- Ecology and Conservation Biology, Institute of Plant Sciences, University of Regensburg, Regensburg, Germany
The correct choice of in vitro pollen germination media (PGM) is crucial in basic and applied pollen research. However, the methodological gaps (e.g., strong focus of current research on model species and cultivated plants along with the lack of general rules for developing a PGM) makes experimenting with pollen difficult. We closed these gaps by compiling a compendium of optimized in vitro PGM recipes from more than 1800 articles published in English, German, and Russian from 1926 to 2019. The compendium includes 1572 PGM recipes successfully used to germinate pollen grains or produce pollen tubes in 816 species representing 412 genera and 114 families (both monocots and dicots). Among the 110 components recorded from the different PGM recipes, sucrose (89% of species), H3BO3 (77%), Ca2+ (59%), Mg2+ (44%), and K+ (39%) were the most commonly used PGM components. PGM pH was reported in 35% of all studies reviewed. Also, we identified some general rules for creating PGM for various groups of species differing in area of research (wild and cultivated species), phylogenetic relatedness (angiosperms vs. gymnosperms, dicots vs. monocots), pollen physiology (bi- and tri-cellular), biochemistry (starchy vs. starchless pollen grains), and stigma properties (dry vs. wet), and compared the component requirements. Sucrose, calcium, and magnesium concentrations were significantly different across most categories indicating that pollen sensitivity to sugar and mineral requirements in PGM is highly group-specific and should be accounted for when composing new PGM. This compendium is an important data resource on PGM and can facilitate future pollen research.
Introduction
Pollen, the male gametophyte, is an evolutionary development in higher plants that ensures successful genetic exchange, establishment, and survival of the species (Ashman et al., 2004; Pacini and Dolferus, 2016). Because of their crucial role in successful seed development (Shivanna and Rangaswamy, 1992; Dafni and Firmage, 2000; Rosbakh et al., 2018), pollen germination (PG), and pollen tube growth (PTG) have been in the focus of many studies ranging from research on physiological and biochemical aspects of these processes (Taylor and Hepler, 1997; Wang et al., 2010; Williams and Reese, 2019) to large-scale screenings of pollen abiotic stress-tolerance (Kakani et al., 2005; Rosbakh et al., 2018). Additionally, pollen is an excellent model system for studying a number of basic processes including plant cell growth, cell wall synthesis, intracellular transport, and cell-cell interaction (Johnson-Brousseau and McCormick, 2004; Boavida and McCormick, 2007). That is why pollen remains one of the most attractive objects in plant research.
In basic and applied research, pollen functioning has been studied with the help of two approaches, in vivo and in vitro. In vivo studies are carried out directly at the stigmatic surface in the natural state, while in vitro approaches rely on a culture medium that simulates conditions of the style-stigma (Rodriguez-Enriquez et al., 2013). The advantage of the in vivo methods is that they consider all natural conditions pollen grains experience on stigma (Dawkins and Owens, 1993; Albert et al., 2018). However, such methods have sometimes proved difficult (Shivanna and Rangaswamy, 1992). Partly, this is due to the involvement of the pistillate tissue that interacts with the growing pollen tubes thereby affecting physiological and biochemical investigations (Shivanna and Rangaswamy, 1992; Zheng et al., 2019; Xu et al., 2020). Moreover, the complex and labor-intensive nature of the in vivo approach (e.g., maintenance of pistil tissue viability and post-experimental sample processing) limits its applicability in large-scale research, such as breeding programs (Kakani et al., 2005) or multispecies ecological screenings (Rosbakh et al., 2018). The comparatively technically simple in vitro approach, which is based on the ability of pollen to germinate and grow without the pistillate tissue, solves this problem making it possible to conduct comprehensive pollen research (Taylor and Hepler, 1997). Although being sometimes criticized for inaccurate replication of the biological context (Rodriguez-Enriquez et al., 2013), the in vitro approach is generally preferred because it provides results comparable to in vivo studies (Sulusoglu and Cavusoglu, 2014; Jayaprakash, 2018; Luo et al., 2020). It is also easier to detect alterations in PG or PTG performance using in vitro approaches (Procissi et al., 2003; Steinebrunner et al., 2003; Cole et al., 2005; Hashida et al., 2007) since the parameters can easily be tested for defects which is difficult to perform in vivo. Notably, in vitro PG rates are considered the best estimate of pollen viability in vivo (Shivanna et al., 1991; Stone et al., 1995).
Typically, an in vitro PG protocol includes cultivating of fresh or stored pollen grains in/on a germination media contained within a hanging drop/well or on a membrane support (Conner, 2011; Soares et al., 2013; Jayaprakash, 2018). In all such protocols, the correct choice of pollen germination media (PGM) remains the most important part, as pollen is highly sensitive to the PGM composition (Dafni et al., 2005). To begin with, pollen of several species requires either liquid (Hoffmann et al., 1990; Golan-Goldhirsh et al., 1991; Montaner et al., 2003) or solid (e.g., with addition of agar) medium (Shivanna and Sawhney, 1995; Jayaprakash, 2018) to germinate, while others can germinate in/on both solid and liquid media (Bilderback, 1981; Boavida and McCormick, 2007). Additionally, most pollen grains need a carbohydrate source to germinate successfully, and sucrose solution is generally used (Montaner et al., 2003; Silva et al., 2016; Lagera et al., 2017). In some cases, other sugars and sugar derivatives such as lactose, maltose, raffinose, and fructose among others, are also used (Shaoling et al., 2005; Hirsche et al., 2017; Lagera et al., 2017; Impe et al., 2019). Furthermore, several inorganic compounds affect in vitro PG with boron being one of the most important element for most species (Brewbaker and Kwack, 1963; Wang et al., 2003; Yao and Zhao, 2004; Fang et al., 2016). Besides boron, minerals such as calcium, magnesium, potassium are also known to have stimulatory effects on PG (Brewbaker and Kwack, 1963; Čapková-Balatková et al., 1980; Song et al., 2009; Biswas and Mondal, 2014; Jayaprakash, 2018). The different compounds in the medium affect the pH that must therefore be adjusted to species-specific values, to allow for optimal conditions for pollen to germinate (Tupý and Řhová, 1984; Fricker et al., 1997; Fan, 2001). Trisaminomethane (Tris) and 2-ethanesulfonic acid (MES) belong to the buffers that has been often used to maintain a constant pH in the medium (Tupý and Řhová, 1984; Holdaway-Clarke et al., 2003). Further, several other substances such as hormones, vitamins, buffers, proteins, lipids, antibiotics, enzymes, plant, and animal extracts are sometimes added to increase the percentage of PG and to accelerate the rate of PTG (Vasil, 1960; de Bruyn, 1966; Boavida and McCormick, 2007; Jayaprakash, 2018). Finally, different plant groups are also suggested to have different PGM requirements (e.g., plants with binucleate vs. trinucleate pollen grains (Hoekstra, 1979; Bergamini and Mulcahy, 1988; Zhang et al., 1997; Gibernau et al., 2003); those with dry vs. wet stigmas (Boavida and McCormick, 2007; Rodriguez-Enriquez et al., 2013); angiosperms vs. gymnosperms (Paoletti and Bellani, 1990); monocots vs. dicots (Jayaprakash, 2018); and in plants with starchy vs. starchless pollen grains (Bellani et al., 1985; Franchi et al., 2007).
Despite the fact that several PGM are widely available (Brewbaker and Kwack, 1963; Hong-Qi and Croes, 1982; Roberts et al., 1983; Rodriguez-Enriquez et al., 2013), experimentation on PG in vitro is still challenged by several problems. Firstly, information on PGM requirements for a species in question is usually extremely scattered in works published in very different journals and/or years. Secondly, although numerous protocols describe methods to induce PG, they are not applicable to all species as they are strongly biased either to model species, such as Arabidopsis thaliana (Boavida and McCormick, 2007; Rodriguez-Enriquez et al., 2013) or domesticated plant species, their cultivars and wild relatives (Roberts et al., 1983; Cheng and Mcomb, 1992; Jayaprakash et al., 2018). In contrast, PG studies for any given wild species are limited (Mortenson et al., 1964; Fernando et al., 2001; Sorkheh et al., 2011). Thirdly, it is not clear whether the available protocols can be generalized to create some general rules for developing a PGM for a single species or various species groups especially those that have not yet been studied.
Here, we close these gaps by compiling a compendium of in vitro PGM recipes available in the published literature. Specifically, we first provide a list of optimized media successfully used to germinate pollen grains and/or produce pollen tubes in different species. In addition, we identify the key PGM components required for various groups of species differing in area of research (wild and cultivated species), phylogenetic relatedness (angiosperms vs. gymnosperms, dicots vs. monocots), pollen physiology (bi- and tri-cellular), biochemistry (starchy vs. starchless pollen grains), and stigma properties (dry vs. wet) that will help to create and/or optimize PGM recipes for the species, for which such information is not available.
Materials and Methods
To extract available information on PGM composition, we first reviewed all studies published from 1926 to 2019 that included keywords ‘‘pollen’’, ‘‘germination,’’ and ‘‘media’’ either in the title or abstract. The literature was searched using the Web of Science database1 with the searched databases including, “Web of Science Core Collection,” “KCI-Korean journal database,” “Medline,” “Russian science citation index,” and “SciELO Citation.” Only publications in English, Russian, and German were considered. The search resulted in 1800 studies.
In the second step, we sorted out the publications that were accessible in a digital form and/or contained information about PGM composition with an estimate of its efficacy to stimulate PG or PTG in the abstract or full text. From each of the 675 studies that fulfilled the selection criteria, we extracted the author name(s), year of publication, full literature reference and whether the publication was available in digital form. For each species/variety studied in the selected publications, we further extracted information on the PGM composition including the ingredients used and their concentrations. When several PGMs were used in a publication, only PGM reported to be most effective, i.e., resulting in maximum PG, longest pollen tubes, or minimum pollen bursting obtained, were extracted. If component concentrations of effective PGMs were given as a range, average values of such ranges were considered.
Additionally, we included unpublished data on PGM composition for 104 Central European plant species collected during PG studies at the University of Regensburg from 2014 to 2020 (S. Rosbakh unpublished).
Data Analysis
For the statistical analysis, all entries in the data set were standardized by recalculating sucrose and agar concentrations to percentages and the rest of the ingredient concentrations to millimolar (mM). The species taxonomy was standardized against the “Plant List” (2013).
In order to infer group-specific concentrations of PGM components, we classified all species present in the data set into several categories: (1) wild vs. cultivated species, (2) angiosperms vs. gymnosperms, (3) dicots vs. monocots plants, (4) bi- vs. tri-nucleate pollen (Brewbaker, 1967), (5) starchy vs. starchless pollen (Baker and Baker, 1979), and (6) dry vs. wet stigmas (Heslop-Harrison and Shivanna, 1977). This analysis was carried out only with entries that included information on the most frequent PGM components (agar, sucrose, H3BO3, Ca2+, Mg2+, K+, and pH). The variation in the PGM ingredients in the species groups were visualized with the help of ggplot2 package (Wickham, 2016) in the R software version 4.0.0 (R Core Team, 2020). A Kruskal–Wallis non-parametric test at a 95% confidence interval was performed to test for significant differences among PGM requirements in the different plant groups.
Results and Discussion
The Compendium of PGM for Pollen Research
The final version of the PGM compendium (Supplementary Appendix 1) is composed of 1572 recipes successfully used to germinate pollen grains and/or produce pollen tubes in 816 species representing 412 genera and 114 families (both monocots and dicots). All together, we recorded 110 components from the different in vitro PGM, used under varying conditions and concentrations. Out of 816 species, 51% (420) and 32% (260) germinated in liquid or solidified (mainly agar with concentrations 0.5–1.5%) media, respectively, while 17% (136) germinated in both solid and in liquid media. The liquid media is preferred when pollen needs to reach a certain turgescence level to germinate (Martin and Brewbaker, 1971; Montaner et al., 2003), but also because water serves other hydrolytic and synthetic reactions (Brink, 1924). However, in some species, cultivation in liquid media leads to pollen bursting, due to quick hydration thus solid (e.g., agarified) media is required (Burke, 2002; Sun et al., 2008; Jayaprakash, 2018). In such media, agar also enables incorporation of sucrose or other stimulants, helps to maintain relative humidity at constant levels and provides appropriate aerobic conditions for adequate PG (Linskens and Stanley, 1974).
Among other components, sucrose (89% of species), H3BO3 (77%), Ca2+ (59%), Mg2+ (44%), and K+ (39%) were the most frequently used while enzymes, vitamins, and amino acids were less common (1, 6, and 1%, respectively); PGM pH values were reported in 35% of all studies reviewed. The high frequency of the former five components in the extracted PGM correspond to other studies on PGM (e.g., Imani, 2012; Jayaprakash, 2018; Wani et al., 2020) and might reflect the wide application of the classic Brewbaker and Kwack PGM (Brewbaker and Kwack, 1963) in pollen research. As for the roles of the most frequent PGM components, sucrose serves as an effective energy source and an osmoticum for PG in vitro (Heslop-Harrison and Heslop-Harrison, 1992; Rodriguez-Enriquez et al., 2013; Selinski and Scheibe, 2014; Reinders, 2016). Pollen bursting and failure to germinate in vitro is often associated with inadequate sucrose concentrations (Baloch et al., 2001). The mineral elements boron and calcium have also been found to play several critical regulatory and structural functions in PG. For instance, boron is required for the pollen wall structure, absorption, and metabolism of sugars by forming a sugar–borate complex, and increases oxygen uptake for metabolism (Vasil, 1960; Sidhu and Malik, 1986; Yang and Li, 1999; Wang et al., 2003). Boron deficiency in PGM, often leads to pollen tube bursting or failed PTG (Cheng and Rerkasem, 1993; Fang et al., 2019).
Similarly, calcium is a central regulator, providing various governing roles in the initiation and regulation of PG through ionic balance and cell signaling (Brewbaker and Kwack, 1963; Steinhorst and Kudla, 2013). Calcium in in vitro PGM has proved essential in pollen tip growth (Steinhorst and Kudla, 2013) with its deficiency leading to morphological abnormalities such as coiling and tip swelling (Shivanna and Rangaswamy, 1992; Taylor and Hepler, 1997). Other combined roles of boron and calcium (e.g., in sugar synthesis and accumulation) have been emphasized in different studies (Shu-juan, 2010; Muengkaew et al., 2017). Elements such as magnesium and potassium were also frequently used ingredients in PGM because of their role in cellular physiological processes, such as osmotic balance and membrane potential (Čapková-Balatková et al., 1980; Taylor and Hepler, 1997; Song et al., 2009; Wu et al., 2011). They also improve the germination and elongation of pollen tubes by enhancing the calcium effect (Brewbaker and Kwack, 1963). The pH of the in vitro germination medium is an important factor controlling PG and pollen tube development in different plant species (Bellani et al., 1997; Munzuroglu et al., 2003; Burke et al., 2004; Zaman, 2011; Fragallah et al., 2019), because it affects physiological processes through enzyme activation or inhibition (Fan, 2001; Bisswanger, 2014). Finally, the comparatively low percentages of PGM components including enzymes, vitamins, and amino acids can be explained by the fact that pollen grains are rich in these components and therefore do not generally require exogenous supply of such substances in the in vitro PGM (Campos et al., 2008; Komosinska-Vassev et al., 2015).
PGM Requirements in Different Plant Groups
The median concentration requirements for the frequently used ingredients varied among the plant groups with different degrees of magnitude. Because data distribution in the majority of the plant groups was skewed, we preferred median values over mean values when discussing the results.
To begin with, successful PG and PTG was observed mainly in a liquid PGM (agar = 0%, Table 1) regardless of the characteristics of the tested species, except for a few cases such as in Poaceae where agar was required for some special needs (see below). The strong dominance of liquid PGM recipes in our compendium could be also explained by high popularity of the “hanging drop” cultivation approach in pollen research (Stanley and Linskens, 1974). Furthermore, pollen of the plant families strongly represented in the compendium (e.g., Solanaceae 10% and Rosaceae 9%), prefer to germinate in liquid medium. Finally, researchers might prefer liquid PGMs over the solid ones because they are slightly more practical (i.e., no need to add agar every time you want to germinate pollen).
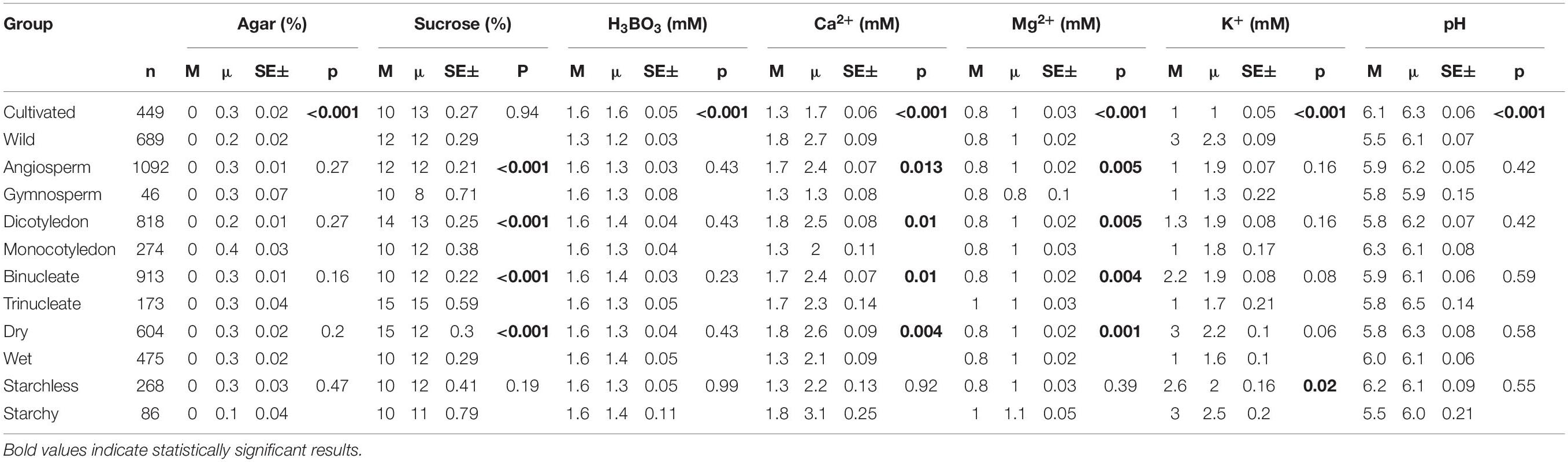
Table 1. The median (M), mean (μ) concentrations, standard error (SE), and p-values of the most frequently used ingredients in in vitro pollen germination media across the categories.
Among other components considered, pollen requirements for H3BO3 (1.6 mM) and pH (range 5.5–6.3) in PGM were similar across all plant groups, except for species with different cultivation status (CW; Table 1). The former being similar could be attributed to the broad usage of Brewbaker–Kwack protocol in our dataset, whose boric acid concentration has proved to be right in many studies (Chauhan et al., 1987; Kumari et al., 2015; Souza et al., 2017). Correspondingly, boric acid is the least variable component in PGMs, markedly affecting PG and PTG (Stanley, 1971) with small deviations making it either inadequate (Cheng and Rerkasem, 1993; Fang et al., 2019) or toxic (Fang et al., 2016). Similarly, slight or drastic changes in the pH media can affect the pollen cytoplasmic pH resulting in slow pollen growth or total growth inhibition (Tupý and Řhová, 1984; Fricker et al., 1997; Fan, 2001). This suggests that boron has equal importance in PG and PTG regardless of the pollen anatomy, morphology, and physiology.
As for the remaining most frequently used PGM components, Ca2+ and Mg2+ were significantly different in all categories except for species with starch vs. starchless pollen (SS). Sucrose concentration was also significantly different in all groups compared, except for SS and CW (Table 1). Notably, although Mg2+ was statistically significant in all categories (except SS), the differences in both median and mean values were marginal (Mg2+ median concentration range: 0.8–1 mM; Table 1). This can be explained by low data variability in our dataset (Supplementary Appendix 1), affected by wide usage of PGM based on the Brewbaker and Kwack PG protocol (Brewbaker and Kwack, 1963). The group-specific difference and possible underlying reasons are discussed in the following paragraphs.
In cultivated plants, the median concentration of H3BO3 (1.6 mM) and pH (6.1) were significantly higher than in wild plants (H3BO3 = 1.3 mM and pH = 5.5). In contrast, wild species had significantly higher concentrations of Ca2+ (1.8 mM) and K+ (3.0 mM) than the cultivated ones (Ca2+ = 1.3 mM, K+ = 1.0 mM; Table 1 and Figure 1). Some studies indicate that pollen of wild species have lower PGM requirements than the cultivated ones (Jayaprakash, 2018) as was observed in the case of boric acid. However, we have no a plausible explanation for the pH differences or why the mineral concentrations were higher in the wild plants than the cultivated. The differences could be attributed to domestication and artificial selection, which may cause cultivars to change their physiological and morphological attributes thus differing from the wild counterparts (Chaudhary, 2013; Gepts, 2014; Liu et al., 2019). Finally, the experimental design and selection of various conditions for domesticated and wild species by different researchers could as well have contributed to the observed differences.
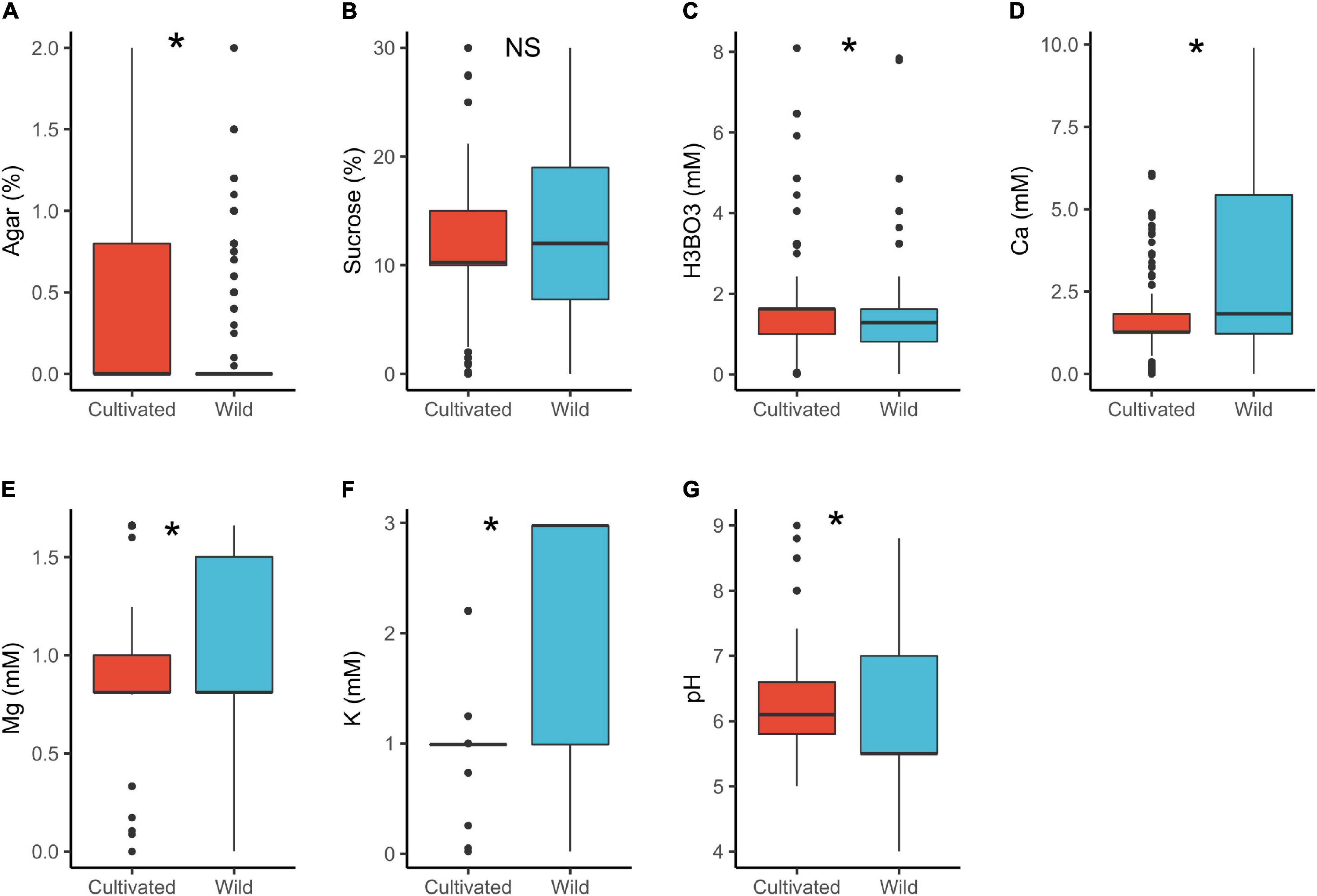
Figure 1. Pollen germination media requirements for cultivated (n = 449) and wild species (n = 689): agar (A), sucrose (B), boric acid (C), calcium (D), magnesium (E), potassium (F), and pH (G). *, significant (p < 0.05); NS, not significant.
In angiosperms, the median concentrations of sucrose (12%) and Ca2+ (1.7 mM) were significantly higher than in gymnosperms (sucrose = 10%, Ca2+ = 1.3 mM; Table 1 and Figure 2). These results are in line with the study by Brewbaker and Kwack (1963) that shows that gymnosperm pollen generally require lower concentration of these substances (Brewbaker and Kwack, 1963). The lower calcium requirements can be attributed to the generally comparatively slow growth of germinating gymnosperm pollen (Brewbaker and Kwack, 1963; Williams, 2012). The dissimilarity in the cytology and wall structure between angiosperms and gymnosperms pollen (e.g., Pacini et al., 1999; Fernando et al., 2005) could probably also contribute to the observed differences. Further, pollen cytology plays a significant role in the metabolic processes (Bergamini and Mulcahy, 1988). For instance, pollen grains of most gymnosperms are wind pollinated (Faegri and van der Pijl, 1979) and hence, released with a low moisture content to reduce weight (Fernando et al., 2005). In order for gymnosperm pollen to germinate, rehydration takes place quickly in the liquid medium/pollination drop within the micropylar (Nepi et al., 2005; Firon et al., 2012). This suggests that a higher osmotic potential (lower salt content) is required probably explaining the lower concentrations of sucrose and Ca2+ observed in the PGM for gymnosperms. Contrary, angiosperm pollen is released with higher moisture content with rehydration being slower, and grows hurriedly, utilizing the available ingredients quickly (Dawkins and Owens, 1993; Pacini et al., 1999; Nepi et al., 2005; Firon et al., 2012). This probably explains the higher concentration of sucrose and Ca2+ in the in vitro media.
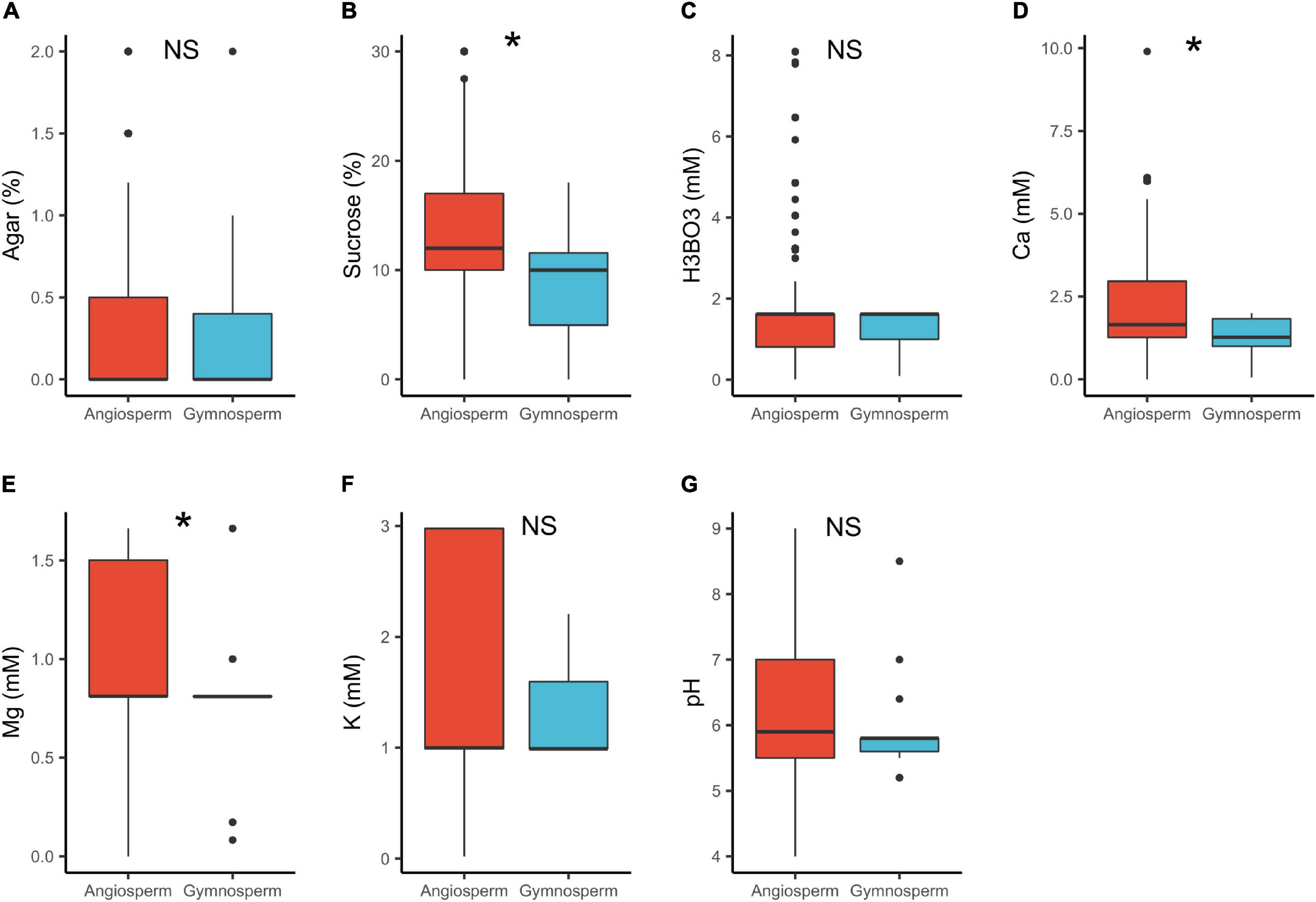
Figure 2. Pollen germination media requirements for angiosperms (n = 1092) and gymnosperms (n = 46): agar (A), sucrose (B), boric acid (C), calcium (D), magnesium (E), potassium (F), and pH (G). *, significant (p < 0.05); NS, not significant.
In dicots, the median concentrations of sucrose (14%) and Ca2+ (1.8 mM) were significantly higher, than in monocots (sucrose = 10%, Ca2+ = 1.3 mM; Table 1 and Figure 3). These results are in accordance with previous research showing that monocot pollen require lower mineral content than dicots (Jayaprakash, 2018). A possible explanation is that monocot pollen is usually recalcitrant (i.e., have high moisture content of 30–40%) as compared to orthodox pollen of dicots (1–5%; Franchi et al., 2011; Jayaprakash, 2018). This suggests therefore, that monocot pollen requires lower ion concentrations in a PGM to reach the turgence level needed to germinate. Additionally, pollen of several monocots such as Typha latifolia L., are released with high concentration of sugars in it (mainly sucrose) and other protective molecules to confer stability after release, an adaptation against desiccation (Wolkers et al., 2001; Pacini et al., 2006). They therefore require low concentrations of these in the media. Contrastingly, the largely orthodox dicot pollen are desiccation tolerant and are released with low moisture and mineral content (Wolkers et al., 2001; Pacini et al., 2006; Franchi et al., 2011), hence requiring a higher supply of these substances in in vitro media.
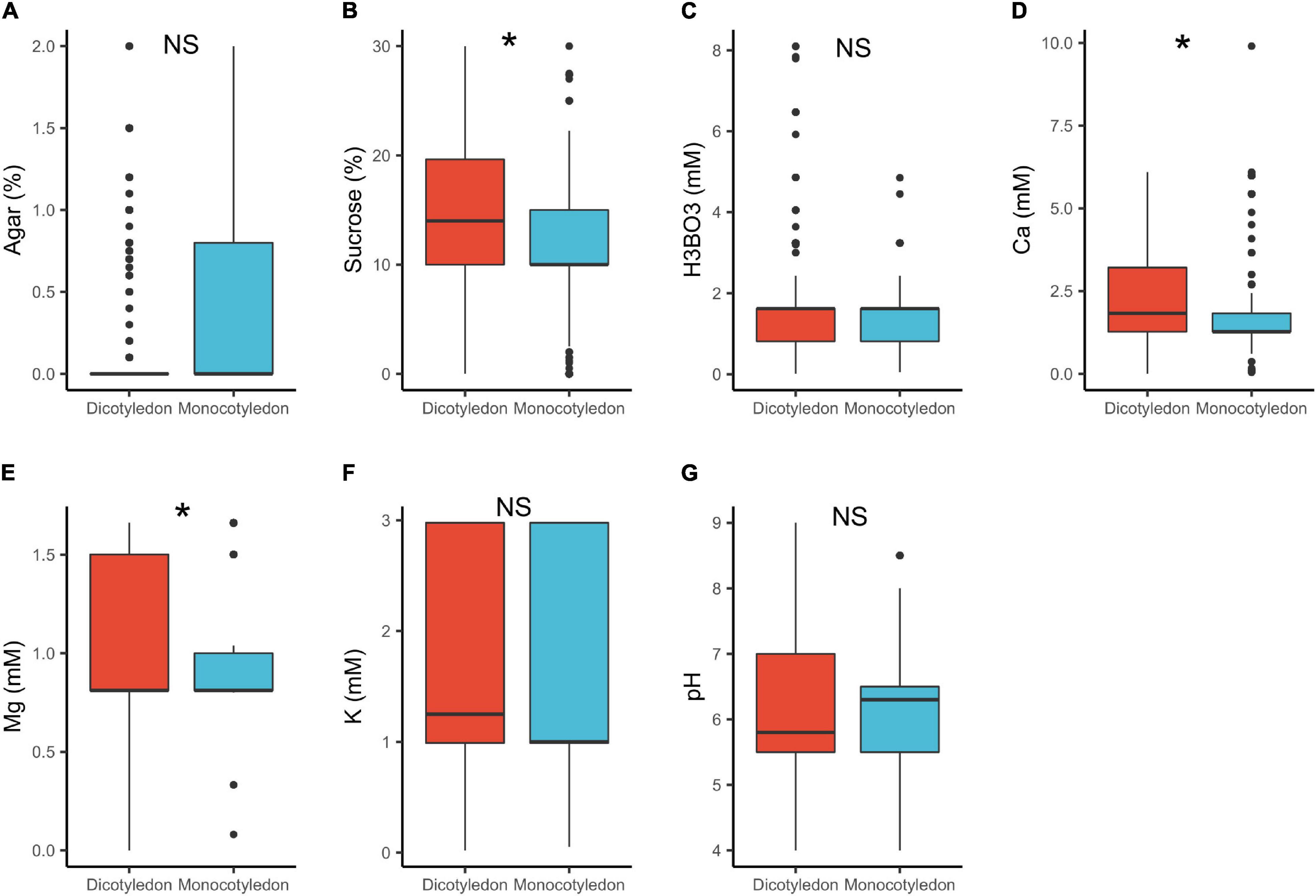
Figure 3. Pollen germination media requirements for dicotyledons (n = 818) and monocotyledons (n = 274): agar (A), sucrose (B), boric acid (C), calcium (D), magnesium (E), potassium (F), and pH (G). *, significant (p < 0.05); NS, not significant.
In tri-nucleated pollen, requirements for sucrose in PGM were significantly higher than in binucleated pollen (15 vs. 10%, respectively; Table 1 and Figure 4). The component requirements in vitro highly depend on the existing endogenous pollen reserves, whether these are sustainable autotrophically and are readily available for metabolism (Read et al., 1993; Stephenson et al., 2003; Carrizo García et al., 2012). Trinucleated pollen is found to be highly dependent on exogeneous supply of substances important for PG and PTG (Mulcahy and Mulcahy, 1988). This can be associated with tricellular pollen germinating faster, a heterochronic evolutionary shift from bicellular pollen (Brewbaker, 1967; Mulcahy and Mulcahy, 1988), accounting for the higher sucrose requirements observed. In contrast, binucleated pollen grains initially rely on their own reserves when germinating in vitro, hence the lower sucrose requirements (Mulcahy and Mulcahy, 1988). Pollen requirements for Ca2+ in PGM were also statistically different between tri- vs. bi-nucleated pollen but the median values were similar (1.7 mM; Table 1) as was the case for Mg2+.
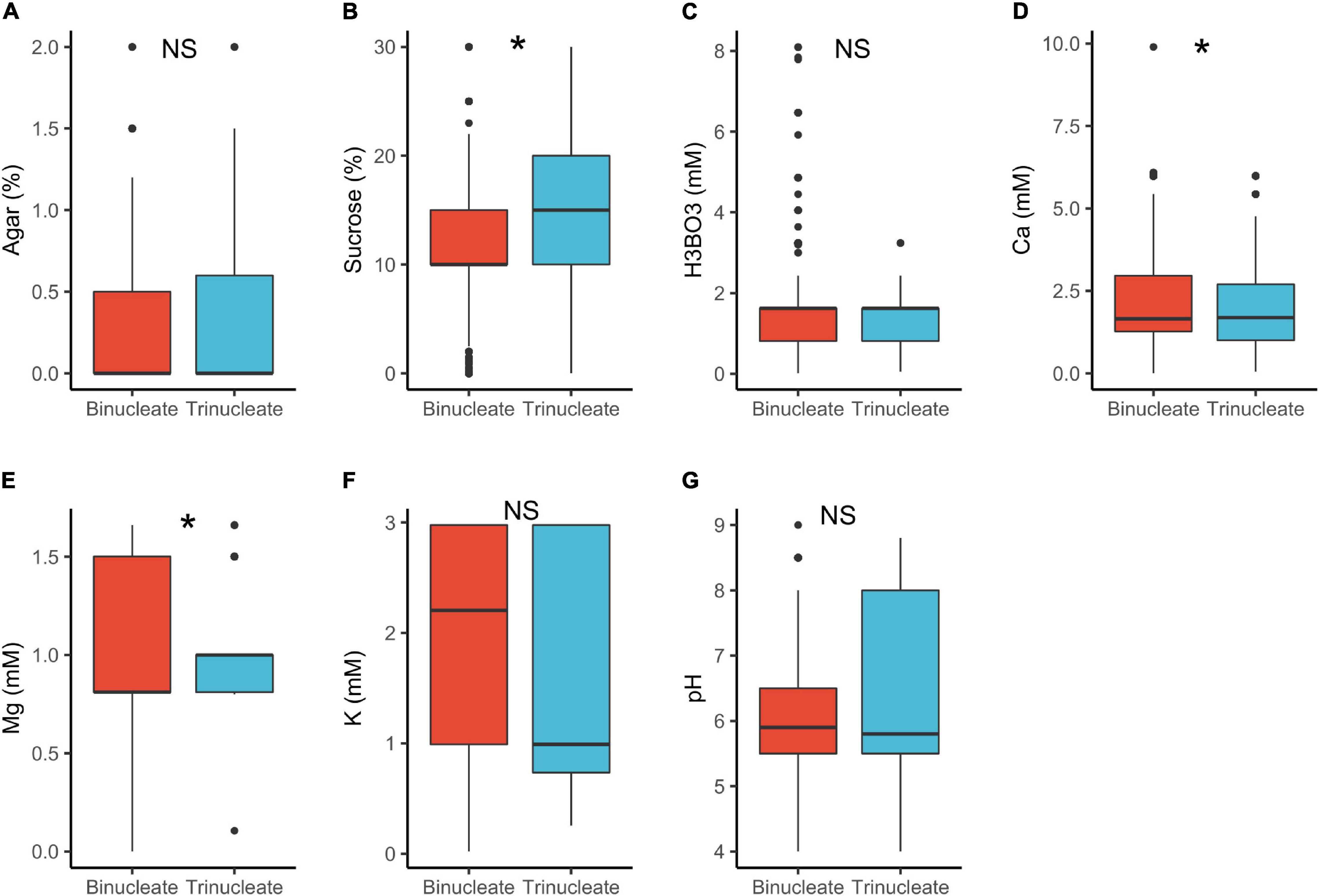
Figure 4. Pollen germination media requirements for binucleate (n = 913) and trinucleate pollen (n = 713): agar (A), sucrose (B), boric acid (C), calcium (D), magnesium (E), potassium (F), and pH (G). *, significant (p < 0.05); NS, not significant.
In plant with dry stigmas, sucrose (15%) and Ca2+ (1.8 mM) requirements were significantly higher than in wet stigma plants (sucrose = 10%, Ca2+ = 1.3 mM; Table 1 and Figure 5). Under natural conditions, wet stigmatic plants produce exudates rich in minerals and sugars (Labarca et al., 1970; Hawker et al., 1983; Kandasamy and Vivekanandan, 1983; Lau et al., 2017) in which pollen germinates (Park and Lord, 2003; Allen and Hiscock, 2010; Rejón et al., 2014). This would suggest that pollen in wet stigmatic plants should as well require a higher exogenous supply of these substances in vitro. However, this was contrary with our results. A possible explanation is that most dry stigmatic plants tend to have trinucleate pollen with high requirements for exogenous minerals (see also above), whereas wet stigmas are associated with binucleated pollen (lower supply; Brewbaker, 1967; Montaner et al., 2003).
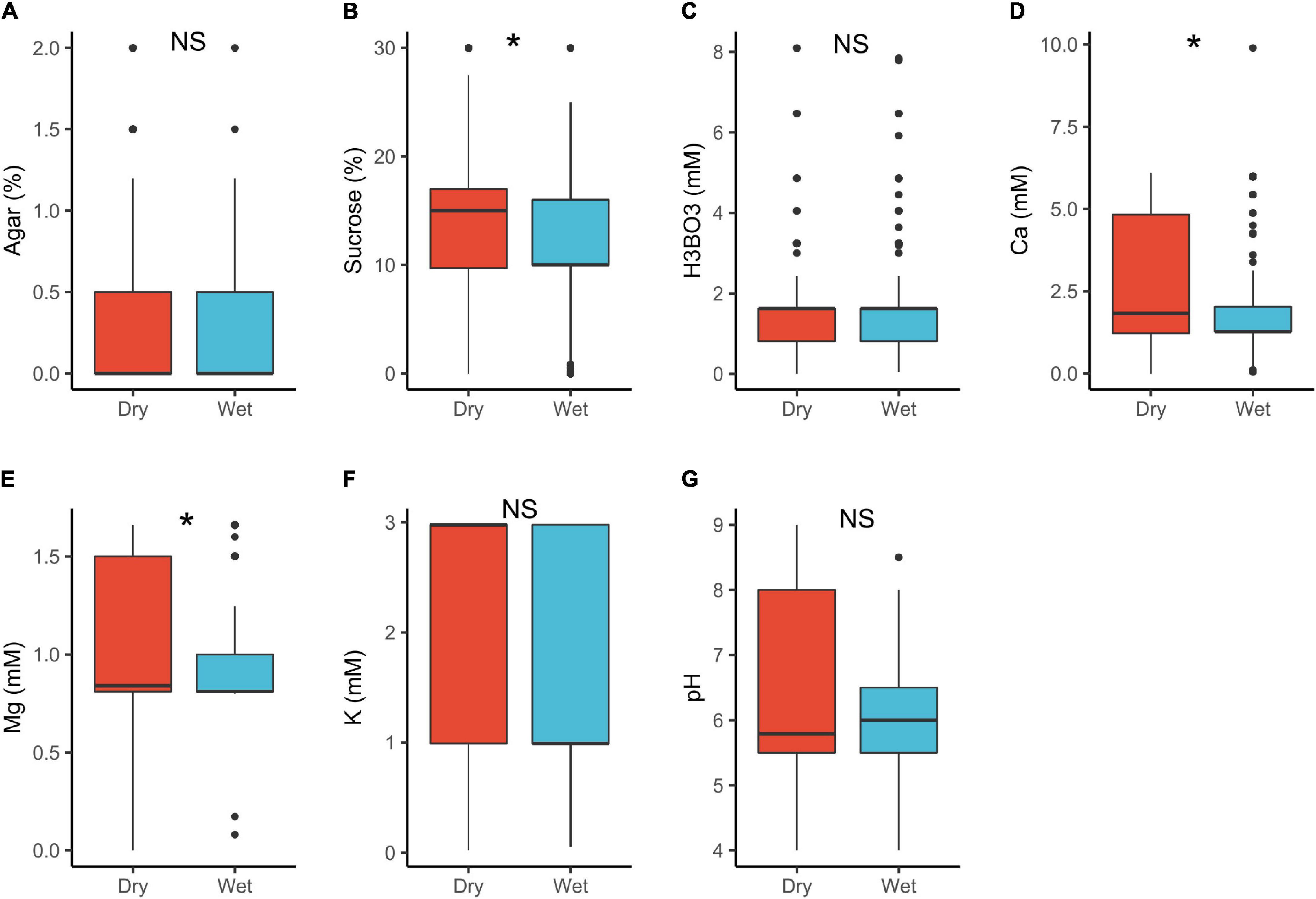
Figure 5. Pollen germination media requirements for dry stigma (n = 604) and wet stigma plants (n = 475): agar (A), sucrose (B), boric acid (C), calcium (D), magnesium (E), potassium (F), and pH (G). *, significant (p < 0.05); NS, not significant.
In plants with starchy vs. starchless pollen, we detected statistically significant differences for K+ requirements only (Table 1 and Figure 6). The lower requirements of starchless pollen (median 2.6 mM) than in starchy (3.0 mM) can be attributed to the fact that the former are usually associated to binucleated pollen which require lower mineral input than in starchy/trinucleate (Lora et al., 2012). Notably, both pollen types can occur in the same anther (Baker and Baker, 1979; Lora et al., 2012) and yet may need different requirements to germinate in vitro (Franchi et al., 2007).
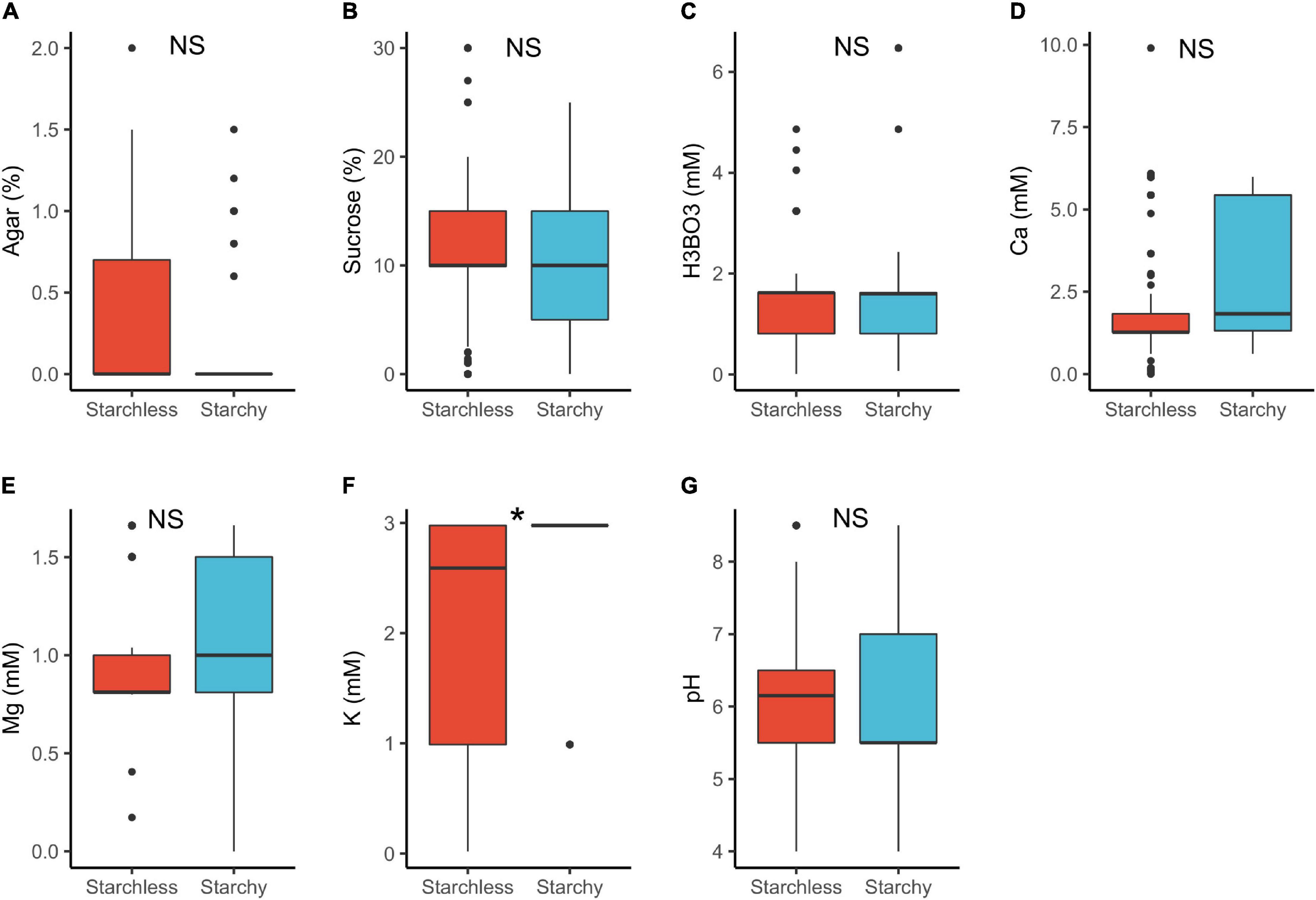
Figure 6. Pollen germination media requirements for starchy (n = 86) and starchless pollen (n = 268): agar (A), sucrose (B), boric acid (C), calcium (D), magnesium (E), potassium (F), and pH (G). *, significant (p < 0.05); NS, not significant.
As for the groupings by taxonomy, plant families Solanaceae (10%), Rosaceae (9%), Fabaceae (8%), Poaceae and Pinaceae at 5% had the strongest representation in the compendium accounting all together for 586 PGM recipes for 285 (35%) species. The high contribution from these five families can be explained by the fact that many species from these families are cultivated plants (e.g., Solanaceae: tomato, potato; Rosaceae: almond, apple, pear, cherry; Fabaceae: peas and beans; Poaceae: rice, wheat, barely; Pinaceae: pines). Nicotiana tabacum L. (27 recipes), Zea mays L. (25), and Lilium longiflorum Thunb. (22), which are also economically (Z. mays) and scientifically (N. tabacum and L. longiflorum) important plants, were the most studied species. N. tabacum L., the tobacco plant, has also been widely used as a model plant in pollen research, as it produces long-living pollen in large quantities (Cheung et al., 1995; Conze et al., 2017). The medium requirements for the most frequently cultivated species as well as model species such as A. thaliana (L.) Heynh. are summarized in the Table 2.
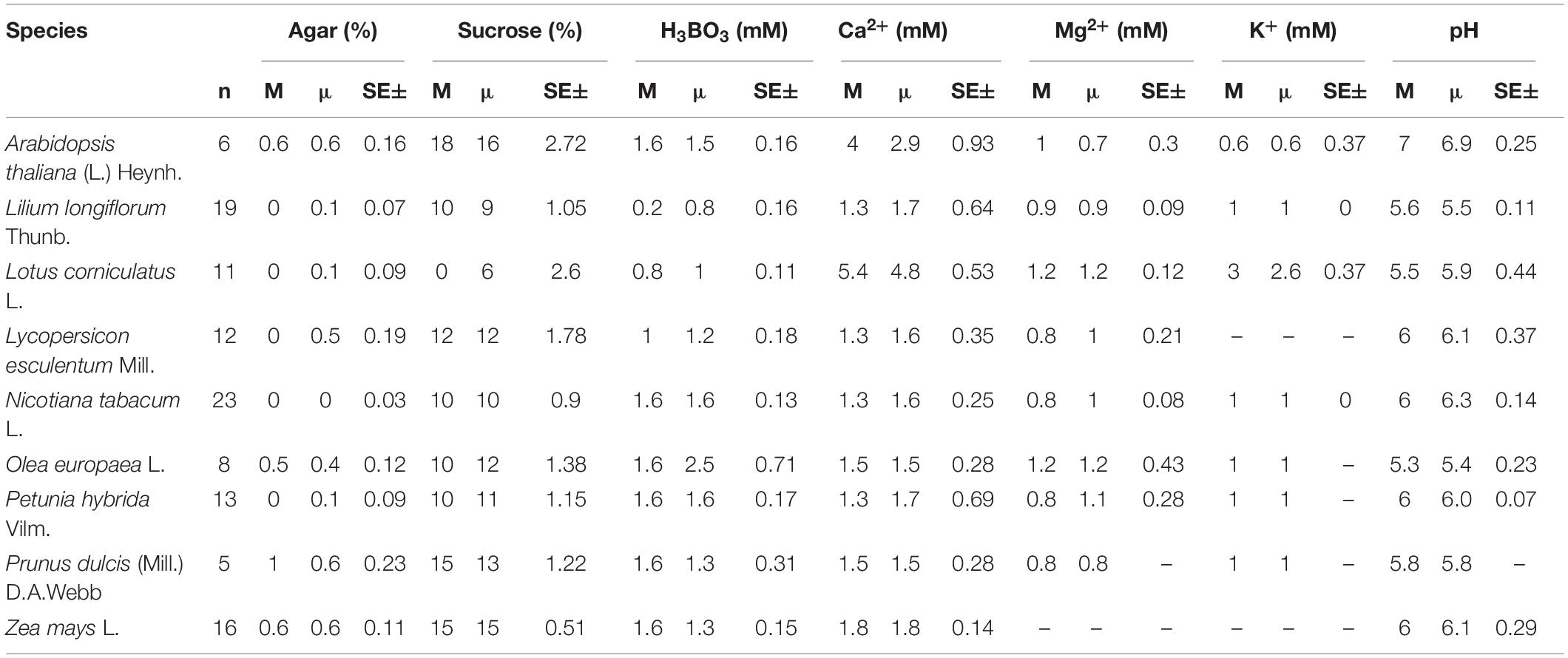
Table 2. The median (M), mean (μ) concentrations, and standard error of the most frequently used ingredients in in vitro pollen germination media for the most studied species.
The PGM requirements for the most used components also tend to be different for the families. Among the frequent families, only Poaceae pollen tends to germinate better and produce pollen tubes on solid medium (0.6% agar; Table 3) with the rest of the families preferring liquid medium. The tendency of Poaceae pollen to germinate in PGM with higher agar concentration can be explained by the dry stigmata in this family. Germinating pollen of plants with dry stigmas has often proved challenging as it is more difficult to imitate the complex interactions on dry stigmatic surfaces (Allen and Hiscock, 2010; Rodriguez-Enriquez et al., 2013). Therefore, the higher agar concentration in PGM of pollen from dry stigmatic plants helps in incorporating the ingredients, maintaining humidity, and adequate aerobic conditions for PG (Linskens and Stanley, 1974). In contrast, the rest of frequent families mostly have wet stigmas with exudates (Allen and Hiscock, 2010) which are easier to imitate in vitro and germinate the pollen in liquid media.
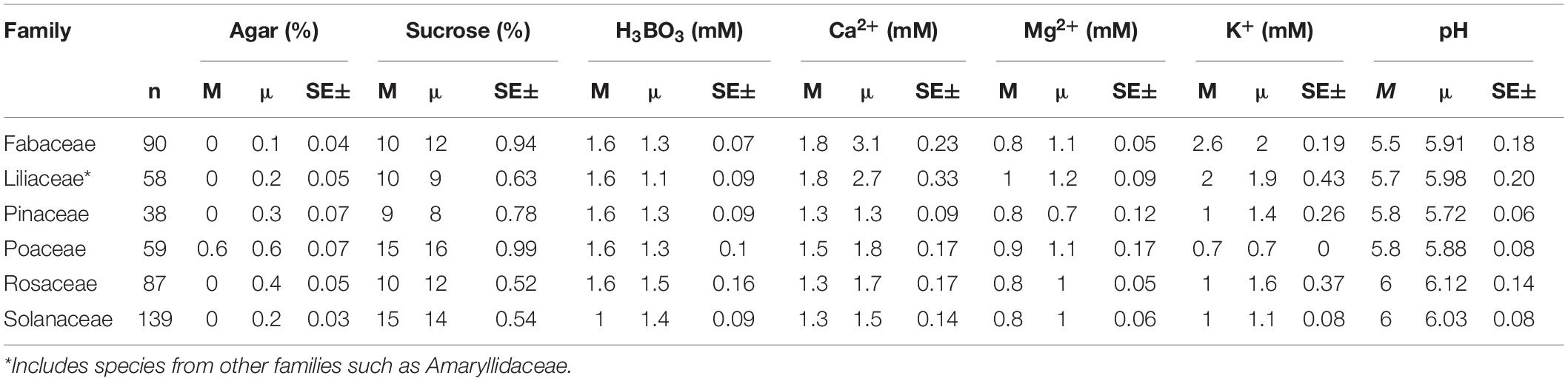
Table 3. The median (M), mean (μ) concentrations, and standard error of the most frequently used ingredients in in vitro pollen germination media for the most represented families.
Furthermore, Poaceae and Solanaceae tend to have comparatively higher sucrose requirements than the other frequent families (15 vs. 9–10%; Table 3). Notably, some Poaceae species such as Triticum aestivum L. even require higher concentrations of other sugars such as maltose (18–30%; Supplementary Appendix 1). This can be explained by Poaceae having trinucleated pollen that mostly rely on the exogenous mineral supply (also see above). Solanaceae having high sucrose requirements can be attributed to the high data variability in our dataset for this family (sucrose concentration range 10–20%). Generally, pollen anatomy, morphology, and physiology can vary considerably among species (Baker and Baker, 1979; Pacini, 1996; Speranza et al., 1997) contributing to observed differences in the media requirements among families (Table 3).
Developing PGM Recipes
The detected (dis)similarities in pollen requirements for the most frequently used PGM components can be used for creating a new PGM where no ready recipe is available in our data set. Below, we suggest a number of “base” recipes for various plant groups:
• Angiosperms: liquid media, 12% sucrose, 1.6 mM H3BO3, 1.7 mM Ca2+, 0.8 mM Mg2+, 1.0 mM K+, and pH 5.9
• Gymnosperms: liquid media, 10% sucrose, 1.6 mM H3BO3, 1.3 mM Ca2+, 0.8 mM Mg2+, 1.0 mM K+, and pH 5.8
• Dicots: liquid media, 14% sucrose, 1.6 mM H3BO3, 1.8 mM Ca2+, 0.8 mM Mg2+, 1.3 mM K+, and pH 5.8
• Monocots: liquid media, 10% sucrose, 1.6 mM H3BO3, 1.3 mM Ca2+, 0.8 mM Mg2+, 1.0 mM K+, and pH 6.3
• Binucleate pollen: liquid media, 10% sucrose, 1.6 mM H3BO3, 1.7 mM Ca2+, 0.8 mM Mg2+, 2.2 mM K+, and pH 5.9
• Trinucleate pollen: liquid media, 15% sucrose, 1.6 mM H3BO3, 1.7 mM Ca2+, 1.0 mM Mg2+, 1.0 mM K+, and pH 5.8
• Dry stigmatic plants: liquid media, 15% sucrose, 1.6 mM H3BO3, 1.8 mM Ca2+, 0.8 mM Mg2+, 3.0 mM K+, and pH 5.8
• Wet stigmatic plants: liquid media, 10% sucrose, 1.6 mM H3BO3, 1.3 mM Ca2+, 0.8 mM Mg2+, 1.0 mM K+, and pH 6.0
• Starchless pollen: liquid media, 10% sucrose, 1.6 mM H3BO3, 1.3 mM Ca2+, 0.8 mM Mg2+, 2.6 mM K+, and pH 6.2
• Starchy pollen: liquid media, 10% sucrose, 1.6 mM H3BO3, 1.8 mM Ca2+, 1.0 mM Mg2+, 3.0 mM K+, and pH 5.5.
For some frequent families, the following from our data set can be used as a guide in creating new PGM:
• Fabaceae: liquid media, 10% sucrose, 1.6 mM H3BO3, 1.8 mM Ca2+, 0.8 mM Mg2+, 2.6 mM K+, and pH 5.5
• Pinaceae: liquid media, 9% sucrose, 1.6 mM H3BO3, 1.3 mM Ca2+, 0.8 mM Mg2+, 1.0 mM K+, and pH 5.8
• Poaceae: 0.6% agar, 15% sucrose, 1.6 mM H3BO3, 1.5 mM Ca2+, 0.9 mM Mg2+, 0.7 mM K+, and pH 5.8
• Rosaceae: liquid media, 10% sucrose, 1.6 mM H3BO3, 1.3 mM Ca2+, 0.8 mM Mg2+, 1.0 mM K+, and pH 6
• Solanaceae: liquid media, 15% sucrose, 1.0 mM H3BO3, 1.3 mM Ca2+, 0.8 mM Mg2+, 1.0 mM K+, and pH 6.
Data Availability Statement
The original contributions presented in the study are included in the article/Supplementary Material, further inquiries can be directed to the corresponding author/s.
Author Contributions
SR designed the study. DT and SR made substantial contributions to the acquisition, analysis, and interpretation of the work. Both authors proofed and corrected the manuscript.
Funding
Research funding was provided by the Deutsche Forschungsgemeinschaft (DFG), Project RO 4909/1-1 (SR).
Conflict of Interest
The authors declare that the research was conducted in the absence of any commercial or financial relationships that could be construed as a potential conflict of interest.
Acknowledgments
We thank Vladimir Orbovic and the two reviewers for their comments on the earlier version of the manuscript.
Supplementary Material
The Supplementary Material for this article can be found online at: https://www.frontiersin.org/articles/10.3389/fpls.2021.709945/full#supplementary-material
Supplementary Appendix 1 | Compendium of pollen germination media.
Footnotes
References
Albert, B., Ressayre, A., Dillmann, C., Carlson, A. L., Swanson, R. J., Gouyon, P.-H., et al. (2018). Effect of aperture number on pollen germination, survival and reproductive success in Arabidopsis thaliana. Ann. Bot. 121, 733–740. doi: 10.1093/aob/mcx206
Allen, A., and Hiscock, S. J. (2010). Molecular communication between plant pollen and pistils. CAB Rev. 5:105053. doi: 10.1079/PAVSNNR20105053
Ashman, T.-L., Knight, T. M., Steets, J. A., Amarasekare, P., Burd, M., Campbell, D. R., et al. (2004). Pollen limitation of plant reproduction: ecological and evolutionary causes and consequences. Ecology 85, 2408–2421. doi: 10.1890/03-8024
Baker, H. G., and Baker, I. (1979). Starch in angiosperm pollen grains and its evolutionary significance. Am. J. Bot. 66, 591–600. doi: 10.1002/j.1537-2197.1979.tb06262.x
Baloch, M. J., Lakho, A. R., Bhutto, H., and Solangi, M. Y. (2001). Impact of sucrose concentrations on in vitro pollen germination of okra, Hibiscus esculentus. Pakistan J. Biol. Sci. 4, 402–403. doi: 10.3923/PJBS.2001.402.403
Bellani, L. M., Pacini, E., and Franchi, G. G. (1985). In vitro pollen grain germination and starch content in species with different reproductive cycle i. Lycopersicum peruvianum Mill. Acta Botan. Neerlandica 34, 59–64. doi: 10.1111/j.1438-8677.1985.tb01852.x
Bellani, L. M., Rinallo, C., Muccifora, S., and Gori, P. (1997). Effects of simulated acid rain on pollen physiology and ultrastructure in the apple. Env. Pollut. 95, 357–362. doi: 10.1016/S0269-7491(96)00127-3
Bergamini, M. G., and Mulcahy, D. L. (1988). The effect of supplemented media on the growth in vitro of bi- and trinucleate pollen. Plant Sci. 55, 213–216. doi: 10.1016/0168-9452(88)90063-5
Bilderback, D. E. (1981). Impatiens pollen germination and tube growth as a bioassay for toxic substances. Env. Health Perspect. 37:95. doi: 10.2307/3429256
Biswas, P., and Mondal, S. (2014). Role of some nutrients on in vitro pollen germination and tube development of Luffa cylindrica (L.) Roem. Ann. Plant Sci. 3, 813–821.
Boavida, L. C., and McCormick, S. (2007). Temperature as a determinant factor for increased and reproducible in vitro pollen germination in Arabidopsis thaliana. Plant J. 52, 570–582. doi: 10.1111/j.1365-313X.2007.03248.x
Brewbaker, J. L. (1967). The distribution and phylogenetic significance of binucleate and trinucleate pollen grains in the angiosperms. Am. J. Bot. 54, 1069–1083. doi: 10.1002/j.1537-2197.1967.tb10735.x
Brewbaker, J. L., and Kwack, B. H. (1963). The essential role of calcium ion in pollen germination and pollen tube growth. Am. J. Bot. 50:859. doi: 10.2307/2439772
Brink, R. A. (1924). The physiology of pollen iii. growth in vitro and in vivo. Am. J. Bot. 11, 351–364. doi: 10.1002/j.1537-2197.1924.tb05781.x
Burke, J. J. (2002). Moisture sensitivity of cotton pollen. Agron. J. 94, 883–888. doi: 10.2134/agronj2002.8830
Burke, J. J., Velten, J., and Oliver, M. J. (2004). In vitro analysis of cotton pollen germination. Agron. J. 96, 359–368. doi: 10.2134/agronj2004.3590
Campos, M. G. R., Bogdanov, S., Almeida-Muradian, L. B., de, Szczesna, T., Mancebo, Y., et al. (2008). Pollen composition and standardisation of analytical methods. J. Apicult. Res. 47, 154–161. doi: 10.1080/00218839.2008.11101443
Čapková-Balatková, V., Hrabìtová, E., and Tupý, J. (1980). Effect of some mineral ions on pollen tube growth and release of proteins in culture. Biol. Plant 22, 294–302. doi: 10.1007/BF02892776
Carrizo García, C., Guarnieri, M., and Pacini, E. (2012). Tomato pollen tube development and carbohydrate fluctuations in the autotrophic phase of growth. Acta Physiol. Plant 34, 2341–2347. doi: 10.1007/s11738-012-1037-4
Chaudhary, B. (2013). Plant domestication and resistance to herbivory. Int. J. Plant Genom. 2013:572784. doi: 10.1155/2013/572784
Chauhan, S. V. S., Yadav, V., and Yadav, D. K. (1987). Studies into the causes of seedlessness in some Bignoniaceae. J. Exp. Bot. 38, 173–177. doi: 10.1093/jxb/38.1.173
Cheng, C., and Mcomb, J. A. (1992). In vitro germination of wheat pollen on raffinose medium. N. Phytol. 120, 459–462. doi: 10.1111/j.1469-8137.1992.tb01793.x
Cheng, C., and Rerkasem, B. (1993). Effects of boron on pollen viability in wheat. Plant Soil 155-156, 313–315. doi: 10.1007/BF00025045
Cheung, A. Y., Wang, H., and Wu, H.-M. (1995). A floral transmitting tissue-specific glycoprotein attracts pollen tubes and stimulates their growth. Cell 82, 383–393. doi: 10.1016/0092-8674(95)90427-1
Cole, R. A., Synek, L., Zarsky, V., and Fowler, J. E. (2005). SEC8, a subunit of the putative Arabidopsis exocyst complex, facilitates pollen germination and competitive pollen tube growth. Plant Physiol. 138, 2005–2018. doi: 10.1104/pp.105.062273
Conner, P. J. (2011). Optimization of in vitro pecan pollen germination. Horts 46, 571–576. doi: 10.21273/HORTSCI.46.4.571
Conze, L. L., Berlin, S., Le Bail, A., and Kost, B. (2017). Transcriptome profiling of tobacco (Nicotiana tabacum) pollen and pollen tubes. BMC Genomics 18:581. doi: 10.1186/s12864-017-3972-3
Dafni, A., and Firmage, D. (2000). ““Pollen viability and longevity: practical, ecological and evolutionary implications,”,” in Pollen and pollination, eds A. Dafni, M. Hesse, and E. Pacini (Vienna: Springer), 113–132.
Dawkins, M. D., and Owens, J. N. (1993). In vitro and in vivo pollen hydration, germination, and pollen-tube growth in white spruce, Picea glauca (Moench) Voss. Intern. J. Plant Sci. 154, 506–521.
de Bruyn, J. A. (1966). The in vitro germination of pollen of Setaria sphacelata. I. Effects of carbohydrates, hormones, vitamins and micronutrients. Physiol. Plant 19, 365–376. doi: 10.1111/j.1399-3054.1966.tb07027.x
Fan, L.-M. (2001). In vitro Arabidopsis pollen germination and characterization of the inward potassium currents in Arabidopsis pollen grain protoplasts. J. Exp. Bot. 52, 1603–1614. doi: 10.1093/jexbot/52.361.1603
Fang, K. F., Du, B. S., Zhang, Q., Xing, Y., Cao, Q. Q., and Qin, L. (2019). Boron deficiency alters cytosolic Ca2+ concentration and affects the cell wall components of pollen tubes in Malus domestica. Plant Biol. 21, 343–351. doi: 10.1111/plb.12941
Fang, K., Zhang, W., Xing, Y., Zhang, Q., Yang, L., Cao, Q., et al. (2016). Boron toxicity causes multiple effects on Malus domestica pollen tube growth. Front. Plant Sci. 7:208. doi: 10.3389/fpls.2016.00208
Fernando, D. D., Lazzaro, M. D., and Owens, J. N. (2005). Growth and development of conifer pollen tubes. Sexual. Plant Reprod 18:195. doi: 10.1007/s00497-005-0013-1
Fernando, D. D., Owens, J. N., Yu, X., and Ekramoddoullah, A. K. M. (2001). RNA and protein synthesis during in vitro pollen germination and tube elongation in Pinus monticola and other conifers. Sexual Plant Reprod 13, 259–264. doi: 10.1007/s004970100069
Firon, N., Nepi, M., and Pacini, E. (2012). Water status and associated processes mark critical stages in pollen development and functioning. Ann. Bot. 109, 1201–1214. doi: 10.1093/aob/mcs070
Fragallah, S., Lin, S., Li, N., Ligate, E., and Chen, Y. (2019). Effects of sucrose, boric acid, pH, and incubation time on in vitro germination of pollen and tube growth of Chinese fir (Cunnighamial lanceolata L.). Forests 10:102. doi: 10.3390/f10020102
Franchi, G. G., Nepi, M., Matthews, M. L., and Pacini, E. (2007). Anther opening, pollen biology and stigma receptivity in the long blooming species, Parietaria judaica L. (Urticaceae). Flora Morphol. Distrib. Funct. Ecol. Plants 202, 118–127. doi: 10.1016/j.flora.2006.03.005
Franchi, G. G., Piotto, B., Nepi, M., Baskin, C. C., Baskin, J. M., and Pacini, E. (2011). Pollen and seed desiccation tolerance in relation to degree of developmental arrest, dispersal, and survival. J. Exp. Bot. 62, 5267–5281. doi: 10.1093/jxb/err154
Fricker, M. D., White, N. S., and Obermeyer, G. (1997). pH gradients are not associated with tip growth in pollen tubes of Lilium longiflorum. J. Cell Sci. 110, (Pt 15) 1729–1740.
Gepts, P. (2014). “Domestication of plants,” in Encyclopedia of Agriculture and Food Systems, ed. Neal K. Van Alfen (Amsterdam: Elsevier), 474–486.
Gibernau, M., Macquart, D., and Diaz, A. (2003). Pollen viability and longevity in two species of Arum. Aroideana 26, 58–62.
Golan-Goldhirsh, A., Schmidhalter, U., Müller, M., and Oertli, J. J. (1991). Germination of Pistacia vera L. pollen in liquid medium. Sexual Plant Reprod 4, 182–187.
Hashida, S.-N., Takahashi, H., Kawai-Yamada, M., and Uchimiya, H. (2007). Arabidopsis thaliana nicotinate/nicotinamide mononucleotide adenyltransferase (AtNMNAT) is required for pollen tube growth. Plant J. 49, 694–703. doi: 10.1111/j.1365-313X.2006.02989.x
Hawker, J. S., Sedgley, M., and Loveys, B. R. (1983). Composition of stigmatic exudate, nectar and pistil of watermelon, Citrullus lanatus (Thunb.) Matsum. & Nakai, before and after pollination. Funct. Plant Biol. 10:257. doi: 10.1071/PP9830257
Heslop-Harrison, Y., and Heslop-Harrison, J. (1992). Germination of monocolpate angiosperm pollen: evolution of the actin cytoskeleton and wall during hydration, activation and tube emergence. Ann. Bot. 69, 385–394. doi: 10.1093/oxfordjournals.aob.a088359
Heslop-Harrison, Y., and Shivanna, K. R. (1977). The receptive surface of the angiosperm stigma. Ann. Bot. 41, 1233–1258. doi: 10.1093/oxfordjournals.aob.a085414
Hirsche, J., García Fernández, J. M., Stabentheiner, E., Großkinsky, D. K., and Roitsch, T. (2017). Differential effects of carbohydrates on Arabidopsis pollen germination. Plant Cell Physiol. 58, 691–701. doi: 10.1093/pcp/pcx020
Hoekstra, F. A. (1979). Mitochondrial development and activity of binucleate and trinucleate pollen during germination in vitro. Planta 145, 25–36. doi: 10.1007/BF00379924
Hoffmann, F., Martin, B. A., Sibley, R. B., and Tsay, S.-S. (1990). Pollen germination is impeded by tap water. Env. Pollut. 63, 179–187. doi: 10.1016/0269-7491(90)90066-l
Holdaway-Clarke, T. L., Weddle, N. M., Kim, S., Robi, A., Parris, C., Kunkel, J. G., et al. (2003). Effect of extracellular calcium, pH and borate on growth oscillations in Lilium formosanum pollen tubes. J. Exp. Bot. 54, 65–72. doi: 10.1093/jxb/erg004
Hong-Qi, Z., and Croes, A. F. (1982). A new medium for pollen germination in vitro. Acta Botanica Neerlandica 31, 113–119. doi: 10.1111/j.1438-8677.1982.tb01597.x
Imani, A. (2012). Standardization of different media for in vitro pollen germination of almond and evaluation of the germination capacity of stored pollen. Afr. J. Biotechnol. 11:1561. doi: 10.5897/AJB11.1561
Impe, D., Reitz, J., Köpnick, C., Rolletschek, H., Börner, A., Senula, A., et al. (2019). Assessment of pollen viability for wheat. Front. Plant Sci. 10:1588. doi: 10.3389/fpls.2019.01588
Jayaprakash, P. (2018). “Pollen germination in vitro,” in Pollination in plants, ed. P. W. Mokwala (Chennai: InTech).
Jayaprakash, P., Sheeba, D., Vikas, V. K., Sivasamy, M., and Sabesan, T. (2018). Development of pollen germination medium to test pollen viability of eggplant and its wild species. Ind. J. Hort. 75:237. doi: 10.5958/0974-0112.2018.00041.5
Johnson-Brousseau, S. A., and McCormick, S. (2004). A compendium of methods useful for characterizing Arabidopsis pollen mutants and gametophytically-expressed genes. Plant J. 39, 761–775. doi: 10.1111/j.1365-313X.2004.02147.x
Kakani, V. G., Reddy, K. R., Koti, S., Wallace, T. P., Prasad, P. V. V., Reddy, V. R., et al. (2005). Differences in in vitro pollen germination and pollen tube growth of cotton cultivars in response to high temperature. Ann. Bot. 96, 59–67. doi: 10.1093/aob/mci149
Kandasamy, M. K., and Vivekanandan, M. (1983). Biochemical composition of stigmatic exudate of Eichhornia crassipes (Mart.) solms. Aquat. Bot. 16, 41–47. doi: 10.1016/0304-3770(83)90050-5
Komosinska-Vassev, K., Olczyk, P., Kaźmierczak, J., Mencner, L., and Olczyk, K. (2015). Bee pollen: chemical composition and therapeutic application. Evid. Based Compl. Altern. Med. 2015, 1–6. doi: 10.1155/2015/297425
Kumari, A., Papenfus, H. B., Kulkarni, M. G., Pošta, M., and van Staden, J. (2015). Effect of smoke derivatives on in vitro pollen germination and pollen tube elongation of species from different plant families. Plant Biol. 17, 825–830. doi: 10.1111/plb.12300
Labarca, C., Kroh, M., and Loewus, F. (1970). The composition of stigmatic exudate from Lilium longiflorum: labeling studies with myo-inositol, d-glucose, and l-proline. Plant Physiol. 46, 150–156. doi: 10.1104/pp.46.1.150
Lagera, A. J., Balinado, L. O., Baldomero, J. R., Rotairo, H. F. I., Tero, N. L., Maghinay, M. S., et al. (2017). Varying sugars and sugar concentrations influence in vitro pollen germination and pollen tube growth of Cassia alata L. J. Young Investig. 1, 42–45.
Lau, J. Y. Y., Pang, C.-C., Ramsden, L., and Saunders, R. M. K. (2017). Stigmatic exudate in the Annonaceae: pollinator reward, pollen germination medium or extragynoecial compitum? J. Integr. Plant Biol. 59, 881–894. doi: 10.1111/jipb.12598
Linskens, H. F., and Stanley, R. G. (1974). Pollen: biology, biochemistry, management [by] HF Linskens [and] RG Stanley. New York, NY: Springer-Verlag.
Liu, W., Chen, L., Zhang, S., Hu, F., Wang, Z., Lyu, J., et al. (2019). Decrease of gene expression diversity during domestication of animals and plants. BMC Evol. Biol. 19:19. doi: 10.1186/s12862-018-1340-9
Lora, J., Herrero, M., and Hormaza, J. I. (2012). Pollen performance, cell number, and physiological state in the early-divergent angiosperm Annona cherimola Mill. (Annonaceae) are related to environmental conditions during the final stages of pollen development. Sexual Plant Reprod 25, 157–167. doi: 10.1007/s00497-012-0187-2
Luo, S., Zhang, K., Zhong, W.-P., Chen, P., Fan, X.-M., and Yuan, D.-Y. (2020). Optimization of in vitro pollen germination and pollen viability tests for Castanea mollissima and Castanea henryi. Scientia Horticulturae 271:109481. doi: 10.1016/j.scienta.2020.109481
Martin, F. W., and Brewbaker, J. L. (1971). The nature of the stigmatic exudate and its role in pollen germination in Pollen. Amsterdam: Elsevier, 262–266.
Montaner, C., Floris, E., and Alvarez, J. M. (2003). Study of pollen cytology and evaluation of pollen viability using in vivo and in vitro test, in borage (Borago officinalis L.). Grana 42, 33–37. doi: 10.1080/00173130310008553
Mortenson, L. R., Peloquin, S. J., and Hougas, R. W. (1964). Germination of Solanum pollen on artificial media. Am. Potato J. 41, 322–328.
Muengkaew, R., Chaiprasart, P., and Wongsawad, P. (2017). Calcium-boron addition promotes pollen germination and fruit set of mango. Intern. J. Fruit Sci. 17, 147–158. doi: 10.1080/15538362.2016.1259085
Mulcahy, G. B., and Mulcahy, D. L. (1988). The effect of supplemented media on the growth in vitro of bi-and trinucleate pollen. Plant Sci. 55, 213–216.
Munzuroglu, O., Obek, E., and Geckil, H. (2003). Effects of simulated acid rain on the pollen germination and pollen tube growth of apple (Malus sylvestris Miller cv. Golden). Acta Biol. Hung. 54, 95–103. doi: 10.1556/ABiol.54.2003.1.10
Nepi, M., Guarnieri, M., Mugnaini, S., Cresti, L., Pacini, E., and Piotto, B. (2005). A modified FCR test to evaluate pollen viability in Juniperus communis L. Grana 44, 148–151. doi: 10.1080/00173130510010576
Pacini, E. (1996). Types and meaning of pollen carbohydrate reserves. Sexual Plant Reprod 9, 362–366. doi: 10.1007/BF02441957
Pacini, E., and Dolferus, R. (2016). “The trials and tribulations of the plant male gametophyte — understanding reproductive stage stress tolerance,” in Abiotic and biotic stress in plants - recent advances and future perspectives, eds A. K. Shanker and C. Shanker (Chennai: InTech).
Pacini, E., Franchi, G. G., and Ripaccioli, M. (1999). Ripe pollen structure and histochemistry of some gymnosperms. Pl. Syst. Evol. 217, 81–99. doi: 10.1007/BF00984923
Pacini, E., Guarnieri, M., and Nepi, M. (2006). Pollen carbohydrates and water content during development, presentation, and dispersal: a short review. Protoplasma 228, 73–77. doi: 10.1007/s00709-006-0169-z
Paoletti, E., and Bellani, L. M. (1990). The in-vitro response of pollen germination and tube length to different types of acidity. Env. Pollut. 67, 279–286. doi: 10.1016/0269-7491(90)90192-F
Park, S.-Y., and Lord, E. M. (2003). Expression studies of SCA in lily and confirmation of its role in pollen tube adhesion. Plant Mol. Biol. 51, 183–189. doi: 10.1023/A:1021139502947
Procissi, A., Guyon, A., Pierson, E. S., Giritch, A., Knuiman, B., Grandjean, O., et al. (2003). Kinky pollen encodes a sabre-like protein required for tip growth in Arabidopsis and conserved among eukaryotes. Plant J. 36, 894–904. doi: 10.1046/j.1365-313X.2003.01933.x
R Core Team (2020). R: A language and environment for statistical computing. R Foundation for Statistical Computing Vienna: R Core Team.
Read, S. M., Clarke, A. E., and Bacic, A. (1993). Stimulation of growth of cultured Nicotiana tabacum W 38 pollen tubes by poly(ethylene glycol) and Cu(II) salts. Protoplasma 177, 1–14. doi: 10.1007/BF01403393
Reinders, A. (2016). Fuel for the road—sugar transport and pollen tube growth. J. Exp. Bot. 67, 2121–2123. doi: 10.1093/jxb/erw113
Rejón, J. D., Delalande, F., Schaeffer-Reiss, C., Carapito, C., Zienkiewicz, K., Alché, J. D. D., et al. (2014). The plant stigma exudate. A biochemically active extracellular environment for pollen germination? Plant Signal Behav. 9:e28274. doi: 10.4161/psb.28274
Roberts, I. N., Gaude, T. C., Harrod, G., and Dickinson, H. G. (1983). Pollen-stigma interactions in Brassica oleracea; a new pollen germination medium and its use in elucidating the mechanism of self incompatibility. Theoret. Appl. Genet. 65, 231–238.
Rodriguez-Enriquez, M. J., Mehdi, S., Dickinson, H. G., and Grant-Downton, R. T. (2013). A novel method for efficient in vitro germination and tube growth of Arabidopsis thaliana pollen. New Phytol. 197, 668–679. doi: 10.1111/nph.12037
Rosbakh, S., Pacini, E., Nepi, M., and Poschlod, P. (2018). An unexplored side of regeneration niche: seed quantity and quality are determined by the effect of temperature on pollen performance. Front. Plant Sci. 9:1036. doi: 10.3389/fpls.2018.01036
Selinski, J., and Scheibe, R. (2014). Pollen tube growth: where does the energy come from? Plant Signal Behav. 9:e977200. doi: 10.4161/15592324.2014.977200
Shaoling, Z., DiXin, C., Lang, K., and Ling, W. (2005). Effects of medium components and pH on pollen germination and tube growth in pear ({\sl Pyrus pyrifolia}). Acta Botanica Boreali-Occidentalia Sinica 25, 225–230.
Shivanna, K. R., and Rangaswamy, N. S. (1992). “Pollen germination and pollen tube growth in vitro,”,” in Pollen biology, eds K. R. Shivanna and N. S. Rangaswamy (Berlin: Springer), 23–31.
Shivanna, K. R., and Sawhney, V. K. (1995). Polyethylene glycol improves the in vitro growth of Brassica pollen tubes without loss in germination. J. Exp. Bot. 46, 1771–1774.
Shivanna, K. R., Linskens, H. F., and Cresti, M. (1991). Pollen viability and pollen vigor. Theor. Appl. Genet. 81, 38–42. doi: 10.1007/BF00226109
Shu-juan, Y. (2010). Effects of calcium and boron on pollen germination and pollen tube growth of Aloe vera L. var. chinesis [J]∗. J. Anhui Agricult. Sci. 2010:14.
Sidhu, R. J. K., and Malik, C. P. (1986). “Metabolic role of boron in germinating pollen and growing pollen tubes,” in Biotechnology and ecology of pollen, eds D. L. Mulcahy, G. B. Mulcahy, and E. Ottaviano (New York, NY: Springer), 373–378.
Silva, L. F. D. O. D., Zambon, C. R., Pio, R., Oliveira, A. F., and de Gonçalves, E. D. (2016). Establishment of growth medium and quantification of pollen grains of olive cultivars in Brazil’s subtropical areas. Bragantia 75, 26–32. doi: 10.1590/1678-4499.213
Soares, T. L., Jesus, O. N., de Santos-Serejo, J. A. D., and Oliveira, E. J. (2013). In vitro pollen germination and pollen viability in passion fruit (Passiflora spp.). Rev. Bras. Frutic. 35, 1116–1126. doi: 10.1590/S0100-29452013000400023
Song, L.-F., Zou, J.-J., Zhang, W.-Z., Wu, W.-H., and Wang, Y. (2009). Ion transporters involved in pollen germination and pollen tube tip-growth. Plant Signal Behav. 4, 1193–1195. doi: 10.4161/psb.4.12.10063
Sorkheh, K., Shiran, B., Rouhi, V., and Khodambashi, M. (2011). Influence of temperature on the in vitro pollen germination and pollen tube growth of various native Iranian almonds (Prunus L. spp.) species. Trees 25, 809–822. doi: 10.1007/s00468-011-0557-7
Souza, E. H., Souza, F. V. D., Rossi, M. L., Packer, R. M., Cruz-Barros, M. A. V., and Martinelli, A. P. (2017). Pollen morphology and viability in Bromeliaceae. An. Acad. Bras. Cienc. 89, 3067–3082. doi: 10.1590/0001-3765201720170450
Speranza, A., Calzoni, G. L., and Pacini, E. (1997). Occurrence of mono- or disaccharides and polysaccharide reserves in mature pollen grains. Sexual Plant Reprod 10, 110–115. doi: 10.1007/s004970050076
Stanley, R. G., and Linskens, H. F. (1974). Pollen: Biology Biochemistry Management. Berlin: Springer.
Steinebrunner, I., Wu, J., Sun, Y., Corbett, A., and Roux, S. J. (2003). Disruption of apyrases inhibits pollen germination in Arabidopsis. Plant Physiol. 131, 1638–1647. doi: 10.1104/pp.102.014308
Steinhorst, L., and Kudla, J. (2013). Calcium - a central regulator of pollen germination and tube growth. Biochim. Biophys. Acta 1833, 1573–1581. doi: 10.1016/j.bbamcr.2012.10.009
Stephenson, A. G., Travers, S. E., Mena-Ali, J. I., and Winsor, J. A. (2003). Pollen performance before and during the autotrophic-heterotrophic transition of pollen tube growth. Philos. Trans. R. Soc. Lond. B Biol. Sci. 358, 1009–1018. doi: 10.1098/rstb.2003.1290
Stone, J. L., Thomson, J. D., and Dent-Acosta, S. J. (1995). Assessment of pollen viability in hand-pollination experiments: A review. Am. J. Bot. 82:1186. doi: 10.2307/2446073
Sulusoglu, M., and Cavusoglu, A. (2014). In vitro pollen viability and pollen germination in cherry laurel (Prunus laurocerasus L.). Sci. World J. 2014:657123. doi: 10.1155/2014/657123
Sun, J.-F., Gong, Y.-B., Renner, S. S., and Huang, S.-Q. (2008). Multifunctional bracts in the dove tree Davidia involucrata (Nyssaceae: Cornales): rain protection and pollinator attraction. Am. Nat. 171, 119–124. doi: 10.1086/523953
Taylor, L. P., and Hepler, P. K. (1997). Pollen germination and tube growth. Annu Rev. Plant Physiol. Plant Mol. Biol. 48, 461–491. doi: 10.1146/annurev.arplant.48.1.461
Tupý, J., and Řhová, L. (1984). Changes and growth effect of pH in pollen tube culture. J. Plant Physiol. 115, 1–10. doi: 10.1016/S0176-1617(84)80045-0
Vasil, I. K. (1960). Studies on pollen germination of certain Cucurbitaceae. Am. J. Bot. 47, 239–247. doi: 10.1002/j.1537-2197.1960.tb07120.x
Wang, H.-J., Huang, J.-C., and Jauh, G.-Y. (2010). Pollen germination and tube growth. Amsterdam: Elsevier, 1–52.
Wang, Q., Lu, L., Wu, X., Li, Y., and Lin, J. (2003). Boron influences pollen germination and pollen tube growth in Picea meyeri. Tree Physiol. 23, 345–351. doi: 10.1093/treephys/23.5.345
Wani, M. S., Hamid, M., Tantray, Y. R., Gupta, R. C., Munshi, A. H., and Singh, V. (2020). In vitro pollen germination of Betula utilis, a typical tree line species in Himalayas. South Afr. J. Bot. 131, 214–221. doi: 10.1016/j.sajb.2020.02.025
Williams, J. H. (2012). Pollen tube growth rates and the diversification of flowering plant reproductive cycles. Intern. J. Plant Sci. 173, 649–661. doi: 10.1086/665822
Williams, J. H., and Reese, J. B. (2019). Evolution of development of pollen performance. Curr. Dev. Biol. 131, 299–336. doi: 10.1016/bs.ctdb.2018.11.012
Wolkers, W. F., McCready, S., Brandt, W. F., Lindsey, G. G., and Hoekstra, F. A. (2001). Isolation and characterization of a D-7 LEA protein from pollen that stabilizes glasses in vitro. Biochim. Biophys. Acta 1544, 196–206. doi: 10.1016/S0167-4838(00)00220-X
Wu, J.-Y., Jin, C., and Zhang, S.-L. (2011). Potassium flux in the pollen tubes was essential in plant sexual reproduction. Plant Signal. Behav. 6, 898–900. doi: 10.4161/psb.6.6.15322
Xu, F., Zheng, L., Yang, Z., and Zhang, S. (2020). Arabidopsis ECERIFERUM3 (CER3) functions to maintain hydration for pollen–stigma recognition during fertilization. J. Plant Biol. 63, 347–359. doi: 10.1007/s12374-020-09257-3
Yang, X., and Li, Y. (1999). Boron plays an important role in the regulation of plant cell growth. Tsinghua Sci. Technol. 4, 1583–1586.
Yao, C.-Y., and Zhao, J. (2004). Effects of calcium and boron on pollen germination and pollen tube growth of Torenia fournieri. J. Wuhan Botanical. Res. 22, 1–7.
Zaman, M. R. (2011). Effect of pH on in vitro pollen germination of fourteen cultivated and wild Species of Cucurbit. J. Bio-Sci. 17, 129–133. doi: 10.3329/jbs.v17i0.7120
Zhang, C., Fountain, D. W., and Morgan, E. R. (1997). In vitro germination of the trinucleate pollen of Limonium perezii. Grana 36, 284–288. doi: 10.1080/00173139709362618
Keywords: cookbook, experiment, medium, in vitro, pollen
Citation: Tushabe D and Rosbakh S (2021) A Compendium of in vitro Germination Media for Pollen Research. Front. Plant Sci. 12:709945. doi: 10.3389/fpls.2021.709945
Received: 14 May 2021; Accepted: 17 June 2021;
Published: 09 July 2021.
Edited by:
Vladimir Orbovic, University of Florida, United StatesReviewed by:
Maria Breygina, Lomonosov Moscow State University, RussiaTaliane Soares, State University of Feira de Santana, Brazil
Copyright © 2021 Tushabe and Rosbakh. This is an open-access article distributed under the terms of the Creative Commons Attribution License (CC BY). The use, distribution or reproduction in other forums is permitted, provided the original author(s) and the copyright owner(s) are credited and that the original publication in this journal is cited, in accordance with accepted academic practice. No use, distribution or reproduction is permitted which does not comply with these terms.
*Correspondence: Donam Tushabe, Donam.Tushabe@biologie.uni-regensburg.de
†ORCID: Sergey Rosbakh, orcid.org/0000-0002-9804-5746