- 1Department of Bioenergy Science and Technology, Chonnam National University, Gwangju, South Korea
- 2Department of Integrative Food, Bioscience and Technology, Chonnam National University, Gwangju, South Korea
- 3Kumho Life Science Laboratory, Chonnam National University, Gwangju, South Korea
As sessile organisms, plants are exposed to constantly changing environments that are often stressful for their growth and development. To cope with these stresses, plants have evolved complex and sophisticated stress-responsive signaling pathways regulating the expression of transcription factors and biosynthesis of osmolytes that confer tolerance to plants. Signaling peptides acting like phytohormones control various aspects of plant growth and development via cell-cell communication networks. These peptides are typically recognized by membrane-embedded receptor-like kinases, inducing activation of cellular signaling to control plant growth and development. Recent studies have revealed that several signaling peptides play important roles in plant responses to abiotic stress. In this mini review, we provide recent findings on the roles and signaling pathways of peptides that are involved in coordinating plant responses to abiotic stresses, such as dehydration, high salinity, reactive oxygen species, and heat. We also discuss recent developments in signaling peptides that play a role in plant adaptation responses to nutrient deficiency stress, focusing on nitrogen and phosphate deficiency responses.
Introduction
As sessile organisms, plants are constantly exposed to a wide range of abiotic stresses, such as drought, high salinity, cold, heat, flooding, and toxic metals in the soil, which negatively affect plant growth, fertility, development, metabolism, photosynthesis, and immune response and impair plant yield and quality in the field (Jeon and Kim, 2013; Suzuki et al., 2014; Ohama et al., 2017; Casal and Balasubramanian, 2019; Hayes et al., 2021). Plants have evolved sophisticated and complex metabolic pathways, signaling modules, such as Ca2+-calcineurin B-like (CBL)-CBL-interacting protein kinases (CIPKs) and mitogen-activated protein kinases (MAPKs) modules, and stress-responsive transcription factors for gaining stress tolerance (Qin et al., 2011; De Zelicourt et al., 2016; Zhu, 2016; Shi et al., 2018; Tang et al., 2020). Recent studies have revealed that signaling peptides acting like phytohormones regulate various biochemical, developmental, and physiological processes to coordinate diverse aspects of plant growth and development (Matsubayashi, 2014; Oh et al., 2018; Fletcher, 2020; Jeon et al., 2021; Kim et al., 2021). Signaling peptides are typically small peptides comprising 5–20 amino acids in length or peptides of 40–100 amino acids; they are processed from precursor proteins or directly translated from small open reading frames without proteolytic processing (Matsubayashi, 2014; Oh et al., 2018). These peptides can be mobile in a long or short distance or membrane-bound and are typically recognized by the membrane-localized leucine-rich repeat (LRR)-receptor-like kinases (RLKs), mostly in association with shape-complementary coreceptors. This ligand-receptor/coreceptor association initiates intracellular signaling to control plant growth and development. Several peptides controlling adaptation and tolerance mechanisms in response to abiotic stress have been identified. In this review, we summarize the roles and signaling pathways of small peptides involved in coordinating plant responses against abiotic stresses, such as dehydration, high salinity, reactive oxygen species (ROS), and heat, and discuss future directions on the peptide research in abiotic stress response. The roles of signaling peptides regulating plant responses under nitrogen or phosphate deficiency are also discussed.
Dehydration Stress Response
CLE Peptides
The CLAVATA3(CLV)/EMBRYO-SURROUNDING REGION-RELATED (CLE) peptides are a major group of signaling peptides in plants (Goad et al., 2017), usually 12–14 amino acids long and processed from a large precursor protein (Strabala et al., 2014). Several CLE peptides play important roles in root meristem maintenance, vasculature tissue and shoot development, and stomata formation and function (Oh et al., 2018; Fletcher, 2020; Jeon et al., 2021). In particular, CLE25 and CLE9 mediate dehydration stress tolerance response in Arabidopsis thaliana (Arabidopsis) (Takahashi et al., 2018; Zhang et al., 2019; Figure 1A).
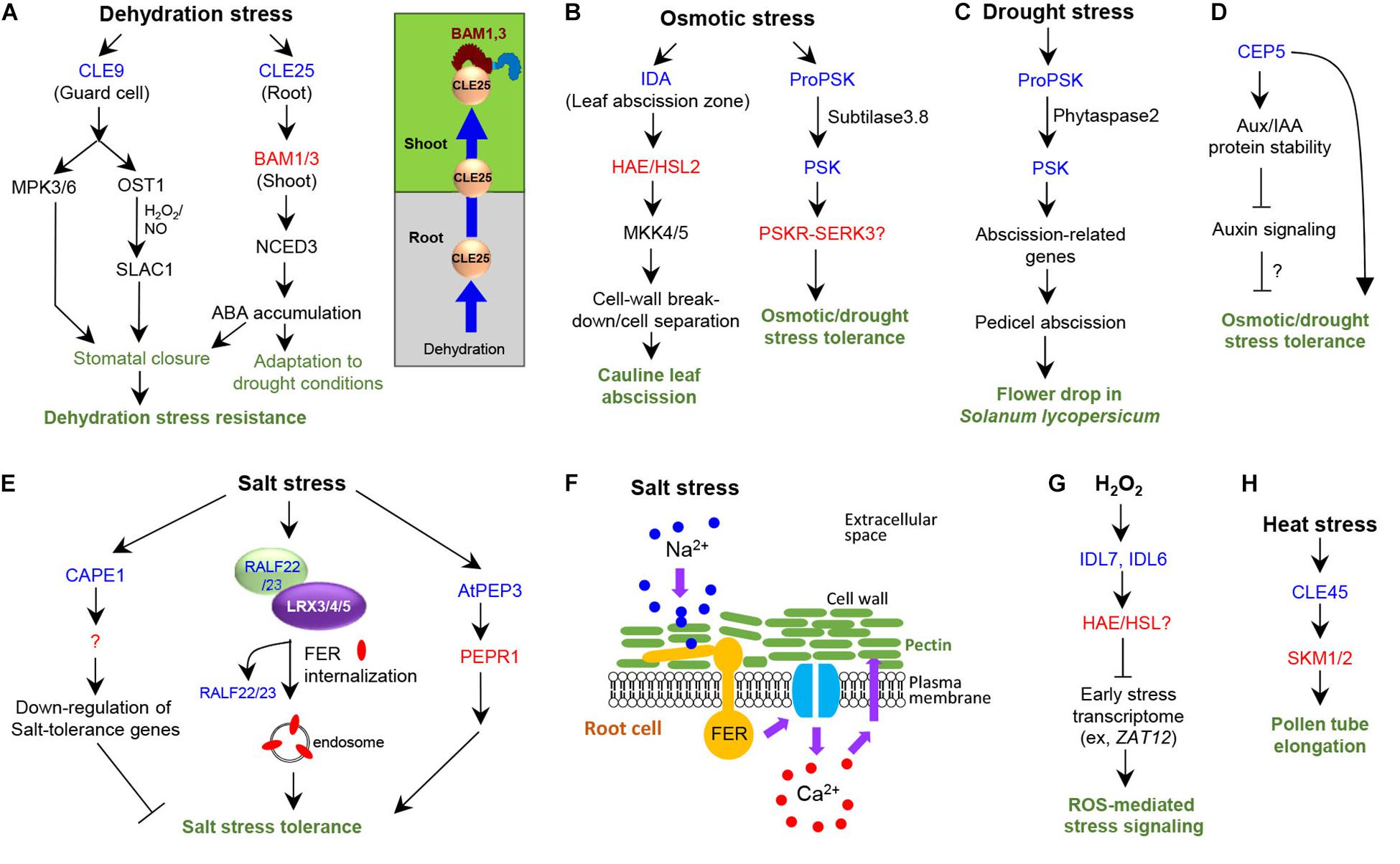
Figure 1. Signaling peptides control abiotic stress responses in plants. Blue and red letters indicate peptides and the cognate receptors, respectively. Plant not specified in the subtitle indicates Arabidopsis. (A) Roles and signaling pathways of CLE9/25 in dehydration stress response. (B) Roles and signaling pathways of IDA and PSK in osmotic stress response. (C) Roles of PSK in flower abscission in tomato under drought stress. (D) Role of CEP5 in osmotic and drought stress tolerance. (E) Roles and signaling pathways in high salinity stress response. (F) Role of FER receptor kinase during high salinity stress response to maintain cell-wall integrity in root cells. (G) Role of IDL6/7 in ROS-mediated stress signaling. (H) Role of CLE45 in pollen tube elongation under heat stress.
CLE25 is a mobile peptide that links water deficit to abscisic acid (ABA)-mediated tolerance to dehydration stress (Takahashi et al., 2018; Figure 1A). Water deficiency upregulates CLE25 expression in root vasculature; peptides then migrate to the leaves, inducing stomatal closure (Takahashi et al., 2018; Figure 1A). CLE25 peptides applied to the roots can induce expression of the gene encoding the enzyme NINE-CIS-EPOXYCAROTENOID DIOXYGENASE3 (NCED3), which cleaves an ABA precursor molecule and generates bioactive ABA (Figure 1A). The cle25 knockout mutants are more sensitive to dehydration than wild type (Takahashi et al., 2018). Grafting assays showed that root-derived CLE25 during dehydration stress is recognized by BARELY ANY MERISTEM1 (BAM1) and BAM2 receptors only in the leaves but not in the roots (Takahashi et al., 2018); the bam1 bam2 mutants are dehydration-sensitive (Takahashi et al., 2018). These results suggest that CLE25–BAM modules convey dehydration signals in the soil from the roots to the leaves in a long-distance signaling to induce ABA accumulation via upregulating NCED3 expression for stress adaptation and resistance against drought conditions.
CLE9 plays a role in enhancing drought tolerance by regulating stomatal closure (Zhang et al., 2019; Figure 1A). Exogenous application of CLE9 peptides or overexpression of CLE9 induces stomatal closure and enhances drought tolerance. Genetic analysis showed that CLE9-induced stomatal closure requires MITOGEN-ACTIVATED PROTEIN KINASE (MAPK)3 (MPK3)/6 and two guard cell ABA-signaling components–protein kinase OPEN STOMATA 1 (OST1) and anion channel protein SLOW ANION CHANNEL-ASSOCIATED 1 (SLAC1) (Zhang et al., 2019; Figure 1A). Moreover, the production of hydrogen peroxide and nitric oxide is stimulated by CLE9 and disappears in NADPH oxidase-deficient or nitric reductase mutants, respectively (Zhang et al., 2019). These findings indicate that CLE9 controls stomatal closure in response to drought stress by sharing OST1 and SLAC1, thereby enhancing drought stress tolerance. CLE9 is specifically expressed in guard cells (Zhang et al., 2019); hence, CLE9, unlike CLE25, acts locally to control stomatal closure. Further, the bam/clv1 mutants respond normally to CLE9 peptides in stomatal closure, indicating that BAM receptors are unlikely to be involved in the recognition of CLE9 peptides (Zhang et al., 2019).
IDA Peptide
The INFLORESCENCE DEFICIENT IN ABSCISSION (IDA) peptide functions in floral organ abscission and lateral root emergence through the LRR-RLKs, HAESA (HAE), and HAESA-LIKE 2 (HSL2) receptors and the MAPK KINASE4 (MKK4)/5-MPK3/6 cascade (Butenko et al., 2003; Stenvik et al., 2006; Cho et al., 2008; Kumpf et al., 2013; Zhu et al., 2019). The extended 20-amino acid Pro-rich motif at the C terminus of IDA, was sufficient to induce floral abscission in Arabidopsis (Stenvik et al., 2008). A 12-amino acid peptide with hydroxylation of a Pro residue at position 7 was identified as the most efficient peptide for signaling activation through HSL2 receptor in Nicotiana benthamiana leaf tissue (Butenko et al., 2014). IDA is involved in drought-induced cauline leaf abscission (Patharkar and Walker, 2016). Expression of HAE and IDA is induced in leaf abscission zones when the leaves are subject to drought conditions (Patharkar and Walker, 2016). Mutant analysis showed that IDA, HAE/HSL2, and MKK4/5 are all necessary for drought-induced cauline leaf abscission (Figure 1B) as they function in floral organ abscission and lateral root emergence (Patharkar and Walker, 2016). IDA induces cell-wall breakdown and thus cell separation, causing these organ abscission events (Stenvik et al., 2006; Zhu et al., 2019; Figure 1B).
PSK Peptide
Phytosulfokine-α (PSK-α) is a pentapeptide sulfated at two Tyr residues (Stührwohldt et al., 2011). Seven Arabidopsis loci encode putative PSK precursor genes (Kaufmann and Sauter, 2019). PSK-PSK receptor (PSKR)/coreceptor SOMATIC EMBRYOGENESIS RECEPTOR KINASE 3 (SERK3) modules promote cell elongation and primary/lateral root growth (Amano et al., 2007; Ladwig et al., 2015; Wang et al., 2015). PSK and subtilases (SBTs), which cleave PSK precursor protein for generating the PSK pentapeptide, play a role in drought stress tolerance in Arabidopsis (Stührwohldt et al., 2021). Four PSK genes–PSK1, PSK3, PSK4, and PSK5–and three SBT genes–SBT1.4, SBT3.7, and SBT3.8–are significantly upregulated in response to osmotic stress, such as mannitol treatment (Stührwohldt et al., 2021). Shoot and root growth is slower in sbt3.8 mutant plants than in wild type under osmotic stress, whereas the osmotic stress-induced sensitive phenotype in sbt3.8 can be recovered by PSK peptide treatment. Arabidopsis plants overexpressing the PSK precursor (proPSK1) exhibit enhanced root and hypocotyl growth and osmotic stress tolerance. ProPSK1 is cleaved by SBT3.8 at the C-terminus of the PSK peptide. ProPSK1 processing depends on the Asp residue directly following the cleavage site and is impaired in the sbt3.8 mutant. Moreover, SBT3.8 overexpression in Arabidopsis improves osmotic stress tolerance and induces shoot and root growth. These results suggest that SBT3.8 mediates PSK peptide processing from the precursor protein proPSK1, thus contributing to drought stress tolerance (Figure 1B).
Phytosulfokine signaling controls drought-induced flower drop in tomato (Reichardt et al., 2020). Overexpression of phytaspase 2 (phyt2), a subtilisin-like protease that cleaves proPSK protein, in tomato plants (Solanum lycopersicum) enhances premature flower abscission, whereas SlPhyt2 knockdown reduces flower drop under drought stress conditions, implying a role of SlPhyt2 in drought-induced abortion of flower and fruit development (Reichardt et al., 2020). Consistently, SlPhyt2 expression is induced in response to drought stress in flower pedicels proximal to the abscission zone. Expression of SlPSK1 and SlPSK6 is coinduced with that of SlPhyt2 by drought stress. Using a proteomics assay with a substrate library, the PSK precursor was identified as a candidate substrate for SlPhyt2. Peptides harboring the PSK pentapeptide of varying sizes can be cleaved by SlPhyt2 in an Asp-specific manner, releasing mature PSK in vitro. Mature PSK induced pedicel abscission in an inflorescence bioassay. PSK treatment upregulated tomato abscission-related polygalacturonase genes and downregulated genes that maintain the abscission zone in an inactive state, indicating that PSK acts as a signal for pedicel abscission in tomato. These findings suggest that the subtilase SlPhyt2, expressed in the pedicel, produces bioactive PSK, which then triggers abscission by inducing cell wall hydrolases in the abscission zone in response to drought stress (Figure 1C).
CEP5 Peptide
The C-terminally encoded peptide (CEP) genes encode proteins comprising an N-terminal secretion signal, a variable domain, one or more CEP domains, and a short C-terminal extension (Ogilvie et al., 2014). The CEP precursor proteins undergo proteolysis and Pro hydroxylation to become a bioactive 15-amino acid CEP peptide (Ohyama et al., 2008). CEPs and the CEP receptors, CEPR1/2, play diverse roles in plant responses to changing environmental conditions, such as the systemic N-acquisition response, sucrose-induced lateral root growth enhancement, and primary root growth suppression, under nutrient starvation conditions, such as carbon and nitrogen limitation (Jeon et al., 2021).
CEP5 negatively regulates primary and lateral root development (Roberts et al., 2016) and plays a role in osmotic and drought stress tolerance in Arabidopsis (Smith et al., 2020). Proteome analysis revealed that CEP5 alters significant portions of the proteins involved in the biological processes “response to stress or to abiotic stimulus”, indicating a potential role of CEP5 in abiotic stress responses. Consistent with this, CEP5-overexpression lines or wild type-seedlings treated with hydroxyprolinated CEP5 peptide exhibit better recovery from drought after re-watering than wild type, indicating that CEP5 can confer drought stress tolerance. CEP5-overexpression line also displays enhanced tolerance in rosette size reduction to osmotic stress and enhanced expression of osmotic stress-inducible transcription factor genes. The xylem intermixed with phloem (xip)/cepr1 cepr2 double mutant did not display rosette size reduction upon osmotic stress treatment, indicating that CEP5 acts independently of the CEPRs. Notably, CEP5-overexpression line displays significant reduction in both DR5-GUS or DR5-LUC activities and expression of auxin-inducible genes, LOB DOMAIN-CONTAINING PROTEIN 18 (LBD18), LBD29, and PIN-FORMED 1 (PIN1). CEP5 stabilizes AUX/IAA proteins, negative regulators of AUXIN RESPONSE FACTORs, by affecting proteasome activity but without altering auxin levels and auxin transport activity (Figure 1D). Whether and how the negative regulatory role of CEP5 in auxin response through AUX/IAA stabilization is linked to drought and osmotic stress tolerance need further investigation. Functional analysis of the cep5 mutants is also necessary to support the results obtained with CEP5 overexpression.
Salinity Stress Response
CAPE Peptides
CAP-derived peptide 1 (CAPE1) is derived from the C-terminus of PATHOGENESIS-RELATED PROTEIN1b (PR-1b), a member of the cysteine-rich secretory proteins, antigen 5, and pathogenesis-related 1 proteins (CAP) superfamily (Chen et al., 2014). CAPE1 was initially identified using a peptidomics approach from tomato leaves (Chen et al., 2014). CAPE1 induces significant anti-pathogen response in tomato, indicating a role for PR-1 in immune signaling (Chen et al., 2014). In Arabidopsis, AtCAPE1, comprising 11 amino acids, negatively regulates salt-stress tolerance (Chien et al., 2015; Figure 1E). Nine potential CAPEs from Arabidopsis were identified as precursor candidates for CAPEs on the basis of sequence similarity to the precursor of tomato CAPE1 and the C-terminal conserved motif, and were named as the precursor Arabidopsis thaliana CAPEs (PROAtCAPEs) (Chien et al., 2015). Among them, PROAtCAPE1 is down-regulated mainly by salt stress. The proatcape1 mutant exhibits resistance to growth inhibition under high-salt conditions, whereas exogenous application of synthetic AtCAPE1 peptide or overexpression of PROAtCAPE1 restores the sensitive phenotype of the mutant, indicating that AtCAPE1 functions as a negative regulator of salt-stress tolerance in Arabidopsis. AtCAPE1also negatively regulates salt-inducible genes, such as those involved in osmolyte biosynthesis, detoxification, and dehydration response (Chien et al., 2015). Hence, AtCAPE1 plays a role in the regulation of salt stress responses in Arabidopsis.
RALF Peptides
Rapid alkalinization factor (RALF) peptides are 5 kDa cysteine-rich peptides inducing rapid alkalinization of the extracellular compartments of plant cells, thus reducing the proton electrochemical potential required for solute uptake and causing cell growth suppression (Blackburn et al., 2020). RALF peptides are involved in root growth, immune responses, guard cell movement, and pollen tube growth and termination during plant reproductive processes (Ge et al., 2017; Mecchia et al., 2017; Blackburn et al., 2020). They are recognized by the LRR-RLK FERONIA (FER) (Stegmann et al., 2017). RALF22/23-FER module regulates salt tolerance by interacting with the cell-wall LRR extensins (LRX)3/4/5 (Zhao et al., 2018; Figure 1E). LRX proteins comprise an N-terminal LRR and a C-terminal extension domain and are localized to the cell wall, playing a role in cell wall-plasma membrane communication (Fabrice et al., 2018). The lrx3/4/5 triple mutant, fer mutant, and RALF22 or RALF23-overexpressing plants all display similar phenotypes, such as reduced plant growth and salt hypersensitivity (Zhao et al., 2018). RALF peptides are physically associated with LRX and FER proteins (Zhao et al., 2018). Salt stress causes dissociation of mature RALF22 peptides from LRX proteins, thereby inducing FER internalization via an endosomal pathway (Zhao et al., 2018; Figure 1E). Therefore, RALF22/23-FER and LRX3/4/5 regulate plant growth and salt tolerance via salt-induced cell wall changes.
FERONIA is also necessary for cell-wall integrity, preventing root cells from bursting during growth under high salt stress (Feng et al., 2018). The glycosylphosphatidylinositol-anchored protein (GPI-AP) LORELEI-like GPI-AP1 (LLG1) interacts directly with FER in the endoplasmic reticulum and is required for both FER localization to the plasma membrane and FER-mediated RHO GTPase signaling (Li et al., 2015). llg1 mutant roots display the same ionic sensitivity and cellular damage caused by a loss of cell-wall integrity as the fer mutant (Feng et al., 2018), indicating that FER-LLG1 interaction is important for maintaining cell-wall integrity in the roots. Fortification of pectin cross-links restores growth and cell-wall integrity of fer seedlings under salt stress (Feng et al., 2018). The FER extracellular domain binds pectin in vitro. FER is necessary for salinity-induced [Ca2+] transients to maintain cell wall integrity during growth recovery, suggesting that FER induces calcium signaling in response to salt stress to maintain cell wall integrity by physically interacting with pectin in the cell wall (Figure 1F). Further investigation is needed to identify the calcium channel responsible for FER-mediated induction of calcium transients and how such transients regulate downstream signaling to repair salt stress-induced cell-wall damage. The ralf1 mutant and a RALF1 RNAi transgenic line did not show significant root growth inhibition, indicating that RALF1 does not display a critical function in regulating root growth under salt stress (Feng et al., 2018). As RALF22/23-FER module regulates plant growth and salt tolerance via salt-induced cell-wall changes (Zhao et al., 2018), RALF22/23 might play a role in FER-mediated protection of root cells from bursting under salt stress.
AtPEP3
Plant elicitor peptides (Peps) are endogenous elicitors of pattern-triggered immunity against bacteria, fungi, and herbivores (Yamaguchi and Huffaker, 2011; Bartels and Boller, 2015). Among eight members of the Arabidopsis thaliana precursor Pep (AtPROPEP) family in Arabidopsis, AtPep3 plays a role in salinity stress response (Nakaminami et al., 2018). Among the AtPROPEP gene family, AtPROPEP3 displays the greatest induction in response to high salinity. Overexpression of AtPROPEP3 or exogenous application of synthetic AtPep3 peptide (30 amino acids) derived from the C-terminal region of PROPEP3 induces salt stress tolerance (Nakaminami et al., 2018). Conversely, AtPROPEP3-RNAi lines are hypersensitive under salinity stress, which is recovered by AtPep3 peptide application. Endogenous AtPep3 peptide was further identified from NaCl-treated plants via mass spectrometry (Nakaminami et al., 2018). AtPEP1-6 peptides bind to the LRR-RLK PEP RECEPTOR1 (PEPR1) and AtPEP1/2 peptides bind to PEPR2 to initiate immune signaling (Yamaguchi et al., 2006, 2010). Although high-salt treatment significantly reduces plant survival of the pepr1, pepr2, and pepr1/2 mutants, exogenous AtPep3 peptide application recovers plant survival only in the pepr2 mutants (Nakaminami et al., 2018), indicating that AtPep3 is recognized by the PEPR1 receptor to induce salinity stress tolerance in plants (Figure 1E). Hence, AtPEP3–PEPR1 module may have a dual function in both salinity stress tolerance and immune responses.
ROS-Mediated Stress Signaling
IDA-LIKE (IDL) Peptides
Bioinformatic analyses identified eight IDL genes with similarity to IDA (Butenko et al., 2003; Vie et al., 2015). In silico analysis showed that IDL6 and IDL7 respond to biotic and abiotic stresses, such as cold, salt, and UV light in the root (Vie et al., 2015). Application of IDL6 and IDL7 peptides to Arabidopsis reduces the expression of early stress-responsive genes, including ZINC FINGER PROTEIN and WRKY genes (Vie et al., 2017). IDL7 expression is rapidly induced by the ROS hydrogen peroxide, and ROS-induced cell death is mitigated in the idl7 mutant. IDL7 peptides can attenuate the rapid ROS burst induced by the bacterial elicitor flagellin 22 (flg22), whereas IDL7 mutation enhances flg22-induced ROS burst. Hence, IDL7 acts as a negative regulator of stress-induced ROS signaling in Arabidopsis (Figure 1G). As IDL6 displays expression patterns similar to IDL7 in response to ROS and IDL6 downregulates similar target genes, IDL6 might act like IDL7 in ROS signaling. Although HAE/HSL2 are likely to perceive IDL6 and IDL7 peptides, the receptors of IDL6/7 peptides remain to be experimentally identified (Vie et al., 2017).
Heat Stress Response
CLE45 Peptide
Environmental stresses negatively affect reproductive development of flowering plants and thus the seed yield (Giorno et al., 2013; De Storme and Geelen, 2014). To date, one signaling peptide is reported to play a role in high temperature stress response in plants (Endo et al., 2013), i.e., the Arabidopsis CLE45-STERILITY-REGULATING KINASE MEMBER1 (SKM1)/SMK2 receptor module in pollen tube growth (Endo et al., 2013). CLE45 prolongs pollen tube growth in in vitro pollen tube culture. Further, the double mutation in SKM1/2 encoding LRR–RLKs causes insensitive phenotype in pollen tube growth in vitro in response to synthetic CLE45 peptide. Photoaffinity labeling experiment with the SKM1-HaloTag protein demonstrated that SKM1 physically interacts with CLE45 peptides. GUS reporter expression analysis and artificial crossing experiments suggest that heat-inducible CLE45 in pistils and SKM1 in pollen function in the same signaling pathway. Suppression of CLE45 expression by RNAi or expression of a kinase-dead version of SKM1 acting as a dominant negative form of SKM1 in the skm1 mutant reduces seed number and size at 30°C but not at room temperature (22°C). Collectively, these findings suggest that the CLE45–SKM1/2 pathway sustains pollen tube growth under high temperatures, leading to successful seed production (Figure 1H).
Nutrient Deficiency Stress Responses
Nitrogen (N) and inorganic phosphate (Pi) are the most important nutrients required by plants, and thus their deficiency profoundly influences the morphology, physiology, growth, and development in plants (Reddy, 2006). Peptides, such as CEPs, CLEs, and RGFs, were identified to play a role in the regulation of nutrient acquisition and to affect plant physiology and growth responses. CEP1 acts as a root-to-shoot mobile signal to promote compensatory N acquisition in the other N-rich parts of the roots (Okamoto et al., 2016). CEP1 peptides originating from the roots under N deficiency are translocated through the xylem to the shoots, where CEP1 peptides are recognized by XIP1/CEPR1 and CEPR2 receptors, generating descending shoot-to-root signals, CEP Downstream 1 (CEPD1) and CEPD2, which belong to the glutaredoxin family (Tabata et al., 2014; Ohkubo et al., 2017; Figure 2A). CEPDs are transported to the roots through the phloem to upregulate the nitrate transporter gene NRT2.1 for promoting N uptake (Ohkubo et al., 2017). CEPD-like 2 originating from the shoots promotes both high-affinity N-uptake in the roots and root-to-shoot transport of nitrate, acting cooperatively with CEPD1/2 (Ota et al., 2020). CEP3 controls nutrient starvation response that suppresses root growth under N-limitation condition, potentially to enhance seedling survival (Delay et al., 2013, 2019; Figure 2B).
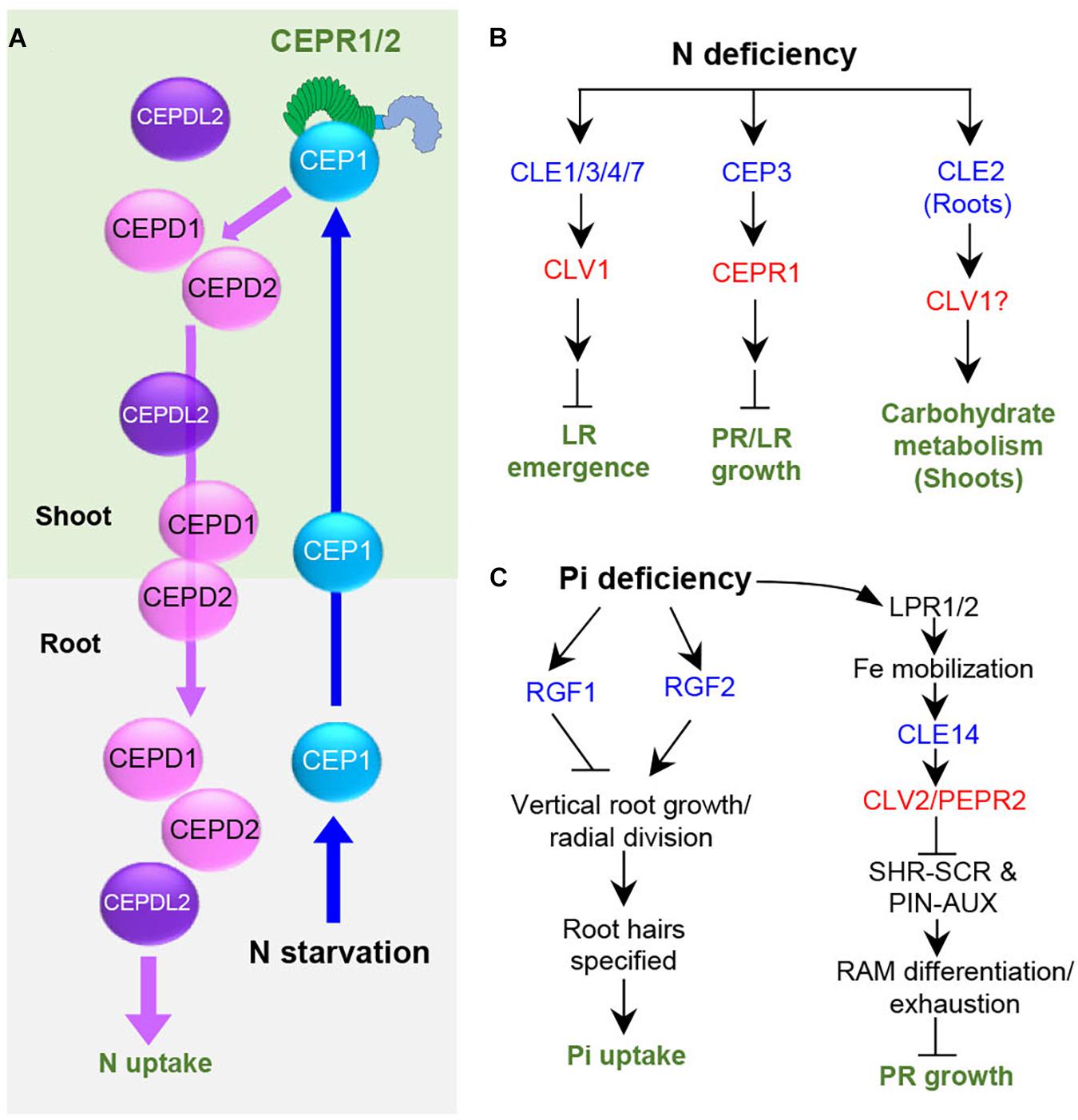
Figure 2. Role and signaling pathways of peptides regulating plant responses under nutritional deficiency stresses in Arabidopsis. Blue and red letters indicate peptides and the cognate receptors, respectively. (A) The systemic N-acquisition response. (B) Plant responses under nitrogen deficiency. (C) Plant responses under phosphate deficiency. N, nitrogen; Pi, inorganic phosphate; LPR, Low Phosphate Root2; LR, lateral root; PR, primary root.
CLE3 inhibits lateral root growth, likely through the receptor CLV1, as the clv1 mutant displayed longer lateral roots under N deficiency and the inhibitory action of CLE3 in lateral root formation was compromised in the clv1 mutant (Araya et al., 2014; Figure 2B). CLE1/3/4/7 genes are upregulated in root pericycle cells by systemic low N levels (Araya et al., 2014), implying that CLE1/3/4/7 act in the combinatorial manner to control lateral root growth. CLE2 expression is upregulated by N supply, dark, and sugar starvation (Ma et al., 2020). The cle2 mutant plants exhibit a severe growth defect with leaf chlorosis under these conditions, whereas cle3 did not display such growth phenotype under the same condition (Ma et al., 2020). Consistent with this phenotype, overexpression of CLE2 in roots increased many different genes in shoots, such as dark-inducible genes and the genes involved in metabolite sensing and regulation of carbohydrate metabolism. These results indicate that CLE2 induced in the roots functions systemically to control carbohydrate metabolism in light-dependent manner (Ma et al., 2020; Figure 2B). It is of interest to identify how a single amino acid difference between CLE2 and CLE3 peptides renders these two similar peptides to regulate different plant responses in different tissues. CLE14 mediates low-phosphate stress signal in roots to trigger RAM differentiation and exhaustion through CLV2/PEPR2 receptors via suppressing SCARECROW (SCR)/SHORT-ROOT (SHR) and PIN/auxin pathway, inhibiting primary root growth (Gutiérrez-Alanís et al., 2017; Figure 2C). This CLE14 signaling pathway allows Arabidopsis plants to adapt to Pi availability around the roots.
Plants alter root development under Pi scarcity to maximize Pi acquisition. Genetic and microscopic expression analyses suggest that ROOT GROWTH FACTOR1 (RGF1) and RGF2 regulate different aspects of root development under Pi-deprivation conditions (Cederholm and Benfey, 2015). RGF2 promotes vertical root growth and radial divisions, whereas RGF1 inhibits radial divisions in the root meristem, specifying root hairs for facilitating Pi uptake (Cederholm and Benfey, 2015; Figure 2C). RGF1 promotes root meristem activity via a mitogen-activated protein kinase cascade by inducing RGF1-INDUCIBLE TRANSCRIPTION FACTOR (RITF), which enhances the stability of a master regulator of root stem cells, PLETHORA2, through ROS signaling (Fernandez et al., 2013; Ou et al., 2016; Shinohara et al., 2016; Song et al., 2016; Fernandez et al., 2020; Lu et al., 2020; Shao et al., 2020; Yamada et al., 2020). It remains to be investigated whether this signaling pathway is involved in RGF-regulated root development caused by Pi deprivation.
Conclusion and Perspectives
Over recent years, several peptides and signaling pathways have been identified to play roles in coordinating plant responses to abiotic stresses. Although the Arabidopsis genome alone encodes several thousands of small coding genes (Takahashi et al., 2019), only few peptides have been reported to be involved in controlling plant abiotic stress responses. Moreover, there exist many questions regarding molecular mechanisms of peptide signaling during abiotic stress responses to be addressed. Functional and molecular understanding on RLKs and the coreceptors that interact with signaling peptides in response to abiotic stress are limited. Questions remain on how peptide precursors are processed into mature peptides, and mature peptides are secreted and transported to the target tissues in long-distance peptide signaling pathways. How peptide-receptor interactions lead to specific developmental controls under abiotic stress need investigation. How peptide-mediated signaling pathways confer plant tolerance to abiotic stresses is also unknown. CLE25 induces active ABA accumulation in the leaves through long-distance signaling from the roots to the leaves in response to dehydration, and CLE9 uses ABA signaling components to enhance drought stress tolerance. It will be of interest to investigate how signaling peptides are linked to conventional phytohormone pathways under abiotic stress for obtaining stress resistance. How changes in local concentrations of nutrients and abiotic stressors are sensed in plants for eliciting peptide signaling will be a challenging issue to be explored. As abiotic stresses accelerated by climate changes are a major threat to crop yields and food security, the identification of plant peptides and signaling pathways will provide new strategies to improve stress tolerance in crops for agricultural sustainability.
Author Contributions
JiK and BJ wrote the draft manuscript. JuK conceived the review outline and wrote and edited the manuscript. All authors contributed to the article and approved the submitted version.
Funding
This work was supported by grants from the Basic Research Lab Program (2020R1A4A2002901) and Mid-Career Researcher Program (2021R1A2C1006296) through the National Research Foundation of Korea (NRF) funded by the Ministry of Science and ICT, and the Next-Generation BioGreen 21 Program from Rural Development Administration, Republic of Korea (PJ013220).
Conflict of Interest
The authors declare that the research was conducted in the absence of any commercial or financial relationships that could be construed as a potential conflict of interest.
References
Amano, Y., Tsubouchi, H., Shinohara, H., Ogawa, M., and Matsubayashi, Y. (2007). Tyrosine-sulfated glycopeptide involved in cellular proliferation and expansion in Arabidopsis. Proc. Natl. Acad. Sci. U.S.A. 104, 18333–18338. doi: 10.1073/pnas.0706403104
Araya, T., Miyamoto, M., Wibowo, J., Suzuki, A., Kojima, S., Tsuchiya, Y. N., et al. (2014). CLE-CLAVATA1 peptide-receptor signaling module regulates the expansion of plant root systems in a nitrogen-dependent manner. Proc. Natl. Acad. Sci. U. S. A. 111, 2029–2034. doi: 10.1073/pnas.1319953111
Bartels, S., and Boller, T. (2015). Quo vadis, Pep? Plant elicitor peptides at the crossroads of immunity, stress, and development. J. Exp. Bot. 66, 5183–5193. doi: 10.1093/jxb/erv180
Blackburn, M. R., Haruta, M., and Moura, D. S. (2020). Twenty years of progress in physiological and biochemical investigation of RALF peptides. Plant Physiol. 182, 1657–1666. doi: 10.1104/pp.19.01310
Butenko, M. A., Patterson, S. E., Grini, P. E., Stenvik, G. E., Amundsen, S. S., Mandal, A., et al. (2003). Inflorescence deficient in abscission controls floral organ abscission in Arabidopsis and identifies a novel family of putative ligands in plants. Plant Cell 15, 2296–2307. doi: 10.1105/tpc.014365
Butenko, M. A., Wildhagen, M., Albert, M., Jehle, A., Kalbacher, H., Aalen, R. B., et al. (2014). Tools and strategies to match peptide-ligand receptor pairs. Plant Cell 26, 1838–1847. doi: 10.1105/tpc.113.120071
Casal, J. J., and Balasubramanian, S. (2019). Thermomorphogenesis. Annu. Rev. Plant Biol. 70, 321–346. doi: 10.1146/annurev-arplant-050718-095919
Cederholm, H. M., and Benfey, P. N. (2015). Distinct sensitivities to phosphate deprivation suggest that RGF peptides play disparate roles in Arabidopsis thaliana root development. New Phytol. 207, 683–691. doi: 10.1111/nph.13405
Chen, Y. L., Lee, C. Y., Cheng, K. T., Chang, W. H., Huang, R. N., Nam, H. G., et al. (2014). Quantitative peptidomics study reveals that a wound-induced peptide from PR-1 regulates immune signaling in tomato. Plant Cell 26, 4135–4148. doi: 10.1105/tpc.114.131185
Chien, P. S., Nam, H. G., and Chen, Y. R. (2015). A salt-regulated peptide derived from the CAP superfamily protein negatively regulates salt-stress tolerance in Arabidopsis. J. Exp. Bot. 66, 5301–5313. doi: 10.1093/jxb/erv263
Cho, S. K., Larue, C. T., Chevalier, D., Wang, H., Jinn, T. L., Zhang, S., et al. (2008). Regulation of floral organ abscission in Arabidopsis thaliana. Proc. Natl. Acad. Sci. U.S.A. 105, 15629–15634. doi: 10.1073/pnas.0805539105
De Storme, N., and Geelen, D. (2014). The impact of environmental stress on male reproductive development in plants: biological processes and molecular mechanisms. Plant Cell Environ. 37, 1–18. doi: 10.1111/pce.12142
De Zelicourt, A., Colcombet, J., and Hirt, H. (2016). The role of MAPK modules and ABA during abiotic stress signaling. Trends Plant Sci. 21, 677–685. doi: 10.1016/j.tplants.2016.04.004
Delay, C., Chapman, K., Taleski, M., Wang, Y., Tyagi, S., Xiong, Y., et al. (2019). CEP3 levels affect starvation-related growth responses of the primary root. J. Exp. Bot. 70, 4763–4774. doi: 10.1093/jxb/erz270
Delay, C., Imin, N., and Djordjevic, M. A. (2013). CEP genes regulate root and shoot development in response to environmental cues and are specific to seed plants. J. Exp. Bot. 64, 5383–5394. doi: 10.1093/jxb/ert332
Endo, S., Shinohara, H., Matsubayashi, Y., and Fukuda, H. (2013). A novel pollen-pistil interaction conferring high-temperature tolerance during reproduction via CLE45 signaling. Curr. Biol. 23, 1670–1676. doi: 10.1016/j.cub.2013.06.060
Fabrice, T. N., Vogler, H., Draeger, C., Munglani, G., Gupta, S., Herger, A. G., et al. (2018). LRX proteins play a crucial role in pollen grain and pollen tube cell wall development. Plant Physiol. 176, 1981–1992. doi: 10.1104/pp.17.01374
Feng, W., Kita, D., Peaucelle, A., Cartwright, H. N., Doan, V., Duan, Q., et al. (2018). The FERONIA receptor kinase maintains cell-wall integrity during salt stress through Ca2+ signaling. Curr. Biol. 28, 666–675 doi: 10.1016/j.cub.2018.01.023
Fernandez, A., Drozdzecki, A., Hoogewijs, K., Nguyen, A., Beeckman, T., Madder, A., et al. (2013). Transcriptional and functional classification of the GOLVEN/ROOT GROWTH FACTOR/CLE-like signaling peptides reveals their role in lateral root and hair formation. Plant Physiol. 161, 954–970. doi: 10.1104/pp.112.206029
Fernandez, A. I., Vangheluwe, N., Xu, K., Jourquin, J., Claus, L. A. N., Morales-Herrera, S., et al. (2020). GOLVEN peptide signalling through RGI receptors and MPK6 restricts asymmetric cell division during lateral root initiation. Nat. Plants 6, 533–543. doi: 10.1038/s41477-020-0645-z
Fletcher, J. C. (2020). Recent advances in Arabidopsis CLE peptide signaling. Trends Plant Sci. 25, 1005–1016. doi: 10.1016/j.tplants.2020.04.014
Ge, Z., Bergonci, T., Zhao, Y., Zou, Y., Du, S., Liu, M. C., et al. (2017). Arabidopsis pollen tube integrity and sperm release are regulated by RALF-mediated signaling. Science 358, 1596–1600. doi: 10.1126/science.aao3642
Giorno, F., Wolters-Arts, M., Mariani, C., and Rieu, I. (2013). Ensuring reproduction at high temperatures: the heat stress response during anther and pollen development. Plants (Basel) 2, 489–506. doi: 10.3390/plants2030489
Goad, D. M., Zhu, C., and Kellogg, E. A. (2017). Comprehensive identification and clustering of CLV3/ESR-related (CLE) genes in plants finds groups with potentially shared function. New Phytol. 216, 605–616. doi: 10.1111/nph.14348
Gutiérrez-Alanís, D., Yong-Villalobos, L., Jiménez-Sandoval, P., Alatorre-Cobos, F., Oropeza-Aburto, A., Mora-Macías, J., et al. (2017). Phosphate starvation-dependent iron mobilization induces CLE14 expression to trigger root meristem differentiation through CLV2/PEPR2 signaling. Dev. Cell 41, 555–570. doi: 10.1016/j.devcel.2017.05.009
Hayes, S., Schachtschabel, J., Mishkind, M., Munnik, T., and Arisz, S. A. (2021). Hot topic: thermosensing in plants. Plant Cell Environ. 44, 2018–2033. doi: 10.1111/pce.13979
Jeon, B. W., Kim, M. J., Pandey, S. K., Oh, E., Seo, P. J., and Kim, J. (2021). Recent advances in peptide signaling during Arabidopsis root development. J. Exp. Bot. 72, 2889–2902. doi: 10.1093/jxb/erab050
Jeon, J., and Kim, J. (2013). Cold stress signaling networks in Arabidopsis. J. Plant Biol. 56, 69–76. doi: 10.1007/s12374-013-0903-y
Kaufmann, C., and Sauter, M. (2019). Sulfated plant peptide hormones. J. Exp. Bot. 70, 4267–4277. doi: 10.1093/jxb/erz292
Kim, M. J., Jeon, B. W., Oh, E., Seo, P. J., and Kim, J. (2021). Peptide signaling during plant reproduction. Trends Plant Sci. doi: 10.1016/j.tplants.2021.02.008 Epub ahead of print.
Kumpf, R. P., Shi, C. L., Larrieu, A., Stø, I. M., Butenko, M. A., Péret, B., et al. (2013). Floral organ abscission peptide IDA and its HAE/HSL2 receptors control cell separation during lateral root emergence. Proc. Natl. Acad. Sci. U.S.A. 110, 5235–5240. doi: 10.1073/pnas.1210835110
Ladwig, F., Dahlke, R. I., Stührwohldt, N., Hartmann, J., Harter, K., and Sauter, M. (2015). Phytosulfokine regulates growth in Arabidopsis through a response module at the plasma membrane that includes CYCLIC NUCLEOTIDE-GATED CHANNEL17, H+-ATPase, and BAK1. Plant Cell 27, 1718–1729. doi: 10.1105/tpc.15.00306
Li, C., Yeh, F. L., Cheung, A. Y., Duan, Q., Kita, D., Liu, M. C., et al. (2015). Glycosylphosphatidylinositol-anchored proteins as chaperones and co-receptors for FERONIA receptor kinase signaling in Arabidopsis. Elife 4:e06587. doi: 10.7554/eLife.06587
Lu, X., Shi, H., Ou, Y., Cui, Y., Chang, J., Peng, L., et al. (2020). RGF1-RGI1, a peptide-receptor complex, regulates Arabidopsis root meristem development via a MAPK signaling cascade. Mol. Plant 13, 1594–1607. doi: 10.1016/j.molp.2020.09.005
Ma, D., Endo, S., Betsuyaku, S., Shimotohno, A., and Fukuda, H. (2020). CLE2 regulates light-dependent carbohydrate metabolism in Arabidopsis shoots. Plant Mol. Biol. 104, 561–574. doi: 10.1007/s11103-020-01059-y
Matsubayashi, Y. (2014). Posttranslationally modified small-peptide signals in plants. Annu. Rev. Plant Biol. 65, 385–413. doi: 10.1146/annurev-arplant-050312-120122
Mecchia, M. A., Santos-Fernandez, G., Duss, N. N., Somoza, S. C., Boisson-Dernier, A., Gagliardini, V., et al. (2017). RALF4/19 peptides interact with LRX proteins to control pollen tube growth in Arabidopsis. Science 358, 1600–1603. doi: 10.1126/science.aao5467
Nakaminami, K., Okamoto, M., Higuchi-Takeuchi, M., Yoshizumi, T., Yamaguchi, Y., Fukao, Y., et al. (2018). AtPep3 is a hormone-like peptide that plays a role in the salinity stress tolerance of plants. Proc. Natl. Acad. Sci. U.S.A. 115, 5810–5815. doi: 10.1073/pnas.1719491115
Ogilvie, H. A., Imin, N., and Djordjevic, M. A. (2014). Diversification of the C-TERMINALLY ENCODED PEPTIDE (CEP) gene family in angiosperms, and evolution of plant-family specific CEP genes. BMC Genomics 15:870. doi: 10.1186/1471-2164-15-870
Oh, E., Seo, P. J., and Kim, J. (2018). Signaling peptides and receptors coordinating plant root development. Trends Plant Sci. 23, 337–351. doi: 10.1016/j.tplants.2017.12.007
Ohama, N., Sato, H., Shinozaki, K., and Yamaguchi-Shinozaki, K. (2017). Transcriptional regulatory network of plant heat stress response. Trends Plant Sci. 22, 53–65. doi: 10.1016/j.tplants.2016.08.015
Ohkubo, Y., Tanaka, M., Tabata, R., Ogawa-Ohnishi, M., and Matsubayashi, Y. (2017). Shoot-to-root mobile polypeptides involved in systemic regulation of nitrogen acquisition. Nat. Plants 3:17029. doi: 10.1038/nplants.2017.29
Ohyama, K., Ogawa, M., and Matsubayashi, Y. (2008). Identification of a biologically active, small, secreted peptide in Arabidopsis by in silico gene screening, followed by LC-MS-based structure analysis. Plant J. 55, 152–160. doi: 10.1111/j.1365-313X.2008.03464.x
Okamoto, S., Tabata, R., and Matsubayashi, Y. (2016). Long-distance peptide signaling essential for nutrient homeostasis in plants. Curr. Opin. Plant Biol. 34, 35–40. doi: 10.1016/j.pbi.2016.07.009
Ota, R., Ohkubo, Y., Yamashita, Y., Ogawa-Ohnishi, M., and Matsubayashi, Y. (2020). Shoot-to-root mobile CEPD-like 2 integrates shoot nitrogen status to systemically regulate nitrate uptake in Arabidopsis. Nat. Commun. 11:641. doi: 10.1038/s41467-020-14440-8
Ou, Y., Lu, X., Zi, Q., Xun, Q., Zhang, J., Wu, Y., et al. (2016). RGF1 INSENSITIVE 1 to 5, a group of LRR receptor-like kinases, are essential for the perception of root meristem growth factor 1 in Arabidopsis thaliana. Cell Res. 26, 686–698. doi: 10.1038/cr.2016.63
Patharkar, O. R., and Walker, J. C. (2016). Core mechanisms regulating developmentally timed and environmentally triggered abscission. Plant Physiol. 172, 510–520. doi: 10.1104/pp.16.01004
Qin, F., Shinozaki, K., and Yamaguchi-Shinozaki, K. (2011). Achievements and challenges in understanding plant abiotic stress responses and tolerance. Plant Cell Physiol. 52, 1569–1582. doi: 10.1093/pcp/pcr106
Reddy, K. J. (2006). “Nutrient stress,” in Physiology and Molecular Biology of Stress Tolerance in Plants, eds K. V. Madhava Rao, A. S. Raghavendra, and K. J. Reddy (Dordrecht: Springer), 187–217. doi: 10.1007/1-4020-4225-6_7
Reichardt, S., Piepho, H. P., Stintzi, A., and Schaller, A. (2020). Peptide signaling for drought-induced tomato flower drop. Science 367, 1482–1485. doi: 10.1126/science.aaz5641
Roberts, I., Smith, S., Stes, E., De Rybel, B., Staes, A., Van De Cotte, B., et al. (2016). CEP5 and XIP1/CEPR1 regulate lateral root initiation in Arabidopsis. J. Exp. Bot. 67, 4889–4899. doi: 10.1093/jxb/erw231
Shao, Y., Yu, X., Xu, X., Li, Y., Yuan, W., Xu, Y., et al. (2020). The YDA-MKK4/MKK5-MPK3/MPK6 cascade functions downstream of the RGF1-RGI ligand-receptor pair in regulating mitotic activity in root apical meristem. Mol. Plant 13, 1608–1623. doi: 10.1016/j.molp.2020.09.004
Shi, Y., Ding, Y., and Yang, S. (2018). Molecular regulation of CBF signaling in cold acclimation. Trends Plant Sci. 23, 623–637. doi: 10.1016/j.tplants.2018.04.002
Shinohara, H., Mori, A., Yasue, N., Sumida, K., and Matsubayashi, Y. (2016). Identification of three LRR-RKs involved in perception of root meristem growth factor in Arabidopsis. Proc. Natl. Acad. Sci. U.S.A. 113, 3897–3902. doi: 10.1073/pnas.1522639113
Smith, S., Zhu, S., Joos, L., Roberts, I., Nikonorova, N., Dai Vu, L., et al. (2020). The CEP5 peptide promotes abiotic stress tolerance, as revealed by quantitative proteomics, and attenuates the AUX/IAA equilibrium in Arabidopsis. Mol. Cell Proteomics 19, 1248–1262. doi: 10.1074/mcp.RA119.001826
Song, W., Liu, L., Wang, J., Wu, Z., Zhang, H., Tang, J., et al. (2016). Signature motif-guided identification of receptors for peptide hormones essential for root meristem growth. Cell Res. 26, 674–685. doi: 10.1038/cr.2016.62
Stegmann, M., Monaghan, J., Smakowska-Luzan, E., Rovenich, H., Lehner, A., Holton, N., et al. (2017). The receptor kinase FER is a RALF-regulated scaffold controlling plant immune signaling. Science 355, 287–289. doi: 10.1126/science.aal2541
Stenvik, G. E., Butenko, M. A., Urbanowicz, B. R., Rose, J. K., and Aalen, R. B. (2006). Overexpression of INFLORESCENCE DEFICIENT IN ABSCISSION activates cell separation in vestigial abscission zones in Arabidopsis. Plant Cell 18, 1467–1476. doi: 10.1105/tpc.106.042036
Stenvik, G. E., Tandstad, N. M., Guo, Y., Shi, C. L., Kristiansen, W., Holmgren, A., et al. (2008). The EPIP peptide of INFLORESCENCE DEFICIENT IN ABSCISSION is sufficient to induce abscission in Arabidopsis through the receptor-like kinases HAESA and HAESA-LIKE2. Plant Cell 20, 1805–1817. doi: 10.1105/tpc.108.059139
Strabala, T. J., Phillips, L., West, M., and Stanbra, L. (2014). Bioinformatic and phylogenetic analysis of the CLAVATA3/EMBRYO-SURROUNDING REGION (CLE) and the CLE-LIKE signal peptide genes in the Pinophyta. BMC Plant Biol. 14:47. doi: 10.1186/1471-2229-14-47
Stührwohldt, N., Bühler, E., Sauter, M., and Schaller, A. (2021). Phytosulfokine (PSK) precursor processing by subtilase SBT3.8 and PSK signaling improve drought stress tolerance in Arabidopsis. J. Exp. Bot. 72, 3427–3440. doi: 10.1093/jxb/erab017
Stührwohldt, N., Dahlke, R. I., Steffens, B., Johnson, A., and Sauter, M. (2011). Phytosulfokine-α controls hypocotyl length and cell expansion in Arabidopsis thaliana through phytosulfokine receptor 1. PLoS One 6:e21054. doi: 10.1371/journal.pone.0021054
Suzuki, N., Rivero, R. M., Shulaev, V., Blumwald, E., and Mittler, R. (2014). Abiotic and biotic stress combinations. New Phytol. 203, 32–43. doi: 10.1111/nph.12797
Tabata, R., Sumida, K., Yoshii, T., Ohyama, K., Shinohara, H., and Matsubayashi, Y. (2014). Perception of root-derived peptides by shoot LRR-RKs mediates systemic N-demand signaling. Science 346, 343–346. doi: 10.1126/science.1257800
Takahashi, F., Hanada, K., Kondo, T., and Shinozaki, K. (2019). Hormone-like peptides and small coding genes in plant stress signaling and development. Curr. Opin. Plant Biol. 51, 88–95. doi: 10.1016/j.pbi.2019.05.011
Takahashi, F., Suzuki, T., Osakabe, Y., Betsuyaku, S., Kondo, Y., Dohmae, N., et al. (2018). A small peptide modulates stomatal control via abscisic acid in long-distance signalling. Nature 556, 235–238. doi: 10.1038/s41586-018-0009-2
Tang, R. J., Wang, C., Li, K., and Luan, S. (2020). The CBL-CIPK calcium signaling network: unified paradigm from 20 years of discoveries. Trends Plant Sci. 25, 604–617. doi: 10.1016/j.tplants.2020.01.009
Vie, A. K., Najafi, J., Liu, B., Winge, P., Butenko, M. A., Hornslien, K. S., et al. (2015). The IDA/IDA-LIKE and PIP/PIP-LIKE gene families in Arabidopsis: phylogenetic relationship, expression patterns, and transcriptional effect of the PIPL3 peptide. J. Exp. Bot. 66, 5351–5365. doi: 10.1093/jxb/erv285
Vie, A. K., Najafi, J., Winge, P., Cattan, E., Wrzaczek, M., Kangasjärvi, J., et al. (2017). The IDA-LIKE peptides IDL6 and IDL7 are negative modulators of stress responses in Arabidopsis thaliana. J. Exp. Bot. 68, 3557–3571. doi: 10.1093/jxb/erx168
Wang, J., Li, H., Han, Z., Zhang, H., Wang, T., Lin, G., et al. (2015). Allosteric receptor activation by the plant peptide hormone phytosulfokine. Nature 525, 265–268. doi: 10.1038/nature14858
Yamada, M., Han, X., and Benfey, P. N. (2020). RGF1 controls root meristem size through ROS signalling. Nature 577, 85–88. doi: 10.1038/s41586-019-1819-6
Yamaguchi, Y., and Huffaker, A. (2011). Endogenous peptide elicitors in higher plants. Curr. Opin. Plant Biol. 14, 351–357. doi: 10.1016/j.pbi.2011.05.001
Yamaguchi, Y., Huffaker, A., Bryan, A. C., Tax, F. E., and Ryan, C. A. (2010). PEPR2 is a second receptor for the Pep1 and Pep2 peptides and contributes to defense responses in Arabidopsis. Plant Cell 22, 508–522. doi: 10.1105/tpc.109.068874
Yamaguchi, Y., Pearce, G., and Ryan, C. A. (2006). The cell surface leucine-rich repeat receptor for AtPep1, an endogenous peptide elicitor in Arabidopsis, is functional in transgenic tobacco cells. Proc. Natl. Acad. Sci. U.S.A. 103, 10104–10109. doi: 10.1073/pnas.0603729103
Zhang, L., Shi, X., Zhang, Y., Wang, J., Yang, J., Ishida, T., et al. (2019). CLE9 peptide-induced stomatal closure is mediated by abscisic acid, hydrogen peroxide, and nitric oxide in Arabidopsis thaliana. Plant Cell Environ. 42, 1033–1044. doi: 10.1111/pce.13475
Zhao, C., Zayed, O., Yu, Z., Jiang, W., Zhu, P., Hsu, C. C., et al. (2018). Leucine-rich repeat extensin proteins regulate plant salt tolerance in Arabidopsis. Proc. Natl. Acad. Sci. U.S.A. 115, 13123–13128. doi: 10.1073/pnas.1816991115
Zhu, J. K. (2016). Abiotic stress signaling and responses in plants. Cell 167, 313–324. doi: 10.1016/j.cell.2016.08.029
Keywords: signaling peptides, receptor-like kinases, dehydration, salt, reactive oxygen species
Citation: Kim JS, Jeon BW and Kim J (2021) Signaling Peptides Regulating Abiotic Stress Responses in Plants. Front. Plant Sci. 12:704490. doi: 10.3389/fpls.2021.704490
Received: 14 May 2021; Accepted: 25 June 2021;
Published: 19 July 2021.
Edited by:
Fuminori Takahashi, Tokyo University of Science, JapanReviewed by:
Farida Minibayeva, Kazan Institute of Biochemistry and Biophysics (RAS), RussiaLinhui Yu, Brookhaven National Laboratory, United States
Copyright © 2021 Kim, Jeon and Kim. This is an open-access article distributed under the terms of the Creative Commons Attribution License (CC BY). The use, distribution or reproduction in other forums is permitted, provided the original author(s) and the copyright owner(s) are credited and that the original publication in this journal is cited, in accordance with accepted academic practice. No use, distribution or reproduction is permitted which does not comply with these terms.
*Correspondence: Jungmook Kim, jungmkim@jnu.ac.kr