- 1School of Agronomy, Anhui Agricultural University, Hefei, China
- 2Tasmanian Institute of Agriculture, University of Tasmania, Hobart, TAS, Australia
- 3School of Horticulture, Anhui Agricultural University, Hefei, China
- 4Department of Field Crop, Faculty of Agriculture, Dicle University, Diyarbakir, Turkey
- 5Department of Botany, University of Narowal, Narowal, Pakistan
High plant density is considered a proficient approach to increase maize production in countries with limited agricultural land; however, this creates a high risk of stem lodging and kernel abortion by reducing the ratio of biomass to the development of the stem and ear. Stem lodging and kernel abortion are major constraints in maize yield production for high plant density cropping; therefore, it is very important to overcome stem lodging and kernel abortion in maize. In this review, we discuss various morphophysiological and genetic characteristics of maize that may reduce the risk of stem lodging and kernel abortion, with a focus on carbohydrate metabolism and partitioning in maize. These characteristics illustrate a strong relationship between stem lodging resistance and kernel abortion. Previous studies have focused on targeting lignin and cellulose accumulation to improve lodging resistance. Nonetheless, a critical analysis of the literature showed that considering sugar metabolism and examining its effects on lodging resistance and kernel abortion in maize may provide considerable results to improve maize productivity. A constructive summary of management approaches that could be used to efficiently control the effects of stem lodging and kernel abortion is also included. The preferred management choice is based on the genotype of maize; nevertheless, various genetic and physiological approaches can control stem lodging and kernel abortion. However, plant growth regulators and nutrient application can also help reduce the risk for stem lodging and kernel abortion in maize.
Introduction
Maize is one of the most widely grown cereal crops and is important for human food, animal feed, industrial raw materials, and biofuel energy (Shiferaw et al., 2011; Anjum et al., 2017). The world population is increasing and is projected to surpass 9.8 billion by 2050 (FAO, 2012). Thus, the crop production must be increased by 50% to the current production level by 2050, to meet food demand of the burgeoning human population (Searchinger et al., 2019; Tanveer, 2020). How to increase maize yield has been a key research question for agronomists for many years (Aslam et al., 2015). The use of high-yield cultivars or hybrid varieties, along with efficient crop husbandry practices, contributes to the increase in maize production; however, there are some inevitable factors, such as climate change, that are still limiting the achievement of maximum yield potential of maize worldwide (Xiao et al., 2008; Martins et al., 2019; Hussen, 2020).
Managing plant density (PD) at the field level is one of the most effective practices and plays a key role in increasing maize yield per unit area (Maddonni and Otegui, 2006; Dhaliwal and Williams, 2020). A low PD results in lower yield production because of smaller number of productive plants per unit area and greater weed infestation (Sharifi et al., 2009; Lashkari et al., 2011; Zhang et al., 2016; Zhao et al., 2019). The use of high PD may provide a high yield (Tokatlidis et al., 2011; Van Ittersum and Cassman, 2013) due to increased leaf area index (LAI) and photosynthetically active radiation (Maddonni and Otegui, 2004; Novacek et al., 2013), and improved dry matter and nitrogen accumulation (Ciampitti and Vyn, 2011; Yan et al., 2017). Conflicting reports have indicated negative effects of high PD, such as mutual shading, intraspecific competition for resources, accelerated leaf senescence, and reduced photosynthesis (Edmeades et al., 2000; Sharifi et al., 2009; Li H. et al., 2010; Li S. K. et al., 2010; Antonietta et al., 2014). In maize, high PD decreased cob length, ear weight, the number of kernels per row, and stalk area by 10.8, 6, 10, and 20% (Testa et al., 2016). Therefore, proper management is required to optimize PD to increase the yield per unit area.
High PD also results in two major problems, stem lodging and kernel abortion, which are casually linked with each other in the context of maize yield. Under intensive crop management, high PD can cause plants to be more susceptible to lodging, which is mainly due to increased stem length and decreased diameter and wall thickness that could diminish flexural rigidity, breaking resistance, and Young's modulus (Wu et al., 2012; Wu and Ma, 2018). High PD increases plant height through greater internode length and smaller stem diameter, promoting the chances of stem lodging further (Wang et al., 2011; Shah et al., 2017). High PD increases competition for nutrients between individual plants, which results in thinner maize stems and a higher risk of lodging (Huang, 2008; Feng et al., 2010; Shah et al., 2017). Moreover, increasing PD also results in reduced quality and intensity of light reaching the maize canopy (Tokatlidis et al., 2011), thus inhibiting the photo-destruction of auxin. More auxin increases the rate of internode elongation, causing the length of internodes to increase (Chen et al., 2018). In addition, plant density influences the quality of light (ratio of red to far-red) during the early growing season and impacts internode length in the lower canopy (Rajcan et al., 2004). Xue et al. (2016a) reported that high PD increases the rate of rapid internode elongation and decreases the duration of rapid internode thickening, causing internodes to increase in length and decrease in diameter. Therefore, as plant density increases, the length of the basal internode significantly increases, whereas the diameter significantly decreases (Novacek et al., 2013). The length of internodes below the ear increases as plant density increases, causing ear and plant height to increase. Elevated ear height increases the center of gravity (Xu et al., 2017). These changes make maize plants more susceptible to bending by the wind. Moreover, under high PD, reduction in mechanical strength and alteration in sugar metabolism also induces stem lodging (Shah et al., 2017; Kamran et al., 2018a).
Kernel abortion is another negative impact of increasing PD in maize, as high PD induces the abortion of young kernels by reducing the ratio of ear growth rate to tassel growth rate (Vega et al., 2001a,b; Sangoi et al., 2002). This reduction favors ear and kernel abortion (Cárcova and Otegui, 2001; Vega et al., 2001a,b). Moreover, high PD increases leaf formation and plant-to-plant competition, accelerates leaf aging, and reduces photosynthesis and the net assimilation rate of individual plants because of decreased carbon and nitrogen supply to the ear, thereby causing kernel abortion (Edmeades et al., 2000; Hiyane et al., 2010; Yan et al., 2010; Antonietta et al., 2014). Under high PD, there is a series of consequences that are detrimental to ear ontogeny and results in barrenness. First, ear differentiation is delayed in relation to tassel differentiation (Sangoi, 2001). Later-initiated ear shoots have a reduced growth rate, resulting in fewer spikelet primordia transforming into functional florets by the time of flowering (Sangoi et al., 2002). Functional florets extrude silks slowly, decreasing the number of fertilized spikelets because of lack of synchrony between anthesis and silking (Sangoi, 2001; Testa et al., 2016). Thus, the risk of kernel abortion increases under high PD because of increased ear height and the development of stems with reduced diameters (Stanger and Lauer, 2007; Novacek et al., 2013). Therefore, kernel abortion and stem lodging in maize under high planting density were discussed in this review.
Regardless of high PD, stem lodging also causes kernel abortion, and at a high PD, it is very difficult to identify the key player that causes kernel abortion. Some preliminary studies indicated that the first week of pollination is crucial for seed set in maize, and the continuous availability of sucrose for the development of seeds is necessary during and after pollination. If stem bending/breakage occurs in dense maize, which may cause an inadequate supply of sucrose, the abortion of kernels could result, which may range from 20 to 50% (Zinselmeier et al., 1999; McLaughlin and Boyer, 2004; Hiyane et al., 2010). It is noticed that stem lodging in maize effects yields most when it occurs at the reproductive stage (Li et al., 2015), as lodging resulted in stalk breakage, which causes a reduction in nutrient flow for developing grains, inducing kernel abortion (Xue et al., 2020). To improve crop yield and ensure food security, we need to eradicate factors that cause complete crop failure. Along with other factors, kernel abortion is significantly noticed along with stem lodging, making stem lodging a prominent factor that needs to be addressed. This phenomenon has not been discussed before; therefore, in this review, we elucidated the link between stem lodging and kernel abortion in maize under high planting density. This study also reviewed recent knowledge of plant characteristics that are important for improving the resistance to stem lodging and kernel abortion (Figure 1). We also discussed further physiological, genetic, agronomic, and management approaches to reduce maize stem lodging and kernel abortion.
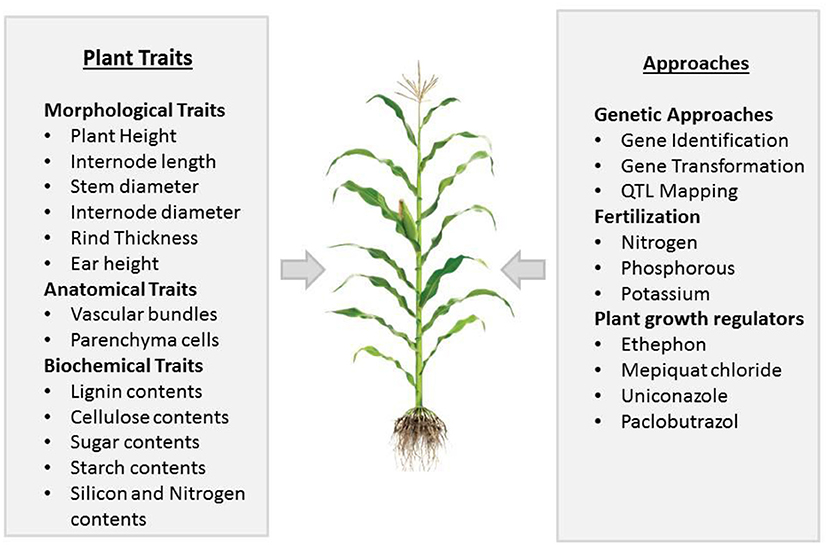
Figure 1. Summary of different plant traits and approaches important in affecting stem lodging and kernel abortion in maize.
Grain Yield Loss Caused by Stem Lodging and Kernel Abortion
Stem lodging significantly reduces maize yield by up to 75% (Cheng et al., 2011; Wen et al., 2019); however, yield reduction caused by lodging depends on its timing and the stage of maize growth (Li et al., 2015). Lodging during the 12th leaf and grain-filling stages can reduce maize yield by 30–38 and 45–48%, respectively (Li et al., 2015; Jun et al., 2017). In addition to grain loss, lodging increases harvest cost and reduces grain quality (Huang et al., 2015; Jun et al., 2017; Xue et al., 2018). Lodging-induced yield reduction is significantly associated with reduced carbon assimilation and mineral translocation during the grain-filling stage, and improved respiration and chlorosis, i.e., loss of chlorophyll content and greater vulnerability to pests and diseases (Zhu et al., 2006; Foulkes et al., 2011; Shah et al., 2017). Stem lodging at the reproductive stage is more detrimental than during the vegetative stage because at an early development stage, the logged stem can be re-erected, whereas the stem cannot be re-erected during the anthesis/grain filling stage after lodging, resulting in greater yield reduction (Berry et al., 2000, 2004; Piñera-Chavez et al., 2016; Jun et al., 2017; Shah et al., 2017).
Kernel setting is associated with a source-sink relationship, which is an important determinant of maize yield (Borrás and Otegui, 2001; Borrás et al., 2003; Borrás and Gambín, 2010; Yu et al., 2015). Kernel abortion can account for 8–12% yield loss during the dry season (Cheng and Lur, 1996). During pollination, any biotic or abiotic stress exacerbates the abortion of kernels, which may reduce the number of final kernels and final yield by up to 95% (Rattalino et al., 2011; Novacek et al., 2013; Testa et al., 2016).
Physiological Regulation of Stem Lodging and Kernel Abortion Under High PD
Morphophysiological Traits
Plant morphological and physiological traits play important roles in determining stem lodging and kernel abortion under high PD. Stem lodging is one of the most severe constraints on the use of high PD in maize (Argenta et al., 2001).
The major and most important morphological feature associated with stem lodging and kernel abortion under high PD is plant height (Yan et al., 2010; Song et al., 2016; Sher et al., 2018). Tall cultivars may be more susceptible to lodging than shorter plant cultivars, which are more resistant to lodging stress (Li et al., 2011). Decreased plant height results in higher lodging resistance because of lower center of gravity and reduced fresh weight, which minimize the risks of lodging (Ransom, 2005; Echezona, 2007). Additionally, plants with lower height also have a small dry matter, and this decreases grain yield. Therefore, maize cultivars with high resistance to stalk lodging should have a lower ear position to decrease center of gravity, larger leaf spacing, and smaller leaf angle above the ear to allow for more light transport to the mid and lower canopy. This plant type has increased stalk lodging resistance and decreased kernel abortion.
Rind thickness is another morphological feature associated with stem lodging. In various studies, rind thickness, internode diameter, and internode length have been used as predictors of stem strength in sorghum (Teetor et al., 2017). Under high PD, rind strength decreased, as evidenced by a decrease in rind penetrometer resistance, which resulted in smaller diameters and weaker stems that broke easier (Stanger and Lauer, 2007). This was also caused by a decrease in the mechanical tensile strength of maize stems under high PD, which resulted in plant lodging and reduced both the yield and quality of maize (Fu et al., 2013). Additionally, positive correlations have been observed among plant height, stem diameter, internodal length, numbers, and lodging index, and it has been concluded that these traits are substantial plant characteristics that influence the vulnerability of plants to lodging (Table 1).
The leaf sheath that surrounds and protects the hollow internodes of a stem also provides plants with great physical support (Hale et al., 2021). In a study, it was found that, on average, the leaf sheath contributed 40, 68, and 38% of the overall stem bending strength, flexural rigidity, and safety factor, respectively, in oat, while it accounted for 11, 24, and 10%, respectively, in wheat plants (Wu and Ma, 2020). Any damage to leaf sheaths may result in the weakening of stem breaking resistance in plants (Wu et al., 2012). Moreover, the leaf sheath of rice varieties showing low stem breaking strength generally died down earlier than varieties having high stem breaking strength (Ookawa and Ishihara, 1992). These studies emphasized the importance of maintaining the vitality of leaf sheath for enhancing stem bending strength.
Leaf angle plays a vital role in the determining the amount of light intercepted by the canopy, and in maize leaves with different leaf angles receive different qualities of light (particularly enriched with far-red, FR) and reduced red (R) radiation under high PD (Lee and Tollenaar, 2007; Hammer et al., 2009). An erect leaf posture improves lodging resistance, and a prostrate leaf stature reduces resistance to lodging under dense planting (Wu and Ma, 2019). A high FR/R ratio triggers many morphophysiological changes in plant architecture, stimulating stem elongation, and favors apical dominance and decrease in stem diameter (Rajcan and Swanton, 2001). Nonetheless, stem lodging and kernel abortion increased, and stem diameter decreased because of mutual shading (Troyer and Rosenbrook, 1983; Valentinuz and Tollenaar, 2004). Such changes make maize stems more susceptible to falls and breakage before kernels reach physiological maturity (Li et al., 2011).
Anatomical and Biochemical Traits
The anatomical characteristics of plants have a significant effect on lodging and kernel abortion under high PD. Stem lodging occurs under high PD when plant-to-plant competition for light, nutrients, water, and carbohydrates increases between the stem (source) and the ear (sink) within the plant, ultimately reducing the vigor of the sclerenchyma cells around the vascular bundles in the stem (Nielsen, 2006; Stanger and Lauer, 2007).
To reduce lodging potential and ear falls, plants rely on their anatomical features to provide shape and strength, bond cells together, and provide rigidity for the whole plant (Wen et al., 2019). However, the development of anatomical features varies significantly with cells, species, and accessions within species (Brett and Waldron, 1990; Hazen et al., 2003). Pith parenchyma cells play a vital role in stabilizing the stem and reducing the risk of local buckling and collapse (Niklas, 1991; Spatz et al., 1993; Kong et al., 2013). Stem stand ability increases with the thickness of the parenchyma layer because parenchyma cells can absorb the effects of environmental forces, such as light, wind, and rain without heating or mechanical damage (Kokubo et al., 1989). About 50–80% of the strength of a maize stalk comes from its outer structure, the rind (Zuber et al., 1980). Wang T. et al. (2015) reported that the crush strength of maize stalks is significantly positively correlated with the ratio of mechanical tissue thickness to internode radius and the ratio of sclerenchyma thickness to internode radius. Xue et al. (2016a) reported that the number of mechanical cell layers, the thickness of mechanical tissue, and the ratio of cortex thickness to internode radius determine ~79% of the Rind penetration strength (RPS) at the third internode. The mechanical strength of maize stalks depends primarily on the cell wall of mechanical tissues in the internode rind (Leroux, 2012). The main components of the cell wall are structural carbohydrates, such as cellulose, hemicellulose, and lignin (Li S. C. et al., 2003; Li Y. et al., 2003; Wang et al., 2006), and, therefore, stalk strength is significantly and positively related to the contents of these materials (Appenzeller et al., 2004; Chen et al., 2007). Sclerenchyma cells around the vascular bundles in the stem are responsible for mechanical strength, and reduction in sugar supplies can reduce the vigor of sclerenchyma cells, inducing stem lodging (Novacek et al., 2013).
In addition to the anatomical characteristics of maize plants, some basic biochemical properties, such as cellulose, hemicellulose, lignin, and soluble sugar content, are essential and have a significant effect on lodging resistance under high PD. When PD increases, it affects the vigor of sclerenchyma cells around vascular bundles and may reduce the synthesis of total non-structural carbohydrates and proteins, and potassium level in the stem, possibly causing stem lodging by altering the source-sink ratio (Wang et al., 2006). Furthermore, decreased stem protein and sugar levels cause senescence of pith tissue and increase stem lodging (Shah et al., 2017). In another study, Wang and Hu (1991) found that lodging-resistant maize varieties exhibited a higher accumulation of carbohydrates and lignin in the stems than susceptible varieties. A non-significant relationship was also observed between the starch content of the stem and lodging resistance (Zhang et al., 2009). Moreover, the accumulation of cellulose, hemicelluloses, and lignin improved the thickening and flexibility of the culm wall (Jones et al., 2001; Tanaka et al., 2003). Typically, stem strength depends on cellulose and lignin content; therefore, plant stems with lower lignin or cellulose levels are susceptible (Shah et al., 2017).
Lignin is one of the main components of and confers rigidity to cell walls; therefore, it is associated with the mechanical stability of plants (Loor et al., 2013). Low lignin content may result in weak mechanical strength of the cell wall and could easily cause stem lodging. Lignification of the cell wall could improve the stability of the cell wall and increase the physical strength of the stem (Chen et al., 2011). Therefore, lignin is an integral component of plant health and function (Chen et al., 2011). The application of silicon and nitrogen could considerably increase the lignin content in hardened cells and increase cellulose content, thereby reducing stem lodging index (Zhang et al., 2010; Chen et al., 2011).
Multiple reasons are involved in kernel abortion, which may be due to failure in pollination or defective ovary, and even abortion is noticed after successful fertilization (Gustin et al., 2018). Under high PD or under a lodging situation, when sources are deficient, plants are used to abort few of the ovaries as a survival tactic (Ruan, 2014; Tardieu et al., 2014). Reduction in the supply of sucrose and concentrations of assimilates causes kernel abortion (Shen et al., 2018). Many studies have discussed that under scarce sucrose conditions, apical kernels are more likely to abort as they have the weakest sink (Shen et al., 2018).
When it comes to the anatomy of female florescence, the kernel at the base of the ear is less likely to abort because even in the most competitive environment they have more photo assimilate supply as compared with the kernels at the terminal parts, which are more likely to abort. The same is the case under water deficit conditions, increasing PD under water deficit conditions increased the risk of kernel abortion for the kernels at the tip of ears (Setter et al., 2001; Setter and Parra, 2010). It is noted that the expression of the TPP gene (trehalose phosphate phosphatase) in the development stage of the ear reduces kernel abortion (Nuccio et al., 2015). Varieties with the TPP transgenes have comparatively lower kernel abortion in high planting density (Hannah et al., 2017). To date, little is known about the molecular mechanism that controls kernel abortion; therefore, there is a need for more studies that are focused on uncovering the exact mechanism of kernel abortion and its reasons. Based on previous studies, there is a hypothesis, on which current studies are relying, that inadequate supplies of carbohydrate and water in the development stage of kernel cause a reduction in kernel set (Gustin et al., 2018) under high PD. The other hypothesis is that reduction in supplies of water causes a reduction in cell expansion, which causes kernels to abort (Oury et al., 2016). The above-mentioned findings have important implications for future maize breeding.
In conclusion, plant characteristics play different roles in controlling the risk of lodging and kernel abortion in maize. These characteristics should be considered in future studies to develop lodging-resistant varieties and reduce kernel abortion.
Role of Stem Sugars in Lodging Resistance and Kernel Abortion
Stem lodging is a significant constraint to the yield of maize at high PD; even high-yielding hybrids can be affected by it (Betra'n et al., 2003; Flint-Garcia et al., 2003). As stated above, stem lodging significantly depends on the distribution of structural chemical constituents of stems (Appenzeller et al., 2004; Chen et al., 2007). Additionally, the roles of structural and nonstructural carbohydrate and sugar contents in lodging resistance and kernel abortion are largely unknown. In this study, we attempted to summarize how sugar content in shoots relates to lodging resistance and kernel abortion, and the mechanisms involved in sugar biosynthesis, transport, and deposition. Sucrose phosphate synthase (SPS) and sucrose synthase genes (SUS) play a role in the accumulation of sugars in stems, and effectively minimize stem lodging and kernel abortion (Zinselmeier et al., 1999; Slewinski, 2012; Mizuno et al., 2018).
Two plausible possibilities are given here to elucidate the co-relationship of stem sugars and lodging. The first is that high sucrose content will require more water in parenchyma cells, thereby increasing cell turgor pressure and creating stiffer cells, thereby combating lodging. The second possibility is the presence of higher concentrations of sugar in the vicinity of the cell, which could facilitate carbohydrate mobilization and help the cell to maintain its integrity, which restricts necrosis that degrades dead cells and tissues and, consequently, compromises stem structure. However, sugar accumulation (controlled by SUS genes) delays senescence until the end of the season and increases resistance to stem lodging; thus, it minimizes kernel abortion and prolongs grain development (Thomas and Howarth, 2000).
Non-structural carbohydrates play a significant role in stem lodging tolerance and kernel abortion. When photosynthesis is at its peak, non-structural carbohydrates are stored in the parenchyma cells and vascular tissues, adding to the physical strength of the stem, thereby improving plant lodging tolerance. During later stages, when photosynthesis is compromised, stored non-structural carbohydrates act as secondary sources for grain filling, significantly reducing kernel abortion (Slewinski, 2012). Sustaining non-structural carbohydrates in the stem has been proposed as an effective way to control stem lodging (Shiferaw et al., 2011). It can be concluded that cultivars with high stem sugar deposits are more likely to resist lodging.
Kernel abortion is mainly caused by an insufficient carbohydrate supply (source-sink relationship; Shen et al., 2020). In the source-sink relationship, the stem buffer system is mainly dependent on sucrose (Daynard et al., 1969). Sugar transportation is regulated by invertase genes and is always noted from the source to sink organs. Initially, sugar is biosynthesized in the leaf by the action of SPS genes used for development, and any surplus is placed in the storage organs by the activity of the SUS genes. The stem in the vegetative growth stage acts as the storage organ (sink), but in later stages, during reproductive growth when sugar is needed for kernel development, the sugar content in the stem tissues begins to act as a source, and a flow from stem to kernel occurs (Slewinski, 2012). Therefore, stem sugars can maintain the supply of sugar for kernel development and can play a role as a limiting factor for kernel abortion. However, the role of stem sugars in important metabolic processes has been neglected in the past, and only recently has its roles in lodging resistance, kernel abortion, and other key metabolic processes been addressed (Saini and Westgate, 1999; McLaughlin and Boyer, 2004; Hiyane et al., 2010).
Sucrose in storage organs displays an apoplasmic movement, and its depletion can affect the total sugar content and total stem dry matter (Sayre et al., 1931; Setter and Flannigan, 1986; Slewinski, 2012). Thus, sugar partitioning is also important in relation to lodging resistance and kernel abortion. ZmSUT1, a member of the sucrose transporter family, is highly expressed in photosynthetic tissues and is responsible for the mobilization of carbohydrates from source to sink tissues (Carpaneto et al., 2005; Slewinski and Braun, 2010). Braun and Slewinski (2009) suggested that characterization of other family member genes could further clarify the roles of these genes in sugar mobilization, and is discussed in detail in later sections of this article. It will be interesting to discover the involvement of sucrose transporter SUT genes in regulating non-structural carbohydrate (NSC) reserves in maize.
In maize, the fertility of the ovaries has a more significant influence on kernel number than that of pollen, which is different from that of wheat, rice, and barley (Boyer and McLaughlin, 2007; Barnabás et al., 2008). During reproductive growth, ovaries require sugar in the form of sucrose for developing embryos, and a reduction in sugar supply results in the abortion of the embryos, resulting in aborted kernels (McLaughlin and Boyer, 2004; Hiyane et al., 2010). Therefore, sugar accumulation should be properly managed to ensure lodging resistance and minimal kernel abortion, because all these factors have an impact on the final yield. This must be understood for an in-depth elucidation of sugar metabolism and transport. A summary of stem sugar storage and translocation in overcoming stem lodging and kernel abortion is shown in Figure 2.
Sugar Biosynthesis and Metabolism
Sugar biosynthesis and metabolism are complex processes affected by different growth and environmental conditions. Briefly, as a result of photosynthesis, sugar is synthesized in the form of sucrose by the catalytic activity of sucrose-phosphate synthase (SPS) and converted into ADP-glucose or UDP-glucose, which is used in plant metabolism by the action of the SuSy enzyme (Hendriks et al., 2003; Kolbe et al., 2005). The SuSy enzyme is encoded by SUS genes that may be present in the cytoplasm, cell wall, and vacuoles (Stein and Granot, 2019). Plants with low activity of SUS genes exhibited stunted growth, whereas the overexpression of SUS genes produced significantly increased growth and strong cell structure with thickened cell walls (Stein and Granot, 2019; Figure 3). At the cellular level, the intercellular biosynthesis of sugar and starch is initiated by ADP-glucose pyrophosphosphorylase (AGPase), which provides ADP-glucose (Geigenberger, 2011). AGPases are primarily encoded in the cytosol and are minimally encoded in amyloplasts and plastids (Burton et al., 2002).
After biosynthesis, sugars reach the sink tissues via the phloem tubes either through the symplast or apoplast pathway. In the apoplasmic unloading pathway, sucrose is unloaded into the cell wall matrix from the sieve element/companion cell (SE/CC) complex mediated by sugars will eventually be exported transporter (SWEET) proteins, which are typically localized on the plasma membrane, to transport sucrose or hexoses down a concentration gradient in an energy-independent manner (Shen et al., 2019). Within the cell wall, sucrose is often hydrolyzed by cell wall invertase (CWIN; with an optimum pH of 5–6) into glucose and fructose (Ruan et al., 2010). Hexoses are then taken up by H+-coupled hexose transporters (HXTs; Ruan, 2014). It is common for CWIN and HXTs to be co-expressed in the apoplasmic unloading region, indicating a synergistic functional relationship between CWIN and HXTs (Weber et al., 2005; Jin et al., 2009; McCurdy et al., 2010; Shen et al., 2019). The direct transfer of sucrose can also occur through plasmodesmata, where vacuolar invertase genes hydrolyze sucrose. SuSy and invertases cleave sucrose differently. SuSy proteins cleave sucrose into reversible UDP-glucose and ADP-glucose, whereas cell wall and vacuolar invertases catalyze an irreversible conversion of sucrose into glucose and fructose (Ma et al., 2019).
Additional sugars are transported to the sink tissues, which are the stem and roots in the case of maize, to be stored and added to the organic matter structural carbohydrates (Ruan, 2014; Stein and Granot, 2019). In maize, the involvement of SUS genes in starch synthesis was first studied by Chourey and Nelson (1976) who found that gene mutants exhibited a 90% reduction in SuSy and an overall 40% reduction in starch accumulation. However, in mutants with overexpressed SUS genes, a considerable increase in the accumulation of ADP-glucose was observed (Li et al., 2013). SUS genes appear to play a role in the development of young photosynthetic tissues and starch accumulation in the non-photosynthetic tissues of the plant (Hänggi and Fleming, 2001). Additionally, SUS genes were localized in the xylem tissue, and their overexpression resulted in increase in xylem cell wall thickness (Coleman et al., 2006; Bahaji et al., 2014; Wei et al., 2015). Hence, SuSy is responsible for directing carbon to cellulose synthesis, which is used in the development of xylem tissues (Barratt et al., 2009).
This raises the question regarding whether these genes protect plants from lodging and kernel abortion. Four cell wall invertase genes, two vacuolar invertase genes, and 21 invertase isogenes are identified in maize (Juarez-Colunga et al., 2018). Grain yield relies mainly on grain number, size, and starch content (Ngoune Tandzi and Mutengwa, 2020). Maize mutants with higher expression of cell wall invertase resulted in a 1.5-fold increase in production, a 20% increase in starch content, and a noticeable increase in the size of ears, grain size, and numbers, which relates invertase genes with resistance to lodging and kernel abortion. Higher activity of cell wall invertase results in increased shoot strength and sugar content, along with a significant increase in seed number and size (Li et al., 2013). It has also been documented that invertases regulate the production of other plant hormones, such as indole acetic acid (IAA), which directly affects kernel development (Chourey et al., 2010). The expression of genes involved in starch biosynthesis and accumulation is controlled by the number of transcription factors; ZmNAC128 and ZmNAC130 transcription factors are involved in sugar accumulation and ZmEREB156 is involved in the regulation of key genes involved in sugar biosynthesis (Huang et al., 2016; Zhang et al., 2019). From these studies, it is inferred that invertase genes can improve lodging resistance in plants.
Genes Involved in Sugar Transport
Photosynthetic tissues are the powerhouses of plant sugars that are biosynthesized into different forms. Later, they are translocated to other organs, accumulated, and used in different development and reproduction processes. Processes involved in the translocation have been well-discussed, but little is known about the genes involved in sugar translocation and accumulation. The carbohydrate partitioning defective1 (Cpd1) gene plays a role in the early production of phloem and controls the distribution of carbohydrates throughout the plant (Julius et al., 2018). In the case of low expression of cpd1, plants are unable to transport sugars from the source to sink tissues, which results in the deposition of sugars in leaves and reduction in sink tissues (stems and roots) causing stunted growth, decreased sturdiness of the stem, and delayed silking and anthesis, which affect kernel size and weight (Julius et al., 2018). Agpsemzm and Agpllzm enzymes play roles in the regulation of starch levels in different tissues (Julius et al., 2018).
In addition to the phloem sieve tissues, other genetic factors are involved in the adequate distribution of carbohydrates throughout plants. Initially, sugars are uploaded to cell walls by the SWEET efflux proteins from source tissue cells, and then to sieve elements and companion cell complexes by sugar transporters (Julius et al., 2017). Sucrose moves passively from high-concentration tissues to low-concentration tissues; sucrose transferase (Sut) genes are responsible for the regulation of this symplasmic and apoplasmic movement of sucrose (Aoki et al., 1999, 2003; Zhang C. et al., 2014). Maize has apoplasmic sucrose movement null mutants for the ZmSut1 gene, which identifies its function as a phloem loading with sucrose from source cell apoplastic regions. Retardation of the ZmSut1 gene results in slow growth and deposition of sucrose in maize leaves (Slewinski et al., 2009; Slewinski and Braun, 2010).
For the identification of ZmSut2 gene functions, null mutants were analyzed, and showed a reduction in growth and decreased ear length and kernel number, indicating that ZmSut2 is responsible for resistance to kernel abortion and contributes to maintaining yields under high-density planting (Leach et al., 2017). Sucrose transporter genes also directly influence maize growth (Leach et al., 2017). Along with the ZmSut genes, ZmSWEET13s (a, b, and c) genes are involved in the translocation from the sink to source tissues, and knockout mutants showed low expression of genes involved in photosynthesis and carbohydrate metabolism (Bezrutczyk et al., 2018).
As the kernel is the main determinant of crop yield, the genetic factors involved in the transportation of sugars and their accumulation need to be elucidated. ZmSWEET4c has been identified in maize and is responsible for the transport and deposition of sugars across the basal endosperm transfer layer, which controls nutrient flow in and out of seeds (Sosso et al., 2015). In wild-type maize, the expression of ZmSWEET4c increases four times that of normal, along with the flow of maximum sugar to developing seeds, thereby supporting seed setting (Chourey et al., 2012). Along with ZmSWEET4c, another gene involved in sugar transport is ZmMn1. The expression of these genes induces the expression of ZmMRP1, which prepares the cell machinery for sugar transport (Sosso et al., 2015).
Linking Stem Lodging With Kernel Abortion Under High PD in Maize
A high PD can increase biomass and yield, but it also increases competition for nutrients and light interception among individual plants (Craine and Dybzinski, 2013). Moreover, plant morphogenesis, stem carbohydrate accumulation, cortex tissue, and mechanical strength are affected by high PD. Thus, maize plants under high PD are more vulnerable to stem lodging (Xue et al., 2016a; Jun et al., 2017). High PD promoted the abortion of kernels before the beginning of grain filling in maize (Sangoi, 2001; Ruffo et al., 2015). Abortion causes permanent losses in seed production and may result in decreased productivity (Salter and Goode, 1967; Hiyane et al., 2010). Floral abortion in maize disrupts carpel development (Hiyane et al., 2010). During the flowering stage, the first week of pollination is crucial for the seed set, during and after pollination. The continuous availability of sucrose for the development of seeds is necessary where an inadequate supply of sucrose results in the abortion of kernels in maize (McLaughlin and Boyer, 2004). Kernel abortion is associated with inhibited photosynthesis and reduced sugar supply to the stems under shade (McLaughlin and Boyer, 2004).
Additionally, under high PD, less interception of light decreases the synthesis of cellulose and lignin in maize, which may cause lodging (Li et al., 2008; Xue et al., 2016b). Stem lodging reduces photosynthesis (Setter et al., 1997; Ma et al., 2014) because of reduced photosynthetic activity, which ultimately reduces the sugar flow in plant storage tissues for metabolic processes. This results in a reduced supply of sucrose to developing kernels, which induces abortion (McLaughlin and Boyer, 2004; Hiyane et al., 2010). However, attack by corn borers increases the occurrence of lodging, reducing CO2 assimilation, biomass accumulation, and carbohydrate partitioning, resulting in yield reduction from water disruption and nutrient translocation to the ear, and pre-harvest losses because of stem lodging and dropped ears (Riedell and Reese, 1999; Steffey and Gray, 2002; Rice, 2006).
Under high PD or lodging situations, reduction in the supply of sucrose and concentrations of assimilates causes kernel abortion (Shen et al., 2018). Many studies have discussed that under scarce sucrose conditions, apical kernels are more likely to abort, as they have the weakest sink (Shen et al., 2018). Kernel abortion can be minimized by adequate supplies of N, organic, and inorganic substances, and improving carbohydrate availability during the pollination stage (Paponov et al., 2020). At high PD, many kernels may not develop an event that occurs in some hybrids following poor pollination resulting from a silking period that is delayed relative to tassel emergence (Otegui, 1997) and/or owing to a limitation in assimilate supply that causes the grain and cob abortion in maize (1985). As stated above, there is no direct relationship between high PD and stem lodging and kernel abortion is mentioned in the literature. However, we attempted to draw a causal link between stem lodging and kernel abortion under high PD. This link needs to be validated in future studies.
Management of Stem Lodging and Kernel Abortion in Maize
The Physiological and Genetic Approach
Genes Involved in Lodging Resistance and Kernel Abortion Reduction
Several studies have shown that various genes are involved in lodging resistance and kernel abortion reduction (Table 2). In cereals, stem diameter, rind penetrometer resistance, and stalk bending strength are determinants of stem lodging (Shah et al., 2017). In more than 250 inbred lines, a genome-wide association study was designed to identify the nucleotides involved in these quantitative traits. A total of 423 QTNs was identified in maize, in addition to 63 cyclin-dependent kinase coding and steroid-binding genes that were identified. Seven of these were classified as transcription factors. These genes are likely involved in cell elongation, stem girth improvement, and development, hence, adding up resistance to lodging; 17 genes for stem stalk bending, 19 for strength/diameter, and 30 for rind penetrometer resistance were identified (Hu et al., 2013; Dante et al., 2014; Zhang et al., 2018).
Other than these factors, lignin is also an evident factor that determines resistance to lodging (Frei, 2013). The maize miR528 family (miR528a and miR528b) regulates the expression of lignin synthesis genes ZmLAC3 and ZmLAC5 in an abundant nitrogen environment, and these genes are highly expressed in the internodes and are responsible for lignin deposition in the maize stem (Sun et al., 2018). Knocking down miR528 results in higher expression of ZmLAC3 and LAC5, which results in higher concentrations of lignin more resistant to lodging (Sun et al., 2018). Somssich (2020) identified the LAC10, PRX42, PRX72, PRX52, and PRX71 proteins as responsible for lignification in stems.
QTLs Involved in Lodging and Kernel Abortion Resistance
Marker-assisted quantitative trait loci (QTLs) are one of the approaches that can be used for the identification of genes and to interrelate qualitative, quantitative, chemical, physiological, and morphological traits. In maize, 22 QTLs for lignin, 2 for starch content, 7 for hemicellulose, and 11 for cellulose were identified, which increased lodging resistance and reduced kernel abortion (Santiago et al., 2016). One QTL for stem sugars was identified to match the SWEET4-3 putative gene locus in maize (Mizuno et al., 2016). Under high PD, plants encounter 43 different QTLs for six different traits: plant height, ear height, reduced stem diameter, delayed days to tassel, delayed days to silk, and anthesis-silking interval (Yuan et al., 2012).
Different QTLs can be identified using a marker-assisted approach. QTLs for lodging resistance mostly overlap with QTLs for stem strength, which reveals factors responsible for stem strength directly related to lodging resistance (Table 3). QTLs were found on chromosomes 2, 4–8, 9, and 10, which are accountable for root lodging. Additionally, 28 QTLs were found in maize with respect to kernel size and shape (Farkhari et al., 2013). Considering QTLs, their heritability, and other genetic factors responsible for quantitative traits and resistance to lodging can be useful for transforming the food chain and ensuring food security.
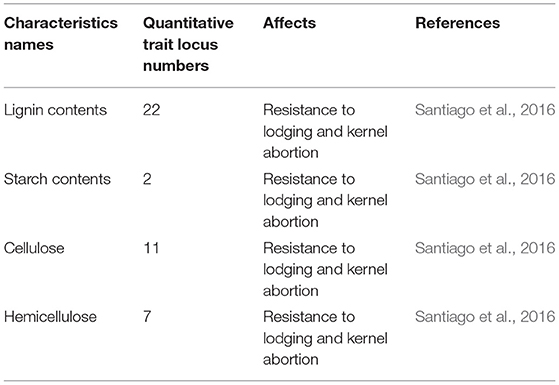
Table 3. Number of quantitative trait loci identified for stem lodging resistance and kernel abortion reduction in maize.
Agronomic Approaches
Planting Time and Method
Planting time influences lodging intensity and kernel abortion in maize (Angel et al., 2019; Zhang et al., 2019). Delays in planting time accelerates plant growth between seedling emergence and silking, which minimizes crop exposure to cumulative incident radiation during the vegetative process. Therefore, lodging may be reduced if crops are sown at the optimum time (Dahiya et al., 2018). However, the optimum planting time differs with the area and environmental conditions (Andrade et al., 1996; Bruns and Abbas, 2006). Similarly, planting time affects kernel abortion (Zhang et al., 2019), and both early and late plantings can cause kernel abortion and reduce the cumulative intercepted photosynthetically active radiation because of delayed leaf area development and high temperature (Otegui et al., 1995; Zhang et al., 2019). During early planting, the effective grain-filling duration is shortened, owing to the maximum daily high temperature from the silking to the blister stage. Similarly, the grain-filling of apical kernels is restrained, which leads to an increase in kernel abortion (Zhang et al., 2019). Contrarily, delayed planting resulted in significant reductions in final kernel number per unit area, and the number of ears at harvest (Cirilo and Andrade, 1994). Moreover, it was suggested that with delayed planting date, radiation and thermal time during the grain-filling period decreased, leading finally to decreased maize kernel weight (Cirilo and Andrade, 1994; Zhou et al., 2017). Li Y. et al. (2003) reported that with delay in sowing date at Shihezi, Xinjiang Uygur Autonomous region, China, grain-filling rate and final kernel weight decreased. Kernel abortion under late planting time is highly associated with less pollen availability, delayed anthesis and silking of individual plants, anthesis-silking interval, decreased number of exposed silks per apical ear, number of pollen grains per square meter, and kernel number per ear (Uribelarrea et al., 2002). Therefore, optimum planting time can improve maize productivity under relatively appropriate climate conditions and with the avoidance of abiotic stresses during the critical period of kernel formation and growth (Arnold and Monteith, 1974; McMaster and Wilhelm, 1997).
In addition to planting time, planting methods also influence the susceptibility of maize plants to lodge and kernel abort (Bakht et al., 2011; Deng et al., 2019). Different planting methods are practiced worldwide at the time of sowing maize crops (Arif et al., 2001; Bakht et al., 2011; Deng et al., 2019). Inappropriate planting methods can result in sterile plants. Ears and plant size remain small, and crops become susceptible to lodging, diseases, and pests, resulting in lower yield per unit area (Liu and Yong, 2008; Bakht et al., 2011). Abdullah et al. (2008) reported that the ridge planting method was better compared with other planting methods examined (bed and flat methods). The ridge planting method reduces lodging and provides good soil conditions for root development and efficient use of irrigation water and nutrients for proper development (Bakht et al., 2006, 2011; Liu and Yong, 2008). Heat stress during fertilization and during flowering significantly reduces pollen viability and seed yield (Edreira et al., 2011; Wu et al., 2020), while adopting an appropriate planting method may alleviate such high temperature-induced negative effect on pollen development. Tao et al. (2013) reported that the ridge planting method enhances the ability of maize to resist heat stress during the grain filling stage, which may reduce kernel abortion. Likewise, the adoption of ridge-furrow with plastic film mulching resulted in reduced lodging index as compared with the well-watered planting method (Li et al., 2020; Li and Li, 2021).
Fertilization
Increasing the planting density and applying N fertilizer are very effective agronomic strategies for raising the yield of modern maize cultivars, but high plant density and excessive N application have led to thinner and taller stalks and increased lodging risk (Li H. et al., 2010; Ciampitti and Vyn, 2012; Shah et al., 2017). Higher nitrogen doses can increase the elongation rate and length of the basal internode and significantly reduce the cellulose content of maize stems (Rajkumara, 2008), thereby decreasing stem strength and increasing lodging rate. Wei et al. (2008) stated that N also increases the development of the upper plant canopy, which decreases basal internode length and, consequently, increases stem lodging. Because of dense canopy development, low light conditions occur, which tend to cause plants to grow vertically, resulting in the development of long internodes and stems with small diameters, and less lignification. This can be managed by the split application of N, rather than high concentration. N application timing should also be considered. During reproductive stages, N application is discouraged to avoid kernel abortion (Bian et al., 2017). Wang et al. (2020) reported that nitrogen application in splits can improve stem lodging resistance of maize under high PD.
Lack of kernel development and enhanced abortion is caused by insufficient supply of carbon and nitrogen assimilates in the ear (Hammad et al., 2020). Nitrogen deficiency may cause kernel abortion, resulting in infertility (Marahatta, 2020). Plants grown under high N levels and high PD have decreased kernel numbers because the floret set is established before silking (Gonzalez et al., 2019; Mueller et al., 2019; Paponov et al., 2020). Thus, an adequate supply of N during the lag phase of the grain-filling stage may reduce kernel abortion (Below et al., 2000; Mueller et al., 2019; Paponov et al., 2020). N deficiency decreases the number of kernels per cob by decreasing maize kernel growth and development (Savin et al., 2006). Optimum N fertilization may increase kernel quantity and weight, resulting in higher crop growth rates, while N deficiency decreases photosynthate production in plants (Worku et al., 2012), consequently reducing the kernel-filling period. Nitrogen deficiency in maize during vegetative growth can cause early maturity (Sharifi and Namvar, 2016) and consequently reduce the kernel-filling period (Mayer et al., 2012). Increasing N availability stimulated ear growth during the bracketing-silking period and during the fast grain-filling phase, consequently resulting in greater maize grain yield (Ning et al., 2018). Moreover, in developing ears, N fertilization likely enhanced the cleavage of sucrose to glucose and fructose in the cob prior to and at silking and the synthesis from glucose and fructose to sucrose in the kernels after silking, thus increasing kernel setting and filling (Ning et al., 2018). Therefore, proper N fertilization can assist in managing kernel abortion and stem lodging in maize under high PD. However, more focus is required to study N application timing and rate, as higher N application is also not beneficial under high PD.
The role of potassium (K) is less evident; but to some extent, it contributes to lodging resistance. An inadequacy of K leads to reduced culm length, diameter, and wall thickness, and plants with inadequate K fertilization exhibit weaker culms than those with proper K fertilization (Mulder, 1954; Shah et al., 2017). K plays an important role in the physical strength of the plant and considerably reduces the lodging index and kernel abortion (Zhang et al., 2010). K can promote lignification in thick-walled cells, thicken collenchyma cells, and increase cellulose content, which reduces the lodging index (Shah et al., 2017). In other studies, the application of K during early stalk development and flowering stages increased structural carbohydrate accumulation and stem strength (Liebhardt and Murdock, 1965; Sun et al., 1989; Li et al., 2012). K deficiency in plants often results in the accumulation of sucrose in source leaves because of insufficient loading of the phloem (Zhao et al., 2001; Cakmak, 2005; Shahzad et al., 2017). K is the major cation in the phloem, and deficiency can lead to poor functioning, including phloem-disrupted metabolism and transport of assimilates, which induces kernel abortion (Shahzad et al., 2017). Epron et al. (2016) and Shahzad et al. (2017) reported that K foliar application may reduce kernel abortion, probably by affecting the phloem transport of assimilates. In another study, it was found that K application increased fertilization by adjusting the period between tasseling and silking, which resulted in a greater number of grain rows, grain cob−1, and produced higher grain weight cob−1 (Ur Rehman and Ishaque, 2011). Moreover, K, in combination with N, has a synergistic influence on the uptake, translocation, and utilization of nutrients, and it reduces the percentage of senescent stalks, lodging and increases crushing strength and rind thickness (Bukhsh et al., 2012).
Growth Regulator Application
Plant growth regulators are artificial chemical compounds used to decrease plant height and other lodging-related plant traits. Some regulators have recently been introduced to control maize lodging (Schluttenhofer et al., 2011). Plant growth regulators can optimize plant morphology and increase yield by regulating endogenous plant hormone signaling and metabolism (Naeem et al., 2012; Zeng et al., 2012). Different plant growth regulators have been applied to maize, such as ethephon (Shekoofa and Emam, 2008), mepiquat chloride (Kamran et al., 2018c), paclobutrazol (Kamran et al., 2020), and uniconazole (Schluttenhofer et al., 2011), in the context of lodging resistance and maize yield improvement; however, the effect on vegetative (and perhaps generative) plant growth is highly dependent on the time of application and dosage of plant growth regulator and probably varies with the maize cultivar used (Hütsch and Schubert, 2018).
Ethephon (2-chloroethyl phosphonic acid) is a plant growth regulator that inhibits stem elongation and promotes stem thickness, thereby improving plant morphological resistance to lodging (Li et al., 2019). Shekoofa and Emam (2008) reported that the application of ethephon is associated with reductions in plant height, leaf area index, and crop growth rate, decreasing lodging by 85–93%, resulting in better kernel filling but also slightly decreasing yield by 2–6% (Khosravi and Anderson, 1991). Yield reduction in maize increases with the application rate of ethephon (Earley and Slife, 1969). However, in another study, ethephon with diethyl aminoethyl hexanoate (DA-6) could offset the yield. Furthermore, the combination of ethephon and DA-6 shortened the length and increased the diameter of internodes below the ear position, improving lodging resistance and the yield of maize (Dong et al., 2006). Ethephon has been observed as a negative regulator of kernel development or grain yield maize because of its negative effects on leaf area development, crop growth rate, and photoassimilate reduction (Shekoofa and Emam, 2008; Gao et al., 2009). However, it has been shown that the application of low concentration of ethephon increases maize kernel yield under high PD (Gao et al., 2009). Given that, more physiological studies are required to explore the role of ethephon further in managing stem lodging and kernel abortion at the same time.
Mepiquat chloride is also a plant growth regulator that mainly reduces the length of internodes in dense plant populations and increases resistance to lodging (Kuai et al., 2015; Kamran et al., 2018b). Kamran et al. (2018b) stated that mepiquat chloride in dense maize populations reduces plant height and ear height and increases stem diameter and lignin content in basal nodes, which ultimately reduces kernel abortion and enhances lodging resistance. The ability of mepiquat chloride to reduce the percentage of lodging results in a more uniform canopy, which further improves grain yield at high density (Kamran et al., 2018b). Similarly, Zhang Q. et al. (2014); Zhang et al. (2017) reported that the application of growth regulators significantly increased the number of kernels per ear and maize yield by increasing the optimal plant density by reducing the lodging percentage.
Paclobutrazol is also a plant growth regulator that mainly reduces the length of the second internode, resulting in reduction in plant height and increased resistance to lodging (Peng et al., 2014). Furthermore, stem diameter, lodging resistance, lignin accumulation, and antioxidant activities were positively affected by its use. Additionally, several studies (Özmen et al., 2003; Dong et al., 2006; Cai et al., 2014) have shown that the canopy of plants is best established by its use, with a significant increase in photosynthetic activity and yield (Wang C. et al., 2015; Xu et al., 2017). Thus, the use of these plant growth regulators increases plant resistance to lodging (Peng et al., 2014; Wang C. et al., 2015).
The application of paclobutrazol increased the mechanical strength of basal internodes and decreased internode length and plant and ear height, thereby ultimately reducing lodging in maize (Kamran et al., 2018a). They also showed that paclobutrazol treatments significantly (P < 0.05) enhanced the ear characteristics (ear length and diameter, kernels ear−1, and 1,000 kernel weight) and grain yield of summer maize when compared with control treatments (Kamran et al., 2018a). The increase in grain yield, in response to paclobutrazol, is attributed partly to decreased investment in above-ground parts, due to a relatively stouter canopy of paclobutrazol-treated plants, as well as enhanced grain filling, in the treated plants due to improved rooting system, which possibly increased the uptake of nutrients and water (Qi, 2012; Kamran et al., 2018b). Given the above findings, at anthesis, the start and duration of pollen production, the start of silking, and anthesis-silking interval were mostly unaffected by paclobutrazol application (Hütsch and Schubert, 2021). Nonetheless, maize grain yield improvements after paclobutrazol application have been attributed to better grain-filling due to broader canopy, delayed onset of senescence and, thus, the start of chlorophyll degradation and improved rooting system (Kamran et al., 2018b, 2020). Kamran et al. (2020) pointed out that higher photosynthetic rate and duration (longer duration of green leaf area) were thought to be mainly responsible for grain yield increases after paclobutrazol treatment.
Uniconazole, a plant growth regulator used mainly to retard plant growth, results in shorter internodes, thereby increasing stem diameter, strengthening the overall stem structure, and increasing lodging resistance (Sellmer et al., 2001). Particularly in maize, the use of uniconazole results in decrease in plant height because of decrease in gibberellins, which results in reduction in cell length but not a reduction in the number of nodes (Schluttenhofer et al., 2011). However, in buckwheat, the results are not much different; lodging resistance and the lodging index were significantly reduced because of reduced plant height and increased lignin content (Wang C. et al., 2015). Schluttenhofer et al. (2011) reported that uniconazole increases lignin content, mechanical strength of the culm, and rind penetration strength, and decreases plant and ear height, which reduces the risk of lodging stress in maize. The application of uniconazole improved maize grain yield by higher kernel number per cob and increased kernel weight due to enhanced seed filling (Ahmad et al., 2018a,b, 2019). A significant increase in cob size was observed when uniconazole was applied at early growth stages, pointing to the impact of application time on maize yield performance (Xu et al., 2004); thus, considering the time of application may result in increased stem lodging and reduced kernel abortion simultaneously under high PD.
Conclusion
Stem lodging and kernel abortion considerably reduce grain yield. This review provides an understanding of stem lodging and kernel abortion mechanisms in maize. Interestingly, we found that genes involved in starch biosynthesis and transportation metabolism are involved in stem lodging resistance and kernel abortion. However, targeting sugar metabolism and via agronomic management, stem lodging and kernel abortion can be reduced under high planting density. Although there is no direct relationship between all the discussed agronomic practices and kernel abortion; however, reducing stem lodging can reduce kernel abortion in maize under high planting density. Thus, further research should be designed to investigate the genes directly involved in stem lodging and, thereby, induce kernel abortion. This will improve our understanding of the molecular basis of maize resistance to lodging. More focused research is needed to elucidate how sugar synthesis, transport, and accumulation processes should be altered to maximize maize resistance to stressful stimuli under high planting density. Further research should be designed to investigate the genes directly involved in stem lodging, and, thereby, induce kernel abortion. This will improve our understanding of the molecular basis of maize resistance to lodging.
Author Contributions
ANS, MT, AA, and YS conceived this review and drafted and finalized the paper. YS and MY helped to improve the draft by providing useful suggestions and information. AAS, MIA, ZW, and WS helped in finalizing the paper. All authors approved the work for publication.
Funding
The work was supported by a grant from China National Key R&D Program (Nos. 2017YFD0301307 and 2017YFD0300204-3) and Anhui Agricultural University Postdoctoral fellowship for ANS.
Conflict of Interest
The authors declare that the research was conducted in the absence of any commercial or financial relationships that could be construed as a potential conflict of interest.
Publisher's Note
All claims expressed in this article are solely those of the authors and do not necessarily represent those of their affiliated organizations, or those of the publisher, the editors and the reviewers. Any product that may be evaluated in this article, or claim that may be made by its manufacturer, is not guaranteed or endorsed by the publisher.
References
Abdullah, G. H., Khan, I. A., Khan, S. A., and Ali, H. (2008). Impact of planting methods and herbicides on weed biomass and some agronomic traits of maize. Pak. J. Weed Sci. Res., 14, 121–130.
Ahmad, I., Kamran, M., Ali, S., Bilegjargal, B., Cai, T., Ahmad, S., et al. (2018a). Uniconazole application strategies to improve lignin biosynthesis, lodging resistance and production of maize in semiarid regions. Field Crop Res. 222, 66–77. doi: 10.1016/j.fcr.2018.03.015
Ahmad, I., Kamran, M., Ali, S., Cai, T., Bilegjargal, B., Liu, T., et al. (2018b). Seed filling in maize and hormones crosstalk regulated by exogenous application of uniconazole in semiarid regions. Environ. Sci. Pollut. Res. 25, 33225–33239. doi: 10.1007/s11356-018-3235-0
Ahmad, I., Kamran, M., Meng, X., Ali, S., Bilegjargal, B., Cai, T., et al. (2019). Effects of plant growth regulators on seed filling, endogenous hormone contents and maize production in semiarid regions. J. Plant Growth Regul. 38, 1467–1480. doi: 10.1007/s00344-019-09949-2
Andrade, F. H., Cirilo, A. G., Uhart, S. A., and Otegui, M. E. (1996). Ecofisiología del Cultivo de Maíz (No. 633.15 584.92041). Balcarce: Dekalb Press.
Angel, M., Astrid, B., Francisca, S., and Jaume, L. (2019). Sowing date affects maize development and yield in irrigated mediterranean environments. Agriculture 9:67. doi: 10.3390/agriculture9030067
Anjum, S. A., Ashraf, U., Tanveer, M., Khan, I., Hussain, S., Shahzad, B., et al. (2017). Drought induced changes in growth, osmolyte accumulation and antioxidant metabolism of three maize hybrids. Front Plant Sci. 8:69. doi: 10.3389/fpls.2017.00069
Antonietta, M., Fanello, D. D., Acciaresi, H. A., and Guiamet, J. J. (2014). Senescence and yield responses to plant density in stay green and earlier-senescing maize hybrids from Argentina. Field Crops Res. 155, 111–119. doi: 10.1016/j.fcr.2013.09.016
Aoki, N., Hirose, T., Scofield, G. N., Whitfeld, P. R., and Furbank, R. T. (2003). The sucrose transporter gene family in rice. Plant Cell Physiol. 44, 223–232. doi: 10.1093/pcp/pcg030
Aoki, N., Hirose, T., Takahashi, S., Ono, K., Ishimaru, K., and Ohsugi, R. (1999). Molecular cloning and expression analysis of a gene for a sucrose transporter in maize (Zea mays L.). Plant Cell Physiol. 40, 1072–1078. doi: 10.1093/oxfordjournals.pcp.a029489
Appenzeller, L., Doblin, M., Barreiro, R., Wang, H., Niu, X., Kollipara, K., et al. (2004). Cellulose synthesis in maize: isolation and expression analysis of the cellulose synthase (CesA) gene family. Cellulose 11, 287–299. doi: 10.1023/B:CELL.0000046417.84715.27
Argenta, G., Silva, P. R. F., and Sangoi, L. (2001). Arranjo de plantas em milho: análise do estado-da- arte. Ciência Rural 31, 1075–1084. doi: 10.1590/S0103-84782001000600027
Arif, M., Ullah, I., Khan, S., Ghani, F., and Yousafzai, H. K. (2001). Response of maize varieties to different planting methods. Sarhad J. Agric. 17, 159–163.
Arnold, S. M., and Monteith, J. L. (1974). Plant development and mean temperature in a Teesdale habitat. J. Ecol. 62, 711–720. doi: 10.2307/2258951
Aslam, M., Zamir, M. S. I., Anjum, S. A., Khan, I., and Tanveer, M. (2015). An investigation into morphological and physiological approaches to screen maize (Zea mays L.) hybrids for drought tolerance. Cereal Res. Commun. 43, 41–51. doi: 10.1556/CRC.2014.0022
Bahaji, A., Li, J., Sánchez-López, Á. M., Baroja-Fernández, E., Muñoz, F. J., Ovecka, M., et al. (2014). Starch biosynthesis, its regulation and biotechnological approaches to improve crop yields. Biotechnol. Adv. 32, 87–106. doi: 10.1016/j.biotechadv.2013.06.006
Bakht, J., Ahmad, S., Tariq, M., Akbar, H., and Shafi, M. (2006). Response of maize to planting methods and fertilizer nitrogen. J. Agric. Biol. Sci. 1, 8–14.
Bakht, J. E. H. A.N., Shafi, M., Rehman, H., Uddin, R., and Anwar, S. (2011). Effect of planting methods on growth, phenology and yield of maize varieties. Pak. J. Bot. 43,1629–1633.
Barnabás, B., Jäger, K., and Fehér, A. (2008). The effect of drought and heat stress on reproductive processes in cereals. Plant Cell Environ. 31, 11–38. doi: 10.1111/j.1365-3040.2007.01727.x
Barratt, D., Paul, D., Kim, F., Marilyn, P., Nikolaus, W., John, L., et al. (2009). Normal growth of Arabidopsis requires cytosolic invertase but not sucrose synthase. Proc. Natl. Acad. Sci. U.S.A. 106, 13124–13129. doi: 10.1073/pnas.0900689106
Below, F. E., Cazetta, J. O., and Seebauer, J. R. (2000). Carbon/nitrogen interactions during ear and kernel development of maize, in Special Publication 29 Physiologies and Modeling Kernel Set in Maize, ed M. Westgate (Madison: Boote Crop Science Society of America), 15–24. doi: 10.2135/cssaspecpub29.c2
Berry, P., Griffin, J., Sylvester-Bradley, R., Scott, R., Spink, J., Baker, C., et al. (2000). Controlling plant form through husbandry to minimise lodging in wheat. Field Crop Res. 67, 59–81. doi: 10.1016/S0378-4290(00)00084-8
Berry, P. M., Sterling, M. J. H., Spink, C. J., Baker, R., Sylvester-Bradley, S. J., Mooney, A. R., et al. (2004). Understanding and reducing lodging cereals. Adv. Agron. 84, 217–271. doi: 10.1016/S0065-2113(04)84005-7
Betra'n, F. J., Beck, D., Ba nziger, M., and Edmeades, G. O. (2003). Secondary traits in parental inbreds and hybrids under stress and non-stress environments in tropical maize. Field Crops Res. 83, 51–65. doi: 10.1016/S0378-4290(03)00061-3
Bezrutczyk, M., Hartwig, T., Horschman, M., Char, S. N., Yang, J., Yang, B., et al. (2018). Impaired phloem loading in zmsweet13a,b,c sucrose transporter triple knock-out mutants in Zea mays. New Phytol. 218, 594–603. doi: 10.1111/nph.15021
Bian, D. H., Liu, M. X., Niu, H. F., Wei, Z. B., Du, X., and Cui, Y. H. (2017). Effects of nitrogen application times on stem traits and lodging of summer maize (Zea mays L.) in the Huang-Huai-Hai Plain. Sci. Agric. Sin. 50, 2294–2304.
Borrás, L., and Gambín, B. L. (2010). Trait dissection of maize kernel weight: towards integrating hierarchical scales using a plant growth approach. Field Crop Res. 118, 1–12. doi: 10.1016/j.fcr.2010.04.010
Borrás, L., and Otegui, M. E. (2001). Maize kernel weight response to postflowering source-sink ratio. Crop Sci. 41, 1816–1822. doi: 10.2135/cropsci2001.1816
Borrás, L., Westgate, M. E., and Otegui, M. E. (2003). Control of kernel weight and kernel water relations by post-flowering source-sink ratio in maize. Ann. Bot. 7, 857–867. doi: 10.1093/aob/mcg090
Boyer, J. S., and McLaughlin, J. E. (2007). Functional reversion to identify controlling genes in multigenic responses: analysis of floral abortion. J. Exp. Bot. 58, 267–277. doi: 10.1093/jxb/erl177
Braun, D. M., and Slewinski, T. L. (2009). Genetic control of carbon partitioning in grasses: roles of sucrose transporters and tie-dyed loci in phloem loading. Plant Physiol. 149, 71–81. doi: 10.1104/pp.108.129049
Brett, C., and Waldron, K., (eds.). (1990). The cell wall and control of cell growth, in Physiology and Biochemistry of Plant Cell Walls (Dordrecht: Springer Netherlands), 89–113. doi: 10.1007/978-94-010-9641-6_4
Bruns, H. A., and Abbas, H. K. (2006). Planting date effects on Bt and non-Bt corn in the mid-south USA. Agron. J. 98,100–106. doi: 10.2134/agronj2005.0143
Bukhsh, M. A. A. H. A., Ahmad, R., Iqbal, J., Maqbool, M. M., Ali, A., Ishaque, M., et al. (2012). Nutritional and physiological significance of potassium application in maize hybrid crop production. Pakistan J. Nutr. 11:187. doi: 10.3923/pjn.2012.187.202
Burton, R. A., Johnson, P. E., Beckles, D. M., Fincher, G. B., Jenner, H. L., Naldrett, M. J., et al. (2002). Characterization of the genes encoding the cytosolic and plastidial forms of ADP-glucose pyrophosphorylase in wheat endosperm. Plant Physiol. 130, 1464–1475. doi: 10.1104/pp.010363
Cai, T., Xu, H., Peng, D., Yin, Y., Yang, W., Ni, Y., et al. (2014). Exogenous hormonal application improves grain yield of wheat by optimizing tiller productivity. Field Crops Res. 155, 172–183. doi: 10.1016/j.fcr.2013.09.008
Cakmak, I. (2005). The role of potassium in alleviating detrimental effects of abiotic stresses in plants. J. Plant. Nutr. Soil Sci. 168, 521–530. doi: 10.1002/jpln.200420485
Cárcova, J., and Otegui, M. (2001). Ear temperature and pollination timing effects on maize kernel set. Crop Sci. 41, 1809–1815. doi: 10.2135/cropsci2001.1809
Carpaneto, A., Geiger, D., Bamberg, E., Sauer, N., Fromm, J., and Hedrich, R. (2005). Phloem- localized, proton-coupled sucrose carrier ZmSUT1 mediates sucrose efflux under the control of the sucrose gradient and the proton motive force. J. Biol. Chem. 280, 21437–21443. doi: 10.1074/jbc.M501785200
Chen, X., Guang, S. Y. H., Wang, C. Y., Yin, Y. P., Ning, T. Y., Shi, C. Y., et al. (2011). Effects of nitrogen and PP333 application on the lignin synthesis of stem in relation to lodging resistance of wheat. Sci. Agric. Sin. 44, 3529–3536.
Chen, Y., Chen, J., and Zhang, Y. (2007). Effect of harvest date on shearing force of maize stems. Livestock Sci. 111, 33–44. doi: 10.1016/j.livsci.2006.11.013
Chen, Y., Zhou, Q., Tian, R., Ma, Z., Zhao, X., Tang, J., et al. (2018). Proteomic analysis reveals that auxin homeostasis influences the eighth internode length heterosis in maize (Zea mays). Sci. Rep. 8, 1–13. doi: 10.1038/s41598-018-23874-6
Cheng, C. Y., and Lur, H. S. (1996). Ethylene may be involved in abortion of the maize caryopsis. Physiol. Plant 98, 245–252. doi: 10.1034/j.1399-3054.1996.980205.x
Cheng, F., Du, X., Liu, M., Jin, X., and Cui, Y. (2011). Lodging of summer maize and the effects on grain yield. J. Maize Sci. 19, 105–108.
Chourey, P. S., Li, Q. B., and Cevallos, J. (2012). Pleiotropy and its dissection through a metabolic gene Miniature1 (Mn1) that encodes a cell wall invertase in developing seeds of maize. Plant Sci. 184, 45–53. doi: 10.1016/j.plantsci.2011.12.011
Chourey, P. S., Li, Q. B., and Kumar, D. (2010). Hormone cross-talk in seed development: two redundant pathways of IAA biosynthesis are regulated differentially in the invertase-deficient miniature seed mutant in maize. Mol. Plant 3, 1026–1036. doi: 10.1093/mp/ssq057
Chourey, P. S., and Nelson, O. E. (1976). The enzymatic deficiency conditioned by the shrunken-1 mutations in maize. Biochem. Genet. 14, 1041–1055. doi: 10.1007/BF00485135
Ciampitti, I. A., and Vyn, T. J. (2011). A comprehensive study of plant density consequences on nitrogen uptake dynamics of maize plants from vegetative to reproductive stage. Field Crops Res. 121, 2–18. doi: 10.1016/j.fcr.2010.10.009
Ciampitti, I. A., and Vyn, T. J. (2012). Physiological perspectives of changes over time in maize yield dependency on nitrogen uptake and associated nitrogen efficiencies: a review. Field Crops Res. 133, 48–67. doi: 10.1016/j.fcr.2012.03.008
Cirilo, A. G., and Andrade, F. H. (1994). Sowing date and maize productivity: II. Kernel number determination. Crop Sci. 34, 1044–1046. doi: 10.2135/cropsci1994.0011183X003400040038x
Coleman, H. D., Ellis, D. D., Gilbert, M., and Mansfield, S. D. (2006). Up-regulation of sucrose synthase and UDP-glucose pyrophosphorylase impacts plant growth and metabolism. Plant Biotechnol. J. 4, 87–101. doi: 10.1111/j.1467-7652.2005.00160.x
Craine, J. M., and Dybzinski, R. (2013). Mechanisms of plant competition for nutrients, water and light. Funct. Ecol. 27, 833–840. doi: 10.1111/1365-2435.12081
Dahiya, S., Kumar, S., Chaudhary, C., and Chaudhary, C. (2018). Lodging: significance and preventive measures for increasing crop production. Int. J. Chem. Stud. 6, 700–705.
Dante, R. A., Sabelli, P. A., Nguyen, H. N., Leiva-Neto, J. T., Tao, Y, Lowe, K. S., et al. (2014). Cyclin-dependent kinase complexes in developing maize endosperm: evidence for differential expression and functional specialization. Planta 239, 493–509. doi: 10.1007/s00425-013-1990-1
Daynard, T. B., Tanner, J. W., and Hume, D. J. (1969). Contribution of stalk soluble carbohydrates to grain yield in corn (Zea mays L.). Crop Sci. 9, 831–834. doi: 10.2135/cropsci1969.0011183X000900060050x
Deng, H. L., Xiong, Y. C., Zhang, H. J., Li, F. Q., Zhou, H., Wang, Y. C., et al. (2019). Maize productivity and soil properties in the Loess Plateau in response to ridge-furrow cultivation with polyethylene and straw mulch. Sci. Rep. 9, 1–13. doi: 10.1038/s41598-019-39637-w
Dhaliwal, D. S., and Williams, M. M. (2020). Understanding variability in optimum plant density and recommendation domains for crowding stress tolerant processing sweet corn. PLoS ONE 15:0228809. doi: 10.1371/journal.pone.0228809
Dong, X. H., Duan, L. S., Meng, F. L., He, Z. P., and Li, Z. H. (2006). Effects of spraying 30% DTA- 6 ethephon solution on yield and straw quality of maize. J. Maize Sci. 14, 138–140.
Dunn, G. J., and Briggs, K. G. (1989). Variation in culm anatomy among barley cultivars differing in lodging resistance. Can. J. Bot. 67, 1838–1843. doi: 10.1139/b89-232
Earley, E. B., and Slife, F. W. (1969). Effect of ethrel on growth and yield of corn. Agron J. 61, 821–823. doi: 10.2134/agronj1969.00021962006100050053x
Echezona, B. C. (2007). Corn-stalk lodging and borer damage as influenced by varying corn densities and planting geometry with soybean (Glycine max. L. Merrill). Int. Agrophy 21, 133–143.
Edmeades, G. O., Bolanos, J., Elings, A., Ribaut, J. M., Bänziger, M., and Westgate, M. E. (2000). The role and regulation of the anthesis-silking interval in maize. Physiol. Model. Kernel Set Maize 29, 43–73. doi: 10.2135/cssaspecpub29.c4
Edreira, J. R., Carpici, E. B., Sammarro, D., and Otegui, M. E. (2011). Heat stress effects around flowering on kernel set of temperate and tropical maize hybrids. Field Crops Res. 123, 62–73. doi: 10.1016/j.fcr.2011.04.015
Epron, D., Cabral, O. M. R., Laclau, J. P., Dannoura, M., Packer, A. P., Plain, C., et al. (2016). In situ 13CO2 pulse labelling of field-grown eucalypt trees revealed the effects of potassium nutrition and through fall exclusion on phloem transport of photosynthetic carbon. Tree Physiol. 36, 6–21. doi: 10.1093/treephys/tpv090
Farkhari, M., Krivanek, A., Xu, Y., Rong, T., Naghavi, M. R., Samadi, B. Y., et al. (2013). Root- lodging resistance in maize as an example for high-through put genetic mapping via single nucleotide polymorphism-based selective genotyping. Plant Breed. 132, 90–98. doi: 10.1111/pbr.12010
Feng, G., Li, Y. Y., Jing, X., Cao, Z. B., Lu, B. S., and Huang, C. L. (2010). Relationship of root and stem characters with lodging resistance of summer maize. J. Henan. Agric. Sci. 11, 20–22.
Flint-Garcia, S. A., Jampatong, C., Darrah, L. L., and McMullen, M. D. (2003). Quantitative trait locus analysis of stalk strength in four maize populations. Crop Sci. 43, 13–22. doi: 10.2135/cropsci,2003.0013
Foulkes, M. J., Slafer, G. A., Davies, W. J., Berry, P. M., Sylvester-Bradley, R., Martre, P., et al. (2011). Raising yield potential of wheat. III. Optimizing partitioning to grain while maintaining lodging resistance. J. Exp. Bot. 62, 469–486. doi: 10.1093/jxb/erq300
Frei, M. (2013). Lignin: characterization of a multifaceted crop component. Sci. World J. 2013:436517. doi: 10.1155/2013/436517
Fu, X. Q., Feng, J., Yu, B., and Gao, Y. J. (2013). Morphological, biochemical and genetic analysis of a brittle stalk mutant of maize inserted by mutator. J. Integr. Agric. 12, 12–18. doi: 10.1016/S2095-3119(13)60200-2
Fu, X. Z., Zhou, X., Xing, F., Ling, L. L., Chun, C. P., Cao, L., et al. (2017). Genome-wide identification, cloning and functional analysis of the zinc/iron-regulated transporter-like protein (ZIP) gene family in trifoliate orange (Poncirus trifoliata L. Raf.). Front. Plant Sci. 8:588. doi: 10.3389/fpls.2017.00588
Gao, B., Zhou, Y. D., Li, D. M., Wang, K. C., and Li, M. (2009). Effect of ethephon on growth and yield of high-yield spring maize. J. Northeast Agric. Univ. 40, 13–17.
Geigenberger, P. (2011). Regulation of starch biosynthesis in response to a fluctuating environment. Plant Physiol. 155, 1566–1577. doi: 10.1104/pp.110.170399
Gonzalez, V. H., Lee, E. A., Lukens, L. L., and Swanton, C. J. (2019). The relationship between floret number and plant dry matter accumulation varies with early season stress in maize (Zea mays L.). Field Crop. Res. 238, 129–138. doi: 10.1016/j.fcr.2019.05.003
Guelette, B. S., Benning, U. F., and Hoffmann-Benning, S. (2012). Identification of lipids and lipid-binding proteins in phloem exudates from Arabidopsis thaliana. J. Exp. Bot. 63, 3603–3616. doi: 10.1093/jxb/ers028
Gustin, J. L., Boehlein, S. K., Shaw, J. R., Junior, W., Settles, A. M., Webster, A., et al. (2018). Ovary abortion is prevalent in diverse maize inbred lines and is under genetic control. Sci. Rep. 8, 1–8. doi: 10.1038/s41598-018-31216-9
Hale, J., Webb, S., Hale, N., Stubbs, C., and Cook, D. D. (2021). A method for assessing axial and temporal effects of the leaf sheath on the flexural stiffness of the maize stem. [PREPRINT]. doi: 10.21203/rs.3.rs-321341/v1
Hammad, H. M., Abbas, F., Ahmad, A., Bakhat, H. F., Farhad, W., Wilkerson, C. J., et al. (2020). Predicting kernel growth of maize under controlled water and nitrogen applications. Int. J. Plant Product. 14, 609–620. doi: 10.1007/s42106-020-00110-8
Hammer, G. L., Dong, Z., McLean, G., Doherty, A., Messina, C., Schussler, J., et al. (2009). Can changes in canopy and/or root system architecture explain historical maize yield trends in the U.S. corn belt? Crop Sci. 49, 299–312. doi: 10.2135/cropsci2008.03.0152
Han, L., Zhu, Y., Liu, M., Zhou, Y., Lu, G., Lan, L., et al. (2017). Molecular mechanism of substrate recognition and transport by the AtSWEET13 sugar transporter. Proc. Natl. Acad. Sci. U.S.A. 114, 10089–10094. doi: 10.1073/pnas.1709241114
Hänggi, E., and Fleming, A. J. (2001). Sucrose synthase expression pattern in young maize leaves: implications for phloem transport. Planta 214, 326–329. doi: 10.1007/s00425-001-0680-6
Hannah, L. C., Shaw, J. R., Clancy, M. A., Georgelis, N., and Boehlein, S. K. (2017). A brittle-2 transgene increases maize yield by acting in maternal tissues to increase seed number. Plant Direct 1:e00029. doi: 10.1002/pld3.29
Hazen, S. P., Wu, Y. J., and Kreps, J. A. (2003). Gene expression profi ling of plant responses to abiotic stress. Funct. Integr. Genomics 3, 105–111. doi: 10.1007/s10142-003-0088-4
Hendriks, J. H. M., Kolbe, A., Gibon, Y., Stitt, M., and Geigenberger, P. (2003). ADP-glucose pyrophosphorylase is activated by posttranslational redox-modification in response to light and to sugars in leaves of arabidopsis and other plant species. Plant Physiol. 133, 838–849. doi: 10.1104/pp.103.024513
Hiyane, R., Hiyane, S., Tang, A. C., and Boyer, J. S. (2010). Sucrose feeding reverses shade-induced kernel losses in maize. Ann. Bot. 106, 395–403. doi: 10.1093/aob/mcq132
Hu, H., Liu, W., Fu, Z., Homann, L., Technow, F., Wang, H., et al. (2013). QTL mapping of stalk bending strength in a recombinant inbred line maize population. Theor. Appl. Genet. 126, 2257–2266. doi: 10.1007/s00122-013-2132-7
Huang, H., Xie, S., Xiao, Q., Wei, B., Zheng, L., Wang, Y., et al. (2016). Sucrose and ABA regulate starch biosynthesis in maize through a novel transcription factor, ZmEREB156. Sci. Rep. 6:27590. doi: 10.1038/srep27590
Huang, J. (2008). Study on density tolerance character of super high-yielding population (MS Dissertation). Shihezi University, Xinjiang, China.
Huang, L., Qiao, J. F., Liu, J. B., Xia, L., Zhu, W. H., Li, C., et al. (2015). Research on the relationship between maize lodging resistance and grain mechanically qualities in different planting density. Acta Agric. Boreali-Sin. 30, 198–201. doi: 10.7668/hbnxb.2015.02.034
Hussen, A. (2020). Review on: response of cereal crops to climate change. Adv. Biosci. Bioeng. 8:63. doi: 10.11648/j.abb.20200804.11
Hütsch, B. W., and Schubert, S. (2018). Maize harvest index and water use efficiency can be improved by inhibition of gibberellin biosynthesis. J. Agron. Crop Sci. 204, 209–218. doi: 10.1111/jac.12250
Hütsch, B. W., and Schubert, S. (2021). Water-use efficiency of maize may be increased by the plant growth regulator paclobutrazol. J. Agron. Crop Sci. 207, 521–534. doi: 10.1111/jac.12456
Imkampe, J., Halter, T., Huang, S., Schulze, S., Mazzotta, S., Schmidt, N., et al. (2017). The arabidopsis leucine-rich repeat receptor kinase BIR3 negatively regulates BAK1 receptor complex formation and stabilizes BAK1. Plant Cell 29, 2285–2303. doi: 10.1105/tpc.17.00376
Jin, Y., Ni, D. A., and Ruan, Y. L. (2009). Posttranslational elevation of cell wall invertase activity by silencing its inhibitor in tomato delays leaf senescence and increases seed weight and fruit hexose level. Plant Cell 21, 2072–2089. doi: 10.1105/tpc.108.063719
Jones, L., Ennos, A. R., and Turner, S. R. (2001). Cloning and characterization of irregular xylem4 (irx4): a severely lignin-deficient mutant of Arabidopsis. Plant J. 260, 205–216. doi: 10.1046/j.1365-313x.2001.01021.x
Juarez-Colunga, S., Lopez-Gonzalez, C., Morales-Elias, N. C., Massange-Sanchez, J. A., Trachsel, S., and Tiessen, A. (2018). Genome-wide analysis of the invertase gene family from maize. Plant Mol. Biol. 97, 385–406. doi: 10.1007/s11103-018-0746-5
Julius, B., Slewinski, T. L., Baker, R. F., Tzin, V., Zhou, S., Bihmidine, S., et al. (2018). Maize carbohydrate partitioning defective1 impacts carbohydrate distribution, callose accumulation, and phloem function. J. Exp. Bot. 69, 3917–3931. doi: 10.1093/jxb/ery203
Julius, B. T., Leach, K. A., Tran, T. M., Mertz, R. A., and Braun, D. M. (2017). Sugar transporters in plants: new insights and discoveries. Plant Cell Physiol. 58, 1442–1460. doi: 10.1093/pcp/pcx090
Jun, X., Xie, U. E., Zhang, R. Z., Wang, W. F., Peng, K. R., Bo, H. O. U., et al. (2017). Research progress on reduced lodging of high-yield and-density maize. J. Integr. Agric. 16, 2717–2725.
Kamran, M., Ahmad, I., Wang, H., Wu, X., Xu, J., Liu, T., et al. (2018c). Mepiquat chloride application increases lodging resistance of maize by enhancing stem physical strength and lignin biosynthesis. Field Crops Res. 224, 148–159 doi: 10.1016/j.fcr.2018.05.011
Kamran, M., Ahmad, S., Ahmad, I., Hussain, I., Meng, X., Zhang, X., et al. (2020). Paclobutrazol application favors yield improvement of maize under semiarid regions by delaying leaf senescence and regulating photosynthetic capacity and antioxidant system during grain-filling stage. Agronomy 10:187. doi: 10.3390/agronomy10020187
Kamran, M., Cui, W., Ahmad, I., Meng, X., Zhang, X., Su, W., et al. (2018a). Effect of paclobutrazol, a potential growth regulator on stalk mechanical strength, lignin accumulation and its relation with lodging resistance of maize. Plant Growth Regul. 84, 317–332. doi: 10.1007/s10725-017-0342-8
Kamran, M., Wennan, S., Ahmad, I., Xiangping, M., Wenwen, C., Xudong, Z., et al. (2018b). Application of paclobutrazol affect maize grain yield by regulating root morphological and physiological characteristics under a semi-arid region. Sci. Rep. 8:4818. doi: 10.1038/s41598-018-23166-z
Khosravi, G., and Anderson, R. I. C. (1991). Growth, yield, and yield components of ethephon-treated corn. Plant Growth Regul. 10, 27–36. doi: 10.1007/BF00035128
Kokubo, A., Kuraishi, S., and Sakurai, N. (1989). Culm strength of barley: correlation among maximum bending stress, cell wall dimensions, and cellulose content. Plant Physiol. 91, 876–882. doi: 10.1104/pp.91.3.876
Kolbe, A., Tiessen, A., Schluepmann, H., Paul, M., Ulrich, S., and Geigenberger, P. (2005). Trehalose 6-phosphate regulates starch synthesis via posttranslational redox activation of ADP-glucose pyrophosphorylase. Proc. Natl. Acad. Sci. U.S.A. 102, 11118–11123. doi: 10.1073/pnas.0503410102
Kong, X., Pan, J., Zhang, D., Jiang, S., Cai, G., and Wang, L. (2013). Identification of mitogen- activated protein kinase kinase gene family and MKK-MAPK interaction network in maize. Biochem. Biophys res. 441, 964–969. doi: 10.1016/j.bbrc.2013.11.008
Kuai, J., Yang, Y., Sun, Y., Zhou, G., Zuo, Q., Wu, J., et al. (2015). Paclobutrazol increases canola seed yield by enhancing lodging and pod shatter resistance in Brassica napus L. Field Crops Res. 180, 10–20. doi: 10.1016/j.fcr.2015.05.004
Kumar, S., Choudhary, P., Gupta, M., and Nath, U. (2018). VASCULAR PLANT ONE-ZINC FINGER1 (VOZ1) and VOZ2 interact with CONSTANS and promote photoperiodic flowering transition. Plant Physiol. 176, 2917–2930. doi: 10.1104/pp.17.01562
Lashkari, M., Madani, H., Ardakani, M. R., Golzardi, F., and Zargari, K. (2011). Effect of plant density on yield and yield components of different corn (Zea mays L.) hybrids. Am-Euras J. Agric. Environ. Sci. 10, 450–457.
Leach, K. A., Tran, T. M., Slewinski, T. L., Meeley, R. B., and Braun, D. M. (2017). Sucrose transporter2 contributes to maize growth, development, and crop yield. J. Integr. Plant Biol. 59, 390–408. doi: 10.1111/jipb.12527
Lee, E. A., and Tollenaar, M. (2007). Physiological basis of successful breeding strategies for maize grain yield. Crop Sci. 47,202–215. doi: 10.2135/cropsci2007.04.0010IPBS
Lehti-Shiu, M. D., and Shiu, S. H. (2012). Diversity, classification and function of the plant protein kinase superfamily. Philos. Trans. R. Soc. Lond. B Biol. Sci. 367, 2619–2639. doi: 10.1098/rstb.2012.0003
Leroux, O. (2012). Collenchyma: a versatile mechanical tissue with dynamic cell walls. Ann. Bot. 110, 1083–1098 doi: 10.1093/aob/mcs186
Li, B., Zhang, J., Cui, H., Jin, L., Dong, S., Liu, P., et al. (2012). Effects of potassium application rate on stem lodging resistance of summer maize under high yield conditions. Acta Agron. Sin. 38, 2093–2099. doi: 10.3724/SP.J.1006.2012.02093
Li, C., and Li, C. (2021). Ridge-furrow with plastic film mulching system decreases the lodging risk for summer maize plants under different nitrogen fertilization rates and varieties in dry semi-humid areas. Field Crops Res. 263:108056. doi: 10.1016/j.fcr.2021.108056
Li, C., Li, C., Ma, B., and Wu, W. (2020). The role of ridge furrow with plastic film mulching system on stem lodging resistance of winter wheat in a dry semi humid region. Agron. J. 112, 885–898. doi: 10.1002/agj2.20073
Li, H., Li, L., Wegenast, T., Longin, C. F., Xu, X., Melchinger, A. E., et al. (2010). Effect of N supply on stalk quality in maize hybrids. Field Crops Res. 118, 208–214. doi: 10.1016/j.fcr.2010.05.005
Li, J., Baroja-Fernández, E., Bahaji, A., Muñoz, F. J., Ovecka, M., Montero, M., et al. (2013). Enhancing sucrose synthase activity results in increased levels of starch and ADP-glucose in maize (Zea mays L.) seed endosperms. Plant Cell Physiol. 54, 282–294. doi: 10.1093/pcp/pcs180
Li, J., Zhang, H., Gong, J., Chang, Y., Dai, Q., Huo, Z., et al. (2011). Effects of different planting methods on the culm lodging resistance of super rice. Sci. Agric. Sin. 44, 2234–2243.
Li, L. L., Gu, W. R., Li, C. F., Li, W. H., Chen, X. C., Zhang, L. G., et al. (2019). Dual application of ethephon and DCPTA increases maize yield and stalk strength. Agron. J. 111, 612–627. doi: 10.2134/agronj2018.06.0363
Li, S. C., Bai, P., Lu, X., Liu, S. Y., and Dong, S. T. (2003). Ecological and planting date effects on maize grain filling. Acta Agron. Sin. 29, 775–778.
Li, S. K., Wang, C. T, and Mo, J. (2010). Potential and Ways to High Yield in Maize. Beijing: Science Press (in Chinese), 217–240.
Li, S. Y., Ma, W., Peng, J. Y., and Chen, Z. M. (2015). Study on yield loss of summer maize due to lodging at the big flare stage and grain filling stage. Sci. Agric. Sin. 19, 3952–3964. doi: 10.3864/j.issn.0578-1752.2015.19.017
Li, W. Y., Yin, Y. P., Yan, S. H., Dai, Z. M., Li, Y., Liang, T. B., Geng, Q. H., et al. (2008). Effect of shading after anthesis on starch accumulation and activities of the related enzymes in wheat grain. Acta Agron. Sin. 34, 632–640. doi: 10.3724/SP.J.1006.2008.00632
Li, Y., Qian, Q., Zhou, Y., Yan, M., Sun, L., Zhang, M., et al. (2003). BRITTLE CULM1, which encodes a COBRA-like protein, affects the mechanical properties of rice plants. Plant Cell 15, 2020–2031. doi: 10.1105/tpc.011775
Liebhardt, W. C., and Murdock, J. T. (1965). Effect of potassium on morphology and lodging of corn. Agron. J. 57, 325–328. doi: 10.2134/agronj1965.00021962005700040004x
Liu, M. X., and Yong, Q. G. (2008). Effects of ridge-furrow tillage on soil water and crop yield in semiarid region, in The 2nd International Conference. Shanghai. doi: 10.1109/ICBBE.2008.395
Loor, J. J., Nikolic, M., Uzun, B., Velasco, L., and Wilson, B. R. (2013). Lignin: characterization of a multifaceted crop component. Sci. World J. 2013:436517.
Ma, D., Xie, R., Liu, X., Niu, X., Hou, P., Wang, K., et al. (2014). Lodging-related stalk characteristics of maize varieties in China since the 1950s. Crop Sci. 54, 2805–2814. doi: 10.2135/cropsci2014.04.0301
Ma, S., Li, Y., Li, X., Sui, X., and Zhang, Z. (2019). Phloem unloading strategies and mechanisms in crop fruits. J. Plant Growth Regul. 38, 494–500. doi: 10.1007/s00344-018-9864-1
Maddonni, G. A., and Otegui, M. E. (2004). Intra-specific competition in maize: early establishment of hierarchies among plants affects final kernel set. Field Crops Res. 85, 1–13. doi: 10.1016/S0378-4290(03)00104-7
Maddonni, G. A., and Otegui, M. E. (2006). Intra-specific competition in maize: contribution of extreme plant hierarchies to grain yield, grain yield components and kernel composition. Field Crops Res. 97, 155–166. doi: 10.1016/j.fcr.2005.09.013
Marahatta, S. (2020). Nitrogen levels influence barrenness and sterility of maize varieties under different establishment methods during hot spring in western terai of nepal. J. Agric. For. Univ. 4:117.
Martins, M. A., Tomasella, J., and Dias, C. G. (2019). Maize yield under a changing climate in the Brazilian Northeast: impacts and adaptation. Agric. Water Manage. 216, 339–350. doi: 10.1016/j.agwat.2019.02.011
Mayer, L. I., Rossini, M. A., and Maddonni, G. A. (2012). Inter-plant variation of grain yield components and grain composition of maize crops grown under contrasting nitrogen supply. Field Crops Res. 125, 98–108. doi: 10.1016/j.fcr.2011.09.004
McCurdy, D. W., Dibley, S., Cahyanegara, R., Martin, A., and Patrick, J. W. (2010). Functional characterization and RNAi-mediated suppression reveals roles for hexose transporters in sugar accumulation by tomato fruit. Mol. Plant 3, 1049–1063. doi: 10.1093/mp/ssq050
McLaughlin, J. E., and Boyer, J. S. (2004). Glucose localization in maize ovaries when kernel number decreases at low water potential and sucrose is fed to the stems. Ann. Bot. 94, 75–86. doi: 10.1093/aob/mch123
McMaster, G. S., and Wilhelm, W. W. (1997). Growing degree-days: one equation, two interpretations. Agric. For. Meteorol. 87, 291–300. doi: 10.1016/S0168-1923(97)00027-0
Mizuno, H., Kasuga, S., and Kawahigashi, H. (2016). The sorghum SWEET gene family: stem sucrose accumulation as revealed through transcriptome profiling. Biotechnol. Biofuels. 9:127. doi: 10.1186/s13068-016-0546-6
Mizuno, H., Kasuga, S., and Kawahigashi, H. (2018). Root lodging is a physical stress that changes gene expression from sucrose accumulation to degradation in sorghum. BMC Plant Biol. 18:2. doi: 10.1186/s12870-017-1218-9
Mueller, S. M., Messina, C. D., and Vyn, T. J. (2019). The role of the exponential and linear phases of maize (Zea mays L.) ear growth for determination of kernel number and kernel weight. Eur. J. Agron. 111:125939. doi: 10.1016/j.eja.2019.125939
Mulder, E. G. (1954). Effect of mineral nutrition on lodging of cereals. Plant Soil 5, 246–306. doi: 10.1007/BF01395900
Munoz-Martinez, F., Garcia-Fontana, C., Rico-Jimenez, M., Alfonso, C., and Krell, T. (2012). Genes encoding Cher-TPR fusion proteins are predominantly found in gene clusters encoding chemosensory pathways with alternative cellular functions. PLoS ONE 7:e45810. doi: 10.1371/journal.pone.0045810
Naeem, M., Khan, M., and Moinuddin, M. A. (2012). Triacontanol: a potent plant growth regulator in agriculture. J. Plant Interact. 7, 129–142. doi: 10.1080/17429145.2011.619281
Ngoune Tandzi, L., and Mutengwa, C. S. (2020). Estimation of maize (Zea mays L.) yield per harvest area: appropriate methods. Agronomy 10:29. doi: 10.3390/agronomy10010029
Nielsen, B. (2006). Stalk Lodging Corn: Guidelines for Preventive Management. West Lafayette, IN: Agronomy Guide, University of Purdue Press.
Niklas, K. J. (1991). Bending stiffness of cylindrical plant organs with a 'core-rind' construction: evidence from juncus effusus leaves. Am. J. Bot. 78, 561–568. doi: 10.1002/j.1537-2197.1991.tb15222.x
Ning, P., Peng, Y., and Fritschi, F. B. (2018). Carbohydrate dynamics in maize leaves and developing ears in response to nitrogen application. Agronomy 8:302. doi: 10.3390/agronomy8120302
Novacek, M. J., Mason, S. C., Galusha, T. D., and Yaseen, M. (2013). Twin rows minimally impact irrigated maize yield, morphology, and lodging. Agron. J. 105, 268–276. doi: 10.2134/agronj2012.0301
Nuccio, M. L., Wu, J., Mowers, R., Zhou, H. P., Meghji, M., Primavesi, L. F., et al. (2015). Expression of trehalose-6-phosphate phosphatase in maize ears improves yield in well-watered and drought conditions. Nat. Biotechnol. 33, 862–869. doi: 10.1038/nbt.3277
Ookawa, T., and Ishihara, K. (1992). Varietal differences of physical characteristics of the culm related to lodging in paddy rice. Jpn. J. Crop Sci. 61, 419–425. doi: 10.1626/jcs.61.419
Otegui, E., Nicolini, G., Ruiz, R. A., and Dodds, P. A. (1995). Sowing date effects on grain yield components for different maize genotypes. Agron. J. 87, 29–33. doi: 10.2134/agronj1995.00021962008700010006x
Otegui, M. E. (1997). Kernel set and flower synchrony within the ear of maize: plant population effects. Crop Sci. 37, 448–455. doi: 10.2135/cropsci1997.0011183X003700020024x
Oury, V., Tardieu, F., and Turc, O. (2016). Ovary apical abortion under water deficit is caused by changes in sequential development of ovaries and in silk growth rate in maize. Plant Physiol. 171, 986–996. doi: 10.1104/pp.15.00268
Özmen, A. D., Özdemír, F., and Türkan, I. (2003). Effects of paclobutrazol on response of two barley cultivars to salt stress. Biol Plant. 46, 263–268. doi: 10.1023/A:1022862929881
Paponov, I. A., Paponov, M., Sambo, P., and Engels, C. (2020). Differential regulation of kernel set and potential kernel weight by nitrogen supply and carbohydrate availability in maize genotypes contrasting in nitrogen use efficiency. Front. Plant Sci. 11:586. doi: 10.3389/fpls.2020.00586
Peng, D., Chen, X., Yin, Y., Lu, K., Yang, W., Tang, Y., et al. (2014). Lodging resistance of winter wheat (Triticum aestivum L.): lignin accumulation and its related enzymes activities due to the application of paclobutrazol or gibberellin acid. Field Crops Res. 157, 1–7. doi: 10.1016/j.fcr.2013.11.015
Peng, H., Meyer, R. S., Yang, T., Whitaker, B. D., Trouth, F., Shangguan, L., et al. (2019). A novel hydroxycinnamoyl transferase for synthesis of hydroxycinnamoyl spermine conjugates in plants. BMC Plant Biol. 19:261. doi: 10.1186/s12870-019-1846-3
Pineiro, M., Gomez-Mena, C., Schaffer, R., Martinez-Zapater, J. M., and Coupland, G. (2003). EARLY BOLTING IN SHORT DAYS is related to chromatin remodeling factors and regulates flowering in Arabidopsis by repressing FT. Plant Cell 15, 1552–1562. doi: 10.1105/tpc.012153
Piñera-Chavez, F. J., Berry, P. M., Foulkes, M. J., Jesson, M. A., and Reynolds, M. P. (2016). Avoiding lodging in irrigated spring wheat. I. Stem and root structural requirements. Field Crops Res. 196, 325–336. doi: 10.1016/j.fcr.2016.06.009
Qi, W. Z. (2012). Morphological and physiological characteristics of corn (Zea mays L.) roots from cultivars with different yield potentials. Eur. J. Agron. 38, 54–63. doi: 10.1016/j.eja.2011.12.003
Rajcan, I., Chandler, K. J., and Swanton, C. J. (2004). Red-far-red ratio of reflected light: a hypothesis of why early-season weed control is important in corn. Weed Sci. 52, 774–778. doi: 10.1614/WS-03-158R
Rajcan, I., and Swanton, C. (2001). Understanding maize-weed competition: resource competition, light quality and the whole plant. Field Crops Res. 71, 139–150. doi: 10.1016/S0378-4290(01)00159-9
Rattalino, E. J. I., Budakli, C. E., Sammarro, D., and Otegui, M. E. (2011). Heat stress effects around flowering on kernel set of temperate and tropical maize hybrids. Field Crops Res. 123, 62–73.
Riedell, W. E., and Reese, R. N. (1999). Maize morphology and shoot CO2 assimilation after root damage by western corn rootworm larvae. Crop Sci. 39, 1332–1340. doi: 10.2135/cropsci1999.3951332x
Ruan, Y. L. (2014). Sucrose metabolism: gateway to diverse carbon use and sugar signaling. Annu. Rev. Plant Biol. 65, 33–67. doi: 10.1146/annurev-arplant-050213-040251
Ruan, Y. L., Jin, Y., Yang, Y. J., Li, G. J., and Boyer, J. S. (2010). Sugar input, metabolism, and signaling mediated by invertase: roles in development, yield potential, and response to drought and heat. Mol. Plant. 3, 942–955. doi: 10.1093/mp/ssq044
Ruan, Z., Zanotti, M., Wang, X., Ducey, C., and Liu, Y. (2012). Evaluation of lipid accumulation from lignocellulosic sugars by Mortierella isabellina for biodiesel production. Bioresour. Technol. 110, 198–205. doi: 10.1016/j.biortech.2012.01.053
Ruffo, M. L., Gentry, L. F., Henninger, A. S., Seebauer, J. R., and Below, F. E. (2015). Evaluating management factor contributions to reduce corn yield gaps. Agron. J. 107, 495–505. doi: 10.2134/agronj14.0355
Saini, H. S., and Westgate, M. E. (1999). Reproductive development in grain crops during drought. Adv. Agron. 68, 59–96. doi: 10.1016/S0065-2113(08)60843-3
Salter, P. J., and Goode, J. E. (1967). Crop responses to water at different stages of growth. Farnham Royal, Buckinghamshire Commonwealth Agricultural Bureaux.
Sangoi, L. (2001). Understanding plant density effects on maize growth and development: an important issue to maximize grain yield. Ciência Rural 31, 159–168. doi: 10.1590/S0103-84782001000100027
Sangoi, L., Gracietti, M. A., Rampazzo, C., and Bianchetti, P. (2002). Response of Brazilian maize hybrids from different eras to changes in plant density. Field Crops Res. 79, 39–51. doi: 10.1016/S0378-4290(02)00124-7
Santiago, R., Malvar, R. A., Barros Rios, J., Samayoa, L. F., and Butrón, A. (2016). Hydroxycinnamate synthesis and association with mediterranean corn borer resistance. J. Agric. Food Chem. 64, 539–551. doi: 10.1021/acs.jafc.5b04862
Savin, R., Prystupa, P., and Araus, J. L. (2006). Hordein composition as affected by post-anthesis source-sink ratio under different nitrogen availabilities. J. Cereal Sci. 44, 113–116. doi: 10.1016/j.jcs.2006.01.003
Sayre, J. D., Morris, V. H., and Richey, F. D. (1931). The effect of preventing fruiting and of reducing the leaf area on the accumulation of sugars in the corn stem1. Agron. J. 23, 751–753. doi: 10.2134/agronj1931.00021962002300090008x
Schluttenhofer, C. M., Massa, G. D., and Mitchell, C. A. (2011). Use of uniconazole to control plant height for an industrial/pharmaceutical maize platform. Ind. Crops Prod. 33, 720–726. doi: 10.1016/j.indcrop.2011.01.009
Searchinger, T., Waite, R., Hanson, C., Ranganathan, J., Dumas, P., Matthews, E., et al. (2019). Creating a Sustainable Food Future: A Menu of Solutions to Feed Nearly 10 Billion People by 2050. Final report, World Resources Institute.
Sellmer, J. C., Adkins, C. R., McCall, I., and Whipker, B. E. (2001). Pampas grass responses to ancymidol, paclobutrazol and uniconazole substrate drenches. Hort Technol. 11, 216–219. doi: 10.21273/HORTTECH.11.2.216
Setter, T. L., and Flannigan, B. A. (1986). Sugar and starch redistribution in maize in response to shade and ear temperature treatment1. Crop Sci. 26, 575–579. doi: 10.2135/cropsci1986.0011183X002600030031x
Setter, T. L., Flannigan, B. A., and Melkonian, J. (2001). Loss of kernel set due to water deficit and shade in maize: carbohydrate supplies, abscisic acid, and cytokinins. Crop Sci. 41, 1530–1540. doi: 10.2135/cropsci2001.4151530x
Setter, T. L., Laureles, E. V., and Mazaredo, A. M. (1997). Lodging reduces yield of rice by self- shading and reductions in canopy photosynthesis. Field Crops Res. 49, 95–106. doi: 10.1016/S0378-4290(96)01058-1
Setter, T. L., and Parra, R. (2010). Relationship of carbohydrate and abscisic acid levels to kernel set in maize under post pollination water deficit. Crop Sci. 50, 980–988. doi: 10.2135/cropsci2009.07.0391
Shah, A. N., Tanveer, M., Rehman, A.u., Anjum, S. A., Iqbal, J., and Ahmad, R. (2017). Lodging stress in cereal-effects and management: an overview. Environ. Sci. Pollut. Res. 24, 5222–5237. doi: 10.1007/s11356-016-8237-1
Shahzad, A. N., Fatima, A., Sarwar, N., Bashir, S., Rizwan, M., Qayyum, M. F., et al. (2017). Foliar application of potassium sulfate partially alleviates pre-anthesis drought-induced kernel abortion in maize. Int. J. Agric. Biol. 19:495. doi: 10.17957/I.J.A.B./15.0317
Sharifi, R. S., and Namvar, A. (2016). Effects of time and rate of nitrogen application on phenology and some agronomical traits of maize (Zea mays L.). Biologija 62, 35–45. doi: 10.6001/biologija.v62i1.3288
Sharifi, R. S., Sedghi, M., and Gholipouri, A. (2009). Effect of population density on yield and yield attributes of maize hybrids. Res. J. Biol. Sci. 4, 375–379.
Shekoofa, A., and Emam, Y. (2008). Plant growth regulator (ethephon) alters maize (Zea maps L.) growth. J. Agron. 7, 41–48. doi: 10.3923/ja.2008.41.48
Shen, S., Li, B. B., Deng, T., Xiao, Z. D., Chen, X. M., Hu, H., et al. (2020). The equilibrium between sugars and ethylene is involved in shading-and drought-induced kernel abortion in maize. Plant Growth Regul. 91, 101–111. doi: 10.1007/s10725-020-00590-8
Shen, S., Ma, S., Liu, Y., Liao, S., Li, J., Wu, L., Kartika, D., et al. (2019). Cell wall invertase and sugar transporters are differentially activated in tomato styles and ovaries during pollination and fertilization. Front. Plant Sci. 10:506. doi: 10.3389/fpls.2019.00506
Shen, S., Zhang, L.i., Liang, X. G., Zhao, X., Lin, S., Qu, L. H., et al. (2018). Delayed pollination and low availability of assimilates are major factors causing maize kernel abortion. J. Exp. Bot. 69, 1599–1613. doi: 10.1093/jxb/ery013
Sher, A., Khan, A., Ashraf, U., Liu, H. H., and Li, J. C. (2018). Characterization of the effect of increased plant density on canopy morphology and stalk lodging risk. Front. Plant Sci. 9:1047. doi: 10.3389/fpls.2018.01047
Shiferaw, B., Prasanna, B. M., Hellin, J., and Bänziger, M. (2011). Crops that feed the world 6. Past successes and future challenges to the role played by maize in global food security. Food Secur. 3:307. doi: 10.1007/s12571-011-0140-5
Slewinski, T. L. (2012). Non-structural carbohydrate partitioning in grass stems: a target to increase yield stability, stress tolerance, and biofuel production. J. Exp. Bot. 63, 4647–4670. doi: 10.1093/jxb/ers124
Slewinski, T. L., and Braun, D. M. (2010). Current perspectives on the regulation of whole-plant carbohydrate partitioning. Plant Sci. 178, 341–349. doi: 10.1016/j.plantsci.2010.01.010
Slewinski, T. L., Meeley, R., and Braun, D. M. (2009). Sucrose transporter1 functions in phloem loading in maize leaves. J. Exp. Bot. 60, 881–892. doi: 10.1093/jxb/ern335
Somssich, M. (2020). Lignification and oxidative enzymes: localization, localization, localization! Plant Physiol. 184, 554–555. doi: 10.1104/pp.20.01021
Song, Y., Rui, Y., Bedane, G., and Li, J. (2016). Morphological characteristics of maize canopy development as affected by increased plant density. PLoS ONE 11:e0154084. doi: 10.1371/journal.pone.0154084
Sosso, D., Luo, D. P., Li, Q. B., Sasse, J., Yang, J., Gendrot, G., et al. (2015). Seed filling in domesticated maize and rice depends on SWEET-mediated hexose transport. Nat. Genet. 47, 1489–1493. doi: 10.1038/ng.3422
Spatz, H., Boomgaarden, C., and Speck, T. (1993). Contribution to the biomechanics of plants. III: experimental and theoretical studies of local buckling. Bot. Acta 106, 254–264.
Stanger, T. F., and Lauer, J. G. (2007). Corn stalk response to plant population and the Bt-European corn borer trait. Agron. J. 99, 657–664. doi: 10.2134/agronj2006.0079
Steffey, K. L., and Gray, M. E. (2002). Management of field crop insect pests, in Illinois Agronomy Handbook, 23rd Edn, eds R. G. Hoeft and E. D. Nafziger (Urbana: University of Illinois), 275–293.
Stein, O., and Granot, D. (2019). An overview of sucrose synthases in plants. Front. Plant Sci. 10:95. doi: 10.3389/fpls.2019.00095
Sun, Q., Liu, X., Yang, J., Liu, W., Du, Q., Wang, H., et al. (2018). MicroRNA528 affects lodging resistance of maize by regulating lignin biosynthesis under nitrogen-luxury conditions. Mol. Plant. 11, 806–814. doi: 10.1016/j.molp.2018.03.013
Sun, S., Dai, J., and Gu, W. (1989). Effect of nitrogen, phosphate and potash fertilizers on lodging and yield in maize. Sci. Agric. Sin. 22, 28–33.
Tanaka, K., Murata, K., Yamazaki, M., Onosato, K., Miyao, A., and Hirochika, H. (2003). Three distinct rice cellulose synthase catalytic subunit genes required for cellulose synthesis in the secondary wall. Plant Physiol. 133:73. doi: 10.1104/pp.103.022442
Tanveer, M. (2020). Tissue-specific reactive oxygen species signalling and ionic homeostasis in Chenopodium quinoa and Spinacia oleracea in the context of salinity stress tolerance (Doctoral dissertation). University of Tasmania. Available online at: https://eprints.utas.edu.au/35306
Tao, Z., Sui, P., Yuan-quan, C., Chao, L. I., Zi jin, N. I. E., Shufen, Y., et al. (2013). (ASC-2012-0679) Subsoiling and ridge tillage alleviate the high temperature stress in spring maize in the north china plain. J. Integr. Agric. 1303. doi: 10.1016/S2095-3119(13)60347-0
Tardieu, F., Parent, B., Caldeira, C. F., and Welcker, C. (2014). Genetic and physiological controls of growth under water deficit. Plant Physiol. 164, 1628–1635. doi: 10.1104/pp.113.233353
Teetor, V. H., Schmalzel, C., and Ray, D. T. (2017). Growing sweet sorghum (Sorghum bicolor L. moench) in clumps potentially reduces lodging in the arid-southwestern United States. Ind. Crops Prod. 107, 458–462. doi: 10.1016/j.indcrop.2017.05.064
Testa, G., Reyneri, A., and Blandino, M. (2016). Maize grain yield enhancement through high plant density cultivation with different inter-row and intra-row spacings. Eur. J. Agron. 72, 28–37. doi: 10.1016/j.eja.2015.09.006
Thomas, H., and Howarth, C. J. (2000). Five ways to stay green. J. Exp. Bot. 51, 329–337. doi: 10.1093/jexbot/51.suppl_1.329
Thompson, D. L. (1963). Stalk strength of corn as measured by crushing strength and rind thickness1. Crop Sci. 4, 323–329. doi: 10.2135/cropsci1963.0011183X000300040013x
Tokatlidis, I. S., Has, V., Melidis, V., Has, I., Mylonas, I., Evgenidis, G., et al. (2011). Maize hybrids less dependent on high plant densities improve resource-use efficiency in rainfed and irrigated conditions. Field Crops Res. 120, 345–351. doi: 10.1016/j.fcr.2010.11.006
Troyer, A. F., and Rosenbrook, R. W. (1983). Utility of higher plant densities for corn performance testing. Crop Sci. 23, 863–867. doi: 10.2135/cropsci1983.0011183X002300050011x
Ur Rehman, A., and Ishaque, M. (2011). Potassium application reduces bareness in different maize hybrids under crowding stress conditions. Pak. J. Agri. Sci. 48, 41–48.
Uribelarrea, M., Cárcova, J., Otegui, M. E., and Westgate, M. E. (2002). Pollen production, pollination dynamics, and kernel set in maize. Crop Sci. 42, 1910–1918. doi: 10.2135/cropsci2002.1910
Valentinuz, O. R., and Tollenaar, M. (2004). Vertical profile of leaf senescence during the grain-filling period in older and newer maize hybrids. Crop Sci. 44, 827–834. doi: 10.2135/cropsci2004.8270
Van Ittersum, M. K., and Cassman, K. G. (2013). Yield gap analysis-Rationale, methods and applications- Introduction to the Special Issue. Field Crops Res. 143, 1–3. doi: 10.1016/j.fcr.2012.12.012
Vega, C. R. C., Andrade, F., and Sadras, V. (2001a). Reproductive partioning and seed set efficiency in soybean, sunflower and maize. Field Crops Res. 72, 163–175. doi: 10.1016/S0378-4290(01)00172-1
Vega, C. R. C., Andrade, F., Sadras, V., Uhart, S. A., and Valentinuz, O. (2001b). Seed number as a function of growth. a comparative study in soybean, sunflower, and maize. Crop Sci. 41, 748–754. doi: 10.2135/cropsci2001.413748x
Wang, C., Hu, D., Liu, X., She, H., Ruan, R., Yang, H., et al. (2015). Effects of uniconazole on the lignin metabolism and lodging resistance of culm in common buckwheat (Fagopyrum esculentum M.). Field Crops Res. 180, 46–53. doi: 10.1016/j.fcr.2015.05.009
Wang, J., Zhu, J., Lin, Q., Li, X., Teng, N., Li, Z., et al. (2006). Effects of stem structure and cell wall components on bending strength in wheat. Chinese Sci. Bull. 51, 815–823. doi: 10.1007/s11434-006-0815-z
Wang, N., Li, F. H., Wang, Z. B., Wang, H. W., Lv, X. L., Zhou, Y. F., et al. (2011). Response to plant density of stem characters of maize hybrids and its relationship to lodging (In Chinese). Crops 3, 67–80.
Wang, Q., Xue, J., Zhang, G., Chen, J., Xie, R., Ming, B., et al. (2020). Nitrogen split application can improve the stalk lodging resistance of maize planted at high density. Agriculture 10:364. doi: 10.3390/agriculture10080364
Wang, Q. Y., and Hu, C. H. (1991). Studies on the anatomical structures of the stalks of maize with different resistance to lodging. Acta Agron. Sin. 70–75.
Wang, T., Zhang, L., Han, Q., Zheng, F., Wang, T., Feng, N., et al. (2015). Effects of stalk cell wall and tissue on the compressive strength of maize. Plant Sci. J. 33, 109–115. doi: 10.11913/PSJ.2095-0837.2015.10109
Wang, Y., and Frei, M. (2012). Stressed food-The impact of abiotic environmental stresses on crop quality. Agric. Ecosyst Environ. 141, 271–286. doi: 10.1016/j.agee.2011.03.017
Weber, H., Borisjuk, L., and Wobus, U. (2005). Molecular physiology of legume seed development. Ann. Rev. Plant Biol. 56, 253–279. doi: 10.1146/annurev.arplant.56.032604.144201
Wei, F. Z., Li, J. C., Wang, C. Y., Qu, H. J., and Shen, X. S. (2008). Effects of nitrogenous fertilizer application model on culm lodging resistance in winter wheat. Acta Agron. Sin. 34, 1080–1085. doi: 10.3724/SP.J.1006.2008.01080
Wei, Z., Wei, Z., Qu, Z., Zhang, L., Zhao, S., Bi, Z., et al. (2015). Overexpression of poplar xylem sucrose synthase in tobacco leads to a thickened cell wall and increased height. PLoS ONE 10:e0120669. doi: 10.1371/journal.pone.0120669
Wen, W., Gu, S., Xiao, B., Wang, C., Wang, J., Ma, L., et al. (2019). In situ evaluation of stalk lodging resistance for different maize (Zea mays L.) cultivars using a mobile wind machine. Plant Methods 15, 1–16. doi: 10.1186/s13007-019-0481-1
Wong, J. H., Kato, T., Belteton, S. A., Shimizu, R., Kinoshita, N., Higaki, T., et al. (2019). Basic proline-rich protein-mediated microtubules are essential for lobe growth and flattened cell geometry. Plant Physiol. 181, 1535–1551. doi: 10.1104/pp.19.00811
Worku, M., Bänziger, M., Erley, G. S., Friesen, D., Diallo, A. O., and Horst, W. J. (2012). Nitrogen efficiency as related to dry matter partitioning and root system size in tropical mid-altitude maize hybrids under different levels of nitrogen stress. Field Crops Res. 130, 57–67. doi: 10.1016/j.fcr.2012.02.015
Wu, W., Huang, J., Cui, K., Nie, L., Wang, Q., Yang, F., et al. (2012). Sheath blight reduces stem breaking resistance and increases lodging susceptibility of rice plants. Field Crops Res. 128, 101–108. doi: 10.1016/j.fcr.2012.01.002
Wu, W., and Ma, B. L. (2018). Assessment of canola crop lodging under elevated temperatures for adaptation to climate change. Agric. For. Meteorol. 248, 329–338. doi: 10.1016/j.agrformet.2017.09.017
Wu, W., and Ma, B. L. (2019). Erect-leaf posture promotes lodging resistance in oat plants under high plant population. Eur. J. Agron. 103, 175–187. doi: 10.1016/j.eja.2018.12.010
Wu, W., and Ma, B. L. (2020). The mechanical roles of the clasping leaf sheath in cereals: two case studies from oat and wheat plants. J. Agron. Crop Sci. 206, 118–129. doi: 10.1111/jac.12362
Wu, W., Shah, F., Duncan, R. W., and Ma, B. L. (2020). Grain yield, root growth habit and lodging of eight oilseed rape genotypes in response to a short period of heat stress during flowering. Agric. For. Meteorol. 287:107954. doi: 10.1016/j.agrformet.2020.107954
Xiao, G., Zhang, Q., Yao, Y., Zhao, H., Wang, R., Bai, H., et al. (2008). Impact of recent climatic change on the yield of winter wheat at low and high altitudes in semi-arid northwestern China. Agric. Ecosyst. Environ. 127, 37–42. doi: 10.1016/j.agee.2008.02.007
Xu, C., Gao, Y., Tian, B., Ren, J., Meng, Q., and Wang, P. (2017). Effects of EDAH, a novel plant growth regulator, on mechanical strength, stalk vascular bundles and grain yield of summer maize at high densities. Field Crops Res. 200, 71–77. doi: 10.1016/j.fcr.2016.10.011
Xu, N., York, K., Miller, P., and Cheikh, N. (2004). Co-regulation of ear growth and internode elongation in corn. Plant Growth Regul. 44, 231–241. doi: 10.1007/s10725-004-5935-3
Xue, J., Gou, L., Zhao, Y., Yao, M., Yao, H., Tian, J., et al. (2016a). Effects of light intensity within the canopy on maize lodging. Field Crops Res. 188, 133–141. doi: 10.1016/j.fcr.2016.01.003
Xue, J., Li, L., Xie, R., Wang, K., Hou, P., Ming, B., et al. (2018). Effect of lodging on maize grain losing and harvest efficiency in mechanical grain harvest. Acta Agron. Sin. 44, 1774–1781. doi: 10.3724/SP.J.1006.2018.01774
Xue, J., Ming, B., Xie, R., Wang, K., Hou, P., and Li, S. (2020). Evaluation of maize lodging resistance based on the critical wind speed of stalk breaking during the late growth stage. Plant Methods 16:148. doi: 10.1186/s13007-020-00689-z
Xue, J., Zhao, Y., Gou, L., Shi, Z., Yao, M., and Zhang, W. (2016b). How high plant density of maize affects basal internode development and strength formation. Crop Sci. 56, 3295–3306 doi: 10.2135/cropsci2016.04.0243
Yan, P., Pan, J., Zhang, W., Shi, J., Chen, X., and Cui, Z. (2017). A high plant density reduces the ability of maize to use soil nitrogen. PLoS ONE 12:0172717. doi: 10.1371/journal.pone.0172717
Yan, Y., Liao, C., Zhang, F., and Li, C. (2010). The causal relationship of the decreased shoot and root growth of maize plants under higher planting density. Plant Nutr. Fertil. Sci. 16, 257–265.
Yang, S., Xie, L., Zheng, S., Li, J., and Yuan, J. (2009). Effects of nitrogen rate and transplanting density on physical and chemical characteristics and lodging resistance of culms in hybrid rice. Acta Agron. Sin. 35, 93–103. doi: 10.3724/SP.J.1006.2009.00093
Yang, X., Song, L., and Xue, H. W. (2008). Membrane steroid binding protein 1 (MSBP1) stimulates tropism by regulating vesicle trafficking and auxin redistribution. Mol. Plant 1, 1077–1087. doi: 10.1093/mp/ssn071
Yokozawa, M., and Hara, T. (1995). Foliage profile, size structure and stem diameter-plant height relationship in crowded corn plant population. Ann. Bot. 76, 271–285. doi: 10.1006/anbo.1995.1096
Yu, S. M., Lo, S. F., and Ho, T. D. (2015). Source–sink communication: regulated by hormone, nutrient, and stress cross-signaling. Trends Plant Sci. 20, 844–857. doi: 10.1016/j.tplants.2015.10.009
Yuan, L., Tang, J., Wang, X., and Li, C. (2012). QTL analysis of shading sensitive related traits in maize under two shading treatments. PLoS ONE 7:e38696. doi: 10.1371/journal.pone.0038696
Zeng, Q., Jiang, L., Wang, D., Huang, S., and Yang, D. (2012). Camptothecin and 10- hydroxycamptothecin accumulation in tender leaves of Camptotheca acuminata saplings after treatment with plant growth regulators. Plant Growth Regul. 68, 467–473. doi: 10.1007/s10725-012-9736-9
Zhang, C., Han, L., Slewinski, T. L., Sun, J., Zhang, J., Wang, Z. Y., et al. (2014). Symplastic phloem loading in poplar. Plant Physiol. 166, 306–313. doi: 10.1104/pp.114.245845
Zhang, F. Z., Jin, Z. X., Ma, G. H., Shang, W. N., Liu, H. Y., Xu, M. L., et al. (2010). Relationship between lodging resistance and chemical contents in culms and sheaths of japonica rice during grain filling. Rice Sci. 17, 311–318. doi: 10.1016/S1672-6308(09)60032-9
Zhang, J., Li, G. H., Song, Y. P., Zhang, W. J., Yang, C. D., Wang, S. H., et al. (2013). Lodging resistance of super-hybrid rice Y Liangyou 2 in two ecological regions. Acta Agron. Sin. 39, 682–692. doi: 10.3724/SP.J.1006.2013.00682
Zhang, Q., Zhang, L., Evers, J., van der Werf, W., Zhang, W., and Duan, L. (2014). Maize yield and quality in response to plant density and application of a novel plant growth regulator. Field Crop Res. 164, 82–89. doi: 10.1016/j.fcr.2014.06.006
Zhang, W., Yu, C., Zhang, K., Zhou, Y., Tan, W., Zhang, L., et al. (2017). Plant growth regulator and its interactions with environment and genotype affect maize optimal plant density and yield. Eur. J. Agron. 91, 34–43. doi: 10.1016/j.eja.2017.09.008
Zhang, W. F., Cao, G. X., Li, X. L., Zhang, H. Y., Wang, C., Liu, Q. Q., et al. (2016). Closing yield gaps in China by empowering smallholder farmers. Nature 537, 671–674. doi: 10.1038/nature19368
Zhang, X., Cheng, J., Wang, B., Yan, P., Dai, H., Chen, Y., et al. (2019). Optimum sowing dates for high-yield maize when grown as sole crop in the North China Plain. Agronomy 9:198. doi: 10.3390/agronomy9040198
Zhang, X. J., Li, H. J., Li, W. J., Xu, Z. J., Chen, W. F., Zhang, W. Z., et al. (2009). The lodging resistance of erect panicle japonica rice in northern China. Sci. Agric. Sin. 2305–2313.
Zhang, Y., Liu, P., Zhang, X., Zheng, Q., Chen, M., Ge, F., et al. (2018). Multi-locus genome-wide association study reveals the genetic architecture of stalk lodging resistance-related traits in maize. Front Plant Sci. 9:611. doi: 10.3389/fpls.2018.00611
Zhao, D. L., Oosterhuis, D. M., and Bednarz, C. W. (2001). Influences of potassium deficiency on photosynthesis, chlorophyll content, and chloroplast ultrastructure of cotton plants. Photosynthetica 39, 103–199. doi: 10.1023/A:1012404204910
Zhao, Y. J., Sen, X., Zhang, Q. S., and Zhang, F. S. (2019). Causes of maize density loss in farmers' fields in Northeast China. J. Integr. Agric. 18, 1680–1689. doi: 10.1016/S2095-3119(18)62101-X
Zheng, K., Wang, Y., and Wang, S. (2019). The non-DNA binding bHLH transcription factor Paclobutrazol Resistances are involved in the regulation of ABA and salt responses in Arabidopsis. Plant Physiol. Biochem. 139, 239–245. doi: 10.1016/j.plaphy.2019.03.026
Zhou, B., Yue, Y., Sun, X., Ding, Z., Ma, W., and Zhao, M. (2017). Maize kernel weight responses to sowing date-associated variation in weather conditions. Crop J. 5, 43–51. doi: 10.1016/j.cj.2016.07.002
Zhu, X. K., Wang, X. J., Guo, K. Q., Guo, W. S., Feng, C. N., and Peng, Y. X. (2006). Stem characteristics of wheat with stem lodging and effects of lodging on grain yield and quality. J. Trit. Crops.
Zinselmeier, C., Jeong, B. R., and Boyer, J. S. (1999). Starch and the control of kernel number in maize at low water potentials. Plant Physiol. 121, 25–36. doi: 10.1104/pp.121.1.25
Zuber (1980). Effect of recurrent selection for crushing strength on several stalk components in maize. Crop Sci. 20, 711–717. doi: 10.2135/cropsci1980.0011183X002000060009x
Zuber, M. S., and Grogan, C. O. (1961). A new technique for measuring stalk strength in corn. Crop Sci. 1, 378–380. doi: 10.2135/cropsci1961.0011183X000100050028x
Keywords: kernel abortion, maize, lodging, sugar metabolism, management, stem lodging, field management, grain yield
Citation: Shah AN, Tanveer M, Abbas A, Yildirim M, Shah AA, Ahmad MI, Wang Z, Sun W and Song Y (2021) Combating Dual Challenges in Maize Under High Planting Density: Stem Lodging and Kernel Abortion. Front. Plant Sci. 12:699085. doi: 10.3389/fpls.2021.699085
Received: 22 April 2021; Accepted: 13 September 2021;
Published: 02 November 2021.
Edited by:
Yared Assefa, Kansas State University, United StatesReviewed by:
Wei Wu, Hainan University, ChinaJun Xue, Chinese Academy of Agricultural Sciences (CAAS), China
Copyright © 2021 Shah, Tanveer, Abbas, Yildirim, Shah, Ahmad, Wang, Sun and Song. This is an open-access article distributed under the terms of the Creative Commons Attribution License (CC BY). The use, distribution or reproduction in other forums is permitted, provided the original author(s) and the copyright owner(s) are credited and that the original publication in this journal is cited, in accordance with accepted academic practice. No use, distribution or reproduction is permitted which does not comply with these terms.
*Correspondence: Youhong Song, y.song@ahau.edu.cn