- 1Department of Plant Sciences, College of Agriculture and Bioresources, University of Saskatchewan, Saskatoon, SK, Canada
- 2Department of Agricultural, Food and Nutritional Science, Faculty of Agricultural, Life and Environmental Sciences, University of Alberta, Edmonton, AB, Canada
To develop crops capable of withstanding challenges posed by climate change, breeding strategies must focus on addressing multiple stresses occurring concurrently in plants. Leaf epidermal structures such as trichomes, stomata, and epidermal cells play an important role in mediating plant defense and could be essential traits that impart wide-ranging tolerance to biotic and abiotic stresses. Consequently, it is important to inform on the underlying diversity in these traits in lentil germplasm (Lens spp.). In this study, we characterized foliar microstructures of 12 genotypes belonging to seven wild and cultivated Lens species. We performed scanning electron microscopy on leaflet and pod surfaces for their qualitative characterization. For quantitative characterization, we observed surface imprints via light microscopy and quantified trichome density (TD), trichome length (TL), stomatal density (SD), epidermal cell density (ECD), and stomatal index (SI) on adaxial and abaxial leaflet surfaces for each genotype. We also assessed the heritability of trichome traits by evaluating interspecific recombinant inbred lines (RILs) derived from the cross Lens culinaris CDC Redberry × Lens tomentosus IG 72805. Comparing foliar microstructures, we found that TD and TL varied widely among cultivated and wild lentil genotypes. However, in most lentil genotypes, the adaxial leaflet surface had lower TD and longer trichomes compared to the abaxial surface. Pubescence on pods comprised five major phenotypes: no trichomes or glabrous pods, very short trichomes at low density, short trichomes at high density, medium-length trichomes at high density, and long trichomes at high density. Leaves of all species were amphistomatous, and SI, SD, and ECD were all higher on the adaxial compared to the abaxial surface. Adaxial surfaces had slightly sunken stomata, which might be an adaptive trait to conserve water. Quantifying TD and TL on the leaflets of interspecific RILs revealed transgressive segregation of these traits, suggesting that TD and TL are quantitative in nature. While taxonomic implications of this study are limited, a detailed description of agronomically relevant morphophysiological traits presented in this paper along with the mode of inheritance of trichomes may serve as a resource for scientists developing lentil adapted to concurrent biotic and abiotic stresses of the future.
Introduction
Lentil (Lens culinaris subsp. culinaris Medikus) is a globally important crop due to its economic, ecological, and nutritive qualities. Worldwide, lentil production exceeds 5.8 million tons, and lentil is cultivated for domestic use and export in 44 countries with various climates spanning six continents (FAO, 2021). Lentil is a legume crop capable of biological nitrogen fixation, which reduces N fertilizer use and increases the yield of subsequent crops (Migliozzi et al., 2015; Niu et al., 2017). The inclusion of lentil in oilseed–cereal rotation systems has also been demonstrated to increase the crop productivity and stabilize yields in changing environments (Liu et al., 2019). Globally, lentil consumption is increasing four to five times more rapidly than other pulse crops because of its relative ease of dehulling and its rapid cooking time (Khazaei et al., 2019). Lentil is relatively inexpensive, packed with essential amino acids, vitamins, and minerals, and has long been consumed in Mediterranean, Middle Eastern, and Afro-Asian cultures (Iqbal et al., 2006; Yadav et al., 2007). Its role as a potential replacement for animal-sourced protein is now being evaluated as a part of a global shift toward environmentally sustainable food systems (Jarpa-Parra, 2018; Warne et al., 2019).
Genus Lens (Miller) of the family Leguminosae consists of seven taxa that were classified into four species based on morphological and genomic differences (Ferguson et al., 2000): the cultivated lentil, L. culinaris subsp. culinaris, and six wild lentil taxa, L. culinaris subsp. orientalis, L. culinaris subsp. tomentosus (Ladizinsky) Ferguson, Maxted, van Slageren and Robertson, L. culinaris subsp. odemensis (Ladizinsky) Ferguson, Maxted, van Slageren and Robertson, L. lamottei Czefr., L. ervoides (Brign.) Grande, and L. nigricans (M. Bieb.) Godron. Most recently, based on genotyping-by-sequencing, Wong et al. (2015) suggested that the seven Lens taxa should be separated into four gene pools based on their ability to produce viable seeds after hybridization with cultivated lentil. They found L. odemensis to be distinct from L. culinaris and suggested that it should be a separate species instead of being a L. culinaris subspecies (Wong et al., 2015). According to the latest classification by Wong et al. (2015), the four gene pools are primary gene pool (L. culinaris, L. orientalis, and L. tomentosus), which have high interspecific crossability with each other; secondary gene pool (L. lamottei and L. odemensis); tertiary gene pool (L. ervoides); and quaternary gene pool (L. nigricans). Species in the same gene pool are reproductively compatible and produce viable seed, whereas species from different gene pools may not hybridize easily due to embryo abortion (Davies et al., 2007). L. nigricans (quaternary gene pool) is the most genetically distant from the cultivated lentil and has not been successfully crossed with L. culinaris (Ogutcen et al., 2018) or any other species.
Lentil is an annual, herbaceous, self-pollinating cool-season food legume with cleistogamous flowers and indeterminate growth habit (Smýkal et al., 2015). The lentil species are naturally distributed widely across diverse landscapes from the Western Mediterranean to Central Asia, an immense east–west region characterized physically by changes in elevation from sea level to high mountains at mid-latitudes. L. orientalis is considered the putative wild progenitor of cultivated lentil and was domesticated in the Fertile Crescent (South Eastern Turkey–Northern Syria) (Sonnante et al., 2009). L. orientalis is widely distributed along the steppes and dry areas of central and southwest Asia and from Turkey to Uzbekistan (Ladizinsky, 1979). Other wild lentil species have a wide, mostly Mediterranean geographical distribution, extending from Southern France to Spain to Morocco, and from Turkey, Syria, Iraq, to Uzbekistan (Smýkal et al., 2015). Wild lentil species are distributed across habitats ranging from open or partially shaded (L. orientalis, L. tomentosus, L. odemensis, and L. nigricans) to mostly shady, such as in pine forests (L. ervoides) (Ferguson and Erskine, 2001). The lentil species also exhibit winter and spring growth habit. It is presumed that during the domestication process, cultivated lentil went through population bottlenecks that narrowed their genetic base (Erskine et al., 1998). Its genetically accessible wild relatives have so far been mainly considered potential sources of genetic diversity and may be of value in breeding for resistance to biotic stresses, mostly for resistance to fungal plant pathogens (Coyne et al., 2020).
Increasing concerns about changes in climate have resulted not only in a shift toward plant-based foods, but also in additional interest in the capacity to breed food crops with greater resilience to climate change. It is becoming increasingly evident that global warming will not only result in an increase in biotic and abiotic stress in plants, but also unique combinations of two or more of these stresses (Pandey et al., 2017). Two of the major stresses of the future that are interlinked and interact with each other include increased pathogen stress and increased severity and frequency of drought (Szczepaniec and Finke, 2019). Recent studies of lentil species show that they employ various strategies to combat abiotic stresses, especially drought (Gorim and Vandenberg, 2017). Trichomes are distinctly more noticeable on L. tomentosus, which was also shown to transpire less water under severe drought stress (Gorim and Vandenberg, 2017, 2018). Various reviews shed light on the role of trichomes and stomata of plants in conferring tolerance to salt stress (Shabala, 2013), water stress (Arve et al., 2011), ozone stress (Oksanen, 2018), insect herbivory (Zavala et al., 2013; Kaur and Kariyat, 2020), pathogens (Melotto et al., 2008), and several other biotic and abiotic stresses (Karabourniotis et al., 2020). Epidermal cell size and number have also been shown to be affected by drought stress (McCree and Davis, 1974; Cutler et al., 1977; Bosabalidis and Kofidis, 2002; Lechner et al., 2008), root penetration stress (Beemster and Masle, 1996), and salt stress (Curtis and Läuchli, 1987). Genetic improvement strategies that include potential benefits of use of traits such as trichome morphology and stomatal characteristics may contribute to the development of lentil germplasm with increased stress tolerance. To date, however, there are no studies that characterize the trichomes, stomata, and epidermal cells of lentil species that could allow the development of breeding strategies to improve the resilience of the cultivated lentil.
In this study, we explored the existing diversity on the surfaces of leaflets and pods in wild and cultivated Lens spp. with respect to the traits of trichomes, stomata, and epidermal cells, with the goal that these morphophysiological traits will be useful to agronomists and breeders looking to identify or develop cultivars better suited to withstand a combination of biotic and abiotic stresses. We examined the foliar surfaces of 12 wild and cultivated lentil genotypes representative of the range of variation across the lentil species. We used the techniques of scanning electron microscopy and Suzuki’s Universal Micro-Printing (SUMP) method (Tanaka et al., 2005), which uses light microscopy, to visualize and characterize surface microstructures. Scanning electron microscopy has been previously used to study leaf epidermal traits to distinguish between and identify different taxa (Esfandani-Bozchaloyi and Zaman, 2018; Bahadur et al., 2019; Gul et al., 2019), and the SUMP method has been used in previous studies to visualize stomatal and epidermal cell anatomy and morphology (Tanaka et al., 2005; Arve et al., 2013; Banik et al., 2016; Carvalho et al., 2016). In this study, we used the imprints obtained from the SUMP method for quantitative characterization, while scanning electron microscopy was used for qualitative characterization of surface microstructural traits in leaflets and pods of Lens spp. While the literature exists on qualitative differences in leaf pubescence of cultivated lentil (Kumar and Solanki, 2014), this trait has not been quantitatively compared across wild and cultivated lentil species. Literature is also sparse on stomatal frequency, stomatal index (SI), and epidermal cell characteristics in lentil germplasm. These basic morphological traits can influence factors such as pathogen entry, insect herbivory, and water-use efficiency, which further influence seed yield and adaptation. Our study fills this gap in the literature and aims to quantitatively assess the variability across 12 wild and cultivated lentil genotypes. This study also quantifies the trichome density (TD) and trichome length (TL) in recombinant inbred lines (RILs) of the hybrid progeny of L. culinaris × L. tomentosus, informing on the inheritance of the trait of pubescence in lentil leaflets.
Materials and Methods
Plant Material and Experimental Design
The study was conducted under controlled conditions in the Phytotron facility of the College of Agriculture and Bioresources at the University of Saskatchewan, Saskatoon, Canada (52°07′58.8″N, 106°37′51.6″W). Table 1 shows the wild and cultivated lentil genotypes evaluated in the experiment. Prior to planting, all seeds were scarified, washed in bleach and Tween, and germinated in 50-ml Erlenmeyer flasks in the dark at 22°C. Germinated seedlings were transplanted into 9-L plastic pots filled with Sunshine Mix 4 (Sun Gro Horticulture, Canada) and kept in a Conviron GR48 growth chamber (Conviron, Winnipeg, Canada). About 500 mL of Hoagland solution was added to each pot before planting. The Hoagland solution contained 5 mM KNO3, 5 mM Ca(NO3)2, 2 mM MgSO4.7H2O, 2 mM KH2PO4, 45 μM Fe chelate (containing FeSO4.7H2O and EDTA 2Na.2H2O), and micronutrients (9.1 μM MnCl2.4H2O, 46.3 μM H3BO3, 0.76 μM ZnSO4.7H2O, 0.32 μM CuSO4.5H2O, and 0.1 μM Na2MoO4.2H2O).
The experiment was set up as a randomized complete block design with 12 genotypes and eight replicates. The chamber was set to 16-h day at 21°C, 8-h night at 15°C, and at ambient humidity. Light intensity ranged from 276 to 441 μmol m–2 s–1 depending on the height of the canopy and placement of the pot. Minimum temperature and maximum temperature during the day were 16.4 and 29.2°C, respectively, and the minimum temperature and maximum temperature during the night were 9.8 and 16.9°C, respectively. Plants were maintained under fully watered conditions for the duration of the study from January 26, 2018 to April 26, 2018.
The interspecific NAM 38 population was developed from the hybrid L. cul. CDC Redberry × L. tom. IG 72805, and single seed descent of 70 seeds was performed from the F2 population for four to six generations. The CDC Redberry parent seed was derived from the inbred seed source that was sequenced to produce the lentil reference genome available at the KnowPulse web portal (Vandenberg et al., 2006; Sanderson et al., 2019). There were 70 RILs of NAM 38 used, varying from the F4 to F6 generation of inbreeding. The NAM 38 lines were grown in the University of Saskatchewan Agriculture Greenhouse in Saskatoon, SK, Canada (52°08′21.3″N, 106°37′54.3″W) from October to December 2018. Temperature was set at 25°C/18°C day/night under a 16-h photoperiod. Temperature during the day ranged from 20.4 to 32.8°C, and the temperature during the night ranged from 16.2 to 21.8°C.
Collection of Leaf Surface Imprints
Imprints of leaf surfaces were made using SUMP disks and SUMP liquid (Sump Laboratory, Tokyo, Japan) as previously described in the study by Tanaka et al. (2005). At the early pod development (R3) stage (Erskine et al., 1990), three plants from each genotype were selected at random for leaf imprinting. Imprints of adaxial and abaxial surfaces of the youngest fully expanded leaflets were then made for a total of 36 plants belonging to the 12 genotypes. For the 70 individuals of the NAM 38 population, imprints were made only of the adaxial surface of the youngest fully expanded terminal leaflets. One leaflet imprint was made for each individual in the population. The impressions of leaflet surfaces were visualized and analyzed with the aid of an EVOS FL inverted microscope (Mill Creek, Washington, United States). Three fields of view were captured for each leaflet sample for both adaxial and abaxial surfaces. Scale for each image was automatically generated by the microscope at the time of visualization. The data obtained for each image were TD, TL, stomatal density (SD), and epidermal cell density (ECD). In a selected area of leaf surface, the number of trichomes, stomata, and epidermal cells was counted manually and subsequently measured using ImageJ software1. Figure 1 shows a representative imprint image used to quantify micromorphological surface traits.
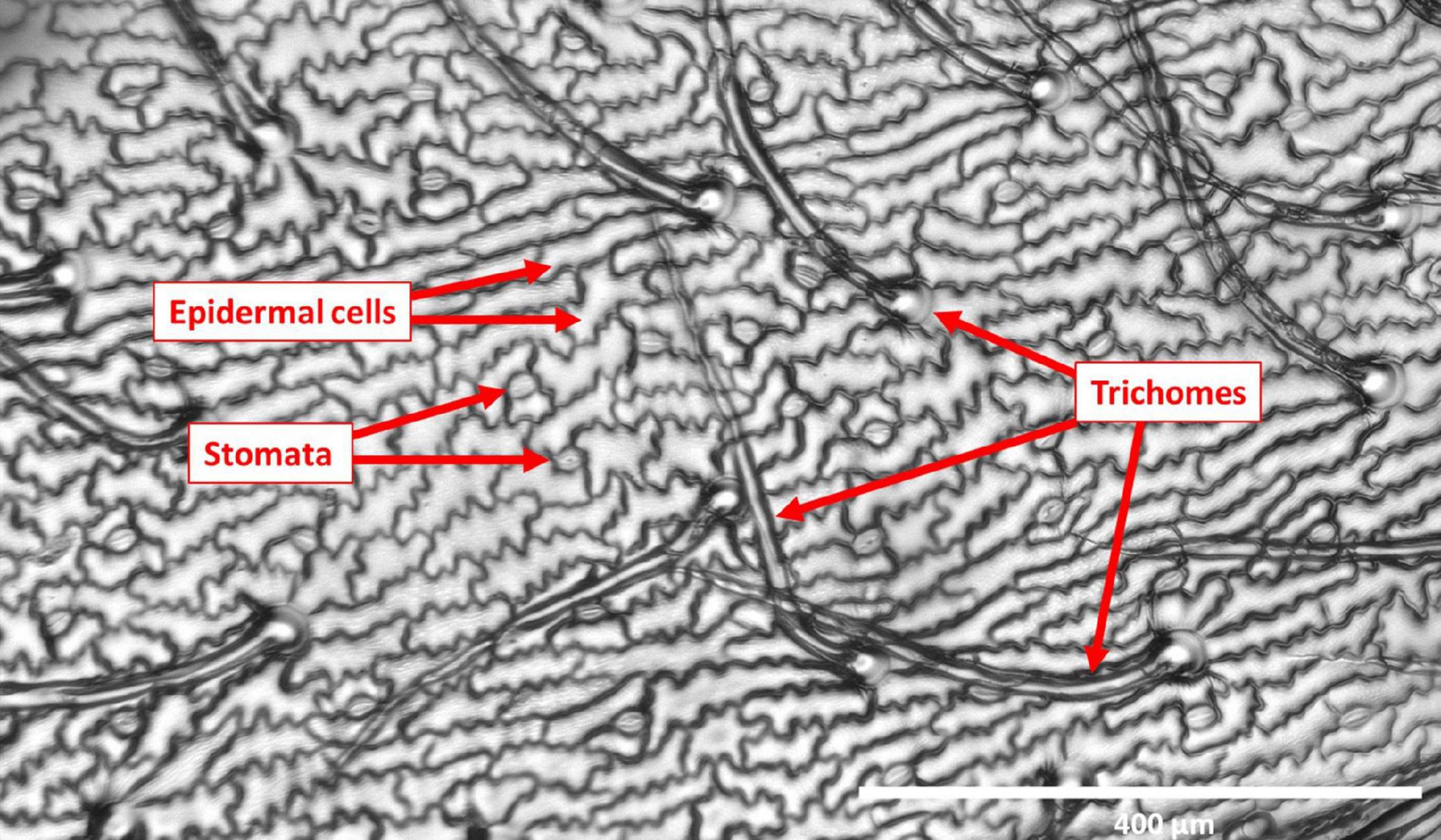
Figure 1. Representative image of a leaflet imprint at 10X magnification used to quantify micromorphological traits on the surface. Scale bar in image = 400 μm.
Trichome length was measured by tracing along the trichomes and using the “Length” measurement on ImageJ using the calibrated scale bar on the images. SIs were then calculated for each genotype using the formula introduced by Salisbury and Oliver (1928):
Scanning Electron Microscopy
Scanning electron microscopy was conducted to visualize the qualitative differences in morphology and topography among leaflets and pods of the 12 lentil genotypes. The youngest fully expanded leaflets of the rachis were sampled. Pods were sampled at the R4 (flat pod) stage (Erskine et al., 1990). Leaf and pod samples were fixed in 100% ethanol at 4°C for at least 24 h. The samples were then subjected to critical point drying using CO2 at approximately 31.5°C and 1200 psi (lb/in2) in a Polaron E3000 Critical Point Dryer (Quorum Technologies Ltd., East Sussex, United Kingdom), then gold-coated in Edwards S150B Sputter Coater (BOC Edwards, United Kingdom), and examined using a Phenom G2 pure desktop SEM (Phenom-World, Eindhoven, Netherlands). Leaflet and pod samples were imaged at 180–185X magnification in Topographic mode for leaflets and normal (full) mode for pods.
Data Analyses
Data for TD, TL, ECD, SD, and SI were fitted using a linear mixed model with the interaction between surface and genotype as a fixed variable and blocking as the random variable. All analyses were done using R Statistical Software (R Core Team, 2019), and significant differences in response variables were calculated using the least-square means method for multiple comparisons at alpha = 5% and adjusting p-value using the Tukey’s method after ensuring homogeneity of variances through Levene’s test from the package “car” (Fox and Weisberg, 2019). The lme function from the package lme4 was used to fit the model, and the function lsmeans from the package emmeans was used to calculate the least-square means (Bates et al., 2015; Lenth, 2019). Data were plotted using SigmaPlot version 11.0 (Systat Software, San Jose, CA, United States).
Results
Characterizing Trichomes in Lentil Genotypes
Scanning electron microscopy revealed that trichomes of all lentil genotypes were simple, unicellular, tapered, non-glandular hair-like structures (Figures 2A–L). Distribution of trichomes was even across the entire leaflet surface in all genotypes except L. lam. IG 110813 and L. erv. L-01-827A, where more trichomes were clustered along the midrib compared to other areas. Trichomes on pods were categorized into five phenotype classes based on visual observations: no trichomes or glabrous pods (L. cul. Indianhead), very short trichomes at low density (L. cul. CDC Redberry), short trichomes at high density (L. erv. L-01-827A), medium-length trichomes at high density (L. tom. IG 72613), and long trichomes at high density (L. tom. IG 72805) (Figures 2I–V, respectively).
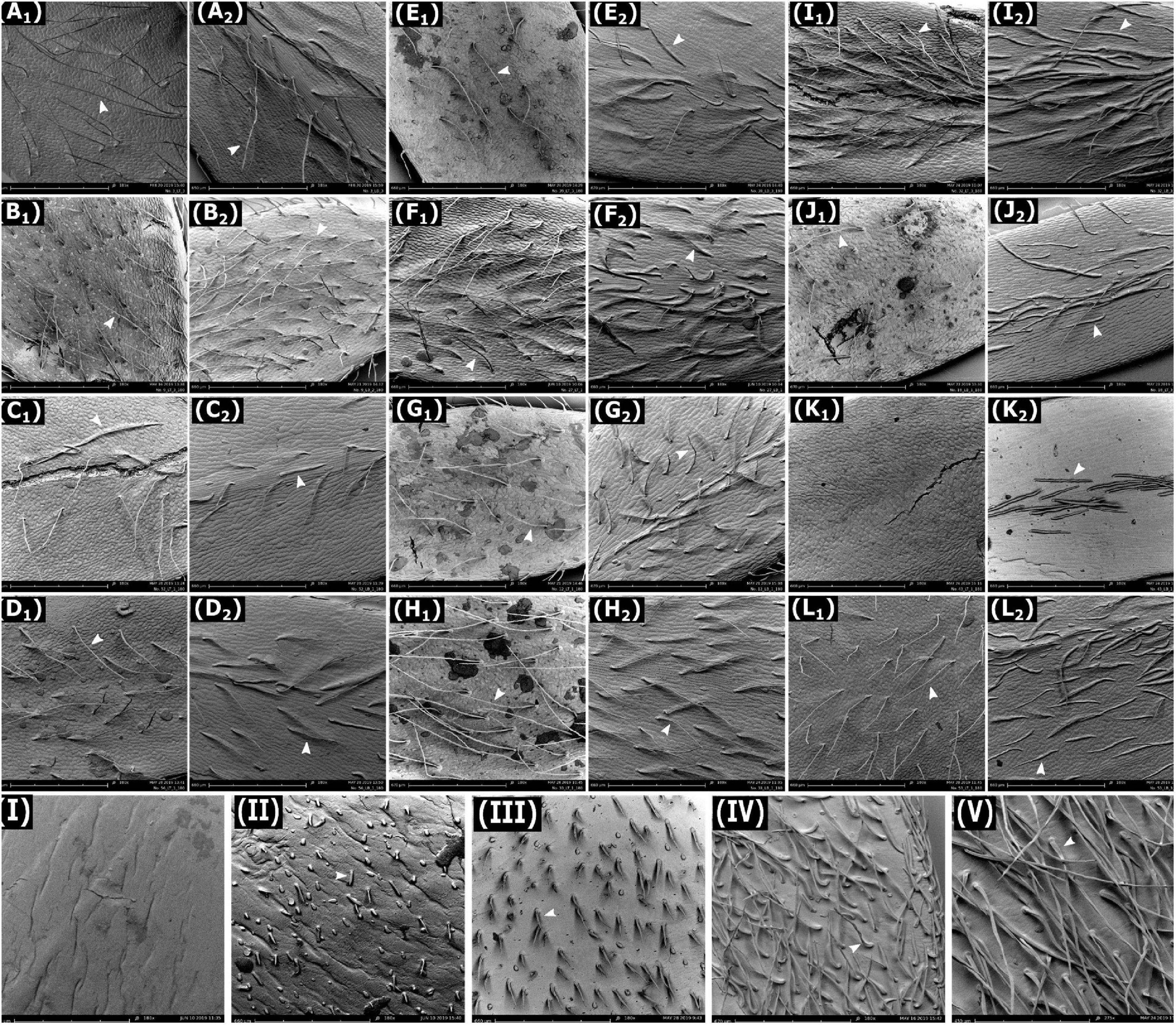
Figure 2. Scanning electron microscopy images of adaxial (indicated as subscript 1) and abaxial (indicated as subscript 2) surfaces of leaflets of lentil genotypes. (A) L. cul. Indianhead and (B) L. cul. CDC Redberry at 185X magnification and the following at 180X magnification: (C) L. cul. CDC Greenstar, (D) L. ori. IG 72643, (E) L. ori. PI 572376, (F) L. tom. IG 72613, (G) L. tom. IG 72614, (H) L. tom. IG 72805, (I) L. ode. IG 72623, (J) L. lam. IG 110813, (K) L. erv. L-01-827A, and (L) L. nig. IG 116024. Images (I–V) are surfaces of lentil pods at 180X magnification for (I) L. cul. Indianhead, (II) L. cul. CDC Redberry, (III) L. erv. L-01-827A, (IV) L. tom. IG 72613, and (V) L. tom. IG 72805. Arrows indicate trichomes. Images (K1) and (I) lack trichomes.
A large range of variation in leaflet TD was observed across wild and cultivated lentil genotypes. TD on adaxial leaflet surfaces ranged from 2 (±0.2) trichomes/mm2 in L. erv. L-01-827A to 20 (±2.8) trichomes/mm2 in L. tom. IG 72613 (Figure 3A). On the abaxial leaflet surface, TD ranged from 4 (±0.2) trichomes/mm2 in L. erv. L-01-827A to 30 (±4.8) trichomes/mm2 in L. nig. IG 116024 (Figure 3A). All lentil genotypes had increased TD on the abaxial leaflet surface, except for L. ori. PI 572376, which had a higher TD on the adaxial leaflet surface. TD between the adaxial and abaxial leaflet surfaces of the same genotype did not differ significantly except in the case of L. nig. IG 116024, where there were 1.8 times more trichomes on the abaxial surface compared to the adaxial surface.
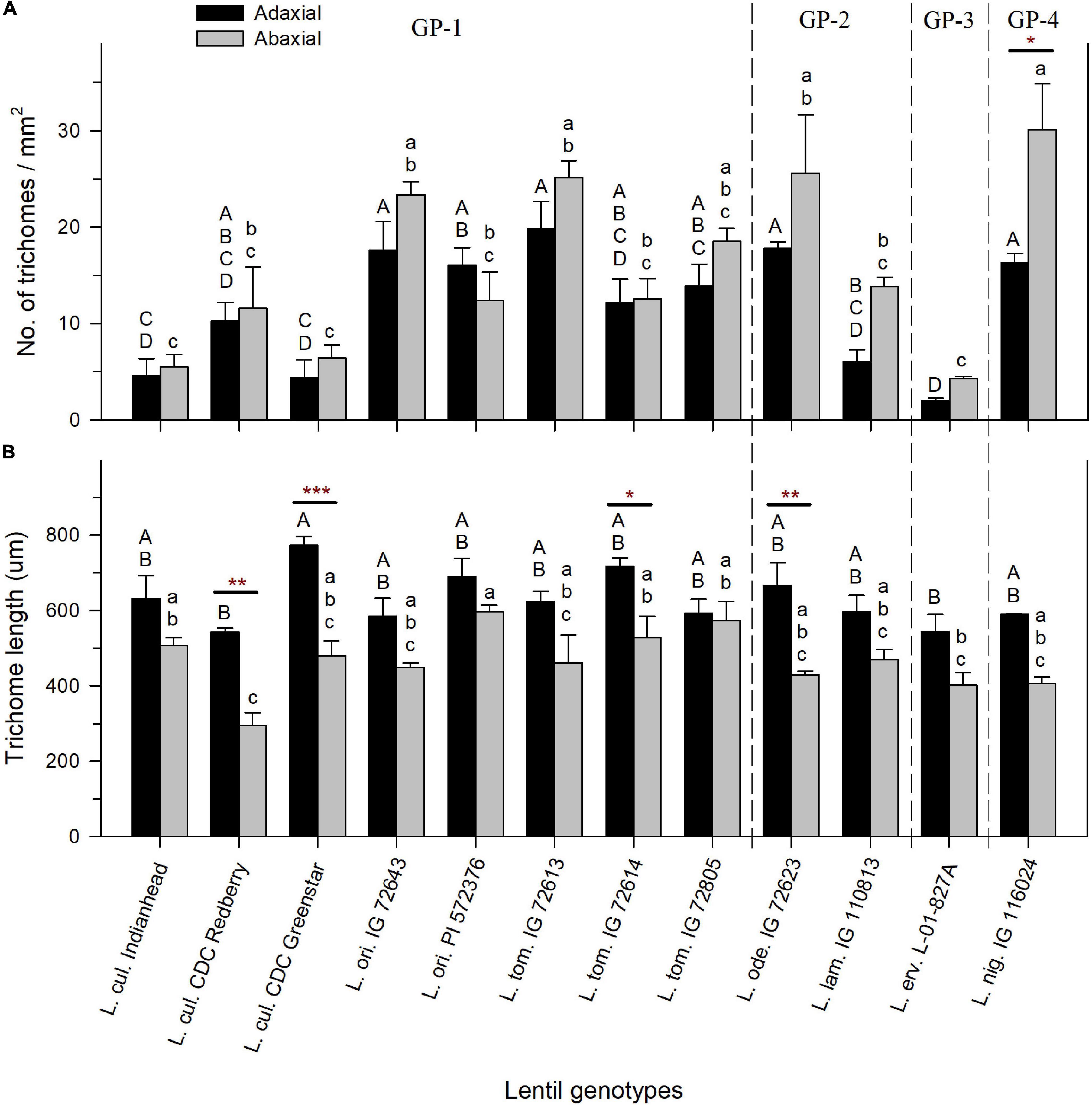
Figure 3. Mean trichome density expressed as the number of trichomes per mm2 (A) and mean trichome length in micrometers (B) on the adaxial and abaxial leaflet surfaces of cultivated and wild lentil genotypes. Asterisks indicate statistical difference between mean density of adaxial and abaxial surfaces of the same genotype based on t-test (Significance: *p < 0.05; **p < 0.01; ***p < 0.001). No asterisk implies no significant difference. Uppercase and lowercase letters show significant differences between adaxial and abaxial surfaces, respectively, across all genotypes at α = 5%. GP-1, GP-2, and GP-3 denote the genotypes belonging to primary, secondary, and tertiary gene pools, respectively, based on Wong et al. (2015).
Trichomes on the adaxial surface were consistently longer compared to those on the abaxial surface in lentil genotypes across all species. On the adaxial surface, the longest TL was observed in L. cul. CDC Greenstar (773 ± 24 μm), and the shortest TL was observed in L. cul. CDC Redberry (543 ± 11 μm) (Figure 3B). On the abaxial surface, trichomes of L. ori. PI 572376 (597 ± 17 μm) and L. cul. CDC Redberry (295 ± 35 μm) were the longest and shortest, respectively (Figure 3B). Difference in TL between adaxial and abaxial surface within each genotype was in most cases non-significant, except for the following four genotypes that had significantly longer trichomes on the adaxial surface compared to the abaxial surface: L. cul. CDC Redberry, L. cul. CDC Greenstar, L. tom. IG 72614, and L. ode. IG 72623.
Characterizing Stomatal and Epidermal Cell Traits in Lentil Genotypes
To determine the type of stomata, cells in contact with the guard cells were only considered subsidiary cell(s) if they appeared to be distinctly different from the neighboring pavement cells in shape, size, or morphology (Gray et al., 2020). All genotypes had anomocytic stomata (Table 2). Additionally, several genotypes had a stomatal complex where one cell in contact with the stomata was significantly smaller than the rest of the neighboring cells and was parallel to the lateral axis of the stomatal pore. These were considered hemi-paracytic stomata as per the definition by Van Cotthem (1970). In all genotypes, stomata on the adaxial surface were slightly sunken below the plane of the epidermal cells, while stomata on the abaxial surface were on the same plane as the epidermal cells.
All genotypes had irregularly shaped epidermal cells. Pattern on the epidermal cell wall was observed for both surfaces of each genotype based on schematic images by Nishida and Christophel (1999). Anticlinal walls of the epidermal cells in most species had a sinuous outline, except for the adaxial surface of L. lam. 110813, which was undulated with fewer curves than other genotypes (Table 2). The degree of convolution of anticlinal walls varied between surfaces: Anticlinal walls of epidermal cells on the abaxial surface were more convoluted than the ones on the adaxial surface.
Stomatal index was influenced by SD and ECD. SI, SD, and ECD were consistently higher on the adaxial leaf surface compared to the abaxial surface across all species (Figures 4A–C). SI on the adaxial surface ranged from 17% (±0.3%) in L. nig. IG 116024 to 27% (±0.8%) in L. erv. L-01-827A, and SI on the abaxial surface ranged from 3% (±0.6%) in L. lam. IG 110813 to 18% (±0.9%) in L. tom. IG 72614 (Figure 4A). There was a significant difference in SI between adaxial and abaxial surfaces in all species except for L. tom., L. ode., and L. nig.
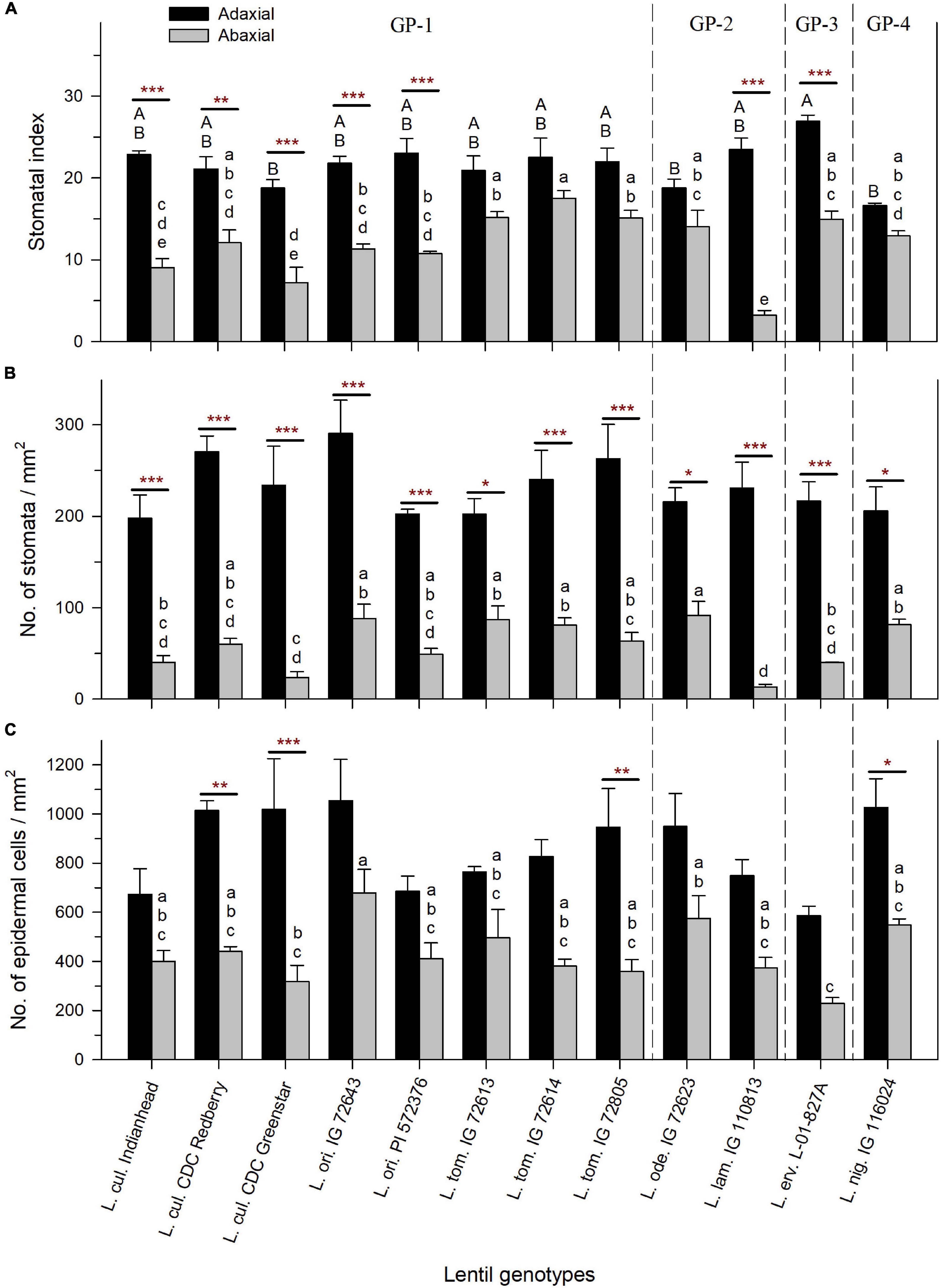
Figure 4. Stomatal index expressed as % (A), stomatal density expressed as the number of stomatal pores per mm2 (B), and epidermal cell density expressed as the number of epidermal cells per mm2 (C) on the adaxial and abaxial leaflet surface of cultivated and wild lentil genotypes. Stars represent the statistical difference between adaxial and abaxial surfaces of the same genotype based on t-test (Significance: *p < 0.05; **p < 0.01; ***p < 0.001). No stars imply no significant difference. Uppercase and lowercase letters show significant differences between the adaxial and abaxial surfaces, respectively, across all genotypes at alpha equals 5%. Absence of letters implies no significant difference. GP-1, GP-2, and GP-3 denote the genotypes from the primary, secondary, and tertiary gene pools, respectively (Wong et al., 2015).
Stomatal density was calculated by counting the number of stomatal pores per square mm on both surfaces. SD on the adaxial surface was not significantly different between genotypes and ranged from 198 (±25) stomata/mm2 (in L. cul. Indianhead) to 291 (±36) stomata/mm2 (in L. ori. IG 72643) (Figure 4B). On the abaxial surface, SD varied significantly between genotypes with L. lam. IG 110813 having the lowest SD of 13 (±3) stomata/mm2 and L. ode. IG 72623 having the highest SD of 92 (±15) stomata/mm2. SD in all wild and cultivated genotypes was significantly higher on the adaxial surface compared to the abaxial surface.
Epidermal cell density followed a similar trend as SD. ECD on the adaxial surface was not significantly different among genotypes and ranged from 585 (±40) epidermal cells/mm2 (in L. erv. L-01-827A) to 1055 (±167) epidermal cells/mm2 (in L. ori. IG 72643) (Figure 4C). On the abaxial surface, ECD was lower and varied from 230 (±23) epidermal cells/mm2 in L. erv. L-01-827A to 679 (±95) epidermal cells/mm2 in L. ori. IG 72643. For most genotypes, ECD on the adaxial and abaxial surfaces did not differ significantly. However, the following genotypes had a significant decrease in ECD on their abaxial surface compared to their adaxial surface: L. cul. CDC Redberry (2.3X difference), L. cul. CDC Greenstar (3.2X difference), L. tom. IG 72805 (2.6X difference), and L. nig. IG 116024 (1.9X difference).
Interaction of Morphological Traits in 12 Lentil Genotypes
Combined analysis of quantitative morphological traits of 12 lentil genotypes via two-factor ANOVA showed significant effects of genotype and leaflet surface characteristics for all traits, while the effects of the interaction between genotype and surface were only significant for the TL and SI (Table 3). Effect of blocking was only significant for TL and was insignificant for all other traits.
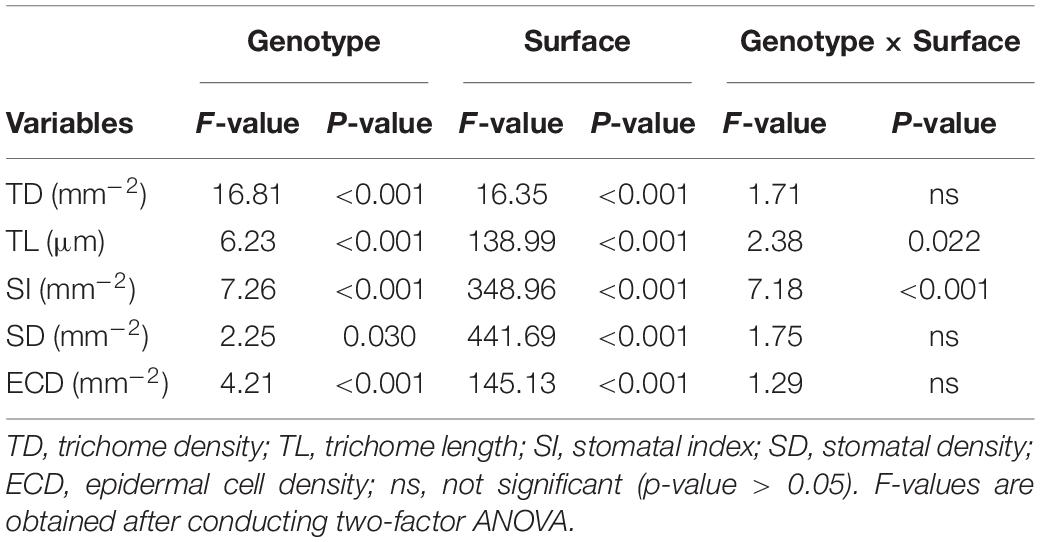
Table 3. Summary of the effects of genotype, adaxial and abaxial leaflet surfaces, and their interaction on micromorphological leaflet traits in wild and cultivated lentil.
Combined correlation analysis for 12 lentil genotypes revealed that TD was negatively correlated with SI, TL, and SD (Figure 5). These negative correlations were not statistically significant and had p-values > 0.05. TL was significantly positively correlated with SI, SD, and ECD. Since SI is a function of stomatal and ECD, all three were significantly positively correlated with each other. For genotypes belonging to wild species, precipitation and altitude at center of origin were not strongly correlated with any trait (correlation coefficient = 0 to 0.35 for positive correlations and −0.28 to 0 for negative correlations).
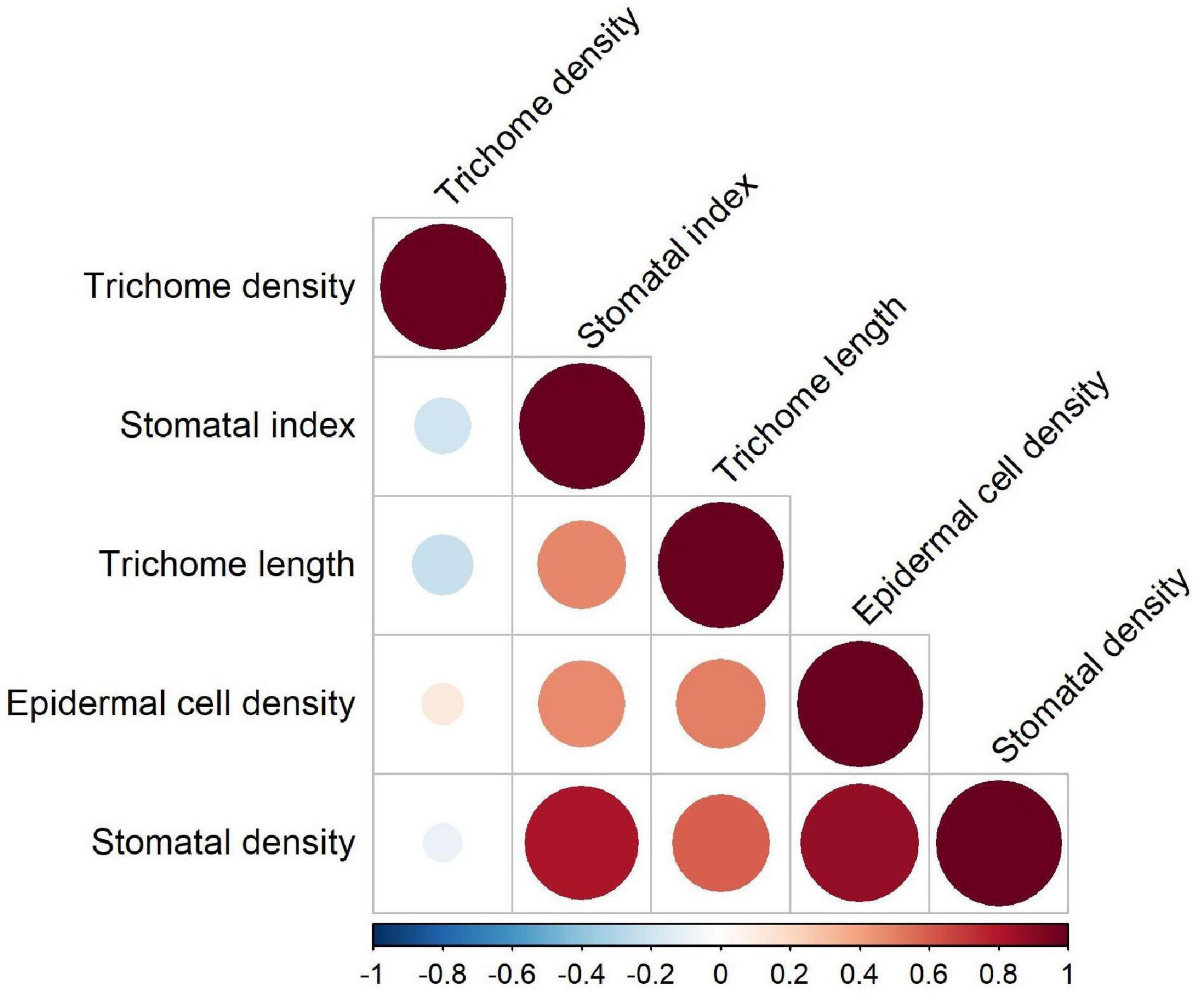
Figure 5. Correlations between five micromorphological traits on the adaxial and abaxial leaflet surfaces of wild and cultivated lentil.
Trichome Density and TL in an Interspecific Hybrid Population of L. culinaris × L. tomentosus
A total of 70 RILs of the NAM 38 population obtained from crossing L. cul. CDC Redberry with L. tom. IG 72805 were assessed for TD and TL on the adaxial leaf surface. These RILs varied from F4 to F6 generation. Histogram and density plots revealed non-symmetric skewed distributions for both traits (Figure 6). In the NAM 38 population, TD ranged from 1.11 mm–2 to 50 mm–2 with the density curve peaking around 10 mm–2 and skewing toward the right (Figure 6A). For reference, TD of cultivated and wild parents of NAM 38 was 1.8 mm–2 and 37.5 mm–2, respectively. Trichome characteristics were observed to be segregating transgressively in the RIL population.
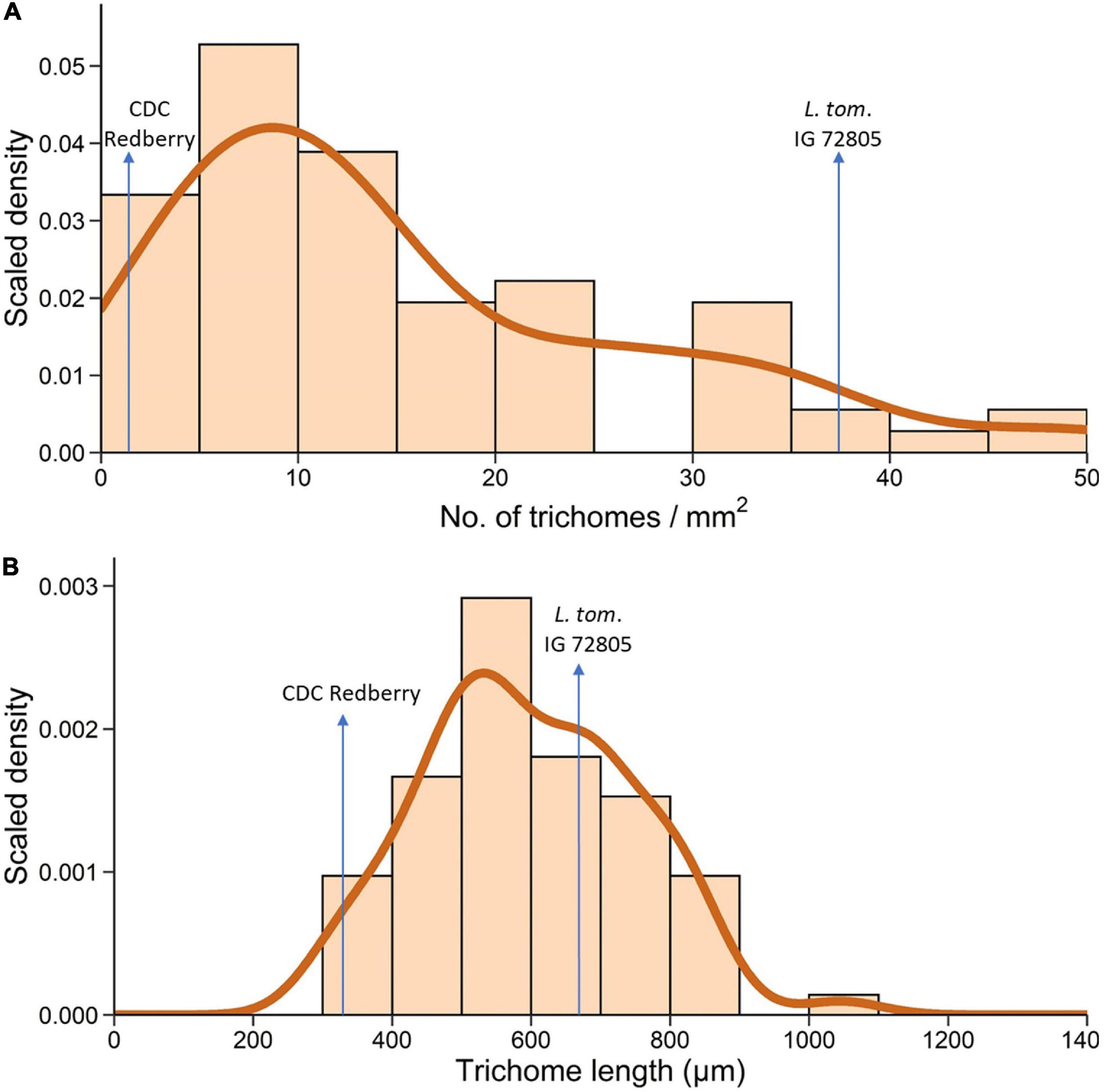
Figure 6. Histogram and density plots of trichome density (A) and trichome length (B) on the adaxial leaflet surface of 70 RILs of L. cul. × L. tom. NAM 38 varying from F4 to F6 generation.
The histogram and the resulting density plot for TL were almost symmetric with a slight skew toward the right. TL in the RILs ranged from 314 μm to 1,046 μm with the density curve peaking around 500 μm and a smaller peak around 700 μm (Figure 6B). TL of NAM 38 parents was 332 μm (L. cul. CDC Redberry) and 670 μm (L. tom. IG 72805).
Discussion
Lentil germplasm exhibits a wide range of variation in surface microstructures of trichomes, stomata, and epidermal cells on its foliar and pod surfaces. Even though trichome type (simple, non-glandular, and unicellular hair-like structures) was uniform across wild and cultivated lentil species, their density and length varied across species. Our results verify the limited amount of available literature on this topic: Variation in the pubescence of cultivated lentil is documented and there are records that describe lentil cultivars and landraces from Spain, the Indian subcontinent, and the western Mediterranean region as having absent, slight, or dense leaf pubescence (Lázaro et al., 2001; Toklu et al., 2009; Kumar and Solanki, 2014). A comprehensive study by Singh et al. (2014) that described various lentil traits across accessions belonging to all seven lentil species also characterized leaf pubescence as absent, slight, or dense. Thus far, leaf pubescence in lentil has only been described as a qualitative trait based on trait descriptors set by IBPGR/ICARDA (IBPGR and ICARDA, 1985). By quantifying TD, our study expands on this information and highlights the variation in leaf pubescence, not only among different species, but for the first time, also on abaxial and adaxial surfaces within the same genotype.
Our study also revealed various types of pubescence characteristics on pod surfaces. Ferguson et al. (2000) stated that L. erv. can be distinguished from other wild species due to its puberulent pods. We confirmed this in our study (Figure 2III) and found that no other species exhibited this phenotype on its pods. In contrast, however, Ferguson et al. (2000) noted L. cul. to have glabrous pods. We found that sparse trichomes may occur in L. cul., for example in CDC Redberry. Leaflet TD in CDC Redberry was also higher than other L. cul. genotypes. Since CDC Redberry is resistant to fungal diseases of ascochyta blight and race 1 of anthracnose (Vandenberg et al., 2006), it is possible that the trait of trichomes contributes to this combined disease resistance and needs to be further investigated. Differences in pubescence were also observed on stems of the different species as corroborated by Hoque et al. (2002), but were not characterized in this study.
Trichome density in genotypes (calculated by taking an average of TD on adaxial and abaxial surface of each genotype) ranged from 3 trichomes/mm2 (in L. erv. L-01-827A) to 23 trichomes/mm2 (in L. nig. IG 116024). TD in other wild and cultivated genotypes varied between these two extremes. Even though no correlation was found between trichome traits and the altitude and precipitation at the center of origin of wild species, it is possible that the differences in TD are due to the allopatric distribution of different lentil species: Presumably, they differentiated genetically and morphologically over time in unique environments. Trichomes play an important role in mediating plant–environment interactions such as defense against biotic and abiotic stressors like UV radiation, herbivores, and pathogens (Karabourniotis et al., 2020), so it is possible that they evolved over time in response to unique stress combinations encountered by each species at their respective centers of origin. Interestingly, drastic differences in TD were also present between genotypes belonging to the same species, even when their centers of origin were the same. For example, even though L. tom. IG 72613 and L. tom. IG 72614 have the same centers of origin, TD on surfaces of L. tom. IG 72614 was half that of L. tom. IG 72613. While this difference between the two L. tom. genotypes was statistically insignificant for either of the adaxial or of the abaxial surfaces, more research is needed to explain the intraspecific variation in TD observed in this study.
Most genotypes had lower TD on their adaxial leaflet surface compared to the abaxial leaflet surface, even though these differences were statistically non-significant. This phenomenon has been observed in other plants adapted to the Mediterranean climate, and it has been shown that the abaxial surface with higher TD is more effective than the adaxial surface at attenuating UV-A and UV-B radiation and protecting developing plants against high visible irradiance (Karabourniotis and Bornman, 1999). Additionally, while TD was generally higher on the abaxial surface, trichomes were shorter on the abaxial surface of all genotypes. This suggests that there might be a compensation between length and density, possibly related to the effect of changes in adaptation to the environment over time. Concurrent with the reduced TD on the adaxial surface, genotypes of all wild and cultivated species had a higher density of slightly sunken stomata on the adaxial surface. Sunken stomata were not observed on the abaxial surface of any genotype. Sunken stomata are a xeromorphic feature aimed at reducing transpiration (Haworth and McElwain, 2008), and it may be that the sunken stomata trait along with longer trichomes in wild and cultivated lentil is an adaptation to conserve water and compensate for reduced trichome cover on the adaxial surface.
Stomatal index was similar when comparing the same leaf surface across all species, but was higher on the adaxial surface compared to the abaxial surface within genotypes (Figure 4A). The same trend was observed for SD and ECD. These results are consistent with those observed by Sánchez-Gómez et al. (2019) in three Spanish lentil cultivars, and those of Lopes and Veiga (1979) who assessed cultivated lentil varieties and lines from the United States, Canada, Chile, and Brazil. However, the latter study observed that accessions with the highest SI on the adaxial surface also had the lowest SI on the abaxial surface. This suggests that there is compensation between the two surfaces with respect to this trait (Lopes and Veiga, 1979). Our study did not support this finding within the same gene pool or species, except in the case of L. ori. PI 572376, which had the highest and lowest SI on the adaxial and abaxial surface, respectively. These observations suggest that each genotype is unique in its physiology and that all lentil genotypes do not regulate their surface microstructural properties in the same manner. Additionally, the higher density of stomata on the adaxial surface in lentil is in contrast to most other amphistomatic plants, which usually have increased SD on the abaxial surface (Xiong and Flexas, 2020). Higher SD on the adaxial surface has been reported, however, in a C3 monocot grass species by Anderson and Briske (1990), but they noticed that this species had a 180° twist at its leaf blade which oriented the adaxial surface of leaves to match the orientation of other grasses that had higher stomata on their abaxial surface. It is not known if Lens spp. exhibits such a phenotype as well. It must be noted here that while amphistomatous plants may have an advantage over hypostomatous plants in terms of having higher gas exchange capacity (Drake et al., 2019), it is unclear if stomatal pore efficiency differs between the two surfaces, and thus, further investigation is needed to elucidate the advantages and disadvantages of amphistomaty, particularly the presence of stomata on the adaxial surface (Drake et al., 2019; Xiong and Flexas, 2020).
The types of stomata and anticlinal wall pattern of epidermal cells in Lens spp. were also determined for the first time via this study. All wild and cultivated genotypes had anomocytic stomata, and most also contained hemi-paracytic stomata, characterized by having one cell in the stomatal complex that was significantly smaller than other neighboring cells and was parallel to the lateral axis of the stomatal pore. It must be noted that in this study, we considered only those cells to be subsidiary cells that appeared distinct from other neighboring cells. Since most cells surrounding the guard cells appeared similar to other cells on the epidermis in shape and size, most stomata were classified as anomocytic. Anticlinal walls of epidermal cells on both adaxial and abaxial surfaces were sinuous in all genotypes except for the adaxial surface of L. lam. 110813. The phenotype of amphistomatic leaves, presence of anomocytic stomata, and adaxial and abaxial surfaces possessing different types of epidermal anticlinal wall patterns have also been reported in some other legumes such as lotus (Stenglein et al., 2003) and faba bean (Ahmad et al., 2010).
After quantifying trichome, stomatal, and epidermal cell traits in Lens spp., we sought to explore the inheritance of trichome traits. Previous studies of lentil pubescence within the cultivated species determined that pubescence is a qualitative trait controlled by a single gene system for which pubescence is dominant to glabrous phenotype in pods, peduncle, and the whole plant (Vandenberg and Slinkard, 1989; Sarker et al., 1999; Hoque et al., 2002). These studies did not go beyond the F2 or F3 generation, and the presence or absence of pubescence was determined through the visual inspection. In our study, after quantifying leaflet TD in the interspecific L. cul. × L. tom. NAM 38 RILs at F4-F6 generation, we found that plants segregated transgressively for TD and had an almost continuous distribution of TD where 45 out of 70 lines had low-medium TD (<15 mm–2) (Figure 6A). Transgressive segregation was also observed for TL and the distribution resembled a bell-shaped curve (Figure 6B). These combined results based on NAM 38 RILs suggest that leaflet pubescence is a quantitative trait or that the alleles of the genes that control plant pubescence are responsible for tissue-specific expression, as speculated by Kumar et al. (2005). This information will be useful from an agronomic perspective for plant breeders wanting to breed the trait of trichomes into cultivated lentil for sustained biotic and abiotic stress tolerance.
Conclusion
Our study highlighted the presence of a wide range of diversity in trichome and stomatal characteristics across the lentil germplasm. Trichome variation observed in wild and cultivated genotypes was not consistent across gene pools or even among lentil genotypes belonging to the same species, suggesting that the regulation of morphophysiological traits of trichomes is uniquely different in each genotype and likely evolved as a response to unique stresses in their centers of origin. Despite these differences, certain trends were observed in the traits of SD, trichomes, and ECD as they are related to the differences between adaxial and abaxial surfaces across the lentil germplasm. TL, SI, SD, and ECD were higher on the adaxial surface compared to the abaxial surface in each genotype, and these traits were significantly correlated with each other. TD was negatively correlated with SI and TL, suggesting that there may be a trade-off between these traits. The adaxial surface of all lentil genotypes, despite having more stomata, exhibited slightly sunken stomata, which might be a xeromorphic adaptation.
The study of interspecific RILs of L. cul. CDC Redberry and L. tom. IG 72805 revealed that TD and TL segregate transgressively. Quantifying and plotting these traits resulted in asymmetric density plots of continuous data, suggesting that the traits of TD and TL are quantitatively inherited. There is potential for harnessing the diversity in pubescence and stomatal traits to create future lentil varieties with desirable characteristics that can contribute to better adaptation to the future challenges of biotic and abiotic stresses.
Species delimitation based on the traits explored in this study alone is not possible since there were not any morphological or anatomical traits of trichomes, stomata, or epidermal cells that were unique to a particular species. However, these are important agronomic morphophysiological traits that have been shown to play a key role in mediating plant responses to biotic and abiotic stresses. TD and TL appear to be quantitatively inherited. If any of these traits are identified as being advantageous for lentil in imparting resistance or tolerance to biotic or abiotic stresses, then the results from this study will help physiologists and breeders make better decisions in selecting species or genotypes that better fit their goal of breeding lentil with improved resistance to concurrent stresses.
Data Availability Statement
The raw data supporting the conclusions of this article will be made available by the authors, without undue reservation.
Author Contributions
IP, LG, KT, and AV contributed to the conception, design, and planning of the experiments. IP performed the experiments, analyzed the data, and wrote the manuscript with input from LG. KT and AV revised the manuscript and approved the submitted version. All authors contributed to the article and approved the submitted version.
Funding
This research received financial support by Saskatchewan Pulse Growers and Canada’s NSERC Industrial Research Chair in Lentil Genetic Improvement at the University of Saskatchewan.
Conflict of Interest
The authors declare that the research was conducted in the absence of any commercial or financial relationships that could be construed as a potential conflict of interest.
Acknowledgments
We would like to acknowledge the technical support provided by staff at the Crop Science Field Laboratory at the University of Saskatchewan, particularly Brent Barlow, Devini De Silva, and Donna Lindsay. We would also like to acknowledge the financial support provided by Saskatchewan Pulse Growers and Canada’s NSERC Industrial Research Chair in Lentil Genetic Improvement at the University of Saskatchewan.
Footnotes
References
Ahmad, K., Khan, M. A., Ahmad, M., Shaheen, N., and Nazir, A. (2010). Taxonomic diversity in epidermal cells of some sub-tropical plant species. Int. J. Agric. Biol. 12, 115–118.
Akgul, A., Akgul, A., Senol, S. G., Yildirim, H., Secmen, O., and Dogan, Y. (2018). An ethnobotanical study in Midyat (Turkey), a city on the silk road where cultures meet. J. Ethnobiol. Ethnomed. 14:12. doi: 10.1186/s13002-017-0201-8
Anderson, V. J., and Briske, D. D. (1990). Stomatal distribution, density and conductance of three perennial grasses native to the southern true prairie of Texas. Am. Midl. Nat. 123, 152–159. doi: 10.2307/2425768
Arve, L. E., Terfa, M. T., Gislerød, H. R., Olsen, J. E., and Torre, S. (2013). High relative air humidity and continuous light reduce stomata functionality by affecting the ABA regulation in rose leaves. Plant. Cell Environ. 36, 382–392. doi: 10.1111/j.1365-3040.2012.02580.x
Arve, L., Torre, S., Olsen, J., and Tanino, K. (2011). “Stomatal responses to drought stress and air humidity,” in Abiotic Stress in Plants - Mechanisms and Adaptations, eds A. Shanker and B. Venkateswarlu (Rijeka: IntechOpen), doi: 10.5772/24661
Bahadur, S., Ahmad, M., Zafar, M., Sultana, S., Begum, N., Ashfaq, S., et al. (2019). Palyno-anatomical studies of monocot taxa and its taxonomic implications using light and scanning electron microscopy. Microsc. Res. Tech. 82, 373–393. doi: 10.1002/jemt.23179
Banik, P., Zeng, W., Tai, H., Bizimungu, B., and Tanino, K. (2016). Effects of drought acclimation on drought stress resistance in potato (Solanum tuberosum L.) genotypes. Environ. Exp. Bot. 126, 76–89. doi: 10.1016/j.envexpbot.2016.01.008
Baran, M., Yıldırım, M., and Yılmaz, A. (2011). Evaluation of ecological design strategies in traditional houses in Diyarbakir. Turkey. J. Clean. Prod. 19, 609–619. doi: 10.1016/j.jclepro.2010.11.001
Bates, D., Mächler, M., Bolker, B., and Walker, S. (2015). Fitting linear mixed-effects models using lme4. J. Stat. Softw. 67, 1–48. doi: 10.18637/jss.v067.i01
Beemster, G. T. S., and Masle, J. (1996). Effects of soil resistance to root penetration on leaf expansion in wheat (Triticum aestivum L.): composition, number and size of epidermal cells in mature blades. J. Exp. Bot. 47, 1651–1662. doi: 10.1093/jxb/47.11.1651
Beranová, R., and Kyselý, J. (2018). Trends of precipitation characteristics in the Czech Republic over 1961-2012, their spatial patterns and links to temperature and the North Atlantic Oscillation. Int. J. Climatol. 38, e596–e606. doi: 10.1002/joc.5392
Bosabalidis, A. M., and Kofidis, G. (2002). Comparative effects of drought stress on leaf anatomy of two olive cultivars. Plant Sci. 163, 375–379. doi: 10.1016/S0168-9452(02)00135-8
Çaçan, E., and Kökten, K. (2017). Current situation of Diyarbakir province in terms of crop production. Middle East J. Sci. 3, 80–91. doi: 10.23884/mejs.2017.3.2.09
Carlisle, L. (2016). Making heritage: The case of black Beluga agriculture on the Northern Great Plains. Ann. Am. Assoc. Geogr. 106, 130–144. doi: 10.1080/00045608.2015.1086629
Carvalho, D. R. A., Vasconcelos, M. W., Lee, S., Koning-Boucoiran, C. F. S., Vreugdenhil, D., Krens, F. A., et al. (2016). Gene expression and physiological responses associated to stomatal functioning in Rosa×hybrida grown at high relative air humidity. Plant Sci. 253, 154–163. doi: 10.1016/j.plantsci.2016.09.018
Coyne, C. J., Kumar, S., von Wettberg, E. J. B., Marques, E., Berger, J. D., Redden, R. J., et al. (2020). Potential and limits of exploitation of crop wild relatives for pea, lentil, and chickpea improvement. Legum. Sci. 2, e36. doi: 10.1002/leg3.36
Curtis, P. S., and Läuchli, A. (1987). The effect of moderate salt stress on leaf anatomy in Hibiscus cannabinus (Kenaf) and its relation to leaf area. Am. J. Bot. 74, 538–542. doi: 10.1002/j.1537-2197.1987.tb08674.x
Cutler, J. M., Rains, D. W., and Loomis, R. S. (1977). The importance of cell size in the water relations of plants. Physiol. Plant. 40, 255–260. doi: 10.1111/j.1399-3054.1977.tb04068.x
Dalkılıç, N. (2012). The architectural analysis of traditional houses of Midyat-Mardin, Turkey. Int. J. Acad. Res. 4:33824139.
Davies, P. A., Lülsdorf, M. M., and Ahmad, M. (2007). “Wild relatives and biotechnological approaches,” in Lentil, eds S. S. Yadav, D. L. McNeil, and P. C. Stevenson (Dordrecht: Springer Netherlands), 225–240. doi: 10.1007/978-1-4020-6313-8_14
Drake, P. L., de Boer, H. J., Schymanski, S. J., and Veneklaas, E. J. (2019). Two sides to every leaf: Water and CO2 transport in hypostomatous and amphistomatous leaves. N. Phytol. 222, 1179–1187. doi: 10.1111/nph.15652
Dubrovsky, M., Svoboda, M. D., Trnka, M., Hayes, M. J., Wilhite, D. A., Zalud, Z., et al. (2009). Application of relative drought indices in assessing climate-change impacts on drought conditions in Czechia. Theor. Appl. Climatol. 96, 155–171. doi: 10.1007/s00704-008-0020-x
Erskine, W., Chandra, S., Chaudhry, M., Malik, I. A., Sarker, A., Sharma, B., et al. (1998). A bottleneck in lentil: widening its genetic base in South Asia. Euphytica 101, 207–211. doi: 10.1023/A:1018306723777
Erskine, W., Muehlbauer, F. J., and Short, R. W. (1990). Stages of development in lentil. Exp. Agric. 26, 297–302. doi: 10.1017/S0014479700018457
Esfandani-Bozchaloyi, S., and Zaman, W. (2018). Taxonomic significance of macro and micro-morphology of Geranium L. species Using Scanning Electron Microscopy. Microsc. Res. Tech. 81, 1520–1532. doi: 10.1002/jemt.23159
Ferguson, M. E., Maxted, N., Slageren, M., Van, and Robertson, L. D. (2000). A re-assessment of the taxonomy of Lens Mill. (Leguminosae, Papilionoideae, Vicieae). Bot. J. Linn. Soc. 133, 41–59. doi: 10.1111/j.1095-8339.2000.tb01536.x
Ferguson, M., and Erskine, W. (2001). “Lentils (Lens L.),” in Plant genetic resources of legumes in the Mediterranean, eds N. Maxted and S. J. Bennett (Dordrecht: Springer Netherlands), 125–133. doi: 10.1007/978-94-015-9823-1_7
Fiala, J. V., Tullu, A., Banniza, S., Séguin-Swartz, G., and Vandenberg, A. (2009). Interspecies transfer of resistance to anthracnose in lentil (Lens culinaris Medic.). Crop Sci. 49, 825–830. doi: 10.2135/cropsci2008.05.0260
Galán, C., Emberlin, J., Domínguez, E., Bryant, R. H., and Villamandos, F. (1995). A comparative analysis of daily variations in the Gramineae pollen counts at Córdoba, Spain and London. Grana 34, 189–198. doi: 10.1080/00173139509429042
Gezer, K., Kaygusuz, O., Çelik, A., and Işıloğlu, M. (2014). Ecological characteristics of truffles growing in Denizli Province, Turkey. J. Food Agric. Environ. 12, 1105–1109. doi: 10.1234/4.2014.5296
Google Maps. (2021). Google Maps. Available online at: https://www.google.com/maps (accessed March 23, 2021)
Gorim, L. Y., and Vandenberg, A. (2017). Evaluation of wild lentil species as genetic resources to improve drought tolerance in cultivated lentil. Front. Plant Sci. 8:1129. doi: 10.3389/fpls.2017.01129
Gorim, L. Y., and Vandenberg, A. (2018). Can wild lentil genotypes help improve water use and transpiration efficiency in cultivated lentil? Plant Genet. Resour. 16, 459–468. doi: 10.1017/S1479262117000399
Gray, A., Liu, L., and Facette, M. (2020). Flanking support: How subsidiary cells contribute to stomatal form and function. Front. Plant Sci. 11:881. doi: 10.3389/fpls.2020.00881
Gul, S., Ahmad, M., Zafar, M., Bahadur, S., Celep, F., Sultana, S., et al. (2019). Taxonomic significance of foliar epidermal morphology in Lamiaceae from Pakistan. Microsc. Res. Tech. 82, 1507–1528. doi: 10.1002/jemt.23316
Gürsoy, S., Özaslan, C., Urğun, M., Kolay, B., and Koç, M. (2014). The effect of sowing time, tillage system and herbicides on weed species density, weed biomass and yield of lentil within a lentil-wheat sequence. Agric. For. 60, 73–85.
Haworth, M., and McElwain, J. (2008). Hot, dry, wet, cold or toxic? Revisiting the ecological significance of leaf and cuticular micromorphology. Palaeogeogr. Palaeoclimatol. Palaeoecol. 262, 79–90. doi: 10.1016/j.palaeo.2008.02.009
Hopkins, J. W. (1938). Agricultural meteorology: Correlation of air temperatures in central and southern Alberta and Saskatchewan with latitude, longitude and altitude. Can. J. Res. 16c, 16–26. doi: 10.1139/cjr38c-002
Hoque, M. E., Mishra, S. K., Kumar, Y., Kumar, R., Tomar, S. M. S., and Sharma, B. (2002). Inheritance and linkage of leaf colour and plant pubescence in lentil (Lens culinaris Medik.). Ind. J. Genet. Plant Breed. 15, 1–8.
Iqbal, A., Khalil, I. A., Ateeq, N., and Sayyar Khan, M. (2006). Nutritional quality of important food legumes. Food Chem. 97, 331–335. doi: 10.1016/j.foodchem.2005.05.011
Jarpa-Parra, M. (2018). Lentil protein: a review of functional properties and food application. An overview of lentil protein functionality. Int. J. Food Sci. Technol. 53, 892–903. doi: 10.1111/ijfs.13685
Karabourniotis, G., and Bornman, J. F. (1999). Penetration of UV-A, UV-B and blue light through the leaf trichome layers of two xeromorphic plants, olive and oak, measured by optical fibre microprobes. Physiol. Plant. 105, 655–661. doi: 10.1034/j.1399-3054.1999.105409.x
Karabourniotis, G., Liakopoulos, G., Nikolopoulos, D., and Bresta, P. (2020). Protective and defensive roles of non-glandular trichomes against multiple stresses: structure–function coordination. J. For. Res. 31, 1–12. doi: 10.1007/s11676-019-01034-4
Kaur, J., and Kariyat, R. (2020). “Role of Trichomes in Plant Stress Biology,” in Evolutionary Ecology of Plant-Herbivore Interaction, eds J. Núñez-Farfán and P. L. Valverde (Cham: Springer International Publishing), 15–35. doi: 10.1007/978-3-030-46012-9_2
Khazaei, H., Subedi, M., Nickerson, M., Martínez-Villaluenga, C., Frias, J., and Vandenberg, A. (2019). Seed protein of lentils: Current status, progress, and food applications. Foods 8:foods8090391. doi: 10.3390/foods8090391
Kumar, J., and Solanki, R. K. (2014). Evaluation of germplasm accessions for agro-morphological traits in lentil. J. Food Legum. 27:275.
Kumar, Y., Mishra, S. K., Tyagi, M. C., Singh, S. P., and Sharma, B. (2005). Linkage between genes for leaf colour, plant pubescence, number of leaflets and plant height in lentil (Lens culinaris Medik.). Euphytica 145, 41–48. doi: 10.1007/s10681-005-0103-z
Ladizinsky, G. (1979). The origin of lentil and its wild genepool. Euphytica 28, 179–187. doi: 10.1007/BF00029189
Lázaro, A., Ruiz, M., De La Rosa, L., and Martín, I. (2001). Relationships between agro/morphological characters and climatic parameters in Spanish landraces of lentil (Lens culinaris Medik.). Genet. Resour. Crop Evol. 48, 239–249. doi: 10.1023/A:1011234126154
Lechner, L., Pereyra-Irujo, G. A., Granier, C., and Aguirrezábal, L. A. N. (2008). Rewatering plants after a long water-deficit treatment reveals that leaf epidermal cells retain their ability to expand after the leaf has apparently reached its final size. Ann. Bot. 101, 1007–1015. doi: 10.1093/aob/mcn029
Liu, K., Blackshaw, R. E., Johnson, E. N., Hossain, Z., Hamel, C., St-Arnaud, M., et al. (2019). Lentil enhances the productivity and stability of oilseed-cereal cropping systems across different environments. Eur. J. Agron. 105, 24–31. doi: 10.1016/j.eja.2019.02.005
Lopes, V. J. X., and Veiga, P. (1979). Comparação entre os componentes da epiderme da folha em variedades e linhagens de lentilha (Lens culinaris Medic.). Rev. Cent. Ciências Rurais 9:325.
McCree, K. J., and Davis, S. D. (1974). Effect of water stress and temperature on leaf size and on size and number of epidermal cells in grain sorghum. Crop Sci. 14, 751–755. doi: 10.2135/cropsci1974.0011183X001400050041x
Melotto, M., Underwood, W., and He, S. Y. (2008). Role of stomata in plant innate immunity and foliar bacterial diseases. Annu. Rev. Phytopathol. 46, 101–122. doi: 10.1146/annurev.phyto.121107.104959
Migliozzi, M., Thavarajah, D., Thavarajah, P., and Smith, P. (2015). Lentil and Kale: Complementary Nutrient-Rich Whole Food Sources to Combat Micronutrient and Calorie Malnutrition. Nutrients 7:nu7115471. doi: 10.3390/nu7115471
Mwakutuya, E. (2006). Epidemiology of stemphylium blight on lentil (Lens culinaris) in Saskatchewan. Saskatoon: University of Saskatchewan.
Nishida, S., and Christophel, D. C. (1999). Leaf anatomy of Beilschmiedia (Lauraceae) in the Neotropics. Nat. Hum. Act. 4, 9–43. doi: 10.24713/nha.4.0_9
Niu, Y., Bainard, L. D., Bandara, M., Hamel, C., and Gan, Y. (2017). Soil residual water and nutrients explain about 30% of the rotational effect in 4-yr pulse-intensified rotation systems. Can. J. Plant Sci. 97, 852–864. doi: 10.1139/cjps-2016-0282
Ogutcen, E., Ramsay, L., von Wettberg, E. B., and Bett, K. E. (2018). Capturing variation in Lens (Fabaceae): Development and utility of an exome capture array for lentil. Appl. Plant Sci. 6:e01165. doi: 10.1002/aps3.1165
Oksanen, E. (2018). Trichomes form an important first line of defence against adverse environment—New evidence for ozone stress mitigation. Plant Cell Environ. 41:13187. doi: 10.1111/pce.13187
Pandey, P., Irulappan, V., Bagavathiannan, M. V., and Senthil-Kumar, M. (2017). Impact of combined abiotic and biotic stresses on plant growth and avenues for crop improvement by exploiting physio-morphological traits. Front. Plant Sci. 8:537. doi: 10.3389/fpls.2017.00537
Piggin, C., Haddad, A., Khalil, Y., Loss, S., and Pala, M. (2015). Effects of tillage and time of sowing on bread wheat, chickpea, barley and lentil grown in rotation in rainfed systems in Syria. Field Crops Res. 173, 57–67. doi: 10.1016/j.fcr.2014.12.014
Salisbury, E. J., and Oliver, F. W. (1928). I. On the causes and ecological significance of stomatal frequency, with special reference to the woodland flora. Phil. Trans. R. Soc. Lond. B 216, 1–65. doi: 10.1098/rstb.1928.0001
Sánchez-Gómez, D., Cervera, M. T., Escolano-Tercero, M. A., Vélez, M. D., de María, N., Diaz, L., et al. (2019). Drought escape can provide high grain yields under early drought in lentils. Theor. Exp. Plant Physiol. 31, 273–286. doi: 10.1007/s40626-018-0136-z
Sanderson, L.-A., Caron, C. T., Tan, R., Shen, Y., Liu, R., and Bett, K. E. (2019). KnowPulse: A web-resource focused on diversity data for pulse crop improvement. Front. Plant Sci. 10:965. doi: 10.3389/fpls.2019.00965
Sarker, A., Erskine, W., Sharma, B., and Tyagi, M. C. (1999). Inheritance and linkage relationship of days to flower and morphological loci in lentil (Lens culinaris Medikus subsp. culinaris). J. Hered. 90, 270–275. doi: 10.1093/jhered/90.2.270
Shabala, S. (2013). Learning from halophytes: physiological basis and strategies to improve abiotic stress tolerance in crops. Ann. Bot. 112, 1209–1221. doi: 10.1093/aob/mct205
Singh, M., Bisht, I. S., Kumar, S., Dutta, M., Bansal, K. C., Karale, M., et al. (2014). Global wild annual Lens collection: A potential resource for lentil genetic base broadening and yield enhancement. PLoS One 9:0107781. doi: 10.1371/journal.pone.0107781
Smýkal, P., Coyne, C. J., Ambrose, M. J., Maxted, N., Schaefer, H., Blair, M. W., et al. (2015). Legume crops phylogeny and genetic diversity for science and breeding. CRC Crit. Rev. Plant Sci. 34, 43–104. doi: 10.1080/07352689.2014.897904
Sonnante, G., Hammer, K., and Pignone, D. (2009). From the cradle of agriculture a handful of lentils: History of domestication. Rend. Fis. Acc. Lincei 20, 21–37. doi: 10.1007/s12210-009-0002-7
Stenglein, S. A., Colares, M. N., Arambarri, A. M., Novoa, M. C., Vizcaíno, C. E., and Katinas, L. (2003). Leaf epidermal microcharacters of the Old World species of Lotus (Leguminosae: Loteae) and their systematic significance. Aust. J. Bot. 51, 459–469. doi: 10.1071/BT02102
Szczepaniec, A., and Finke, D. (2019). Plant-vector-pathogen interactions in the context of drought stress. Front. Ecol. Evol. 7:262. doi: 10.3389/fevo.2019.00262
Tanaka, Y., Sano, T., Tamaoki, M., Nakajima, N., Kondo, N., and Hasezawa, S. (2005). Ethylene inhibits abscisic acid-induced stomatal closure in Arabidopsis. Plant Physiol. 138, 2337L–2343. doi: 10.1104/pp.105.063503
Toklu, F., Biçer, B., and Karaköy, T. (2009). Agro-morphological characterization of the Turkish lentil landraces. Afr. J. Biotechnol. 8:828. doi: 10.5897/AJB09.828
Ulukanligil, M., Seyrek, A., Aslan, G., Ozbilge, H., and Atay, S. (2001). Environmental pollution with soil-transmitted helminths in Sanliurfa, Turkey. Mem. Inst. Oswaldo Cruz 96, 903–909. doi: 10.1590/s0074-02762001000700004
Van Cotthem, W. (1970). Classification of stomatal types. Bot. J. Linn. Soc. 63, 235–246. doi: 10.1111/j.1095-8339.1970.tb02321.x
Vandenberg, A., and Slinkard, A. E. (1989). Inheritance of four new qualitative genes in lentil. J. Hered. 80, 320–322. doi: 10.1093/oxfordjournals.jhered.a110861
Vandenberg, A., Banniza, S., Warkentin, T. D., Ife, S., Barlow, B., McHale, S., et al. (2006). CDC Redberry lentil. Can. J. Plant Sci. 86, 497–498. doi: 10.4141/P05-071
Velasco-Jiménez, M. J., Alcázar, P., Domínguez-Vilches, E., and Galán, C. (2013). Comparative study of airborne pollen counts located in different areas of the city of Córdoba (south-western Spain). Aerobiologia 29, 113–120. doi: 10.1007/s10453-012-9267-x
Wade, A. J., Black, E., Brayshaw, D. J., El-Bastawesy, M., Holmes, P. A. C., Butterfield, D., et al. (2010). A model-based assessment of the effects of projected climate change on the water resources of Jordan. Philos. Trans. A Math. Phys. Eng. Sci. 368, 5151–5172. doi: 10.1098/rsta.2010.0207
Warne, T., Ahmed, S., Byker Shanks, C., and Miller, P. (2019). Sustainability dimensions of a North American lentil system in a changing world. Front. Sustain. Food Syst. 3:88. doi: 10.3389/fsufs.2019.00088
Wong, M. M. L., Gujaria-Verma, N., Ramsay, L., Yuan, H. Y., Caron, C., Diapari, M., et al. (2015). Classification and characterization of species within the genus Lens using genotyping-by-sequencing (GBS). PLoS One 10:0122025. doi: 10.1371/journal.pone.0122025
Xiong, D., and Flexas, J. (2020). From one side to two sides: the effects of stomatal distribution on photosynthesis. New Phytol. 228, 1754–1766.
Yadav, S. S., Stevenson, P. C., Rizvi, A. H., Manohar, M., Gailing, S., and Mateljan, G. (2007). “Uses and consumption,” in Lentil: An ancient crop for modern times, eds S. S. Yadav, D. L. McNeil, and P. C. Stevenson (Dordrecht: Springer Netherlands), 33–46. doi: 10.1007/978-1-4020-6313-8_4
Keywords: lentil genotypes, trichomes, stomatal index, epidermal cells, wild lentil
Citation: Patel I, Gorim LY, Tanino K and Vandenberg A (2021) Diversity in Surface Microstructures of Trichomes, Epidermal Cells, and Stomata in Lentil Germplasm. Front. Plant Sci. 12:697692. doi: 10.3389/fpls.2021.697692
Received: 20 April 2021; Accepted: 15 June 2021;
Published: 12 July 2021.
Edited by:
Sergio J. Ochatt, INRA, UMR 1347 Agroécologie, FranceReviewed by:
Saraj Bahadur, Hainan University, ChinaJun-Ho Song, Korea Institute of Oriental Medicine (KIOM), South Korea
Copyright © 2021 Patel, Gorim, Tanino and Vandenberg. This is an open-access article distributed under the terms of the Creative Commons Attribution License (CC BY). The use, distribution or reproduction in other forums is permitted, provided the original author(s) and the copyright owner(s) are credited and that the original publication in this journal is cited, in accordance with accepted academic practice. No use, distribution or reproduction is permitted which does not comply with these terms.
*Correspondence: Albert Vandenberg, YmVydC52YW5kZW5iZXJnQHVzYXNrLmNh