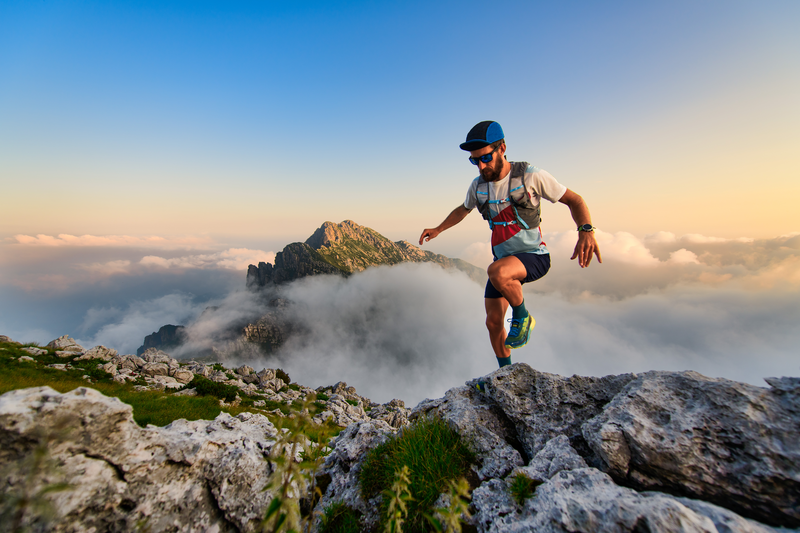
94% of researchers rate our articles as excellent or good
Learn more about the work of our research integrity team to safeguard the quality of each article we publish.
Find out more
ORIGINAL RESEARCH article
Front. Plant Sci. , 02 August 2021
Sec. Crop and Product Physiology
Volume 12 - 2021 | https://doi.org/10.3389/fpls.2021.694153
This article is part of the Research Topic Behind the Smoke and Mirrors: Reflections on Improving Cannabis Production and Investigating Medical Potential View all 16 articles
Cultivation of hemp (Cannabis sativa L.) in tropical and subtropical regions can be challenging if the flowering behavior of a given cultivar is unknown, poorly understood, or not accurately selected for the photoperiod. Identifying cultivars adapted to local environmental conditions is key to optimizing hemp vegetative and flowering performance. We investigated the effects of varying light cycles in regulating extension growth and flowering response of 15 essential oil and 12 fiber/grain hemp cultivars both indoors and outdoors. Plants were subjected to 11 photoperiods in the controlled rooms ranging from 12 to 18 h, and natural day length in the field. The critical photoperiod threshold was identified for seven essential oil cultivars and two fiber/grain cultivars. “Cherry Wine-CC,” “PUMA-3,” and “PUMA-4” had the shortest critical day length between 13 h 45 min and 14 h. The flowering of essential oil cultivars was generally delayed by 1–2 days when the photoperiod exceeded 13 h compared with 12 h, and flowering was further delayed by 7–8 days when the photoperiod exceeded 14 h. In fiber/grain cultivars, flowering was generally delayed by 1–3 days when the day length exceeded 14 h. Flowering for most essential oil cultivars was delayed by 5–13 days under a 14-h photoperiod compared with 13 h 45 min, suggesting a photoperiod difference as little as 15 min can significantly influence the floral initiation of some essential oil cultivars. Cultivars represented by the same name but acquired from different sources can perform differently under the same environmental conditions, suggesting genetic variation among cultivars with the same name. Average days to flower of fiber/grain cultivars was correlated with reported cultivar origin, with faster flowering occurring among northern cultivars when compared with southern cultivars. Plant height generally increased as the day length increased in essential oil cultivars but was not affected in fiber/grain cultivars. In addition, civil twilight of ~2 μmol·m−2·s−1 was discovered to be biologically effective in regulating hemp flowering. Collectively, we conclude that most of the essential oil cultivars and some southern fiber/grain cultivars tested express suitable photoperiods for tropical and sub-tropical region cultivation.
Cultivation of hemp (Cannabis sativa L.) within the USA was restricted in 1937 following the passage of the Marihuana Tax Act. Similarly, hemp cultivation was prohibited throughout the western world during most of the twentieth century (Cherney and Small, 2016; Congressional Research Service, 2019). With the legal status of Cannabis production shifting in the USA following the passage of the 2014 and 2018 farm bills (Agricultural Act of 2014, P.L. 113-79; Agriculture Improvement Act of 2018, P.L. 115-334), restrictions on hemp production were relaxed and interest in hemp cultivation thereafter rapidly increased. Within the USA, the classification of Cannabis is based upon the concentration of Δ9-tetrahydrocannabinol (THC) present in plant tissue. Plants with a concentration of ≤ 0.3% THC on a dry weight basis are legally recognized as industrial hemp, whereas plants containing >0.3% are recognized as marijuana, a Schedule I drug as defined by the Controlled Substances Act of 1967 (Congressional Research Service, 2019). Industrial hemp is commercially cultivated for its fiber, seed, and secondary metabolites [such as cannabidiol (CBD) and cannabigerol (CBG)]. Hemp is used to produce a wide variety of industrial and consumer products including food and beverages, personal care products, nutritional supplements, therapeutic products, fabrics and paper, and construction materials (Congressional Research Service, 2019). In 2016, the global fiber hemp market was valued at nearly $700 million with an expected growth rate of 10–20%, whereas the hemp-derived CBD market in 2022 is expected to be more than 2-fold greater than it was in 2018 to become a $1.3 billion-dollar market (Hemp Business Journal, 2018; Anderson et al., 2019).
Hemp fiber quality can be largely influenced by flowering time, sex characteristics, and other environmental factors independent of heritable genetic variation (Petit et al., 2020). “Technical maturity” for fiber production of monoecious hemp is reached at peak flowering of male plants (Mediavilla et al., 2001). At the onset of flowering, the nutrient flow is shifted from the development of stem and leaves to flower and seeds (Salentijn et al., 2019). High primary bast fiber content with a low secondary bast fiber content in fiber hemp is considered advantageous for textile production. However, the primary bast fiber layer experiences a proportional decrease during the flowering stage, whereas the secondary bast fiber fraction increase along the stems (Mediavilla et al., 2001; Salentijn et al., 2019). Harvest after the flowering of the male hemp plant will result in fiber loss and reduction of fiber fineness (Keller et al., 2001). Thus, precise prediction of flowering time is essential for determining fiber hemp harvest time and maximizing fiber quality. On the other hand, hemp is naturally dioecious (male and female flowers on separate plants) with monoecious (male and female flowers on the same plant) cultivars existing. Male plants in dioecious genotypes have a finer fiber and superior for textile production, whereas monoecious genotypes are more uniform in plant height and better for the dual harvest of fiber and seed (Salentijn et al., 2019). Understanding the sex composition of fiber/grain hemp cultivars is beneficial for breeding purposes and is critical for selecting the ideal cultivar for a specific production purpose.
Hemp can be challenging to cultivate in tropical and subtropical regions, given high temperatures, high humidity, and ample presence of disease and pests. However, relatively short daylengths experienced in tropical and subtropical environments arguably present the greatest challenge to the successful cultivation of hemp at lower latitudes. Hemp is considered an annual, dioecious, short-day plant (SDP) originating from temperate regions of Central Asia. Most hemp varieties are photoperiodic, and thus flowering of hemp is dependent upon day length or photoperiod. Cannabis has adapted to a wide range of climates and latitudes (23–52°N) and thus can possess large variability in its sensitivity to day length (Zhang et al., 2018). Timing of transition from vegetative growth to flowering is key for high yield and acceptable fiber quality of hemp (Amaducci et al., 2012). Earlier seasonal planting under critical daylength can extend the vegetative growth period before late-summer flowering, which is expected to occur generally 4–5 weeks after the summer solstice in the northern hemisphere, dependent upon hemp variety and latitude (Cherney and Small, 2016; Anderson et al., 2019). Relatively short day length experienced in tropical and subtropical regions result in reduced vegetative growth and early seasonal transition to flowering that ultimately limits stem elongation and fiber biomass yield, key factors for successful commercial cultivation of industrial hemp (Cosentino et al., 2012; Hall et al., 2014). Thus, genotypes of hemp adapted to higher latitudes would be expected to perform poorly when cultivated in tropical and subtropical environments due to premature flowering and the negative influence it has on plant growth and yield (Amaducci et al., 2008; Cosentino et al., 2012; Hall et al., 2012).
Hemp expresses broad genetic diversity in hemp photoperiod requirements for vegetative-to-reproductive transition requirements, similar to that seen in other major crops (e.g., maize; Navarro et al., 2017). Identifying plant genotypes adapted to light conditions of a region is key to the successful cultivation of photoperiod crops, such as hemp (Jung and Müller, 2009; Cho et al., 2017). Zhang et al. (2018) discovered that Cannabis could be generally categorized in three northern hemispheric haplogroups distinguished by geographical location (north of 40°N, 30 to 40°N, and south of 30°N); however, a myriad of photoperiod responses can be observed when breeding among haplogroups. Hemp selected for fiber production is generally believed to be a quantitative SDP with a relatively long photoperiod, around 14 h (dependent upon the origin of the plant material). Excluding European varieties, the photoperiod response of most industrial hemp is poorly documented. “Kompolti” (Hungarian variety) and “Futura 77” (French variety) have an estimated maximal optimum photoperiod of 13.8 and 14 h, respectively (Heslop-Harrison and Heslop-Harrison, 1969). An estimated photoperiod of roughly 14 h was identified by Amaducci et al. (2008) for five European hemp cultivars as the most important single factor controlling flowering date. Flowering was increasingly delayed at longer photoperiods, but a 24-h photoperiod did not prevent “Fedrina 74” (French variety) and “Kompolti Hybrid TC” (Hungarian variety) from flowering (Van der Werf et al., 1994; Lisson et al., 2000). A Portuguese fiber variety was reported to have a maximum optimal photoperiod of 9 h, although the critical photoperiod is somewhere between 20 and 24 h (Heslop-Harrison and Heslop-Harrison, 1972; Lisson et al., 2000). In contrast to European hemp, the flowering of Chilean and US Kentucky hemp varieties occurred promptly under a photoperiod of 14 h or fewer but was considerably delayed or failed to flower when photoperiod exceeded 16 h (Borthwick and Scully, 1954). A subtropical Australian variety, “BundyGem,” had a critical photoperiod between 13 h 40 min and 14 h 40 min, and plant maturity was significantly delayed when day length exceeded 14 h 40 min (Hall et al., 2014). A photoperiod of 11–12 h has been reported to induce flowering of Thai hemp (Sengloung et al., 2009). While most of the studies on hemp have been conducted in the field or greenhouses, plant responses to environmental factors in a more strictly controlled environment, such as growth chambers, are very limited.
Until recently, Cannabis plants grown for recreational use have largely been cultivated indoors using artificial lighting. Given that most of these cultivation operations were conducted before the legalization of marijuana and were thus illegal, critical photoperiods of these types of Cannabis plants are not documented in the literature, and information is limited. A day length of 12 and 18 h are common practices to induce flowering or keep plants vegetative, respectively (Potter, 2014). Moher et al. (2020) indicated that C. sativa “802,” although not categorized as hemp given its 15–20% THC content, had a critical photoperiod between 15 and 16 h. Growth chamber environments are ideal for investigating the photoperiodism of hemp. With artificial lighting (typically from light-emitting diodes) being the only radiation source indoors, the photoperiod is strictly controlled by the hours of light operation. In tropical and subtropical regions, the vegetation of hemp under long days can be achieved in protected environments, such as greenhouses, by manipulating photoperiod utilizing end-of-day extension lighting and night interruption techniques that have been utilized in the production of other common SDPs (Lane et al., 1965; Vince-Prue and Canham, 1983; Runkle et al., 1998; Zhang and Runkle, 2019). However, since hemp is often cultivated outdoors to reduce production costs, it is imperative that it is germinated or transplanted at timing with respect to natural photoperiod. Prediction of flowering time in response to a specific, known photoperiod is thus critical to support successful production both outdoors and indoors and optimize select hemp varieties for a diverse range of growing regions. To directly address these needs, we investigated 15 cultivars of essential oil hemp, and 12 cultivars of fiber/grain hemp with seven growth rooms to (i) empirically define critical photoperiod thresholds to induce vegetative to floral transition in diverse hemp cultivars; (ii) compare critical photoperiod thresholds to flowering dates within a subtropical field environment; and (iii) quantify the physiological response of hemp cultivars under different photoperiod treatments.
Seeds, cuttings, or plants of all essential oil cultivars were obtained from three different sources (Supplementary Table 1). Cultivars were selected based on commercial interest and availability. Seeds of five essential oil cultivars, “Cherry Wine-BS,” “Cherry Blossom-BS,” “Cherry*T1-BS,” “Berry Blossom-BS,” and “Cherry Blossom-Tuan-BS,” were sown in 72 round cell propagation sheets (DPS72, The HC Companies, Twinsburg, OH) within Pro-Mix soilless substrate (HP Mycorrhizae Pro-Mix; Premier Tech Horticulture Ltd., Quakertown, PA) containing 65–75% peat, 8–35% perlite, dolomite limestone, and mycorrhizae on November 19, 2019. Cuttings of the other 10 essential oil cultivars, “ACDC-AC,” “Super CBD-AC,” “Cherry-AC,” “Wife-AC,” “Cherry Blossom-BC,” “JL Baux-CC,” “ACDC-CC,” “Cherry Wine-CC,” “Cherry-CC,” and “Wife-CC,” were propagated on November 25, 2019. Each cultivar was propagated from identical mother stock plants to reduce potential genetic diversity among replicates. Stems of plant propagules were dipped into rooting hormone (Dip'N Grow; Dip'N Grow Inc., Clackamas, OR) containing 1,000 mg/L indole-3-butyric acid (IBA)/500 mg/L naphthaleneacetic acid (NAA) and then inserted into 3.8 cm Rockwool cubes (Grodan; ROXUL Inc., Milton, Canada) that were pre-soaked with water exhibiting a pH of 5.8 as per manufacturer recommendations. Both seeded trays and cuttings were grown at 25°C under 24-h photoperiod and were hand irrigated daily as needed.
After roots were well-established (~21 days), the most uniform rooted propagules of each cultivar were selected and transplanted into 1.1 L containers (SVD-450, T.O. Plastics, Clearwater, MN) filled with Pro-Mix soilless substrate and top-dressed with 5 g of Osmocote Plus 15-9-12 5-6 month slow-release fertilizer (Everris NA, Inc.; Dublin, OH) containing 7% ammoniacal and 8% nitrate nitrogen, 9% phosphate and 12% soluble potash on December 17, 2019. Plants were randomly assigned to seven identical controlled rooms with 10 replicates per cultivar in each room and were cultivated at 25°C under a photoperiod of 18 h (0600–2400 HR) for vegetative growth. Plants were irrigated for 4 min every 5 days for the first 2 weeks and 4 min every 3 days thereafter as controlled by an automatic irrigation system.
After 3 weeks of vegetative growth following transplant, seven lighting treatments were randomly assigned to each controlled room on January 7, 2020. Ten plants of each hemp cultivar were grown at 25°C under the photoperiod of 12 h (0600–1800 HR), 12 h 30 min (0600–1830 HR), 13 h (0600–1900 HR), 13 h 30 min (0600–1930 HR), 13 h 45 min (0600–1945 HR), 14 h (0600–2000 HR), and 18 h (0600–2400 HR) provided by light-emitting diodes (LEDs) (VYPR 2p; Fluence Bioengineering, Inc., Austin, TX). Lighting treatments were maintained until the termination of the experiment 5 weeks following the vegetative growth period.
Propagation of cuttings and germination of seeds was conducted indoors in an environmentally controlled propagation room at the Mid-Florida Research and Education Center (Apopka, FL). Air temperatures were maintained in all indoor grow rooms utilizing air conditioners set to 25°C. Air temperature and relative humidity data were collected by thermocouples installed at plant canopy height, and data was recorded using a wireless data logging station (HOBO RX3000; Onset Computer Corporation, Bourne, MA) every 10 min. The average temperature in the propagation room was 24.9 ± 0.04°C. Within the propagation room, a 24-h photoperiod was provided by fluorescent lamps (E-conolight; Sturtevant, WI) as sole-source lighting. The photosynthetic photon flux density (PPFD) on the propagation bench was measured by a quantum sensor (MQ-500; Apogee Instruments Inc., Logan, UT) at 10 representative positions at seedling canopy level. The average PPFD that cuttings and seedlings received was 53.9 ± 3.02 and 73.5 ± 3.61 μmol·m−2·s−1, respectively, with a daily light integral of ~4.7 and 6.4 mol·m−2·d−1, respectively.
Following the transplant, all plants were cultivated in seven identical environmentally controlled rooms. Each room was equipped with two sole-source LEDs (VYPR 2p; Fluence Bioengineering, Inc., Austin, TX) regulated by a timer (Titan Controls Apollo 8; Hawthorne Gardening Company, Vancouver, WA) to provide varying controlled photoperiod treatments. A PPFD of ~300 and 330 μmol·m−2·s−1 was maintained at plant canopy height at the onset of the vegetative and flowering stages, respectively. Average temperature, relative humidity, and light intensity for vegetative and flowering stages for each lighting treatment are reported in Supplementary Table 2.
The flowering of female hemp plants is defined as the appearance of dual, fork-shaped stigmas protruding from tubular bracts (Hall et al., 2012) being visible at the apical meristem or decimal code of 2201 defined by Mediavilla et al. (1998). The flowering of male hemp plants is defined when five radial segments of the first pointed male bud open and start to release pollen (Hall et al., 2012) or decimal code of 2101 (Mediavilla et al., 1998). Plant height (from the substrate surface to the tallest meristem) was measured at the initiation of lighting treatments and flowering. Extension growth was calculated by subtracting initial plant height from height at flowering. Days to flower and plant sex were recorded when plants initiated flowering. Boolean evaluation of plant flowering status (flowering percentage) was conducted at the end of week 5 following the initiation of lighting treatments.
The experiment was conducted using a complete randomized design with seven lighting treatments and multiple replicates. Each plant was considered an experimental unit. Data were pooled from multiple replicates and were analyzed with a restricted maximum likelihood mixed model analysis in JMP® Pro 15 (SAS Institute, Inc., Cary, NC) with post hoc mean separation tests performed using Tukey's honest significant difference test at P ≤ 0.05. Normality of the residuals was evaluated with QQ-plots and the Anderson-Darling A2 statistic goodness of fit test in JMP. Homogenous variances were tested using Levene's test.
Twelve fiber/grain hemp cultivars from seven different source origins were purchased, including Canada cultivars— “CFX-1” and “Joey;” Poland cultivar— “Tygra”; Serbia cultivar— “Helena”; Italy cultivars— “Carmagnola Selezionata,” “Fibranova,” and “Eletta Campana”; North China cultivar— “HAN-FN-H;” Central China cultivars— “HAN-NE” and “HAN-NW”; and South China cultivars— “PUMA-3” and “PUMA-4.” Seeds were sown in 72 round cell propagation sheets (DPS72, The HC Companies, Twinsburg, OH) filled with Pro-Mix HP soilless substrate on February 18, 2020. They were placed under a mist bench in a greenhouse and grown at 25°C under natural daylight supplemented with 1,000 W metal halide lighting to maintain an 18-h photoperiod. Seedlings were misted for 1 min at 8 a.m., 12 p.m., and 5 p.m. each day.
Seedlings possessing the most uniform height were selected three weeks after germination when roots were well-established and transplanted into 1.1 L containers, as described above, with Fafard 4P potting media (Sun Gro Horticulture Canada Ltd., Agawam, MA) containing 48% peat, 30% pine bark, 10% perlite, and 12% vermiculite, top-dressed with 5 g of Osmocote Plus slow-release fertilizer as described above. Plants were randomly assigned to seven identical environmentally controlled rooms with 10 replicates for “CFX-1,” “Tygra,” “Helena,” “Eletta Campana,” “HAN-FN-H,” and “HAN-NE”; 9 replicates for “PUMA-3;” 7 replicates for “Joey” and “Fibranova;” 6 replicates for “PUMA-4”; and 4 replicates for “Carmagnola Selezionata” and “HAN-NW” due to poor germination rates. Plants were grown at 25°C under an 18-h photoperiod (0600–2400 HR) for vegetative growth until the initiation of photoperiod treatments.
Seven lighting treatments were randomly assigned to each controlled room after 2 weeks of plant vegetative growth on March 23, 2020. Twelve fiber/grain hemp cultivars were subjected to seven photoperiod treatments: 12 h (0600–1800 HR), 13 h 30 min (0600–1930 HR), 13 h 45 min (0600–1945 HR), 14 h (0600–2000 HR), 14 h 30 min (0600–2030 HR), 14 h 45 min (0600 HR−2045 HR), and 18 h (0600–2400 HR) provided by LEDs. Treatments were selected based on the common photoperiod range of fiber/grain cultivars documented in the literature and the expected photoperiod of tropical and subtropical regions. Lighting treatments were maintained for 5 weeks before the termination of the experiment.
Germination of seedlings was conducted in a research greenhouse under a mist bench. Greenhouse heaters and fans were controlled by an environmental control system (Wadsworth Control System, Arvada, CO) and operated when greenhouse temperature was ≤ 16°C or ≥ 24°C, respectively. Seedlings were misted for a duration of 1 min three times per day utilizing a programmable irrigation controller (Sterling 12; Superior Controls Co., Inc., Valencia, CA) and subjected to an 18-h photoperiod (from 0700 HR to 0100 HR) with 11 h of ambient solar radiation (from 0700 HR to 1800 HR) and 8 h of supplemental metal halide 7,500°K lamps (UltraSun 1,000 W; Hawthorne Hydroponics LLC., Vancouver, WA) that operated from 1700 HR to 0100 HR. Greenhouse environmental conditions were recorded every 15 min by a weather station data logger (WatchDog 2475; Spectrum Technologies, Inc., Aurora, IL). Average air temperature, relative humidity, photosynthetic active radiation, and daily light integral was 24.0 ± 0.08°C, 60.1 ± 0.45%, 261.6 ± 5.07 μmol·m−2·s−1, and 22.6 ± 0.44 mol·m−2·d−1, respectively.
After transplant in 1.1 L containers, all plants were cultivated in seven identical environmentally controlled rooms, as described previously. Average air temperature, relative humidity, and light intensity for the vegetative stage and flowering stage for each lighting treatment were also reported in Supplementary Table 2.
Plant height, recorded from the substrate surface to the tallest meristem, was measured at the initiation of lighting treatments and at flowering. Days to flower and plant sex were recorded when plants started to flower, as defined previously. The flowering of monoecious plants was defined when a female or male flowering occurred as defined previously or by decimal code of 2301 and 2304, respectively (Mediavilla et al., 1998). Boolean evaluation of plant flowering status (flowering percentage) was conducted at the end of week 5 after the initiation of the lighting treatments. Experimental design and data analysis were conducted as described for Expt. 1.
Based on results of Expts. 1 and 2, an expanded photoperiod trial was designed with select essential oil, fiber, and grain cultivars to better understand the effect of photoperiodism on a broader scale.
Six fiber/grain hemp cultivars, “Carmagnola Selezionata,” “Helena,” “Eletta Campana,” “HAN-FN-H,” “PUMA-3,” and “PUMA-4,” were propagated as described in Expt. 2 on May 24, 2020. Cuttings of 10 essential oil cultivars, “ACDC-AC,” “Super CBD-AC,” “Cherry-AC,” “Cherry Blossom-BC,” “Cherry Wine-BS,” “Cherry Blossom-BS,” “Cherry*T1-BS,” “JL Baux-CC,” “ACDC-CC,” and “Cherry-CC,” were propagated as described in Expt. 1 on June 18, 2020. Both cuttings and seeded trays were placed under a mist bench that misted 8 s every 20 min in a greenhouse and grown at 25°C under natural daylight with supplemental metal halide lamps as described in Expt. 2 maintaining an 18-h photoperiod. Plants grew vegetatively under the mist bench in the greenhouse for 3–4 weeks before being transplanted into 1.1 L containers and assigned to lighting treatments.
Seedlings or clones of the 10 essential oil cultivars were thinned and transplanted as described in Expt. 2 with Pro-Mix HP soilless substrate on June 18, 2020, for fiber cultivars, and July 11, 2020, for essential oil cultivars. Slow-release fertilizer was applied as described in Expt. 1. All plants were cultivated for vegetative growth for 7 days and then randomly assigned to identical environmentally controlled rooms under different lighting treatments with five replicates per cultivar.
Six lighting treatments were randomly assigned to each controlled room as proposed: 12 h 30 min (0600–1830 HR), 13 h (0600–1900 HR), 14 h 30 min (0600–2030 HR), 14 h 45 min (0600–2045 HR), 15 h (0600–2100 HR), and 15 h 30 min (0600–2130 HR). Different photoperiods were provided by LEDs as described previously. Photoperiod lighting treatments were maintained for 5 weeks before the termination of the experiment. Ten essential oil cultivars were selected based on the results from Expt. 1 and were evaluated from 14 h 30 min to 15 h 30 min.
A Boolean evaluation of flowering status (flowering percentage) was conducted as described previously. Environmental conditions of the greenhouse during the vegetative stage were as described in Expt. 2, and the environmental conditions of the controlled rooms during the flowering stage were as described in Expt. 1 and provided in Supplementary Table 2. Experimental design and data analysis were conducted as described for Expt. 1.
Fourteen essential oil and 12 fiber/grain cultivars were evaluated for flowering response time under natural daylength, field-grown conditions following seedling establishment of fiber/grain cultivars and rooting of clonally propagated essential oil cultivars. Fiber/grain seeds were sown in 72-cell trays within Pro-Mix HP soilless substrate on April 30, 2020, and propagated as described in Expt. 2. Seedlings were watered daily by hand as needed. Fourteen essential oil cultivars were clonally propagated as described in Expt. 2 on May 1, 2020. Rooted plants were transplanted into the field on June 3, 2020.
The field trial was designed using plasticulture production techniques with Chapin Turbulent Flow-Deluxe drip tape (Catalog # 11714142N, Jain Irrigation USA, Watertown, NY) placed below the plastic emitting 0.76 L h−1 per dripper at 68.9 kPa spaced 0.10 m between drippers. Plants were spaced 0.9 m apart within rows, and rows were spaced 1.5 m apart between row centers. Total plot lengths were 3.7 m, including walking allies. The total trial area was 0.9 ha. Trials received 2 h of drip irrigation per day. A soluble fertilizer with micronutrients (Peter 20-20-20; ICL Specialty Fertilizers, Dorchester County, SC, USA) was applied every 14 days at 8.8 kg N ha−1 for an accumulated rate of 48 kg N ha−1 (six applications total).
The experiment was conducted using a complete randomized block design comprised of an essential oil trial and a fiber/grain trial. Both trials contain three replicates of each cultivar. Each plot/replicate within the trial consisted of three plants. Flowering time was measured as defined previously. During the civil twilight period (sun 6–0° below the horizon), light intensity was recorded every 2 min manually in an open field with a quantum sensor (MQ-500; Apogee Instruments Inc., Logan, UT) for 3 days. A restricted maximum likelihood mixed model analysis in JMP® Pro 15 (SAS Institute, Inc., Cary, NC) was performed to estimate genetic means of flowering time.
Critical photoperiod differed significantly among essential oil cultivars (Table 1). In addition, a significant effect was observed on flowering percentages of the essential oil cultivars. As expected, all essential oil cultivars in Expt. 1 flowered in response to 12-h photoperiod, and no plants flowered in response to 18 h (Table 1). One cultivar, “Cherry Wine-CC,” was identified with a critical photoperiod below 14 h, with no flowers developed above 14 h. Four cultivars expressed 100% floral initiation at the longest photoperiod (14 h, excluding 18 h control), with an additional six cultivars that demonstrated a complete floral initiation (>50%) when cultivated under 14 h of light. For this reason, expanded photoperiod treatments of up to 15 h 30 min were evaluated for select essential oil cultivars (Expt. 3). Of the 10 essential oil cultivars evaluated within Expt. 3, five cultivars expressed a majority (>50%) of floral initiation between 15 h and 15 h 30 min. Four of them, including “Cherry-AC,” “Cherry Blossom-BS,” “ACDC-CC,” and “Cherry-CC,” have been identified with a critical photoperiod within this range. In addition, “Cherry Wine-CC” had the shortest, critical photoperiod identified between 13 h 45 min and 14 h. The critical photoperiod for “Super CBD-AC” and “Cherry Blossom-BC” occurred between 14 h 45 min and 15 h. For the rest of the cultivars, “ACDC-AC” had a significant flowering reduction when the photoperiod was extended from 15 h to 15 h 30 min. “Wife-AC” flowered significantly less under 13 h 30 min compared with 13 h, but the critical photoperiod is likely >14 h. Similarly, the percentage flowering of “Berry Blossom-BS,” “Cherry Blossom-Tuan-BS,” and “Wife-CC” decreased when photoperiod was increased from 13 h 45 min to 14 h. Our results suggest that a photoperiod difference of as little as 15 min could significantly influence floral initiation and development of some essential oil hemp cultivars. Moreover, floral initiation can occur at varying rates when the photoperiod is close to the critical threshold of some cultivars.
Less variation in critical photoperiod thresholds was observed for fiber/grain hemp than essential oil cultivars. Like essential oil cultivars, all fiber/grain cultivars flowered in response to a 12-h photoperiod (Table 1). For the majority of the fiber cultivars (8 of 12), plants did not flower under an 18-h photoperiod. “CFX-1,” “Joey,” “Tygra,” and “Helena” flowered in response to a photoperiod of 18 h and did not remain vegetative like the majority of the other fiber hemp cultivars evaluated in this study, thus suggesting their critical photoperiod could be above 18 h. The critical photoperiod of PUMA 3 and 4 was identified between 13 h 45 min and 14 h, but the floral initiation was greatly reduced by more than 70% when day length exceeded 13 h. Similarly, the flowering of HAN-NE and HAN-NW was also greatly reduced when day length exceeded 14 h 30 min. To verify the critical photoperiod of “CFX-1,” “Joey,” “Tygra,” and “Helena,” seeds were germinated on February 18, 2020, and placed under a 24-h photoperiod in a greenhouse. All four cultivars flowered on April 20, 2020, under a 24-h photoperiod. This observation was consistent with previous reports where a 24-h photoperiod did not prevent the flowering of “Fedrina 74” and “Kompolti Hybrid TC” and that the critical photoperiod of a Portuguese fiber hemp variety from Coimbra is between 20 and 24 h (Heslop-Harrison and Heslop-Harrison, 1972; Van der Werf et al., 1994). In addition, “CFX-1” and “Joey” formed flower buds during the 3-week propagation stage in the greenhouse in Expt. 2. Available literature supports that primordium formation in hemp varieties occurs in response to quantitative short days, and the photoperiod inductive phase is jointly affected by photoperiod and temperature (Lisson et al., 2000; Hall et al., 2012). However, Spitzer-Rimon et al. (2019) argued that Cannabis could enter the reproductive phase under both long-day and short-day conditions because “solitary flowers,” which are developed in the axil of each stipulate leaf, are differentiated under such conditions. Flower induction of “solitary flowers” is likely age-dependent and is controlled not by photoperiod but rather internal signals. Therefore, they reported that Cannabis could be considered a day-neutral plant where floral initiation is not dependent upon photoperiod requirements. These “solitary flowers,” which can be referred to as sex-indicating flowers or pre-flowers, are believed to be the start of calyx development in hemp and are not photoperiod dependent (Green, 2017; Williams, 2020). In our study, long-day conditions did not prevent the floral initiation of “CFX-1,” “Joey,” “Tygra,” and “Helena.” Thus, they are likely day-neutral cultivars given floral initiation occurred in response to a 24-h photoperiod.
Hall et al. (2012) suggested that the hemp juvenile phase was not affected by photoperiod, and the length of the juvenile phase is either determined by the development of reproductive organs or the apical meristem, which is independently timed to produce flowering signals. In our study, the length of the juvenile phase was observed to be cultivar-specific, with “CFX-1” being the shortest and “Helena” is the longest among the four day-neutral cultivars (Figure 2). Traits, such as days to maturity and cannabinoid production, have been identified to be nearly entirely controlled by genetics. However, the environment can play a significant role in other traits, such as yield and plant height, and thus the influence of environment and genetics are likely needed to be considered collectively (Campbell et al., 2019). Different hemp cultivars have been suggested to have different lengths of juvenile phase and photosensitive phase, largely in association with geographic origin. Cultivars adapted to northern latitudes tend to have a short life cycle and grow and flower faster within their limited growing seasons, whereas cultivars adapted to southern latitudes and closer to the equator tend to flower later to ensure sufficient vegetative growth before short days occur (Amaducci et al., 2008; Small, 2015; Zhang et al., 2018). This theory is supported by our study results where cultivars of northern origin (“CFX,” “Joey,” “Tygra,” etc.) responded to a longer photoperiod and flowered faster than southern cultivars (“PUMA-3,” “PUMA-4,” “HAN-NW,” etc.) having a shorter critical day length threshold (Figure 2). Thus, understanding the juvenile phase and photosensitivity is essential for selecting the right hemp cultivar for a target region.
Some plant species can respond to light even at a very low intensity and are thus considered highly photosensitive. For these species of plants, civil twilight, or the period that occurs shortly before sunrise and after sunset when the sun is between 0 and 6° below the horizon, may still be biologically effective to the plant's photoperiodism response (Salisbury, 1981; Kishida, 1989). For example, rice (Oryza sativa) is light-insensitive to twilight both at dusk and dawn; perilla (Perilla frutescens) and Biloxi soybean (Glycine max) are light-insensitive at dusk but more light-sensitive at dawn; and cocklebur (Xanthium saccharatum) is both light-sensitive at dusk and dawn (Takimoto and Ikeda, 1961). For hemp, Borthwick and Scully (1954) suggested that 0.12 ft-candle or more would sufficiently prevent hemp from flowering, suggesting hemp is extremely sensitive to light. In our experience, light intensity as little as 2 μmol·m−2·s−1 can cause light pollution and disrupt the flowering for “Cherry Blossom-BS” in the greenhouse. To evaluate the effect of twilight on hemp, we conducted field trials (Expt. 4) to investigate the performance of essential oil, fiber, and grain hemp cultivars under natural day light conditions. The average light intensity during civil twilight period in our study was 2.4 ± 0.54 μmol·m−2·s−1, which is within the light range reported by Kishida (1989). By comparing plant response and photoperiod to sunrise to sunset daylengths and civil twilight lengths, our results support the hypothesis that flowering performance of hemp is affected by civil twilight (Figure 1). Most flowering data from the field trial were in alignment with our trials from the controlled rooms (Expt. 1 to 3) and plants flowered around the critical photoperiod we tested, with a few exceptions. “Cherry Wine-BS”, “Cherry Blossom-BS”, “Cherry-AC”, “Super CBD”, and “ACDC-AC” flowered later and slower in the field compared with the controlled rooms (Figure 1). It is possible that the day length changes under the natural conditions are slower to occur and not as drastic compared with conditions imposed in the controlled rooms and therefore plants would respond to day length changes slower under natural conditions. On the contrary, “Cherry Wine-CC” and “PUMA-4” flowered earlier, suggesting that these cultivars might be more sensitive to the dark period. In addition, differences in individual perception of flowering initiation may have led to the reduced correlation between field and growth chamber floral initiation dates collected for these cultivars. Additional years of field trials will aid in the importance of civil twilight's effect on Cannabis flowering. Collectively, we believe that civil twilight length and the slow progression of day length changes under natural conditions should be taken into consideration for the biologically effective photoperiod for hemp flowering. Additional rigorous experiments involving artificial dawn and dusk regimes in controlled rooms is needed to further verify the hypothesis.
Figure 1. Flowering date of essential oil, grain, and fiber cultivars after transplanting from greenhouse to field conditions on June 3, 2020. Points depict genetic means, and error bars represent 95% confidence intervals. The floating text depicts dates of 15-min daylength intervals (top), sunrise to sunset daylengths (middle), and civil twilight lengths (bottom).
Flowering response was delayed as flowering photoperiod increased. In both essential oil and fiber/grain cultivars, plants subjected to 12-h photoperiod had an average flowering time of 13–14 days (Figure 2). This is supported by Borthwick and Scully (1954) where 10–14 days of short-day photoperiod was sufficient for flower induction in at least some of the Chilean and Kentucky varieties.
Figure 2. Days to flower after lighting treatment initiated and height extension growth at the flowering of (A) essential oil and (B) fiber/grain cultivars under different photoperiods, cultivars, and/or sex from Expt. 1 and 2. Means sharing a letter are not statistically different by Tukey's honest significance difference test at P ≤ 0.05. Error bars indicate standard error.
Among essential oil cultivars, flowering was generally delayed by 1–2 days when photoperiod exceeded 13 h compared with 12 h, and flowering was significantly delayed by 7–8 days when photoperiod exceed 14 h (Figure 2). Across cultivars and regardless of sources, “ACDC” and “Super CBD” flowered the fastest, with an average flowering time of 13 days after initiation of the critical photoperiod. “Wife-AC,” “Wife-CC,” and “Cherry Wine” had an average flowering time of 21 d, suggesting these cultivars took longer to either perceive the photoperiod or to complete flower formation and initiation.
Essential oil hemp cultivars demonstrated delayed floral initiation at longer photoperiods and significant genetic variation in floral initiation across photoperiod treatments. Variance in observed photosensitivity is likely a result of genetic variation that influenced floral initiation response to light cues. Flowering for most essential oil cultivars was delayed by 5–13 days under a 14-h photoperiod compared with 13 h 45 min (Figure 3 and Supplementary Figure 1). Flowering of “ACDC-AC” and “Cherry*T1-BS” was significantly delayed by 4 and 6 days, respectively, under 13 h 45 min compared with 12 h. Moreover, the delayed flowering of “Cherry-CC” started at 13 h 30 min and in “Wife-CC,” 13 h. This suggests that floral initiation of these cultivars was more sensitive to photoperiod than others. In contrast, no significant differences were observed in days to flower among different treatments of “Wife-AC,” “Berry Blossom-BS,” and “Cherry Blossom-Tuan-BS,” suggesting the flowering formation and initiation were rather similar under different day lengths, as long as they were below the critical photoperiod.
Figure 3. Comparison of (A) days to flower and (B) height extension growth at flowering of 10 essential oil cultivars with the same name but different sources in Expt. 1. All data were pooled from 10 replications except “ACDC-AC” (n = 9). NS indicates insignificant treatment effects. NA indicates less than four valid data under such treatment. Means sharing a letter are not statistically different by Tukey's honest significance difference test at P ≤ 0.05. Error bars indicate standard error.
Cultivars represented by the same name acquired from different sources performed differently in days to flower. A photoperiod of 13 h 30 min significantly delayed the flowering of “ACDC-CC” but not “ACDC-AC,” whereas 13 h 45 min delayed the flowering of “ACDC-AC” but not “ACDC-CC” (Figure 3). Delay of flowering in “Cherry-CC” started under a photoperiod of 13 h 30 min. In comparison, flowering occurred 30 min later at 14 h for “Cherry-AC.” A photoperiod of 13 h delayed flowering of “Wife-CC” by 8 days compared with 12 h, but not in “Wife-AC.” Similarly, flowering of “Cherry Wine-BS” was significantly delayed by 7 days under a 13-h photoperiod compared with a 12-h photoperiod. However, no differences in flowering were observed in “Cherry Wine-CC.” In conjunction with Sawler et al. (2015), these results indicated that plants with the same cultivar names from different sources could have varying genetics and subsequently performed differently.
Most fiber/grain cultivars tested did not flower under an 18-h photoperiod (Table 1). Flowering was delayed by 1–3 days if the photoperiod exceeded 14 h, and no differences were observed among treatments beyond 14 h (Figure 2). This is consistent with the theory that hemp is a quantitative SDP and has a photoperiod of roughly 14 h where flowering would occur promptly below 14 h and flowering would be delayed under a longer photoperiod (Borthwick and Scully, 1954; Heslop-Harrison and Heslop-Harrison, 1969; Amaducci et al., 2008; Hall et al., 2014). When subjected to the critical photoperiod, average days to flower was shortest among cultivars from northern latitudes and longest among those from southern latitudes with a gradient response correlated to the cultivar's genetic origin. More specifically, Canada/Northern Europe cultivars flowered 4–11 days after lighting transition. Cultivars from comparatively lower latitudinal regions (North China/Italy) flowered from 12 to 16 days, whereas South China cultivars flowered 21–25 days (Figure 2 and Supplementary Figure 2). Moreover, different photoperiod treatments did not affect the flowering of “CFX-1,” “Joey,” “Tygra,” “Carmagnola Selezionata,” and “Helena” (Supplementary Figure 2). Collectively, considering that 24-h day length did not prevent “CFX-1,” “Joey,” “Tygra,” and “Helena” from flowering and their flowering process was not influenced by imposed photoperiods (Table 1 and Supplementary Figure 2), these four fiber cultivars are likely photoperiod insensitive or day-neutral.
Temperature differences and other stresses such as nutrient deficiencies can result in differences in flowering time (Amaducci et al., 2008; Hall et al., 2012). Amaducci et al. (2008) indicated that high temperature would accelerate flowering by decreasing the duration between the formation of flower primordia and full flowering, and thus modeling had been used to predict flowering time based on day length and temperature. Hall et al. (2012) also indicated that the photoperiod inductive phase of hemp is jointly influenced by air temperature and photoperiod. In our study, growing conditions including air temperature and nutrient fertility are nearly identical in each environmentally controlled room and thus did not contribute to differences in plant flowering performances. As recorded in this study, the flowering response was, therefore, free from the confounding influence of temperature and nutrient deficiency (excluding Expt. 4) and thus provides an enhanced foundational understanding of relationships between photoperiod and flowering response in hemp.
Collectively, based upon study findings and available literature, we believe the hemp juvenile phase to be controlled by genetics rather than photoperiod or temperature. The pre-flowering of the single sex-indicating flower at the axillary is photo insensitive. The response to photoperiod from pre-flowering to flowering at the apical meristem is affected by both photoperiod and temperature and can be either quantitative (most cultivars) or day-neutral (such as “CFX-1,” “Joey,” “Tygra,” and “Helena”), dependent upon cultivar.
Hemp cultivated within northern latitudes generally has a longer stem and greater biomass due to the late flowering and prolonged vegetative phase (Hall et al., 2014; Tang et al., 2016; Salentijn et al., 2019). The highest stem yield is usually obtained by late-maturing cultivars (Höppner and Mange-Hartmann, 2007). Generally, plant height increased as day length increased, as would be expected from increased photosynthesis. In our study, plant height extension growth was 47–102% greater under a 14-h day length compared with the 12-h day length, the control group in nine essential oil cultivars, while the imposed photoperiods did not affect the remaining six cultivars (Figure 2). The longer stem could have resulted from a longer vegetative stage caused by the delay in floral initiation, which has been reported on a variety of crops (Craig and Runkle, 2013; Zhang and Runkle, 2019). This is also supported by Höppner and Mange-Hartmann (2007), where stem length is positively correlated with the vegetative phase duration. However, under certain photoperiod treatments, flowering was delayed, but the height extension growth was not affected; this included “Cherry Wine-BS,” “Cherry Wine-CC,” and “Wife-CC” under 13 h, “ACDC-CC” under 13 h 30 min, “ACDC-AC” and “Cherry*T1-BS” under 13 h 45 min, and “Cherry-CC” under both 13 h 30 min and 13 h 45 min (Figure 3 and Supplementary Figure 1). Results indicated that flowering initiation of essential oil hemp was more sensitive than extension growth in response to photoperiods. In addition, unlike flowering, extension growth of essential oil cultivars with the same name but from different sources generally responded similarly, except “Cherry Wine.” Campbell et al. (2019) indicated that plant height was collectively influenced by both environment (e.g., irrigation) and genetics, accounting for 38 and 36% of the variance, respectively. We concluded that similar height extension growth among essential oil cultivars under different treatments was due to similar irrigation applications.
In contrast to essential oil cultivars, extension growth of fiber/grain cultivars was not affected by photoperiod, except “CFX-1” and “HAN-NE” (Supplementary Figure 3). Height extension of “HAN-NE” was 58–64% greater when day length exceeds 14 h due to the later flower initiation development. Interestingly, stem extension of “CFX-1” was shorter under an 18-h photoperiod compared with a 13 h 30 min photoperiod. We believe “CFX-1” is photo insensitive, and individual variances caused this difference.
Plant sex was recorded and calculated across the lighting treatments for fiber/grain hemp cultivars (Table 2). Among all the fiber/grain hemp cultivars tested, most cultivars had a relatively equal proportion of male and female plants in general with a small occurrence of monoecious plant development, which is consistent with Hall et al. (2012). “Tygra” had the highest proportion of monoecious plants (55.7%) with a small proportion of female plant development (4.3%). “Helena” was one-third monoecious. Most of “CFX-1” were female plants with no monoecious plants that developed. The sex of more than 25% of “HAN-NE,” “HAN-NW,” “PUMA-3,” and “PUMA-4” could not be determined due to a lack of flowering response throughout our experiment.
Overall, the flowering of female and monoecious plants was delayed by 1–2 days compared with male plants (Figure 3). Our observations were supported by Borthwick and Scully (1954) findings where the greater flowering delay occurred in male plants compared with female plants under long photoperiods. Hall et al. (2012) and Van der Werf et al. (1994) suggested extending the day length would alter the sex proportion of flowering hemp plants and that male and monoecious plants would fail to flower when photoperiod exceeded the optimal day length. Additionally, Van der Werf et al. (1994) suggested that unlike male hemp plants, female flowering would be less influenced by photoperiod. We did not observe such trends in fiber hemp cultivars, and no flowering pattern or changes in flowering percentage were identified when the photoperiod exceeded the optimal 14 h. Female hemp also has a significantly shorter extension growth than male and monoecious plants at flowering (Figure 3).
This research reported flowering and growth of 27 hemp cultivars in response to different photoperiods under both indoor controlled and outdoor natural environments. Most of the essential oil cultivars and some southern fiber/grain cultivars (such as “PUMA-3” and “PUMA-4”) tested express suitable photoperiods for tropical and sub-tropical region cultivation. Pre-flowering of hemp is photo insensitive, but the response to photoperiod from pre-flowering to flowering can be either quantitative or day neutral. Depending on photosensitivity, a photoperiod difference of as little as 15 min significantly influenced the floral initiation of some essential oil cultivars. Northern fiber/grain hemp cultivars had a shorter juvenile phase and faster flowering than cultivars from southern latitudes. Cultivar name may not be enough to finely estimate photoperiod response for essential oil cultivars. The flowering performance of hemp appears to be influenced by civil twilight, and thus this should be considered when attempting to time cultivation to maximize vegetative and flowering response. Male plants flower faster than female and monoecious plants. Plant height generally increased as the day length increased in essential oil cultivars but not in fiber/grain cultivars.
The raw data supporting the conclusions of this article will be made available by the authors, without undue reservation.
MZ performed the conceptualization, data curation, formal analysis, investigation, methodology, original draft, review and editing (lead), supervision, validation, and visualization. SA performed the conceptualization, data curation, formal analysis, investigation, methodology, supervision, validation, visualization, and review and editing (supporting). ZB performed funding acquisition, project administration, supervision, resources, and review and editing (supporting). BP performed the conceptualization, methodology, project administration, supervision, resources, and review and editing (supporting). All authors contributed to the article and approved the submitted version.
This project was made possible by financial support from Green Roads LLC, Roseville Farms LLC, and the UF/IFAS Office of the Dean and Research.
The authors declare that the research was conducted in the absence of any commercial or financial relationships that could be construed as a potential conflict of interest.
All claims expressed in this article are solely those of the authors and do not necessarily represent those of their affiliated organizations, or those of the publisher, the editors and the reviewers. Any product that may be evaluated in this article, or claim that may be made by its manufacturer, is not guaranteed or endorsed by the publisher.
We would like to acknowledge Brandon White and Chris Halliday for their technical support; James Johnston and Dillan Raab for their hard work and effort in maintaining experimental plants and collecting phenotypic data; Jerry Fankhauser and Sandra Alomar for administrative assistance; Green Point Research, ANO Colorado LLC, and Green Roads LLC for donating the cultivars used in this research; and all members of the University of Florida IFAS Industrial Hemp Pilot Project for their collaboration.
The Supplementary Material for this article can be found online at: https://www.frontiersin.org/articles/10.3389/fpls.2021.694153/full#supplementary-material
Amaducci, S., Colauzzi, M., Bellocchi, G., Cosentino, S. L., Pahkala, K., Stomph, T. J., et al. (2012). Evaluation of a phenological model for strategic decisions for hemp (Cannabis Sativa L.) biomass production across European sites. Ind. Crops Prod. 37, 100–110. doi: 10.1016/j.indcrop.2011.11.012
Amaducci, S., Colauzzi, M., Bellocchi, G., and Venturi, G. (2008). Modelling post-emergent hemp phenology (Cannabis sativa L.): theory and evaluation. Eur. J. Agron. 28, 90–102. doi: 10.1016/j.eja.2007.05.006
Anderson, E., Baas, D., Thelen, M., Burns, E., Chilvers, M., Thelen, K., et al. (2019). Industrial Hemp Production in Michigan. Available online at: https://www.canr.msu.edu/hemp/uploads/files/industrialhempinfosheet_2019-05-24.pdf (accessed February 20, 2021).
Borthwick, H. A., and Scully, N. J. (1954). Photoperiodic responses of hemp. Botanical Gazette 116, 14–29. doi: 10.1086/335843
Campbell, B. J., Berrada, A. F., Hudalla, C., Amaducci, S., and McKay, J. K. (2019). Genotype× environment interactions of industrial hemp cultivars highlight diverse responses to environmental factors. Agrosyst. Geosci. Environ. 2, 1–11. doi: 10.2134/age2018.11.0057
Cherney, J. H., and Small, E. (2016). Industrial hemp in North America: production, politics and potential. Agronomy 6:58. doi: 10.3390/agronomy6040058
Cho, L. H., Yoon, J., and An, G. (2017). The control of flowering time by environmental factors. Plant J. 90, 708–719. doi: 10.1111/tpj.13461
Congressional Research Service (2019). Defining Hemp: A Fact Sheet. Available online at: https://www.everycrsreport.com/files/20190322_R44742_1b0195c6aa7e2cad29256c85a8574347c1ee833d.pdf (accessed October 18, 2020).
Cosentino, S. L., Testa, G., Scordia, D., and Copani, V. (2012). Sowing time and prediction of flowering of different hemp (Cannabis sativa L.) genotypes in southern Europe. Ind. Crops Prod. 37, 20–33. doi: 10.1016/j.indcrop.2011.11.017
Craig, D. S., and Runkle, E. S. (2013). A moderate to high red to far-red light ratio from light-emitting diodes controls flowering of short-day plants. J. Am. Soc. Hort. Sci. 138, 167–172. doi: 10.21273/JASHS.138.3.167
Hall, J., Bhattarai, S. P., and Midmore, D. J. (2012). Review of flowering control in industrial hemp. J. Natural Fibers 9, 23–36. doi: 10.1080/15440478.2012.651848
Hall, J., Bhattarai, S. P., and Midmore, D. J. (2014). Effect of industrial hemp (Cannabis sativa L.) planting density on weed suppression, crop growth, physiological responses, and fibre yield in the subtropics. Renew. Bioresources 2, 1–7. doi: 10.7243/2052-6237-2-1
Hemp Business Journal (2018). Farm Bill Effect: Impact on U.S. and Global Hemp Markets. Available online at: https://www.hempbizjournal.com/farm-bill-effect-impacts-on-us-hemp-market-and-global-hemp-market/ (accessed July 10, 2020).
Heslop-Harrison, J., and Heslop-Harrison, Y. (1969). Cannabis sativa L. The induction of flowering. Some case studies. MacMillan Co. Pty. Ltd.
Heslop-Harrison, J., and Heslop-Harrison, Y. (1972). Sexuality of Angiosperms. Physiology of Development: From Seeds to Sexuality. New York, NY: Academic Press.
Höppner, F., and Mange-Hartmann, U. (2007). Yield and quality of fibre and oil of fourteen hemp cultivars in Northern Germany at two harvest dates. Landbauforschung Volkenrode 57, 219–232.
Jung, C., and Müller, A. E. (2009). Flowering time control and applications in plant breeding. Trends in Plant Sci. 14, 563–573. doi: 10.1016/j.tplants.2009.07.005
Keller, A., Leupin, M., Mediavilla, V., and Wintermantel, E. (2001). Influence of the growth stage of industrial hemp on chemical and physical properties of the fibres. Ind. Crops Prod. 13, 35–48. doi: 10.1016/S0926-6690(00)00051-0
Kishida, Y. (1989). Changes in light intensity at twilight and estimation of the biological photoperiod. Jpn. Agr. Res. Qrtly. 22, 247–252.
Lane, H. C., Cathey, M., and Evans, L. T. (1965). The dependence of flowering in several long-day plants on the spectral composition of light extending the photoperiod. Amer. J. Bot. 52, 1006–1014. doi: 10.1002/j.1537-2197.1965.tb07278.x
Lisson, S. N., Mendham, N. J., and Carberry, P. S. (2000). Development of a hemp (Cannabis sativa L.) simulation model 2. The flowering response of two hemp cultivars to photoperiod. Austr. J. Exp. Agr. 40, 413–417. doi: 10.1071/EA99059
Mediavilla, V., Jonquera, M., Schmid-Slembrouck, I., and Soldati, A. (1998). Decimal code for growth stages of hemp (Cannabis sativa L.). J. Intl. Hemp Assn. 5:65.
Mediavilla, V., Leupin, M., and Keller, A. (2001). Influence of the growth stage of industrial hemp on the yield formation in relation to certain fibre quality traits. Ind. Crops Prod. 13, 49–56. doi: 10.1016/S0926-6690(00)00052-2
Moher, M., Jones, M., and Zheng, Y. (2020). Photoperiodic response of in vitro Cannabis sativa plants. Hort Sci. 56, 108–113. doi: 10.21273/HORTSCI15452-20
Navarro, J. A. R., Willcox, M., Burgueño, J., Romay, C., Swarts, K., Trachsel, S., et al. (2017). A study of allelic diversity underlying flowering-time adaptation in maize landraces. Nat. Genet. 49, 476–480. doi: 10.1038/ng.3784
Petit, J., Salentijn, E. M., Paulo, M. J., Thouminot, C., van Dinter, B. J., Magagnini, G., et al. (2020). Genetic variability of morphological, flowering, and biomass quality traits in hemp (Cannabis sativa L.). Front. Plant Sci. 11:102. doi: 10.3389/fpls.2020.00102
Potter, D. J. (2014). “Cannabis horticulture,” in Handbook of Cannabis (Oxford: Oxford University Press). doi: 10.1093/acprof:oso/9780199662685.003.0004
Runkle, E. S., Heins, R. D., Cameron, A. C., and Carlson, W. H. (1998). Flowering of herbaceous perennials under various night interruption and cyclic lighting treatments. HortScience 33, 672–677. doi: 10.21273/HORTSCI.33.4.672
Salentijn, E. M., Petit, J., and Trindade, L. M. (2019). The complex interactions between flowering behavior and fiber quality in hemp. Front. Plant Sci. 10:614. doi: 10.3389/fpls.2019.00614
Salisbury, F. B. (1981). Twilight effect: initiating dark measurement in photoperiodism of Xanthium. Plant Physiol. 67, 1230–1238. doi: 10.1104/pp.67.6.1230
Sawler, J., Stout, J. M., Gardner, K. M., Hudson, D., Vidmar, J., Butler, L., et al. (2015). The genetic structure of marijuana and hemp. PLoS ONE 10:e0133292. doi: 10.1371/journal.pone.0133292
Sengloung, T., Kaveeta, L., and Nanakorn, W. (2009). Effect of sowing date on growth and development of Thai hemp (Cannabis sativa L.). Kasetsart J. 43, 423–431.
Small, E. (2015). Evolution and classification of Cannabis sativa (marijuana, hemp) in relation to human utilization. Bot. Rev. 81, 189–294. doi: 10.1007/s12229-015-9157-3
Spitzer-Rimon, B., Duchin, S., Bernstein, N., and Kamenetsky, R. (2019). Architecture and florogenesis in female Cannabis sativa plants. Front. Plant Sci. 10:350. doi: 10.3389/fpls.2019.00350
Takimoto, A., and Ikeda, K. (1961). Effect of twilight on photoperiodic induction in some short day plants. Plant Cell Physiol. 2, 213–229. doi: 10.1093/oxfordjournals.pcp.a077680
Tang, K., Struik, P. C., Yin, X., Thouminot, C., Bjelkova, M., Stramkale, V., et al. (2016). Comparing hemp (Cannabis sativa L.) cultivars for dual- purpose production under contrasting environments. Ind. Crop. Prod. 87, 33–44. doi: 10.1016/j.indcrop.2016.04.026
Van der Werf, H. M. G., Haasken, H. J., and Wijlhuizen, M. (1994). The effect of daylength on yield and quality of fibre hemp (Cannabis sativa L.). Eur. J. Agron. 3, 117–123. doi: 10.1016/S1161-0301(14)80117-2
Vince-Prue, D., and Canham, A. (1983). “Horticultural significance of photmorphogenesis,” in Photomorphogenesis. Photomorphogenesis, eds W. Shropshire and H. Mohr (Heidelberg: Springer-Verlag), 518–544. doi: 10.1007/978-3-642-68918-5_20
Williams, A. (2020). Hemp Breeding and the Uses of Photoperiod Manipulation. Creative Components 560.
Zhang, M., and Runkle, E. S. (2019). Regulating flowering and extension growth of poinsettia using red and far-red light-emitting diodes for end-of-day lighting. HortScience 54, 323–327. doi: 10.21273/HORTSCI13630-18
Keywords: critical photoperiod, twilight, subtropical, cultivar, extension growth, genetic variation, origin, sex
Citation: Zhang M, Anderson SL, Brym ZT and Pearson BJ (2021) Photoperiodic Flowering Response of Essential Oil, Grain, and Fiber Hemp (Cannabis sativa L.) Cultivars. Front. Plant Sci. 12:694153. doi: 10.3389/fpls.2021.694153
Received: 12 April 2021; Accepted: 28 June 2021;
Published: 02 August 2021.
Edited by:
Derek Stewart, The James Hutton Institute, United KingdomReviewed by:
Gerhard Buck-Sorlin, Agrocampus Ouest, FranceCopyright © 2021 Zhang, Anderson, Brym and Pearson. This is an open-access article distributed under the terms of the Creative Commons Attribution License (CC BY). The use, distribution or reproduction in other forums is permitted, provided the original author(s) and the copyright owner(s) are credited and that the original publication in this journal is cited, in accordance with accepted academic practice. No use, distribution or reproduction is permitted which does not comply with these terms.
*Correspondence: Brian J. Pearson, YnBlYXJzb25AdWZsLmVkdQ==
Disclaimer: All claims expressed in this article are solely those of the authors and do not necessarily represent those of their affiliated organizations, or those of the publisher, the editors and the reviewers. Any product that may be evaluated in this article or claim that may be made by its manufacturer is not guaranteed or endorsed by the publisher.
Research integrity at Frontiers
Learn more about the work of our research integrity team to safeguard the quality of each article we publish.