- 1Department of Viticulture and Enology, University of California, Davis, Davis, CA, United States
- 2Department of Agronomy, Biotechnology and Food Science, Public University of Navarra, Pamplona, Spain
- 3Semios Biotechnologies Toronto, Toronto, ON, Canada
Climate change models predict lower precipitation and higher air temperatures that will negatively affect viticultural regions. Irrigation of vineyards will be crucial for mitigating abiotic stress during the growing season. However, the environmental impact of irrigation requires consideration for ensuring its sustainability in the future. We evaluated the standard irrigation practices on grapevine water use efficiency, berry flavonoid composition, vineyard water footprint, and arbuscular mycorrhizal fungi-grapevine symbiosis in two seasons with contrasting amounts of precipitation. The irrigation treatments consisted of weekly replacement of 25, 50, and 100% of crop evapotranspiration (ETc) during two growing seasons. Irrigation in grapevine vineyards mitigated the water scarcity when precipitation during the dormant season was not sufficient. The results provided field data supporting that despite the low rainfall recorded in one of the seasons, increasing the amount of irrigation was not advised, and replacing 50% ETc was sufficient. In this treatment, berry composition was improved with increased contents of total soluble solids, anthocyanins, and flavonols, and a stable flavonoid profile without an economic decrease in yield. In addition, with 50% ETc, the mycorrhizal symbiosis was not compromised and water resources were not highly impacted. Altogether, our results provide fundamental knowledge for viticulturists to design an appropriate irrigation schedule under the future warming scenarios with minimal environmental impact in semi-arid regions facing warming trends.
Introduction
Global warming trends due to climate change are likely to continue at the current rate, leading to temperature increases of 1.5–4.5°C between 2030 and 2052 (IPCC et al., 2018). Furthermore, changes in precipitation patterns, frequencies of heatwaves, droughts, and a general increase in evapotranspiration (ET) rates are also expected (IPCC et al., 2018). These changes would in turn, affect soil moisture, ground water table, storage of water in reservoirs, and the salinization of shallow aquifers (Bates et al., 2008).
The effects of climate change are leading to an increasingly negative water balance during the grapevine growing season (Martínez-Lüscher et al., 2017, 2020). The effects have been an advance of grapevine phenology, diminution of yield and a lack of cultivar trait expression at the farm gate (Costa et al., 2016; Mosedale et al., 2016; Torres et al., 2018a; Venios et al., 2020). Different approaches have been used in recent years to cope with these effects, such as the use of drought-tolerant rootstocks, clones and/or varieties, improved training systems or increased row spaces, and irrigation applications (van Leeuwen et al., 2019). Although winegrowers still prioritize canopy and soil management and changes in harvest date and winemaking techniques over water management (Neethling et al., 2017), use of irrigation in vineyards is inevitable in historically non-irrigated areas due to the warming trends (Costa et al., 2016; Resco et al., 2016). Furthermore, many of the viticulture areas of the world rely on irrigation for consistent production (Torres et al., 2021b), such as California, where irrigation is required to overcome the dry and warm summers. Thus, irrigated agriculture in California is the largest consumer of fresh water, which accounted for about 50% of the total water supplied to the state in 2011–2015 (CDWR, 2019). However, water resources, particularly groundwater, have reached a critical state due to extended drought periods, and overuse by irrigated agriculture (Wilson et al., 2020). In fact, Alam et al. (2019) recently reported that increases in water demand and decreases in surface water supply caused by a warming climate might negatively affect groundwater storage, especially in regions like the San Joaquin Valley of California, where groundwater reserve is already stressed. Therefore, evidence support the necessity of finding sustainable practices in vineyard production systems for long-time exploitation of natural resources, such as water and soil.
Water footprint (WF) is the volume of water used per unit of food produced. It was suggested as an indicator of the total water used for grape production to promote sustainable and efficient use of water in viticulture (Mekonnen and Hoekstra, 2011). WF is the sum of three components; the green WF or water from precipitation, the blue WF or irrigation water sourced from surface or groundwater resources, and the gray WF that is the amount of fresh water required to assimilate pollutants to meet specific water quality standards (Mekonnen and Hoekstra, 2011). The agriculture sector accounted for 92% of the total WF (about 8,360 billion m3/year; Mekonnen and Hoekstra, 2011). The WF of irrigated crops recently came under scrutiny due to socioeconomic concerns and the need to reduce it (Cominelli et al., 2009; D'Ambrosio et al., 2020). Intrinsic water use efficiency (iWUE) is the ratio of moles of CO2 assimilated and moles of water transpired by the plant (Tomás et al., 2014). It is an indicator of how efficient the grapevine is, utilizing water to produce photosynthates. Deficit irrigation strategies were developed to reduce the amount of water applied to the grapevine, substantially reducing the WF and increasing the iWUE. The other aim in applying water deficits was to maintain or improve grape berry composition (Terry and Kurtural, 2011). Deficit irrigation methods apply a predetermined fraction of the crop evapotranspiration (ETc), during a portion of the growing season (Torres et al., 2021a). A large and growing body of literature investigated how these strategies, including various timing, duration, and severity, affected grapevine physiology and consequently, berry composition regarding sugar and anthocyanin accumulation and the subsequent chemical composition in wines (Chaves et al., 2010, Intrigliolo and Castel, 2008; Keller et al., 2016; da Silva et al., 2018; Torres et al., 2021b). Nevertheless, the WF of these strategies at a local scale in warm climates has not been addressed thoroughly.
Arbuscular mycorrhizal fungi (AMF) are soil-borne fungi that establish mutualistic relationships with terrestrial plants including grapevines, being key components of the viticulture production systems (Trouvelot et al., 2015; Torres et al., 2018a). The symbiosis of grapevines with AMF may be affected by stress factors related to climate change (Compant et al., 2010). In addition, previous studies demonstrated that management practices strongly shaped bacterial and fungal communities in vineyard soils (Coller et al., 2019; Vink et al., 2021), which may modulate grapevine responses to environmental stresses. Under controlled conditions, the association of grapevines with AMF enhanced berry quality (i.e., increased phenolic content) when potted grapevines were subjected to deficit irrigation and elevated temperature (Torres et al., 2018b,c), whereas berry flavonoid metabolism was upregulated in AMF-inoculated grapevines in vineyards (Torres et al., 2021a).
Previous work indicated that different replacements of the ETc affected grapevine physiology (water status and gas exchange parameters), leading to a different carbon allocation between source and sink organs (Torres et al., 2021b). This study also demonstrated that replacing 50% of the ETc was sufficient to sustain the grapevine performance through the enhancement of sugar transport that could slow down the detrimental effect of water deficits on yield (Torres et al., 2021b). Based on the above-mentioned literature, it was hypothesized that (1) water deficits may increase grapevine iWUE, promoting the balance between vegetative and reproductive growth and improving berry composition in a hot climate and (2) deficit irrigation strategies may exert a different pressure on water resources and AMF associated with grapevines. Therefore, the aim of this study was to evaluate three applied water amounts based on different fractions of the ETc for maintaining berry quality without compromising yield and minimizing their environmental impact concerning total WF and AMF colonization rates. This work covered the effect of irrigation strategies on different interrelated elements of vineyard production systems such as the soil water storage or the AMF abundance together with the productive characteristics in terms of yield and quality, advancing in the knowledge of a more sustainable water management.
Materials and Methods
Plant Material and Experimental Design
The experiment was conducted on Cabernet Sauvignon (clone FPS08) on 110R rootstock during two consecutive seasons (2018–2019 to 2019–2020) in Oakville, CA (38.428°N, 122.409°W). Grapevines were planted in 2011 with a spacing of 2.4 by 2.0 m (row × vine) with a row orientation of North West-South East. The grapevines were trained to a bilateral cordon on a vertically shoot positioned trellis with a cordon height 96 cm above vineyard floor and pruned to 30 spurs (15 spurs/m) and one bud per spur. The experiment was designed as a randomized complete block with a one-way arrangement of the following fractions of ETc replacement treatments: (i) 25% ETc, (ii) 50% ETc, and (iii) 100% ETc. Each treatment was replicated six times with five grapevines in each treatment replicate. The three middle vines within the treatment replicate were used for data collection and the two on distal ends were treated as buffer plants. Plants were irrigated weekly with two drip emitters per vine. Other cultural practices were standard for the area and conducted before treatment application.
Weather Data and Applied Water Amounts Treatments
Weather data (Figure 1A) were obtained from the California Irrigation Management Information System (CIMIS, station #77, Oakville, CA) located 160 m from the experimental vineyard (CIMIS, 2020).
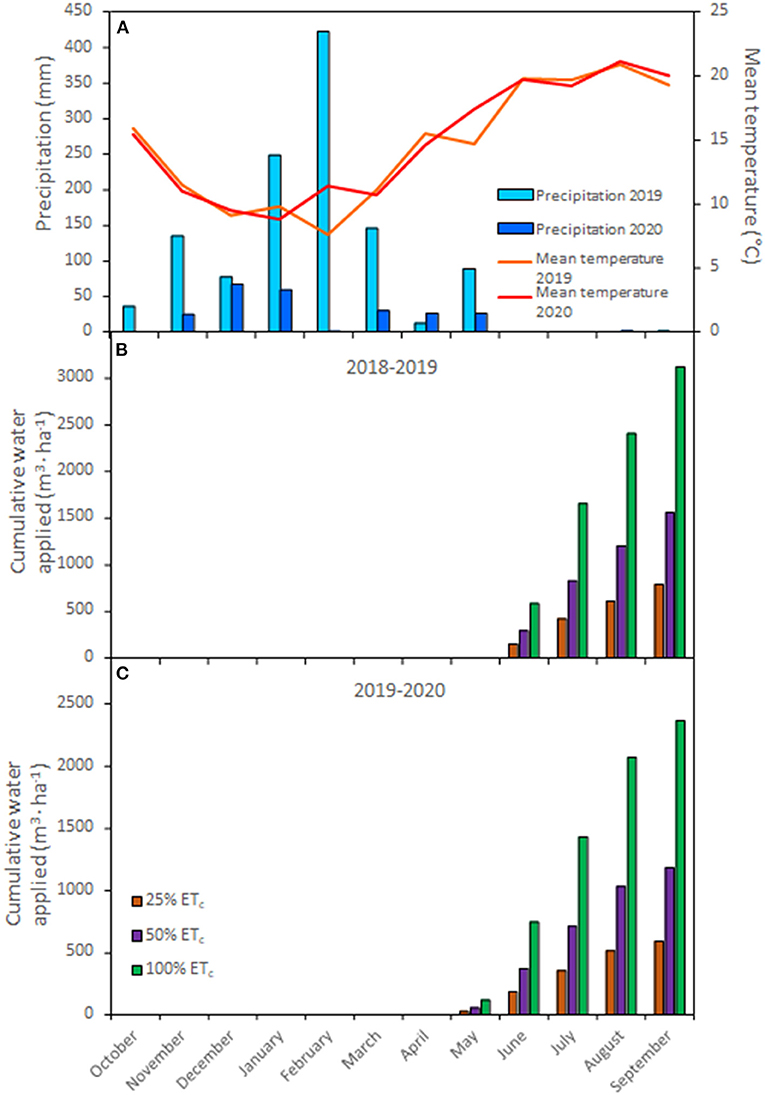
Figure 1. Mean air temperature (°C) and precipitation (mm) during the 2018–2019 and 2019–2020 growing seasons (A) Weather data were obtained from the CIMIS weather station # 77 (Oakville, CA) located at the research site. Cumulative water applied (m3 · ha−1) to Cabernet Sauvignon grapevines (clone FPS08) according to the different replacements of crop evapotranspiration (25, 50, and 100% ETc) during the 2018–2019 (B) and 2019–2020 (C) growing seasons in Oakville, CA.
The applied water amounts treatments consisted of replacing different fractions of ETc. Vineyard ETc was calculated using the following equation: ETc = ETo × Kc, where ETo is the reference ET and Kc is the crop coefficient. The ETo was measured by the California Irrigation Monitoring Information System station 77, 160 m from this experimental vineyard. The evaluation of vineyard Kc over the course of the growing season was calculated using the shade cast beneath grapevines grown with 100% ETo application from April 1 and the vertical shoot-positioned (VSP)-specific equation developed by Williams (2014) and adjusted for row spacing. The irrigation treatments were imposed when stem water potential was lower than −1 MPa combined with a visual assessment of the first symptoms of water stress in petioles and leaves (June 2019 and May 2020) to harvest (no irrigation was used after harvest) of each growing season (Figures 1B,C). Different irrigation treatments were implemented through the variation of the emitter output per grapevine in the drip line from 8 L · h−1 to 2 L · h−1 and 4 L · h−1, respectively, and the irrigation pump was scheduled to run based on the application rate of the 100% ETc treatment plots. Thus, the 25, 50, and the 100% ETc plots received 4 L · h−1, 8 L · h−1, and 16 L · h−1 per grapevine, respectively.
iWUE and Crop Water Use Efficiency
Leaf gas exchange was measured with a CIRAS-3 portable infrared gas analyzer system (PP Systems, Amesbury, MA, USA) featuring a broad-leaf chamber with a 4.5 cm2 window. For each sample date and treatment replicate, three measurements were made ca. solar noon (11:30 to 13:30 h) on a healthy and fully expanded leaf within a minute to obtain an accurate range of leaf gas exchange and then, values were averaged. The cuvette was oriented perpendicularly to sunlight, which was always in saturating conditions (average of internal PAR = 1969 ± 135 μmol · m−2 s−1). Chamber conditions were set up at 40% relative humidity, a CO2 concentration of 400 μmol·mol−1, and using a flow to the chamber of 300 mL·min−1. The iWUE was calculated as the ratio between carbon assimilation rate (AN) and stomatal conductance (gs) and expressed as μmol CO2 ·mmol H2O−1. The crop water use efficiency (WUEc) was calculated as the ratio between yield expressed as kg·ha−1 and the amount of water applied to each plot (m3 · ha−1) as reported by Medrano et al. (2015).
Carbon Isotope Composition of Musts (δ13C)
Carbon stable isotope composition (δ13C) was measured in the berry must at harvest following the protocol described by Gaudillère et al. (2002). A 2 mL aliquot was centrifuged at 14,119 × g to remove suspended solids. Then, 5 μL of the supernatant was pipetted in thin capsules, dried overnight at 60°C, and encapsulated using tweezers. Isotopic analyses were performed at the UC Davis Stable Isotope facility, using a PDZ Europa ANCA-GSL elemental analyzer interfaced to a PDZ Europa 20–20 isotope ratio mass spectrometer (Sercon Ltd., Cheshire, United Kingdom) following the methods described in Brillante et al. (2020). Results for each of the samples were normalized to the reference standard MAB using the following equation (1) and expressed in delta notation:
where Rsample and Rstandard are the absolute 13C/12C ratios for sample and standard. The values of δ13C are reported in parts per thousand with respect to the Vienna Pee Dee Belemnite (VPDB) international reference.
Yield Components and Leaf Biomass
The harvest commenced when the berry total soluble solids (TSS) reached ca. 24°Brix on an average in all treatments on September 25, 2019 [114 days after flowering (DAF)] and September 8, 2020 (115 DAF), respectively. Clusters per vine were counted and weighed on a top-loading balance. One vine per treatment replicate was then defoliated and leaves were weighed to obtain leaf mass per area unit (tonnes · ha−1). Leaf area was measured with a LI-3100 Area meter (LI-COR, Lincoln, NE, USA) on a subsample of leaves and then it was related to dry mass (e.g., via specific leaf area (SLA) cm2 · g−1). The total dry mass of leaves collected within a known ground surface area was converted into leaf area index (LAI) by multiplying with the SLA. In the following winter of each season, shoots were pruned, and weighed on a top-loading balance to determine pruning mass. The yield to pruning mass ratio was calculated as the ratio between the yield and the pruning mass in the following dormant season.
Berry Size and Primary Metabolites
At harvest, 60 berries were randomly collected from the three middle grapevine of each replicate (n = 6) during both growing seasons and immediately processed. Berries were weighed, averaged, and berry mass was obtained during the growing season. At harvest, berries were gently pressed by hand to express the juice. The TSS were determined using a temperature-compensating digital refractometer (Atago PR-32, Bellevue, WA, USA). Must pH and titratable acidity (TA) were determined with an autotitrator (Metrohm 862 Compact Titrosampler, Herisau, Switzerland). TA was estimated by titration with 0.1 N sodium hydroxide to an end point of 8.3 pH and reported as g · L−1 of tartaric acid.
Berry Skin Flavonoid Composition
Berry skin flavonoid composition was determined in 20 berries randomly collected from each treatment replicate (n = 6). Berries were gently peeled and skins were freeze-dried (Cold Trap 7,385,020, Labconco, Kansas City, MO, USA) and ground with a tissue lyser (MM400, Retsch, Germany). Fifty (50) mg of the resultant powder was extracted in methanol: water: 7 M hydrochloric acid (70:29:1, V:V:V) to simultaneously determine flavonol and anthocyanin concentration and profile as previously described in Martínez-Lüscher et al. (2019). Briefly, extracts were filtered (0.45 μm, Thermo Fisher Scientific, San Jose, CA, USA) and analyzed using an Agilent 1,260 series reversed-phase high performance liquid chromatography (HPLC) system (Agilent 1,260, Santa Clara, CA, USA) coupled to a diode array detector. Separation was performed on a reversed-phase C18 column LiChrospher® 100, 250 × 4 mm with a 5 μm particle size and a 4 mm guard column of the same material at 25°C with a flow rate of 0.5 mL/min. Chromatographic conditions were previously reported in Martínez-Lüscher et al. (2019). Commercial standards of malvidin-3-O-glucoside and quercetin-3-O-glucoside (Sigma-Aldrich, St. Louis, MO, USA) were used for the quantification of anthocyanins and flavonols, respectively.
AMF Colonization
Intraradical AMF colonization was measured after harvest of each season where root samples (mainly root hairs) from the three middle grapevines per treatment replicate were collected at a depth of 15 and 20 cm away from the vine trunk. Root samples were cleaned, cleared, and stained according to methods described in Koske and Gemma (1989). Fifty root segments per replicate (~1 cm each) were examined under the microscope to determine intraradical AMF colonization as previously described in Torres et al. (2016, 2021a). Briefly, the extension of mycorrhizal colonization was determined by estimating its product in width and length according to a scale range between 0 and 10, where 0 is the complete absence of fungal structures. Then, the incidence of mycorrhizal colonization was estimated by dividing the number of root segments with the presence of fungal structures and the total observed segments. The intensity of the colonization was calculated as the product between the extension and incidence, and the result was expressed as a percentage of colonization.
WF Assessment
WF was calculated following the methods described in Zotou and Tsihrintzis (2017) with minor modifications. Briefly, WF was derived as the sum of the green, blue, and gray WFs and expressed in m3 of water consumed per tonne of fruit harvested. Green, blue, and gray components were given by following equations:
where Pm is the monthly effective precipitation expressed in m3 · ha−1 after applying a conversion factor of 10 and Y is the yield of grapevines expressed in tonne·ha−1.
where WUm is the total amount of irrigation water received by the grapevines monthly expressed in m3−. ha−1 and Y is the yield of grapevines expressed in tonne·ha−1
where α is the percentage of fertilizer that leaches to the receiving aquatic system; AR is the amount of fertilizer applied to the grapevines expressed in kg · ha−1; cmax is the maximum acceptable concentration of fertilizer in the aquatic system (mg · L −1); and cnat is the natural concentration of the pollutant in the aquatic system (mg · L−1). Pm values were obtained from the CIMIS station placed in the vineyard. For gray component calculation, only nitrogen fertilization was considered, given the environmental issues derived from its use in agriculture (UC Davis, 2016). The percentage of nitrogen entering the water system of the area was assumed 10% according to Mekonnen and Hoekstra (2011). The maximum acceptable concentration of nitrogen (45 mg · L−1) was obtained from CDFA California Department of Food Agriculture (2020). According to Hoekstra et al. (2011), the natural concentration of pollutants was taken equal to zero, as proposed when data were missing.
Statistical Analyses
Statistical analyses were conducted with R studio version 3.6.1 (RStudio: Integrated Development for R., Boston, MA, USA) for Windows. After normality assessment, data were submitted to an ANOVA to assess the statistical differences between the different irrigation treatments. Means ± SEs were calculated and when the F value was significant (P ≤ 0.05), a Tukey's “Honest Significant Difference” (HSD) post hoc test was executed by using “agricolae” 1.2–8 R package (de Mendiburu, 2016). Pearson correlation analyses were conducted with the same software.
Results
Weather Conditions
Mean day temperature and precipitation recorded by the CIMIS station are presented in Figure 1A. The measurements/records started in October of the previous year to account for the rain received during the dormant season. In the second year (2020) of the experiment, precipitation was five times less compared to the long term averages, with an exceptionally arid February. Mean day temperatures were similar between both years (14.5 and 14.9°C, respectively). However, the 2020 growing season had 17 more days with high temperatures (over 30°C). Therefore, irrigation in 2020 started 1 month earlier than in 2019 (Figures 1B,C). Cumulative water applied at the end of the season reflected the different water applications across treatments where 100% ETc plots received 2,335 and 1,772 m3 · ha−1 more in 2019 and 2020 growing seasons, respectively, compared with 25% ETc plots.
Grapevine iWUE, WUEc, Must δ13C, Growth, and Yield Components Were Affected by Irrigation Treatments
The 25 and 50% ETc irrigation treatments increased the iWUE, starting around véraison (DAF 57 for 2019 and DAF 62 for 2020) until harvest (Figures 2A,B). At harvest, 25 and 50% ETc improved the iWUE between 18.6 and 29.2% in 2019 and between 29.2 and 42.9% in 2020, respectively, compared with the 100% ETc treatment. The WUEc was higher with the 25 and 50% ETc compared with 100% ETc in 2019, whereas only 25% ETc improved WUEc in 2020 (Figure 2C). The berry must δ13C was greater in treatments subjected to higher water deficits (Figure 2D). Thus, berry must δ13C was higher in 25 and 50% ETc treatments in 2019 while in 2020, only in 25% ETc was significantly higher compared with 100% ETc treatment.
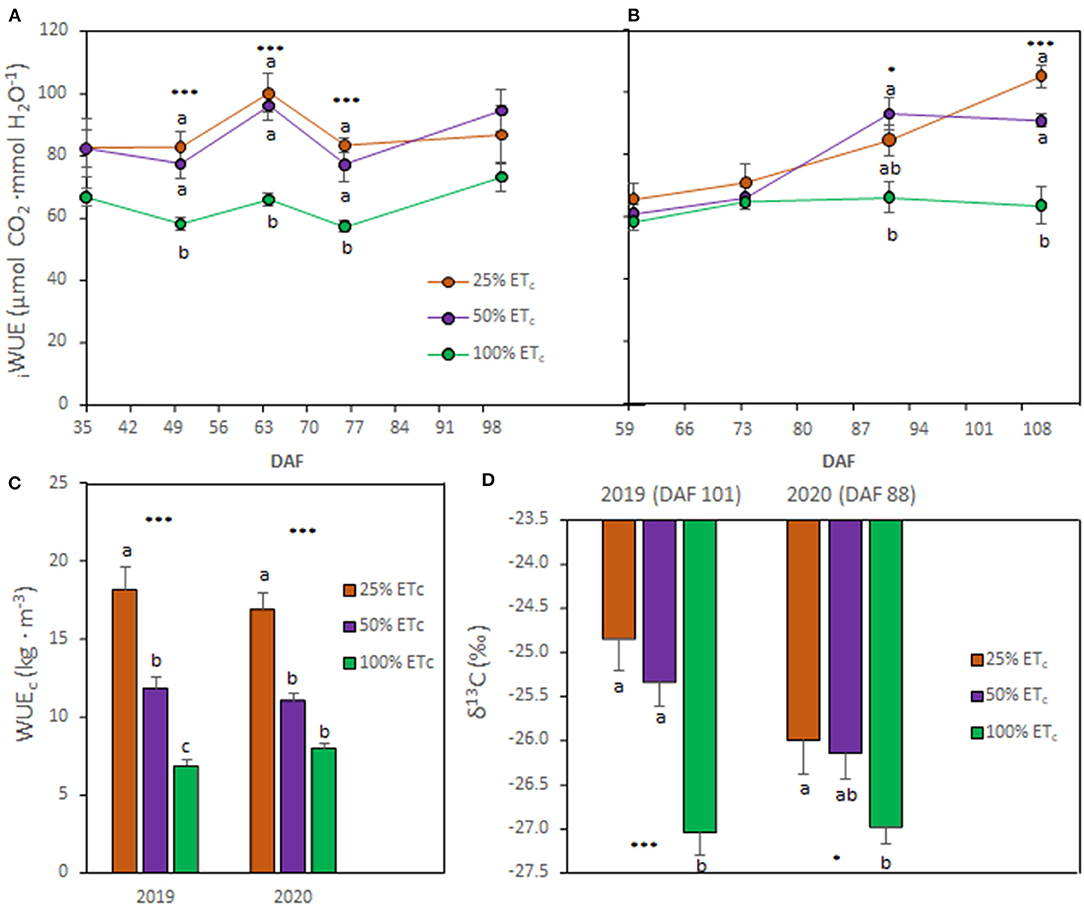
Figure 2. Intrinsic water use efficiency (iWUE, A and B), crop WUE (WUEc, C), and δ13C of berry must (D) of Cabernet Sauvignon grapevines (clone FPS08), subjected to different replacements of crop evapotranspiration (25, 50, and 100% ETc) and collected through 2018–2019 and 2019–2020 growing seasons in Oakville, CA. Values represent means ± SE (n = 6). At each time point, different letters indicate significant differences (p ≤ 0.05) between irrigation treatments according to one-way ANOVA followed by Tukey HSD test. *, and *** indicate significance at 5, and 0.1% probability levels, respectively. DAF, Days after flowering.
Yield components and vegetative growth responded to irrigation treatments during both growing seasons (Table 1). Cluster mass decreased with 25% ETc, whereas 100% ETc increased it. The number of clusters per vine ranged between 56 and 59 and between 53 and 55 in 2019 and 2020 growing seasons, respectively, and did not differ between treatments. Likewise, a significant enhancement of vegetative growth was measured by increases in LAI and total leaf biomass per area unit area with the 100% ETc. Yield to pruning mass ratio was only affected by treatments in the 2019 growing season where 25% ETc decreased it compared with other treatments.
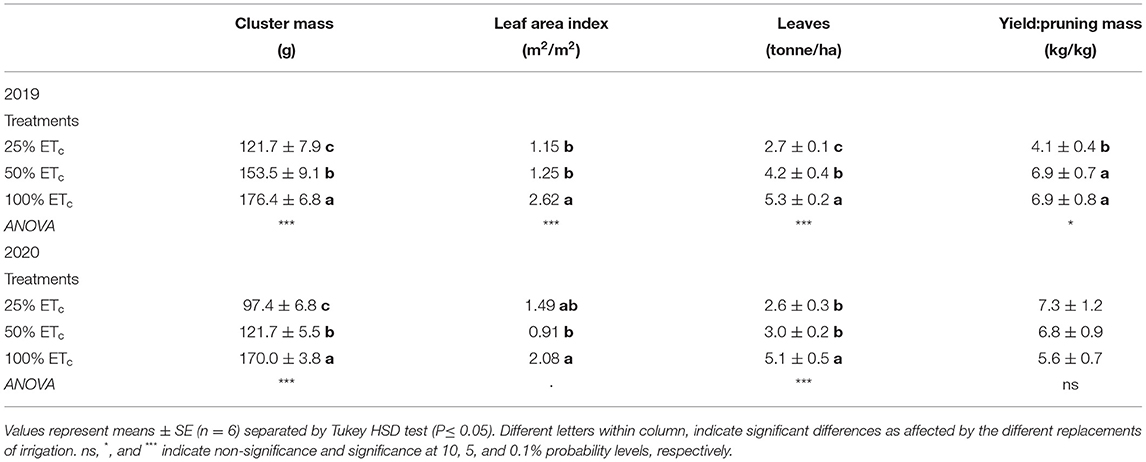
Table 1. Reproductive and vegetative growth of Cabernet Sauvignon grapevines (clone FPS08) subjected to different replacement of crop evapotranspiration (25, 50, and 100% ETc), collected in Oakville, CA, in 2018–2019 and 2019–2020 seasons.
Berry Composition Was Affected by Replacement of Different ETc Fractions
Berry mass was affected by the applied water treatments during berry ripening in both seasons (Figures 3A,B) where it was the highest with the 100% ETc treatment. We did not measure any treatment differences in TSS, pH, or TA in 2019 (Table 2). In the 2020 growing season, 100% ETc treatment resulted in a lower TSS but higher must pH at harvest, compared with other treatments.
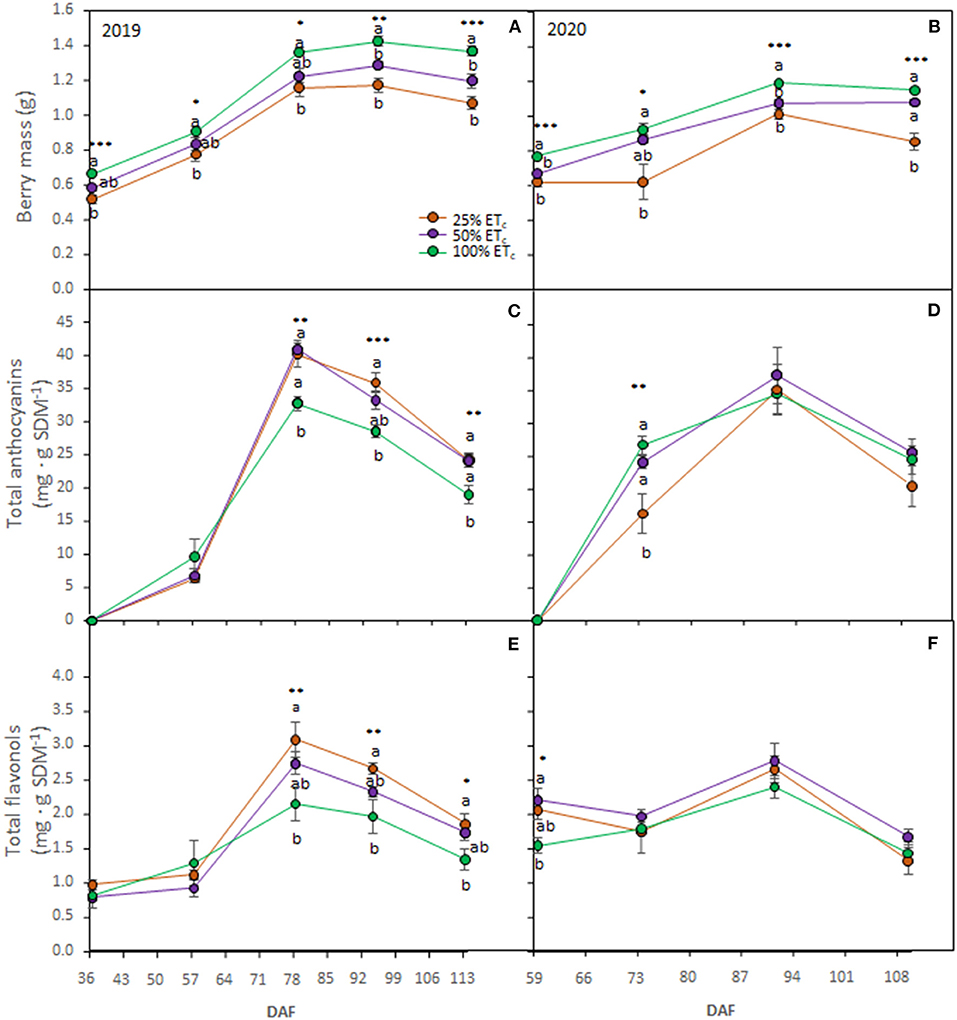
Figure 3. Berry mass (A, B), total anthocyanins (C, D), and total flavonols (E, F) in berry skin dry mass (SDM) of Cabernet Sauvignon grapevines (clone FPS08), subjected to different replacements of crop evapotranspiration (25, 50, and 100% ETc) and collected through 2018–2019 and 2019–2020 growing seasons in Oakville, CA. Values represent means ± SE (n = 6). At each time point, different letters indicate significant differences (p ≤ 0.05) between irrigation treatments according to one-way ANOVA followed by Tukey HSD test. *, **, and *** indicate significance at 5, 1, and 0.1% probability levels, respectively. DAF, Days after flowering.
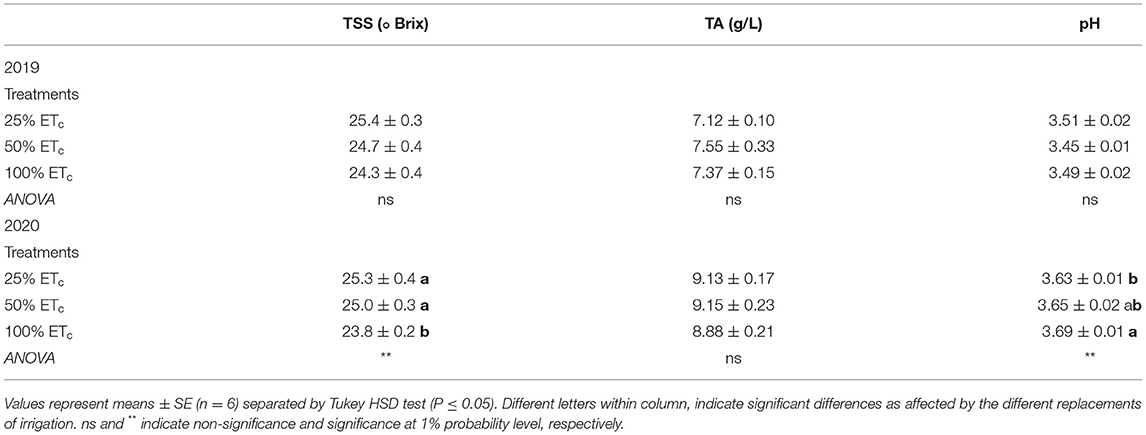
Table 2. Total soluble solids (TSS), titratable acidity (TA) and pH of Cabernet Sauvignon grapevines (clone FPS08) subjected to different replacement of crop evapotranspiration (25, 50, and 100% ETc), collected at harvest in Oakville, CA, in 2018–2019 and 2019–2020 seasons.
In 2019, the 100% ETc decreased berry anthocyanin and flavonol contents from DAF 78 to harvest (DAF 114) (Figures 3C,E). In 2020, berry anthocyanin and flavonol contents were not affected by treatments except at prevéraison (DAF 59) where 100% ETc decreased berry skin flavonol (Figure 3F). At véraison in 2020, the 25% ETc had a significantly lower content of anthocyanins (Figure 3D) compared with other treatments.
Malvidin derivatives were the most abundant anthocyanins found in the Cabernet Sauvignon berry skins, ranging from 74 to 78% and 65 to 74% in 2019 and 2020 growing seasons, respectively (Table 3). The treatments strongly affected anthocyanin compounds in both seasons. In 2019, at harvest, 50 and 100% ETc increased the proportion of peonidins and 25% ETc had a significantly higher proportion of petunidin derivatives.
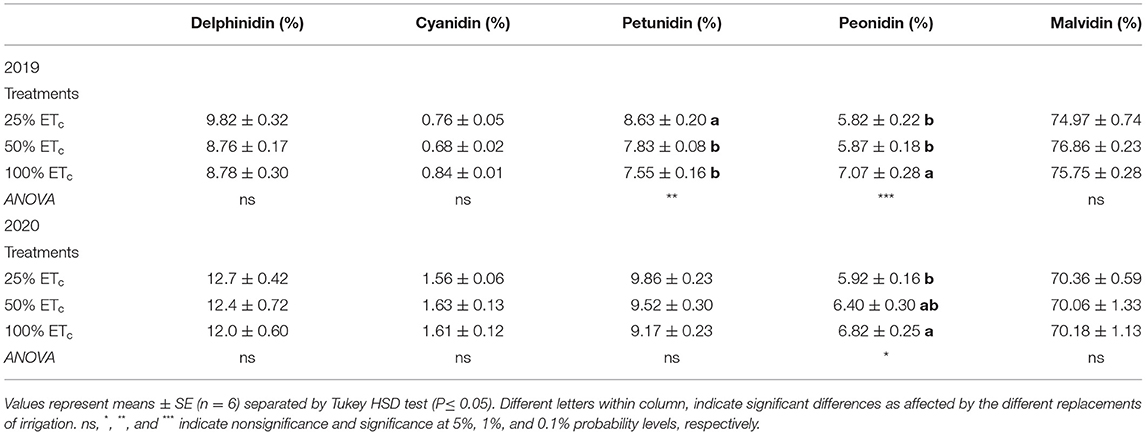
Table 3. Anthocyanin composition (%) of Cabernet Sauvignon grapevines (clone FPS08) subjected to different replacement of crop evapotranspiration (25, 50, and 100% ETc), collected at harvest in Oakville, CA, in 2018–2019 and 2019–2020 seasons.
Flavonol composition was only affected by irrigation treatments during the 2020 growing season at harvest (Table 4). Myricetin and quercetin derivatives were the main flavonols found in Cabernet Sauvignon berry skins and both accounted for about 75% of the total amount. The most restrictive applied water treatment increased proportion quercetins and kaempferols, while 100% ETc increased myricetins and syringetins.
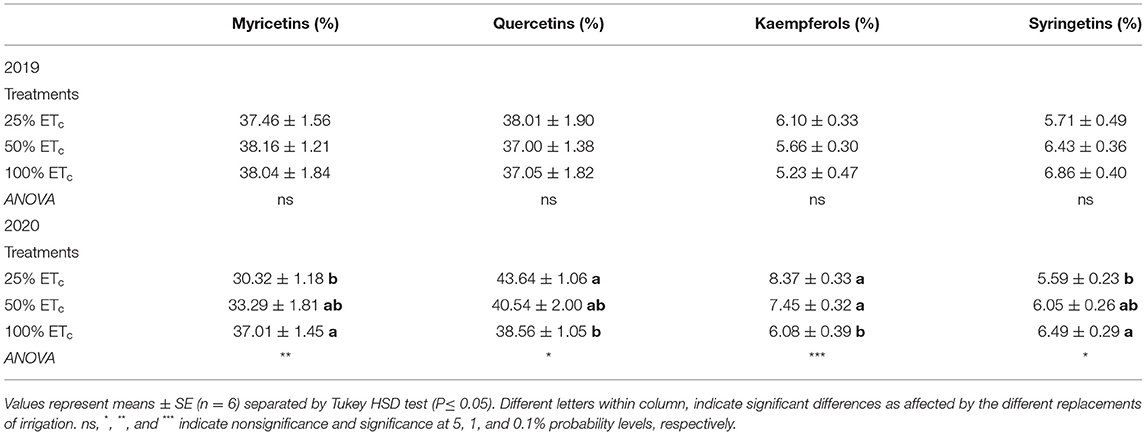
Table 4. Flavonol composition (%) of Cabernet Sauvignon grapevines (clone FPS08) subjected to different replacement of crop evapotranspiration (25, 50, and 100% ETc), collected at harvest in Oakville, CA, in 2018–2019 and 2019–2020 seasons.
Applied Water Amounts Influenced the Native Mycorrhizal Colonization of Grapevine Roots
The analysis of native mycorrhizal colonization of grapevines indicated that 100% ETc decreased the abundance of mycorrhizal structures compared with other treatments (Figure 4A). Thus, in 100% ETc, AMF colonization decreased by 10% in both growing seasons (Figure 4B) compared with the AMF colonization measured in 25% ETc where the fungal structures were especially abundant (Figure 4C). The AMF colonization of 100% ETc was 5% lower when compared with 50% ETc.
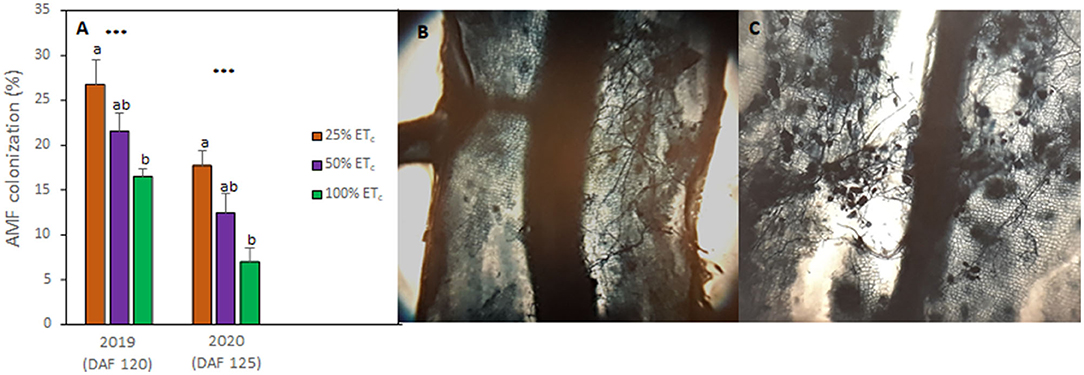
Figure 4. Arbuscular mycorrhizal fungi (AMF) colonization rates of Cabernet Sauvignon grapevines (clone FPS08) subjected to different replacement of crop evapotranspiration (25, 50, and 100% ETc), collected in Oakville, CA, in 2018–2019 and 2019–2020 growing seasons (A). Bars represent means ± SE (n = 6). At each growing season, different letters indicate significant differences (p ≤ 0.05) between irrigation treatments according to one-way ANOVA followed by Tukey HSD test. *** indicate significance at 0.1% probability level. Microscopic image (×100) of fungal structures Cabernet Sauvignon roots present in half of the root fragment (B) and in the whole fragment (C).
Applied Water Amounts Affected the Total WF and Its Components
Analysis of the effect of different applied water amounts on WF components indicated the same pattern regardless of the growing season (Figures 5A,B). The 25% ETc increased the greenWF and grayWF components and decreased the blueWF. Conversely, the 100% ETc resulted in lower greenWF and grayWF components and but higher blueWF. The totalWF increased with the 25% ETc treatment and decreased with the 100% ETc. This effect was more prominent during the second growing season (Figure 5B).
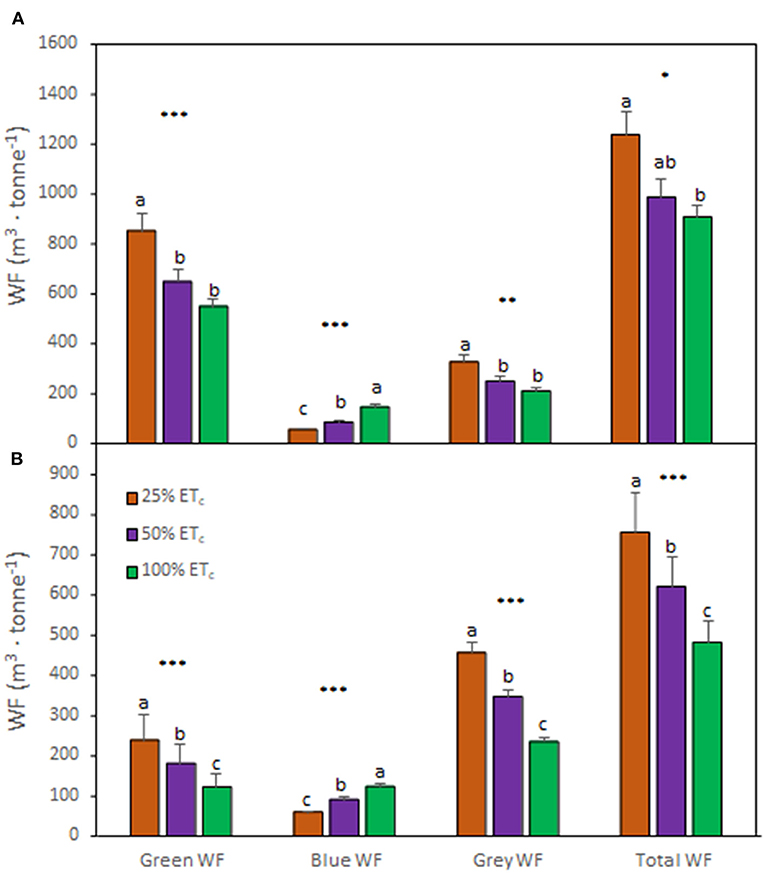
Figure 5. Water footprint (WF) components of Cabernet Sauvignon grapevines (clone FPS08) subjected to different replacement of crop evapotranspiration (25% ETc, 50% ETc, and 100% ETc), collected in Oakville, CA, in 2018–2019 (A) and 2019–2020 (B) growing seasons. Bars represent means ± SE (n = 6). At each WF component, different letters indicate significant differences (p ≤ 0.05) between irrigation treatments according to one-way ANOVA followed by Tukey HSD test. *, **, and *** indicate significance at 5, 1, and 0.1% probability levels, respectively.
Relationships Between Water Use and Grapevine Characteristics
To determine relationships between water use and grapevine characteristics, correlation analyses were conducted on the pooled data of both seasons (Figure 6). The AMF colonization rates showed direct relationships with the totalWF (Figure 6A, r = 0.80, p ≤ 0.0001) and with the δ13C of berry must (Figure 6D, r = 0.57, p ≤ 0.0001). The totalWF was positively correlated with the δ13C berry must (Figure 6B, r = 0.42, p = 0.011). There was no discernable relation between LAI and the totalWF (Figure 6C, r = −0.14, p = 0.432). However, an indirect relationship between LAI and the δ13C (Figure 6F, r = −0.52, p = 0.001) was evident. Finally, iWUE measured at harvest was positively correlated with the δ13C (Figure 6E, r = 0.33, p = 0.047).
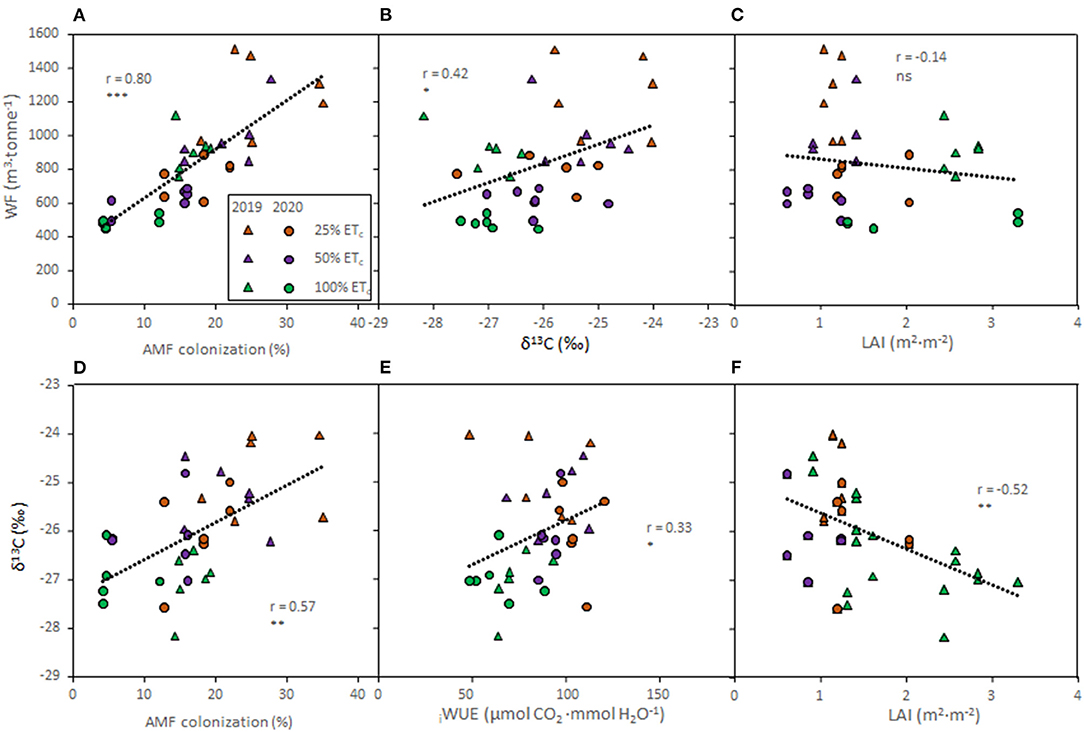
Figure 6. Relationships between total water footprint (WF) and arbuscular mycorrhizal fungi (AMF) colonization rates (A); WF and δ13C of berry must (B); and WF and leaf area index (LAI, C); δ13C of berry must and AMF (D); δ13C and intrinsic water use efficiency (iWUE, E); and δ13C and LAI (F) of Cabernet Sauvignon grapevines (clone FPS08) subjected to different replacement of crop evapotranspiration (25, 50, and 100% ETc), collected in Oakville, CA, in 2018–2019 (triangles) and 2019–2020 (circles) growing seasons. For each combination, straight lines correspond to the linear regression lines fitted for the pooled data of all treatments in both seasons. ns, *, **, and *** indicate non-significance and significance at 5, 1, and 0.1% probability levels, respectively.
Discussions
Improving WUE by Optimizing Applied Water Amounts and Their Effects on Berry Quality
This study evaluated the effect of applied water amounts based on the replacement of fractions of the ETc for maintaining berry quality while minimizing yield losses due to the environmental impact (i.e., totalWF and AMF colonization rates). Results covered two seasons that strongly differed in the precipitation supply. Compared with the average total amount of precipitation received by the area in the last decade (768 mm, CIMIS), 2019 growing season was a rainy period with 970.3 mm precipitation, while 2020 was a hyperarid growing season with only 234.2 mm of precipitation. In spite of the differences in total precipitation, the response of Cabernet Sauvignon grapevines to water deficits was consistent across both seasons and our results corroborated that deficit irrigation may mitigate the effects of water scarcity (Costa et al., 2016; Fraga et al., 2018; Torres et al., 2021b).
The results achieved in this study indicated that 25% and 50% ETc treatments were effective in improving iWUE (to ca. 100 μmol CO2 ·mmol H2O−1) compared with previous studies reporting a compilation of data from Cabernet Sauvignon (iWUE ranged 62 and 64 μmol CO2 ·mmol H2O−1) and other cultivars (11.2–103 μmol CO2 ·mmol H2O−1) (Tomás et al., 2014; Bota et al., 2016). The iWUE decreased when the fractions of applied ETc increased as previously reported by Keller et al. (2016) in a 3-year field experiment conducted on the same Cabernet Sauvignon clone as used in this study. Likewise, WUEc calculated as the ratio between yield and water applied was also enhanced with the decreased water supply. The berry must δ13C enhanced under stronger water deficits conditions corroborating previous studies with different grapevine cultivars (Bchir et al., 2016; Spangenberg and Zufferey, 2018; Brillante et al., 2020; Yu et al., 2021). The iWUE and the berry must δ13C also indicated a linear relationship in accordance to previous research (Bchir et al., 2016). Previous work indicated that δ13C of grape must is a reliable indicator of plant water status and leaf gas exchange in vineyard systems, which in turn, are crucial for the identification of plant water status zones leading to better irrigation decisions and informed management (Brillante et al., 2020). The present study also provided evidence that δ13C is a convenient tool without intensive labor and time inputs for the assessment of environmental impacts (i.e., WF and/or AMF) of deficit irrigation strategies.
Increased applied water amounts led to greater canopy size and yields (Torres et al., 2021b). There was a strong negative relationship between the berry must δ13C and grapevine vegetative growth measured as LAI. Previous studies reported a linear relationship between the δ13C and the carbon assimilation rates (Bchir et al., 2016; Brillante et al., 2020) and consequently with vegetative growth estimated as pruning mass (Brillante et al., 2020; Yu et al., 2021). Yield achieved in this experiment ranged from 4.8 to 10.4 kg · vine−1 (Torres et al., 2021b) in accordance to a previous study conducted in a vineyard at a similar density (Munitz et al., 2020). Thus, 100% ETc may double the yield compared with the 25% ETc as previously reported by Keller et al. (2016). This suggested that the effect of applied water on yield components is consistent in spite of the climate difference, planting space, and grapevine age.
Under our experimental conditions, primary metabolites (i.e., sugars and organic acids) were affected by applied water amounts in the second season, where 100% ETc accounted for lower TSS but higher pH. Increased water content in berries was associated with a lower concentration of sugars due to a dilution effect (Terry and Kurtural, 2011; Keller et al., 2016). Conversely, the lower pH in 25% ETc grapevines was related to exacerbated organic acid degradation under high temperatures by water deficit (Martínez-Lüscher et al., 2017, 2020). Berry skin flavonol and anthocyanin contents decreased with the 100% ETc in 2019 but not in 2020. Although several studies reported increases in berry flavonoid content under mild or moderate water deficit (Gambetta et al., 2020), field research conducted in California resulted in contradictory results when severe water deficits were combined with a long hang time (Brillante et al., 2018; Yu et al., 2020, 2021).
In general, 100% ETc irrigation treatment reduced the proportion of petunidin derivatives and increased the proportion of peonidin derivatives leading to a decreased ratio between tri-hydroxylated and di-hydroxylated anthocyanins, which was suggested to be less chemically stable for winemaking purposes (Torres et al., 2020). Likewise, previous studies have reported an increase in the ratio between tri-hydroxylated and di-hydroxylated anthocyanins when grapevines were subjected to water deficits given the upregulation of the relevant anthocyanin biosynthetic genes (Castellarin et al., 2007; Martínez-Lüscher et al., 2014; Cook et al., 2015; Savoi et al., 2017). In addition, these forms were more persistent through hang time, making tri-hydroxylated flavonoids more abundant as maturity progressed (Brillante et al., 2017). Flavonol composition was modified by applied water amounts in 2020 growing, where proportions of myricetin and syringetin derivatives increased and quercetin and kaempferol derivatives decreased with 100% ETc. Given that quercetin and kaempferol are important antioxidants in red wines, this shift in the composition may impact the antioxidant properties of wine (Dabeek and Ventura-Marra, 2019; Torres et al., 2020). In previous work, it was reported that 100% ETc irrigation increased the net carbon assimilation and improved the grapevine water status, leading to higher soluble sugar and starch contents in leaves with the highest yields, and vegetative biomasses (Torres et al., 2021b). However, the greatest leaf area to fruit ratios measured in this treatment showed a clear sign of disproportionate leaf biomass growth, which presumably impacted berry metabolism. Thus, both studies highlighted the importance of management of water deficits to ensure grape berry composition optimization, improving water use sustainability by rewarding quality over quantity in arid and semiarid regions (Medrano et al., 2015; Romero-Azorín and García-García, 2020).
Reducing Environmental Costs Through Irrigation Management Optimization
Decreasing irrigation amounts increased AMF colonization in accordance with previous studies (Schreiner et al., 2007). The symbiotic relationship of AMF with grapevines provided several adaptive advantages, such as improved abiotic and biotic stress resistance, enhanced nutrient uptake, and grapevine growth (Trouvelot et al., 2015; Torres et al., 2018a). Previous research suggested that these effects might be related to the altered regulation of nutrient transport, cell wall-related, phenylpropanoid, and stilbene biosynthesis genes driven by AMF colonization (Bruisson et al., 2016; Balestrini et al., 2017). Additionally, it was recently reported that AMF may enhance the content of flavonoids in berries (Torres et al., 2019, 2021a), leading to improved berry composition and antioxidant properties in spite of the lack of effect on petiole nutrient contents (Torres et al., 2021a). However, vineyard management practices may affect the soil structure and the composition of the rhizosphere-living microbiota (Coller et al., 2019; Vink et al., 2021), as well as the microbiota associated with grapevine roots, which is mainly composed by Rhizophagus and Glomus genus (Schreiner, 2020), likely affecting the effectiveness of the symbiosis. The relationship between AMF and berry must δ13C suggested that productivity of high quality grapes could still be sustained in this region with less water input because the root system of the grapevines may perform more efficiently due to greater AMF colonization.
The totalWF measured in this study ranged between 484.3 and 1237.7 m3 ·tonne −1 across treatments and growing seasons, in accordance with previous studies assessing the WF of grapevine cultivation (Mekonnen and Hoekstra, 2011). This variation in the totalWF was related to the amount applied (determining blueWF) and the differences in precipitation between the two seasons (determining greenWF). Indeed, the previous research speculated that changes in temperature and precipitation may affect the proportional contribution of blue and green WF to the totalWF (Zotou and Tsihrintzis, 2017). Previous studies reported that vineyards accounted for a higher WF compared with other crops such as olives, wheat, and other fruit trees (Zotou and Tsihrintzis, 2017; D'Ambrosio et al., 2020). Wine grape growers require appropriate irrigation schedules that reduce blueWF and increase greenWF leading to a decreased totalWF for increasing sustainability of vineyards. Under our experimental conditions, 25% ETc strongly decreased the blueWF, however, this came with a dramatic increase in the grayWF component, which led to increased totalWF. Conversely, 100% ETc decreased totalWF to lower values than those reported in Zotou and Tsihrintzis (2017), presumably because of the differences of standard yields recorded in Mesogeia area (Greece), where the authors conducted their research, and Napa Valley, CA, USA where this study was performed. A recent study reported that the current values of blueWF and grayWF are unsustainable (D'Ambrosio et al., 2020). The actual runoff of the surface water is not sufficient to satisfy the irrigation requirements and/or dilute the pollutant load associated with the diffuse and point sources to reduce it below the maximum acceptable concentration (D'Ambrosio et al., 2020). These results highlighted that the management of natural resources, specifically water management, is paramount for the sustainability of the wine industry under future constraints (Schultz, 2016; Romero-Azorín and García-García, 2020; Wilson et al., 2020). Thus, our data suggested that values ranging between 600 and 1000 m3·tonne −1 of the totalWF may ensure a high iWUE of grapevines (Bota et al., 2016), optimum LAI, and profitable yields, which maintained the balance between vegetative and reproductive growths (Torres et al., 2021b). Nevertheless, it is noteworthy to address that WF assessment also presents some limitations given that the water consumed by an irrigated crop is often a mix of residual soil moisture from previous precipitation and irrigation events (residual green and blue WFs) and that the reference ET (ETo) is strongly dependent on the local climate (Fereres et al., 2017).
Conclusions
We aimed to evaluate the standard irrigation practices on grapevine WUE, berry flavonoid composition, vineyard WF, and AMF-grapevine symbiosis in two seasons with contrasting amounts of precipitation. Irrigation in grapevine vineyards mitigated the water scarcity when precipitation during the dormant season was not sufficient. This study provided field data supporting that despite the low rainfall recorded in one of the seasons, an increase in the amount of irrigation was not advised. Thus, irrigating grapevines with the replacement of the 50% ETc was still adequate in spite of the warming trends. In this treatment, berry composition was improved with increased contents of TSS, anthocyanins, and flavonols, and a stable flavonoid profile without an economic decrease in yield. In addition, with 50% ETc, the mycorrhizal symbiosis was not compromised and water resources were not highly impacted. Altogether, this study provides fundamental knowledge for viticulturists to design an appropriate irrigation schedule under the future constraints.
Data Availability Statement
The raw data supporting the conclusions of this article will be made available by the authors, without undue reservation.
Author Contributions
SK conceived and designed the study and acquired the funding. NT, RY, JM-L, and EK executed the trial. NT and RY collected and curated the data. All authors contributed to the writing of the manuscript and approved the final version.
Conflict of Interest
The authors declare that the research was conducted in the absence of any commercial or financial relationships that could be construed as a potential conflict of interest.
Publisher's Note
All claims expressed in this article are solely those of the authors and do not necessarily represent those of their affiliated organizations, or those of the publisher, the editors and the reviewers. Any product that may be evaluated in this article, or claim that may be made by its manufacturer, is not guaranteed or endorsed by the publisher.
Acknowledgments
The authors acknowledge the University of California Agriculture and Natural Resources for providing partial funding during the execution of the trial. A graduate stipend was provided to RY and EK.
References
Alam, S., Gebremichael, M., Li, R., Dozier, J., and Lettenmaier, D. P. (2019). Climate change impacts on groundwater storage in the Central Valley, California. Clim. Change 157, 387–406. doi: 10.1007/s10584-019-02585-5
Balestrini, R., Salvioli, A., Dal Molin, A., Novero, M., Gabelli, G., Paparelli, E., et al. (2017). Impact of an arbuscular mycorrhizal fungus versus a mixed microbial inoculum on the transcriptome reprogramming of grapevine roots. Mycorrhiza 27, 417–430. doi: 10.1007/s00572-016-0754-8
Bates, B., Kundzewicz, Z. W., Wu, S., and Palutikof, J. (2008). “Climate change and water,” in Technical Paper of the Intergovernmental Panel on Climate Change, IPCC Secretariat, Geneva, 210.
Bchir, A., Escalona, J. M., Galléc, A., Hernández-Montes, E., Tortosa, I., Brahama, M., et al. (2016). Carbon isotope discrimination (δ13C) as an indicator of vine water status and water use efficiency (WUE): Looking for the most representative sample and sampling time. Agric. Water Manage. 167, 11–20. doi: 10.1016/j.agwat.2015.12.018
Bota, J., Tomás, M., Flexas, J., Medrano, H., and Escalona, J. M. (2016). Differences among grapevine cultivars in their stomatal behavior and water use efficiency under progressive water stress. Agric. Water Manag. 164 (Part 1), 91–99. doi: 10.1016/j.agwat.2015.07.016
Brillante, L., Martínez-Lüscher, J., and Kurtural, S.K. (2018). Applied water and mechanical canopy management affect berry and wine phenolic and aroma composition of grapevine (Vitis vinifera L., cv. Syrah) in Central California. Sci. Hortic. 227, 261–271. doi: 10.1016/j.scienta.2017.09.048
Brillante, L., Martínez-Lüscher, J., Yu, R., and Kurtural, S. K. (2020). Carbon isotope discrimination (δ13C) of grape musts is a reliable tool for zoning and the physiological ground-truthing of sensor maps in precision viticulture. Front. Environ. Sci. 8:561477. doi: 10.3389/fenvs.2020.561477
Brillante, L., Martínez-Lüscher, J., Yu, R., Plank, C. M., Sanchez, L., Bates, T. L., et al. (2017). Assessing spatial variability of grape skin flavonoids at the vineyard scale based on plant water status mapping. J. Agric. Food Chem. 65, 5255–5265. doi: 10.1021/acs.jafc.7b01749
Bruisson, S., Maillot, P., Schellenbaum, P., Walter, B., Gindro, K., and Deglène-Benbrahim, L. (2016). Arbuscular mycorrhizal symbiosis stimulates key genes of the phenylpropanoid biosynthesis and stilbenoid production in grapevine leaves in response to downy mildew and grey mould infection. Phytochemistry 131, 92–99. doi: 10.1016/j.phytochem.2016.09.002
Castellarin, S. D., Pfeiffer, A., Sivilotti, P., Degan, M., Peterlunger, E., and Di Gaspero, G. (2007). Transcriptional regulation of anthocyanin biosynthesis in ripening fruits of grapevine under seasonal water deficit. Plant Cell Environ. 30, 1381–1399. doi: 10.1111/j.1365-3040.2007.01716.x
CDFA California Department of Food Agriculture. (2020). About Fertilizer. Available online at: https://www.cdfa.ca.gov/is/ffldrs/about_fertilizer.html. (accessed January 15, 2021).
CDWR California Department of Water Resources. (2019). California water plan. Update 2018. Available online at: https://water.ca.gov/-/media/DWR-Website/Web-Pages/Programs/California-Water-Plan/Docs/Update2018/Final/California-Water-Plan-Update-2018.pdf (accessed July 25, 2021).
Chaves, M. M., Zarrouk, O., Francisco, R., Costa, J. M., Santos, T., Regalado, A. P., et al. (2010). Grapevine under deficit irrigation: hints from physiological and molecular data. Ann. Bot. 105, 661–676. doi: 10.1093/aob/mcq030
CIMIS California Department of Water Resources (2020). Weather Observation Data: University of California at Davis. Available online at: www.cimis.water.ca.gov (accessed October 15, 2020).
Coller, E., Cestaro, A., Zanzotti, R., Bertoldi, D., Pindo, M., Larger, S., et al. (2019). Microbiome of vineyard soils is shaped by geography and management. Microbiome 7, 140. doi: 10.1186/s40168-019-0758-7
Cominelli, E., Galbiati, M., Tonelli, C., and Bowler, C. (2009). Water: the invisible problem, access to fresh water is considered to be a universal and free human right, but dwindling resources and a burgeoning population are increasing its economic Value. EMBO Rep. 10, 671–676. doi: 10.1038/embor.2009.148
Compant, S., van der Heijden, M. G. A., and Sessitsch, A. (2010). Climate change effects on beneficial plant-microorganism interactions. FEMS Microbiol. Ecol. 73, 197–214. doi: 10.1111/j.1574-6941.2010.00900.x
Cook, M. G., Zhang, Y., Nelson, C. J., Gambetta, G., Kennedy, J. A., and Kurtural, S. K. (2015). Anthocyanin composition of merlot is ameliorated by light microclimate and irrigation in central California. Am. J. Enol. Vitic. 66, 266 LP-278. doi: 10.5344/ajev.2015.15006
Costa, J. M., Vaz, M., Escalona, J., Egipto, R., Lopes, C., Medrano, H., et al. (2016). Modern viticulture in southern Europe: vulnerabilities and strategies for adaptation to water scarcity. Agric. Water Manage. 164, 5–18. doi: 10.1016/j.agwat.2015.08.021
da Silva, J. R., Pereira-Rodrigues, W., Souza-Ferreira, L., de Paula-Bernado, W., Sousa-Paixão, J., Patterson, A. E., et al. (2018). Deficit irrigation and transparent plastic covers can save water and improve grapevine cultivation in the tropics. Agric. Water Manage. 202, 66–80. doi: 10.1016/j.agwat.2018.02.013
Dabeek, W. M., and Ventura-Marra, M. (2019). Dietary quercetin and kaempferol: bioavailability and potential cardiovascular-related bioactivity in humans. Nutrients 11:2288. doi: 10.3390/nu11102288
D'Ambrosio, E., Gentile, F., and De Girolamo, A. M. (2020). Assessing the sustainability in water use at the basin scale through water footprint indicators. J. Clean. Prod. 244:118847. doi: 10.1016/j.jclepro.2019.118847
de Mendiburu, M. F. (2016). Package ‘agricolae.’ Statistical Procedures for Agricultural Research. Version 1.3–0.
Fereres, E., Villalobos, F. J., Orgaz, F., Minguez, M. I., van Halsema, G., and Perry, C. J. (2017). Commentary: On the water footprint as an indicator of water use in food production. Irrig. Sci. 35, 83–85. doi: 10.1007/s00271-017-0535-y
Fraga, H., García de Cortázar-Atauri, I., and Santos, J. A. (2018). Viticultural irrigation demands under climate change scenarios in Portugal. Agric. Water Manage. 196, 66–74. doi: 10.1016/j.agwat.2017.10.023
Gambetta, G. A., Herrera, J. C., Dayer, S., Feng, Q., Hochberg, U., and Castellarin, S. D. (2020). The physiology of drought stress in grapevine: towards an integrative definition of drought tolerance. J. Exp. Bot. 71, 4658–4676. doi: 10.1093/jxb/eraa245
Gaudillère, J.-P., Van Leeuwen, C., and Ollat, N. (2002). Carbon isotope composition of sugars in grapevine, an integrated indicator of vineyard water status. J. Exp. Bot. 53, 757–763. doi: 10.1093/jexbot/53.369.757
Hoekstra, A. Y., Chapagain, A. K., Aldaya, M. M., and Mekonnen, M. M. (2011). The Water Footprint Assessment Amnual-Setting the Global Standard. Earthscan, London, WATER Footprint Network.
Intrigliolo, D. S., and Castel, J. R. (2008). Effects of irrigation on the performance of grapevine cv. Tempranillo in Requena, Spain. Am. J. Enol. Vitic. 59, 30–38.
IPCC, Hoegh-Guldberg, O., Jacob, D., Taylor, M., Bindi, M., Brown, S., Camilloni, I., et al. (2018). “Impacts of 1.5°C Global warming on natural and human systems,” in Global warming of 1.5°C, In Press An IPCC Special Report on the impacts of global warming of 1.5°C above pre-industrial levels and related global greenhouse gas emission pathways, in the context of strengthening the global response to the threat of climate change, sustainable development, and efforts to eradicate poverty eds V. Masson-Delmotte., P. Zhai., H.-O. Pörtner., D. Roberts., J. Skea., P.R. Shukla, et al. (IPCC).
Keller, M., Romero, P., Gohil, H., Smithyman, R. P., Riley, W. R., Casassa, L. F., et al. (2016). Deficit irrigation alters grapevine growth, physiology, and fruit microclimate. Am. J. Enol. Vitic. 67, 426–435. doi: 10.5344/ajev.2016.16032
Koske, R. E., and Gemma, J. N. (1989). A modified procedure for staining roots to detect VA mycorrhizas. Mycol. Res. 92, 486–488. doi: 10.1016/s0953-7562(89)80195-9
Martínez-Lüscher, J., Brillante, L., and Kurtural, S. K. (2019). Flavonol profile is a reliable indicator to assess canopy architecture and the exposure of red wine grapes to solar radiation. Front. Plant Sci. 10:10. doi: 10.3389/fpls.2019.00010
Martínez-Lüscher, J., Chen, C. C. L., Brillante, L., and Kurtural, S. K. (2017). Partial solar radiation exclusion with color shade nets reduces the degradation of organic acids and flavonoids of grape berry (Vitis vinifera L.). J. Agric. Food Chem. 65, 10693–10702. doi: 10.1021/acs.jafc.7b04163
Martínez-Lüscher, J., Chen, C. C. L., Brillante, L., and Kurtural, S. K. (2020). Mitigating heat wave and exposure damage to “Cabernet sauvignon” wine grape with partial shading under two irrigation amounts. Front. Plant Sci. 11, 579192. doi: 10.3389/fpls.2020.579192
Martínez-Lüscher, J., Sanchez-Dias, M., Delrot, S., Aguirreolea, J., Pascual, I., and Gomès, E. (2014). Ultraviolet-B radiation and water deficit interact to alter flavonol and anthocyanin profiles in grapevine berries through transcriptomic regulation. Plant Cell Physiol. 55, 1925–1936. doi: 10.1093/pcp/pcu121
Medrano, H., Tomás, M., Martorell, S., Escalona, J. M., Pou, A., Fuentes, S., et al. (2015). Improving water use efficiency of vineyards in semi-arid regions. a review. Agron. Sustain. Dev. 35, 499–517. doi: 10.1007/s13593-014-0280-z
Mekonnen, M. M., and Hoekstra, A. Y. (2011). National Water Footprint Accounts: the Green, Blue and Grey Water Footprint of Production and Consumption, Value of Water Research Report Series No. 50, UNESCO-IHE, Delft, the Netherlands.
Mosedale, J. R., Abernethy, K. E., Smart, R. E., Wilson, R. J., and Maclean, I. M. D. (2016). Climate change impacts and adaptive strategies: lessons from the grapevine. Glob. Change Biol. 22, 3814–3828. doi: 10.1111/gcb.13406
Munitz, S., Netzer, Y., and Schwartz, A. (2020). Sustained and regulated deficit irrigation of field-grown Merlot grapevines. Aust. J. Grape Wine Res. 23, 87–94. doi: 10.1111/ajgw.12241
Neethling, E., Petitjean, T., Quénol, H., and Barbeau, G. (2017). Assessing local climate vulnerability and winegrowers' adaptive processes in the context of climate change. Mitig. Adapt. Strat. Global Change 22, 777–803. doi: 10.1007/s11027-015-9698-0
Resco, P., Iglesias, A., Bardají, I., and Sotés, V. (2016). Exploring adaptation choices for grapevine regions in Spain. Reg. Environ. Change 16, 979–993. doi: 10.1007/s10113-015-0811-4
Romero-Azorín, P., and García-García, J. (2020). The Productive, economic, and social efficiency of vineyards using combined drought-tolerant rootstocks and efficient low water volume deficit irrigation techniques under Mediterranean semiarid conditions. Sustainability 12:1930. doi: 10.3390/su12051930
Savoi, S., Wong, D. C. J., Degu, A., Herrera, J. C., Bucchetti, B., Peterlunger, E., et al. (2017). Multi-Omics and integrated network analyses reveal new insights into the systems relationships between metabolites, structural genes, and transcriptional regulators in developing grape berries (Vitis vinifera L.) exposed to water deficit. Front. Plant Sci. 8:1124. doi: 10.3389/fpls.2017.01124
Schreiner, R. P. (2020). Depth structures the community of arbuscular mycorrhizal fungi amplified from grapevine (Vitis vinifera L.) roots. Mycorrhiza 30, 149–160. doi: 10.1007/s00572-020-00930-6
Schreiner, R. P., Tarara, J. M., and Smithyman, R. P. (2007). Deficit irrigation promotes arbuscular colonization of fine roots by mycorrhizal fungi in grapevines (Vitis vinifera L.) in an arid climate. Mycorrhiza 17, 551–562. doi: 10.1007/s00572-007-0128-3
Schultz, H. R. (2016). Global climate change, sustainability, and some challenges for grape and wine production. J. Wine Econ. 11, 181–200. doi: 10.1017/jwe.2015.31
Spangenberg, J. E., and Zufferey, V. (2018). Changes in soil water availability in vineyards can be traced by the carbon and nitrogen isotope composition of dried wines. Sci. Total Environ. 635, 178–187. doi: 10.1016/j.scitotenv.2018.04.078
Terry, D. B., and Kurtural, S. K. (2011). Achieving vine balance of Syrah with mechanical canopy management and regulated deficit irrigation. Am. J. Enol. Vitic. 62, 426–437. doi: 10.5344/ajev.2011.11022
Tomás, M., Medrano, H., Escalona, J. M., Martorell, S., Pou, A., Ribas-Carbó, M., and Flexas, J. (2014). Variability of water use efficiency in grapevines. Environ. Exp. Bot. 103, 148–157. doi: 10.1016/j.envexpbot.2013.09.003
Torres, N., Goicoechea, N., and Antolín, M. C. (2018a). Arbuscular Mycorrhizal Symbiosis as a promising resource for improving berry quality in grapevines under changing environments. Front. Plant Sci. 9:897. doi: 10.3389/fpls.2018.00897
Torres, N., Goicoechea, N., and Antolín, M. C. (2018b). Influence of irrigation strategy and mycorrhizal inoculation on fruit quality in different clones of Tempranillo grown under elevated temperatures. Agric. Water Manag. 202, 285–298. doi: 10.1016/j.agwat.2017.12.004
Torres, N., Goicoechea, N., Morales, F., and Antolín, M. C. (2016). Berry quality and antioxidant properties in Vitis vinifera cv. Tempranillo as affected by clonal variability, mycorrhizal inoculation and temperature. Crop Past. Sci. 67, 961–977. doi: 10.1071/CP16038
Torres, N., Hilbert, G., Antolín, M. C., and Goicoechea, N. (2019). Aminoacids and flavonoid profiling in Tempranillo berries can be modulated by the Arbuscular mycorrhizal fungi. Plants. 8:400. doi: 10.3390/plants8100400
Torres, N., Martínez-Lüscher, J., Porte, E., Yu, R., and Kurtural, S. K. (2020). Impacts of leaf removal and shoot thinning on cumulative daily light intensity and thermal time and their cascading effects of grapevine (Vitis vinifera L.) berry and wine chemistry in warm climates. Food Chem. 343:128447. doi: 10.1016/j.foodchem.2020.128447
Torres, N., Yu, R., and Kurtural, S. K. (2021a). Arbuscular mycorrhizal fungi inoculation and applied water amounts modulate the response of young grapevines to mild water stress in a hyper-arid season. Front. Plant Sci. 11:622209. doi: 10.3389/fpls.2020.622209
Torres, N., Yu, R., Martínez-Lüscher, J., Kostaki, E., and Kurtural, S. K. (2021b). Application of fractions of crop evapotranspiration affects carbon partitioning of grapevine differentially in a hot climate. Front. Plant Sci. 12:633600. doi: 10.3389/fpls.2021.633600
Torres, N., Zamarreño, A., Goicoechea, N., and Antolín, M. C. (2018c). Changes in ABA conjugation/catabolism could account for the effects of AMF inoculation on Tempranillo (Vitis vinifera L.) fruit quality under climate change scenarios. Plant Sci. 274, 383–393. doi: 10.1016/j.plantsci.2018.06.009
Trouvelot, S., Bonneau, L., Redecker, D., van Tuinen, D., Adrian, M., and Wipf, D. (2015). Arbuscular mycorrhiza symbiosis in viticulture: a review. Agron. Sustain. Dev. 35, 1449–1467. doi: 10.1007/s13593-015-0329-7
UC Davis (2016). California Nitrogen Assessment Shows the State of the Science on Nitrogen use and Pollution California Paves the Way for Reconciling Agriculture and the Environment. Available online at: https://climatechange.ucdavis.edu/news/first-state-level-nitrogen-assessment-shows-state-science-nitrogen-use-and-pollution/#:~:text=Much%20excess%20nitrogen%20from%20crops,is%20lost%20as%20air%20pollution. (accessedJanuary10, 2020).
van Leeuwen, C., Destrac-Irvine, A., Dubernet, M., Duchêne, E., Gowdy, M., Marguerit, E., et al. (2019). An update on the impact of climate change in viticulture and potential adaptations. Agronomy 9:514. doi: 10.3390/agronomy9090514
Venios, X., Korkas, E., Nisiotou, A., and Banilas, G. (2020). Grapevine responses to heat stress and global warming. Plants 9:1754. doi: 10.3390/plants9121754
Vink, S. N., Chrysargyris, A., Tzortzakis, N., and Falcao-Salles, J. (2021). Bacterial community dynamics varies with soil management and irrigation practices in grapevines (Vitis vinifera L.). Agric., Ecosyst. Environ,. Appl. Soil Ecol. 158:103807. doi: 10.1016/j.apsoil.2020.103807
Williams, L. E. (2014). Determination of evapotranspiration and crop coefficients for a Chardonnay vineyard located in a cool climate. Am. J. Enol. Vitic. 65, 159–169. doi: 10.5344/ajev.2014.12104
Wilson, T. G., Kustas W, P., Alfieri, J. G., Anderson, M,C, Gaoa, F., Prueger, J. H., et al. (2020). Relationships between soil water content, evapotranspiration, and irrigation measurements in a California drip-irrigated Pinot noir vineyard. Agric. Water Manag. 237:106186. doi: 10.1016/j.agwat.2020.106186
Yu, R., Brillante, L., Martinez-Luscher, J., and Kurtural, S. K. (2020). Spatial variability of soil and plant water status and their cascading effects on grapevine physiology are linked to berry and wine chemistry. Front. Plant Sci. 11:790. doi: 10.3389/fpls.2020.00790
Yu, R., Zaccaria, D., Kisekka, I., and Kurtural, S. K. (2021). Soil apparent electrical conductivity and must carbon isotope ratio provide indication of plant water status in wine grape vineyards. Precision Agric. 9787. doi: 10.1007/s11119-021-09787-x
Keywords: arbuscular mycorrhizal fungi, berry quality, climate change, deficit irrigation, water footprint, water scarcity, water use efficiency
Citation: Torres N, Yu R, Martínez-Lüscher J, Kostaki E and Kurtural SK (2021) Effects of Irrigation at Different Fractions of Crop Evapotranspiration on Water Productivity and Flavonoid Composition of Cabernet Sauvignon Grapevine. Front. Plant Sci. 12:712622. doi: 10.3389/fpls.2021.712622
Received: 20 May 2021; Accepted: 06 August 2021;
Published: 01 September 2021.
Edited by:
Sergio Tombesi, Catholic University of the Sacred Heart, ItalyReviewed by:
Erna Blancquaert, Stellenbosch University, South AfricaRupam Kapoor, University of Delhi, India
Copyright © 2021 Torres, Yu, Martínez-Lüscher, Kostaki and Kurtural. This is an open-access article distributed under the terms of the Creative Commons Attribution License (CC BY). The use, distribution or reproduction in other forums is permitted, provided the original author(s) and the copyright owner(s) are credited and that the original publication in this journal is cited, in accordance with accepted academic practice. No use, distribution or reproduction is permitted which does not comply with these terms.
*Correspondence: Sahap Kaan Kurtural, skkurtural@ucdavis.edu