- 1Irrigated Agriculture Research and Extension Center, Washington State University, Prosser, WA, United States
- 2Department of Plant Sciences, North Dakota State University, Fargo, ND, United States
- 3Grain Legume Genetics and Physiology Research Unit, United States Department of Agriculture - Agricultural Research Service (USDA-ARS), Prosser, WA, United States
Bean common mosaic necrosis virus (BCMNV) is a major disease in common bean (Phaseolus vulgaris L.). Host plant resistance is the primary disease control. We sought to identify candidate genes to better understand the host-pathogen interaction and develop tools for marker-assisted selection (MAS). A genome-wide association study (GWAS) approach using 182 lines from a race Durango Diversity Panel (DDP) challenged by BCMNV isolates NL-8 [Pathogroup (PG)-III] and NL-3 (PG-VI), and genotyped with 1.26 million single-nucleotide polymorphisms (SNPs), revealed significant peak regions on chromosomes Pv03 and Pv05, which correspond to bc-1 and bc-u resistance gene loci, respectively. Three candidate genes were identified for NL-3 and NL-8 resistance. Side-by-side receptor-like protein kinases (RLKs), Phvul.003G038700 and Phvul.003G038800 were candidate genes for bc-1. These RLKs were orthologous to linked RLKs associated with virus resistance in soybean (Glycine max). A basic Leucine Zipper (bZIP) transcription factor protein is the candidate gene for bc-u. bZIP protein gene Phvul.005G124100 carries a unique non-synonymous mutation at codon 14 in the first exon (Pv05: 36,114,516 bases), resulting in a premature termination codon that causes a nonfunctional protein. SNP markers for bc-1 and bc-u and new markers for I and bc-3 genes were used to genotype the resistance genes underpinning BCMNV phenotypes in the DDP, host group (HG) differentials, and segregating F3 families. Results revealed major adjustments to the current host-pathogen interaction model: (i) there is only one resistance allele bc-1 for the Bc-1 locus, and differential expression of the allele is based on presence vs. absence of bc-u; (ii) bc-1 exhibits dominance and incomplete dominance; (iii) bc-1 alone confers resistance to NL-8; (iv) bc-u was absent from HGs 2, 4, 5, and 7 necessitating a new gene symbol bc-ud to reflect this change; (v) bc-ud alone delays susceptible symptoms, and when combined with bc-1 enhanced resistance to NL-3; and (vi) bc-ud is on Pv05, not Pv03 as previously thought. These candidate genes, markers, and adjustments to the host-pathogen interaction will facilitate breeding for resistance to BCMNV and related Bean common mosaic virus (BCMV) in common bean.
Introduction
Bean common mosaic necrosis virus (BCMNV) and Bean common mosaic virus (BCMV) are related positive-stranded RNA viruses in the Potyvirus genus that infect common bean (Phaseolus vulgaris L.) worldwide. BCMNV and BCMV are transmitted by infected seeds and from plant to plant by several aphid species in a non-persistent manner. Seed-borne transmission plagues subsistence farmers and other growers who rely on planting their own “bin-run” seed. These viruses can cause greater than 80% yield loss in common bean production fields (Morales, 2003). The primary disease control is host plant resistance. Resistance to BCMNV and BCMV in the common bean is regulated by the dominant I gene and six recessive alleles (bc-1, bc-12, bc-2, bc-22, bc-3, and bc-u) distributed across four loci (Drijfhout, 1978). Strain diversity is classified into eight pathogroups (PG) based on interactions with 12 host group (HG) differential cultivars possessing different resistance gene combinations, PG I to VII referenced in Drijfhout et al. (1978) and PG VIII identified by Feng et al. (2015).
A few of the host resistance genes are well studied. The eIF4E [Eukaryotic translation initiation factor 4E; Phvul.006G168400 in Phytozome v13 info: P. vulgaris_G19833 v2.1 (Schmutz et al., 2014)] protein is a candidate gene for bc-3 on chromosome Pv06 (Naderpour et al., 2010). eIF4E has a reported role in potyviral infection in other crops such as peas (Pisum sativum; Swisher Grimm and Porter, 2020), melon (Cucumis melo; Nieto et al., 2006), tomato (Solanum lycopersicum; Parrella et al., 2002), and pepper (Capsicum annuum; Ruffel et al., 2004). A missense single-nucleotide polymorphism (SNP) within eIF4E is diagnostic for bc-3, and a KASP marker designed for the SNP is used for marker-assisted selection (MAS) of bc-3 in common bean (Hart and Griffiths, 2013). The I gene induces a temperature-independent hypersensitive response (HR) against BCMNV and either an immune or temperature-dependent HR response to BCMV strains (Drijfhout et al., 1978). The HR for I gene, without protection by any recessive genes (bc-12, bc-22, and bc-3), often results in the death of the plant. The I gene on Pv02 is linked with a cluster of seven NBS-LRR genes (Vallejos et al., 2006). An SNP marker just downstream from the NBS-LRR cluster is currently used for MAS of the I gene (Bello et al., 2014). Candidate genes for bc-1, bc-2, and bc-u have not been identified. However, bc-12 is located on Pv03 (Miklas et al., 2000) and linked with a SCAR marker (SBD5) that is used for MAS of the gene (Vandemark and Miklas, 2005). Drijfhout (1978) characterized bc-u as a non-specific helper gene needed by the other five recessive bc genes to express resistance. bc-u was moderately linked (23 cM) with bc-12 on Pv03 in a recombinant inbred population (Strausbaugh et al., 1999).
Candidate gene analysis of targeted traits is facilitated by new molecular tools for common bean, including millions of SNPs identified by resequencing genotypes and diversity panels (Cichy et al., 2015; Moghaddam et al., 2016; Lobaton et al., 2018; Wallace et al., 2018; Oladzad et al., 2019; Diaz et al., 2020; Wu et al., 2020), 6 and 12 K SNP BeadChip assays (Song et al., 2015; Miklas et al., 2020), and assembled bean genomes representing different gene pools, such as the Andean G19833 landrace (Chaucha Chuga; Schmutz et al., 2014) and “UI-111” cultivar for race Durango (DDP). These genomic tools contributed to candidate gene discovery for several traits in common bean, including the NAC candidate gene for bgm-1 resistance to Bean golden yellow mosaic virus (BGYMV; Soler-Garzón et al., 2021), a bHLH transcription factor for the seed pigment P gene (McClean et al., 2018), and truncated CRINKLY4 kinase acting as a decoy to condition Co-x anthracnose resistance (Richard et al., 2021).
We sought to take advantage of a genome-wide association study (GWAS) approach to unravel the genetics of resistance to BCMNV in the Durango Diversity Panel (DDP). The objectives were to identify genomic regions associated with bc-1 and bc-u resistance genes leading to candidate gene discovery and identification of polymorphisms to exploit for MAS.
Materials and Methods
Plant Materials
The DDP consists of 182 dry bean lines (cultivars, breeding lines, germplasm releases, and landraces) primarily from race Durango of the Middle American gene pool. Díaz and Blair (2006) grouped race Durango and race Jalisco genotypes because they were closely related as determined by microsatellite markers. Undoubtedly the DDP contains some Jalisco genotypes as well; however, we did not differentiate them. The panel was developed to provide a historical representation of pinto, great northern, pink, and small red market class genotypes released by North American breeding programs from the late 1930s to about 2010 (Supplementary Table S1).
Nine F2 populations were generated from crosses among DDP lines selected as parents according to their reactions to BCMNV inoculations and genotypes based on SNP markers linked to the I, bc-1, and bc-u genes (described below). Subsequently, the F2 populations were genotyped using the same SNP markers. About 241 F2 plants among the nine populations were genotyped. We selected and advanced 34 F2 plants (Supplementary Table S2) to further validate the segregation of the genes and linked markers. About 16 true-breeding F2:3 families were used as controls for each gene in a homozygous state, and the remaining 22 families had at least one gene in a heterozygous state. A total of 1,284 F2:3 plants were evaluated for BCMNV reaction and genotyped for the linked SNP markers (Supplementary Table S3).
Evaluation of BCMNV Reaction
Screening of 182 DDP lines and 34 F3 families for BCMNV reaction using strains NL-8 D (henceforth NL-8) from PG 3 (PG-III) and NL-3 D (henceforth NL-3) from PG 6 (PG-VI) was conducted in the USDA-ARS greenhouses at Prosser (Washington, United States) under controlled conditions (22–28°C temperature range and 14-h photoperiod using artificial lights as necessary). The “D” suffix is indicative that NL-8 and NL-3 trace back to Drijfhout (1978) original isolates. Completed sequences for both isolates have been reported in previous phylogenetic studies (Larsen et al., 2005, 2011). Two sets of each DDP line and ~18 seeds per F2:3 family were grown in 9 cm3 pots containing three seeds each and a commercial potting mix (Sun Gro Horticulture, Bellevue, WA, United States). For each set, the primary leaves, when 80 to 100% fully expanded at approximately 10 days after planting (DAP), were mechanically inoculated (Drijfhout, 1978) with one of the BCMNV strains. Phenotypic data based on visual characterization and differential cultivars reactions were recorded at weekly intervals from 1 to 5 wpi (weeks post-inoculation).
The plant reactions to virus inoculations were categorized as, NS: no apparent symptoms on inoculated leaves and no systemic symptoms; VN: restricted vein necrosis on inoculated leaves, no systemic symptoms; VN+: restricted vein necrosis on inoculated leaves, with some small patches (10 mm2) of systemic restricted vein necrosis on upper trifoliolate leaves observed from 3 to 5 wpi; NLL: local necrotic lesions on inoculated leaves, no systemic symptoms; TN: lethal systemic top necrosis by 7–10 dpi (days post-inoculation), resulting in plant death; dTN: delayed TN beginning >11 dpi, most often resulting in plant death; M: leaf curling and plant stunting with severe systemic chlorotic mosaic symptoms; mM: mild systemic chlorotic mosaic symptoms; and dM: delayed severe systemic chlorotic mosaic symptoms observed 2–4 wpi. dM initiating at 2 wpi occurred in plants with NS, and at 4 wpi in plants with mM symptoms. The differential cultivars with reactions to BCMNV strains in parentheses (NL-8/NL-3): HG-1, “Sutter Pink” (M/M); HG-3, “Olathe” (NS/mM); HG-6, “Othello” (NS/NS); HG-8, “Widusa” (TN/TN); HG-9, “Top Crop” (VN/TN); HG-10, “Beryl” (VN/VN); and HG-11, “92US1006” (NLL/NLL) were used as controls.
DNA Extraction and SNP Calling
DNA was extracted from each DDP member following the protocol described previously (Tobar-Piñón et al., 2021). Each DNA sample was sequenced at Hudson/Alpha Institute of Biotechnology (Huntsville, AL, United States) using Illumina technology to an average depth of 8X. WGS raw data have been deposited into the NCBI SRA database with accession BioProject PRJNA386820 (Supplementary Table S1).1
Reads of DDP were indexed, aligned, and sorted to the reference Andean G19833 P. vulgaris v2.1 genome sequence using BWA-MEM (Li, 2013) and Samtools (Li et al., 2009).2 A total of 11,518,066 variants [single nucleotide variants (SNVs), insertions or deletions (InDels), and short tandem repeats (STRs)], with at least 20 samples genotyped, were mapped to the 11 chromosomes and scaffolds of the G19833 v2.1 reference genome by next generation sequencing experience platform (NGSEP) pipeline (Perea et al., 2016; Lobaton et al., 2018). The maximum base quality score was set to 30, and the minimum base quality for reporting a variant was set to 40. All SNP markers detected with less than 50% missing values and a minor allele frequency (MAF) 0.05 were retained to perform imputation with the ImputeVCF module in NGSEP, which is a reimplementation of the Hidden Markov Model (HMM) applied in the package fastPHASE (Scheet and Stephens, 2006). Annotation of variants was performed using the command Annotate by NGSEP.
Genomic DNA in DDP and additional lines for SNP marker testing was isolated from 20 mg of leaf tissue collected from an individual plant for each line grown in the USDA-ARS greenhouses at Prosser, WA, United States, using a Qiagen DNeasy 96 Plant Kit (Hilden, Germany). For individual F3 plants, total DNA was extracted from four-leaf disks (approximately 30 mm2) according to the alkaline extraction method described by Xin et al. (2003) with modifications. Briefly, the method includes Buffer A (50 mM NaOH, 1% Tween® 20) to degrade cell walls and Buffer B (100 mM Tris-HCl, 1.7 mM EDTA pH 8) to neutralize DNA and bind proteins. About 200 μl of Buffer A were added, mixed by centrifuging at 1,500 rpm for 1 min, and incubated at 95°C for 10 min. About 120 μl of Buffer B was added, mixed by centrifuging at 1,500 rpm at room temperature for 5 min. A 1:7 dilution of the extracted DNA was placed in a 96-well plate with a final volume of 100 μl. At last, a 5 μl of DNA template dilution was used for PCR.
Genome-Wide Association Study
Phenotypic and genotypic information was integrated for conducting GWAS based on a multi-locus random-SNP-effect mixed linear model (mrMLM) described by Wang et al. (2016) and implemented in the “mrMLM” R package (Wen et al., 2018). A kinship matrix was generated using the efficient mixed-model association (EMMA) algorithm implemented in the GAPIT R package (Lipka et al., 2012) with corrections for kinship and population structure. Five PCs generated from GAPIT were included as covariates. The Bonferroni test was implemented to control the experiment-wise type I error rate at 0.05. GWAS results were plotted using CMPlot v3.62 (Yin, 2016), and IntAssoPlot v3 (He et al., 2020) was used to represent regional and single gene-based marker-trait associations graphically.
Candidate Gene Identification and Development of SNP Markers
Candidate genes located within the associated genomic regions were identified by aligning with the G19833 reference transcriptome v2.1, perusing available scientific literature, and synteny comparison with soybean (Glycine max).3 Exon sequences of each bc-1 and bc-u candidate gene were amplified across select genotypes (Supplementary Table S4) using PCR primers designed with Primer3 software (Koressaar and Remm, 2007; Untergasser et al., 2012). Two standard PCRs, for each sample, were replicated in a volume of 25 μl contained 1.8 mM MgCl2, 0.4 mM of the dNTPs mix (Promega™, Madison, WI), 0.25 μM of each primer (forward and reverse), 25 ng of genomic DNA, and 1 unit Taq DNA polymerase (Promega), in 1 × PCR buffer (Promega), under the following amplification conditions: heating for 2 min at 95°C, followed by 38 cycles at 94°C for 20 s, annealing specific temperature for each primer set for 30 s and 72°C for 90 s, and a final extension at 72°C for 5 min. All the PCR amplifications were performed in a PCR Eppendorf Mastercycler (Eppendorf AG, Hamburg, Germany). PCR fragments were visualized by gel electrophoresis on 2% (w+v) agarose. The PCR fragments were purified and Sanger sequenced by Eurofins MWG Operon (Louisville, KY, United States). Sequence trimming, alignment, and polymorphism discovery were performed with Geneious 9.1.2 software (Kearse et al., 2012).
A set of allele-specific primers were designed according to Wang et al. (2005) for polymorphic SNPs identified in coding regions of the sequenced bc-1 and bc-u candidate genes using the Primer3 software (Koressaar and Remm, 2007; Untergasser et al., 2012). Similar primers were designed for one missense SNP detected by GWAS, linked to the I gene. These markers were named S03_4203361 (bc-1 gene), Pvbzip1_A_C (bc-u gene), and S02_48908259 (I gene). Fragments were amplified by PCR on an Eppendorf Mastercycler using a PCR volume of 20 μl containing 1.5 mM MgCl2, 0.2 mM of the dNTPs mix (Promega), 0.15 μM of each primer (two allele-specific forward primers and the common reverse primer), 1X EvaGreen™ (Biotium, Fremont, CA), 20 ng of genomic DNA, 1X Taq buffer, and 0.1 μl Taq1 polymerase (Promega) under the following thermal profile: an initial denaturation step at 94°C for 2 min, then 38 cycles of denaturation at 92°C for 20 s, annealing for 20 s (the temperature was specific to each primer trio), and extension at 72°C for 20 s, and a final extension at 72°C for 5 min. Melting point analysis for allele determination of the template DNA was performed with a fluorescence-detecting thermocycler (LightCycler™ 4890 Instrument II, Roche, Basal Switzerland) with EvaGreen™ fluorescent dye (Biotium). Fluorescent detection was performed for 1 min at 95°C and the melting curve step ramping was performed from 65 to 95°C in increments of 1°C every 20 s. Screening for the bc-3 gene in DDP lines was also performed using the primer assay designed by Hart and Griffiths (2013), which we modified for the temperature melting (Tm)-shift assay, SNP genotyping method (Wang et al., 2005). The same PCR amplification protocol described above was used, except for modifying the annealing temperature to 69°C (Table 1).
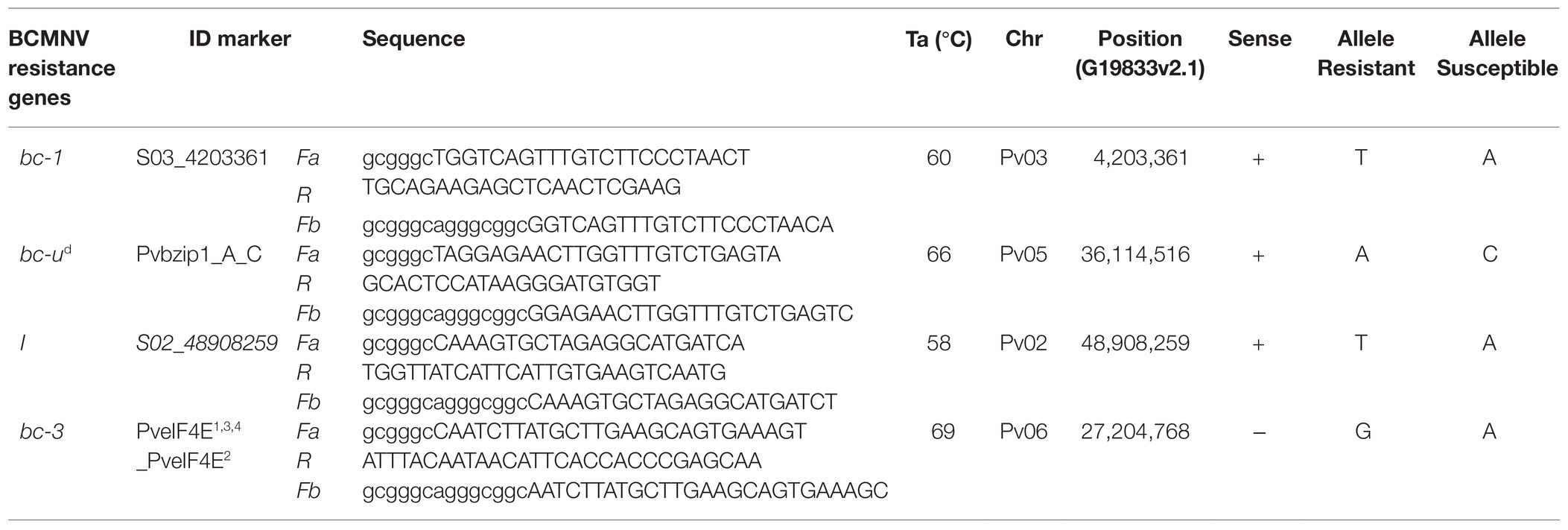
Table 1. Primers were used to generate markers for bc-1, bc-ud, I, and bc-3 genes conditioning resistance to Bean common mosaic necrosis virus (BCMNV) and Bean common mosaic virus (BCMV).
Results
Bean Common Mosaic Necrosis Virus Screening
A total of 182 DDP lines were evaluated for reaction to BCMNV strains NL-3 (PG-VI) and NL-8 (PG-III) under greenhouse conditions. The DDP lines could be separated into two major groups based on M, dM, and mM vs. TN, VN, VN+, and NLL symptoms indicating absence (96 lines) and presence of the dominant I gene (61 lines), respectively (Table 2; Supplementary Table S5). The I gene marker assayed across the DDP lines verified these groupings, except for AC Polaris with mM symptoms, which lacked the I gene but possessed the marker. Based on symptoms, 25 DDP lines did not fit the above groups due to segregating reactions (seven lines), NS due to the presence of the bc-3 gene (six lines) as verified by the PveIF4E1,3,4_PveIF4E2 marker, or NS due to the presence of the bc-22 allele based on the published information. Comparing the reactions to both BCMNV strains resulted in nine categories (Table 2) with mM to NL-3 and NS to NL-8 (26.4% of the lines) and TN to both strains (17.0%) representing the two largest categories, and NLL to both strains (1.1%) was the category with the fewest number of lines.
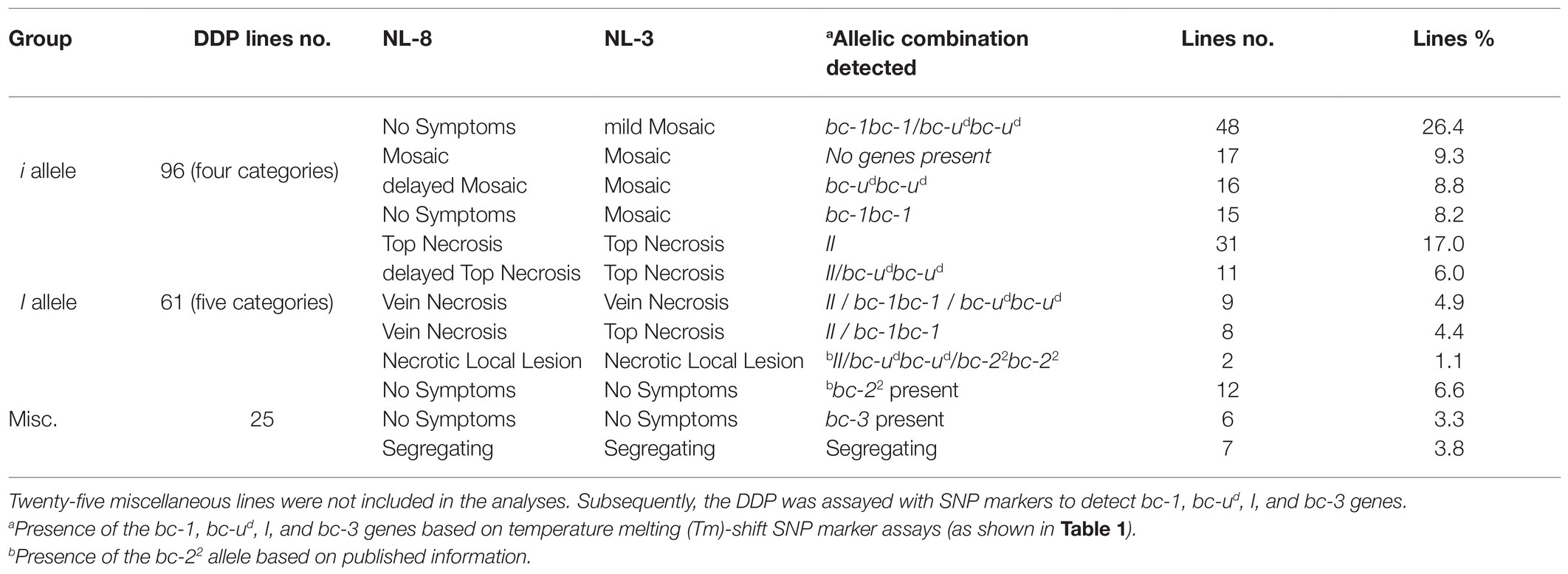
Table 2. Phenotypic reactions to BCMNV isolates NL-8 and NL-3observed in Durango Diversity Panel (DDP; 182 lines), classified into two major groups: recessive I gene vs. dominant I gene, with four and five phenotype sub-categories, respectively.
Genome-Wide Association Analysis
Genome-wide association studies were performed to identify genetic regions associated with BCMNV resistance using the filtered 1,269,044 biallelic SNPs and the DDP lines homozygous recessive i for the I gene (Table 2). The first GWAS was conducted with 48 DDP lines with partial mM resistance and 48 lines with susceptible M reactions to NL-3 strain. A second GWAS was performed with 63 DDP lines with resistant NS and 33 lines with susceptible M or dM reactions to NL-8. The Manhattan plots revealed two different loci associated with partial resistance to NL-3 and one locus for resistance to NL-8 strain (Figures 1A,B). These loci, exceeding the Bonferroni-corrected α = 0.05 threshold (p = 3.9E-08), were mapped to Pv03: 4.1 Mb and Pv05: 36.1 Mb.
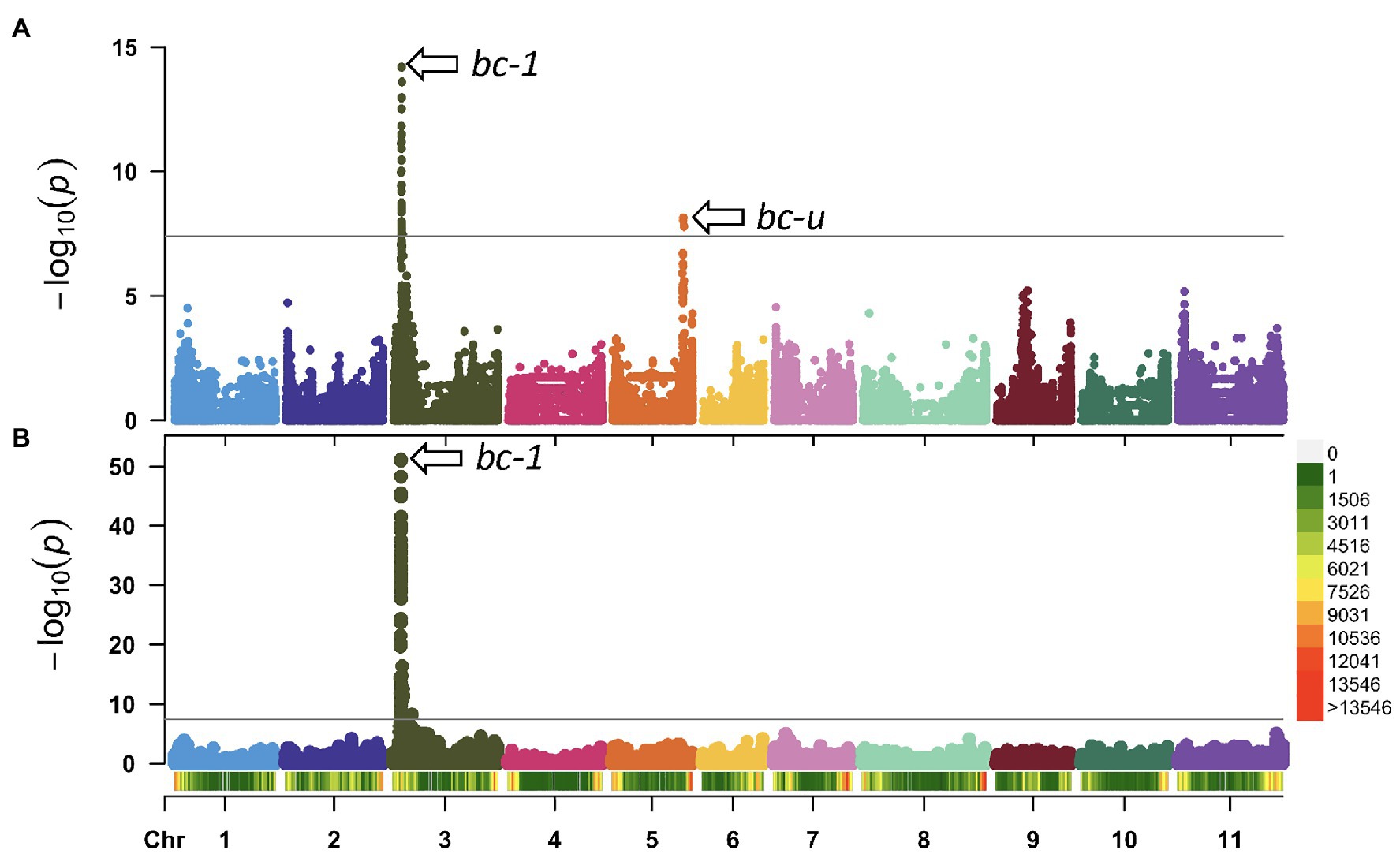
Figure 1. Manhattan plots of common mosaic necrosis virus (BCMNV) resistance in DDP. The gray horizontal line represents the genome-wide significance threshold of p = 3.98 × 10−8. (A) genome-wide association study (GWAS) using lines exhibiting mild mosaic (mM) and lines with mosaic (M) symptoms inoculated with NL-3 strain, two strong associations on Pv03 (bc-1) and Pv05 (bc-ud). (B) GWAS using lines with no symptoms (NS) compared with lines showing mosaic (M) symptoms against NL-8 strain, peak detected on Pv03 (bc-1). Bottom: Density bar (color-coded) showing genome distribution of 1,269,044 biallelic SNPs within 1 Mb window size in the DDP.
The locus on chromosome Pv03, detected by both GWAS populations, is associated with the recessive bc-12 allele (Drijfhout, 1978; Strausbaugh et al., 1999) linked to the SDB5 SCAR marker (Miklas et al., 2000) located on Pv03 at 4,204,238 bp in the Andean G19833 v2.1 reference genome. The second locus associated with resistance to NL-3 on chromosome Pv05 is putatively the bc-u recessive gene. This Pv05 location is inconsistent with the Pv03 location for bc-u observed by Strausbaugh et al. (1999). For this putative interaction model, the bc-12 and bc-u gene combination conditions partial mM resistance (Kelly, 1997) to NL-3, and bc-12 alone conditions NS resistance to NL-8. The bc-12 and bc-u combination for HG-3 fits the model of Drijfhout (1978) with bc-u acting as complementary strain-unspecific helper gene necessary for the expression of bc-12 resistance to BCMNV or BCMV strains. However, bc-12 mediated resistance to NL-8 strain in the absence of bc-u does not fit the model of Drijfhout. His model for HG-2 has bc-1 and bc-u contributing NS resistance to NL-8. For our model going forward, bc-1 and bc-12 are the same alleles, and the differential reaction observed with NL-3 and NL-8 strains is due to the presence vs. absence of bc-u.
Based on the whole-genome sequences for the DDP, there were 29 gene models spanning the peak region (3,861,624 bp–4,364,674 bp, determined by the significance threshold in Figure 1) on Pv03 where bc-1 maps. About 19 models possessed 225 SNVs, 16 InDels, and seven STRs in coding regions. Two genes in high linkage disequilibrium (LD), Phvul.003G038700 and Phvul.003G038800, were identified from a literature survey and synteny block analysis as candidate genes for bc-1 (Figure 2). Synteny between common bean and soybean [G. max (L.) Merrill] revealed that these two bc-1 candidate genes were orthologous to soybean Glyma.02g121900 and Glyma.02g122000 gene models (Table 3). These soybean gene models were identified in separate studies to be candidate genes for resistance to the Tobacco ringspot virus (TRSV; Chang et al., 2016) and Soybean mosaic virus (SMV; Maroof et al., 2010; Ilut et al., 2015). These candidate genes encode protein receptor-like protein kinases (RLK; Chang et al., 2016) with a potential role in delaying virus replication and affecting the systemic spread of BCMV (Maroof et al., 2010; Feng et al., 2017).
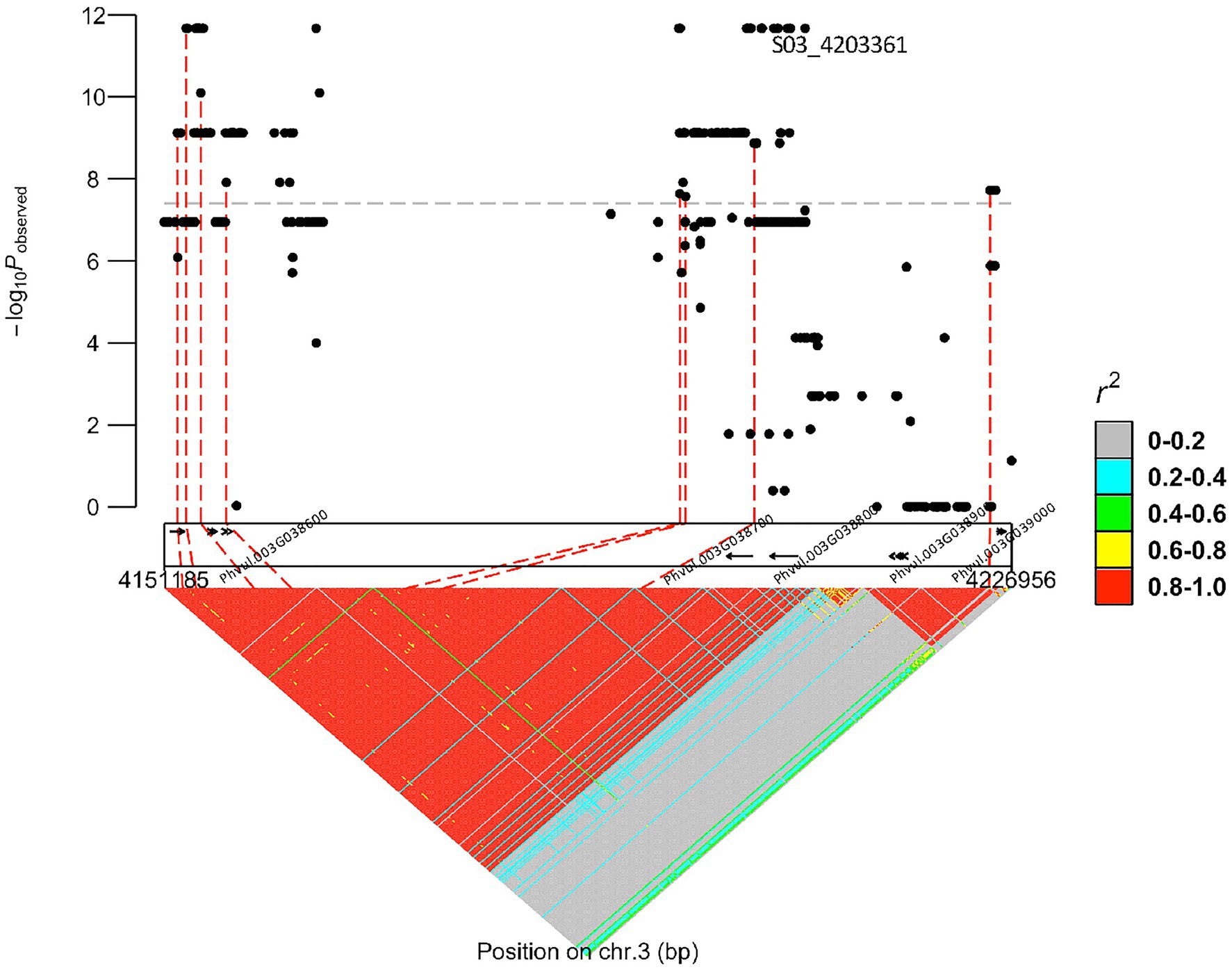
Figure 2. bc-1 regional marker-trait associations plot depicting the receptor-like protein kinases (RLK) candidate genes (arrows) for bc-1 inside the dotted box rectangle.
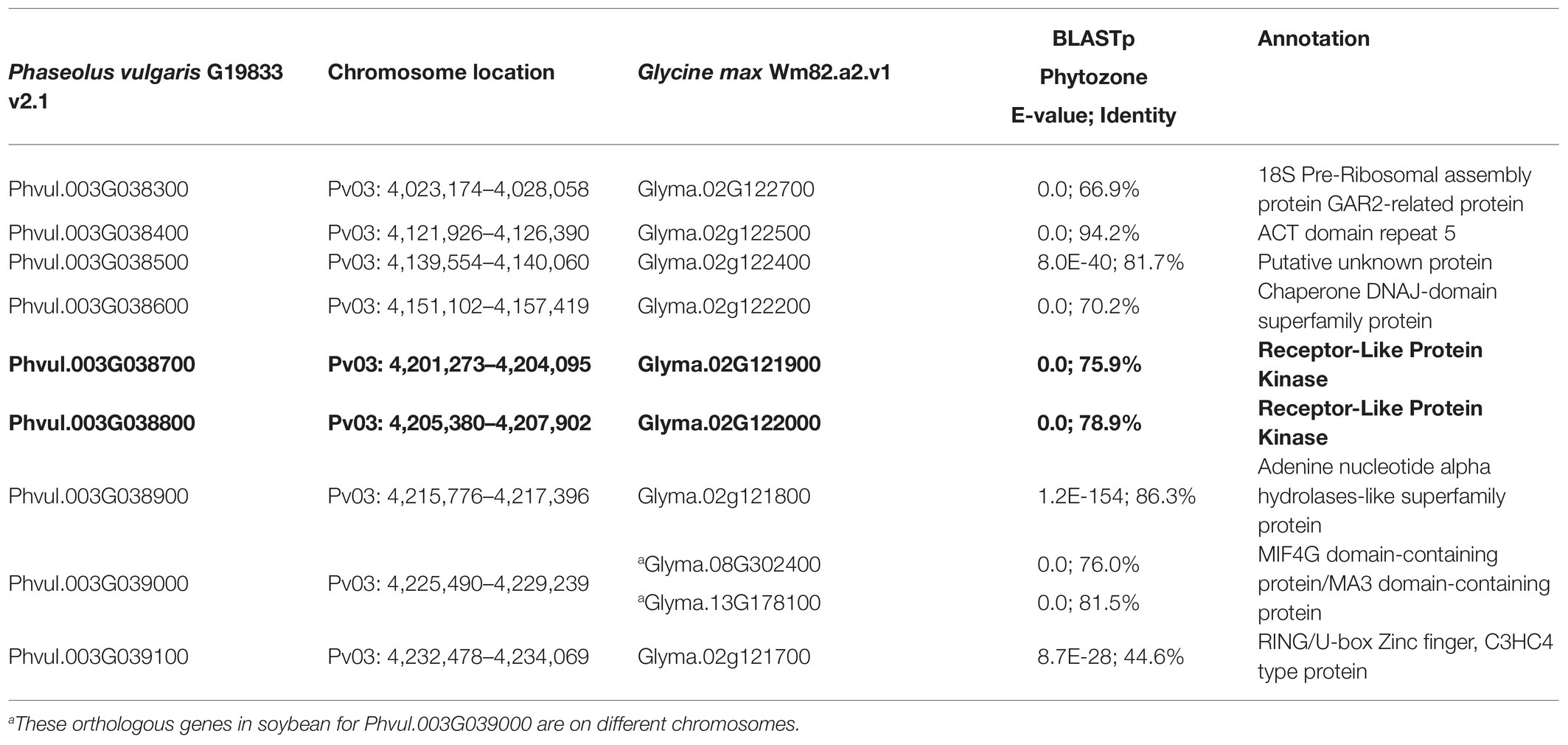
Table 3. Synteny analysis between common bean and soybean comparing bc-1 and Rsv4 candidate genes (bold type), respectively.
Phvul.003G039000, which encodes a MIF4G (middle domain of the eIF4G) domain-containing protein, is another putative candidate gene for bc-1 (Table 3). MIF4G conditions resistance to Rice yellow mottle virus (RYMV) in Oryza sativa (Albar et al., 2006). MIF4G is absent from the orthologous Gm02 region, so we conducted a protein–protein BLAST of the Phvul.003G039000 MIF4G protein, against the G. max, Wm82.a2.v1 reference genome. Similar protein sequence identity alignments on chromosomes Gm08 (Glyma.08G302400) and Gm13 (Glyma.13G178100; E-value = 0; identity = 76–81.5%, respectively) were obtained from the BLAST search. Glyma.13G178100 is 0.66 Mb upstream from the Rsv1 gene conditioning resistance to SMV and BCMV (Wu et al., 2019), which increases support of MIF4G (Phvul.003G039000) as a candidate gene for potyvirus resistance in common bean.
There were 15 gene models spanning the peak region (Pv05: 36,000,235–36,127,721 bases) for bc-u, with 289 SNVs, 58 InDels, and 18 STRs (Table 4). Coding variants were discovered in Phvul.005G122700 (one missense variant), Phvul.005G124000 (one synonymous variant), Phvul.005G124100 [two 3′ UTR (Un-Translated Regions), three 5′ UTR, one stop-gain, and three synonymous variants], and Phvul.005G124200 (three 3′ UTR variants; Figure 3A). No variants were found in Phvul.005G12320, Phvul.005G123600, and Phvul.005G123900 gene models. GWAS results revealed the stop-gain mutation in Phvul.005G124100 as the highest associated SNP for the bc-u gene (Figure 3B). A stop-gain mutation results in a premature termination codon that causes a shortening and likely reduced protein function. The Phvul.005G124100 gene model contains basic Leucine Zipper (bZIP) transcription factor and Delay of Germination 1 (DOG1) domains. bZIP transcription factors have been related to pathogen infection, stress signaling, seed maturation, and flower development in different crop species (Kaminaka et al., 2006; Liao et al., 2008; Alves et al., 2013; Gao et al., 2014). The Arabidopsis bZIP ortholog TGA9 (AT1G08320; BLASTp E-value = 7.74E-180; identity = 60%) is an active regulatory factor in autophagy, a physiological response in host-pathogen interactions (Wang et al., 2020). Consequently, of the 15 gene models, Phvul.005G124100 was chosen as the most likely candidate gene for bc-u.
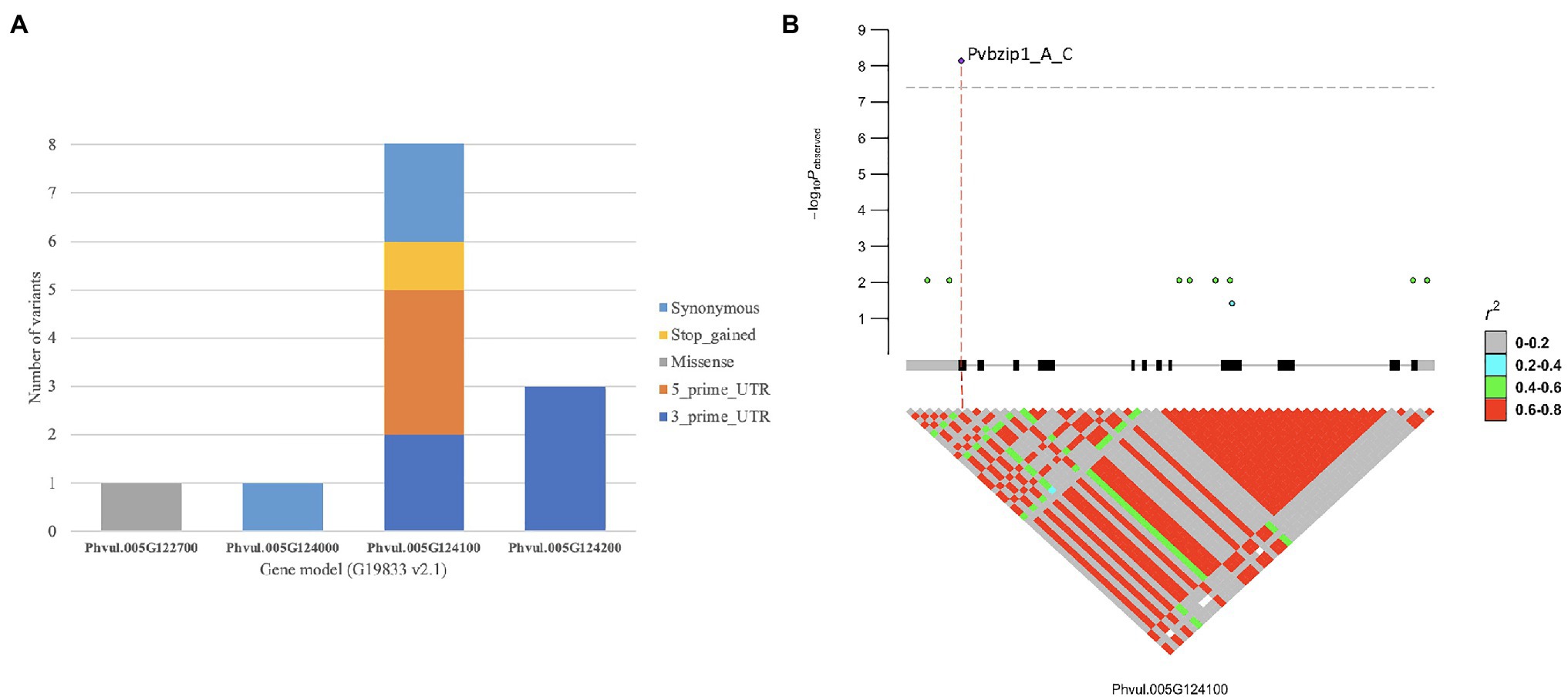
Figure 3. Candidate genes in bc-u region detected by GWAS: (A) coding variants distributed across gene models for the bc-ud gene region, and (B) Phvul.005G124100 basic Leucine Zipper (bZIP) gene-based marker-trait associations plot.
A separate GWAS, using DDP lines with (96 lines) and without (61 lines) I gene, detected a significant interval of 48.0–49.4 Mb on Pv02 as expected (Figure 4A). The 48.8 peak position was near the cluster of seven NBS-LRR (R gene motifs) gene models, from 48,786,657 to 48,895,789 base pairs, previously reported to have a putative association with the I gene (Vallejos et al., 2006; Bello et al., 2014). The high protein sequence identity ranging from 53.4 to 82.0% among the seven NBS-LRR genes limited the marker saturation for this region (Figure 4B). Genomic regions with repetitive DNA present a technical challenge for calling high-quality variants for use in GWAS and subsequent candidate gene analysis (Treangen and Salzberg, 2012). The GWAS results identified one missense SNP with a high p-value (p = 5.7E-80) on Pv02: 48,908,259 bases within the Phvul.002G324100 gene model encoding a PLATZ (Plant AT-rich sequence and zinc-binding) transcription factor family protein. This SNP is in high LD with the SNP identified by Bello et al. (2014), with both SNPs exhibiting 99.4% accuracy for detecting the I gene in the DDP. AC-Polaris expressing mild mosaic (mM) symptoms to NL-3, indicating the absence of I gene, possessed both SNPs (false positive), which was the only mismatch for these I gene-linked markers in the DDP.
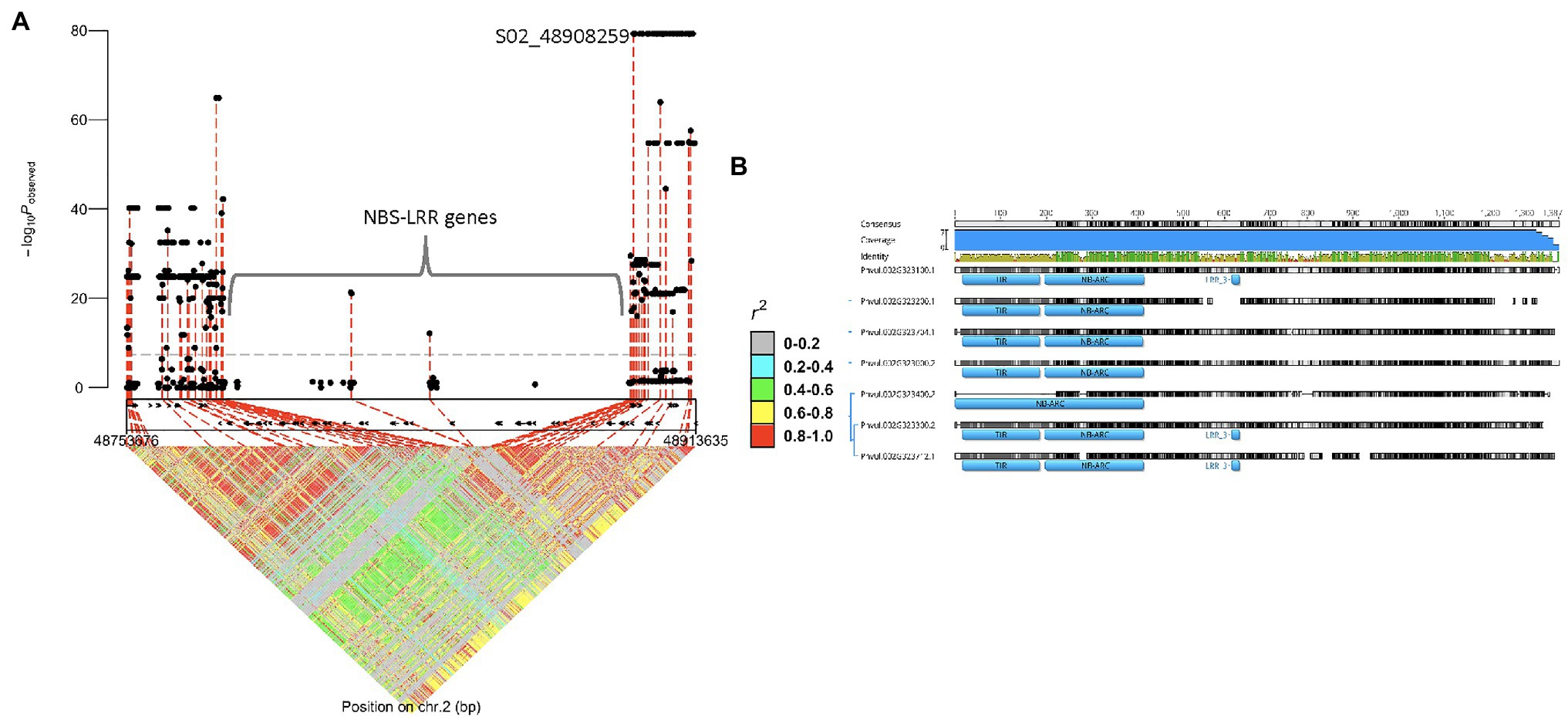
Figure 4. Regional association plot displaying GWAS results and candidate genes for I gene: (A) I gene regional marker-trait associations and linkage disequilibrium plots, and (B) alignment of the amino acid sequences of NBS-LRR cluster proteins.
Sequencing Candidate Genes
Twenty-five lines representing different BCMNV/BCMV HGs were chosen for targeted sequencing for the ORF of bc-1 and bc-u candidate genes (Supplementary Tables S6–S8; Supplementary Figures S1 and S2). For these lines, sequences of bc-1 candidate genes aligned to G19833 genome identified 22 missense SNPs, 10 synonymous SNPs, and one InDel in-frame insertion for Phvul.003G038700, and 27 missense SNPs, 13 synonymous SNPs, one in-frame InDel insertion and two in-frame InDel deletion variants for Phvul.003G038800 gene. No introns were detected for either of the RLK bc-1 candidate genes. Variants found in Phvul.003G038700 and Phvul.003G038800 were not polymorphic between lines reported as bc-1 and bc-12 in previous studies (Drijfhout, 1978; Strausbaugh et al., 1999). This result and segregation analyses below further support bc-1 and bc-12 as the same allele, which exhibits a differential effect based on the presence vs. absence of bc-ud.
For the ORF sequences of candidate gene Phvul.003G39000 MIF4G domain-containing protein, there were 16 missense SNPs, 12 synonymous SNPs, and two InDels (one in-frame InDel deletion and another splice region variant) found. These variants were grouped in six haplotypes, of which two haplotypes (Hap1 and Hap2) were identified in lines with bc-1 gene and Andean background, and four haplotypes (Hap3–Hap6) in lines with Durango background, but only Hap3 possessed the bc-1 gene (Supplementary Table S7).
The sequence of Phvul.005G124100 (bZIP), a candidate gene for bc-u, was analyzed by BLASTn to the G19833 genome (Supplementary Table S8). Multiple mutations were detected, including one stop-gain SNP in the first exon, two synonymous SNPs in the ninth exon, and one synonymous SNP in the twelfth exon. The protein sequence for the stop-gain mutation (Pv05:36,114,516 bases) exhibited a nonfunctional protein containing 14 amino acids in the Common Red landrace (Supplementary Figure S1). The unique nonsynonymous mutation comprises a single base transversion at codon 14 in the first exon. A preliminary gene symbol, bc-ud, is proposed for this mutation Pvbzip1_A_C due to its high frequency (55%) in the DDP. The “d” superscript signifies the bc-u allele of race Durango origin.
Temperature Melting-Shift Genotyping With Candidate SNP Markers
Single-nucleotide polymorphisms identified in coding regions of candidate genes for bc-1 and bc-ud were converted to Tm-shift assays (Wang et al., 2005). S03_420336 is a missense SNP in the RLK (Phvul.003G038700) candidate gene for bc-1 (Supplementary Figure S2). Due to the high number of missense variants found in the candidate genes for bc-1, this SNP was chosen based on primer design parameters such as % GC content and self-complementary score. Pvbzip1_A_C is the unique nonsynonymous SNP variant found in the Phvul.005G124100 candidate for the bc-ud gene. Additional Tm-shift assays were developed for SNPs to track the I and bc-3 genes. S02_48908259 is a missense SNP in Phvul.002G324100 PLATZ transcription factor, which is ~67 kb downstream from the NBS-LRR gene cluster and tightly linked with the I gene. The missense SNP at 27,204,768 bases (G19833 v2.1) in Phvul.006G168400 was used for the PveIF4E1,3,4_PveIF4E2 assay for bc-3 gene developed by Hart and Griffiths (2013). These Tm-shift assay markers (Supplementary Figures S3–S6) were used for genotyping the DDP, and progeny of the F2 and F3 populations for the presence of bc-1, bc-ud, I, and bc-3 to ascertain the resistance genes underpinning the phenotypic responses to BCMNV.
DDP Assays
The nine phenotypic categories for reaction to NL-8 and NL-3 strains in the DDP had distinct fixed genotypes for the I, bc-1, and bc-ud markers (Table 2; Supplementary Table S5). DDP lines with susceptible M symptoms to both strains lacked the resistance marker allele for all three resistance genes. Lines with dM to NL-8 and M to NL-3 had only the bc-ud resistance allele, indicating bc-ud alone had a minor effect in delaying, by about 7d, susceptible reactions to some BCMNV strains. DDP lines with only the bc-1 resistance allele were resistant (NS) to NL-8 and susceptible (M) to NL-3. DDP lines with both the bc-1 and bc-ud resistance alleles were similarly (NS) resistant to NL-8 but had partial resistance (mM) to NL-3.
Lines with resistant I and bc-ud alleles exhibited a similar effect of dTN to NL-8 strain by about 1 week, whereas these same lines had TN to NL-3. DDP lines with resistance I and bc-1 alleles exhibited resistant VN to NL-8 and susceptible TN to NL-3. However, lines with the bc-ud resistance allele in addition to I + bc-1 alleles expressed VN to both strains, which shows a critical role for bc-ud in protecting I + bc-1 lines against NL-3 strain. DDP lines with TN to both strains possessed just the I gene unprotected by any of the recessive “bc” genes.
Host Group Assays
Differential genotypes distributed across the 12 HGs for BCMNV/BCMV and one unassigned genotype UI-129 were assayed for the I, bc-1, and bc-ud linked SNP markers (Table 5). All HGs (HG-2, HG-3, HG-5, HG-9, and HG-10) reported in previous studies with bc-1 or bc-12 carried the bc-1 linked S03_4203361 SNP and the SBD5 SCAR markers. The SBD5 marker, located on Pv03 (4,204,236–4,205,565 bases), is in high LD with the S03_4203361 SNP in the DDP lines (data not shown). HG-1 differentials, not expected to possess bc-1, were mixed for the presence of bc-1 linked markers. For the HG-2, HG-5, and HG-9 genotypes previously reported to possess bc-1, none had the bc-ud gene marker, whereas most of HG-3 and HG-10 genotypes, previously reported to possess bc-12, also possessed bc-ud, with few exceptions. The Andean lines Redlands Greenleaf B (HG-3) and Amanda (HG-10) did not possess bc-ud.
A pattern for the presence of bc-ud and its effect when present can be hypothesized for race Durango lines across the host differentials in Table 5 and Supplementary Table S8. Briefly, bc-ud is present in race Durango lines in HG-3, HG-6, HG-10, and HG-11. All these HGs possess the bc-12 (formerly) or bc-22 alleles. Conversely, bc-ud is absent in HG-2, HG-4, and HG-9, which possess the alternative bc-1 and bc-2 alleles.
Allelism Test in F2:3 Families
Fixed Genotypes
The observed segregations for phenotypic reactions to BCMNV strains across F2:3 families from F2 plants with the same genotypes for I, bc-1, and bc-ud based on linked markers are summarized in Table 6 and presented in greater detail in Supplementary Tables S9 and S10. For even more detail, the genotype and phenotype for each F3 plant are in Supplementary Table S3. Each resistant gene-linked SNP marker fit expected codominant 1:2:1 ratio in all segregating populations and families (df = 2.0, p ≤ 0.05: 5.99). Accordingly, F2 individuals homozygous for the gene-linked markers bred true in the F3 generation. The true-breeding F2:3 families for one or more markers matched the phenotypes of the parents and other DDP lines with the same marker genotypes (Supplementary Table S5). For example, F2:3 plants or families fixed for I + bc-1 linked markers exhibited VN to NL-8 and TN to NL-3 (e.g., parental lines “Orion” and “Powderhorn”); I + bc-ud exhibited dTN to NL-8 and TN to NL-3 (“Gemini” and “Lariat”); and finally, I + bc-1 + bc-ud exhibited VN to both strains (“Beryl” and “Kodiak”). It was previously thought that the VN response to NL-3 strain was conditioned by the I + bc-12 combination (Drijfhout, 1978; Kelly, 1997; Miklas et al., 2000), whereas the results indicate bc-1 requires bc-ud to confine NL-3 strain to the inoculated leaf.
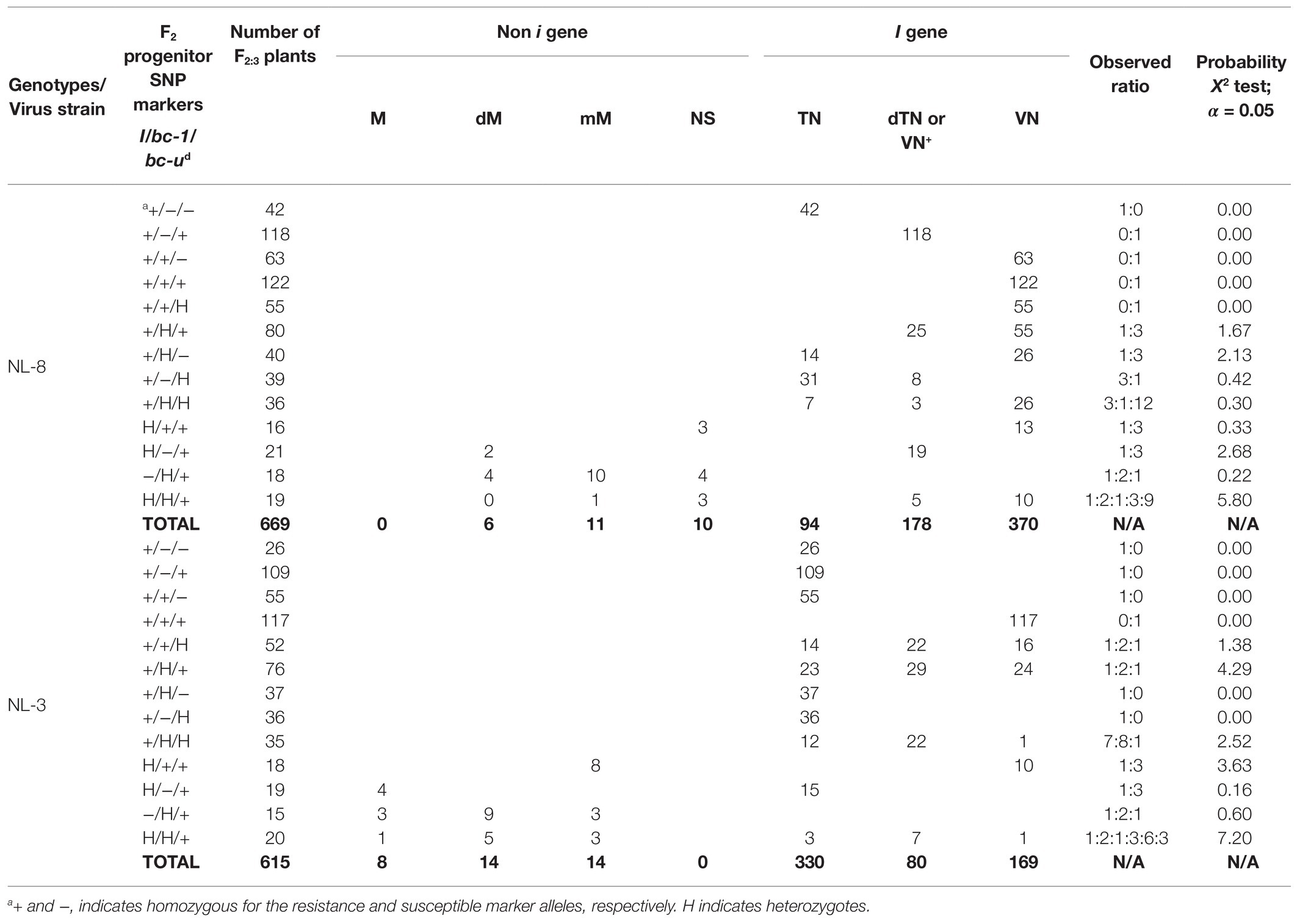
Table 6. Phenotypic reactions to BCMNV NL-8 and NL-3 strains, pooled across F2:3 families from multiple crosses with the same F2 genotype based on SNP genetic markers linked with I (S02_48908259), bc-1 (S03_4203361), and bc-ud (Pvbzip1_A_C) genes.
Segregating Families: NL-8 Reactions
The F2:3 families fixed for I gene and bc-1 genes (II/bc-1bc-1) all exhibited VN to NL-8 regardless of the allelic state for bc-ud. When the I gene was fixed and bc-1 was segregating, the observed phenotypes fit a 1 susceptible (TN or dTN) to 3 resistant (VN) ratio. The resistant VN reaction for II/Bc-1bc-1 heterozygotes was unexpected. Delayed TN by 1 week (dTN) was the susceptible reaction when bc-ud was fixed (II/bc-udbc-ud) and TN when (II/Bc-udBc-ud) or heterozygous (II/Bc-udbc-ud) were absent. When both bc-u and bc-1 were segregating and the I gene was fixed a dihybrid 3(TN):1(dTN):12(VN) segregation ratio was observed, which fit with the segregation ratios observed above for each gene.
The segregation of bc-1 in an F2:3 family with no I gene and fixed for bc-ud exhibited a 1(dM):2(mM):1(NS) segregation ratio for reaction to NL-8. The heterozygous ii/Bc-1bc-1/bc-udbc-ud individuals had partial mM resistance. In the absence of I or bc-1, individual homozygous for bc-ud (ii/Bc-1Bc-1/bc-udbc-ud) had mosaic symptoms delayed (dM) by 1 week, whereas individual homozygous for both bc-1 and bc-ud (ii/bc-1bc-1/bc-udbc-ud) had no symptoms.
Segregating Families: NL-3 Reactions
The F2:3 families fixed for I and bc-1 genes (II/bc-1bc-1) and segregating for bc-ud, exhibited 1(TN):2(dTN):1VN phenotypic segregation ratio to NL-3 strain. In this case, the heterozygous II/bc-1bc-1/Bc-udbc-ud genotype exhibited dTN, and homozygous genotypes II/bc-1bc-1/Bc-udBc-ud and II/bc-1bc-1/bc-udbc-ud had TN and VN, respectively. For F2:3 families with I and bc-ud genes fixed (II/bc-udbc-ud) and bc-1 segregating a 1(TN):2(VN+):1(VN) phenotypic segregation ratio was observed with most heterozygous genotypes for bc-1 (II/Bc-1bc-1/bc-udbc-ud) exhibiting VN+ (48% of plants) or dTN (33%) and a few VN (19%). For these II/Bc-1bc-1/bc-udbc-ud genotypes, VN+ and dTN symptoms started to appear in some plants at 3 wpi (Supplementary Table S3). The variable reactions for the heterozygotes may result from slight differences in virus titer or environmental conditions between inoculations. The homozygous individuals II/Bc-1Bc-1/bc-udbc-ud and II/bc-1bc-1/bc-udbc-ud had TN and VN, respectively.
The segregation of bc-1 in an F2:3 family with no I gene and fixed for bc-ud exhibited a 1(M):2(dM):1(mM) segregation ratio for reaction to NL-3. The heterozygous individuals ii/Bc-1bc-1/bc-udbc-ud had mosaic symptoms delayed by 1 week (dM). The homozygous ii/Bc-1Bc-1/bc-udbc-ud and ii/bc-1bc-1/bc-udbc-ud had M and mM, respectively.
Discussion
Genome-wide association study of the DDP confirmed the Bc-1 locus on Pv03 and located the Bc-u locus on Pv05. High-density SNP genotyping of the DDP using WGS enabled GWAS to detect narrow peak intervals for both loci and facilitate the discovery of candidate genes. The candidate gene markers developed for both loci were used to examine the phenotypic segregations in F2 populations and select F2:3 families. The candidate gene markers for bc-1 and bc-ud (formerly bc-u) and Tm-shift primers developed for bc-3 and the new marker for I gene, were further used to examine the resistance genotypes in the DDP and HG differentials. Ultimately, the marker for bc-ud was key for the discovery of major adjustments to the host-pathogen model from Drijfhout (1978) seminal work on pathogenicity and resistance to BCMNV/BCMV in common bean.
First, we show that there is only one recessive resistance allele bc-1 for the Bc-1 locus. This finding is supported by disease reaction and molecular marker analyses in the segregating populations and assays of the DDP and host differential cultivars. Furthermore, sequencing data for the RLK candidate gene exons did not detect any polymorphic variants between bc-1 (RGC) and bc-12 (Olathe) genotypes. So, instead of two alleles (bc-1 and bc-12), the differential expression for bc-1 results from the absence vs. presence of the bc-ud resistance allele.
Another new finding for bc-1 is the dominant and incompletely dominant inheritance observed in F2:3 families. Heterozygous Bc-1bc-1 and homozygous bc-1bc-1 genotypes with I gene fixed exhibited dominant VN symptoms to NL-8. Bc-1bc-1 heterozygotes with I and bc-ud genes fixed exhibited incomplete dominance (VN+) to NL-3 strain, which for most testing scenarios would reflect dominant inheritance because the vein necrosis reaction does not appear systemically until 3–5 wpi. Miklas et al. (2000) tagged bc-12 with the SBD5 SCAR marker using near-isogenic lines (NILs) they thought differed for bc-1 alleles (bc-1 vs. bc-12). They reported the order of dominance as bc-12>bc-1. However, in retrospect, each of their NILs possessed the dominant I and recessive bc-ud genes but differed for presence vs. absence of bc-1. This explains the dominant inheritance for resistance to NL-3 observed in an F2 population obtained from a cross between the NILs.
We also observed incomplete dominance for bc-1 in the absence of I gene (ii genotypes) and homozygous presence of bc-ud, but in this case, the disease reactions for the Bc-1bc-1 heterozygotes, mM to NL-8 and dM to NL-3, more closely resembled the homozygous (Bc-1Bc-1) susceptible dM and M reactions, respectively. We were unable to examine the inheritance of bc-1 in the absence of I and bc-ud genes because our crosses did not segregate for those genotypes. However, DDP lines (“Sapphire” and “Croissant”) with markers indicating the presence of the recessive bc-1 only were resistant (NS) to NL-8 and susceptible (M) to NL-3, indicating bc-1 by itself is effective in conditioning resistance against some BCMNV strains.
A second major change to the host-pathogen interaction model is that bc-u is not ubiquitous across HGs 2–7, which lack the I gene. This new finding necessitated the need to mark this absence of bc-u in HGs where it was previously thought to be present. Therefore, we added the “d” superscript to track this specific allele going forward. The HGs 2, 4, 5, and 7 lacked bc-ud, whereas bc-ud was present in HG-6 and most HG-3 genotypes. For HGs with I gene, bc-ud was absent in HG-8 and HG-9a and 9b and present in HG-11 as expected, but it was also present in some HG-10 genotypes, which was unexpected.
All DDP lines in HG-3 and HG-10 possessed bc-ud, whereas bc-ud is absent in many snap beans or lines of Andean origin within these HGs. Redlands Greenleaf B (RGB), an Andean line categorized as HG-3, and reported previously to possess the bc-u gene, showed the wild type Bc-u protein form. Strausbaugh et al. (2003) observed quantitative differences in levels of partial resistance among lines in HG-3 inoculated with NL-3, and we hypothesize that these quantitative differences are due to different/independent “bc-u”-like helper genes. A homologous bZIP domain gene Phvul.011G093700 on Pv11 (G19833 v2.1: 9,431,906–9,439,104 base pairs) with 73.9% similarity to Phvul.005G124100 is under investigation as an alternative helper gene for HG-3 lines with bc-1, such as RGB, and Amanda in HG-10.
Further investigation of why some HG-1 differentials, such as Bountiful and stringless green refugee (SGR), that are susceptible to all BCMNV and BCMV strains possess the bc-1 linked marker, whereas others, like Dubbele Witte and Sutter Pink, do not, is warranted. Myers et al. (1996) observed segregation for susceptible and resistant reactions in an F2 cross between Sutter Pink (susceptible) and SGR (resistant) inoculated with BCMV strain Blackeye cowpea mosaic virus (BCMV-BlCMV). A single resistance gene from SGR with dominant inheritance was observed when symptoms were recorded 3 wpi and with incomplete dominance when recorded 7 wpi. They indicated SGR possessed bc-u and perhaps bc-u or some other genetic factor was conferring resistance to BCMV-BlCMV. The marker results reveal that SGR possesses bc-1 but not bc-u. However, we similarly observed dominant gene action at 3 wpi and partial dominance at 5 wpi to BCMNV for some F2:3 families segregating for bc-1. Larsen and Miklas (2010) observed bc-1 conferred resistance to Peanut mottle virus (PeMoV), and in subsequent F2 populations (unpublished data) observed dominant gene action.
bc-u (now bc-ud) is relocated on Pv05, and independent from bc-1, contrary to the loose (23 cM) linkage observed between them by Strausbaugh et al. (1999). Perhaps the loose linkage was observed because some of the RILs were either misclassified as partially resistant to NL-3 when in fact they were susceptible or vice versa. They classified RILs with Bc-uBc-u/bc-12bc-12 genotype as expressing mM reactions, whereas we observed such genotypes to have susceptible M reactions to NL-3 in this study. These differences may be influenced by recording disease reactions every week for 5 wpi, whereas they recorded disease reactions once at 3 wpi.
Another new finding for bc-ud is that it conditioned a delay in the onset of susceptible reactions by about 1 wpi when bc-1 was absent and significantly enhanced the level of resistance in the presence of bc-1. Bc-1Bc-1/bc-udbc-ud genotypes inoculated with NL-8 exhibited dTN and dM symptoms in the presence vs. absence of I gene, respectively, whereas bc-1bc-1/bc-udbc-ud genotypes inoculated with NL-3 exhibited resistant VN and partially resistant mM symptoms. Moreover, II/bc-1bc-1/Bc-udbc-ud genotype exhibited dTN to NL-3, indicating bc-ud in heterozygous condition with both I and bc-1 genes fixed delayed the onset of TN by about 1 wpi.
Plants with the I gene can be useful for understanding the mechanisms of other recessive resistance genes (bc-ud, bc-1) when present in combination with the I gene. The I gene alone conditions HR to BCMNV strains in common bean leading to TN and eventual plant death. This TN symptom in beans is similar to the phenomenon known as trailing necrosis, where HR does not prevent the pathogen from moving systemically from cell to cell, and in turn expands the HR to other cells and tissues (Balint-Kurti, 2019). Although HR is associated with pathogen resistance, when it results in systemic cell to cell movement and eventual plant death, it can be considered a consequence of pathogen compatibility. Whether the HR mechanism leads to systemic cell death (TN), delayed TN, or restricted vein necrosis (VN) is controlled by other proteins that recognize specific pathogen effectors, such as receptor molecules like RLK and NBS-LRR proteins. Moreover, several families of transcription factors have been discovered to regulate programmed cell death and HR, including members of bZIP, MBY, NAC, ERF, and WRKY (Burke et al., 2020).
In the present study, we identified a candidate gene for bc-ud that encodes a bZIP transcription factor. This candidate gene (Phvul.005G124100) is located on Pv05 from 36,113,780 to 36,120,803 bases. The results for bc-ud predict a loss-function of bZIP protein due to the presence of a stop-gain variant. The silenced protein combined with the I and bc-1 genes affects complete resistance characterized by restricted vein necrosis on the inoculated primary leaves to BCMNV strain NL-3. Several studies have demonstrated the importance of bZIP transcription factors modulating different regulatory networks and signaling pathways related to physiological processes (Alves et al., 2013). Some of these processes are related to biotic stress, particularly in the defense against pathogens. In Arabidopsis thaliana, the bZIP protein TGA9 (AT1G08320), which regulates the autophagy under stress conditions, showed in TGA9–2 and TGA9–3 mutants, significative repression of autophagy activation under stress but not completely blocked (Wang et al., 2020). Another bZIP in A. thaliana, AtbZIP10, has been identified as a positive regulator of the pathogen-induced hypersensitive cell-death response (HR) and in basal defense response. AtbZIP10 interacts with LSD1 (lesions simulating disease resistance 1), a protein that protects against cell death mediated by oxidative stress signals (Kaminaka et al., 2006). Genetic interactions have been reported between bZIP transcription factors and the tobacco (Nicotiana tabacum) NPR1 gene, an NBS-LRR class protein conferring resistance to Tobacco mosaic virus (TMV; Liu et al., 2002). When only bc-ud is combined with the I gene, the HR response is delayed by about 1 week in response to NL-8.
Receptor-like protein kinases play a relevant role in interaction networks between plants and viruses (Tang et al., 2017; Macho and Lozano-Duran, 2019). Proteins with receptor-like domains interact with viral movement proteins promoting the viral movement into tubules within plasmodesmata (Amari et al., 2010). The bc-1 recessive gene was found to affect the systemic spread of BCMV in common beans (Feng et al., 2017, 2018). We identified two RLKs as candidate genes for bc-1 in this study. The two RLK genes on Pv03 in the common bean are orthologous with two RLK genes on Gm02 in soybean reported separately to condition resistance to TRSV and SMV (Ilut et al., 2015; Chang et al., 2016). Both RLK genes were identified as candidate genes for the Rsv4 gene, which presents a dominant or semi-dominant resistance to SMV in soybean (Ilut et al., 2015). Another study in soybean revealed a major QTL near Rsv4, which conditioned resistance to Clover yellow vein virus (ClYVV). This QTL was named d-cv gene to acknowledge the recessive inheritance as different from the Rsv4 gene (Abe et al., 2019). In summary, the synteny analysis between common bean and soybean shows that the genomic regions possessing bc-1 and Rsv4 genes are orthologous and involved in resistance to multiple ssRNA (+) viruses. Finally, Phvul.003G039000, which encodes a MIF4G domain-containing protein, cannot be discounted as a candidate gene for bc-1. However, a MIF4G annotated gene is absent from the orthologous Rsv4 region on Gm02.
Many breeding programs are moving toward the introgression of the bc-3 (eIF4e) resistance gene to control BCMNV and BCMV. However, a new BCMV isolate has been identified, 1755a (PG-VIII), which causes mosaic and deformed leaf symptoms in some common bean lines with the bc-3 gene (Feng et al., 2015). This study shows that bc-ud and bc-1 can be introgressed in breeding lines to achieve suitable and improved resistance to BCMNV. Perhaps, bc-ud combined with bc-3 will provide resistance to the new BCMV strain. Deploying bc-ud in a snap and Andean beans that are nearly ubiquitous for bc-1 would broaden the virus resistance of those market classes and gene pools. The SNP markers developed will be useful for deploying bc-ud and bc-1 resistance genes in common bean, and those tools combined with the identified candidate genes will help to further elucidate the host-pathogen interaction between potyviruses and common bean.
Data Availability Statement
The original contributions presented in the study are publicly available. This data can be found here: NCBI SRA database with accession Bioproject PRJNA386820.
Author Contributions
AS-G and PNM conceived and designed the experiments, and wrote the paper. AS-G conducted the genomics analyses, genotyping, and phenotyping. PEM generated SNP data. All authors contributed to the article and approved the submitted version.
Conflict of Interest
The authors declare that the research was conducted in the absence of any commercial or financial relationships that could be construed as a potential conflict of interest.
Acknowledgments
We thank James Myers for his helpful review of the manuscript.
Supplementary Material
The Supplementary Material for this article can be found online at: https://www.frontiersin.org/articles/10.3389/fpls.2021.699569/full#supplementary-material
Footnotes
1. ^https://www.ncbi.nlm.nih.gov/bioproject/?term=PRJNA386820
References
Abe, J., Wang, Y., Yamada, T., Sato, M., Ono, T., Atsumi, G., et al. (2019). Recessive resistance governed by a major quantitative trait locus restricts Clover yellow vein virus in mechanically but not graft-inoculated cultivated soybeans. Mol. Plant Microbe Interact. 32, 1026–1037. doi: 10.1094/MPMI-12-18-0331-R
Albar, L., Bangratz-Reyser, M., Hébrard, E., Ndjiondjop, M. N., Jones, M., and Ghesquière, A. (2006). Mutations in the eIF(iso)4G translation initiation factor confer high resistance of rice to Rice yellow mottle virus. Plant J. 47, 417–426. doi: 10.1111/j.1365-313X.2006.02792.x
Alves, M., Dadalto, S., Gonçalves, A., De Souza, G., Barros, V., and Fietto, L. (2013). Plant bZIP transcription factors responsive to pathogens: a review. Int. J. Mol. Sci. 14, 7815–7828. doi: 10.3390/ijms14047815
Amari, K., Boutant, E., Hofmann, C., Schmitt-Keichinger, C., Fernandez-Calvino, L., Didier, P., et al. (2010). A family of plasmodesmal proteins with receptor-like properties for plant viral movement proteins. PLoS Pathog. 6:e1001119. doi: 10.1371/journal.ppat.1001119
Balint-Kurti, P. (2019). The plant hypersensitive response: concepts, control and consequences. Mol. Plant Pathol. 20, 1163–1178. doi: 10.1111/mpp.12821
Bello, M. H., Moghaddam, S. M., Massoudi, M., McClean, P. E., Cregan, P. B., and Miklas, P. N. (2014). Application of in silico bulked segregant analysis for rapid development of markers linked to bean common mosaic virus resistance in common bean. BMC Genomics 15:903. doi: 10.1186/1471-2164-15-903
Burke, R., Schwarze, J., Sherwood, O. L., Jnaid, Y., McCabe, P. F., and Kacprzyk, J. (2020). Stressed to death: The role of transcription factors in plant programmed cell death induced by abiotic and biotic stimuli. Front. Plant Sci. 11:1235. doi: 10.3389/fpls.2020.01235
Chang, H.-X., Brown, P. J., Lipka, A. E., Domier, L. L., and Hartman, G. L. (2016). Genome-wide association and genomic prediction identifies associated loci and predicts the sensitivity of Tobacco ringspot virus in soybean plant introductions. BMC Genomics 17:153. doi: 10.1186/s12864-016-2487-7
Cichy, K. A., Porch, T. G., Beaver, J. S., Cregan, P., Fourie, D., Glahn, R. P., et al. (2015). A Phaseolus vulgaris diversity panel for Andean bean improvement. Crop Sci. 55, 2149–2160. doi: 10.2135/cropsci2014.09.0653
Díaz, L. M., and Blair, M. W. (2006). Race structure within the Mesoamerican gene pool of common bean (Phaseolus vulgaris L.) as determined by microsatellite markers. Theor. Appl. Genet. 114, 143–154. doi: 10.1007/s00122-006-0417-9
Diaz, S., Ariza-Suarez, D., Izquierdo, P., Lobaton, J. D., de la Hoz, J. F., Acevedo, F., et al. (2020). Genetic mapping for agronomic traits in a MAGIC population of common bean (Phaseolus vulgaris L.) under drought conditions. BMC Genomics 21:799. doi: 10.1186/s12864-020-07213-6
Drijfhout, E. (1978). Genetic interaction between Phaseolus vulgaris and bean common mosaic virus with implications for strain identification and breeding for resistance. Versl. Landbouwkd. Onderz. 872, 1–89.
Drijfhout, E., Silbernagel, M., and Burke, D. (1978). Differentiation of strains of bean common mosaic virus. Neth. J. Plant Pathol. 84, 13–26. doi: 10.1007/BF01978099
Feng, X., Guzmán, P., Myers, J. R., and Karasev, A. V. (2017). Resistance to Bean common mosaic necrosis virus conferred by the bc-1 gene affects systemic spread of the virus in common bean. Phytopathology 107, 893–900. doi: 10.1094/PHYTO-01-17-0013-R
Feng, X., Myers, J. R., and Karasev, A. V. (2015). Bean common mosaic virus isolate exhibits a novel pathogenicity profile in common bean, overcoming the bc-3 resistance allele coding for the mutated eIF4E translation initiation factor. Phytopathology 105, 1487–1495. doi: 10.1094/PHYTO-04-15-0108-R
Feng, X., Orellana, G. E., Myers, J. R., and Karasev, A. V. (2018). Recessive resistance to bean common mosaic virus conferred by the bc-1 and bc-2 genes in common bean (Phaseolus vulgaris) affects long-distance movement of the virus. Phytopathology 108, 1011–1018. doi: 10.1094/PHYTO-01-18-0021-R
Gao, M., Zhang, H., Guo, C., Cheng, C., Guo, R., Mao, L., et al. (2014). Evolutionary and expression analyses of basic zipper transcription factors in the highly homozygous model grape PN40024 (Vitis vinifera L.). Plant Mol. Biol. Report. 32, 1085–1102. doi: 10.1007/s11105-014-0723-3
Hart, J. P., and Griffiths, P. D. (2013). A series of eIF4E alleles at the Bc-3 locus are associated with recessive resistance to Clover yellow vein virus in common bean. Theor. Appl. Genet. 126, 2849–2863. doi: 10.1007/s00122-013-2176-8
He, F., Ding, S., Wang, H., and Qin, F. (2020). IntAssoPlot: An R package for integrated visualization of genome-wide association study results with gene structure and linkage disequilibrium matrix. Front. Genet. 11:260. doi: 10.3389/fgene.2020.00260
Ilut, D. C., Lipka, A. E., Jeong, N., Nyuk, D., Hyun, D., Ji, K., et al. (2015). Identification of haplotypes at the Rsv 4 genomic region in soybean associated with durable resistance to Soybean mosaic virus. Theor. Appl. Genet. 129, 453–468. doi: 10.1007/s00122-015-2640-8
Kaminaka, H., Näke, C., Epple, P., Dittgen, J., Schütze, K., Chaban, C., et al. (2006). bZIP10-LSD1 antagonism modulates basal defense and cell death in Arabidopsis following infection. EMBO J. 25, 4400–4411. doi: 10.1038/sj.emboj.7601312
Kearse, M., Moir, R., Wilson, A., Stones-Havas, S., Cheung, M., Sturrock, S., et al. (2012). Geneious basic: an integrated and extendable desktop software platform for the organization and analysis of sequence data. Bioinformatics 28, 1647–1649. doi: 10.1093/bioinformatics/bts199
Kelly, J. D. (1997). A review of varietal response to bean common mosaic potyvirus in Phaseolus vulgaris. Plant Var. Seeds 10, 1–6.
Koressaar, T., and Remm, M. (2007). Enhancements and modifications of primer design program Primer3. Bioinformatics 23, 1289–1291. doi: 10.1093/bioinformatics/btm091
Larsen, R. C., Druffel, K. L., and Wyatt, S. D. (2011). The complete nucleotide sequences of Bean common mosaic necrosis virus strains NL-5, NL-8 and TN-1. Arch. Virol. 156, 729–732. doi: 10.1007/s00705-011-0945-8
Larsen, R. C., and Miklas, P. N. (2010). Mapping resistance to Peanut mottle virus in common bean. Annu. Rep. Bean Improv. Coop. 53, 50–51.
Larsen, R. C., Miklas, P. N., Druffel, K. L., and Wyatt, S. D. (2005). NL-3 K strain is a stable and naturally occurring interspecific recombinant derived from Bean common mosaic necrosis virus and Bean common mosaic virus. Phytopathology 95, 1037–1042. doi: 10.1094/PHYTO-95-1037
Li, H. (2013). Aligning sequence reads, clone sequences and assembly contigs with BWA-MEM. Available at: http://github.com/lh3/bwa (Accessed October 16, 2020).
Li, H., Handsaker, B., Wysoker, A., Fennell, T., Ruan, J., Homer, N., et al. (2009). The sequence alignment/map format and SAMtools. Bioinformatics 25, 2078–2079. doi: 10.1093/bioinformatics/btp352
Liao, Y., Zhang, J.-S., Chen, S.-Y., and Zhang, W.-K. (2008). Role of soybean GmbZIP132 under abscisic acid and salt stresses. J. Integr. Plant Biol. 50, 221–230. doi: 10.1111/j.1744-7909.2007.00593.x
Lipka, A. E., Tian, F., Wang, Q., Peiffer, J., Li, M., Bradbury, P. J., et al. (2012). GAPIT: genome association and prediction integrated tool. Bioinformatics 28, 2397–2399. doi: 10.1093/bioinformatics/bts444
Liu, Y., Schiff, M., Marathe, R., and Dinesh-Kumar, S. P. (2002). Tobacco Rar1, EDS1 and NPR1/NIM1 like genes are required for N-mediated resistance to tobacco mosaic virus. Plant J. 30, 415–429. doi: 10.1046/j.1365-313X.2002.01297.x
Lobaton, J. D., Miller, T., Gil, J., Ariza, D., de la Hoz, J. F., Soler, A., et al. (2018). Resequencing of common bean identifies regions of inter-gene pool introgression and provides comprehensive resources for molecular breeding. Plant Genome 11:170068. doi: 10.3835/plantgenome2017.08.0068
Macho, A. P., and Lozano-Duran, R. (2019). Molecular dialogues between viruses and receptor-like kinases in plants. Mol. Plant Pathol. 20, 1191–1195. doi: 10.1111/mpp.12812
Maroof, M. A. S., Tucker, D. M., Skoneczka, J. A., Bowman, B. C., Tripathy, S., and Tolin, S. A. (2010). Fine mapping and candidate gene discovery of the Soybean mosaic virus resistance gene, Rsv4. Plant Genome J. 3:14. doi: 10.3835/plantgenome2009.07.0020
McClean, P. E., Bett, K. E., Stonehouse, R., Lee, R., Pflieger, S., Moghaddam, S. M., et al. (2018). White seed color in common bean (Phaseolus vulgaris) results from convergent evolution in the P (pigment) gene. New Phytol. 219, 1112–1123. doi: 10.1111/nph.15259
Miklas, P., Chilagane, L., Fourie, D., Nchimbi, S., Soler-Garzon, A., Hart, J. P., et al. (2020). QTL for resistance to angular leaf spot and rust in Tanzania vs South Africa for the Andean panel & Rojo/Cal 143 RIL population. Annu. Rep. Bean Improv. Coop. 63, 83–84.
Miklas, P. N., Larsen, R. C., Riley, R., and Kelly, J. D. (2000). Potential marker-assisted selection for bc-12 resistance to bean common mosaic potyvirus in common bean. Euphytica 3, 211–219.
Moghaddam, S. M., Mamidi, S., Osorno, J. M., Lee, R., Brick, M., Kelly, J., et al. (2016). Genome-wide association study identifies candidate loci underlying agronomic traits in a middle american diversity panel of common bean. Plant Genome 9, 1–21. doi: 10.3835/plantgenome2016.02.0012
Morales, F. J. (2003). “Common bean,” in Virus and Virus-like Diseases of Major Crops in Developing Countries. eds. G. Loebenstein and G. Thottappilly (Dordrecht: Springer), 425–445.
Myers, J. R., Strausbaugh, C. A., Forster, R. L., Stewart-Williams, K. D., and McClean, P. E. (1996). Genetics of blackeye cowpea mosaic virus (BlCMV) resistance in bean. Annu. Rep. Bean Improv. Coop. 39, 162–163.
Naderpour, M., Lund, O. S., Larsen, R., and Johansen, E. (2010). Potyviral resistance derived from cultivars of Phaseolus vulgaris carrying bc-3 is associated with the homozygotic presence of a mutated eIF4E allele. Mol. Plant Pathol. 11, 255–263. doi: 10.1111/j.1364-3703.2009.00602.x
Nieto, C., Morales, M., Orjeda, G., Clepet, C., Monfort, A., Sturbois, B., et al. (2006). An eIF4E allele confers resistance to an uncapped and non-polyadenylated RNA virus in melon. Plant J. 48, 452–462. doi: 10.1111/j.1365-313X.2006.02885.x
Oladzad, A., Porch, T., Rosas, J. C., Moghaddam, S. M., Beaver, J., Beebe, S. E., et al. (2019). Single and multi-trait GWAS identify genetic factors associated with production traits in common bean under abiotic stress environments. Genes Genomes Genet. 9, 1881–1892. doi: 10.1534/g3.119.400072
Parrella, G., Ruffel, S., Moretti, A., Morel, C., Palloix, A., and Caranta, C. (2002). Recessive resistance genes against potyviruses are localized in colinear genomic regions of the tomato (Lycopersicon spp.) and pepper (Capsicum spp.) genomes. Theor. Appl. Genet. 105, 855–861. doi: 10.1007/s00122-002-1005-2
Perea, C., De La Hoz, J. F., Cruz, D. F., Lobaton, J. D., Izquierdo, P., Quintero, J. C., et al. (2016). Bioinformatic analysis of genotype by sequencing (GBS) data with NGSEP. BMC Genomics 17:498. doi: 10.1186/s12864-016-2827-7
Richard, M. M. S., Gratias, A., Alvarez Diaz, J. C., Thareau, V., Pflieger, S., Meziadi, C., et al. (2021). A common bean truncated CRINKLY4 kinase controls gene-for-gene resistance to the fungus Colletotrichum lindemuthianum. J. Exp. Bot. 72, 3569–3581. doi: 10.1093/jxb/erab082
Ruffel, S., Caranta, C., Palloix, A., Lefebvre, V., Caboche, M., and Bendahmane, A. (2004). Structural analysis of the eukaryotic initiation factor 4E gene controlling potyvirus resistance in pepper: exploitation of a BAC library. Gene 338, 209–216. doi: 10.1016/j.gene.2004.05.010
Scheet, P., and Stephens, M. (2006). A fast and flexible statistical model for large-scale population genotype data: applications to inferring missing genotypes and haplotypic phase. Am. J. Hum. Genet. 78, 629–644. doi: 10.1086/502802
Schmutz, J., McClean, P. E., Mamidi, S., Wu, G. A., Cannon, S. B., Grimwood, J., et al. (2014). A reference genome for common bean and genome-wide analysis of dual domestications. Nat. Genet. 46, 707–713. doi: 10.1038/ng.3008
Soler-Garzón, A., Oladzadabbasabadi, A., Beaver, J., Beebe, S., Lee, R. K., Lobaton, J., et al. (2021). NAC candidate gene marker for bgm-1 and interaction with QTL for resistance to Bean golden yellow mosaic virus in common bean. Front. Plant Sci. 12:628443. doi: 10.3389/fpls.2021.628443
Song, Q., Jia, G., Hyten, D. L., Jenkins, J., Hwang, E.-Y., Schroeder, S. G., et al. (2015). SNP assay development for linkage map construction, anchoring whole genome sequence and other genetic and genomic applications in common bean. Genes Genomes Genet. 5, 2285–2290. doi: 10.1534/g3.115.020594
Strausbaugh, C. A., Miklas, P. N., Singh, S. P., Myers, J. R., and Forster, R. L. (2003). Genetic characterization of differential reactions among host group 3 common bean cultivars to nl-3 k strain of Bean common mosaic necrosis virus. Phytopathology 93, 683–690. doi: 10.1094/PHYTO.2003.93.6.683
Strausbaugh, C. A., Myers, J. R., Forster, R. L., and McClean, P. E. (1999). Bc-1 and Bc-u - two loci controlling bean common mosaic virus resistance in common bean are linked. J. Am. Soc. Hortic. Sci. 124, 644–648. doi: 10.21273/JASHS.124.6.644
Swisher Grimm, K. D., and Porter, L. D. (2020). Development and validation of KASP markers for the identification of Pea seedborne mosaic virus pathotype P1 resistance in Pisum sativum. Plant Dis. 104, 1824–1830. doi: 10.1094/PDIS-09-19-1920-RE
Tang, D., Wang, G., and Zhou, J. M. (2017). Receptor kinases in plant-pathogen interactions: more than pattern recognition. Plant Cell 29, 618–637. doi: 10.1105/tpc.16.00891
Tobar-Piñón, M. G., Mafi Moghaddam, S., Lee, R. K., Villatoro Mérida, J. C., DeYoung, D. J., Reyes, B. A., et al. (2021). Genetic diversity of Guatemalan climbing bean collections. Genet. Resour. Crop. Evol. 68, 639–656. doi: 10.1007/s10722-020-01013-3
Treangen, T. J., and Salzberg, S. L. (2012). Repetitive DNA and next-generation sequencing: computational challenges and solutions. Nat. Rev. Genet. 13, 36–46. doi: 10.1038/nrg3117
Untergasser, A., Cutcutache, I., Koressaar, T., Ye, J., Faircloth, B. C., Remm, M., et al. (2012). Primer3--new capabilities and interfaces. Nucleic Acids Res. 40:e115. doi: 10.1093/nar/gks596
Vallejos, C. E., Astua-Monge, G., Jones, V., Plyler, T. R., Sakiyama, N. S., and Mackenzie, S. A. (2006). Genetic and molecular characterization of the I locus of Phaseolus vulgaris. Genetics 172, 1229–1242. doi: 10.1534/genetics.105.050815
Vandemark, G. J., and Miklas, P. N. (2005). Genotyping common bean for the potyvirus resistance alleles I and bc-12 with a multiplex real-time polymerase chain reaction assay. Phytopathology 95, 499–505. doi: 10.1094/PHYTO-95-0499
Wallace, L., Arkwazee, H., Vining, K., and Myers, J. R. (2018). Genetic diversity within snap beans and their relation to dry beans. Gen. Dent. 9:587. doi: 10.3390/genes9120587
Wang, J., Chuang, K., Ahluwalia, M., Patel, S., Umblas, N., Mirel, D., et al. (2005). High-throughput SNP genotyping by single-tube PCR with Tm-shift primers. Biotechniques 39, 885–893. doi: 10.2144/000112028
Wang, P., Nolan, T. M., Yin, Y., and Bassham, D. C. (2020). Identification of transcription factors that regulate ATG8 expression and autophagy in Arabidopsis. Autophagy 16, 123–139. doi: 10.1080/15548627.2019.1598753
Wang, S. B., Feng, J. Y., Ren, W. L., Huang, B., Zhou, L., Wen, Y. J., et al. (2016). Improving power and accuracy of genome-wide association studies via a multi-locus mixed linear model methodology. Sci. Rep. 6, 1–10. doi: 10.1038/srep19444
Wen, Y.-J., Zhang, H., Ni, Y.-L., Huang, B., Zhang, J., Feng, J.-Y., et al. (2018). Methodological implementation of mixed linear models in multi-locus genome-wide association studies. Brief. Bioinform. 19, 700–712. doi: 10.1093/bib/bbw145
Wu, J., Wang, L., Fu, J., Chen, J., Wei, S., Zhang, S., et al. (2020). Resequencing of 683 common bean genotypes identifies yield component trait associations across a north–south cline. Nat. Genet. 52, 118–125. doi: 10.1038/s41588-019-0546-0
Wu, M., Liu, Y. N., Zhang, C., Liu, X. T., Liu, C. C., Guo, R., et al. (2019). Molecular mapping of the gene(s) conferring resistance to soybean mosaic virus and Bean common mosaic virus in the soybean cultivar Raiden. Theor. Appl. Genet. 132, 3101–3114. doi: 10.1007/s00122-019-03409-x
Xin, Z., Velten, J. P., Oliver, M. J., and Burke, J. J. (2003). High-throughput DNA extraction method suitable for PCR. Biotechniques 34, 820–826. doi: 10.2144/03344rr04
Yin, L. (2016). CMplot. Available at: https://github.com/YinLiLin/CMplot (Accessed March 17, 2021).
Keywords:Phaseolus vulgaris, potyvirus, autophagy, hypersensitive response, transcription factors, marker-assisted selection, receptor-like kinases, synteny
Citation: Soler-Garzón A, McClean PE and Miklas PN (2021) Genome-Wide Association Mapping of bc-1 and bc-u Reveals Candidate Genes and New Adjustments to the Host-Pathogen Interaction for Resistance to Bean Common Mosaic Necrosis Virus in Common Bean. Front. Plant Sci. 12:699569. doi: 10.3389/fpls.2021.699569
Edited by:
Peng Zhang, The University of Sydney, AustraliaReviewed by:
Laura Ashlee Ziems, The University of Sydney, AustraliaAlexander Karasev, University of Idaho, United States
Copyright © 2021 Soler-Garzón, McClean and Miklas. This is an open-access article distributed under the terms of the Creative Commons Attribution License (CC BY). The use, distribution or reproduction in other forums is permitted, provided the original author(s) and the copyright owner(s) are credited and that the original publication in this journal is cited, in accordance with accepted academic practice. No use, distribution or reproduction is permitted which does not comply with these terms.
*Correspondence: Phillip N. Miklas, phil.miklas@usda.gov, orcid.org/0000-0002-6636-454X