- 1Area of Plant Physiology, Department of Organisms and Systems Biology, Oviedo University, Oviedo, Spain
- 2Functional Genomics Center, Zurich, Switzerland
- 3Swiss Institute of Bioinformatics, Lausanne, Switzerland
- 4Department of Plant and Microbial Biology & Zurich and Basel Plant Science Center, University of Zurich, Zurich, Switzerland
- 5Servicio Regional de Investigación y Desarrollo Agroalimentario (SERIDA), Finca Experimental La Mata, Grado, Spain
- 6Department of Biology and Geology, Physics and Inorganic Chemistry, Rey Juan Carlos University, Móstoles, Spain
- 7Department of Biochemistry and Molecular Biology, Institute of Oncology of the Principality of Asturias, Oviedo University, Móstoles, Spain
The gametophyte of ferns reproduces either by sexual or asexual means. In the latter, apogamy represents a peculiar case of apomixis, in which an embryo is formed from somatic cells. A proteomic and physiological approach was applied to the apogamous fern Dryopteris affinis ssp. affinis and its sexual relative D. oreades. The proteomic analysis compared apogamous vs. female gametophytes, whereas the phytohormone study included, in addition to females, three apogamous stages (filamentous, spatulate, and cordate). The proteomic profiles revealed a total of 879 proteins and, after annotation, different regulation was found in 206 proteins of D. affinis and 166 of its sexual counterpart. The proteins upregulated in D. affinis are mostly associated to protein metabolism (including folding, transport, and proteolysis), ribosome biogenesis, gene expression and translation, while in the sexual counterpart, they account largely for starch and sucrose metabolism, generation of energy and photosynthesis. Likewise, ultra-performance liquid chromatography-tandem spectrometry (UHPLC-MS/MS) was used to assess the levels of indol-3-acetic acid (IAA); the cytokinins: 6-benzylaminopurine (BA), trans-Zeatine (Z), trans-Zeatin riboside (ZR), dyhidrozeatine (DHZ), dyhidrozeatin riboside (DHZR), isopentenyl adenine (iP), isopentenyl adenosine (iPR), abscisic acid (ABA), the gibberellins GA3 and GA4, salicylic acid (SA), and the brassinosteroids: brassinolide (BL) and castasterone (CS). IAA, the cytokinins Z, ZR, iPR, the gibberellin GA4, the brassinosteoids castasterone, and ABA accumulated more in the sexual gametophyte than in the apogamous one. When comparing the three apogamous stages, BA and SA peaked in filamentous, GA3 and BL in spatulate and DHRZ in cordate gametophytes. The results point to the existence of large metabolic differences between apogamous and sexual gametophytes, and invite to consider the fern gametophyte as a good experimental system to deepen our understanding of plant reproduction.
Introduction
Understanding the factors underlying plant reproduction represents a challenge to which many research groups contribute given its enormous repercussions. In contrast to animals, in which the germ line differentiates early in development, flowering plants have no distinct germ line (Poethig et al., 1986). Instead, totipotent meristematic cells proceed through a long period of vegetative development before they eventually form complex sexual organs, the flowers (Vyskot and Hobza, 2004). Most angiosperms reproduce sexually through seeds, but the formation of seeds by asexual means is also possible, a process called apomixis. The production of seeds without sexual union is considered the holy grail of agriculture (Grossniklaus et al., 1998, 2001; Hofmann, 2010), but the molecular mechanisms operating behind apomixis remain unclear. Although plants with apomictic reproductive ability exceed 400 species (Grimanelli et al., 2003; Koltunow and Grossniklaus, 2003), no major seed crops are apomictic. Likewise, the distribution of apomictic taxa across plant lineages is uneven, with estimations of 0.1% in angiosperms, up to 10% in ferns, and with little or no evidence of its existence in gymnosperms, mosses, liverworts or hornworts (Lovis, 1978; Asker and Jerling, 1992; Dyer et al., 2012). Apomixis is especially frequent in the Dryopteridaceae family, which, together with Pteridaceae, comprises around 70% of the reported apomictic fern species (Liu et al., 2012).
Ferns represent a major vascular plant group, in which haploid and diploid generations are completely separated, and the gametophyte is an appealing experimental system to deal with reproduction. Moreover, in ferns, apomixis is an important mode of asexual reproduction, which has evolved several times independently within the group (Ekrt and Koutecký, 2016). Apomixis in ferns includes “apogamy,” the formation of sporophytes from somatic cells of the prothallium, and “agamospory” (or diplospory), which represents the production of unreduced (diplo) spores in the fronds. In contrast, in gametophytes reproducing sexually, there are two organogenic events, producing antheridia and archegonia, bearing the male and female gametes, respectively. Performing molecular analyses in ferns has been elusive, as they exhibit higher chromosome numbers and larger genomes than mosses and seed plants (Barker and Wolf, 2010), which made it difficult to obtain genomic data. However, the advent of next-generation sequencing (NGS) technologies, by which it is possible to characterize the transcriptome in plants, represents a small but information reach-target compared to complete genome characterization (Ward et al., 2012). The variation in gene expression, induced by whatever environmental or endogenous conditions, can be examined in non-model organisms because these techniques have become more feasible as automation and efficiency have reduced costs. Until present, some transcriptome and proteome data sets have been published for ferns, which include the species Pteridium aquilinum (Der et al., 2011), Ceratopteris richardii (Salmi et al., 2010; Cordle et al., 2012), Blechnum spicant (Valledor et al., 2014), Lygodium japonicum (Aya et al., 2015), and D. affinis ssp. affinis (Grossmann et al., 2017; Wyder et al., 2020). Over the last case, both transcriptomic and proteomic analyses were performed by using next-generation sequencing (NGS) and shotgun proteomics by tandem mass spectrometry (MS/MS).
Several papers have been published to deepen on sexual and asexual reproduction in ferns. Two of them were driven in the model fern species C. richardii, looking for genes associated with female expression, and also male expression mediated by the pheromone antheridiogen in gametophytes (Atallah et al., 2018; Chen et al., 2019). Also, a comparative transcriptome analyses was done by Fu and Chen (2019) between apogamous and sexual gametophytes in Adiantum reniforme var. sinense. Previously, Bui et al. (2017) in C. richardii reported an AINTEGUMENTA-LIKE unigene, inducing the sporophyte formation without fertilization. It mirrors BABY BOOM (BBM) gene, a transcription factor of AP2/ERF family, which in angiosperms are known to promote somatic embryogenesis. Likewise, Domzalska et al. (2017) found proteins differently regulated during somatic embryogenesis in the tree fern Cyathea delgadii. Freshly, in the apogamous species, we have done a RNA-Seq approach to compare gene expression profiles of one- and two-dimensional gametophytes, finding several thousands of genes differentially expressed, and related to different aspect of either vegetative or reproductive behavior of the gametophyte (Wyder et al., 2020). In summary, with the increasing availability of genomic data from non-model species, better approaches will improve the sensitivity in protein identification for species distantly related to models.
On the other hand, phytohormones take part in the regulation of almost all phases of plant development, and also mediate the responses to various environmental stresses (Hicks, 1894; Rademacher, 2000; Dobrev et al., 2002). In ferns, growth and development of gametophyte and sporophyte are controlled by plant growth regulators, receiving especial attention their applications on morphogenesis, and its great repercussion on the sporophyte multiplication of ornamental ferns (Amaki and Higuchi, 1992; Fernández and Revilla, 2003; Somer et al., 2010; Rybczynski et al., 2018; Singh and Johari, 2018). The impact of phytohormones on gametophyte reproduction has been scrutinized in several species, some of them governed by an antheridiogen system, such as B. spicant, being these compounds devoted to promote genetic exchange, and linked chemically to gibberellin-related diterpenoids (Yamane, 1998; Menéndez et al., 2006a,b; Kazmierczak, 2010; Tanaka et al., 2014; Valledor et al., 2014). In B. spicant, Menéndez et al. (2006b) found similar levels of the gibberellins GA4, GA7, and GA20 in male and female gametophytes, while ABA has been reported to act as an antheridiogen antagonist in C. richardii (Warne and Hickok, 1989). In the sexual fern Asplenium nidus, which lacks antheridiogens, a significant increase in the content of the cytokinins iP and iPR was found in female gametophytes, and also qualitative differences in the content of gibberellins between the gametophyte and sporophyte generations were reported (Menéndez et al., 2011). In the gametophytes of Polystichum aculeatum and Dryopteris filix-mas, qualitative and quantitative changes of phytohormone content associated with the regulation of growth and gametangia formation, were recently documented (Kosakivska et al., 2019, 2020). On the other hand, variations in the content of phytohormones have been noted in D. affinis ssp. affinis (Menéndez et al., 2006c), associated to apogamy process. Yet, little attention has been paid so far to other phytohormones such as SA or brassinosteroids in basal branching vascular plants (i.e., lycophytes and ferns) (Sun et al., 2010; Choudhary et al., 2012). The former was traditionally associated with pathogen defense, together with jasmonic acid (de Vries et al., 2018), and the latter, in junction with auxins and gibberellins, is part of a key subset of plant hormones considered major determinants of plant growth and development (Ross and Reid, 2010; Gómez-Garay et al., 2018).
For a better understanding of the functions of the phytohormones and even possible interactions between them, exhaustive determination of their contents is of great importance (Bai et al., 2010). Analyses of phytohormones have focused on auxins, gibberellins, cytokinins, abscisic acid, or ethylene, while others like jasmonates or brassinosteroids were recently added, as standards and protocols became available. In the last few years, the advent of rapid, sensitive, accurate and efficient methods, even when small amounts of sample are available, has made possible to broaden the number of compounds analyzed (Du F. et al., 2012; Porfírio et al., 2016; Delatorre et al., 2017). HPLC-MS is the most accurate method to perform quantitative analysis of endogenous phytohormones or plant regulators (Pan et al., 2008, 2010; Delatorre et al., 2017), and at present day, a step forward is the use of UHPLC to detect those small amounts of phytohormones in fresh tissue, giving even better results on a wide spectrum of plant hormones.
In this work, a physiological and proteomic study was carried out in the fern D. oreades and its close relative D. affinis ssp. affinis (hereafter referred to as D. affinis). D. oreades is a sexual diploid and thus its gametophytes are haploid. D. affinis is also diploid but has two distinct genomes, one from D. oreades and one from an unknown “pure” D. affinis ancestor (Fraser-Jenkins, 1980). D. affinis is obligately apogamous and its gametophytes are diploid with the same genome constitution as that of sporophytes (Sheffield et al., 1983). Thus, this species pair provides an experimental system for investigating the gene expression an phytohormonal changes linked to sexual or apogamous reproduction. Specifically, our analyses involved (1) a proteomic comparison between sexual and apogamous gametophytes, and (2) the assessment of the endogenous content of fourteen phytohormones in sexual gametophytes and in three developmental stages of apogamous gametophytes: one-dimensional or filamentous; and two-dimensional or spatulate, and heart-shaped or cordate.
Materials and Methods
Plant Material and Growth Conditions
Spores of D. affinis were obtained from sporophytes growing in Turón valley (Asturias, Spain), 477 m a.s.l., 43°12′10″N−5°43′43″W. In the case of D. oreades, spores were collected from sporophytes growing in Burgos, Neila lagoons, 1920 m a.s.l., 42°02′48″N−3°03′44″W. Spores were released from sporangia, soaked in water for 2 h, and then washed for 10 min with a solution of NaClO (0.5%) and Tween 20 (0.1%). Then, they were rinsed three times with sterile, distilled water. Spores were centrifuged at 1,300 g for 3 min between rinses, and then cultured in 500-mL Erlenmeyer flasks containing 100 mL of liquid Murashige and Skoog (MS) medium (Murashige and Skoog, 1962). Unless otherwise noted, media were supplemented with 2% sucrose (w/v), and the pH adjusted to 5.7 with 1 or 0.1 M NaOH. The cultures were kept at 25°C under cool-white fluorescent light (70 μmol m−2s−1) with a 16:8 h light: dark photoperiod, and put on an orbital shaker (75 rpm).
Following spore germination, gametophytes of both species go through three sequential growth stages, as in most ferns (Nayar and Kaur, 1971): filamentous, because of one-dimensional growth; spatulate, when growth becomes two-dimensional; and cordate (heath-shaped), when a notch meristem is formed in the middle of the upper margin. Gametophytes of D. affinis were collected at these three stages (Figures 1A–C), and, in the case of cordate ones, with visible signs (under a light microscope) of an evolving apogamic center (Figure 1D), composed of smaller and darker isodiametric cells. Filamentous gametophytes were collected 30 days after the start of spore culture. Spatulate and cordate gametophytes were obtained by transferring 30-day old filamentous gametophytes to 200 mL flask containing 25 mL of MS medium supplemented with 2% sucrose (w/v) and 0.7% agar, and being collected after 20 or 30 additional days, respectively. Gametophytes of D. oreades needed around 6 months to become cordate and reach sexual maturity (Figures 1E,F). They were then picked up and, in all cases, only female reproductive organs (i.e., archegonia) were observed under a light microscope. Samples of the three types of apogamous gametophytes (filamentous, spatulate and cordate) and sexual cordate, were weighted before and after being lyophilized for 48 h (Telstar-Cryodos) and stored in Eppendorf tubes on a freezer at −80°C until required.
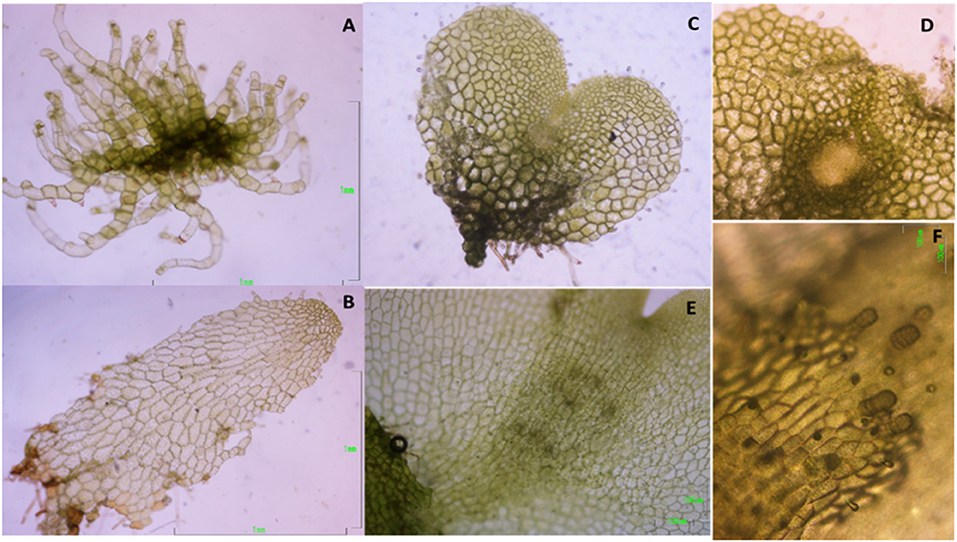
Figure 1. Morphological features in the growth stages of the apogamous fern Dryopteris affinis: (A) filamentous; (B) spatulate; (C) cordate or heart-shaped; (D) detail of first steps in the embryo development; and its sexual relative D. oreades: (E) archegonium cushion in the middle of gametophyte; (F) archegonia.
Protein Extraction
From the cordate apogamous and cordate sexual gametophytes (three samples each), an amount of 20 mg dry weight of gametophytes were homogenized using a Silamat S5 shaker (Ivoclar Vivadent, Schaan, Liechtenstein). Homogenized samples were solubilized in 800 μL of buffer A [0.5 M Tris-HCL pH 8.0, 5 mM EDTA, 0.1 M Hepes-KOH, 4 mM DTT, 15 mM EGTA, 1 mM PMSF, 0.5% PVP and 1 × protease inhibitor cocktail (Roche, Rotkreuz, Switzerland)] using a Potter homogenizer (Thermo Fisher Scientific, Bremen, Germany).
Proteins were extracted in two steps: first, the homogenate was subjected to centrifugation at 16,200 g for 10 min at 4°C on a table top centrifuge and, second, the supernatant was subjected to ultracentrifugation at 117–124 kPa (~100,000 g) for 45 min at 4°C. Post-ultracentrifugation the supernatant contained the soluble protein fraction. The pellet from the first ultracentrifugation was re-dissolved in 200 μL of buffer B (40 mM Tris base, 40 mM DTT, 4% SDS, 1 × protease inhibitor cocktail (Roche, Rotkreuz, Switzerland) to extract membrane proteins using ultracentrifugation as described before. The supernatant after the second ultracentrifugation step contained the membrane protein fraction. Ultracentrifugation was performed using an Airfuge (Beckman Coulter, Pasadena, CA).
Protein concentrations were determined using a Qubit Fluorometer (Invitrogen, Carlsbad, CA). For 1 D gel electrophoresis, ~1 mg protein per each soluble and membrane fraction was loaded separately onto a 0.75 mm tick, 12% SDS-PAGE mini-gel. Samples were treated with sample loading buffer and 2 M DTT, heated at 99°C for 5 min, followed by a short cooling period on ice, and loaded onto the gel. 1D gel electrophoresis was performed at 150 V and 250 mA for 1 h in 1X Running Buffer.
Protein Separation and In-gel Digestion
After 1D SDS-PAGE each gel lane was cut into six 0.4 cm wide sections using a custom-made gel cutter, resulting in 48 slices. These slices were further fragmented into smaller pieces and subjected to 10 mM DTT (in 25 mM AmBic pH8) for 45 min at 56°C and 50 mM Iodoacetamide for 1 h at room temperature in the dark, prior to trypsin digestion at 37°C overnight (Baerenfaller et al., 2008). The small pieces were washed twice with 100 μL of 100 mM NH4HCO3/50% acetonitrile, and washed once with 50 μL acetonitrile. All three supernatants were discarded and peptides digested with 20 μL trypsin (5 ng/μL in 10 mM Tris/2 mM CaCl2, pH 8.2) and 50 μL buffer (10 mM Tris/2 mM CaCl2, pH 8.2). After microwave-heating for 30 min at 60°C, the supernatant was removed and gel pieces extracted once with 150 μL 0.1% TFA/50% acetonitrile. All supernatants were combined and dried, and samples were then dissolved in 15 μL 0.1% formic acid/3% acetonitrile and transferred to auto-sampler vials for liquid chromatography (LC)-MS/MS where 5 μL were injected.
Protein Identification, Verification, and Bioinformatic Downstream Analyses
MS/MS and peptide identification (Orbitrap XL) were performed accordingly (Grossmann et al., 2017). Scaffold software (version Scaffold 4.2.1, Proteome Software Inc., Portland, OR) was used to validate MS/MS-based peptide and protein identifications. Mascot results were analyzed together using the MudPIT option. Peptide identifications were accepted if they scored better than 95.0% probability as specified by the Peptide Prophet algorithm with delta mass correction, and protein identifications were accepted if the Protein Prophet probability was above 95%. Proteins that contained same peptides and could not be differentiated based on MS/MS alone were grouped to satisfy the principles of parsimony using scaffolds cluster analysis option. Only proteins that met the above criteria were considered positively identified for further analysis. The amount of random matches was evaluated by performing the Mascot searches against a database containing decoy entries and checking how many decoy entries (proteins or peptides) passed the applied quality filters. The peptide FDR and protein FDR was estimated at 2 and 1%, respectively, indicating the stringency of the analyses.
A semi-quantitative spectrum counting analysis was conducted. The “total spectrum count” for each protein and each sample was reported, and these spectrum counts were averaged for each species, D. affinis (DA) and D. oreades (DO). Then, one “1” was added to each average (DA and DO) in order to prevent division by zero and then a log2-ratio of the averaged spectral counts from DA vs. DO was calculated. Proteins were considered to be differentially expressed if this log2Ratio is above 0.99. This refers to at least twice as much peptide spectrum match (PSM) assignments in one group compared to the other. Also, in order to have a functional understanding of the identified proteins, we blasted the whole protein sequences of all identified proteins against Sellginella moellendorfii and Arabidopsis thaliana Uniprot sequences and retrieved the best matching identifier from each of them, along with the corresponding e-value, accepting blast-hits which evaluate below 1E-7. These better described ortholog identifiers are then used in further downstream analysis.
Protein Analysis Using STRING-DB Platform
The identifiers of the genes from the apogamous and sexual gametophyte samples were used as input for String version 11.0 analysis and networks were built based on high confidence in the ranking (0.7).
Endogenous Phytohormone Analyses
The extraction of phytohomones was done from 0.2 g fresh weight of each of the four gametophyte samples (filamentous, spatulate and cordate apogamous; and cordate sexual), according to the protocol of Pan et al. (2008) with modifications from Delatorre et al. (2017), which avoid derivatization and purification of the samples, and six replicates were considered for sample. The phytohormones analyzed were: ABA, IAA, the cytokinins iP, iPR, Z, ZR, DHZ, DHZR, the gibberellins GA3 and GA4, SA, and the brassinosteroids BL and CS (Sigma-Aldrich, St. Louis, MO, USA). The following deuterated standards were employed: IAA-d5, SA-d6, DHZ-d3, and ABA-d6 (20 ng); GA9-d2 and CS-d5 (40 ng) and BA-d7 (10 ng). All of them were supplied by Olchemim Ltd. (Czech Republic) except d6-SA obtained from Sigma Aldrich (St. Louis, MO, USA). Starting from these internal standards, known samples homogenized in liquid N2, were extracted with 1 ml of extraction buffer (2-propanol/Mili Q water/Hydrochloric acid; 2:1:0.002; v/v/v) in 2 mL propylene Eppendorf tubes (Eppendorf Safe Lock England), and agitated by repeated inversion (60 rpm) for 20 min, at 4°C, in the dark. The resulting homogenate was transferred to a teflon tube (Oak Rifge Centrifuge Tube, Thermo Scientific, England) with 1.8 ml dichloromethane and reextracted by repeated and inverted agitation for 30 min, at 4°C in the dark. After 30 min standing, three phases were obtained, an aqueous, a material debris and an organic phase. The organic bottom phase was recovered and the intermediate debris phase was also re-extracted again. The propanol of combined extracts was evaporated under N2 flow. Resulting dried extracts were resuspended in 150 μL of 100% methanol and filtered through a 0.2 μm regenerated cellulose Captiva Premium Syringe filter (Agilent Technologies, California, USA). The chromatographic separation takes place on a reverse phase chromatographic column (Zorbax SB-C18 2.1 × 50 mm) coupled to a Zorbax plus eclipse pre-column (C18 2.1 × 5 mm) at 40°C. Two solvents are used as mobile phases with a flow rate of 0.45 mL / min: MeOH acidified with 0.1% formic acid (solvent A) and ammonium formate 10 mM, pH 4 (solvent B).
Samples were injected into UHPLC System (1290 Infinity binary LC system, Agilent Technologies, Madrid Spain). The UHPLC was coupled to a Triple Quadrupole (6460 Triple Quad, LC/MS equipped with ESI-Ion Source). All compounds were separated and quantified following the protocol described by Delatorre et al. (2017).
Statistical Analyses of Phytohormone Content
Deviation from normality and homogeneity of variance were tested respectively with Shapiro-Wilk and Levene tests. One-way ANOVA was carried out with a Tukey-HSD test to group samples. From the obtained means squares estimates, values were used to identify the role played by each kind of gametophyte in overall variability through the Principal Component Analysis (PCA). This PCA was performed on the mean values recorded for 14 phytohormones assessed from four kind of gametophytes. From this PCA, a bioplot graph was drawn in an attempt to classify the endogenous content of phytohormones into similarity groups. All statistical analyses were completed in R environment with R Studio (Team, 2016) or the free software from Past.uio 3.26 (Hammer, 2001).
Results
Identification of Differentially Expressed Genes by LC-MS/MS
The proteomic analyses generated a total of 879 quantifiable proteins (Supplementary Table 1), rejecting those proteins not having a homolog and those having two DA/DO proteins the same homolog and repetitions. The “differential” protein regulation between the apogamous species and its sexual relative took into account proteins that were not “borderline,” exhibiting a total of three assigned spectra, and rejecting those with less counts. According to this criterium, from the total number of annotated proteins, 206 and 166 were upregulated in 2 month-old apogamictic and sexual gametophytes, respectively (Figure 2). One-third of total annotated proteins (35.7%) had BLAST hits to proteins from A. thaliana, followed by Oryza sativa (4.7%). The remain matches of proteins are distributed among several species such as the seed plants Nicotiana tabacum, Zea mays, Glycine max, Solanum tuberosum, etc., animals like Bos taurus, Mus musculus, Caenorhabditis elegans, seedless plants like the moss Physcomitrella patens or the lycophyte Sellaginella moellemdorfii, and microorganisms such as Saccharomyces cerevisiae, Bacillus sp., etc. (Figure 3).
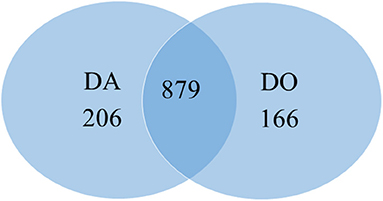
Figure 2. Venn diagram totaling the number of proteins similarly and differently regulated in heart-shaped gametophytes from the apomictic fern Dryopteris affinis (DA), and its sexual relative D. oreades (DO).
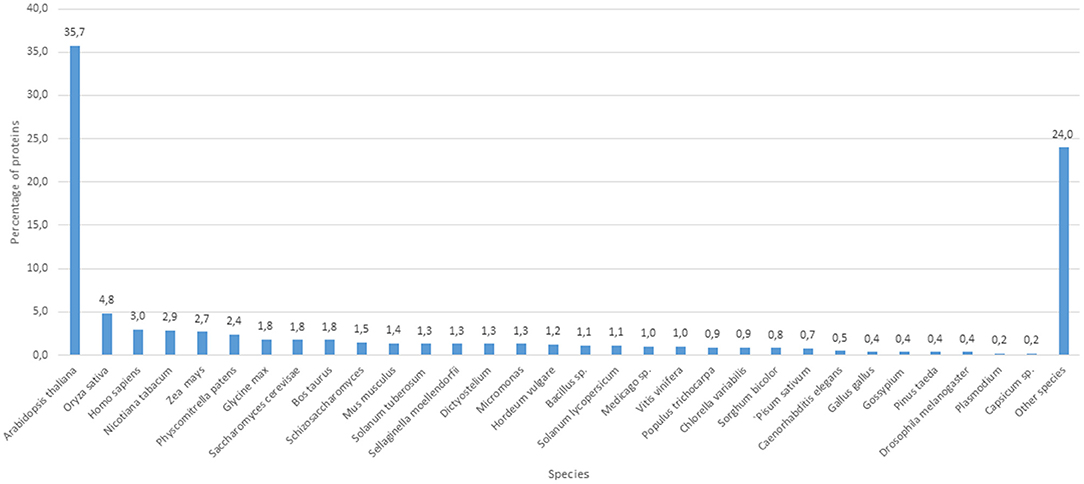
Figure 3. Number of protein clusters obtained from the apogamous gametophytes of Dryopteris affinis and its sexual relative D. oreades, with the best hits to species belonging to the phylogenetic groups specified.
Over-represented Categories for Differentially Regulated Proteins in Apogamous and Its Sexual Relative Gametophyte
The collections of differentially regulated proteins were analyzed by using the STRING database (http://String-db.org). Regarding those of the apogamous species, the results show 206 nodes and 1,047 edges, and a PPI enrichment p-value = 1.0 e−16; in the sexual relative, 166 nodes and 259 edges were scored, with a PPI enrichment p-value = 5.55 e−16. In both of cases, data reflected that our proteins have more interactions among themselves than what would be expected for a random set of proteins of similar size, drawn from the genome. Thus, the proteins are at least partially biologically connected, as a group.
In order to obtain a better knowledge about the function of both collections of proteins, Gene Ontology (GO), and KEGG enrichment analyses provided by the String platform were obtained. In total, 206 and 166 proteins differentially regulated were clustered to a number of GO and KEGG pathways significantly enriched (FDR's). Regarding the GO databank, proteins were checked according to standard categories of biological function, molecular function and cellular components (Figure 4). In both species, more than half of differentially regulated proteins (60–70%) counted for general metabolic and cellular processes (Figure 4A). The apogamous species, totals more proteins involved in the primary metabolism like amino acids, peptides and proteins (including folding, transport and proteolysis), nucleic acids and cofactors, nitrogen and sulfur metabolism, ribosome biogenesis, gene expression and translation. Instead, the sexual counterpart accumulated more proteins involved in generation of precursor metabolites and energy, and photosynthesis. In addition, it was found more proteins involved in response to hormones in this species. There are also biological functions such as response to stimulus or metabolism linked to carbohydrates, in which both species show a similar increase in the number of proteins scored but different profiles when detailing each kind of processes.
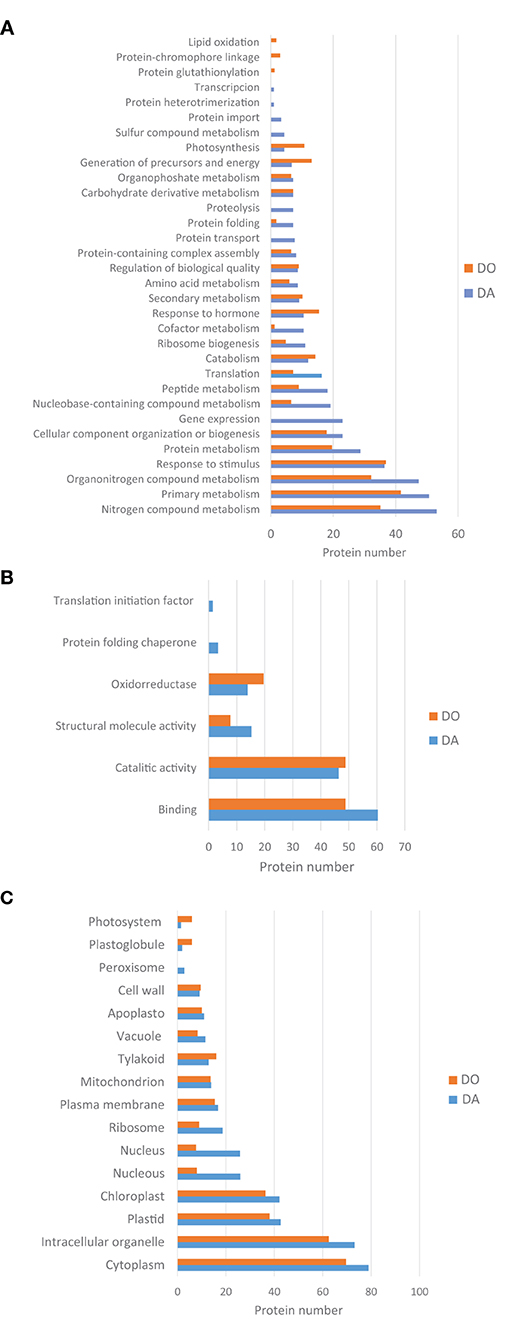
Figure 4. GO classification of proteins differentially upregulated in the apogamous fern Dryopteris affinis and its sexual relative D. oreades, in the three main categories: (A) biological function; (B) molecular function; and (C) cellular component.
Under “molecular function” category, the list of proteins differentially regulated was headed by binding activity in both species, being a ten percent higher in the apogamous gametophyte (Figure 4B). This activity is dominated by organic cyclic compounds and ion binding, in both gametophytes, respectively, followed by oxidoreductase activity, higher in the sexual gametophyte. The apogamous gametophytes expressed more proteins related to structural molecule activity, protein folding chaperone and translation initiation factor.
Additionally, under “cellular components” most part of the proteins was linked to cytoplasm and intracellular organelles, especially plastids and chroloplasts (Figure 4C). Both species upregulated proteins involved in cell wall, plasma membrane, and mitochondrion. In the apogamous gametophytes, it was significant the presence of proteins linked to nucleus, ribosome and peroxisome. On the other hand, in the sexual relative, increased the content of proteins associated to the photosynthetic machinery such as tylakoids, plastoglobule, and photosystems.
KEGG clustering showed an important expression of proteins associated to ribosome, aminoacid metabolism and proteasome in both species, being higher in the apogamous gametophyte (Figure 5). In this species, proteins drop in protein processing in endoplasmic reticulum, porphyrin and chlorophyll metabolism, spliceosome, RNA degradation, arginine biosynthesis, etc. In the sexual counterpart, proteins are involved in starch and sucrose metabolism, amino sugar and nucleotide sugar metabolism, photosynthesis, oxidative phosphorylation, lipid metabolism, etc. A selection of those proteins differentially regulated is reported in Tables 1, 2.
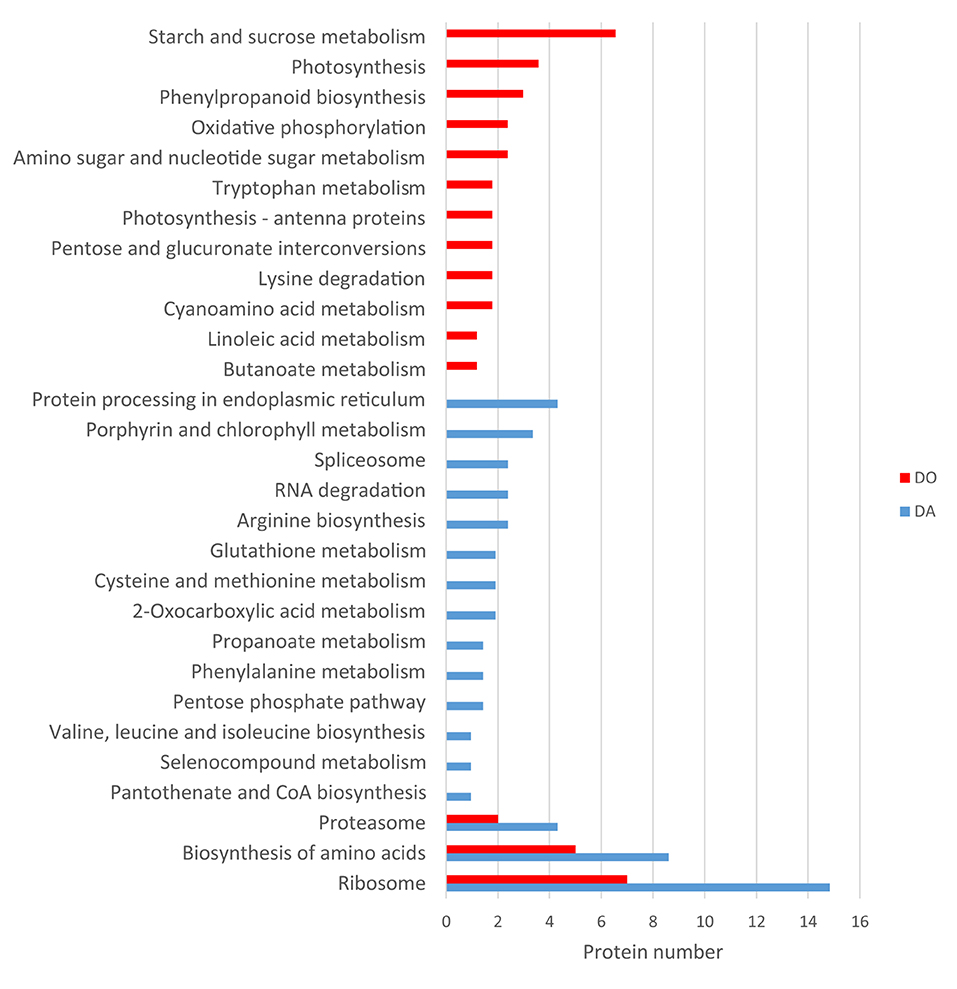
Figure 5. KEGG classification of proteins differentially upregulated in the apogamous fern Dryopteris affinis and its sexual relative D. oreades.
Quantification of Endogenous Phytohormones
The endogenous levels of the plant growth regulators analyzed are shown in Table 3. The auxin IAA was found in a greater quantity in D. oreades gametophytes than in any growth stage of D. affinis, and in spatulate compared to filamentous and cordate gametophytes. Regarding cytokinins, BA peaked in the filamentous gametophytes of D. affinis, and Z and ZR showed low levels in D. affinis (<0.1 ng/g FW) and markedly higher in the sexual counterpart.
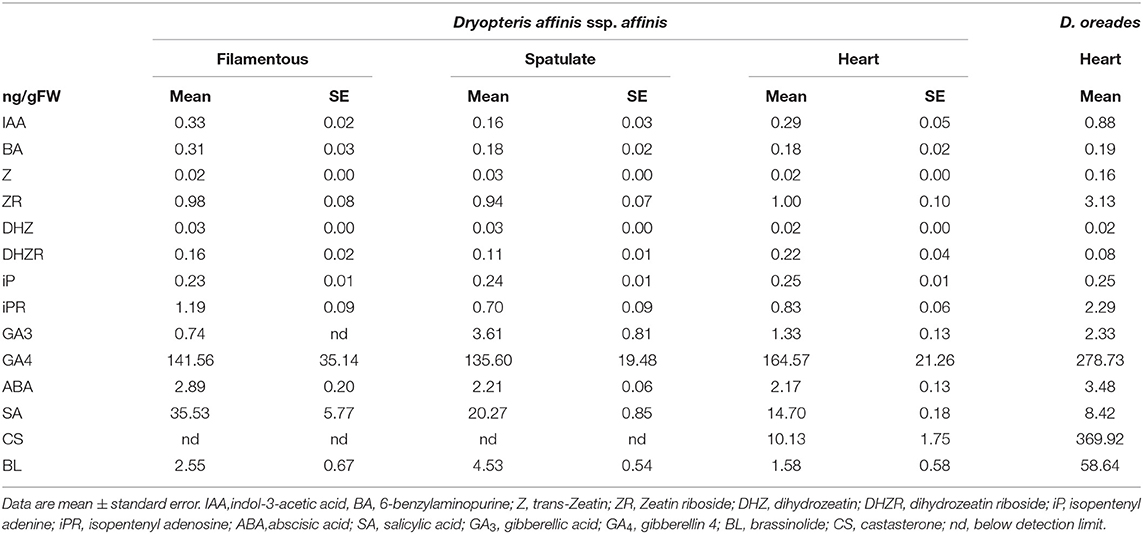
Table 3. Endogenous content of phytohormones in three growth stages of apogamous fern Dryopteris affinis and one stage of its relative D. oreades.
No differences were found in the content of DHZ, while the levels of its riboside, DHZR, significantly shrank in spatulate gametophytes of D. affinis compared to cordate gametophytes, dropping considerably in the sexual parent. Finally, no differences were found regarding the isoprenoid cytokinin iP, while the levels of its riboside, iPR, was lower in the spatulate and cordate than in the filamentous apogamous gametophytes, and also than in the sexual gametophytes.
The levels of plant growth inhibitor ABA increased in the sexual gametophytes and no differences were observed among the three developmental stages of apogamous gametophytes. Salicylic acid showed substantial differences among the four gametophyte samples. Levels up to 35 ng/g FW were detected in filamentous apogamous gametophytes, falling significantly in sexual ones. In relation to the tested gibberellins, high levels of GA4 were noticed in sexual gametophytes, being also significant in apogamous ones. In addition, GA3 reached a maximum in spatulate apogamous gametophytes. Finally, on one hand, the brassinosteroid CS was undetected from filamentous and spatulate apogamous samples, and low in cordate apogamous gametophytes. On the other hand, this phytohormone was 10 times more abundant in sexual than in apogamous gametophytes. BL is 25 times more abundant in sexual than in apogamous gametophytes (but only one sample was achieved). In addition, significant differences were found between spatulate and the other apogamous stages.
Principal Component Analyses (PCA) revealed that one component (PC1) included the most part of the variance (90%), splitting sexual and apogamous gametophytes (Figure 6). Biplot shows the phytohormones grouping into the four gametophyte samples. DHZ and DHZR are common to filamentous and spatulate D. affinis gametophytes, and GA3 is the hormone which differentiates both groups of samples. Apogamous filamentous samples showed variation with a great dispersion, and they shared differences with both spatulate and cordate stages by the cytokinin BA and salicylic acid.
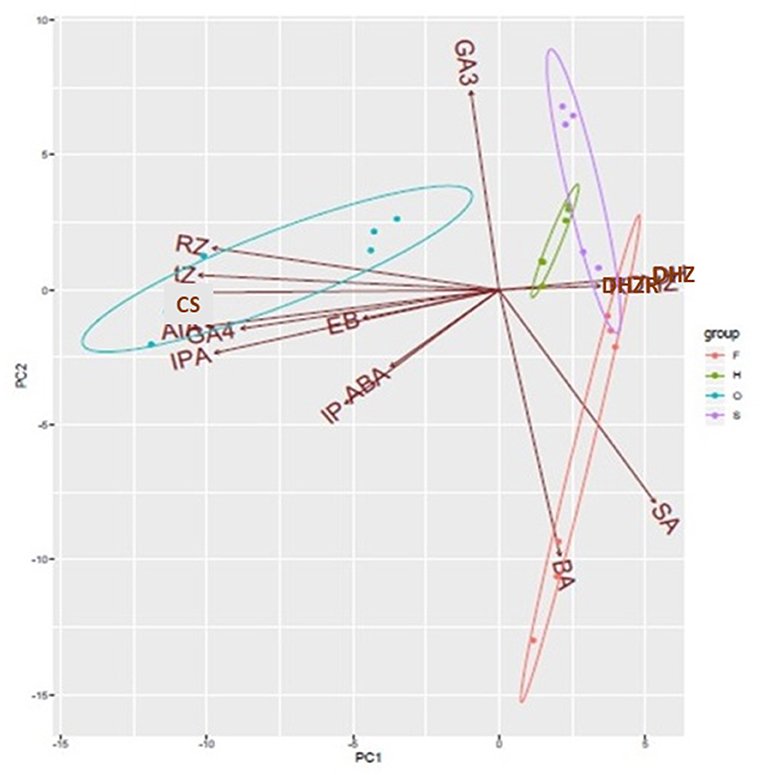
Figure 6. PCA biplot showing the contribution of all variables to the variation in the endogenous content of phytohormones in filamentous (F), spatulate (S), and heart-shaped (H) gametophytes of the apogamous species Dryopteris affinis, and heart-shaped gametophytes (O) of its sexual relative D. oreades. Ellipses encircle group samples from the same origin. IAA, indol-3-acetic acid; BA, 6-benzylaminopurine; Z, trans-Zeatin; Zr, Zeatin riboside; DHZ, dihydrozeatin; DHZR, dihydrozeatin riboside; iP, isopentenyl adenine; iPR, isopentenyl adenosine; ABA, abscisic acid; SA, salicylic acid; GA3, gibberellic acid; GA4, gibberellin 4; BL, brassinolide; CS, castasterone.
Discussion
Our results revealed substantial differences in the protein content between apogamous and sexual gametophytes of two closely related woodfern species. Specifically, D. affinis and D. oreades showed differences in terms of the expression of proteins involved in some biological, molecular and cellular aspects of plant development. Part of these differences can be explained by the distinct reproductive systems. Furthermore, although both species share the D. oreades genome, D. affinis has an additional genome (Fraser-Jenkins, 1980) whose expression also contributes to the divergent proteomic profiles.
Apogamous Gametophytes Upregulate Proteins Related to Protein Folding, Transport, Targeting, Proteolysis, Ribosome Biogenesis, Gene Expression and Translation
It has been reported an important role of protein metabolism on apomixis in angiosperms (Schmidt, 2020). Certainly, cellular processes related to proteins, such as in transport, folding, targeting or proteolysis are upregulated in the gametophyte of our apogamous species. For instance, we found RAN3, a small GTP-binding nuclear protein, required for the import of protein into the nucleus, RNA export, chromatin condensation and the control of cell cycle (Yano et al., 2006); and a puromycin-sensitive aminopeptidase, which plays an essential role during prophase I of meiosis for correct meiotic recombination in both male and female gametophytes (Sánchez-Morán et al., 2004). In addition, protein folding chaperones such as HSP60, HSP60-2, and the translation initiation factors FUG1, IF3-4 or ELF 3C, were found. Then, two signal chloroplastic recognition particles: cpSRP43 (CAO) and cpSRP54, involved in protein heterotrimerization, deserve to be mentioned. They act as a highly specific chaperones for LHCPs, preventing aggregation and being able to dissolve aggregates (Falk and Sinning, 2010; Falk et al., 2010). G-protein signaling is an integral part of the G-protein network important in many agronomic traits, including architecture and grain yield, and which is lost in many monocots (Bhatnagar and Pandey, 2020). In D. affinis, another interesting annotation is the chaperone protein htpG family protein or SHD, which encodes an ortholog of GRP94, an ER-resident HSP90-like protein involved in regulation of meristem size and organization. This protein is suggested to be required for the correct folding and/or complex formation of CLV proteins (Ishiguro et al., 2002), and it is also involved in resistance to tunicamycin- or high calcium-induced ER stress (Chong et al., 2015). Indeed, Hsp70s are key components that facilitate folding of de novo synthesized proteins, assist translocation of precursor proteins into organelles, and are responsible for degradation of damaged proteins under stress conditions (Lee et al., 2009).
In apogamous gametopytes, we also found proteins related to the ribosome organelle, including a wide number of processes such as ribosome biogenesis, processing, gene expression, translation and cell cycle control. As an example, the protein CPR, member of metallopeptidase M24 family, is involved in the regulation of rRNA processing and ribosome assembly, and is required for expression of cell cycle genes such as CYCD3-1, RNR2A, and CDKB1-1, playing a role in the entry into mitosis. Besides, it promotes, in a dose- and auxin-dependent manner, organ growth by stimulating both cell proliferation and expansion, via the regulation of RBR1 levels (Horváth et al., 2006). The list also included an emb3010 or 40S ribosomal protein S6-2, which may play an important role in controlling cell growth and proliferation through the selective translation of particular classes of mRNAs (Zhou et al., 2015).
Ribosome assembly and function are correlated with the activity of RNA binding proteins, often associated to ribonucleoprotein complexes of RNA as well as helicases (Schmidt, 2020). The former includes proteins like the phosphoprotein AtLa1, required for normal ribosome biogenesis and embryogenesis (Fleurdépine et al., 2007), and the latter, a 50S ribosomal protein L13 or embryo defective 1473, involved in translation and biological processes such as embryo development ending in seed dormancy or stress tolerance (Moin et al., 2016).
RNA helicases are considered of crucial importance for gene regulation of developmental processes, exerting an epigenetic control. In the apogamous species, two helicases, to unwind nucleic acids, were reported: the emb1138, member of the DEAD-box ATP–dependent RNA helicase 3, and At3G62310, a probable pre-mRNA-splicing factor ATP-dependent RNA helicase DEAH2, which might be involved in pre-mRNA splicing. The DEAD-box helicases are involved in various aspects of RNA metabolism, including nuclear transcription, pre-mRNA splicing, ribosome biogenesis, nucleocytoplasmic transport, translation, RNA decay and organellar gene expression. Different studies provided evidence of RNA helicases to be likely involved in regulating apomictic development (Schmidt, 2020). In A. thaliana, mutants for the RNA helicase MNEME form unreduced gametophytes, resembling apospory (Schmidt et al., 2011). Also, in apomictic Brachiaria brizantha and in Hypericum perforatum, BrizHELIC and a of MATERNAL EFFECT EMBYO ARREST29, are differentially expressed in tissues of apomictic plants (Silveira et al., 2012; Barcaccia and Albertini, 2013). In our work, a homologue of MATERNAL EFFECT EMBRYO ARREST 59 (MEE59) is up regulated in the apogamous gametophyte, but its biological function is unknown. Furthermore, we found TFL2, a chromo domain-containing protein LHP1, structural component of heterochromatin, and the transducin/WD40 repeat-like superfamily protein, which is a component of the PAF1 complex (PAF1C), involved in epigenetic gene repression of several floral homeotic genes, such as FLT, that regulates flowering time, and is required for maintenance of vernalization-induced repression of FLC (Kotake et al., 2003; Zhang et al., 2003; Xu and Shen, 2008). An interesting finding is the ortologue of the transcriptional regulation protein Methyl-CpG-binding domain-containing protein 10, required for nucleolar dominance that leads to the silencing of rRNA genes inherited from one progenitor in interspecific hybrids (Preuss et al., 2008). It must be noted that D. affinis ssp. affinis is actually a fertile hybrid in which both sporophyte and gametophyte have two genomes from two different species (Fraser-Jenkins, 1980). Finally, it is reported the mitochondrial pentatricopeptide repeat-containing protein At1g63130, a trans-acting siRNA (ta-siRNA) generating locus (Yoshikawa et al., 2005).
Some annotated proteins may be involved on reproduction, such as D-3-phosphoglycerate dehydrogenase 1, which apart from participating in the plastidial phosphorylated pathway of serine biosynthesis (PPSB) (Benstein et al., 2013), might act on pollen development, megagametogenesis or seed dormancy (Toujani et al., 2013). Also, a DC1 domain-containing protein, associated to the generation of lipid signaling molecules in pistil (Qin et al., 2009), a dihydrolipoyl dehydrogenase 1, highly expressed in developing seeds, and the translation elongation factor emb2726, member of the broad mutant family found in Arabidopsis, EMBRYO DEFECTIVE (Meinke, 2020).
Our results give support to a role of the metabolism of arginin, precursor of polyamines, on gametophyte reproduction, due to the increase of the protein argininosuccinate synthase and carbamoyl-phosphate synthase. A possible role of polyamines on gametophyte development is a recurring data in our previous analyses in these fern species (Grossmann et al., 2017; Wyder et al., 2020). Finally, the metabolism of cell wall pectins acquires some importance with the protein UGD2, which is involved in the biosynthesis of UDP-glucuronic acid (UDP-GlcA), providing nucleotide sugars for cell-wall polymers. A possible connection to the apogamy will need further research.
Sexual Gametophytes Upregulate Proteins Related to Starch and Sucrose Metabolism, Generation of Precursor Metabolites and Energy, and Photosynthesis
Starch and sucrose metabolism appear to be relevant processes in the sexual gametophytes. Specifically, among other proteins upregulated in the studied female gametophytes, we found alpha and beta amylase, pfkB-like carbohydrate kinase family protein, phosphoglucan, water dikinase (PWD), cytosolic fructose 1-6-bisphosphatase, a probable sucrose-phosphate synthase 3, glucose-1-phosphate adenylyltransferase, chloroplastic glucose-6-phosphate isomerase 1, etc. In Ceratopteris thalictroides, proteins linked to photosynthesis were over-represented in hermaphrodite gametophytes compared to male ones induced by antheridiogens, which had smaller size (Chen et al., 2019).
Regarding the generation of precursor metabolites and energy annotations, several proteins of both aerobic or anaerobic paths, such as the citrate cycle (TCA cycle), oxidative phosporilation or glucolysis/gluconeogenesis, significantly increased in sexual gametophytes. Moreover, in these gametophytes the number of proteins upregulated in photosynthesis and antenna proteins was double that of the apogamous one, and they act on chromophore-linkage and assembly and repair of the photosystetic apparatus (PSBB, PSAB, LHCB5, LHCB3, LHCA3, VAR2, PSB27, TLP18.3, PORA, PORC). PORA, for instance, may function as a photoprotectant during the transitory stage from dark to light, and also in photomorphogenesis and throughout the plant life under specific light conditions (Paddock et al., 2012). There are also proteins associated with pigment biosynthetic processes, such as the magnesium-chelatases ALB1 and GUN5, which can have other functions as GUN5 acting in ABA signaling (Du S. Y. et al., 2012).
In our experiments, both sexual and apogamous gametophytes were cultured under similar conditions of light, photoperiod, humidity, nutrients, or pH. Although it was not scored, the growth rate of gametophytes was clearly slower in D. oreades than in D. affinis. However, final size of the sexual gametophyte was bigger than that of the apogamous one. In the gametophytes, although scarce, there are some data supporting differences in photochemical efficiency linked to sex gender, increasing in female individuals, which thus could provide more energy for the development of archegonia, fertilization and the further growth of sporophytes (Valledor et al., 2014; Slate et al., 2017; Chen et al., 2019). Our data suggest that the sexual gametophytes are more energy-demanding than the apogamous counterparts. We propose that the gametophyte of D. affinis devotes all resources to quickly grow and differentiate an embryo, while the female gametophytes have to attain a more complex organization level, building a decisive central area, the archegonium cushion, a several cell layers thick which is the place where the archegonia and future embryo will develop. At first glance, the larger and more complex sexual gametophyte could thus demand more energy than the smaller and fast-growing apogamous gametophytes.
The lipid metabolism seems especially important in the sexual gametophytes, upregulating proteins involved in linoleic acid metabolism such as the lipoxygenases LOX1 and LOX 3, as well as the peroxisomal fatty acid beta-oxidation multifunctional protein MFP2. These proteins are linked to functions such as growth and development, pest resistance, senescence or responses to wounding (Vellosillo et al., 2007), and in the tree fern C. delgadii, key enzymes in fatty acid biosynthesis were told to have an important role during embryogenesis (Domzalska et al., 2017).
Other proteins found in the sexual gametophyte are related to different aspects of reproduction, such as embryo defective 1579, involved in embryo development ending in seed dormancy, several LEA proteins such as LEA4-1 or ECP63, which may be linked to the BHLH109-mediated regulation of somatic embryogenesis (Nowak and Gaj, 2016), and Villin-2, a Ca(2+)-a regulated actin-binding protein, required for the construction of actin collars in pollen tubes (van der Honing et al., 2012; Qu et al., 2013). In addition, NAP1-related protein 2 acts as histone H2A/H2B chaperone in nucleosome assembly, being essential, together NRP1, for the maintenance of cell proliferation and differentiation in postembryonic root growth, and for intramolecular and intermolecular somatic homologous recombination (Zhu et al., 2006). Furthermore, we annotated two interesting proteins: an ADP-ribosylation factor-like protein, member of ARF GTPase family partaking in cell division, expansion, and cellulose production; and the monocopper oxidase-like protein SKU5, a GPI-anchor protein involved in directed root tip growth (Sedbrook et al., 2002).
Other interesting protein is a flavone 3'-Omethyltransferase, involved in melatonin biosynthesis (Byeon et al., 2014), and the protein glutamate decarboxylase 1, which catalysis the production of GABA (Bouché et al., 2004). Finally, it deserves to mention the protein FIB2, a S-adenosyl-L-methionine-dependent methyltransferase, which has been speculated to be involved on methylation of RNAs and proteins, including histones, having an important role on defense against bacterial pathogens (Seo et al., 2019).
Phytohormones: Hormonal Content and Protein Regulation
Information acquired so far about the physiology and molecular events operating inside this free-leaving fern generation is scarce. As far as we know, the present study represents the first assessment of a large number of phytohormones in fern gametophytes, and shows changes in phytohormone profiles related to reproductive systems in non-model species.
The presence of SA at higher levels in the filamentous apogamous gametophytes could be related to a stressful environment as could be in vitro culture itself, especially at the beginning of gametophyte development (Kosakivska et al., 2019; Wyder et al., 2020). SA influences a wide range of processes, including seedling establishment and responses to abiotic and biotic stresses (Vlot et al., 2009), being one of the first features in plant-microbe interactions that is present in basal-branching algae (Pieterse et al., 2012; Hori et al., 2014). Although this phytohormone showed higher levels at the filamentous stage, the proteomic data point out an important presence of its metabolic pathways along the whole gametophyte development, as shown later.
Although very simple morphologically, gametophytes go through successive developmental changes until sexual maturity. An early crucial event in the formation of the two-dimensional gametophyte involves the re-orientation of cell plate, from a transverse to a longitudinal alignment (Nayar and Kaur, 1971; Banks, 1999; Racusen, 2002). Our results suggest a possible connection between the cytokinin BA and the filamentous morphology, and between GA3 and the spatulate shape. Cytokinins might affect the rate and pattern of cell division, cell elongation and cell differentiation in ferns (Romanov, 2009). Although gametophytes of B. spicant and Osmunda regalis became shorter, widened and lacking meristem in response to cytokinins (Menéndez et al., 2006a; Greer et al., 2012), these phytohormones induced transition from one- to two-dimensional growth in C. richardii (Spiro et al., 2004). The increase of GA3 in our spatulate gametophytes is consistent with previous data with other sexual and apogamous fern species such as B. spicant, Anemia phillitidis, and Polystichum aculeatum (Kazmierczak, 2003; Menéndez et al., 2006b,c, 2009; Kosakivska et al., 2020).
The levels of IAA, Z, ZR, iPR, the gibberellin GA4, the brassinosteoid castasterone and ABA were higher in sexual cordate gametophytes than in the apogamous cordate ones. The primary role of zeatin riboside in reproductive processes has been stated in ferns (Abul et al., 2010; Vedenecheva and Sytnik, 2013; Vedenicheva and Kosakivska, 2016, 2018). In the last case, zeatin and seatin riboside levels increased in the sporophyte of the fern D. filix-mas when sporulation happens, while zeatin and zeatin riboside content became higher during intensive vegetative growth in P. acculeatum. Recently, an increase of IAA, ZR, iP, iPR, GA3 and ABA in the cordate gametophyte of P. aculeatum has been documented by the same authors (Kosakivska et al., 2020), pointing out a key role of these phytohormones in the regulation of growth and development of the cushion. The iP bases cytokinins are characteristic of mosses and ferns, being more difficult to be detected zeatin derivatives (Johri, 2008). Additionally, in cordate gametophytes of D. filix-mas an increase in the IAA and Z, at the formation of both sexual organs, archegonia and antheridia, has been reported (Kosakivska et al., 2019). These authors concluded also that GA3 was dominant at all stages of gametophyte development, reaching the highest content during the development of the sexual organs. The gibberellin GA4 prevails over GA3 either in apogamic or sexual development, being especially high in the latter. Previously, high levels of GA4 and GA7 were assessed in D. affinis, as an apogamous embryo evolved (Menéndez et al., 2006c), and in gametophytes governed by an antheridiogen system, GA4 has been associated with the induction of antheridia, and also with contributing to the genetic exchange (Tanaka et al., 2014). The accumulation of GA4 in the cordate sexual gametophytes of D. oreades suggests a wider role on gametophyte development not yet dilucidated by the gibberellins.
The sexual gametophytes also had a noticeable increase in ABA. It has recently been reported that the origin of the core ABA signaling pathway in seed plants might lie in the sexual differentiation of ferns (McAdam et al., 2016). In female gametophytes of D. oreades, a possible connection of ABA with their antheridiogen-release capacity could be to protect themselves against the activity of these pheromone, preserving the female condition (Hickok, 1983; Banks et al., 1993). Regarding the active brassinosteroids analyzed, both BL and CS were detected. Many processes have been linked with brassinosteroids function such as cell elongation, cell division, reproductive and vascular development, stress responses, or senescence, but information about their role on ferns is scarce (Gómez-Garay et al., 2018). Interestingly, the amount of CS was high, pointing at some possible role of brassinosteroids on sexual development of gametophytes in D. oreades. Moreover, CS was identified in most of the ferns studied by Yokota et al. (2017) but not BL.
As noted above, our proteomic analysis revealed a myriad of proteins related to the biosynthesis and/or function of the analyzed phytohormones, giving strong support to the important role of these regulators on gametophyte development. In the apogamous gametophyte, the histidine phosphotransfer protein AHP1, linked to cytokinin signaling was annotated (Hwang et al., 2002), and the protein, emb22 (also named Gurke or Pasticcino3), which is an acetyl-CoA carboxylase 1, related to cell proliferation and tissue patterning (Baud et al., 2003), embryo morphogenesis (Torres-Ruiz et al., 1996); or supressing cytokinin activity (Faure et al., 1998). Also the protein Cand1, required for SCFTIR activity, participating in several resposes such as response to auxin (Cheng et al., 2004). In addition, there are proteins on defense or stress, such as prohibitin-3, required to regulate ethylene-mediated signaling, and nitric oxide (NO)-mediated responses (Van Aken et al., 2007; Wang et al., 2010).
On the other hand, sexual gametophytes overexpressed the plasma membrane-type ATPase 1, which has been suggested to be involved in brassinosteroid signaling (Ladwig et al., 2015); tryptophan synthase beta type 2 (TSBtype2), acting on the auxin biosynthesis (Zhao, 2014); proteins linked to the ABA response, such as those of NAD(P)-binding Rossmann-fold superfamily proteins (Ghelis et al., 2008); to polyamines or proline as arginase/deacetylase (Patel et al., 2017), whose over-expression decreases susceptibility to the fungal pathogen Botrytis cinerea (Brauc et al., 2012). We also found Nudix hydrolase homolog 8, which may act on SA signaling (Fonseca and Dong, 2014).
Another interesting protein is a transcriptional activator belonging to RNA-binding (RRM/RBD/RNP motifs) family protein, antagonist and promoter of polycomb LHP1 gene regulation activity, to regulate the transcription of stress-responsive and flowering genes (Latrasse et al., 2011). Besides, it may function as a suppressor of cell-autonomous immune responses involving glucosinolates, SA, and JA pathways toward pathogenic bacteria and fungi (Le Roux et al., 2014). Finally, glutathione S-transferase TAU 20 is another protein upregulated in the sexual gametophyte, involved in the regulation of far-red light influence on development as a regulator of the interplay between light and JA signaling (Chen et al., 2007, 2017) and playing a role in gravitropic signal transduction (Schenck et al., 2013).
Conclusions
Qualitative and quantitative differences in protein and phytohormone profiles between apogamous and sexual gametophytes are reported. Our results indicate that phytohormone contents vary either between cordate apogamous gametophytes and their sexual counterpart. Our main conclusions were: (1) Seven out of fourteen phytohormones accumulated more in the sexual (female) gametophyte, especially auxin IAA, the cytokinins Z, ZR, iPR, the active gibberellin GA4, and the active brassinosteoids CS. (2) The proteins upregulated in the apogamous species are associated with the primary metabolism of aminoacids, peptides and proteins (including folding, transport and proteolysis), nucleic acids and cofactors, nitrogen metabolism, ribosome biogenesis, translation and gene expression and stress response. (3) The sexual counterpart accumulated more proteins coping with starch and sucrose metabolism, generation of energy and photosynthesis, lipid oxidation and response to hormones. Asexual vs. sexual gametophyte involves two different metabolic scenarios, with apogamous reproduction more connected to stress responses while sexual reproduction is more resource demanding.
Data Availability Statement
The datasets presented in this study can be found in online repositories. The names of the repository/repositories and accession number(s) can be found in the article/Supplementary Material.
Author Contributions
HF and UG: conceive the project. HF, AR, and MC: in vitro culture of ferns and sampling. LQ: supplied plant material. AR and VQ: statistical analyses. JG: performed bioinformatics. VG: protein extraction and purification. IF and LR: phytohormones analyses. All authors discussed the results and contributed to the final manuscript.
Funding
This work was supported by the University of Zurich and Project PRIME-XS-0002520 funded by the European Union's 7th Framework Program.
Conflict of Interest
The authors declare that the research was conducted in the absence of any commercial or financial relationships that could be construed as a potential conflict of interest.
Publisher's Note
All claims expressed in this article are solely those of the authors and do not necessarily represent those of their affiliated organizations, or those of the publisher, the editors and the reviewers. Any product that may be evaluated in this article, or claim that may be made by its manufacturer, is not guaranteed or endorsed by the publisher.
Acknowledgments
We thank the University of Oviedo for a grant from International Mobility of Research Staff, accordingly to the collaboration agreement CESSTT1819 and the Functional Genomics Center Zurich for access to its infrastructure.
Supplementary Material
The Supplementary Material for this article can be found online at: https://www.frontiersin.org/articles/10.3389/fpls.2021.718932/full#supplementary-material
Abbreviations
ABA, abscisic acid; BA, 6-benzylaminopurine; BL, brassinolide; CS, castasterone; DA, Dryopteris affinis; DO, Dryopteris oreades; DHZ, dihydrozeatin; DHZR, dihydrozeatin riboside; GA3, gibberellic acid; GA4, gibberellin GA4; IAA, indole-3-acetic acid; iP, isopentenyl adenine; iPR, isopentenyl adenosine; JA, jasmonic acid; MS/MS, mass spectrometry; MS, Murashige and Skood mineral medium (1962); SA, salicylic acid; ZR, trans-zeatin riboside; Z, trans-zeatin.
References
Abul, Y., Menéndez, V., Gómez-Campo, C., Revilla, M. A., Lafont, F., et al. (2010). Occurence of plant growth regulators in Psilotum nudum. J. Plant Physiol. 167, 1211–1213. doi: 10.1016/j.jplph.2010.03.015
Amaki, W., and Higuchi, H. (1992). A possible propagation system of Nephrolepis, Asplenium, Pteris, Adiantum and Rumohra (Arachniodes) through tissue culture. Acta Hortic. 300, 237–244. doi: 10.17660/ActaHortic.1992.300.33
Atallah, N. M., Vitek, O., Gaiti, F., Tanurdzic, M., and Banks, J. A. (2018). Sex determination in Ceratopteris richardii is accompanied by transcriptome changes that drive epigenetic reprogramming of the young gametophyte. Genes Genomes Genetics 8, 2205–2214. doi: 10.1534/g3.118.200292
Aya, K., Kobayashi, M., Tanaka, J., Ohyanagi, H., Suzuki, T., et al. (2015). De novo transcriptome assembly of a fern, Lygodium japonicum, and a web resource database, Ljtrans DB. Plant Cell Physiol. 56:e5. doi: 10.1093/pcp/pcu184
Baerenfaller, K., Grossmann, J., Grobei, M. A., Hull, R., Hirsch-Hoffmann, M., et al. (2008). Genome-scale proteomics reveals Arabidopsis thaliana gene models and proteome dynamics. Science 320, 938–941. doi: 10.1126/science.1157956
Bai, Y., Du, F., and Liu, H. (2010). Determination strategies of phytohormones: recent advances. Anal. Methods 2, 1867–1873. doi: 10.1039/c0ay00471e
Banks, J. A. (1999). Gametophyte development in ferns. Annu. Rev. Plant Physiol. Plant Mol. Biol. 50, 163–183. doi: 10.1146/annurev.arplant.50.1.163
Banks, J. A., Hickok, L. G., and Webb, M. A. (1993). The programming of sexual phenotype in the homosporous fern Ceratopteris richardii. Int. J. Plant Sci. 154, 522–534. doi: 10.1086/297135
Barcaccia, G., and Albertini, E. (2013). Apomixis in plant reproduction: a novel perspective on an old dilemma. Plant Reprod. 26, 159–179. doi: 10.1007/s00497-013-0222-y
Barker, M. S., and Wolf, P. G. (2010). Unfurling fern biology in the genomics age. Bioscience 60, 177–185. doi: 10.1525/bio.2010.60.3.4
Baud, S., Guyon, V., Kronenberger, J., Wuillème, S., Miquel, M., et al. (2003). Multifunctional acetyl-CoA carboxylase 1 is essential for very long chain fatty acid elongation and embryo development in Arabidopsis. Plant J. Cell Mol. Biol. 33, 75–86. doi: 10.1046/j.1365-313X.2003.016010.x
Benstein, R. M., Ludewig, K., Wulfert, S., Wittek, S., Gigolashvili, T., et al. (2013). Arabidopsis phosphoglycerate dehydrogenase1 of the phosphoserine pathway is essential for development and required for ammonium assimilation and tryptophan biosynthesis. Plant Cell 25, 5011–5029. doi: 10.1105/tpc.113.118992
Bhatnagar, N., and Pandey, S. (2020). Heterotrimeric G-protein interactions are conserved despite regulatory element loss in some plants. Plant Physiol. 22:01309. doi: 10.1104/pp.20.01309
Bouché, N., Fait, A., Zik, M., and Fromm, H. (2004). The root-specific glutamate decarboxylase (GAD1) is essential for sustaining GABA levels in Arabidopsis. Plant Mol. Biol. 55, 315–325. doi: 10.1007/s11103-004-0650-z
Brauc, S., De Vooght, E., Claeys, M., Geuns, J. M. C., Höfte, M., and Angenon, G. (2012). Overexpression of arginase in Arabidopsis thaliana influences defence responses against Botrytis cinerea. Plant Biol. 14, 39–45. doi: 10.1111/j.1438-8677.2011.00520.x
Bui, L. T., Pandzic, D., Youngstrom, C. E., Wallace, S., Irish, E. E., Szövényi, P., et al. (2017). A fern AINTEGUMENTA gene mirrors BABY BOOM in promoting apogamy in Ceratopteris richardii. Plant J. 90, 122–132. doi: 10.1111/tpj.13479
Byeon, Y., Lee, H. Y., Lee, K., and Back, K. (2014). Caffeic acid O-methyltransferase is involved in the synthesis of melatonin by methylating N-acetylserotonin in Arabidopsis. J. Pineal Res. 57, 219–227. doi: 10.1111/jpi.12160
Chen, C. Y., Ho, S. S., Kuo, T. Y., Hsieh, H. L., and Cheng, Y. S. (2017). Structural basis of jasmonate-amido synthetase FIN219 in complex with glutathione S-transferase FIP1 during the JA signal regulation. Proc. Natl. Acad. Sci. U.S.A. 114, E1815–E1824. doi: 10.1073/pnas.1609980114
Chen, I. C., Huang, I. C., Liu, M. J., Wang, Z. G., Chung, S. S., and Hsieh, H. L. (2007). Glutathione S-transferase interacting with far-red insensitive 219 is involved in phytochrome A-mediated signaling in arabidopsis. Plant Physiol. 143, 1189–1202. doi: 10.1104/pp.106.094185
Chen, X., Chen, Z., Huang, W., Fu, H., Wang, Q., Wang, Y., et al. (2019). Proteomic analysis of gametophytic sex expression in the fern Ceratopteris thalictroides. PLoS ONE 14:e0221470. doi: 10.1371/journal.pone.0221470
Cheng, Y., Dai, X., and Zhao, Y. (2004). AtCAND1, a Heat-repeat protein that participates in auxin signaling in Arabidopsis. Plant Physiol. 135, 1020–1026. doi: 10.1104/pp.104.044495
Chong, L. P., Wang, Y., Gad, N., Anderson, N., Shah, B., and Zhao, R. (2015). A highly charged region in the middle domain of plant endoplasmic reticulum (ER)-localized heat-shock protein 90 is required for resistance to tunicamycin or high calcium-induced ER stresses. J. Exp. Bot. 66, 113–124. doi: 10.1093/jxb/eru403
Choudhary, S. P., Yu, J. Q., Yamaguchi-Shinozaki, K., Shinozaki, K., and Tran, L. S. P. (2012). Benefits of brassinosteroid crosstalk. Trends Plant Sci. 17, 594–605. doi: 10.1016/j.tplants.2012.05.012
Cordle, A., Irish, E., and Cheng, C.l.L. (2012). Gene expression associated with apogamy commitment in Ceratopteris richardii. Sex. Plant Reprod. 25, 293–304. doi: 10.1007/s00497-012-0198-z
de Vries, S., de Vries, J., Teschke, H., von Dahlen, J. K., Rose, L. E., and Gould, S. B. (2018). Jasmonic and salicylic acid response in the fern Azolla filiculoides and its cyanobiont. Plant Cell Environ. 41, 2530–2548. doi: 10.1111/pce.13131
Delatorre, C., Rodríguez, A., Rodríguez, L., Majada, J. P., Ordás, R. J., and Feito, I. (2017). Hormonal profiling: Development of a simple method to extract and quantify phytohormones in complex matrices by UHPLC–MS/MS. J. Chromatogr. B Analyt. Technol. Biomed. Life Sci. 1040, 239–249. doi: 10.1016/j.jchromb.2016.11.007
Der, J. P., Barker, M. S., Wickett, N. J., dePamphilis, C. W., and Wolf, P. G. (2011). De novo characterization of the gametophyte transcriptome in bracken fern, Pteridium aquilinum. BMC Genomics 12:99. doi: 10.1186/1471-2164-12-99
Dobrev, P., Kamínek, M., Ivanov Dobrev, P., Kamínek, M., Dobrev, P., and Kamínek, M. (2002). Fast and efficient separation of cytokinins from auxin and abscisic acid and their purification using mixed-mode solid-phase extraction. J. Chromatogr. A 950, 21–29. doi: 10.1016/S0021-9673(02)00024-9
Domzalska, L., Kedracka-Krok, S., Jankowska, U., Grzyb, M., Sobczak, M., Rybczyński, J. J., et al. (2017). Proteomic analysis of stipe explants reveals differentially expressed proteins involved in early direct somatic embryogenesis of the tree fern Cyathea delgadii Sternb. Plant Sci. 258, 61–76. doi: 10.1016/j.plantsci.2017.01.017
Du, F., Ruan, G., and Liu, H. (2012). Analytical methods for tracing plant hormones. Anal. Bioanal. Chem. 403, 55–74. doi: 10.1007/s00216-011-5623-x
Du, S. Y., Zhang, X. F., Lu, Z., Xin, Q., Wu, Z., et al. (2012). Roles of the different components of magnesium chelatase in abscisic acid signal transduction. Plant Mol. Biol. 80, 519–537. doi: 10.1007/s11103-012-9965-3
Dyer, R. J., Savolainen, V., and Schneider, H. (2012). Apomixis and reticulate evolution in the Asplenium monanthes fern complex. Ann. Bot. 110, 1515–1529. doi: 10.1093/aob/mcs202
Ekrt, L., and Koutecký, P. (2016). Between sexual and apomictic: Unexpectedly variable sporogenesis and production of viable polyhaploids in the pentaploid fern of the Dryopteris affinis agg. (Dryopteridaceae). Ann. Bot. 117, 97–106. doi: 10.1093/aob/mcv152
Falk, S., Ravaud, S., Koch, J., and Sinning, I. (2010). The C terminus of the Alb3 membrane insertase recruits cpSRP43 to the thylakoid membrane. J. Biol. Chem. 285, 5954–5962. doi: 10.1074/jbc.M109.084996
Falk, S., and Sinning, I. (2010). cpSRP43 is a novel chaperone specific for light-harvesting chlorophyll a,b-binding proteins. J. Biol. Chem. 285, 21655–21661. doi: 10.1074/jbc.C110.132746
Faure, J. D., Vittorioso, P., Santoni, V., Fraisier, V., Prinsen, E., et al. (1998). The PASTICCINO genes of Arabidopsis thaliana are involved in the control of cell division and differentiation. Development 125, 909–918. doi: 10.1242/dev.125.5.909
Fernández, H., and Revilla, M. A. (2003). In vitro culture of ornamental ferns. Plant Cell Tissue Organ Cult. 73, 1–13. doi: 10.1023/A:1022650701341
Fleurdépine, S., Deragon, J. M., Devic, M., Guilleminot, J., and Bousquet-Antonelli, C. (2007). A bona fide La protein is required for embryogenesis in Arabidopsis thaliana. Nucleic Acids Res. 35, 3306–3321. doi: 10.1093/nar/gkm200
Fonseca, J. P., and Dong, X. (2014). Functional characterization of a Nudix Hydrolase AtNUDX8 upon pathogen attack indicates a positive role in plant immune responses. PLoS ONE 9:e114119. doi: 10.1371/journal.pone.0114119
Fraser-Jenkins, C. R. (1980). Dryopteris Affinis: A New Treatment for a Complex Species in the European Pteridophyte Flora (Vol. 10). Berlin: Botanischer Garten und Botanisches Museum.
Fu, Q., and Chen, L. Q. (2019). Comparative transcriptome analysis of two reproductive modes in Adiantum reniforme var. sinense targeted to explore possible mechanism of apogamy. BMC Genet. 20:55. doi: 10.1186/s12863-019-0762-8
Ghelis, T., Bolbach, G., Clodic, G., Habricot, Y., Miginiac, E., Sotta, B., et al. (2008). Protein tyrosine kinases and protein tyrosine phosphatases are involved in abscisic acid-dependent processes in Arabidopsis seeds and suspension cells. Plant Physiol. 148, 1668–1680. doi: 10.1104/pp.108.124594
Gómez-Garay, A., Gabriel y Galán, J. M., Cabezuelo, A., Pintos, B., Prada, C., and Martín, L. (2018). “Ecological significance of brassinosteroids in three temperate ferns,” in Current Advances in Fern Research, ed H. Fernández (New York, NY: Springer International Publishing), 1–544.
Greer, G. K., Dietrich, M. A., Devol, J. A., Rebert, A., American, S., et al. (2012). The effects of exogenous cytokinin on the morphology and gender expression of Osmunda regalis gametophytes. Amer. Fern J. 102, 32–46. doi: 10.1640/0002-8444-102.1.32
Grimanelli, D., García, M., Kaszas, E., Perotti, E., and Leblanc, O. (2003). Heterochronic expression of sexual reproductive programs during apomictic development in Tripsacum. Genetics 165, 1521–1531. doi: 10.1093/genetics/165.3.1521
Grossmann, J., Fernández, H., Chaubey, P. M., Valdés, A. E., Gagliardini, V., et al. (2017). Proteogenomic analysis greatly expands the identification of proteins related to reproduction in the apogamous fern Dryopteris affinis ssp. affinis. Front. Plant Sci. 8:336. doi: 10.3389/fpls.2017.00336
Grossniklaus, U., Koltunow, A. M., and van Lookeren Campagne, M. (1998). A bright future for apomixis. Trends Plant Sci. 3, 415–416. doi: 10.1016/S1360-1385(98)01338-7
Grossniklaus, U., Nogler, G. A., and van Dijk, P. J., (2001). How to avoid sex: the genetic control of developmental aspects. Plant Cell (July) 13, 1491–1497. doi: 10.2307/3871381
Hammer, Ø. (2001). PAST: Paleontological statistics software package for education and data analysis. Palaeontol. Electron. 4:9. Available online at: http://palaeo-electronica.org/2001_1/past/issue1_01.htm
Hickok, L. G. (1983). Abscisic acid blocks antheridiogen-induced antheridium formation in gametophytes of the fern Ceratopteris. Can. J. Bot. 61, 888–892. doi: 10.1139/b83-098
Hicks, G. H. (1894). Nourishment of the Embryo and importance of the endosperm in viviparous mangrove plants. Bot. Gazette 19, 327–330. doi: 10.1086/327078
Hofmann, N. R. (2010). Apomixis and gene expression in Boechera. Plant Cell 22:539. doi: 10.1105/tpc.110.220312
Hori, K., Maruyama, F., Fujisawa, T., Togashi, T., Yamamoto, N., et al. (2014). Klebsormidium flaccidum genome reveals primary factors for plant terrestrial adaptation. Nat. Commun. 5, 2–6. doi: 10.1038/ncomms4978
Horváth, B. M., Magyar, Z., Zhang, Y., Hamburger, A. W., Bakó, L., et al. (2006). EBP1 regulates organ size through cell growth and proliferation in plants. EMBO J. 25, 4909–4920. doi: 10.1038/sj.emboj.7601362
Hwang, I., Chen, H. C., and Sheen, J. (2002). Two-component signal transduction pathways in Arabidopsis. Plant Physiol. 129, 500–515. doi: 10.1104/pp.005504
Ishiguro, S., Watanabe, Y., Ito, N., Nonaka, H., Takeda, N., et al. (2002). SHEPHERD is the Arabidopsis GRP94 responsible for the formation of functional CLAVATA proteins. EMBO J. 21, 898–908. doi: 10.1093/emboj/21.5.898
Johri, M. (2008). Hormonal regulation in green plant lineage families. Physiol. Mol. Biol. Plants Int. J. Funct. Plant Biol. 14, 23–38. doi: 10.1007/s12298-008-0003-5
Kazmierczak, A. (2003). Induction of cell division and cell expansion at the beginning of gibberellin A3-induced precocious antheridia formation in Anemia phyllitidis gametophytes. Plant Sci. 165, 933–939. doi: 10.1016/S0168-9452(03)00217-6
Kazmierczak, A. (2010). “Gibberellic acid and ethylene control male sex determination and development of anemia phyllititdis gametophytes,” in Working With Ferns. Issues and Applications, eds H. Fernández, A. Kumar, and A Revilla (New York, NY: Springer), 49–65.
Koltunow, A. M., and Grossniklaus, U. (2003). Apomixis: a developmental perspective. Annu. Rev. Plant Biol. 54, 547–574. doi: 10.1146/annurev.arplant.54.110901.160842
Kosakivska, I. V., Romanenko, K., Voytenko, L., Vasyuk, V., Schcherbatiuk, M., and Babenko, L. (2019). Hormonal complex of gametophytes of Dryopteris filix-mass (Dryopteridaceae) in vitro culture. Plant Physiol. Biochem. Cell Mol. Biol. 76, 260–269. doi: 10.15407/ukrbotj76.03.260
Kosakivska, I. V., Vasyuk, V. A., Voytenko, L. V., Shcherbatiuk, M. M., Romanenko, K. O., and Babenko, L. M. (2020). Endogenous phytohormones of fern Polystichum aculeatum (L.) Roth gametophytes at different stages of morphogenesis in vitro culture. Cytol. Genet. 54, 23–30. doi: 10.3103/S0095452720010089
Kotake, T., Takada, S., Nakahigashi, K., Ohto, M., and Goto, K. (2003). Arabidopsis terminal Flower 2 gene encodes a heterochromatin protein 1 homolog and represses both FLOWERING LOCUS T to regulate flowering time and several floral homeotic genes. Plant Cell Physiol. 44, 555–564. doi: 10.1093/pcp/pcg091
Ladwig, F., Dahlke, R. I., Stührwohldt, N., Hartmann, J., Harter, K., and Sauter, M. (2015). Phytosulfokine regulates growth in arabidopsis through a response module at the plasma membrane that includes cyclic nucleotide-gated channel17, H+-ATPase, and BAK1. Plant Cell 27, 1718–1729. doi: 10.1105/tpc.15.00306
Latrasse, D., Germann, S., Houba-Hérin, N., Dubois, E., Bui-Prodhomme, D., et al. (2011). Control of flowering and cell fate by LIF2, an RNA binding partner of the polycomb complex component LHP1. PLoS ONE 6:e16592. doi: 10.1371/journal.pone.0016592
Le Roux, C., Del Prete, S., Boutet-Mercey, S., Perreau, F., Balagué, C., et al. (2014). The hnRNP-Q Protein LIF2 participates in the plant immune response. PLoS ONE 9:e99343. doi: 10.1371/journal.pone.0099343
Lee, S., Lee, D. W., Lee, Y., Mayer, U., Stierhof, Y. D., et al. (2009). Heat shock protein cognate 70-4 and an E3 ubiquitin ligase, CHIP, mediate plastid-destined precursor degradation through the ubiquitin-26S proteasome system in Arabidopsis. Plant Cell 21, 3984–4001. doi: 10.1105/tpc.109.071548
Liu, H.-M., Dyer, R. J. R. J., Guo, Z.-Y., Meng, Z., Li, J.-H., and Schneider, H. (2012). The evolutionary dynamics of apomixis in ferns: a case study from Polystichoid ferns. J. Bot. 2012, 1–11. doi: 10.1155/2012/510478
Lovis, J. D. (1978). Evolutionary patterns and processes in ferns. Adv. Bot. Res. 4, 229–415. doi: 10.1016/S0065-2296(08)60371-7
McAdam, S. A. M., Brodribb, T. J., Banks, J. A., Hedrich, R., Atallah, N. M., et al. (2016). Abscisic acid controlled sex before transpiration in vascular plants. Proc. Natl. Acad. Sci. U.S.A. 113, 12862–12867. doi: 10.1073/pnas.1606614113
Meinke, D. W. (2020). Genome-wide identification of EMBRYO-DEFECTIVE (EMB) genes required for growth and development in Arabidopsis. New Phytol. 226, 306–325. doi: 10.1111/nph.16071
Menéndez, V., Abul, Y., Bohanec, B., Lafont, F., and Fernández, H. (2011). The effect of exogenous and endogenous phytohormones on the in vitro development of gametophyte and sporophyte in Asplenium nidus L. Acta Physiol. Plant. 33, 2493–2500. doi: 10.1007/s11738-011-0794-9
Menéndez, V., Revilla, M. A., Bernard, P., Gotor, V., and Fernández, H. (2006a). Gibberellins and antheridiogen on sex in Blechnum spicant L. Plant Cell Rep. 25:1104. doi: 10.1007/s00299-006-0149-y
Menéndez, V., Revilla, M. A., Fal, M. A., and Fernández, H. (2009). The effect of cytokinins on growth and sexual organ development in the gametophyte of Blechnum spicant L. Plant Cell Tissue Organ Cult. 96, 245–250. doi: 10.1007/s11240-008-9481-y
Menéndez, V., Revilla, M. A., and Fernández, H. (2006b). Growth and gender in the gametophyte of Blechnum spicant L. Plant Cell Tissue Organ Cult. 86, 47–53. doi: 10.1007/s11240-006-9095-1
Menéndez, V., Villacorta, N. F., Revilla, M. A., Gotor, V., Bernard, P., and Fernández, H. (2006c). Exogenous and endogenous growth regulators on apogamy in Dryopteris affinis (Lowe) Fraser-Jenkins sp. affinis. Plant Cell Rep. 25, 85–91. doi: 10.1007/s00299-005-0041-1
Moin, M., Bakshi, A., Saha, A., Dutta, M., Madhav, S. M., and Kirti, P. B. (2016). Rice ribosomal protein large subunit genes and their spatio-temporal and tress regulation. Front. Plant Sci. 7:1284. doi: 10.3389/fpls.2016.01284
Murashige, T., and Skoog, F. (1962). A revised medium for rapid growth and bioassays with tobacco tissue cultures. Plant Physiol. 15, 473–497. doi: 10.1111/j.1399-3054.1962.tb08052.x
Nayar, B. K., and Kaur, S. (1971). Gametophytes of homosporous ferns. Bot. Rev. 37, 295–396. doi: 10.1007/BF02859157
Nowak, K., and Gaj, M. D. (2016). Stress-related function of bHLH109 in somatic embryo induction in Arabidopsis. J. Plant Physiol. 193, 119–126. doi: 10.1016/j.jplph.2016.02.012
Paddock, T., Lima, D., Mason, M. E., Apel, K., and Armstrong, G. A. (2012). Arabidopsis light-dependent protochlorophyllide oxidoreductase A (PORA) is essential for normal plant growth and development. Plant Mol. Biol. 78, 447–460. doi: 10.1007/s11103-012-9873-6
Pan, X., Welti, R., and Wang, X. (2008). Simultaneous quantification of major phytohormones and related compounds in crude plant extracts by liquid chromatography-electrospray tandem mass spectrometry. Phytochemistry 69, 1773–1781. doi: 10.1016/j.phytochem.2008.02.008
Pan, X., Welti, R., and Wang, X. (2010). Quantitative analysis of major plant hormones in crude plant extracts by high-performance liquid chromatography-mass spectrometry. Nat. Protoc. 5, 986–992. doi: 10.1038/nprot.2010.37
Patel, J., Ariyaratne, M., Ahmed, S., Ge, L., Phuntumart, V., Kalinoski, A., et al. (2017). Dual functioning of plant arginases provides a third route for putrescine synthesis. Plant Sci. 262, 62–73. doi: 10.1016/j.plantsci.2017.05.011
Pieterse, C. M. J., Van der Does, D., Zamioudis, C., Leon-Reyes, A., and Van Wees, S. C. M. (2012). Hormonal modulation of plant immunity. Annu. Rev. Cell Dev. Biol. 28, 489–521. doi: 10.1146/annurev-cellbio-092910-154055
Poethig, R. S., Coe, E. H., and Johri, M. M. (1986). Cell lineage patterns in maize embryogenesis: a clonal analysis. Dev. Biol. 117, 392–404. doi: 10.1016/0012-1606(86)90308-8
Porfírio, S., Gomes da Silva, M. D. R., Peixe, A., Cabrita, M. J., and Azadi, P. (2016). Current analytical methods for plant auxin quantification–a review. Anal. Chim. Acta 902, 8–21. doi: 10.1016/j.aca.2015.10.035
Preuss, S. B., Costa-Nunes, P., Tucker, S., Pontes, O., Lawrence, R. J., et al. (2008). Multimegabase silencing in nucleolar dominance involves siRNA-directed DNA methylation and specific methylcytosine-binding proteins. Mol. Cell 32, 673–684. doi: 10.1016/j.molcel.2008.11.009
Qin, Y., Leydon, A. R., Manziello, A., Pandey, R., Mount, D., et al. (2009). Penetration of the stigma and style elicits a novel transcriptome in pollen tubes, pointing to genes critical for growth in a pistil. PLoS Genet. 5:e1000621. doi: 10.1371/journal.pgen.1000621
Qu, X., Zhang, H., Xie, Y., Wang, J., Chen, N., and Huang, S. (2013). Arabidopsis villins promote actin turnover at pollen tube tips and facilitate the construction of actin collars. Plant Cell 25, 1803–1817. doi: 10.1105/tpc.113.110940
Racusen, R. H. (2002). Early development in fern gametophytes: Interpreting the transition to prothallial architecture in terms of coordinated photosynthate production and osmotic ion uptake. Ann. Bot. 89, 227–240. doi: 10.1093/aob/mcf032
Rademacher, W. (2000). Growth retardants: effects on gibberellin biosynthesis and other metabolic pathways. Annu. Rev. Plant Physiol. Plant Mol. Biol. 51, 501–531. doi: 10.1146/annurev.arplant.51.1.501
Romanov, G. A. (2009). How do cytokinins affect the cell? Russian J. Plant Physiol. 56, 268–290. doi: 10.1134/S1021443709020174
Ross, J. J., and Reid, J. B. (2010). Evolution of growth-promoting plant hormones. Funct. Plant Biol. 37, 795–805. doi: 10.1071/FP10063
Rybczynski, J. J., Tomiczak, K., Grzyb, M., and Mikula, A. (2018). “Morphogenic events in ferns: single and multicellular explants in vitro,” in Current Advances in Fern Research, ed H. Fernández (New York, NY: Springer International Publishing), 99–120.
Salmi, M. L., and Bushart, T. J., R. S. (2010). “Cellular, molecular, and genetic changes during the development of ceratopteris richardii gametophytes,” in Working With Ferns. Issues and Applications, eds H. Fernández, A. Kumar, and A. Revilla (New York, NY: Springer), 11–24.
Sánchez-Morán, E., Jones, G. H., Franklin, F. C. H., and Santos, J. L. (2004). A puromycin-sensitive aminopeptidase is essential for meiosis in Arabidopsis thaliana. Plant Cell 16, 2895–2909. doi: 10.1105/tpc.104.024992
Schenck, C. A., Nadella, V., Clay, S. L., Lindner, J., Abrams, Z., and Wyatt, S. E. (2013). A proteomics approach identifies novel proteins involved in gravitropic signal transduction. Am. J. Bot. 100, 194–202. doi: 10.3732/ajb.1200339
Schmidt, A. (2020). Controlling apomixis: shared features and distinct characteristics of gene regulation. Genes 11:329. doi: 10.3390/genes11030329
Schmidt, A., Wuest, S. E., Vijverberg, K., Baroux, C., Kleen, D., and Grossniklaus, U. (2011). Transcriptome analysis of the arabidopsis megaspore mother cell uncovers the importance of RNA helicases for plant germline development. PLoS Biol. 9:1001155. doi: 10.1371/journal.pbio.1001155
Sedbrook, J. C., Carroll, K. L., Hung, K. F., Masson, P. H., and Somerville, C. R. (2002). The Arabidopsis SKU5 gene encodes an extracellular glycosyl phosphatidylinositol-anchored glycoprotein involved in directional root growth. Plant Cell 14, 1635–1648. doi: 10.1105/tpc.002360
Seo, J. S., Diloknawarit, P., Park, B. S., and Chua, N. H. (2019). Elf18-induced long noncoding RNA 1 evicts fibrillarin from mediator subunit to enhance PATHOGENESIS-RELATED GENE 1 (PR1) expression. New Phytol. 221, 2067–2079. doi: 10.1111/nph.15530
Sheffield, E., Laird, S., and Bell, P. (1983). Ultrastructural aspects of sporogenesis in the apogamous fern Dryopteris borreri. J. Cell Sci. 63, 125–134. doi: 10.1242/jcs.63.1.125
Silveira, É. D., Guimarães, L. A., de Dusi, D. M. A., da Silva, F. R., Martins, N. F., et al. (2012). Expressed sequence-tag analysis of ovaries of Brachiaria brizantha reveals genes associated with the early steps of embryo sac differentiation of apomictic plants. Plant Cell Rep. 31, 403–416. doi: 10.1007/s00299-011-1175-y
Singh, A. P., and Johari, D. (2018). “Scope of ferns in horticulture and economic development,” in Current Advances in Fern Research, ed H. Fernández (New York, NY: Springer International Publishing), 153–175.
Slate, M. L., Rosenstiel, T. N., and Eppley, S. M. (2017). Sex-specific morphological and physiological differences in the moss Ceratodon purpureus (Dicranales). Ann. Bot. 120, 845–854. doi: 10.1093/aob/mcx071
Somer, M., Arbes,ú, R., Menéndez, V., Revilla, M. A., and Fernández, H. (2010). Sporophyte induction studies in ferns in vitro. Euphytica 171, 203–210. doi: 10.1007/s10681-009-0018-1
Spiro, M. D., Torabi, B., and Cornell, C. N. (2004). Cytokinins induce photomorphogenic development in dark-grown gametophytes of Ceratopteris richardii. Plant Cell Physiol. 45, 1252–1260. doi: 10.1093/pcp/pch146
Sun, Y., Fan, X. Y., Cao, D. M., Tang, W., He, K., Zhu, J. Y., et al. (2010). Integration of brassinosteroid signal transduction with the transcription network for plant growth regulation in Arabidopsis. Dev. Cell 19, 765–777. doi: 10.1016/j.devcel.2010.10.010
Tanaka, J., Yano, K., Aya, K., Hirano, K., Takehara, S., et al. (2014). Antheridiogen determines sex in ferns via a spatiotemporally split gibberellin synthesis pathway. Science 346, 469–473. doi: 10.1126/science.1259923
Torres-Ruiz, R. A., Lohner, A., and Jurgens, G. (1996). The GURKE gene is required for normal organization of the apical region in the Arabidopsis embryo. Plant J. 10, 1005–1016. doi: 10.1046/j.1365-313X.1996.10061005.x
Toujani, W., Muñoz-Bertomeu, J., Flores-Tornero, M., Rosa-Téllez, S., Anoman, A. D., et al. (2013). Functional characterization of the plastidial 3-phosphoglycerate dehydrogenase family in Arabidopsis. Plant Physiol. 163, 1164–1178. doi: 10.1104/pp.113.226720
Valledor, L., Menéndez, V., Canal, M. J., Revilla, M. A., and Fernández, H. (2014). Proteomic approaches to sexual development mediated by antheridiogen in the fern Blechnum spicant L. Proteomics 14, 1–11. doi: 10.1002/pmic.201300166
Van Aken, O., Pečenkov,á, T., van de Cotte, B., De Rycke, R., Eeckhout, D., et al. (2007). Mitochondrial type-I prohibitins of Arabidopsis thaliana are required for supporting proficient meristem development. Plant J. 52, 850–864. doi: 10.1111/j.1365-313X.2007.03276.x
van der Honing, H. S., Kieft, H., Emons, A. M. C., and Ketelaar, T. (2012). Arabidopsis VILLIN2 and VILLIN3 are required for the generation of thick actin filament bundles and for directional organ growth. Plant Physiol. 158, 1426–1438. doi: 10.1104/pp.111.192385
Vedenecheva, N. P., and Sytnik, K. M. (2013). Cytokinins localization and dynamics in different parts of Equisetum arvense L. Dop. Nac. akad. nauk Ukr. 11, 150–156.
Vedenicheva, N. P., and Kosakivska, I. V. (2016). Endogenous cytokinins of water fern Salvinia natans (Salviniaceae), Ukr. Bot. J. 72, 277–282. doi: 10.15407/ukrbotj73.03.277
Vedenicheva, N. P., and Kosakivska, I. V. (2018). Endogenous cytokinins dynamics during development of perennial fern Dryopteris filix-mas and Polystichum aculeatum. Ukr. Bot. J. 75, 384–391. doi: 10.15407/ukrbotj75.04.384
Vellosillo, T., Martínez, M., López, M. A., Vicente, J., Cascón, T., et al. (2007). Oxylipins produced by the 9-lipoxygenase pathway in Arabidopsis regulate lateral root development and defense responses through a specific signaling cascade. Plant Cell 19, 831–846. doi: 10.1105/tpc.106.046052
Vlot, A. C., Dempsey, D. A., and Klessig, D. F. (2009). Salicylic acid, a multifaceted hormone to combat disease. Annu. Rev. Phytopathol. 47, 177–206. doi: 10.1146/annurev.phyto.050908.135202
Vyskot, B., and Hobza, R. (2004). Gender in plants: sex chromosomes are emerging from the fog. Trends Genet. Elsevier Curr. Trends 20, 432–438. doi: 10.1016/j.tig.2004.06.006
Wang, Y., Ries, A., Wu, K., Yang, A., and Crawford, N. M. (2010). The Arabidopsis prohibitin gene phb3 functions in nitric oxide-mediated responses and in hydrogenperoxide-induced nitric oxide accumulation. Plant Cell 22, 249–259. doi: 10.1105/tpc.109.072066
Ward, J., Ponnala, L., and Weber, C. (2012). Strategies for transcriptome analysis in nonmodel plants. Am. J. Bot. 99, 267–276. doi: 10.3732/ajb.1100334
Warne, T. R., and Hickok, L. G. (1989). Evidence for a gibberellin biosynthetic origin of Ceratopteris antheridiogen. Plant Physiol. 89, 535–538. doi: 10.1104/pp.89.2.535
Wyder, S., Rivera, A., Valdés, A. E., Jesús, M., Gagliardini, V., Fernández, H., et al. (2020). Plant physiology and biochemistry differential gene expression profiling of one- and two-dimensional apogamous gametophytes of the fern Dryopteris affinis ssp. affinis. Plant Physiol. Biochem. 148, 302–311. doi: 10.1016/j.plaphy.2020.01.021
Xu, L., and Shen, W. H. (2008). Polycomb silencing of KNOX genes confines shoot stem cell niches in Arabidopsis. Curr. Biol. 18, 1966–1971. doi: 10.1016/j.cub.2008.11.019
Yamane, H. (1998). Fern antheridiogens. Int. Rev. Cytol. 184, 1–6. doi: 10.1016/S0074-7696(08)62177-4
Yano, A., Kodama, Y., Koike, A., Shinya, T., Kim, H. J., et al. (2006). Interaction between methyl CpG-binding protein and Ran GTPase during cell division in tobacco cultured cells. Ann. Bot. 98, 1179–1187. doi: 10.1093/aob/mcl211
Yokota, T., Ohnishi, T., Shibata, K., Asahina, M., Nomura, T., et al. (2017). Occurrence of brassinosteroids in non-flowering land plants, liverwort, moss, lycophyte and fern. Phytochemistry 136, 46–55. doi: 10.1016/j.phytochem.2016.12.020
Yoshikawa, M., Peragine, A., Park, M. Y., and Poethig, R. S. (2005). A pathway for the biogenesis of trans-acting siRNAs in Arabidopsis. Genes Dev. 19, 2164–2175. doi: 10.1101/gad.1352605
Zhang, H., Ransom, C., Ludwig, P., and van Nocker, S. (2003). Genetic analysis of early flowering mutants in Arabidopsis defines a class of pleiotropic developmental regulator required for expression of the flowering-time switch flowering Locus C. Genetics 164, 347–358. doi: 10.1093/genetics/164.1.347
Zhou, X., Liao, W.-J., Liao, J.-M., Liao, P., and Lu, H. (2015). Ribosomal proteins: functions beyond the ribosome. J. Mol. Cell Biol. 7, 92–104. doi: 10.1093/jmcb/mjv014
Keywords: apogamy, apomixis, Dryopteris affinis ssp. affinis, Dryopteris oreades, fern, gametophyte, plant growth regulator, proteomic
Citation: Fernández H, Grossmann J, Gagliardini V, Feito I, Rivera A, Rodríguez L, Quintanilla LG, Quesada V, Cañal MJ and Grossniklaus U (2021) Sexual and Apogamous Species of Woodferns Show Different Protein and Phytohormone Profiles. Front. Plant Sci. 12:718932. doi: 10.3389/fpls.2021.718932
Received: 01 June 2021; Accepted: 23 September 2021;
Published: 12 November 2021.
Edited by:
Paloma Moncaleán, Neiker Tecnalia, SpainReviewed by:
Danuše Tarkowská, Academy of Sciences of the Czech Republic, CzechiaFulvio Pupilli, National Research Council (CNR), Italy
Copyright © 2021 Fernández, Grossmann, Gagliardini, Feito, Rivera, Rodríguez, Quintanilla, Quesada, Cañal and Grossniklaus. This is an open-access article distributed under the terms of the Creative Commons Attribution License (CC BY). The use, distribution or reproduction in other forums is permitted, provided the original author(s) and the copyright owner(s) are credited and that the original publication in this journal is cited, in accordance with accepted academic practice. No use, distribution or reproduction is permitted which does not comply with these terms.
*Correspondence: Helena Fernández, ZmVybmFuZGV6ZWxlbmFAdW5pb3ZpLmVz