2018), and GhGH3 (Yu et al., 2018), among others, have been studied. Compared with other plant hormones, BR has high physiological activity and low content in plants, and it strongly induces nutritional and reproductive growth (Qanmber et al., 2019c). EXO gene family members have been shown to perform diverse functions in Arabidopsis in addition to the regulation of BR responsive genes. The results of our study identified the GhEXO family in the upland cotton to provide the foundation for deeper investigations into the functions of GhEXO genes in cotton.
GhEXO Gene Evolution
In the present study, for the first time, we identified the EXO gene family in cotton and analyzed its properties. It is believed that, around 5 to 10 Mya (million years ago), hybrids between diploid cotton of two different species gave rise to the heterotetraploid variety of cotton G. hirsutum and G. barbadense. G. arboretum evolved into the At subgenome and G. raimondii into the Dt gene subgroup (Li et al., 2015; Huang et al., 2020, 2021; Yang et al., 2020). In this study, total 175 EXO genes were identified in different varieties of cotton (Ga, Gh, Gr, and Gb), one other dicotyledonous species (Arabidopsis), two monocotyledonous species (O. sativa and S. bicolor), a moss (P. patens), and a lycophyte (S. moellendorffii). Interestingly, the number of EXO genes identified in the two allotetraploid cotton species G. hirsutum and G. barbadense was almost the sum of the number of EXO genes in diploid cotton varieties G. arboreum and G. raimondii, which strengthens the conclusion that the allotetraploid cotton species are derived from the hybridization and polyploidization of two diploid species (Yang et al., 2020; Huang et al., 2021). Next, the genome size of G. arboreum is larger than the genome of G. raimondii (Huang et al., 2021), and in this regard more EXO genes were identified in G. arboreum than in G. raimondii. Furthermore, the number of EXO gene family members were same in the two included monocot species (O. sativa and S. bicolor), and together with these findings we can speculate that the EXO gene family was conserved during evolution. Additionally, the presence of EXO genes in the two primitive plants P. patens and S. moellendorffii indicate that the EXO genes constitute an ancient gene family that has persisted during evolution in the plant kingdom. And with the substantial chromosome rearrangements in eukaryotes and cotton-specific whole-genome duplications, it has expanded and evolved in the cotton genome.
Our phylogenetic analysis of EXO protein sequences from the nine different plant species classified all EXO proteins into five major clades (I–V). Clade II contains the most EXO family members, while Clade V contains the least. More precisely, Clade III contains EXO proteins from all species included in the analysis (dicots, monocots, moss, and lycophyte), while Clades I and II contain only dicot and monocot EXO proteins, demonstrating that Clade III is the most primitive group, and that the proteins in Clades I and II may be specific to angiosperms. In contrast Clade V only contains EXO proteins from the primitive plants P. patens and S. moellendorffii. The absence of EXO genes in algae leads us to hypothesize that the EXO genes might have appeared in moss after the separation of moss and algae during evolution. Furthermore, the phylogenetic analysis of all the EXO proteins from the four cotton species divided them into four clades, corresponding to Clade I–IV except Clade V in the evolution analysis of different species. However, Clade III contains the most genes, although Clade II also contains numerous genes. These two clades contain almost 70% of the cotton EXO genes, which may be related to the fact that the EXO genes of each species are not evenly distributed in each clade. This phylogenetic analysis of cotton distinctly showing the clustering of orthologous/paralogous gene pairs and supporting the hybridization and polyploidization of cotton species (Li et al., 2015; Zhang et al., 2015; Huang et al., 2021).
Conserved amino acid residue analysis in the two dicot species Arabidopsis and G. hirsutum and the monocot species rice (O. sativa) revealed remarkably similar N- and C-terminal amino acid residues, demonstrating that the EXO proteins are highly conserved in dicots and monocots and the composition of each amino acid is not detected to be biased in any area. This corresponds to the last two motifs in the analysis of upland cotton conserved motifs, which are contained in all GhEXO protein sequences. Moreover, it has been found that the structure of a gene permits its evolutionary analysis, and the pattern of exon/intron distribution is useful for its function (Roy and Gilbert, 2006; Liu et al., 2021). In our study, exon/intron analysis indicated that most of the GhEXO genes have no introns, only 25% or fewer contain introns. Similarly, conserved protein motif distribution patterns were observed in almost all of the GhEXO proteins, and the proteins exhibiting similar gene structure and protein motif distribution pattern were generally in the same clade. Previous analyses of the cotton WOX (Yang et al., 2017) and YABBY gene families (Yang et al., 2018) showed similar results. Taken together, these findings strongly suggest that the EXO gene family has been conserved in plants during the course of evolution.
Expansion of the GhEXO Gene Family
During plant evolution, many gene families such as GRX (Malik et al., 2020) and AAI (Qanmber et al., 2019c) underwent expansion, and the number of family members increased compared to related species or, in the case of polyploids, their parental species. Here, from the phylogenetic analysis, we found that the orthologous/paralogous gene pairs in all nine plant species were closely clustered, illustrating evolutionary expanded model of the EXO gene family. Also, the two tetraploid cotton species G. hirsutum and G. barbadense showed a significant increase (almost 2-fold) (Yang et al., 2020) in the number of identified EXO genes compared to their diploid progenitors (G. arboreum and G. raimondii) as well as all of the other plants included in the analysis (dicots, monocots, moss, and lycophyte). Therefore, the quantities of GhEXO family genes and the polyploid effect are mutually sufficient and necessary conditions. Cotton provides a powerful model system in which to study cell elongation and the effects of polyploidy.
Here, the large number of intron-less GhEXO genes suggests that at the early expansion stage, genes containing more introns could be lost during the passage of time (Roy and Penny, 2007; Liu et al., 2021), and these genes then formed advanced families with reduced numbers of introns (Roy and Gilbert, 2005, 2006; Tan et al., 2018). Furthermore, insertion/deletion events result in structural differences that permit the estimation of evolutionary timing (Lecharny et al., 2003; Liu et al., 2021), and the genes with fewer or no introns evolve more rapidly because introns experience weak selection pressure even though exons/introns focus more on functional roles, whether the sequence is conserved (Frugoli et al., 1998; Cai et al., 2021). The exon/intron and motif distribution patterns in most gene families are conserved, and many gene families with fewer or no introns have been identified (Li et al., 2019; Qanmber et al., 2019b; Quan et al., 2019). This is also consistent with the fact that equilibrium intron numbers in species with large population sizes will be lower, due to a host of successful intron loss mutations and few successful intron mutations (Liu et al., 2021). Therefore, we deem that the GhEXO gene family is a high-quality gene family, with intron loss during evolution and differentiation during the early expansion phase of evolution.
It has been reported that the gene family expands with the duplication of genes (Xu et al., 2012; Zeng et al., 2021). Previous studies have shown that cotton experienced genome duplication events during evolution, and subsequently there were increases in the sizes of many gene families. Duplicated gene pairs may exhibit functional differentiation, such as partial or complete loss of function, the gain of a new function, or conservation of the original function (Vandepoele et al., 2003; Campbell et al., 2019; Huang et al., 2021). The 39 GhEXO genes showed uneven distribution on the 18 chromosomes of G. hirsutum. Except for GhEXO3, GhEXO4t, and GhEXO5, all other orthologo were located on homologous chromosomes. Previously, a translocation event was identified between the second and third chromosomes of the At subgenome (Zhang et al., 2015; Yang et al., 2020), which might be the explanation that GhEXO3 (the gene on At and Dt sub-genomes), GhEXO4, and GhEXO5 are not on homologous chromosomes compared with its orthologs.
In this study, most of the EXO genes were found to originate from WGD or segmental duplication events, while only three genes showed evidence of having been derived from tandem duplication. However, no singletons or proximal duplication was found investigated how WGD or segmental duplication expanded many gene families, while it reported that tandem duplication generated an increased number of introns along with new genes (Iwamoto et al., 1998; Hartmann et al., 2018). Furthermore, homologous locus relationship between the At and Dt sub-genomes showed that 15 GhEXO genes are present in colinear blocks, indicating that these were active during evolution. Additionally, analysis of the selection pressure (positive selection, negative selection, and purifying selection) by computing the non-synonymous (Ka) to synonymous substitution (Ks) ratios (Ka/Ks) suggests that all orthologous/paralogous gene pairs had Ka > Ks values which is evidence that purifying selection pressure acted on the GhEXO genes which in turn conserved their function despite the expansion of the gene family. Therefore, the main method of amplification of the GhEXO gene family with conserved gene function is gene duplication. In summary, GhEXO genes are an ancient gene family that existed in the early stages of evolution and under the pressure of purifying selection. Its expansion in the genome originates from genome-wide duplication and fragment duplication and conforms to the polyploid effect.
Diverse Expression Patterns of the GhEXO Genes
We investigated the expression patterns of the GhEXO genes in various tissues and fiber samples and found that the tested GhEXO genes were expressed in all tissues. However, GhEXO12_At/Dt and GhEXO13_At/Dt were advantageously expressed in 0, 5, 10, and 20 DPA fiber tissues. Similarly, GhEXO8_At/Dt, GhEXO14_At/Dt, GhEXO16_At/Dt, GhEXO17_At/Dt, GhEXO18_At/Dt, and GhEXO19_At/Dt were expressed in the roots, while all other genes had uneven expression patterns. There are almost no very similar expression patterns among family members, which means that GhEXO family members function in various organizations. These highly enriched fiber-related genes might be important genetic targets for fiber breeding in cotton.
It has been reported that EXO expression is relatively high in the tissue of high concentrations of auxin and auxin can induce the expression of EXO in AtEM201 (Farrar et al., 2003); EXO ectopic expression was observed in the hypocotyl of etiolated seedings and in root vascular tissue of GA treatment (Farrar, 2000); BR-deficient dwf1-6 and CPD antisense plants showed weaker EXO expression, and exogenous BL resulted in increased EXO transcript levels in both wild-type and dwf1-6 plants (Coll-Garcia et al., 2004). In addition, these three phytohormones can promote plant growth and development, corresponding to the function of EXO. Hence, we investigated the expression patterns of GhEXO genes in response to exposure to three plant hormones (IAA, GA, and BL) and observed variable response patterns. For example, GhEXO3_At/Dt, GhEXO4_At/Dt, GhEXO7_At/Dt, GhEXO8_At/Dt, and GhEXO17_At/Dt were upregulated while GhEXO1_At/Dt, GhEXO9_At/Dt, GhEXO10_At/Dt, GhEXO11_At/Dt, GhEXO12_At/Dt, and GhEXO14_At/Dt were completely downregulated by all hormone treatments. GhEXO3_At/Dt, GhEXO4_At/Dt, GhEXO7_At/Dt, GhEXO8_At/Dt, GhEXO15_At/Dt, and GhEXO17_At/Dt showed upregulation at all time points in response to BL. These genes might be the key genes involved in the responses to the relevant hormones, and they could prove to be important genetic targets for future cotton breeding programs.
GhEXO7_At Mediates BR-Regulated Plant Growth and Development
As reports, BRs was considered to promote growth primarily through cell expansion or elongation (Oh et al., 2020). BR-deficient mutants show plant dwarfing, hypocotyls and root cells are shortened to varying degrees, and the two-dimensional expansion of leaf cells is restricted (Yang et al., 2014; Oh et al., 2020). A limited number of studies have explored the function of EXO genes in different plant species; however, ours is the first to characterize the EXO gene family in cotton. A previous report indicated that inhibition of EXO gene expression does not cause abnormal phenotypes (Coll-Garcia et al., 2004), while exo knockout mutants exhibited dwarfing due to reduced cell expansion (Schroder et al., 2009). Similarly, overexpression of EXO genes gave plants with increased vegetative growth accompanied by increased expression of BR-up-regulated genes. Additionally, EXO genes show weak expression in BR-deficient mutants, while enhanced expression of EXO genes in BR-treated plants has been reported (Coll-Garcia et al., 2004).
In our study, MM 4-64 was used to co-locate with GhEXO7_At-GFP in N. benthamiana leaf cells confirmed that GhEXO7_At is a plasma membrane protein. MM 4-64 is a membrane dye that can specifically bind to the plasma membrane in a short time and emit high-intensity red fluorescence. In the early stage, it was used to study the endocytosis of yeast cells (Vida and Emr, 1995). Now, it is widely used in the medical field, such as the staining of cardiomyocytes (Chen et al., 2015). In addition, it has also been used to stain Arabidopsis root cells, Brassica napus L. seeds and tobacco cells in plant kingdom (Jelínková et al., 2010, 2015; Rigal et al., 2015; Lin et al., 2019). Ectopic expression of GhEXO7_At in Arabidopsis gave transgenic plants that exhibited increased vegetative growth and root growth as compared to the control Col-0 plants. Histological observations show that it has stronger cell expansion than WT and this is exactly the mechanism by which BR promotes growth. Deeper investigation revealed that BR biosynthesis genes (DWF4 and CPD) showed downregulated expression, while the fatty acid elongate gene KCS1 and the cell elongation gene EXP5 were upregulated in GhEXO7_At-overexpression lines, demonstrating BR-mediated growth and development by the GhEXO7_At gene in Arabidopsis. In addition to affecting cell expansion, BRs can also affect cell division (Oh et al., 2020), but we have not observed this aspect. These results were further verified in cotton plants in which GhEXO7_At gene expression was decreased 70–80% by VIGS. We observed reduced plant growth and development, including reduced leaf length, fiber length, and root length, in silenced plants as compared to the control plants. However, we found that not only the transcription level of GhEXO7_At was suppressed in silent plants, but also other GhEXO genes from the same phylogenetic clade as GhEXO7_At. We speculate that the phenotype of silent plants is caused by the specific expression of these genes in different tissues. GhEXO7_At was most significantly inhibited, so we think it is the main effector gene. Taken together, we conclude that GhEXO7_At gene mediates BR-regulated plant growth and development in cotton.
Conclusion
Although the function of certain EXO genes has previously been demonstrated in Arabidopsis, the function of the EXO gene family in cotton is unclear. In the present study, we identified 175 EXO genes in nine plant species, including 21 EXO genes in G. arboreum, 39 in G. hirsutum, 19 in G. raimondii, and 40 in G. barbadense. A phylogenetic analysis grouped the 175 EXO proteins into five major clades. Approximately 75% of the GhEXO genes have no introns. Conserved amino acid sequence signatures of EXO proteins from Arabidopsis, rice, and G. hirsutum showed that the EXO protein sequences are highly evolutionarily conserved. Collinearity analysis verified that segmental duplication and WGD events were the main drivers of the expansion of the cotton EXO gene family. Furthermore, gene expression analysis indicated that the GhEXO genes were without exception expressed in eight tested cotton tissues, and also evidenced that the expression of these genes can be regulated by hormone treatments, indicating that GhEXO genes mediate plant responses to hormonal stress. We found that GhEXO7_At is a plasma membrane-localized protein, and overexpression of GhEXO7_At enhanced growth in Arabidopsis, suggesting that it mediates the effect of BR on plant growth. Silencing GhEXO7_At in cotton caused a decrease in leaf length, leaf width, plant height, and fiber length. Our results will be not only helpful to understanding the evolution of cotton EXO genes, but will also provide important candidate genes for genetic engineering in cotton.
Data Availability Statement
The datasets presented in this study can be found in online repositories. The names of the repository/repositories and accession number(s) can be found in the article/Supplementary Material.
Author Contributions
SL: experimentation and writing. GC: methodology and formal analysis. ZL: writing and editing. GQ: conceptualization and writing — review, and editing. LL: software and data curation. JZ: formal analysis and review and editing. SM: methodology and data curation. ZY: supervision. FL: supervision. All authors contributed to the article and approved the submitted version.
Funding
This project was supported by the Funds for Creative Research Groups of China (Grant No. 31621005), State Key Laboratory of Cotton Biology Open Fund (No. CB2020A02), and National Natural Science Foundation of China (Project 32001597).
Conflict of Interest
The authors declare that the research was conducted in the absence of any commercial or financial relationships that could be construed as a potential conflict of interest.
Publisher’s Note
All claims expressed in this article are solely those of the authors and do not necessarily represent those of their affiliated organizations, or those of the publisher, the editors and the reviewers. Any product that may be evaluated in this article, or claim that may be made by its manufacturer, is not guaranteed or endorsed by the publisher.
Acknowledgments
We thank Xin Li and Peng Huo (Zhengzhou Research Center, Institute of Cotton Research of CAAS, Zhengzhou) for their technical assistance.
Supplementary Material
The Supplementary Material for this article can be found online at: https://www.frontiersin.org/articles/10.3389/fpls.2021.719889/full#supplementary-material
Supplementary Figure 1 | Phylogenetic analysis of EXO protein sequences from four cotton species.
Supplementary Figure 2 | Chromosomal locations of predicted GhEXO gene family members in the G. hirsutum genome.
Supplementary Figure 3 | Gene structure analysis of the GhEXO family in G. hirsutum. (A) Protein motif distribution patterns of GhEXO proteins. (B) Intro/exon structures of the GhEXO genes.
Supplementary Figure 4 | The expression levels of six other GhEXO genes in the GhEXO7_At silenced plants. Student’s t-test: ∗p < 0.05, ∗∗p < 0.01.
Supplementary Figure 5 | Multiple sequence alignments of conserved motif data from Arabidopsis, rice, and G. hirsutum.
Supplementary Figure 6 | Statistical analyses of leaf length (A), leaf width (B), and plant height (C) vector control and VIGS: GhEXO7_At plants. Student’s t-test: ∗p < 0.05. (D) Mature fiber length in the vector control and VIGS: GhEXO7_At plants. Comparison of total root length (E) and root fresh weight (F) of GhEXO7_At-OE and WT Student’s t-test: ∗p < 0.05, ∗∗p < 0.01, ∗∗∗p < 0.001.
Supplementary Table 1 | Oligonucleotide primers used in this study.
Supplementary Table 2 | Duplication type information for the 39 GhEXO genes.
Supplementary Table 3 | Calculated expression values and primary data from qRT-PCR assays in this study.
Footnotes
- ^ https://www.arabidopsis.org/
- ^ https://phytozome-next.jgi.doe.gov/
- ^ https://grand.biocloud.net/
- ^ https://cottonfgd.org/
- ^ http://www.arabidopsis.org
- ^ http://www.ebi.ac.uk/InterProScan/
- ^ http://smart.emblheidelberg.de/
- ^ https://www.expasy.org/resources/protparam
- ^ https://www.evolgenius.info/evolview/
- ^ https://github.com/CJ-chen/TBtools
References
Ali, F., Qanmber, G., Li, Y., Ma, S., Lu, L., Yang, Z., et al. (2019). Genome-wide identification of Gossypium INDETERMINATE DOMAIN genes and their expression profiles in ovule development and abiotic stress responses. J. Cotton Res. 2:3. doi: 10.1186/s42397-019-0021-6
Ali, F., Qanmber, G., Wei, Z., Yu, D., Li, Y., Gan, L., et al. (2020). Genome-wide characterization and expression analysis of geranyl geranyl diphosphate synthase genes of cotton (Gossypium spp.) in plant development and abiotic stresses. BMC Genomics 21:561. doi: 10.1186/s12864-020-06970-8
Bailey, T. L., Williams, N., Misleh, C., and Li, W. W. (2006). MEME: discovering and analyzing DNA and protein sequence motifs. Nucleic Acids Res. 34(Suppl._2), W369–W373.
Cai, L., Arnold, B. J., Xi, Z., Khost, D. E., Patel, N., Hartmann, C. B., et al. (2021). Deeply altered genome architecture in the endoparasitic flowering plant Sapria himalayana Griff.(Rafflesiaceae). Curr. Biol. 31, 1002.e9–1011.e9.
Campbell, M. A., Hale, M. C., McKinney, G. J., Nichols, K. M., and Pearse, D. E. (2019). Long-term conservation of ohnologs through partial tetrasomy following whole-genome duplication in Salmonidae. G3 Genes Genomes Genet. 9, 2017–2028. doi: 10.1534/g3.119.400070
Chen, B., Zhang, C., Guo, A., and Song, L.-S. (2015). In situ single photon confocal imaging of cardiomyocyte T-tubule system from Langendorff-perfused hearts. Front. Physiol. 6:134. doi: 10.3389/fphys.2015.00134
Chen, C., Chen, H., He, Y., and Xia, R. (2018). TBtools, a toolkit for biologists integrating various biological data handling tools with a user-friendly interface. BioRxiv [Preprint]. doi: 10.1101/289660v2
Chen, Z. J., Sreedasyam, A., Ando, A., Song, Q., De Santiago, L. M., Hulse-Kemp, A. M., et al. (2020). Genomic diversifications of five Gossypium allopolyploid species and their impact on cotton improvement. Nat. Genet. 52, ages525–ages533.
Choe, S. (2006). Brassinosteroid biosynthesis and inactivation. Physiol. Plant. 126, 539–548. doi: 10.1111/j.1399-3054.2006.00681.x
Clough, S. J., and Bent, A. F. (1998). Floral dip: a simplified method for Agrobacterium-mediated transformation of Arabidopsis thaliana. Plant J. 16, 735–743. doi: 10.1046/j.1365-313x.1998.00343.x
Coll-Garcia, D., Mazuch, J., Altmann, T., and Müssig, C. (2004). EXORDIUM regulates brassinosteroid-responsive genes. FEBS Lett. 563, 82–86. doi: 10.1016/s0014-5793(04)00255-8
Crooks, G. E., Hon, G., Chandonia, J.-M., and Brenner, S. E. (2004). WebLogo: a sequence logo generator. Genome Res. 14, 1188–1190. doi: 10.1101/gr.849004
Dellagi, A., Birch, P. R., Heilbronn, J., Avrova, A. O., Montesano, M., Palva, E. T., et al. (2000). A potato gene, erg-1, is rapidly induced by Erwinia carotovora ssp. atroseptica, Phytophthora infestans, ethylene and salicylic acid. J. Plant Physiol. 157, 201–205. doi: 10.1016/s0176-1617(00)80191-1
Du, X., Huang, G., He, S., Yang, Z., Sun, G., Ma, X., et al. (2018). Resequencing of 243 diploid cotton accessions based on an updated A genome identifies the genetic basis of key agronomic traits. Nat. Genet. 50, 796–802. doi: 10.1038/s41588-018-0116-x
Farrar, K. (2000). Exordium a Novel Gene in Arabidopsis Identified by Promoter Trapping. Durham: Durham University.
Farrar, K., Evans, I. M., Topping, J. F., Souter, M. A., Nielsen, J. E., and Lindsey, K. (2003). EXORDIUM–a gene expressed in proliferating cells and with a role in meristem function, identified by promoter trapping in Arabidopsis. Plant J. 33, 61–73. doi: 10.1046/j.1365-313x.2003.01608.x
Friedrichsen, D. M., Nemhauser, J., Muramitsu, T., Maloof, J. N., Alonso, J., Ecker, J. R., et al. (2002). Three redundant brassinosteroid early response genes encode putative bHLH transcription factors required for normal growth. Genetics 162, 1445–1456. doi: 10.1093/genetics/162.3.1445
Frugoli, J. A., McPeek, M. A., Thomas, T. L., and McClung, C. R. (1998). Intron loss and gain during evolution of the catalase gene family in angiosperms. Genetics 149, 355–365. doi: 10.1093/genetics/149.1.355
Gao, X., Britt, R. C. Jr., Shan, L., and He, P. (2011). Agrobacterium-mediated virus-induced gene silencing assay in cotton. J. Vis. Exp. 54:e2938.
Hartmann, T., Bernt, M., and Middendorf, M. (2018). EqualTDRL: illustrating equivalent tandem duplication random loss rearrangements. BMC Bioinformatics 19:192. doi: 10.1186/s12859-018-2170-x
He, H., Dong, Q., Shao, Y., Jiang, H., Zhu, S., Cheng, B., et al. (2012). Genome-wide survey and characterization of the WRKY gene family in Populus trichocarpa. Plant Cell Rep. 31, 1199–1217. doi: 10.1007/s00299-012-1241-0
Hu, B., Jin, J., Guo, A.-Y., Zhang, H., Luo, J., and Gao, G. (2015). GSDS 2.0: an upgraded gene feature visualization server. Bioinformatics 31, 1296–1297. doi: 10.1093/bioinformatics/btu817
Hu, Y., Chen, J., Fang, L., Zhang, Z., Ma, W., Niu, Y., et al. (2019). Gossypium barbadense and Gossypium hirsutum genomes provide insights into the origin and evolution of allotetraploid cotton. Nat. Genet. 51, 739–748. doi: 10.1038/s41588-019-0371-5
Huang, G., Huang, J.-Q., Chen, X.-Y., and Zhu, Y.-X. (2021). Recent advances and future perspectives in cotton research. Annu. Rev. Plant Biol. 72, 437–462. doi: 10.1146/annurev-arplant-080720-113241
Huang, G., Wu, Z., Percy, R. G., Bai, M., and Zhu, Y. (2020). Genome sequence of Gossypium herbaceum and genome updates of Gossypium arboreum and Gossypium hirsutum provide insights into cotton A-genome evolution. Nat. Genet. 52, 516–524. doi: 10.1038/s41588-020-0607-4
Iwamoto, M., Maekawa, M., Saito, A., Higo, H., and Higo, K. (1998). Evolutionary relationship of plant catalase genes inferred from exon-intron structures: isozyme divergence after the separation of monocots and dicots. Theor. Appl. Genet. 97, 9–19. doi: 10.1007/s001220050861
Jelínková, A., Malínská, K., Simon, S., Kleine-Vehn, J., Paøezová, M., Pejchar, P., et al. (2010). Probing plant membranes with FM dyes: tracking, dragging or blocking? Plant J. 61, 883–892. doi: 10.1111/j.1365-313x.2009.04102.x
Jelínková, A., Müller, K., Fílová-Paøezová, M., and Petrášek, J. (2015). NtGNL1a ARF-GEF acts in endocytosis in tobacco cells. BMC Plant Biol. 15:272. doi: 10.1186/s12870-015-0621-3
Jones, P., Binns, D., Chang, H.-Y., Fraser, M., Li, W., McAnulla, C., et al. (2014). InterProScan 5: genome-scale protein function classification. Bioinformatics 30, 1236–1240. doi: 10.1093/bioinformatics/btu031
Krzywinski, M., Schein, J., Birol, I., Connors, J., Gascoyne, R., Horsman, D., et al. (2009). Circos: an information aesthetic for comparative genomics. Genome Res. 19, 1639–1645. doi: 10.1101/gr.092759.109
Kumar, S., Stecher, G., Peterson, D., and Tamura, K. (2012). MEGA-CC: computing core of molecular evolutionary genetics analysis program for automated and iterative data analysis. Bioinformatics 28, 2685–2686. doi: 10.1093/bioinformatics/bts507
Lecharny, A., Boudet, N., Gy, I., Aubourg, S., and Kreis, M. (2003). Introns in, introns out in plant gene families: a genomic approach of the dynamics of gene structure. J. Struct. Funct. Genomics 3, 111–116. doi: 10.1007/978-94-010-0263-9_11
Letunic, I., Doerks, T., and Bork, P. (2015). SMART: recent updates, new developments and status in 2015. Nucleic Acids Res. 43, D257–D260.
Li, F., Fan, G., Lu, C., Xiao, G., Zou, C., Kohel, R. J., et al. (2015). Genome sequence of cultivated Upland cotton (Gossypium hirsutum TM-1) provides insights into genome evolution. Nat. Biotechnol. 33, 524–530.
Li, F., Fan, G., Wang, K., Sun, F., Yuan, Y., Song, G., et al. (2014). Genome sequence of the cultivated cotton Gossypium arboreum. Nat. Genet. 46, 567–572.
Li, J., Yu, D., Qanmber, G., Lu, L., Wang, L., Zheng, L., et al. (2019). GhKLCR1, a kinesin light chain-related gene, induces drought-stress sensitivity in Arabidopsis. Sci. China Life Sci. 62, 63–75. doi: 10.1007/s11427-018-9307-y
Lichius, A., and Zeilinger, S. J. (2019). Application of membrane and cell wall selective fluorescent dyes for live-cell imaging of filamentous fungi. J. Vis. Exp. 153:e60613.
Lin, Y.-X., Xin, X., Yin, G.-K., He, J.-J., Zhou, Y.-C., Chen, J.-Y., et al. (2019). Membrane phospholipids remodeling upon imbibition in Brassica napus L. seeds. Biochem. Biophys. Res. Commun. 515, 289–295. doi: 10.1016/j.bbrc.2019.05.100
Liu, H., Lyu, H. M., Zhu, K., Van de Peer, Y., and Cheng, Z. M. (2021). The emergence and evolution of intron-poor and intronless genes in intron-rich plant gene families. Plant J. 105, 1072–1082. doi: 10.1111/tpj.15088
Liu, Z., Ge, X., Yang, Z., Zhang, C., Zhao, G., Chen, E., et al. (2017). Genome-wide identification and characterization of SnRK2 gene family in cotton (Gossypium hirsutum L.). BMC Genet. 18:54. doi: 10.1186/s12863-017-0517-3
Liu, Z., Qanmber, G., Lu, L., Qin, W., Liu, J., Li, J., et al. (2018). Genome-wide analysis of BES1 genes in Gossypium revealed their evolutionary conserved roles in brassinosteroid signaling. Sci. China Life Sci. 61, 1566–1582. doi: 10.1007/s11427-018-9412-x
Livak, K. J., and Schmittgen, T. D. (2001). Analysis of relative gene expression data using real-time quantitative PCR and the 2- ΔΔCT method. Methods 25, 402–408. doi: 10.1006/meth.2001.1262
Ma, Z., He, S., Wang, X., Sun, J., Zhang, Y., Zhang, G., et al. (2018). Resequencing a core collection of upland cotton identifies genomic variation and loci influencing fiber quality and yield. Nat. Genet. 50, 803–813. doi: 10.1038/s41588-018-0119-7
Malik, W. A., Wang, X., Wang, X., Shu, N., Cui, R., Chen, X., et al. (2020). Genome-wide expression analysis suggests glutaredoxin genes response to various stresses in cotton. Int. J. Biol. Macromol. 153, 470–491. doi: 10.1016/j.ijbiomac.2020.03.021
Molnar, G., Bancoş, S., Nagy, F., and Szekeres, M. (2002). Characterisation of BRH1, a brassinosteroid-responsive RING-H2 gene from Arabidopsis thaliana. Planta 215, 127–133. doi: 10.1007/s00425-001-0723-z
Müssig, C., and Altmann, T. (2003). Genomic brassinosteroid effects. J. Plant Growth Regul. 22, 313–324. doi: 10.1007/s00344-003-0061-4
Nguyen, H. H., Lee, M. H., Song, K., Ahn, G., Lee, J., and Hwang, I. (2018). The A/ENTH domain-containing protein AtECA4 is an adaptor protein involved in cargo recycling from the trans-Golgi network/early endosome to the plasma membrane. Mol. Plant. 11, 568–583. doi: 10.1016/j.molp.2018.01.001
Oh, M.-H., Honey, S. H., and Tax, F. E. (2020). The control of cell expansion, cell division, and vascular development by brassinosteroids: a historical perspective. Int. J. Mol. Sci. 21:1743. doi: 10.3390/ijms21051743
Paterson, A. H., Wendel, J. F., Gundlach, H., Guo, H., Jenkins, J., Jin, D., et al. (2012). Repeated polyploidization of Gossypium genomes and the evolution of spinnable cotton fibres. Nature 492, 423–427.
Qanmber, G., Ali, F., Lu, L., Mo, H., Ma, S., Wang, Z., et al. (2019a). Identification of Histone H3 (HH3) genes in Gossypium hirsutum revealed diverse expression during ovule development and stress responses. Genes 10:355. doi: 10.3390/genes10050355
Qanmber, G., Liu, J., Yu, D., Liu, Z., Lu, L., Mo, H., et al. (2019b). Genome-wide identification and characterization of the PERK gene family in Gossypium hirsutum reveals gene duplication and functional divergence. Int. J. Mol. Sci. 20:1750. doi: 10.3390/ijms20071750
Qanmber, G., Lu, L., Liu, Z., Yu, D., Zhou, K., Huo, P., et al. (2019c). Genome-wide identification of GhAAI genes reveals that GhAAI66 triggers a phase transition to induce early flowering. J. Exp. Bot. 70, 4721–4736. doi: 10.1093/jxb/erz239
Qanmber, G., Yu, D., Li, J., Wang, L., Ma, S., Lu, L., et al. (2018). Genome-wide identification and expression analysis of Gossypium RING-H2 finger E3 ligase genes revealed their roles in fiber development, and phytohormone and abiotic stress responses. J. Cott. Res. 1:4. doi: 10.1186/s42397-018-0004-z
Quan, C., Bai, Z., Zheng, S., Zhou, J., Yu, Q., Xu, Z., et al. (2019). Genome-wide analysis and environmental response profiling of phosphate-induced-1 family genes in rice (Oryza sativa). Biotechnol. Biotechnol. Equip. 33, 627–638. doi: 10.1080/13102818.2019.1604157
Ren, R., Gao, J., Yin, D., Li, K., Lu, C., Ahmad, S., et al. (2021). Highly efficient leaf base protoplast isolation and transient expression systems for orchids and other important monocot crops. Front. Plant Sci. 12:626015. doi: 10.3389/fpls.2021.626015
Ren, Z., Yu, D., Yang, Z., Li, C., Qanmber, G., Li, Y., et al. (2017). Genome-wide identification of the MIKC-type MADS-box gene family in Gossypium hirsutum L. Unravels their roles in flowering. Front. Plant Sci. 8:384. doi: 10.3389/fpls.2017.00384
Rigal, A., Doyle, S. M., and Robert, S. (2015). Live cell imaging of FM4-64, a tool for tracing the endocytic pathways in Arabidopsis root cells. Methods Mol. Biol. 1242, 93–103. doi: 10.1007/978-1-4939-1902-4_9
Roy, S. W., and Gilbert, W. (2005). Complex early genes. Proc. Natl. Acad. Sci. U.S.A. 102, 1986–1991.
Roy, S. W., and Gilbert, W. (2006). The evolution of spliceosomal introns: patterns, puzzles and progress. Nat. Rev. Genet. 7, 211–221. doi: 10.1038/nrg1807
Roy, S. W., and Penny, D. (2007). A very high fraction of unique intron positions in the intron-rich diatom Thalassiosira pseudonana indicates widespread intron gain. Mol. Biol. Evol. 24, 1447–1457. doi: 10.1093/molbev/msm048
Saini, S., Sharma, I., and Pati, P. K. (2015). Versatile roles of brassinosteroid in plants in the context of its homoeostasis, signaling and crosstalks. Front. Plant Sci. 6:950. doi: 10.3389/fpls.2015.00950
Sano, T., Kuraya, Y., Amino, S., and Nagata, T. (1999). Phosphate as a limiting factor for the cell division of tobacco BY-2 cells. Plant Cell Physiol. 40, 1–8. doi: 10.1093/oxfordjournals.pcp.a029464
Schroder, F., Lisso, J., Lange, P., and Mussig, C. (2009). The extracellular EXO protein mediates cell expansion in Arabidopsis leaves. BMC Plant Biol. 9:20. doi: 10.1186/1471-2229-9-20
Sousa, A. O., Assis, E. T. C. M., Pirovani, C. P., Alvim, F. C., and Costa, M. G. C. (2014). Phosphate-induced-1 gene from Eucalyptus (EgPHI-1) enhances osmotic stress tolerance in transgenic tobacco. Genet. Mol. Res. 13, 1579–1588. doi: 10.4238/2014.march.12.10
Subramanian, B., Gao, S., Lercher, M. J., Hu, S., and Chen, W.-H. (2019). Evolview v3: a webserver for visualization, annotation, and management of phylogenetic trees. Nucleic Acids Res. 47, W270–W275.
Tan, J., Miao, Z., Ren, C., Yuan, R., Tang, Y., Zhang, X., et al. (2018). Evolution of intron-poor clades and expression patterns of the glycosyltransferase family 47. Planta 247, 745–760. doi: 10.1007/s00425-017-2821-6
Tanaka, K., Asami, T., Yoshida, S., Nakamura, Y., Matsuo, T., and Okamoto, S. (2005). Brassinosteroid homeostasis in Arabidopsis is ensured by feedback expressions of multiple genes involved in its metabolism. Plant Physiol. 138, 1117–1125. doi: 10.1104/pp.104.058040
Vandepoele, K., Simillion, C., and Van de Peer, Y. (2003). Evidence that rice and other cereals are ancient aneuploids. Plant Cell 15, 2192–2202. doi: 10.1105/tpc.014019
Vida, T. A., and Emr, S. D. (1995). A new vital stain for visualizing vacuolar membrane dynamics and endocytosis in yeast. J. Cell Biol. 128, 779–792. doi: 10.1083/jcb.128.5.779
Wang, K., Wang, Z., Li, F., Ye, W., Wang, J., Song, G., et al. (2012). The draft genome of a diploid cotton Gossypium raimondii. Nat. Genet. 44, 1098–1103.
Wang, L., Yang, Z., Zhang, B., Yu, D., Liu, J., Gong, Q., et al. (2018). Genome-wide characterization and phylogenetic analysis of GSK gene family in three species of cotton: evidence for a role of some GSKs in fiber development and responses to stress. BMC Plant Biol. 18:330. doi: 10.1186/s12870-018-1526-8
Wang, M., Tu, L., Yuan, D., Zhu, D., Shen, C., Li, J., et al. (2019). Reference genome sequences of two cultivated allotetraploid cottons, Gossypium hirsutum and Gossypium barbadense. Nat. Genet. 51, 224–229. doi: 10.1038/s41588-018-0282-x
Wei, Z., and Li, J. (2020). Regulation of brassinosteroid homeostasis in higher plants. Front. Plant Sci. 11:583622. doi: 10.3389/fpls.2020.583622
Witte, C.-P., Noël, L., Gielbert, J., Parker, J., and Romeis, T. (2004). Rapid one-step protein purification from plant material using the eight-amino acid StrepII epitope. Plant Mol. Biol. 55, 135–147. doi: 10.1007/s11103-004-0501-y
Xu, G., Guo, C., Shan, H., and Kong, H. (2012). Divergence of duplicate genes in exon–intron structure. Proc. Natl. Acad. Sci. U.S.A. 109, 1187–1192. doi: 10.1073/pnas.1109047109
Yang, C.-J., Zhang, C., Lu, Y.-N., Jin, J.-Q., and Wang, X.-L. (2011). The mechanisms of brassinosteroids’ action: from signal transduction to plant development. Mol. Plant 4, 588–600. doi: 10.1093/mp/ssr020
Yang, Z. (2007). PAML 4: phylogenetic analysis by maximum likelihood. Mol. Biol. Evol. 24, 1586–1591. doi: 10.1093/molbev/msm088
Yang, Z., Gong, Q., Qin, W., Yang, Z., Cheng, Y., Lu, L., et al. (2017). Genome-wide analysis of WOX genes in upland cotton and their expression pattern under different stresses. BMC Plant Biol. 17:113. doi: 10.1186/s12870-017-1065-8
Yang, Z., Gong, Q., Wang, L., Jin, Y., Xi, J., Li, Z., et al. (2018). Genome-wide study of YABBY genes in upland cotton and their expression patterns under different stresses. Front. Genet. 9:33. doi: 10.3389/fgene.2018.00033
Yang, Z., Qanmber, G., Wang, Z., Yang, Z., and Li, F. (2020). Gossypium genomics: trends, scope, and utilization for cotton improvement. Trends Plant Sci. 25, 488–500. doi: 10.1016/j.tplants.2019.12.011
Yang, Z. R., Zhang, C. J., Yang, X. J., Liu, K., Wu, Z. X., Zhang, X. Y., et al. (2014). PAG1, a cotton brassinosteroid catabolism gene, modulates fiber elongation. New Phytol. 203, 437–448. doi: 10.1111/nph.12824
Yin, Y., Wang, Z. Y., Moragarcia, S., Li, J., Yoshida, S., Asami, T., et al. (2002). BES1 accumulates in the nucleus in response to brassinosteroids to regulate gene expression and promote stem elongation. Cell 109, 181–191. doi: 10.1016/s0092-8674(02)00721-3
Yoo, S.-D., Cho, Y.-H., and Sheen, J. (2007). Arabidopsis mesophyll protoplasts: a versatile cell system for transient gene expression analysis. Nat. Protoc. 2:1565. doi: 10.1038/nprot.2007.199
Yu, D., Qanmber, G., Lu, L., Wang, L., Li, J., Yang, Z., et al. (2018). Genome-wide analysis of cotton GH3 subfamily II reveals functional divergence in fiber development, hormone response and plant architecture. BMC Plant Biol. 18:350. doi: 10.1186/s12870-018-1545-5
Zeng, X., Li, H., Li, K., Yuan, R., Zhao, S., Li, J., et al. (2021). Evolution of the Brassicaceae-specific MS5-Like family and neofunctionalization of the novel MALE STERILITY 5 gene essential for male fertility in Brassica napus. New Phytol. 229, 2339–2356. doi: 10.1111/nph.17053
Keywords: brassinosteroid, GhEXO, G. hirsutum, phylogenetic analysis, gene duplication, ectopic expression
Citation: Li S, Liu Z, Chen G, Qanmber G, Lu L, Zhang J, Ma S, Yang Z and Li F (2021) Identification and Analysis of GhEXO Gene Family Indicated That GhEXO7_At Promotes Plant Growth and Development Through Brassinosteroid Signaling in Cotton (Gossypium hirsutum L.). Front. Plant Sci. 12:719889. doi: 10.3389/fpls.2021.719889
Received: 03 June 2021; Accepted: 05 August 2021;
Published: 16 September 2021.
Edited by:
Honghong Wu, Huazhong Agricultural University, ChinaReviewed by:
Xianchen Zhang, Anhui Agricultural University, ChinaLan Zhu, Huazhong Agricultural University, China
Copyright © 2021 Li, Liu, Chen, Qanmber, Lu, Zhang, Ma, Yang and Li. This is an open-access article distributed under the terms of the Creative Commons Attribution License (CC BY). The use, distribution or reproduction in other forums is permitted, provided the original author(s) and the copyright owner(s) are credited and that the original publication in this journal is cited, in accordance with accepted academic practice. No use, distribution or reproduction is permitted which does not comply with these terms.
*Correspondence: Zuoren Yang, yangzuoren@caas.cn; Fuguang Li, aylifug@caas.cn
†These authors have contributed equally to this work