- 1Department of Horticultural Sciences, Faculty of Agriculture and Environment, The Islamia University of Bahawalpur, Bahawalpur, Pakistan
- 2Environmental Horticulture Department and Mid-Florida Research and Education Center, Institute of Food and Agricultural Sciences, University of Florida, Apopka, FL, United States
- 3Institute of Horticultural Sciences, University of Agriculture Faisalabad, Faisalabad, Pakistan
- 4Dr. M. Ajmal Khan Institute of Sustainable Halophyte Utilization, University of Karachi, Karachi, Pakistan
- 5Institute of Soil Sciences, University of Agriculture Faisalabad, Faisalabad, Pakistan
- 6Institute of Plant Ecology, Justus-Liebig-University Giessen, Giessen, Germany
- 7The UWA Institute of Agriculture, The University of Western Australia, Perth, WA, Australia
Alpinia zerumbet (Zingiberaceae) is a unique ornamental and medicinal plant primarily used in food ingredients and traditional medicine. While organic amendments such as biochar (BC) and compost (Co) have been demonstrated to improve plant productivity, no studies have examined their effects on the growth, physiology, and secondary metabolites of A. zerumbet. This study evaluated the impact of the amendment of BC, Co, or a biochar and compost mixture (BC+Co) on modifying and improving the growth, photosynthesis, antioxidant status, and secondary metabolism of A. zerumbet grown on sandy loam soil. The morpho-physiological and biochemical investigation revealed variation in the response of A. zerumbet to organic amendments. The amendment of BC and BC+Co significantly increased net photosynthetic rates of plants by more than 28%, chlorophyll a and b contents by 92 and 78%, respectively, and carboxylation efficiency by 50% compared with those grown in the sandy loam soil without amendment. Furthermore, the amendment significantly decreased plant oxidative stress, measured as leaf free proline and glycine betaine. Enzymatic antioxidant activity, total phenols, and flavonoids also varied in their response to the organic amendments. In conclusion, this study shows that BC and/or Co amendments are an efficient and sustainable method for improving the metabolite contents and reducing oxidative stress in A. zerumbet.
Introduction
There is increasing interest in soil amendments, such as biochar (BC) and compost (Co) for the sustainable production of high-value medicinal plants and crops (Basak et al., 2021; Liu et al., 2021; Nigam et al., 2021). BC is a recalcitrant, black carbonaceous, porous, and low-density material produced by the pyrolysis of various biological residues, such as crop residue, wood waste, manure, food waste, forest residue, and sewage sludge (Wang et al., 2020). BC amendment to soils can improve the photosynthesis, growth, and yield of crop plants (Ali et al., 2019; He et al., 2020; Mansoor et al., 2020; Wang et al., 2021). Compost is an organic residue product produced by aerobic biological decomposition (biodegradation process). Compost amendment has been shown to alter plant metabolism, improving plant growth and productivity (Liang et al., 2021).
Plant metabolites, such as phenolics and flavonoids, are a vital group of active constituents of medicinal plants with therapeutic importance (Zulfiqar et al., 2021). These antioxidants play a crucial role in mitigating oxidative stress by regulating reactive oxygen species (Hasanuzzaman et al., 2020; Zulfiqar et al., 2021). The therapeutic properties of medicinal plants depend on these metabolites and antioxidant activity, which can be improved by amending soils with organic materials (Liu et al., 2021; Mehdizadeh et al., 2021; Nigam et al., 2021). BC application to medicinal plants has been reported to affect plant metabolites and the antioxidant system under stress conditions (Liu et al., 2021; Nigam et al., 2021). Similarly, soils amended with compost can influence these traits in medicinal plants (Burducea et al., 2019). The combined application of BC and Co could have additional benefits for improving medicinal plant production (Zulfiqar et al., 2019a). However, less is known about the interactive effects of the compost and BC application on the physiological and biochemical pathways of medicinal plants under non-stressed conditions.
This study considered shell ginger (Alpinia zerumbet (Pers.) B.L. Burtt. and R.M. Sm.), a member of the family Zingiberaceae, and a tall herbaceous crop that is widespread and important in tropical and subtropical regions of China, Taiwan, Japan, and Brazil. Shell ginger is commonly grown as a landscape and cut-foliage species. It has many pharmacological properties due to its chemical constituents, such as flavonoids, phenolic acids, phenylpropanoid glycosides, kava pyrones, sterols, and terpene (Lim, 2016; Chan et al., 2017). For instance, the fruits are used to treat gastrointestinal and cardiovascular diseases, rhizomes are consumed as spices, stem fibers are used to produce paper, kariyushi wear, and textiles, and the essential oil from leaves is used in cosmetics (e.g., perfume, soap, skincare, and deodorant) and insect repellent. To determine the beneficial effects of BC and Co on shell ginger, we investigated the effect of BC and Co, individually and combined, on the growth, physiology, and secondary metabolites of shell ginger, which will be of interest for both growers and the pharmaceutical industry.
Materials and Methods
Experimental Location and Plant Material
The experiment was established in the floriculture research area of the Institute of Horticulture, University of Agriculture Faisalabad, Pakistan (31°300 N, 73°100 E, altitude 213 m), under natural daylight conditions in typical subtropical climate conditions from April 12, 2017 to March 20, 2018. A 40% shading net was placed above the metal canopy to prevent high light intensities and temperatures on sunny days. Alpinia plants (with 18-cm height, 85-g fresh weight, six-leaf stage) produced from tissue culture were purchased from a local nursery in Pakistan (Best Garden Nursery, Faisalabad).
Selection and Preparation of Raw Material
Sandy loam soil (65 sand, 20 silt, and 15% clay) was taken from the top layer (~6–22 cm depth) of a field (31°300 N, 73°100 E, altitude 213 m), air-dried, ground, and sieved.
Biochar was produced by the slow pyrolysis of chopped wheat straw (Triticum aestivum L.) prepared at 450°C for 2-h resident time. Prior to the soil amendment, the BC was air-dried for 4 days and grounded. Compost was manufactured from plant leaf residue (the Institute of Horticultural Sciences, University of Agriculture Faisalabad, Pakistan) and sieved to <2mm. The compost and/or BC were mixed with the soil before pot filling. Plants were grown in earthen pots (with 28-cm top diameter, 24-cm base diameter, and 22-cm height). The soil, BC, and Co properties are listed in Table 1.
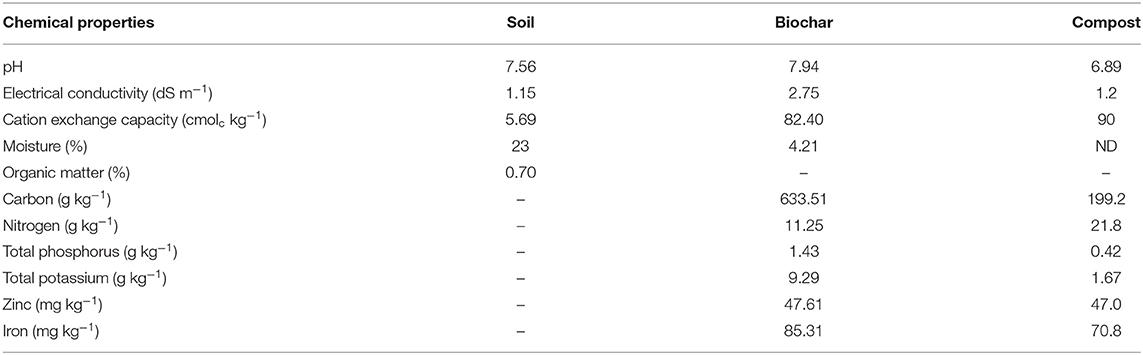
Table 1. Chemical characteristics and nutritional composition of wheat straw biochar, compost, and soil used in the study.
Preparation of Soil Potting Mixtures
Four different soil mixtures were tested as follows: (1) soil only as control (S), (2) BC soil amendment (5% by weight), (3) Co soil amendment (5% by weight), and (4) BC+Co (5 + 5% by weight).
Experimental Setup
The experiment had a randomized block design with four replications. The median light intensity received by A. zerumbet plants was about 150 μmol photons m−2 s−1, with a 95% quantile of 1,230 μmol photons m−2 s−1. The median temperature was about 25°C. Plants were tap-watered by hand on alternate days. Seedlings were provided with a half-strength Hoagland nutrient medium after 7 days. The daily photoperiod ranged from 14 to 16 h. The impact of the soil amendments on growth, gas exchange, photosynthetic pigments, and antioxidants was studied for 12 months.
Plant Sampling and Analysis
Morphological characteristics, gas exchanges, chlorophyll contents, some secondary metabolites, and activities of the oxidative-related enzymes of shell ginger plants grown in the four treatments were examined.
Plant Growth Parameters
The non-destructive vegetative parameters, such as plant height, tiller number, shoot diameter, and leaf number, were recorded at the end of the experiment (March 2018). Plant height was determined as the distance from the root base to the top of the plant. The main shoot diameter was measured with a digital Vernier caliper (India Tools and Instruments Co., Mumbai, India). Four plants (n = 4) in each treatment were separated into leaf and root tissue, and the fresh mass of each was measured with a digital balance. Leaf area was determined with an LI-3000C portable area meter (LI-COR, Lincoln, NE, USA) and subsequently used to calculate the leaf area/leaf mass ratio [specific leaf area (SLA)].
Gas Exchange Measurements
Gas exchange parameters were measured on March 12, 2018. Photosynthetic rate (Pn), stomatal conductance (gs), transpiration rate (E), internal CO2 concentration (Ci), water use efficiency (Pn/E), and carboxylation efficiency (COE, Pn/CO2) concentration were analyzed on fully expanded mature leaves (i.e., midportion; two per replicate) on a sunny day between 10:00 and 12:30 with a portable CO2 IR gas analyzer (Analytical Development Company, Hoddesdon, England).
Chlorophyll Contents
Eight days before the end of the experiment (March 12, 2018), two mature leaves per plant were excised from the middle portion of the main shoot (i.e., six replicates per treatment); 0.5 g was mortared and kept overnight (dark) in 80% acetone at −4°C. The extract was centrifuged (Z 306; HERMLE Labortechnik, Wehingen, Germany) at 10,000 × g for 5 min. The absorbance of the supernatant was read at 663 and 645 nm using a UV-1900 spectrophotometer (BMC, Canada). Chlorophyll concentrations (i.e., a, b, and total chlorophyll) were calculated following the protocol of Arnon (1949).
Leaf Free Proline Content
A mature leaf sample (0.5 g) was isolated from the middle portion of a plant (i.e., four replicates per treatment) and mixed with 10 ml of 3% (w/v) sulfosalicylic acid (MP Biomedicals, Inc., Solon, OH, USA) according to the study by Bates et al. (1973). The samples were filtered; 2.0 ml filtrate was mixed with 2.0 ml acid ninhydrin solution; and 2.0 ml glacial acetic acid (GAA) (MP Biomedicals, Inc.) in a test tube. Acid ninhydrin was prepared by mixing 1.25 g ninhydrin (C6H4COCOCOH2O, BDH, Anala R, England) with 30 ml GAA and 20 ml of 6 M H3PO4. The optical density of the filtrate was measured at 520 nm using a UV-1900 spectrophotometer (BMC, Canada).
Glycine Betaine Content
Leaf material (0.5 g) for each replicate was shaken occasionally in 10 ml toluene (0.5%) and kept at 4°C overnight. After filtration and centrifugation, 1 ml filtrate was added to 1 ml of 2 N sulfuric acid and 200 μl potassium triiodide (KI3) in a test tube. Samples were cooled at 4°C for 1 h in a chiller before adding 2.8 ml ice-cooled deionized H2O and 5 ml 1,2-dichloroethane. The absorbance of the organic layer (lower layer) was recorded spectrophotometrically (UV-1900 spectrophotometer; BMC, Canada) at 365 nm. The glycine betaine (GB) concentrations were recorded against a standard curve following the study by Grieve and Grattan (1983).
Preparation of Extracts for Biochemical Assays
Fresh leaf material (1 g) was pulverized in a mortar and extracted with 25 ml solvent (i.e., methanol, ethanol, acetone, or water). An orbital shaker (Kalsterin, YR 40, Montpellier, France) was used to continually stir at 150 × g for 24 h to homogenize the extract before centrifuging at 10,000×g for 10 min. The supernatant was filtered using the Whatman filter paper (No. 1) (Tisch Scientific, Cleves, OH, USA). The filtrate was used for further screening.
Quantification of Total Phenolic Content
The total phenolic content (TPC) of the leaf extract was quantified at 765 nm using a UV-1900 spectrophotometer (BMC, Canada), as described by Folin and Ciocalteu (1927). Briefly, 1 ml leaf extract was mixed with 4 ml sodium carbonate (20%) and 5 ml Folin–Ciocalteu solution (10%) and incubated for 1 h in a water bath. Absorbance was read at 765 nm (Optizen POP, Mecasys Co., Ltd., Korea). The TPC was expressed as gallic acid equivalents (GAE). Of note, 1 ml of each standard was mixed with 4 ml sodium carbonate (20%) and 5 ml Folin–Ciocalteu reagent, and the absorption was measured after 1 h at 765 nm using a UV-1900 spectrophotometer (BMC, Canada). A calibration curve was prepared using absorbance as a function of concentration. Finally, GAE was calculated as follows:
where T is the TPC (mg GAE per g plant extract), C is the gallic acid concentration determined from the calibration curve (mg ml−1), V is the volume of extract (ml), and M is the weight (g) of pure plant extract.
Determination of Total Flavonoid Content
The total flavonoid content (TFC) of the prepared leaf extract was estimated using the AlCl3 colorimetric method described by Chang et al. (2006) using quercetin as a standard. The extract or standard solution (0.5 ml) was mixed with 2 ml distilled water and 0.15 ml of 5% NaNO2 solution. After 6 min of incubation, 0.15 ml of 10% AlCl3 solution was added. After 6 min, 1 M NaOH was added to the mixture. Finally, 3 ml methanol was added for an end volume of 5 ml. The reaction mixture was mixed thoroughly and incubated at room temperature for 45 min. Absorbance was measured using a UV-1900 spectrophotometer (BMC, Canada) at 510 nm. The TFC was expressed as catechin equivalents from the linear regression curve of catechin.
Antioxidant Assays
Determination of 2,2-Diphenyl-1-Picrylhydrazyl (DPPH) Radical Scavenging Activity
Total free radical scavenging activity was measured using 2,2-diphenyl-1-picrylhydrazyl (DPPH) as per the method described by Yen and Chen (1995). Briefly, 0.5 g leaf tissue was homogenized in 10 ml acetone. Then, 3 ml leaf extract (i.e., three replicates) was added to 1 ml DPPH methanol solution (0.004%), vigorously shaken, and incubated in the dark for 30 min at room temperature. Absorbance was read at 517 nm using a UV-1900 spectrophotometer (BMC, Canada). A low absorbance reading indicates high radical scavenging activity. DPPH inhibition was calculated as follows:
Determination of Reducing Power Assay
The reducing power capacity of the leaf extract was evaluated by direct electron donation to reduce Fe3+ (CN)6 to Fe2+ (CN)6 using the method described by Yadav et al. (2014). Briefly, 1 ml prepared extract was mixed with 2.5 ml potassium ferricyanide (1%) and 2.5 ml phosphate buffer (0.2 M, pH 6.6). The mixture was incubated in a water bath at 50°C for 20 min before adding 2.5 ml trichloroacetic acid (1% w/v). The reaction mixture was centrifuged at 3,000 × g for 10 min. The supernatant (2.5 ml) was mixed with 0.5 ml ferric chloride (0.1%) and 2.5 ml deionized water. Absorbance was read at 700 nm using a UV-1900 spectrophotometer (BMC, Canada).
Enzymatic Antioxidant Extraction and Assay
Leaf sample (0.5 g) was homogenized in 4 ml sodium phosphate buffer (0.05 M, pH 7.8), comprising 2% (w/v) polyvinylpyrrolidone (PVP) and 1.0 mM ethylenediaminetetraacetic acid (EDTA). The homogenate was centrifuged (Z 306; HERMLE Labortechnik, Wehingen, Germany) at 10,000 × g for 17 min at 4°C. The supernatant (i.e., protein extract) was used in the catalase (CAT) and peroxidase (POD) assays and to determine total soluble proteins (TSP).
Catalase Activity
Catalase activity was determined according to the method described by Chance and Maehly (1955). Briefly, 0.1 ml of the reaction mixture contains 0.9 ml of 5.9 mM H2O2, 2 ml of 50 mM phosphate buffer, and 0.1 ml protein extract. The changes in absorbance were measured at 240 nm using a UV-1900 spectrophotometer (BMC, Canada). Absorbance was read at 30-s intervals for 5 min to study H2O2 decomposition, reflecting CAT activity (μmol min−1 mg−1 protein).
Peroxidase Activity
Peroxidase activity was determined in leaf extracts using the method of Chance and Maehly (1955). The reaction mixture contained 0.1 ml protein extract, 0.4 ml guaiacol (20 mM), 0.5 ml H2O2 (40 mM), and 2 ml sodium phosphate buffer (50 mM). Absorbance was read at 470 nm in 20-s intervals with a UV-1900 spectrophotometer (BMC, Canada). The absorbance slope was used to calculate POD activity (μmol min−1 mg−1 protein).
Superoxide Dismutase Activity
Superoxide dismutase activity was assayed using the method of Van Rossum et al. (1997). The reaction mixture contained 0.4 ml distilled water, 0.1 ml methionine, 0.1 ml Triton-X, 0.25 ml phosphate buffer (pH 7.8), 0.5 ml nitro blue tetrazolium, 0.5 ml riboflavin, and 0.5 ml protein extract. The mixture was kept in the light for 20 min before recording the absorbance at 560 nm.
Total Soluble Proteins
Total soluble proteins were determined according to the protocol described by Bradford (1976). The absorbance of the prepared reaction mixture (0.2 ml protein extract, 0.02 ml Coomassie blue dye, and 0.78 ml deionized water) was read at 595 nm with a UV-1900 spectrophotometer (BMS, Canada).
Statistical Analysis
All collected data were subjected to ANOVA using SPSS software version 11.0 (SPSS, Chicago, IL, USA). If significance occurred among treatments, means were separated by the least significance difference (LSD) at p < 0.05 level. SigmaPlot software version 12.0 (Systat, San Jose, CA, USA) was used to display the means and SE of the dataset.
Results
Effect of Organic Amendments on Plant Growth
The application of BC and/or Co significantly affected the measured growth parameters of plants. The BC and BC+Co treatments increased plant height by 40 and 47%, respectively, relative to the control (Figure 1). The BC, Co, and BC+Co treatments increased tiller number (about two-fold) and stem diameter, compared to the control, more so in the two BC treatments (Figure 1). Leaf fresh weights increased in the three amended treatments, relative to the control, more so in the BC+Co treatment (Table 2). The BC and BC+Co treatments increased leaf numbers by 65% compared to the control. Leaf areas of plants grown in the three amendment media were more than doubled with respect to those of plants grown in the control medium. The SLA of plants grown in BC+Co was the highest among the treatments (Table 2).
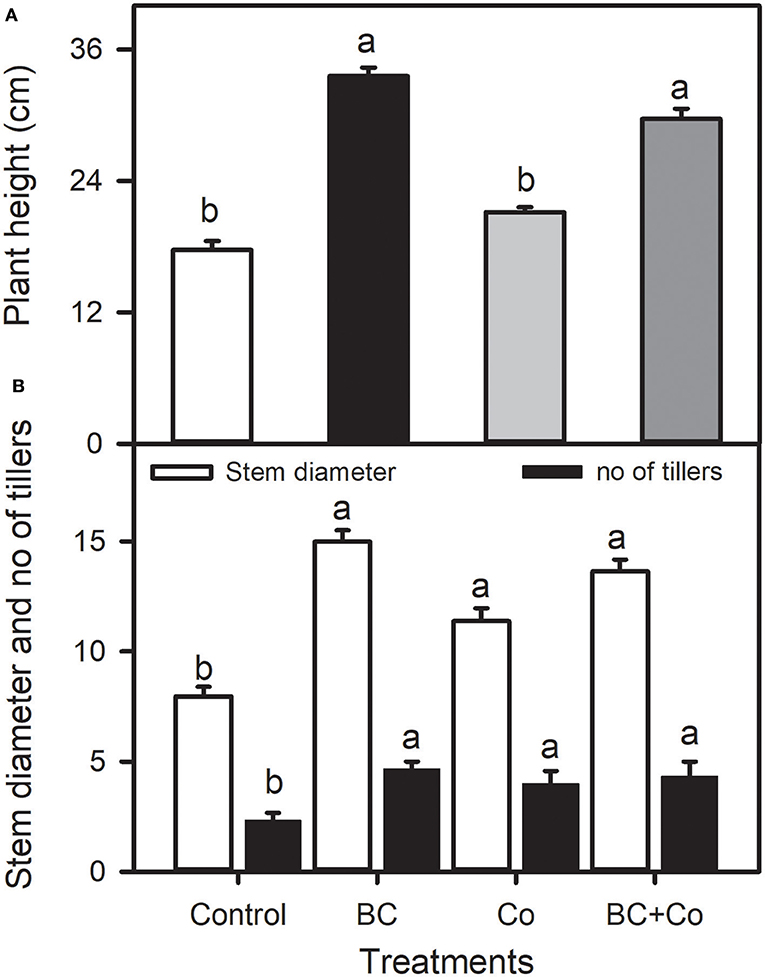
Figure 1. Plant height (A), and stem diameter and tiller number (B) of Alpinia zerumbet treated with biochar (BC), compost (Co), and biochar+compost mixture (BC+Co). Data are the mean ± SE (n = 6). Different letters above the bars represent significant differences according to the least significance difference (LSD) post hoc test at the p < 0.05 level.

Table 2. Impact of organic amendments (biochar, compost, and biochar+compost) on growth parameters (means ± SE) of Alpinia zerumbet.
Effect of Organic Amendments on Leaf Gas Exchange
Net photosynthesis rate (Pn) increased by 19 and 26% in the BC and BC+Co treatments, respectively, compared to control (Table 3). The Co treatment did not significantly increase Pn, relative to the control (Table 3). Stomatal conductance (gs) decreased (32%) sharply only in the Co treatment. Internal CO2 concentration declined in the three amended treatments, relative to the control. COE increased by 15% in the BC and BC+Co treatments. Transpiration rate (E) increased by 40, 12, and 57%, respectively, in the BC, Co, and BC+Co treatments, relative to the control (Table 3).
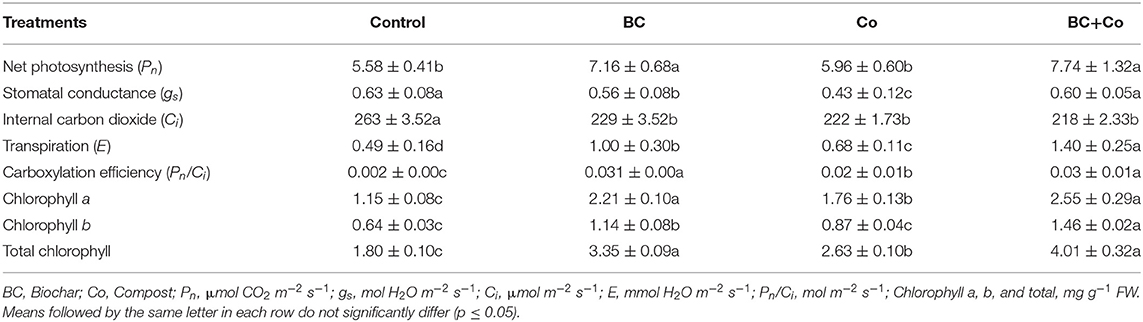
Table 3. Impact of organic amendments (biochar, compost, and biochar+compost) on leaf gas exchange and chlorophyll contents (means ± SE) of A. zerumbet.
Effect of Organic Amendments on Chlorophyll Contents
Chlorophyll a, b, and total chlorophyll contents increased in the three amended treatments, more so in the BC+Co treatment, relative to the control (Table 3).
Effect of Organic Amendments on Biochemical Parameters
Leaf free proline and GB contents showed a differential decrease response in the three amended treatments, relative to the control (Figure 2). The TFC increased 1.5-fold in the BC treatment but did not change in the Co or BC+Co treatments, compared to the control (Figure 3). TPC increased by 15% in the BC and BC+Co treatments, but the Co treatment did not influence this trait, relative to the control. The TSP in plants grown in BC were similar to those grown in the control medium but decreased in the Co and BC+Co treatments by 15%, relative to the control (Figure 3).
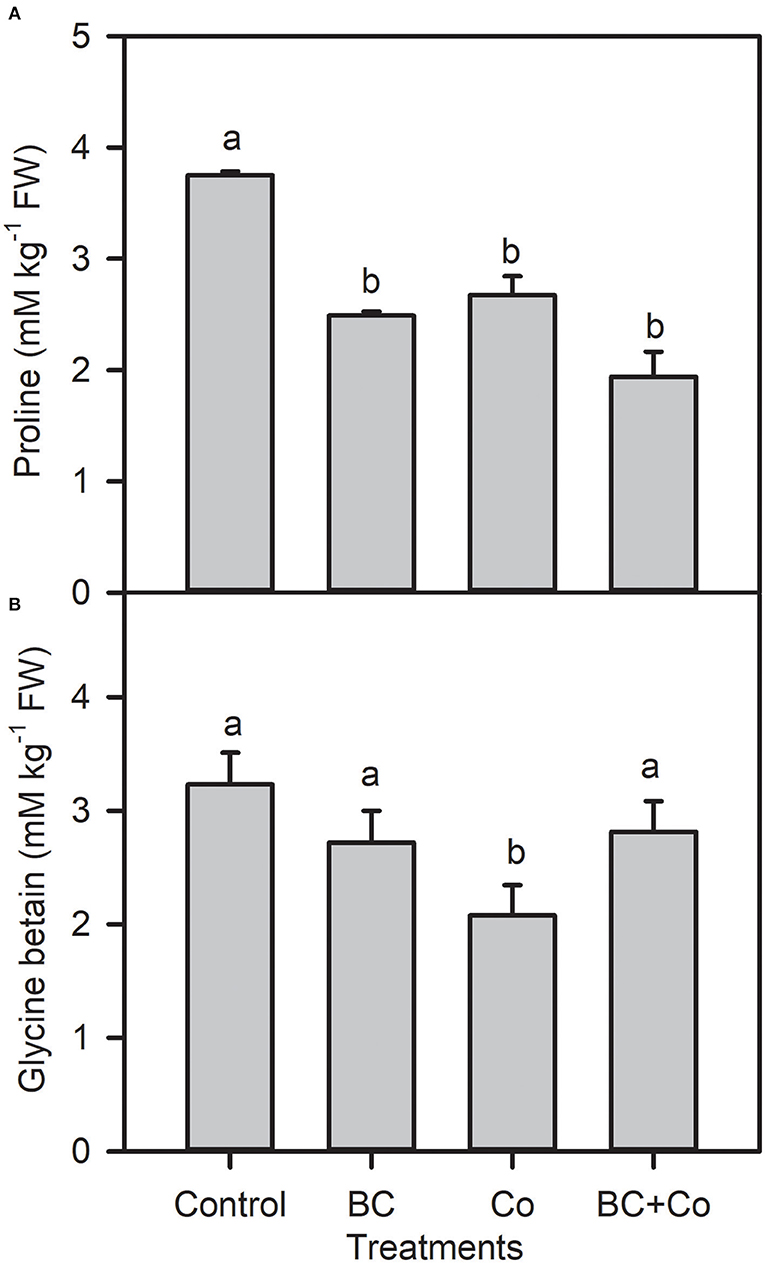
Figure 2. Leaf proline (A) and glycine betaine (B) contents of A. zerumbet treated with BC, Co, and BC+Co. Data are the mean ± SE (n = 6). Different letters above the bars represent significant differences according to the LSD post hoc test at the p < 0.05 level.
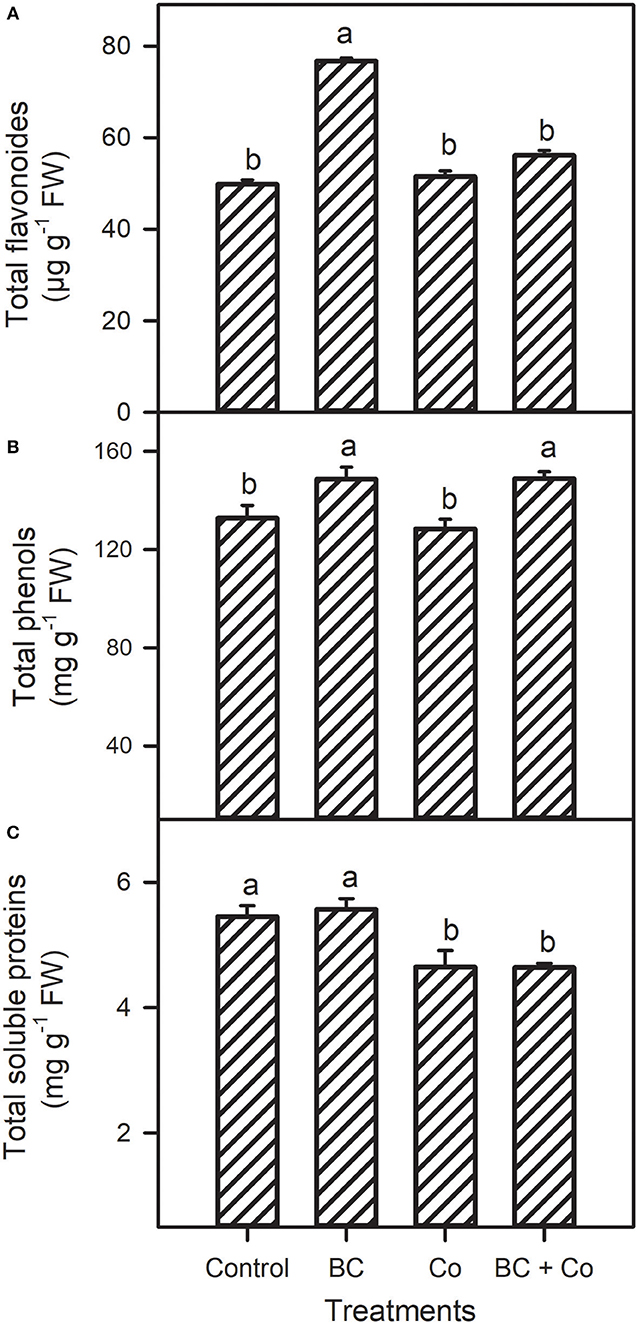
Figure 3. Leaf total flavonoids (A), phenolics (B), and soluble proteins (C) of A. zerumbet treated with BC, Co, and BC+Co. Data are the mean ± SE (n = 6). Different letters above the bars represent significant differences according to the LSD post hoc test at the p < 0.05 level.
Antioxidant capacity by DPPH declined in the three amended treatments, relative to the control. The antioxidant activity by reducing power assay increased in the BC and BC+Co treatments more than the Co treatment (Figure 4). The CAT activity did not change in any treatment, with respect to the control, while POD increased by 60% and SOD activities increased by 18% in plants grown in BC+Co medium compared with those grown in the control medium. SOD of plants grown in BC also increased by 18% compared to the control medium (Figure 5).
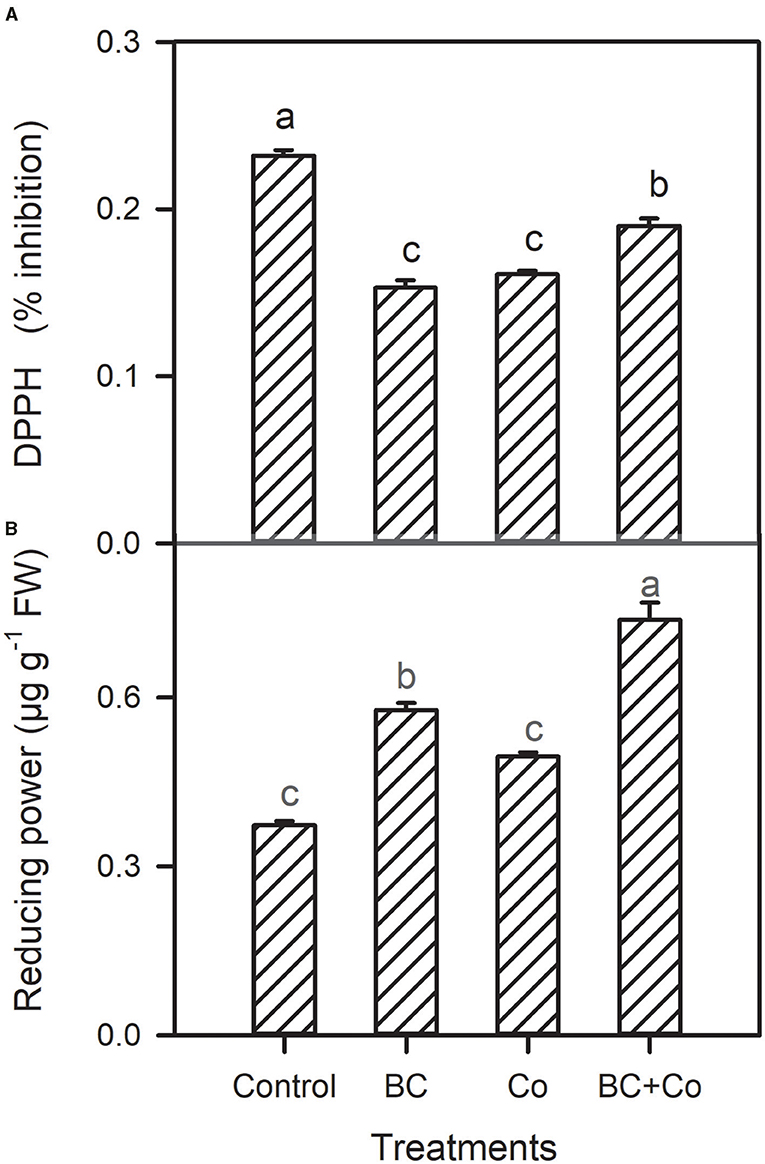
Figure 4. Leaf DPPH % inhibition (A) and reducing power (B) of A. zerumbet plants treated with BC, Co, and BC+Co. Data are the mean ± SE (n = 6). Different letters above the bars represent significant differences according to the LSD post hoc test at the p < 0.05 level.
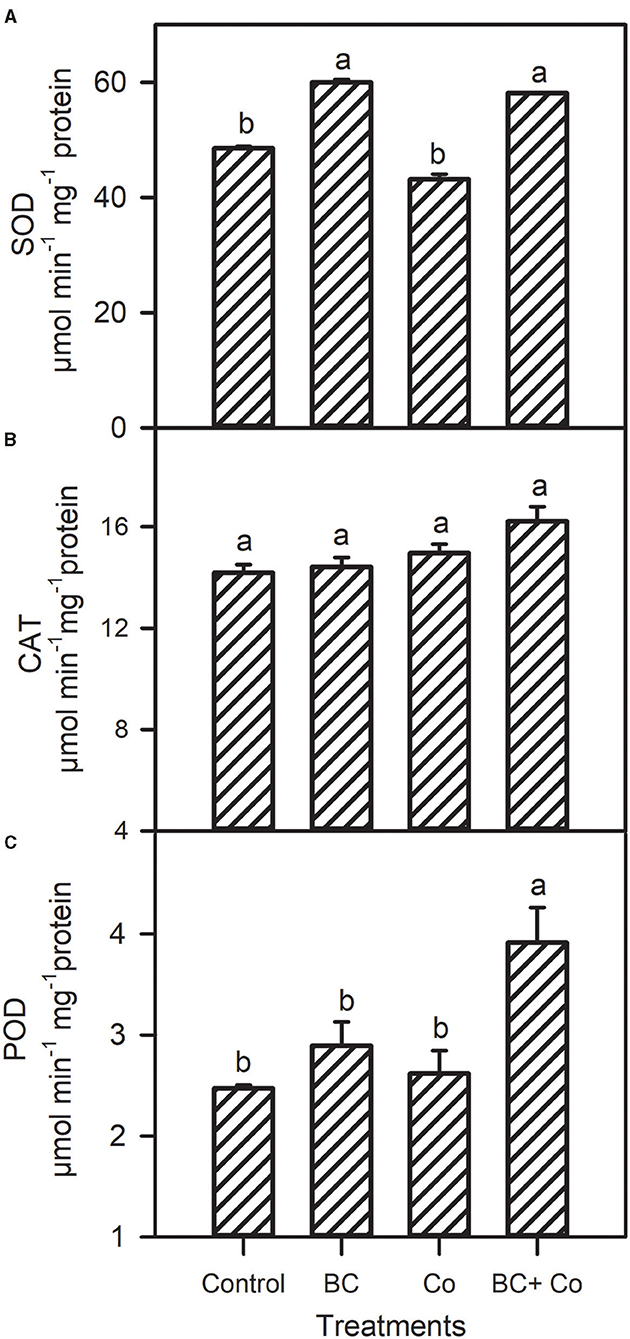
Figure 5. Superoxide dismutase (SOD) (A), catalase (CAT) (B), and peroxidase (POD) (C) antioxidant enzyme activities of A. zerumbet plants treated with BC, Co, and BC+Co. Data are the mean ± SE (n = 6). Different letters above the bars represent significant differences according to the LSD post hoc test at the p < 0.05 level.
Discussion
Plant Growth
The application of BC, Co, and BC+Co increased total biomass in A. zerumbet. The increase in plant height, tiller number, stem diameter, and leaf and root fresh weights in response to organic amendments could be associated with enhanced photosynthesis, as reported elsewhere (Agegnehu et al., 2016; Singh et al., 2018; Sánchez-Monedero et al., 2019; Rasool et al., 2021a). In contrast, Schmidt et al. (2014) reported a small and mostly nonsignificant effect of BC and combined applications of BC and Co on grapevines grown in nutrient-poor soil.
The organic soil amendment increased leaf fresh weight in A. zerumbet due to changes in various leaf-related parameters, such as an increased leaf canopy and foliage area to facilitate net photosynthesis, particularly in the BC+Co treatment. In contrast, the presence of BC and Co in the growth medium had a smaller increase in leaf fresh weight.
The BC+Co treatment improved root development in A. zerumbet more than BC or Co alone. This is consistent with previous studies where combined BC and Co application increased soil moisture and nutrient retention and thus root growth more than individual applications (Sorrenti et al., 2019; Abideen et al., 2020a; Teodoro et al., 2020). The positive effect of BC on root growth could be due to changes in physical soil condition, such as soil pH, water holding capacity, and hormonal effects, thus accelerating root growth and improving overall growth (Somerville et al., 2020).
Physiological Attributes
Higher photosynthetic rates in A. zerumbet were observed in the BC (19%) and BC+Co (26%) treatments than the compost treatment, which was similar to the control and could be related to improved COE at decreased intracellular CO2 concentration. Other studies have also reported higher Pn with BC and BC+Co amendments relative to the control (Xu et al., 2015; Seehausen et al., 2017).
The BC+Co mixture increased transpiration the most in A. zerumbet, while Co alone had no effect. BC alone and combined increased soil water availability and soil water holding capacity, thus increasing transpiration. Hence, higher transpiration was associated with higher Pn for Alpinia plants grown in BC+Co. Plants grown in soil amended with BC and Co can minimize substantial water losses through stomatal closure and transpiration (Kammann and Graber, 2015), which helps maintain water balance and leaf turgidity. Thus, organic soil improvements ultimately support photosynthetic performance (Xu et al., 2015), while decreasing Ci, Ci/Ca, and gs.
Chlorophyll a and b contents of Alpinia increased in the BC and BC+Co treatments, as reported for Phragmites karka (Agegnehu et al., 2016). Higher chlorophyll contents are accompanied by higher Pn in plants grown in BC and BC+Co. The high chlorophyll contents in Alpinia could be related to improved soil physical and chemical properties that facilitated the plant absorption of nutrients, especially nitrogen concentration (Almaroai and Eissa, 2020), and may increase light absorption to increase photosynthesis. Increased chlorophyll contents act as a proxy for leaf maximum carboxylation rate, which could be supported by increased water flux and carbon acquisition with BC and Co application to soil. Increased chlorophyll in the presence of BC and Co triggers nitrogen supply for photosynthetic enzymes and indirectly enhances COE (Luo et al., 2018).
Biochemical Parameters
Total flavonoid and polyphenol contents increased in the BC treatments but were unaffected or decreased in the sole Co treatment. The synthesis of phenolic compounds was associated with higher antioxidant activity (reducing power activity), which helps plants detoxify reactive oxygen species, indicating that BC is useful for enhancing plant antioxidant capability and protecting plants from oxidative stress by increasing phenolic acid concentrations. The antioxidative balance is important for optimal leaf photosynthesis and biomass production, as reflected in the higher POD and SOD activities in Alpinia amended with BC+Co, which increased plant biomass. Plant antioxidants act as a natural defense system against various stresses that induce excessive production of reactive oxygen species (Ahmad et al., 2010, 2019; Kohli et al., 2019; Zulfiqar and Ashraf, 2020). Numerous studies have shown that organic amendments improve the systematic resistance of antioxidant enzymes in plants (Quartacci et al., 2017; Rehman et al., 2019; Zulfiqar et al., 2019b; Abideen et al., 2020a,b; Rasool et al., 2021b).
Plants subjected to climatic variations could accumulate osmotic substances in cells, such as proline, soluble sugars, and various betaines, which function as osmoprotectants (Zulfiqar et al., 2020). In Alpinia, the soil organic amendments decreased proline and GB accumulation due to osmoprotectant mechanisms. The accumulation of nitrogenous compounds also declined, which was related to strong enzymatic and non-enzymatic defense in Alpinia. An elevated plant antioxidative defense system is related to reduced energy demands for catabolism and increased demand for anabolism (biomass synthesis) with BC and Co amendment (Ali et al., 2017; Quartacci et al., 2017; Huang et al., 2019).
Conclusions
Organic soil amendments (i.e., BC, Co, and BC+Co) had a positive effect on the growth and physiobiochemical response of A. zerumbet. In particular, BC+Co significantly increased the growth, chlorophyll content, photosynthesis, and antioxidant defense system activity, and it also reduced the proline and GB accumulation (Table 4). The results support the view that BC alone or combined with Co alters the physiobiochemical characteristics of A. zerumbet. Further research studies are needed to evaluate the agronomic and environmental benefits of A. zerumbet supplied with different feedstock-based BCs at different concentrations and combined with compost, especially under field conditions.
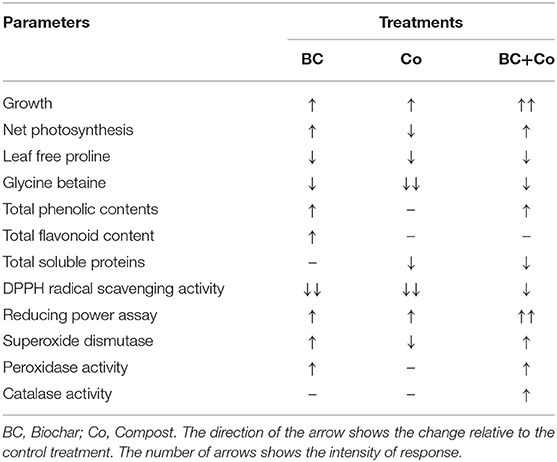
Table 4. Representation of changes in different parameters of A. zerumbet after treatment with biochar, compost, and biochar+compost.
Data Availability Statement
The raw data supporting the conclusions of this article will be made available by the authors, without undue reservation.
Author Contributions
FZ designed and undertook the experiment, contributed to the collection and interpretation of data, and drafted the manuscript. All authors contributed to editing and revising the manuscript, and they read and approved the final manuscript.
Conflict of Interest
The authors declare that the research was conducted in the absence of any commercial or financial relationships that could be construed as a potential conflict of interest.
Publisher's Note
All claims expressed in this article are solely those of the authors and do not necessarily represent those of their affiliated organizations, or those of the publisher, the editors and the reviewers. Any product that may be evaluated in this article, or claim that may be made by its manufacturer, is not guaranteed or endorsed by the publisher.
References
Abideen, Z., Koyro, H. W., Huchzermeyer, B., Ansari, R., Zulfiqar, F., and Gul, B. (2020b). Ameliorating effects of biochar on photosynthetic efficiency and antioxidant defence of Phragmites karka under drought stress. Plant. Biol. 22, 259–266. doi: 10.1111/plb.13054
Abideen, Z., Koyro, H. W., Huchzermeyer, B., Gul, B., and Khan, M. A. (2020a). Impact of a biochar or a biochar-compost mixture on water relation, nutrient uptake, and photosynthesis of Phragmites karka. Pedosphere 30, 466–477. doi: 10.1016/S1002-0160(17)60362-X
Agegnehu, G., Bass, A. M., Nelson, P. N., and Bird, M. I. (2016). Benefits of biochar, compost and biochar–compost for soil quality, maize yield and greenhouse gas emissions in a tropical agricultural soil. Sci. Total. Environ. 543, 295–306. doi: 10.1016/j.scitotenv.2015.11.054
Ahmad, P., Jaleel, C. A., Salem, M. A., Nabi, G., and Sharma, S. (2010). Roles of enzymatic and nonenzymatic antioxidants in plants during abiotic stress. Crit. Rev. Biotechnol. 30, 161–175. doi: 10.3109/07388550903524243
Ahmad, P., Tripathi, D. K., Deshmukh, R., Singh, V. P., and Corpas, F. J. (2019). Revisiting the role of ROS and RNS in plants under changing environment. Environ. Exp. Bot. 161, 1–3. doi: 10.1016/j.envexpbot.2019.02.017
Ali, A., Guo, D., Zhang, Y., Sun, X., Jiang, S., Guo, Z., et al. (2017). Using bamboo biochar with compost for the stabilization and phytotoxicity reduction of heavy metals in mine-contaminated soils of China. Sci. Rep. 7, 1–12. doi: 10.1038/s41598-017-03045-9
Ali, S., Rizwan, M., Noureen, S., Anwar, S., Ali, B., Naveed, M., et al. (2019). Combined use of biochar and zinc oxide nanoparticles foliar spray improved the plant growth and decreased the cadmium accumulation in rice (Oryza sativa L.) plant. Environ. Sci. Pollut. Res. 26, 11288–11299. doi: 10.1007/s11356-019-04554-y
Almaroai, Y. A., and Eissa, M. A. (2020). Effect of biochar on yield and quality of tomato grown on a metal-contaminated soil. Sci. Hortic. 265:109210. doi: 10.1016/j.scienta.2020.109210
Arnon, D. T. (1949). Copper enzyme in isolated chloroplasts polyphenol oxidase in Beta vulgaris. Plant Physiol. 24, 1–15. doi: 10.1104/pp.24.1.1
Basak, B. B., Saha, A., Sarkar, B., Kumar, B. P., Gajbhiye, N. A., and Banerjee, A. (2021). Repurposing distillation waste biomass and low-value mineral resources through biochar-mineral-complex for sustainable production of high-value medicinal plants and soil quality improvement. Sci. Total Environ. 760:14339. doi: 10.1016/j.scitotenv.2020.143319
Bates, L. S., Waldren, R. P., and Teare, I. D. (1973). Rapid determination of free proline for water-stress studies. Plant Soil. 39, 205–207. doi: 10.1007/BF00018060
Bradford, M. M. (1976). A rapid and sensitive method for the quantitation of microgram quantities of protein utilizing the principle of protein-dye binding. Anal. Biochem. 72, 248–254. doi: 10.1016/0003-2697(76)90527-3
Burducea, M., Zheljazkov, V. D., Lobiuc, A., Pintilie, C. A., Virgolici, M., Silion, M., et al. (2019). Biosolids application improves mineral composition and phenolic profile of basil cultivated on eroded soil. Sci. Hortic. 249, 407–418. doi: 10.1016/j.scienta.2019.02.004
Chan, E. W. C., Wong, S. K., and Chan, H. T. (2017). Alpinia zerumbet, a ginger plant with a multitude of medicinal properties: An update on its research findings. J. Chin. Pharm. Sci. 26, 775–788. doi: 10.5246/jcps.2017.11.088
Chance, B., and Maehly, A. C. (1955). Assay of catalases and peroxidases. Meth. Enzymol. 2, 764–775. doi: 10.1016/S0076-6879(55)02300-8
Chang, C. H., Lin, H. Y., Chang, C. Y., and Liu, Y. C. (2006). Comparisons on the antioxidant properties of fresh, freeze-dried and hot-air-dried tomatoes. J. Food Eng. 77, 478–485. doi: 10.1016/j.jfoodeng.2005.06.061
Folin, O., and Ciocalteu, V. (1927). On tyrosine and tryptophane determinations in proteins. J. Biol. Chem. 73, 627–650. doi: 10.1016/S0021-9258(18)84277-6
Grieve, C. M., and Grattan, S. R. (1983). Rapid assay for determination of water soluble quaternary ammonium compounds. Plant Soil. 70, 303–307. doi: 10.1007/BF02374789
Hasanuzzaman, M., Bhuyan, M. H. M. B., Zulfiqar, F., Raza, A., Mohsin, S. M., Mahmud, J. A., et al. (2020). Reactive oxygen species and antioxidant defense in plants under abiotic stress: revisiting the crucial role of a universal defense regulator. Antioxidants 9:681. doi: 10.3390/antiox9080681
He, Y., Yao, Y., Ji, Y., Deng, J., Zhou, G., Liu, R., et al. (2020). Biochar amendment boosts photosynthesis and biomass in C3 but not C4 plants: A global synthesis. GCB Bioenergy 12, 605–617. doi: 10.1111/gcbb.12720
Huang, M., Zhang, Z., Zhu, C., Zhai, Y., and Lu, P. (2019). Effect of biochar on sweet corn and soil salinity under conjunctive irrigation with brackish water in coastal saline soil. Sci. Hortic. 250, 405–413. doi: 10.1016/j.scienta.2019.02.077
Kammann, C., and Graber, E. R. (2015). “Biochar effects on plant ecophysiology,” in Biochar for Environmental Management: Science, Technology and Implementation, eds J. Lehmann and S. Joseph (Abingdon: Routledge), 391–420.
Kohli, S. K., Khanna, K., Bhardwaj, R., Abd_Allah, E. F., Ahmad, P., and Corpas, F. J. (2019). Assessment of subcellular ROS and NO metabolism in higher plants: multifunctional signaling molecules. Antioxidants 8:641. doi: 10.3390/antiox8120641
Liang, J. F., Li, Q. W., Gao, J. Q., Feng, J. G., Zhang, X. Y., Hao, Y. J., et al. (2021). Biochar-compost addition benefits Phragmites australis growth and soil property in coastal wetlands. Sci. Total Environ. 769:145166. doi: 10.1016/j.scitotenv.2021.145166
Lim, T. K. (2016). “Alpinia zerumbet,” in Edible Medicinal and Non-Medicinal Plants (Cham: Springer), 196–213.
Liu, D., Ding, Z., Ali, E. F., Kheir, A. M., Eissa, M. A., and Ibrahim, O. H. (2021). Biochar and compost enhance soil quality and growth of roselle (Hibiscus sabdariffa L.) under saline conditions. Sci. Rep. 11:6. doi: 10.1038/s41598-021-88293-6
Luo, X., Croft, H., Chen, J. M., Bartlett, P., Staebler, R., and Froelich, N. (2018). Incorporating leaf chlorophyll content into a two-leaf terrestrial biosphere model for estimating carbon and water fluxes at a forest site. Agric. Forest. Meteorol. 248, 156–168. doi: 10.1016/j.agrformet.2017.09.012
Mansoor, S., Kour, N., Manhas, S., Zahid, S., Wani, O. A., et al. (2020). Biochar as a tool for effective management of drought and heavy metal toxicity. Chemosphere 271:129458. doi: 10.1016/j.chemosphere.2020.129458
Mehdizadeh, L., Farsaraei, S., and Moghaddam, M. (2021). Biochar application modified growth and physiological parameters of Ocimum ciliatum L. and reduced human risk assessment under cadmium stress. J. Hazard. Mater. 409:124954. doi: 10.1016/j.jhazmat.2020.124954
Nigam, N., Khare, P., Ahsan, M., Yadav, V., et al. (2021). Biochar amendment reduced the risk associated with metal uptake and improved metabolite content in medicinal herbs. Physiol. Plant. 13393. doi: 10.1111/ppl.13393
Quartacci, M. F., Sgherri, C., and Frisenda, S. (2017). Biochar amendment affects phenolic composition and antioxidant capacity restoring the nutraceutical value of lettuce grown in a copper-contaminated soil. Sci. Hortic. 215, 9–14. doi: 10.1016/j.scienta.2016.12.002
Rasool, M., Akhter, A., and Haider, M. S. (2021b). Molecular and biochemical insight into biochar and Bacillus subtilis induced defense in tomatoes against Alternaria solani. Sci. Hort. 285:110203. doi: 10.1016/j.scienta.2021.110203
Rasool, M., Akhter, A., Soja, G., and Haider, M. S. (2021a). Role of biochar, compost and plant growth promoting rhizobacteria in the management of tomato early blight disease. Sci. Rep. 11:6092. doi: 10.1038/s41598-021-85633-4
Rehman, M., Liu, L., Bashir, S., Saleem, M. H., Chen, C., Peng, D., et al. (2019). Influence of rice straw biochar on growth, antioxidant capacity and copper uptake in ramie (Boehmeria nivea L.) grown as forage in aged copper-contaminated soil. Plant Physiol. Biochem. 138, 121–129. doi: 10.1016/j.plaphy.2019.02.021
Sánchez-Monedero, M. A., Cayuela, M. L., Sánchez-García, M., Vandecasteele, B., D'Hose, T., and López, G. (2019). Agronomic evaluation of biochar, compost and biochar-blended compost across different cropping systems: Perspective from the European project FERTIPLUS. Agronomy 9:225. doi: 10.3390/agronomy9050225
Schmidt, H. P., Kammann, C., Niggli, C., Evangelou, M. W., Mackie, K. A., and Abiven, S. (2014). Biochar and biochar-compost as soil amendments to a vineyard soil: Influences on plant growth, nutrient uptake, plant health and grape quality. Agric. Ecosys. Environ. 191, 117–123. doi: 10.1016/j.agee.2014.04.001
Seehausen, M. L., Gale, N. V., Dranga, S., Hudson, V., Liu, N., Michener, J., et al. (2017). Is there a positive synergistic effect of biochar and compost soil amendments on plant growth and physiological performance? Agronomy 7:13. doi: 10.3390/agronomy7010013
Singh, C., Tiwari, S., Gupta, V. K., and Singh, J. S. (2018). The effect of rice husk biochar on soil nutrient status, microbial biomass and paddy productivity of nutrient poor agriculture soils. Catena 171, 485–493. doi: 10.1016/j.catena.2018.07.042
Somerville, P. D., Farrell, C., May, P. B., and Livesley, S. J. (2020). Biochar and compost equally improve urban soil physical and biological properties and tree growth, with no added benefit in combination. Sci. Total Environ. 706:135736. doi: 10.1016/j.scitotenv.2019.135736
Sorrenti, G., Muzzi, E., and Toselli, M. (2019). Root growth dynamic and plant performance of nectarine trees amended with biochar and compost. Sci. Hortic. 257:108710. doi: 10.1016/j.scienta.2019.108710
Teodoro, M., Trakal, L., Gallagher, B. N., Šimek, P., Soudek, P., Pohorelý, M., et al. (2020). Application of co-composted biochar significantly improved plant-growth relevant physical/chemical properties of a metal contaminated soil. Chemosphere 242:125255. doi: 10.1016/j.chemosphere.2019.125255
Van Rossum, M. W. P. C., Alberda, M., and Van der Plas, L. H. W. (1997). Role of oxidative damage in tulip bulb scale micropropagation. Plant Sci. 130, 207–216. doi: 10.1016/S0168-9452(97)00215-X
Wang, D., Jiang, P., Zhang, H., and Yuan, W. (2020). Biochar production and applications in agro and forestry systems: a review. Sci. Total Environ. 723. doi: 10.1016/j.scitotenv.2020.137775
Wang, S., Zheng, J., Wang, Y., Yang, Q., Chen, T., Chen, Y., et al. (2021). Photosynthesis, chlorophyll fluorescence, and yield of peanut in response to biochar application. Front. Plant Sci. 12:1000. doi: 10.3389/fpls.2021.650432
Xu, C. Y., Hosseini-Bai, S., Hao, Y., Rachaputi, R. C., Wang, H., Xu, Z., et al. (2015). Effect of biochar amendment on yield and photosynthesis of peanut on two types of soils. Environ. Sci. Pollut. Res. 22, 6112–6125. doi: 10.1007/s11356-014-3820-9
Yadav, M., Yadav, A., and Yadav, J. P. (2014). In vitro antioxidant activity and total phenolic content of endophytic fungi isolated from Eugenia jambolana Lam. Asian Pac. J. Trop. Med. 7, S256–S261. doi: 10.1016/S1995-7645(14)60242-X
Yen, G. C., and Chen, H. Y. (1995). Antioxidant activity of various tea extracts in relation to their antimutagenicity. J. Agric. Food Chem. 43, 27–32. doi: 10.1021/jf00049a007
Zulfiqar, F., Akram, N. A., and Ashraf, M. (2020). Osmoprotection in plants under abiotic stresses: new insights into a classical phenomenon. Planta 251:3. doi: 10.1007/s00425-019-03293-1
Zulfiqar, F., and Ashraf, M. (2020). Bioregulators: unlocking their potential role in regulation of the plant oxidative defense system. Plant Mol. Biol. 105, 11–41. doi: 10.1007/s11103-020-01077-w
Zulfiqar, F., Chen, J., Finnegan, P. M., Younis, A., Nafees, M., Zorrig, W., et al. (2021). Application of trehalose and salicylic acid mitigates drought stress in sweet basil and improves plant growth. Plants 10:1078. doi: 10.3390/plants10061078
Zulfiqar, F., Younis, A., Asif, M., Abideen, Z., Allaire, S. E., and Shao, Q. S. (2019a). Evaluation of container substrates containing compost and biochar for ornamental plant Dracaena deremensis. Pak. J. Agric. Sci. 56, 613–619. doi: 10.21162/PAKJAS/19.7515
Keywords: antioxidant system, medicinal plant, organic amendments, Zingiberaceae, proline, glycine betaine
Citation: Zulfiqar F, Chen J, Younis A, Abideen Z, Naveed M, Koyro H-W and Siddique KHM (2021) Biochar, Compost, and Biochar–Compost Blend Applications Modulate Growth, Photosynthesis, Osmolytes, and Antioxidant System of Medicinal Plant Alpinia zerumbet. Front. Plant Sci. 12:707061. doi: 10.3389/fpls.2021.707061
Received: 08 May 2021; Accepted: 26 July 2021;
Published: 23 August 2021.
Edited by:
María Serrano, Miguel Hernández University of Elche, SpainReviewed by:
Salar Farhangi-Abriz, University of Tabriz, IranParvaiz Ahmad, Sri Pratap College Srinagar, India
Copyright © 2021 Zulfiqar, Chen, Younis, Abideen, Naveed, Koyro and Siddique. This is an open-access article distributed under the terms of the Creative Commons Attribution License (CC BY). The use, distribution or reproduction in other forums is permitted, provided the original author(s) and the copyright owner(s) are credited and that the original publication in this journal is cited, in accordance with accepted academic practice. No use, distribution or reproduction is permitted which does not comply with these terms.
*Correspondence: Faisal Zulfiqar, ch.faisal.zulfiqar@gmail.com, orcid.org/0000-0001-5428-5695; Kadambot H. M. Siddique, kadambot.siddique@uwa.edu.au; orcid.org/0000-0001-6097-4235