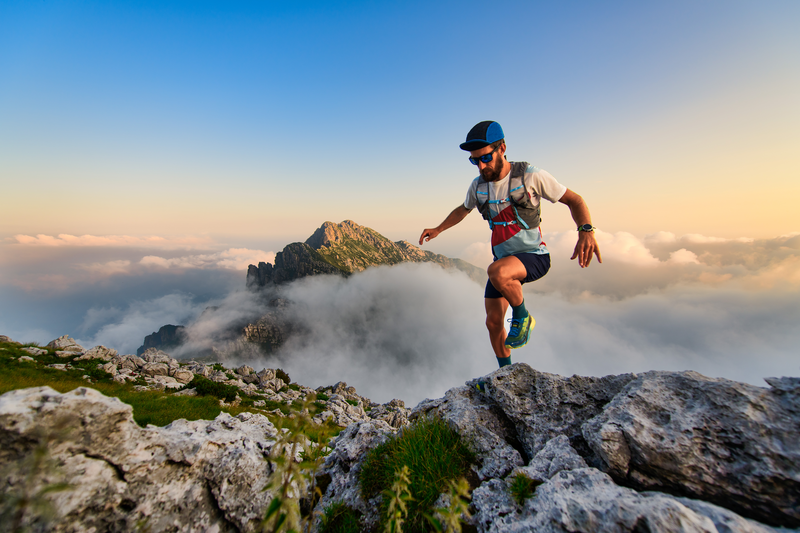
94% of researchers rate our articles as excellent or good
Learn more about the work of our research integrity team to safeguard the quality of each article we publish.
Find out more
ORIGINAL RESEARCH article
Front. Plant Sci. , 23 July 2021
Sec. Plant Abiotic Stress
Volume 12 - 2021 | https://doi.org/10.3389/fpls.2021.702892
This article is part of the Research Topic Photosynthetic Efficiency under Multiple Stress Conditions: Prospects for Increasing Crop Yields View all 24 articles
Rice yield has decreased due to climate variability and change in Colombia. Plant growth regulators have been used as a strategy to mitigate heat stress in different crops. Therefore, this study aimed to evaluate the effect of foliar applications of four growth regulators [auxins (AUX), brassinosteroids (BR), cytokinins (CK), or gibberellins (GA)] on physiological (stomatal conductance, total chlorophyll content, Fv/Fm ratio, plant canopy temperature, and relative water content) and biochemical (Malondialdehyde (MDA) and proline contents) variables in two commercial rice genotypes exposed to combined heat stress (high day and nighttime temperatures). Two separate experiments were carried out using plants of two rice genotypes, Fedearroz 67 (“F67”) and Fedearroz 2000 (“F2000”) for the first and second experiments, respectively. Both trials were analyzed together as a series of experiments. The established treatments were as follows: absolute control (AC) (rice plants grown under optimal temperatures (30/25°C day/nighttime temperatures), heat stress control (SC) [rice plants only exposed to combined heat stress (40/30°C)], and stressed rice plants and sprayed twice (5 days before and after heat stress) with a plant growth regulator (stress+AUX, stress+BR, stress+CK, or stress+GA). The results showed that foliar CK sprays enhanced the total chlorophyll content in both cultivars (3.25 and 3.65 mg g−1 fresh weight for “F67” and “F2000” rice plants, respectively) compared to SC plants (2.36 and 2.56 mg g−1 fresh weight for “F67,” and “F2000” rice plants, respectively). Foliar CK application also improved stomatal conductance mainly in “F2000” rice plants compared to their heat stress control (499.25 vs.150.60 mmol m−2s−1). Foliar BR or CK sprays reduced plant canopy temperature between 2 and 3°C and MDA content in plants under heat stress. The relative tolerance index suggested that foliar CK (97.69%), and BR (60.73%) applications helped to mitigate combined heat stress mainly in “F2000” rice plants. In conclusion, foliar BR or CK applications can be considered an agronomic strategy to help to ameliorate the negative effect of combined heat stress conditions on the physiological behavior of rice plants.
Rice (Oryza sativa) belongs to the Gramineae family and is one of the most cultivated cereals around the world together with maize and wheat (Bajaj and Mohanty, 2005). Rice occupied 617,934 ha with a national production volume of 2,937,840 t and an average yield of 5.02 t ha−1 in 2020 (Federarroz (Federación Nacional de Arroceros), 2021).
Rice crops are being affected by global warming, causing different types of abiotic stress, such as high temperatures and drought periods (Wassmann et al., 2009; Chandio et al., 2020). Climate change has increased the global temperature around the world; it has been predicted that temperature will increase by 1.0–3.7°C during the twenty-first century, which will potentially increment the frequency and magnitude of heat stress events (IPCC, 2013). This increase in environmental temperatures has affected rice, generating a decrease between 6 and 7% in crop production (Lesk et al., 2016). On the other hand, climate variability has also generated adverse environmental conditions in crops such as periods of intense drought or high temperatures in the tropical and subtropical regions (Feller and Vaseva, 2014). Additionally, variability phenomena such as the “El Niño” phenomenon can cause heat stress conditions and aggravate the damage on crops in some tropical regions (Iizumi et al., 2014; Amanullah et al., 2017). In Colombia, rice-producing areas will expect increases in temperatures between 2 and 2.5°C by 2050, reducing rice yields and making an impact on product flows to markets and supply chains (Ramirez-Villegas et al., 2012).
The majority of rice crops are grown in regions where temperatures are close to the optimum range for crop growth (Shah et al., 2011). It has been reported that the optimal average temperatures for rice growth and development are usually 28 and 22°C for day and night, respectively (Kilasi et al., 2018; Calderón-Páez et al., 2021). Temperatures above those thresholds can cause moderate or severe heat stress periods during sensitive rice development stages (tillering, anthesis, flowering, and grain filling), negatively affecting grain yield (Shah et al., 2011). These yield reductions have been mainly associated with prolonged heat stress periods (more than 7 days) that affect plant physiology (Shah et al., 2011; Sánchez-Reinoso et al., 2014). Heat stress can cause a series of irreversible damages in plant metabolism and development due to the interaction of different factors such as the stress duration, or the maximum temperature reached (Porch Clay and Hall, 2013; Zhou et al., 2014).
Heat stress affects different physiological and biochemical processes in plants (Bita and Gerats, 2013; Chavez-Arias et al., 2018). Leaf photosynthesis is one of the most susceptible processes to heat stress in rice plants since the photosynthetic rate can decrease by 50% when daytime temperatures exceed 35°C (Restrepo-Diaz and Garces-Varon, 2013). The physiological response varies according to the type of heat stress in rice plants. For example, the photosynthetic rate and stomatal conductance are inhibited when plants are exposed to high day temperatures (33–40°C) or to a combination of high day and night temperatures (35 to 40°C for day, and 28 to 30°C for night) (Lü et al., 2013; Fahad et al., 2016; Chaturvedi et al., 2017). A high nighttime temperature (30°C) caused a moderate inhibition of photosynthesis but enhanced nighttime respiration (Fahad et al., 2016; Alvarado-Sanabria et al., 2017). Heat stress also affects leaf chlorophyll content, the ratio of variable to maximum chlorophyll fluorescence (Fv/Fm), and the activation of Rubisco in rice plants regardless of the period of stress (Cao et al., 2009; Yin et al., 2010; Sánchez-Reinoso et al., 2014).
Biochemical changes are another aspect of plant acclimatization to heat stress (Wahid et al., 2007). Proline content has been used as a biochemical indicator of stress in plants (Ahmed and Hassan, 2011). Proline plays an important role in plant metabolism since it acts as a source of C or N and as a membrane stabilizer under high temperature conditions (Sánchez-Reinoso et al., 2014). High temperatures also affect membrane stability by lipid peroxidation, leading to malondialdehyde (MDA) production (Wahid et al., 2007). Therefore, the MDA content has also been used to know the structural integrity of cell membranes under heat stress (Cao et al., 2009; Chavez-Arias et al., 2018). Finally, combined heat stress [37/30°C (day/night)] increased the electrolyte leakage percentage and MDA content in rice plants (Liu et al., 2013).
The use of plant growth regulators (GRs) has been evaluated to mitigate the negative effects of heat stress since these substances are actively involved in plant responses or mechanisms to develop physiological protection against this kind of stress (Peleg and Blumwald, 2011; Yin et al., 2011; Ahammed et al., 2015). The exogenous application of GRs has shown a positive response in plant tolerance to heat stress in different crops. Studies have observed that plant hormones such as gibberellins (GA), cytokinins (CK), auxins (AUX), or brassinosteroids (BR) have caused an increase in different physiological and biochemical variables (Peleg and Blumwald, 2011; Yin et al., 2011; Mittler et al., 2012; Zhou et al., 2014). In Colombia, the exogenous application of GRs and their effects are still not well-elucidated or studied in rice crops. However, a previous study showed that foliar BR applications enhanced rice tolerance by improving leaf gas exchange properties, chlorophyll, or proline content in rice seedlings (Quintero-Calderón et al., 2021).
Cytokinins mediate plant responses to abiotic stress, including heat stress (Ha et al., 2012). Also, it has been reported that exogenous applications of CK can reduce heat damages. For example, exogenous applications of zeatin improved the photosynthesis rate, chlorophyll a and b contents, and electron transport efficiency during heat stress in creeping bentgrass (Agrotis estolonífera) (Xu and Huang, 2009; Jespersen and Huang, 2015). Exogenous applications of zeatin also improved the antioxidant activity and enhanced the synthesis of different proteins, decreasing the damage of reactive oxygen species (ROS), and the production of malondialdehyde (MDA) in plant tissues (Chernyad'ev, 2009; Yang et al., 2016; Kumar et al., 2020).
Gibberellic acid applications have also exhibited a positive response to heat stress. Studies have shown that GA biosynthesis mediates different metabolic pathways and enhances tolerance under high temperature conditions (Alonso-Ramirez et al., 2009; Khan et al., 2020). Abd El-Naby et al. (2020) found that exogenous foliar application of GA (25 or 50 mg*L) increased the photosynthetic rate and antioxidant activity in heat-stressed orange plants compared to control plants. It has also been observed that the exogenous application of GA enhanced the relative water content, chlorophyll and carotenoids content, and diminished lipid peroxidation in date palm (Phoenix dactylifera) under heat stress (Khan et al., 2020). Auxins also play an essential role in regulating adaptive growth responses to high temperature conditions (Sun et al., 2012; Wang et al., 2016). This growth regulator works as a biochemical marker during different processes such as the synthesis or degradation of proline under abiotic stress (Ali et al., 2007). Also, AUX enhance the antioxidant activity, causing a decrease in MDA in the plant because of lower lipid peroxidation (Bielach et al., 2017). Sergiev et al. (2018) observed that the proline content increased when garden pea (Pisum sativum) plants were treated with auxin analogs [1-(2-chloroethoxycarbonyl –methyl)-4-naphthalene sulfonic acid calcium salt (TA-12) and 1-(2-dimethylaminoethoxicarbonylmethyl) naphthalene chlormethylate (TA-14)] under heat stress. In the same experiment, they also observed that the MDA levels were lower in treated plants compared to plants without AUX application.
Brassinosteroids are another group of growth regulators that have been used to mitigate the effects of heat stress. Ogweno et al. (2008) reported that exogenous BR sprays increased the net photosynthetic rate, stomatal conductance, and maximum carboxylation rate of Rubisco in tomato (Solanum lycopersicum) plants under heat stress for 8 days. The foliar spray of epibrassinosteroid caused a higher net photosynthetic rate in cucumber (Cucumis sativus) plants under heat stress (Yu et al., 2004). Also, exogenous applications of BR delayed chlorophyll degradation and enhanced water use efficiency and maximum quantum yield of PSII photochemistry in heat-stressed plants (Holá et al., 2010; Thussagunpanit et al., 2015).
Rice crops are facing periods of high day and night temperatures due to climate change and variability (Lesk et al., 2016; Garcés, 2020; Federarroz (Federación Nacional de Arroceros), 2021). In plant phenotyping, the use of plant nutrients or biostimulants has been studied as a strategy to mitigate heat stress in rice-cultivating areas (Alvarado-Sanabria et al., 2017; Calderón-Páez et al., 2021; Quintero-Calderón et al., 2021). Additionally, the use of biochemical and physiological variables (leaf canopy temperature, stomatal conductance, chlorophyll fluorescence parameters, chlorophyll and relative water contents, malondialdehyde and proline synthesis) are reliable tools to screen rice under heat stress at local and international levels (Sánchez-Reinoso et al., 2014; Alvarado-Sanabria et al., 2017; Sarsu et al., 2018). However, research on the use of foliar plant hormone sprays in rice plants is still scarce at the local level. Therefore, the study of physiological and biochemical responses to the use of plant growth regulators is important to propose practical agronomic strategies to ameliorate the negative effects of combined heat stress periods in rice. In consequence, the objective of this research was to evaluate the effect of the foliar applications of four plant growth regulators (AUX, CK, GA, and BR) on the physiological (stomatal conductance, chlorophyll fluorescence parameters, and relative water content) and biochemical (photosynthetic pigments, malondialdehyde, and proline content) variables of two commercial rice genotypes subjected to combined heat stress (high day/nighttime temperatures).
Two separate experiments were carried out in this study. Rice seeds of genotypes Fedearroz 67 (F67: genotype bred under high-temperature conditions in the last decade) and Fedearroz 2000 (F2000: genotype bred in the last decade of the twentieth century that shows tolerance to White Leaf Virus) were used in the first and second experiments, respectively. Both genotypes are widely grown by Colombian farmers. Seeds were sown in 10 L trays (39.6 cm length × 28.8 cm width × 16.8 cm height), containing sandy loam soil with 2% organic matter. Five pregerminated seeds were planted in each tray. The trays were set in a greenhouse at the Faculty of Agricultural Sciences of the Universidad Nacional de Colombia, Bogotá campus (43°50′56″ N, 74°04′051″ W), at an altitude of 2,556 m above sea level (m.a.s.l.), from October to December 2019 for the first experiment (Fedearroz 67), and in the same season in 2020 for the second experiment (Fedearroz 2000).
The environmental conditions in the greenhouse during each sowing season were as follows: 30/25°C day/night temperature, 60–80% relative humidity, and a natural photoperiod of 12 h (photosynthetically active radiation of 1,500 μmol (photons) m−2 s−1 at noon). At 20 days after seed emergence (DAE), plants were fertilized following the amounts of each element according to Sánchez-Reinoso et al. (2019): 670 mg of N per plant, 110 mg of P per plant, 350 mg of K per plant, 68 mg of Ca per plant, 20 mg of Mg per plant, 20 mg of S per plant, 17 mg of Si per plant, 10 mg of B per plant, 17 mg of Cu per plant, and 44 mg of Zn per plant. Rice plants were maintained under those conditions up to 47 DAE in each experiment as rice plants reached the phenological stage V5 during that period. Previous studies have shown that this phenological stage is an appropriate time to perform heat stress studies in rice plants (Sánchez-Reinoso et al., 2014; Alvarado-Sanabria et al., 2017).
Two separated applications of foliar growth regulators were performed in each experiment. The first group of foliar plant hormone sprays was performed 5 days before heat stress treatments (42 DAE) to prepare plants for environmental stress. Then, the second foliar sprays were carried out 5 days after subjecting plants to stress conditions (52 DAE). Four plant hormones were used, and the characteristics of each active ingredient sprayed in this study are mentioned in Supplementary Table 1. The concentrations of the foliar growth regulators used were as follows: (i) auxins (1-Naphthaleneacetic acid: NAA) at 5 × 10−5 M; (ii) gibberellins (Gibberellic acid: GA3) at 5 × 10−5 M; (iii) cytokinins (Trans-Zeatin) at 1 × 10−5 M; and (iv) brassinosteroids [Spirostan-6-one, 3,5-dihydroxy-, (3b,5a,25R)] at 5 × 10−5 M. These concentrations were selected since they showed a positive response and increased plant tolerance to heat stress (Zahir et al., 2001; Wen et al., 2010; El-Bassiony et al., 2012; Salehifar et al., 2017). Rice plants without any plant growth regulator sprays were only treated with distilled water. All rice plants were sprayed using a hand sprayer. The application volume was 20 ml H2O per plant, wetting the upper and lower leaf surfaces. All foliar applications were performed with an agricultural adjuvant (Agrotin, Bayer CropScience, Colombia) at a dose of 0.1% (v/v). The distance between the pot and the atomizer sprayer was 30 cm.
Heat stress treatments were carried out 5 days after the first foliar sprays (47 DAE) in each experiment. Rice plants were transferred from the greenhouse to growth chambers of 294 L of capacity (MLR-351H, Sanyo, Illinois, US) to establish heat stress or continue with the previous environmental conditions (47 DAE). The combined heat stress treatment was performed by setting the chambers with the following day/nighttime temperatures: a period of high daytime [40°C for 5 h (from 11:00 to 16:00)] and nighttime [30°C for 5 h (from 19:00 to 24:00)] for 8 consecutive days. Stress temperatures and exposure time were selected based on previous studies (Sánchez-Reinoso et al., 2014; Alvarado-Sanabria et al., 2017). On the other hand, a group of plants transferred to the growth chamber was kept at the same temperature of the greenhouse (30°C day/25°C night) for 8 consecutive days.
At the end of the experiment, the following groups of treatments were obtained: (i) growth temperature conditions + distilled water applications [absolute control (AC)], (ii) heat stress conditions + distilled water applications [heat stress control (SC)], (iii) heat stress condition + auxins (AUX) applications, (iv) heat stress condition + gibberellins (GA) applications, (v) heat stress condition + cytokinins (CK) applications, and (vi) heat stress condition + brassinosteroids (BR) applications. These groups of treatments were used in both genotypes (F67 and F2000). All treatments were arranged in a completely randomized design with five replicates, and each replicate consisted of one plant. Each plant was used for readings of the variables determined at the end of the experiment. The experiment lasted 55 DAE.
Stomatal conductance (gs) was determined using a portable porometer (SC-1, METER Group Inc., USA) with a range from 0 to 1,000 mmol m−2s−1, and a sample chamber aperture of 6.35 mm. Measurements were taken by clipping the sensor of the porometer onto a fully expanded mature leaf from the main tiller of the plant. gs readings were taken between 11:00 and 16:00 h on three leaves per plant in each treatment, and values were averaged.
The RWC was determined following the method described by Ghoulam et al. (2002). A fully expanded leaf used to determine gs was also used to measure RWC. The fresh weight (FW) was immediately determined after harvesting using a digital balance. Subsequently, leaves were placed inside plastic recipients with water under dark conditions and room temperature (22°C) for 48 h. Then, they were weighed on a digital balance to record the turgid weight (TW). Turgid leaves were dried in an oven at 75°C for 48 h and their dry weight (DW) was registered.
The relative chlorophyll content was determined using a chlorophyll meter (atLeaf meter, FT Green LLC, US) and expressed as atLeaf units (Dey et al., 2016). The readings of maximum quantum efficiency of PSII (Fv/Fm ratio) were recorded using a continuous excitation chlorophyll fluorimeter (Handy PEA, Hansatech Instruments, UK). Before taking Fv/Fm measurements, leaves were adapted to darkness using leaf clips for 20 min (Restrepo-Diaz and Garces-Varon, 2013). After leaf adaptation in the dark, Baseline (F0) and maximum fluorescence (Fm) were measured. The variable fluorescence (Fv = Fm – F0), the ratio of variable to maximum fluorescence (Fv/Fm), the maximum quantum yield of PSII photochemistry (Fv/F0), and the Fm/F0 ratio were calculated based on these data (Baker, 2008; Li et al., 2017). The readings of relative chlorophyll and chlorophyll fluorescence parameters were taken on the same leaves used for gs readings.
Approximately 800 mg of leaf fresh weight was collected for biochemical variables. Then, leaf samples were homogenized in liquid nitrogen and stored for further analysis. The spectral determination method used to estimate chlorophyll a, b, and carotenoid content on tissue was based on the methodology and the equations described by Wellburn (1994). Leaf tissue samples (30 mg) were collected and homogenized in 3 mL of 80% acetone. Then, the samples were centrifuged (Model 420101, Becton Dickinson Primary Care Diagnostics, USA) at 5,000 rpm for 10 min to remove particles. The supernatant was diluted to a final volume of 6 ml by adding 80% acetone (Sims and Gamon, 2002). Chlorophyll content was determined at 663 (chlorophyll a) and 646 (chlorophyll b) nm, whereas carotenoids were estimated at 470 nm using a spectrophotometer (Spectronic BioMate 3 UV-vis, Thermo, USA).
The thiobarbituric acid (TBA) method described by Hodges et al. (1999) was used to estimate membrane lipid peroxidation (MDA). Approximately 0.3 g of leaf tissue was also homogenized in liquid nitrogen. The samples were centrifuged at 5,000 rpm, and then the absorbances were measured at 440, 532, and 600 nm with the spectrophotometer. Finally, an extinction coefficient (157 M·ml−1) was used to obtain the MDA concentration.
Proline content was determined for all treatments using the method described by Bates et al. (1973). Ten milliliters of a 3% aqueous solution of sulfosalicylic acid was added to the stored samples, which were then filtered with Whatman paper (No. 2). Subsequently, 2 mL of this filtrate was reacted with 2 mL of ninhydrin acid and 2 mL of glacial acetic acid. The mixture was placed in a water bath at 90°C for 1 h. The reaction was stopped by incubation on ice. The resulting solution was dissolved in 4 mL of toluene by shaking the test tubes vigorously using a vortex shaker. Absorbance readings were determined at 520 nm with the same spectrophotometer used in the quantification of photosynthetic pigments (Spectronic BioMate 3 UV-Vis, Thermo, Madison, WI, USA).
The methodology described by Gerhards et al. (2016) was used to calculate plant canopy temperature and CSI. Thermal photographs were taken with a FLIR 2 camera (FLIR Systems Inc., Boston, MA, USA) with an accuracy of ±2°C at the end of the stress period. A white surface was placed behind plants for photography. Similarly, two plants were considered as the reference pattern. These plants were placed on a white surface; one of them was covered with an agricultural adjuvant (Agrotin, Bayer CropScience, Bogotá, Colombia) to simulate the opening of all stomata [wet pattern (Twet)], and the other was a leaf without any application [dry pattern (Tdry)] (Castro-Duque et al., 2020). The photography was taken with a distance of 1 m between the camera and the pot.
The relative tolerance index was calculated indirectly to determine the tolerance of the treated genotypes evaluated in this research, using the stomatal conductance (gs) of treated plants in relation to control plants (plants without stress treatment and application of growth regulators). RTI was obtained using the equation adapted from Chávez-Arias et al. (2020).
All physiological variables described above were determined and recorded at 55 DAE in each experiment using fully expanded leaves collected from the upper portion of the canopy. Also, the measurements were carried out inside the growth chamber to avoid altering the environmental conditions in which the plants were grown.
Data from the first and second experiments were analyzed together as a series of experiments. Each treatment group consisted of five plants, and each plant was an experimental unit. An analysis of variance (ANOVA) was performed (P ≤ 0.05). When significant differences were found, the comparative Tukey post hoc test was used at P ≤ 0.05. Percentage values were transformed using the arcsine function. Data were analyzed using the Statistix v 9.0 software (Analytical Software, Tallahassee, FL, USA), and SigmaPlot (version 10.0; Systat Software, San Jose, CA, USA) was used for the figures. A principal analysis of components was performed with InfoStat 2016 software (analytical software, Universidad Nacional de Córdoba, Argentina) to identify the better plant growth regulators in the study.
Table 1 summarizes the analysis of variance that shows the effect of the experiments, the different treatments, and their interaction on leaf photosynthetic pigments (chlorophyll a, b, total content, and carotenoids), malondialdehyde (MDA) and proline contents, stomatal conductance (gs), relative water content (RWC), chlorophyll content, chlorophyll α fluorescence parameters, plant canopy temperature (PCT) (°C), crop stress index (CSI), and relative tolerance index of rice plants at 55 DAE.
Table 1. Summary of the analysis of variance between experiment (genotypes) and heat stress treatments on the physiological and biochemical variables of rice plants.
Differences (P ≤ 0.01) in the interaction between experiments and treatments on leaf photosynthetic pigments, relative chlorophyll content (Atleaf readings), and the chlorophyll α fluorescence parameters are shown in Table 2. High day/nighttime temperatures improved the total chlorophyll and carotenoids contents. Rice seedlings without any foliar plant hormone sprays (2.36 mg g−1 for “F67,” and 2.56 mg g−1 for “F2000”) showed a lower total chlorophyll content compared to plants grown at optimal temperature conditions (2.67 mg g−1 for “F67,” and 2.80 mg g−1 for “F2000”) in both experiments. Additionally, rice seedlings under heat stress and treated with AUX and GA sprays also showed a decrease in the chlorophyll content in both genotypes (AUX = 1.96 mg g−1 and GA = 1.45 mg g−1 for “F67;” AUX = 2.24 mg g−1 and GA = 1.43 mg g−1 for “F2000”) under heat stress conditions. Foliar BR treatments caused a slight increase in this variable in both genotypes under heat stress conditions. Finally, foliar CK sprays showed the highest photosynthetic pigment values of all treatments (AUX, GA, BR, SC, and AC treatments) in genotypes F67 (3.24 mg g−1) and F2000 (3.65 mg g−1). The relative chlorophyll content (Atleaf units) was also diminished by the combined heat stress. The highest values were also recorded in plants sprayed with CK in both genotypes (41.66 for “F67”, and 49.30 for “F2000”). The Fv and Fv/Fm ratio showed significant differences between the treatments and cultivars (Table 2). In general, the cultivar F67 was less affected by heat stress than “F2000” in these variables. The Fv and Fv/Fm ratio were more affected in the second experiment. Stressed “F2000” seedlings without any plant hormone sprays registered the lowest Fv (2120.15) and Fv/Fm ratio (0.59) values; however, foliar CK sprays helped these variables to recover (Fv: 2591.89, and Fv/Fm ratio: 0.73), obtaining similar readings to those recorded in “F2000” plants grown under optimal temperature conditions (Fv: 2955.35, and Fv/Fm ratio: 0.72). There were no significant differences in the initial fluorescence (F0), maximum fluorescence (Fm), maximum quantum yield of PSII photochemistry (Fv/F0), and the Fm/F0 ratio. Finally, BR showed similar trends to those observed with the application of CK (2545.06 for Fv and 0.73 for Fv/Fm ratio).
Table 2. Effect of combined heat stress (40°/30°C day/night) on leaf photosynthetic pigments [total chlorophyll (Chl total), chlorophyll a (Chl a), chlorophyll b (Chl b) and carotenoids Cx+c)], relative chlorophyll content (Atleaf units), and chlorophyll fluorescence parameters (initial fluorescence (F0), maximum fluorescence (Fm), variable fluorescence (Fv), maximum efficiency of PSII (Fv/Fm), maximum quantum yield of PSII photochemistry (Fv/F0), and the Fm/F0 ratio) in plants of two rice genotypes [Fedearroz 67 (F67) and Fedearroz 2000 (F2000)] at 55 days after emergence (DAE).
The relative water content (RWC) of rice plants subjected to the different treatments showed differences (P ≤ 0.05) in the interaction between experiments and foliar treatments (Figure 1A). The SC treatment registered the lowest values in both genotypes (74.01% for F67 and 76.6% for F2000). A significant increase in RWC was registered in rice plants of both genotypes treated with different plant hormones under heat stress. In general, the foliar CK, GA, AUX or BR applications enhanced RWC, reaching similar values to those of plants grown under optimal conditions during the experiments. The values recorded for the absolute control and foliar sprayed plants were around 83% for both genotypes. On other hand, gs also showed significant differences (P ≤ 0.01) in the interaction between experiments and treatments (Figure 1B). Absolute control plants (AC) also registered the highest values in each genotype (440.65 mmol m−2s−1 for F67 and 511.02 mmol m−2s−1 for F2000). Rice plants only subjected to combined heat stress showed the lowest gs values for both genotypes (150.60 mmol m−2s−1 for F67 and 171.32 mmol m−2s−1 for F2000). gs was also enhanced by foliar applications of all plant growth regulators. The effects of foliar plant hormone sprays were more notorious in “F2000” rice plants sprayed with CK. This group of plants did not show differences compared to the absolute control plants (511.02 for AC and 499.25 mmol m−2s−1for CK).
Figure 1. Effect of combined heat stress (40°/30°C day/night) on the relative water content (RWC) (A), stomatal conductance (gs) (B), malondialdehyde production (MDA) (C), and proline content (D) in plants of two rice genotypes (F67 and F2000) at 55 days after emergence (DAE). The treatments evaluated in each genotype were: absolute control (AC), heat stress control (SC), heat stress + auxins (AUX), heat stress + gibberellins (GA), heat stress + cytokinins (CK), and heat stress + brassinosteroids (BR). Each column represents the mean of five data ± standard error (n = 5). Bars followed by different letters indicate statistically significant differences according to the Tukey test (P ≤ 0.05). Equal letters indicate that means are not statistically significant ( ≤ 0.05).
MDA (P ≤ 0.01) and proline (P ≤ 0.01) contents also showed significant differences in the interaction between experiments and plant hormone treatments (Figures 1C,D). An increase was observed in lipid peroxidation in SC treatments in both genotypes (Figure 1C); however, plants treated with foliar growth regulator sprays showed a decrease in lipid peroxidation for both genotypes. In general, the use of plant hormones (CK, AUX, BR, or GA) caused a reduction in lipid peroxidation (MDA content). Differences were not obtained between AC plants and plants under heat stress and sprayed with plant hormones in both genotypes (observed values between 4.38 and 6.77 μmol g−1 of FW in “F67” plants and 2.84 and 9.18 μmol g−1 of FW “F2000” plants). On the other hand, proline synthesis was lower in “F67” plants than in “F2000” plants under combined stress. The foliar AUX or BR applications also caused a higher proline production in rice plants subjected to heat stress in both experiments. It was observed that the use of these hormones notoriously increased the amino acid content (30.44 and 18.34 μmol g−1 of FW for AUX and BR, respectively) in “F2000” plants (Figure 1D).
The effect of foliar plant growth regulator sprays and combined heat stress on plant canopy temperature, and relative tolerance index (RTI) is shown in Figures 2A,B. AC plants registered temperatures close to 27°C on the plant canopy whereas SC plants showed a canopy temperature around 28°C for both genotypes. It was also observed that foliar CK and BR treatments caused a higher decrease in the plant canopy temperature between 2 and 3°C compared to SC plants (Figure 2A). The RTI showed similar behavior to the other physiological variables, showing significant differences (P ≤ 0.01) in the interactions between experiments and treatments (Figure 2B). SC plants showed lower plant tolerance in both genotypes (34.18 and 33.52% for “F67” and “F2000” rice plants, respectively). The foliar application of plant hormones improved the RTI in plants exposed to stressful temperatures. This effect was more notorious in “F2000” plants sprayed with CK, registering an RTI of 97.69. On the other hand, significant differences were only observed on the crop stress index (CSI) of rice plants subjected to the stress condition (P ≤ 0.01) in the factor foliar sprays (Figure 2C). Rice plants only exposed to combined heat stress showed the highest stress index values (0.816). The stress index was lower (values between 0.6 and 0.67) when rice plants were sprayed with the different plant hormones. Finally, rice plants grown under optimal conditions showed a value of 0.138.
Figure 2. Effect of combined heat stress (40°/30°C day/night) on plant canopy temperature (A), the relative tolerance index (RTI) (B), and crop stress index (CSI) (C) of two commercial rice genotypes (F67 and F2000) exposed to different heat treatments. The treatments evaluated in each genotype were: absolute control (AC), heat stress control (SC), heat stress + auxins (AUX), heat stress + gibberellins (GA), heat stress + cytokinins (CK), and heat stress + brassinosteroids (BR). The combined heat stress consisted of exposing rice plants to high day/nighttime temperatures (40°/30°C day/night). Each column represents the mean of five data ± standard error (n = 5). Bars followed by different letters indicate statistically significant differences according to the Tukey test (P ≤ 0.05). Equal letters indicate that means are not statistically significant ( ≤ 0.05).
The principal component analysis (PCA) showed that the variables evaluated at 55 DAE explained 66.1% of the physiological and biochemical responses of heat-stressed rice plants and treated with growth regulator sprays (Figure 3). Vectors represent the variables, whereas points identify plant growth regulators (GR). The vectors of gs, chlorophyll content, maximum quantum efficiency of PSII (Fv/Fm), and biochemical readings (TChl, MDA, and proline) have angles close to the origin, showing that there is a high correlation between plant physiological behavior and these variables. The rice seedlings grown at optimal temperatures (AC) and F2000 plants treated with CK and BR form a single group (V). Also, most of the plants treated with GR form another single group (IV), and the GA treatment in F2000 forms a single group (II). In contrast, heat-stressed rice seedlings without any foliar plant hormone sprays (SC for both genotypes) (group I and III) are located in the sector opposite to group V, showing a negative effect of heat stress on plant physiological and biochemical responses.
Figure 3. Effect of combined heat stress (40°/30°C day/night) on the biplot analysis of plants of two rice genotypes (F67 and F2000) at 55 days after emergence (DAE). Abbreviations: AC F67, absolute control F67; SC F67, heat stress control F67; AUX F67, heat stress + auxins F67; GA F67, heat stress + gibberellins F67; CK F67, heat stress + cytokinins F67; BR F67, heat stress + brassinosteroids F67; AC F2000, absolute control F2000; SC F2000, heat stress control F2000; AUX F2000, heat stress + auxins F2000; GA F2000, heat stress + gibberellins F2000; CK F2000, heat stress + cytokinins, and BR F2000, heat stress + brassinosteroids F2000.
Variables such as chlorophyll content, stomatal conductance, Fv/Fm ratio, CSI, MDA, RTI, and proline content are useful to understand the rice genotypes acclimatization and evaluate the impact of agronomic strategies under heat stress (Sarsu et al., 2018; Quintero-Calderón et al., 2021). The objective of the experiment was to evaluate the effect of the application of four growth regulators on the physiological and biochemical variables of rice seedlings subjected to combined heat stress. The seedling test is a simple and rapid method that allows the simultaneous evaluation of rice plants depending on the size or conditions of the available infrastructure (Sarsu et al., 2018). The results of this study showed that the combined heat stress caused different physiological and biochemical responses on both rice genotypes, indicating an acclimatization process. These results also showed that the foliar growth regulator sprays (principally cytokinins and brassinosteroids) helped rice acclimatization to combined heat stress since gs, RWC, and Fv/Fm ratio, photosynthetic pigments, and proline content were mainly favored.
The application of growth regulators helped the plant water status of rice plants under heat stress conditions, which can be associated with a higher gs and lower plant canopy temperature. This study showed that rice plants treated mainly with CK or BR registered higher gs values and lower PCT than SC treatment in “F2000” plants (susceptible genotype). Previous studies have also found that gs and PCT are accurate physiological indicators to determine the acclimatization response of rice plants and the effect of agronomic strategies to heat stress (Restrepo-Diaz and Garces-Varon, 2013; Sarsu et al., 2018; Quintero-Calderón et al., 2021). Foliar CK or BR enhanced gs under stress conditions since these plant hormones can help the stomatal opening by interacting with the synthesis of other signaling molecules such as ABA (stomatal closing promoter under abiotic stress) (Macková et al., 2013; Zhou et al., 2014). Stomatal opening promotes leaf cooling and helps to decrease canopy temperature (Sonjaroon et al., 2018; Quintero-Calderón et al., 2021). For these reasons, plant canopy temperature could be lower in rice plants sprayed with CK or BR under combined heat stress.
The high-temperature stresses diminish the content of photosynthetic pigments in leaves (Chen et al., 2017; Ahammed et al., 2018). In this study, the photosynthetic pigments tended to decrease in both genotypes when rice plants were under heat stress conditions and without any plant growth regulator sprays (Table 2). Feng et al. (2013) also reported a substantial decrease in the leaf chlorophyll content in two wheat genotypes exposed to heat stress. Exposure to high temperatures usually results in low chlorophyll content that can be caused by decreased chlorophyll biosynthesis, pigment degradation, or their combined effect under heat stress (Fahad et al., 2017). However, rice plants mainly treated with CK and BR increased the leaf photosynthetic pigment concentration under heat stress. Similar results were found by Jespersen and Huang (2015) and Thussagunpanit et al. (2015) who observed that leaf chlorophyll values increased after the application of Zeatin and epibrassinolide hormones in creeping bentgrass and rice plants exposed to heat stress, respectively. A plausible explanation why CK and BR helped the leaf chlorophyll content under combined heat stress is because CK can up-regulate the initiation of continuous induction of expression promoters (such as senescence-activated promoter (SAG12) or HSP18 promoter), reducing leaf chlorophyll loss, delaying leaf senescence, and enhancing plant heat resistance (Liu et al., 2020). BR can protect leaf chlorophyll and improve leaf chlorophyll content by activating or inducing the synthesis of enzymes involved in chlorophyll biosynthesis under stress (Sharma et al., 2017; Siddiqui et al., 2018). Finally, both plant hormones (CK and BR) also help the expression of heat shock proteins, improving different metabolic acclimatization processes such as the increase in chlorophyll biosynthesis (Sharma et al., 2017; Liu et al., 2020).
The parameters of chlorophyll a fluorescence are a fast and non-destructive technique that allows estimating the plant's tolerance or acclimatization to abiotic stress conditions (Chaerle et al., 2007; Kalaji et al., 2017). Parameters such as the Fv/Fm ratio have been used as plant acclimatization indicators to stressful conditions (Alvarado-Sanabria et al., 2017; Chávez-Arias et al., 2020). In this research, SC plants showed the lowest values of this variable, mainly in “F2000” rice plants. Yin et al. (2010) also found that the Fv/Fm ratio significantly decreased at temperatures higher than 35°C in rice leaves at maximum tillering. According to Feng et al. (2013), a low Fv/Fm ratio under heat stress suggests a decrease in the capture and conversion rate of excitation energy by PSII reaction centers, indicating disorganization of PSII reaction centers under heat stress conditions. This observation allows us to infer that the disorder in the photosynthetic machinery appeared to be more pronounced in sensitive cultivars (Fedearroz 2000) than in resistant ones (Fedearroz 67).
The use of CK or BR mainly enhanced PSII efficiency under combined heat stress. Similar results were obtained by Thussagunpanit et al. (2015), who observed that the application of BR enhanced the PSII efficiency under heat stress in rice plants. Kumar et al. (2020) also found that chickpea plants treated with CK (6-Benzyladenine) and exposed to heat stress increased the Fv/Fm ratio, concluding that foliar CK application helped the activity of PSII by activating the operation of the zeaxanthin pigment cycle. Also, foliar BR sprays favored the PSII photosynthetic machinery under combined stress conditions, suggesting that the application of this plant hormone resulted in lower dissipation of excitation energy in the PSII antennae and promoted the accumulation of chloroplastic small heat shock proteins (Ogweno et al., 2008; Kothari and Lachowiec, 2021).
The MDA and proline content generally increased when plants were under abiotic stress compared to plants grown under optimal conditions (Alvarado-Sanabria et al., 2017). Previous studies also identified that MDA and proline levels are biochemical makers used to understand the acclimatization process or the effect of agronomic practices in rice plants under high day or nighttime temperatures (Alvarado-Sanabria et al., 2017; Quintero-Calderón et al., 2021). Those studies also reported that MDA and proline contents were generally higher in rice plants exposed to high night or daytime temperatures, respectively. However, the application of foliar CK and BR helped to decrease MDA and increase proline levels, mainly in the tolerant genotype (Fedearroz 67). CK sprays could help the overexpression of cytokinin oxidase/dehydrogenase that increased the content of protective compounds such as betaine, proline, etc. (Liu et al., 2020). BR promote the induction of osmoprotectants such as betaines, sugars, and amino acids, including free proline, maintaining cell osmotic balance under many unfavorable environmental conditions (Kothari and Lachowiec, 2021).
The crop stress index (CSI) and relative tolerance index (RTI) are used to determine if the treatments evaluated help to mitigate different stresses (abiotic and biotic) and have a positive effect on plant physiology (Castro-Duque et al., 2020; Chávez-Arias et al., 2020). CSI values can range between zero and one, representing the non-stressed and stressed conditions, respectively (Lee et al., 2010). The values of heat-stressed plants (SC) showed a CSI between 0.8 and 0.9 (Figure 2C), suggesting that rice plants were negatively affected under combined stress. However, foliar BR (0.6) or CK (0.6) sprays mainly caused a decrease in this index under abiotic stress compared to SC rice plants. In “F2000” plants, the RTI showed a higher increase with the use of CK (97.69%), and BR (60.73%) compared to SC (33.52%), suggesting that these plant growth regulators also helped to improve rice tolerance to combined heat stress. These indexes have been suggested for the management of stressful situations in different species. Studies performed by Lee et al. (2010) showed that two cotton cultivars under moderate water stress had a CSI around 0.85, whereas well-irrigated cultivars registered CSI values between 0.4 and 0.6, concluding that this index is an indicator of the cultivar adaptability to water stress conditions. Additionally, Chávez-Arias et al. (2020) evaluated the effect of synthetic elicitors as a strategy to mitigate combined stress in cape gooseberry plants, finding that plants sprayed with these compounds displayed a higher RTI (65%) compared to plants only exposed to combined stress (biotic and abiotic stress). Based on the above, CK and BR can be considered an agronomic strategy to induce rice tolerance to combined heat stress, since these plant growth regulators caused positive biochemical and physiological responses.
In the last years, rice studies in Colombia have focused on the evaluation of genotypes with resistance to high day or nighttime temperatures using physiological or biochemical traits (Sánchez-Reinoso et al., 2014; Alvarado-Sanabria et al., 2021). However, the analysis of practical, economic, and profitable techniques has gained importance in the last years in order to propose crop integrated management to ameliorate the effects of combined heat stress periods in the country (Calderón-Páez et al., 2021; Quintero-Calderón et al., 2021). For this reason, the physiological and biochemical responses of rice plants to combined heat stress (40°C day/30°C night) observed in this study indicate that the foliar CK or BR sprays may be an appropriate crop management technique to mitigate the adverse effects of moderate heat stress periods. These treatments increased the tolerance (low CSI and high RTI) of the two rice genotypes, showing common trends in the physiological and biochemical responses of plants under combined heat stress. The principal responses of rice plants were a decrease in gs, total chlorophyll, Chl α and β, and carotenoids content. Additionally, plants registered an increase in the damage of the PSII (reducing the values of chlorophyll fluorescence parameters such as Fv/Fm ratio), and lipid peroxidation. On the other hand, these negative effects were mitigated and the proline content increased when rice plants were treated with CK and BR (Figure 4).
Figure 4. Concept model of the impact of the combined heat stress and foliar plant growth regulators sprays in rice plants. Red arrows and blue arrows indicate the negative or positive effect of interaction between combined heat stress and foliar BR (brassinosteroids) and CK (cytokinins) applications on the physiological and biochemical responses, respectively. gs: stomatal conductance; Total Chl: total chlorophyll content; Chl α: Chlorophyl α content; Chl β: Chlorophyl β content; Cx+c: Carotenoids content.
In summary, the physiological and biochemical responses of this study revealed that Fedearroz 2000 rice plants were more susceptible to the combined heat stress period than Fedearroz 67 rice plants. All growth regulators evaluated in this research (auxins, gibberellins, cytokinins, or brassinosteroids) demonstrated a certain level of mitigation of combined heat stress. However, cytokinins and brassinosteroids induced better plant acclimatization, since both plant growth regulators increased the chlorophyll content, chlorophyll α fluorescence parameters, gs, and RWC and decreased MDA content and canopy temperature, compared to rice plants without any application. The foregoing allows us to conclude that the use of plant growth regulators (cytokinins and brassinosteroids) is a useful tool for managing stress conditions due to severe heat stress in rice crops when periods of high temperatures are expected.
The original contributions presented in the study are included in the article, further inquiries can be directed to the corresponding author.
AP-B and HR-D: conceptualization, writing—review, editing, data curation, and formal analysis. AP-B, HR-D, and GG-V: methodology, investigation, writing—original draft, and validation. HR-D and GG-V: resources, supervision, project administration, and funding acquisition. All authors agreed to be accountable for the content of the work.
This research was partially supported by the Convocatoria para la Financiación Parcial de Proyectos de Tesis de Doctorado y Maestría of the Facultad de Ciencias Agrarias, Universidad Nacional de Colombia, Campus Bogotá.
The authors declare that the research was conducted in the absence of any commercial or financial relationships that could be construed as a potential conflict of interest.
All claims expressed in this article are solely those of the authors and do not necessarily represent those of their affiliated organizations, or those of the publisher, the editors and the reviewers. Any product that may be evaluated in this article, or claim that may be made by its manufacturer, is not guaranteed or endorsed by the publisher.
The Supplementary Material for this article can be found online at: https://www.frontiersin.org/articles/10.3389/fpls.2021.702892/full#supplementary-material
Abd El-Naby, S. K. M. A, Abdelkhalek, A., Baiea, M. H. M., and Amin, O. A. (2020). Mitigation of heat stress effects on washington navel orange by using melatonin, gibberellin and salicylic treatments. Plant Archiv. 20 (Suppl. 1), 3523–3534.
Ahammed, G. J., Xia, X. J., Li, X., Shi, K., Yu, J. Q., and Zhou, Y. H. (2015). Role of brassinosteroid in plant adaptation to abiotic stresses and its interplay with other hormones. Curr. Protein Peptide Sci. 16, 462–473. doi: 10.2174/1389203716666150330141427
Ahammed, G. J., Xu, W., Liu, A., and Chen, S. (2018). COMT1 silencing aggravates heat stress induced reduction in photosynthesis by decreasing chlorophyll content, photosystem II activity, and electron transport efficiency in tomato. Front. Plant Sci. 9:998. doi: 10.3389/fpls.2018.00998
Ahmed, J., and Hassan, M. (2011). Evaluation of seedling proline content of wheat genotypes in relation to heat tolerance. Bangladesh J. Bot. 40, 17–22. doi: 10.3329/bjb.v40i1.7991
Ali, Q., Ashraf, M., and Athar, H. U. R. (2007). Exogenously applied proline at different growth stages enhances growth of two maize cultivars grown under water deficit conditions. Pak. J. Bot. 39, 1133–1144.
Alonso-Ramirez, A., Rodriguez, D., Reyes, D., Jimenez, J. A., Nicolas, G., Lopez-Climent, M., et al. (2009). Evidence for a role of gibberellins in salicylic acid-modulated early plant responses to abiotic stress in arabidopsis seeds. Plant Physiol. 150, 1335–1344. doi: 10.1104/pp.109.139352
Alvarado-Sanabria, O. H., Garces-Varon, G. A., and Restrepo-Diaz, H. (2017). The effects of night-time temperatures on physiological and biochemical traits in rice. Notulae Botanicae Horti Agrobotanici Cluj-Napoca 45, 157–163. doi: 10.15835/nbha45110627
Alvarado-Sanabria, O. H., Garces-Varon, G. A., and Restrepo-Diaz, H. (2021). Effect of two nocturnal temperatures on chlorophyll fluorescence parameters of flag leaves and panicle characteristics in seven rice (Oryza sativa L.) genotypes. Pak. J. Bot. 53, 97–103. doi: 10.30848/PJB2021-1(32)
Amanullah, Fahad, S., Anwar, S., Baloch, S. K., Saud, S., Alharby, H., et al. (2017). Rice crop responses to global warming: an overview. Rice—technology and production. Plant Sci. 8:1147. doi: 10.5772/68035
Bajaj, S., and Mohanty, A. (2005). Recent advances in rice biotechnology-towards genetically superior transgenic rice. Plant Biotech. J. 3, 275–307. doi: 10.1111/j.1467-7652.2005.00130.x
Baker, N. R. (2008). Chlorophyll fluorescence: a probe of photosynthesis in vivo. Ann. Rev. Plant Bio. 59, 89–113. doi: 10.1146/annurev.arplant.59.032607.092759
Bates, L. S., Waldren, R. P., and Teare, I. D. (1973). Rapid determination of free proline for water-stress studies. Plant Soil 39, 205–207. doi: 10.1007/BF00018060
Bielach, A., Hrtyan, M., and Tognetti, V. B. (2017). Plants under stress: involvement of auxin and cytokinin. Int. J. Mol. Sci. 18:1427. doi: 10.3390/ijms18071427
Bita, C. E., and Gerats, T. (2013). Plant tolerance to high temperature in a changing environment: scientific fundamentals and production of heat stress-tolerant crops. Front. Plant Sci. 4:273 doi: 10.3389/fpls.2013.00273
Calderón-Páez, S. E., Cueto-Niño, Y. A., Sánchez-Reinoso, A. D., Garces-Varon, G., Chávez-Arias, C. C., and Restrepo-Díaz, H. (2021). Foliar boron compounds applications mitigate heat stress caused by high daytime temperatures in rice (Oryza sativa L.) Boron mitigates heat stress in rice. J. Plant Nutr. doi: 10.1080/01904167.2021.1921202
Cao, Y.-Y. H, Duan, L.-N., Yang, Z.-Q., Wang, L.-J., and Liu Yang, J.-C. (2009). Effect of high temperature during heading and early filling on grain yield and physiological characteristics in Indica rice. Acta Agronom. Sin. 35, 512–521. doi: 10.1016/S1875-2780(08)60071-1
Castro-Duque, N. E., Chávez-Arias, C. C., and Restrepo-Díaz, H. (2020). Foliar glycine betaine or hydrogen peroxide sprays ameliorate waterlogging stress in cape gooseberry. Plants 9:644. doi: 10.3390/plants9050644
Chaerle, L., Leinonen, I., Jones, H. G., and Van Der Straeten, D. (2007). Monitoring and screening plant populations with combined thermal and chlorophyll fluorescence imaging. J. Exp. Bot. 58, 773–784. doi: 10.1093/jxb/erl257
Chandio, A. A., Magsi, H., and Ozturk, I. (2020). Examining the effects of climate change on rice production: case study of Pakistan. Environ. Sci. Pollut. Res. 27, 7812–7822. doi: 10.1007/s41748-021-00210-z
Chaturvedi, A. K., Bahuguna, R. N., Shah, D., Pal, M., and Jagadish, S. V. K. (2017). High temperature stress during flowering and grain filling offsets beneficial impact of elevated CO2 on assimilate partitioning and sink-strength in rice. Sci. Rep. 7, 1–13. doi: 10.1038/s41598-017-07464-6
Chávez-Arias, C. C., Gómez-Caro, S., and Restrepo-Díaz, H. (2020). Mitigation of the impact of vascular wilt and soil hypoxia on cape gooseberry plants by foliar application of synthetic elicitors. Hortscience 55, 121–132. doi: 10.21273/HORTSCI14550-19
Chavez-Arias, C. C., Ligarreto-Moreno, G. A., and Restrepo-Díaz, H. (2018). Evaluation of heat stress period duration and the interaction of daytime temperature and cultivar on common bean. Environ. Exp. Bot. 155, 600–608. doi: 10.1016/j.envexpbot.2018.08.012
Chen, J., Nolan, T. M., Ye, H., Zhang, M., Tong, H., Xin, P., et al. (2017). Arabidopsis WRKY46, WRKY54, and WRKY70 transcription factors are involved in brassinosteroid-regulated plant growth and drought responses. Plant Cell 29, 1425–1439. doi: 10.1105/tpc.17.00364
Chernyad'ev, I. I. (2009). The protective action of cytokinins on the photosynthetic machinery and productivity of plants under stress (review). Appl. Biochem. Microbio. 45, 351–362. doi: 10.1134/S0003683809040012
Dey, A. K., Sharma, M., and Meshram, M. R. (2016). An analysis of leaf chlorophyll measurement method using chlorophyll meter and image processing technique. Procedia Comput. Sci. 85, 286–292. doi: 10.1016/j.procs.2016.05.235
El-Bassiony, A. M., Ghoname, A. A., El-Awadi, M. E., Fawzy, Z. F., and Gruda, N. (2012). Ameliorative effects of brassinosteroids on growth and productivity of snap beans grown under high temperature. Gesunde Pflanzen 64, 175–182. doi: 10.1007/s10343-012-0286-x
Fahad, S., Bajwa, A. A., Nazir, U., Anjum, S. A., Farooq, A., Zohaib, A., et al. (2017). Crop production under drought and heat stress: plant responses and management options. Front. Plant Sci. 8:1147. doi: 10.3389/fpls.2017.01147
Fahad, S., Hussain, S., Saud, S., Khan, F., Hassan, S., Amanullah, et al. (2016). Exogenously applied plant growth regulators affect heat-stressed rice pollens. J. Agron. Crop Sci. 202, 139–150. doi: 10.1111/jac.12148
Federarroz (Federación Nacional de Arroceros) (2021). Online Statistical Database: Area, Production and Yields in Colombia. Available online at: http://www.fedearroz.com.co/new/apr_public.php
Feller, U., and Vaseva, I. I. (2014). Extreme climatic events: impacts of drought and high temperature on physiological processes in agronomically important plants. Front. Environ. Sci. 2:442. doi: 10.3389/fenvs.2014.00039
Feng, B., Liu, P., Li, G., Dong, S. T., Wang, F. H., Kong, L. A., et al. (2013). Effect of heat stress on the photosynthetic characteristics in flag leaves at the grain-filling stage of different heat-resistant winter wheat varieties. J. Agron. Crop Sci. 200, 143–155. doi: 10.1111/jac.12045
Garcés, G. (2020). Adaptación del sector arrocero colombiano al cambio y la variabilidad climática. Arroz 68: 38–48.
Gerhards, M., Rock, G., Schlerf, M., and Udelhoven, T. (2016). Water stress detection in potato plants using leaf temperature, emissivity, and reflectance. Int. J. Appl. Earth Observ. Geoinform. 53, 27–39. doi: 10.1016/j.jag.2016.08.004
Ghoulam, C., Foursy, A., and Fares, K. (2002). Effects of salt stress on growth, inorganic ions and proline accumulation in relation to osmotic adjustment in five sugar beet cultivars. Environ. Exp. Bot. 47, 39–50. doi: 10.1016/S0098-8472(01)00109-5
Ha, S., Vankova, R., Yamaguchi-Shinozaki, K., Shinozaki, K., and Tran, L.-S. P. (2012). Cytokinins: metabolism and function in plant adaptation to environmental stresses. Trends Plant Sci. 17, 172–179. doi: 10.1016/j.tplants.2011.12.005
Hodges, D. M., DeLong, J. M., Forney, C. F., and Prange, R. K. (1999). Improving the thiobarbituric acid-reactive-substances assay for estimating lipid peroxidation in plant tissues containing Anthocyanin and other interfering compounds. Planta 207, 604–611. doi: 10.1007/s004250050524
Holá, D., Rothov,á, O., Kočov,á, M., Kohout, L., and Kvasnica, M. (2010). The effect of brassinosteroids on the morphology, development and yield of field-grown maize. Plant Growth Regul. 61, 29–43. doi: 10.1007/s10725-010-9446-0
Iizumi, T., Luo, J.-J., Challinor, A. J., Sakurai, G., Yokozawa, M., Sakuma, H., et al. (2014). Impacts of El Niño Southern Oscillation on the global yields of major crops. Nat. Commun. 5:3712. doi: 10.1038/ncomms4712
IPCC (2013). Climate Change 2013: The Physical Science Basis. Contribution of Working Group I to the Fifth Assessment Report of the Intergovernmental Panel on Climate Change. Cambridge: Cambridge University Press.
Jespersen, D., and Huang, B. (2015). Proteins associated with heat-induced leaf senescence in creeping bentgrass as affected by foliar application of nitrogen, cytokinins, and an ethylene inhibitor. Proteomics 15, 798–812. doi: 10.1002/pmic.201400393
Kalaji, H. M., Schansker, G., Brestic, M., Bussotti, F., Calatayud, A., Ferroni, L., et al. (2017). Frequently asked questions about chlorophyll fluorescence, the sequel. Photosynth. Res. 132, 13–66. doi: 10.1007/s11120-016-0318-y
Khan, A., Bilal, S., Khan, A. L., Imran, M., Shahzad, R., Al-Harrasi, A., et al. (2020). Silicon and gibberellins: synergistic function in harnessing aba signaling and heat stress tolerance in date palm (Phoenix dactylifera L.). Plants 9:620. doi: 10.3390/plants9050620
Kilasi, N. L., Singh, J., Vallejos, C. E., Ye, C., Jagadish, S. V. K., Kusolwa, P., et al. (2018). Heat stress tolerance in rice (Oryza sativa L.): identification of quantitative trait loci and candidate genes for seedling growth under heat stress. Front. Plant Sci. 9:1598. doi: 10.3389/fpls.2018.01578
Kothari, A., and Lachowiec, J. (2021). Roles of brassinosteroids in mitigating heat stress damage in cereal crops. Int. J. Mol. Sci. 22:2706. doi: 10.3390/ijms22052706
Kumar, P., Yadav, S., and Singh, M. P. (2020). Bioregulators application improved heat tolerance and yield in chickpea (Cicer arietinum L.) by modulating zeaxanthin cycle. Plant Physiol. Rep. 25, 677–688. doi: 10.1007/s40502-020-00555-z
Lee, W. S., Alchanatis, V., Yang, C., Hirafuji, M., Moshou, D., and Li, C. (2010). Sensing technologies for precision specialty crop production. Comput. Electron. Agric. 74, 2–33. doi: 10.1016/j.compag.2010.08.005
Lesk, C., Rowhani, P., and Ramankutty, N. (2016). Influence of extreme weather disasters on global crop production. Nature 529, 84–87. doi: 10.1038/nature16467
Li, Z., Tan, X. F., Lu, K., Liu, Z. M., and Wu, L. L. (2017). The effect of CaCl2 on calcium content, photosynthesis, and chlorophyll fluorescence of tung tree seedlings under drought conditions. Photosynthetica 55, 553–560. doi: 10.1007/s11099-016-0676-x
Liu, Q., Wu, X., Ma, J., Li, T., Zhou, X., and Guo, T. (2013). Effects of high air temperature on rice grain quality and yield under field condition. Agron. J.105, 446–454. doi: 10.2134/agronj2012.0164
Liu, Y., Zhang, M., Meng, Z., Wang, B., and Chen, M. (2020). Research progress on the roles of cytokinin in plant response to stress. Int. J. Mol. Sci. 21:6574. doi: 10.3390/ijms21186574
Lü, G. H., Wu, Y. F., Bai, W. B., Bao, M. A., Wang, C. Y., and Song, J. Q. (2013). Influence of high temperature stress on net photosynthesis, dry matter partitioning and rice grain yield at flowering and grain filling stages. J. Integr. Agric. 12, 603–609. doi: 10.1016/S2095-3119(13)60278-6
Macková, H., Hronková, M., Dobrá, J., Turečková, V., Novák, O., Lubovská, Z., et al. (2013). Enhanced drought and heat stress tolerance of tobacco plants with ectopically enhanced cytokinin oxidase/dehydrogenase gene expression. J. Exp. Bot. 64, 2805–2815. doi: 10.1093/jxb/ert131
Mittler, R., Finka, A., and Goloubinoff, P. (2012). How do plants feel the heat? Trends Biochem. Sci. 37, 118–125. doi: 10.1016/j.tibs.2011.11.007
Ogweno, J. O., Song, X. S., Shi, K., Hu, W. H., Mao, W. H., Zhou, Y. H., et al. (2008). Brassinosteroids alleviate heat-induced inhibition of photosynthesis by increasing carboxylation efficiency and enhancing antioxidant systems in lycopersicon esculentum. J. Plant Grow. Regul. 27, 49–57. doi: 10.1007/s00344-007-9030-7
Peleg, Z., and Blumwald, E. (2011). Hormone balance and abiotic stress tolerance in crop plants. Curr. Opin. Plant Bio. 14, 290–295. doi: 10.1016/j.pbi.2011.02.001
Porch Clay, T. G., and Hall, A. E. (2013) Heat tolerance. Genom. Breed. Clim. Resilient Crops 2, 167–202. doi: 10.1007/978-3-642-37048-9_4
Quintero-Calderón, E. H., Sánchez-Reinoso, A. D., Chávez-Arias, C. C., Garces-Varon, G., and Restrepo-Díaz, H. (2021). Rice seedlings showed a higher heat tolerance through the foliar application of biostimulants. Notulae Botanicae Horti Agrobotanici Cluj-Napoca 49:12120. doi: 10.15835/nbha49112120
Ramirez-Villegas, J., Salazar, M., Jarvis, A., and Navarro-Racines, C. E. (2012). A way forward on adaptation to climate change in Colombian agriculture: perspectives towards 2050. Clim. Change 115, 611–628. doi: 10.1007/s10584-012-0500-y
Restrepo-Diaz, H., and Garces-Varon, G. (2013). Response of rice plants to heat stress during initiation of panicle primordia or grain-filling phases. J. Stress Physiol. Biochem. 9, 319–325.
Salehifar, M., Rabiei, B., Afshar Mohammadian, M., and Asghari, J. (2017). Physiological and fluorescence reaction of four rice genotypes to exogenous application of iaa and kinetin under drought stress. Notulae Scientia Biologicae 9:378. doi: 10.15835/nsb9310091
Sánchez-Reinoso, A. D., Garcés-Varón, G., and Restrepo-Díaz, H. (2014). Biochemical and physiological characterization of three rice cultivars under different daytime temperature conditions. Chilean J. Agric. Res. 74, 373–379. doi: 10.4067/S0718-58392014000400001
Sánchez-Reinoso, A. D., Nieto, M. F., Dossmann, J., Camacho-Tamayo, J. H., and Restrepo-Díaz, H. (2019). Nutrient uptake, partitioning, and removal in two modern high-yielding Colombian rice genotypes. J. Plant Nutri. 42, 1–15. doi: 10.1080/01904167.2019.1659334
Sarsu, F., Ghanim, A., Das, P., Bahuguna, R. N., Kusolwa, P. M., Ashraf, M., et al. (2018). Pre-Field Screening Protocols for Heat-Tolerant Mutants in Rice. Cham: Springer Nature.
Sergiev, I., Todorova, D., Shopova, E., Jankauskiene, J., Jankovska-Bortkevič, E., and Jurkonien, e S. (2018). Effects of auxin analogues and heat stress on garden pea. Zemdirbyste-Agricult 105, 243–248. doi: 10.13080/z-a.2018.105.031
Shah, F., Huang, J., Cui, K., Nie, L., Shah, T., Chen, C., et al. (2011). Impact of high-temperature stress on rice plant and its traits related to tolerance. J. Agric. Sci. 149, 545–556. doi: 10.1017/S0021859611000360
Sharma, I., Kaur, N., and Pati, P. K. (2017). Brassinosteroids: a promising option in deciphering remedial strategies for abiotic stress tolerance in rice. Front. Plant Sci. 8:2151. doi: 10.3389/fpls.2017.02151
Siddiqui, H., Hayat, S., and Bajguz, A. (2018). Regulation of photosynthesis by brassinosteroids in plants. Acta Physiologiae Plantarum 40. doi: 10.1007/s11738-018-2639-2
Sims, D. A., and Gamon, J. A. (2002). Relationships between leaf pigment content and spectral reflectance across a wide range of species, leaf structures and developmental stages. Rem. Sens. Environ. 81, 337–354. doi: 10.1016/S0034-4257(02)00010-X
Sonjaroon, W., Jutamanee, K., Khamsuk, O., Thussagunpanit, J., Kaveeta, L., and Suksamrarn, A. (2018). Impact of brassinosteroid mimic on photosynthesis, carbohydrate content and rice seed set at reproductive stage under heat stress. Agric. Nat. Res. 52, 234–240. doi: 10.1016/j.anres.2018.09.001
Sun, J., Qi, L., Li, Y., Chu, J., and Li, C. (2012). PIF4–mediated activation of YUCCA8, expression integrates temperature into the auxin pathway in regulating Arabidopsis hypocotyl growth. PLoS Genet. 8:e1002594. doi: 10.1371/journal.pgen.1002594
Thussagunpanit, J., Jutamanee, K., Sonjaroon, W., Kaveeta, L., Chai-Arree, W., Pankean, P., et al. (2015). Effects of brassinosteroid and brassinosteroid mimic on photosynthetic efficiency and rice yield under heat stress. Photosynthetica 53, 312–320. doi: 10.1007/s11099-015-0106-5
Wahid, A., Gelani, S., Ashraf, M., and Foolad, M. R. (2007). Heat tolerance in plants: an overview. Environ. Exp. Bot. 61, 199–223. doi: 10.1016/j.envexpbot.2007.05.011
Wang, R., Zhang, Y., Kieffer, M., Yu, H., Kepinski, S., and Estelle, M. (2016). HSP90 regulates temperature-dependent seedling growth in Arabidopsis by stabilizing the auxin co-receptor F-box protein TIR1. Nat. Commun. 7:10269. doi: 10.1038/ncomms10269
Wassmann, R., Jagadish, S. V. K., Heuer, S., Ismail, A., Redona, E., Serraj, R., et al. (2009). Chapter 2 climate change affecting rice production. Adv. Agron. 52, 59–122. doi: 10.1016/S0065-2113(08)00802-X
Wellburn, A. R. (1994). The spectral determination of chlorophylls a and b, as well as total carotenoids, using various solvents with spectrophotometers of different resolution. J. Plant Physiol. 144, 307–313. doi: 10.1016/S0176-1617(11)81192-2
Wen, F., Zhang, Z., Bai, T., Xu, Q., and Pan, Y. (2010). Proteomics reveals the effects of gibberellic acid (GA3) on salt-stressed rice (Oryza sativa L.) shoots. Plant Sci. 178, 170–175. doi: 10.1016/j.plantsci.2009.11.006
Xu, Y., and Huang, B. (2009). Effects of foliar-applied ethylene inhibitor and synthetic cytokinin on creeping bentgrass to enhance heat tolerance. Crop Sci. 49:1876. doi: 10.2135/cropsci2008.07.0441
Yang, D., Li, Y., Shi, Y., Cui, Z., Luo, Y., Zheng, M., et al. (2016). Exogenous cytokinins increase grain yield of winter wheat cultivars by improving stay-green characteristics under heat stress. PLoS ONE 11:e0155437. doi: 10.1371/journal.pone.0155437
Yin, B., Zhang, Y., and Zhang, Y. (2011). Effects of plant growth regulators on growth and yields characteristics in adzuki beans (Phaseolus angularis). Front. Agric. China 5, 519–523. doi: 10.1007/s11703-011-1150-y
Yin, Y., Li, S., Liao, W., Lu, Q., Wen, X., and Lu, C. (2010). Photosystem II photochemistry, photoinhibition, and the xanthophyll cycle in heat-stressed rice leaves. J. Plant Physiol. 167, 959–966. doi: 10.1016/j.jplph.2009.12.021
Yu, J. Q., Huang, L. F., Hu, W. H., Zhou, Y. H., Mao, W. H., Ye, S. F., et al. (2004). A role for brassinosteroids in the regulation of photosynthesis in Cucumis sativus. J. Exp. Bot. 55, 1135–1143. doi: 10.1093/jxb/erh124
Zahir, Z. A., Asghar, H. N., and Arshad, M. (2001). Cytokinin and its precursors for improving growth and yield of rice. Soil Bio. Biochem. 33, 405–408. doi: 10.1016/S0038-0717(00)00145-0
Keywords: stomatal conductance, Fv/Fm ratio, high daytime and nighttime temperature, lipid peroxidation, plant acclimatization
Citation: Pantoja-Benavides AD, Garces-Varon G and Restrepo-Díaz H (2021) Foliar Growth Regulator Sprays Induced Tolerance to Combined Heat Stress by Enhancing Physiological and Biochemical Responses in Rice. Front. Plant Sci. 12:702892. doi: 10.3389/fpls.2021.702892
Received: 30 April 2021; Accepted: 23 June 2021;
Published: 23 July 2021.
Edited by:
Fabricio Eulalio Leite Carvalho, Corporacion Colombiana de Investigacion Agropecuaria (Agrosavia)—CI La Suiza, ColombiaReviewed by:
Sung Kyeom Kim, Kyungpook National University, South KoreaCopyright © 2021 Pantoja-Benavides, Garces-Varon and Restrepo-Díaz. This is an open-access article distributed under the terms of the Creative Commons Attribution License (CC BY). The use, distribution or reproduction in other forums is permitted, provided the original author(s) and the copyright owner(s) are credited and that the original publication in this journal is cited, in accordance with accepted academic practice. No use, distribution or reproduction is permitted which does not comply with these terms.
*Correspondence: Hermann Restrepo-Díaz, aHJlc3RyZXBvZEB1bmFsLmVkdS5jbw==
Disclaimer: All claims expressed in this article are solely those of the authors and do not necessarily represent those of their affiliated organizations, or those of the publisher, the editors and the reviewers. Any product that may be evaluated in this article or claim that may be made by its manufacturer is not guaranteed or endorsed by the publisher.
Research integrity at Frontiers
Learn more about the work of our research integrity team to safeguard the quality of each article we publish.