- 1Crop Genomics and Bioinformatics Center, Nanjing Agricultural University, Nanjing, China
- 2National Key Laboratory of Crop Genetics and Germplasm Enhancement, Nanjing Agricultural University, Nanjing, China
- 3Huaiyin Institute of Agriculture Sciences of Xuhuai Region in Jiangsu, Huaian, China
Wheat production is increasingly threatened by the fungal disease, Fusarium head blight (FHB), caused by Fusarium spp. The introduction of resistant varieties is considered to be an effective measure for containment of this disease. Mapping of FHB-resistance quantitative trait locus (QTL) has promoted marker-assisted breeding for FHB resistance, which has been difficult through traditional breeding due to paucity of resistance genes and quantitative nature of the resistance. The lab of Ma previously cloned Fhb1, which inhibits FHB spread within spikes, and fine mapped Fhb4 and Fhb5, which condition resistance to initial infection of Fusarium spp., from FHB-resistant indigenous line Wangshuibai (WSB). In this study, these three QTLs were simultaneously introduced into five modern Chinese wheat cultivars or lines with different ecological adaptations through marker-assisted backcross in early generations. A total of 14 introgression lines were obtained. All these lines showed significantly improved resistance to the fungal infection and disease spread in 2-year field trials after artificial inoculation. In comparison with the respective recipient lines, the Fhb1, Fhb4, and Fhb5 pyramiding could reduce the disease severity by 95% and did not systematically affect plant height, productive tiller number, kernel number per spike, thousand grain weight, flowering time, and unit yield (without Fusarium inoculation). These results indicated the great value of FHB-resistance QTLs Fhb1, Fhb4, and Fhb5 derived from WSB, and the feasibility and effectiveness of early generation selection for FHB resistance solely based on linked molecular markers.
Introduction
Fusarium head blight (FHB) or scab is a global fungal disease in wheat caused by Fusarium spp., particularly Fusarium graminearum Schwabe (teleomorph: Gibberella zeae) (Ma et al., 2020). Apart from reducing yield and deteriorating grain quality, the pathogen produces mycotoxins, such as deoxynivalenol (DON), in kernels that are harmful to human and livestock health (Gilbert and Tekauz, 2000). In China, wheat FHB epidemics frequently occur in the middle and lower reaches of the Yangtze River and the south of the Huang-Huai area, where the flowering stage of wheat often meets with a warm and humid environment. However, due to global warming and changes in cultivation practices in recent years, FHB occurrence has become more and more frequent in the north and west of China. Of the measures that could be taken to control FHB (Chen et al., 2019; Ma et al., 2020), deployment of FHB-resistant cultivars is fundamental and favored by farmers for its environmental friendliness and cost-effectiveness.
Fusarium head blight resistance is a quantitative trait controlled by polygenes and greatly affected by the environment. Making matters more complicated is that it could take different forms, for instance, type I resistance (against initial infection), type II resistance (against fungal spread within the spike), type III resistance (low toxin accumulation in kernels), type IV resistance (lower kernel infection rate), and type V resistance (host tolerance) (Schroeder and Christensen, 1963; Miller et al., 1985; Mesterházy, 1995). These factors pose great difficulties on phenotype evaluation because of the requirement for suitable facilities, different inoculation methods and assessments, repeated trials, and considerable labor and time investment, and thus limit the efficiency of FHB resistance improvement through conventional breeding. The advent of marker-assisted selection (MAS) provides a very promising option to overcome these problems (Dudley, 1993; Lee, 1995; Miedaner et al., 2006; Buerstmayr et al., 2009; Xue et al., 2010a; Nayak et al., 2017; Jia et al., 2018; Ma et al., 2020). Until now, more than 432 quantitative trait loci (QTLs) for FHB resistance have been mapped in wheat (Ma et al., 2020), of which many for type I and type II resistances overlap with QTLs for other types of resistance, indicating the principal roles of type I and type II resistances in controlling FHB. Some of these QTLs have been applied to MAS-based FHB resistance improvement with success (Miedaner et al., 2006; Buerstmayr et al., 2009; Xue et al., 2010a; Salameh et al., 2011; Bernardo et al., 2013; Zhang et al., 2016; Jia et al., 2018; Brar et al., 2019a; Li et al., 2019a); however, most of them still require verification due to small effects and large confidence intervals.
No accessions or lines showing immunity to FHB have been found among wheat germplasm. In wheat breeding programs worldwide, FHB-resistant Sumai 3, a wheat cultivar developed from the cross of Funo with Taiwanxiaomai by Suzhou Institute of Agricultural Sciences, China, and its derivatives are the main sources of FHB resistance (Ban and Suenaga, 2000; Buerstmayr et al., 2003; Frohberg et al., 2006; Marza et al., 2006; Badea et al., 2008; Anderson et al., 2012, 2019; Bernardo et al., 2013; Li et al., 2019c). The utilization of Sumai 3-derived resistance genes has only been partially successful so far because of the difficulty in simultaneous improvement of the resistance and agronomic traits. Moreover, the use of a single resistant source could potentially diminish genetic diversity. Wangshuibai (WSB), an indigenous wheat accession in Jiangsu, China, is highly resistant to FHB and carries QTL for different types of FHB resistance (Lin et al., 2004, 2006; Zhou et al., 2004; Mardi et al., 2005; Yu et al., 2007). Using a recombinant inbred line population, WSB was found to possess type I resistance QTL on chromosomes 3A, 4B (Fhb4), and 5A (Fhb5), type II resistance QTL on chromosomes 2A, 3B (Fhb1), and 6B (Fhb2), and type IV resistance QTL on chromosomes 2A, 3B, 4B, and 7D (Lin et al., 2004, 2006; Li et al., 2008; Ma et al., 2008). To speed up utilization of the WSB QTL, Fhb1 has been cloned (Li et al., 2019b), and Fhb2, Fhb4, and Fhb5 have been mapped to small intervals (Xue et al., 2010b, 2011; Jia et al., 2018).
Evaluation of the QTL effects in different genetic backgrounds is of great importance for marker-assisted breeding. WSB Fhb1, Fhb4, and Fhb5 have been individually validated using near-isogenic lines developed with Mianyang 99–323 as the recurrent parent (Xue et al., 2010a). This study investigated the effects of Fhb1, Fhb4, and Fhb5 pyramiding in five modern Chinese wheat cultivars or lines on FHB resistance and a few major agronomic traits.
Materials and Methods
Plant Materials
NMAS022 is a near-isogenic line carrying Fhb1, Fhb2, Fhb4, and Fhb5, developed through marker-assisted backcross with WSB as the donor parent and FHB-susceptible common wheat line, PH691, as the recurrent parent and is similar to WSB in FHB resistance and PH691 in other traits. The recipients included semi-winter white wheat lines, Bainong418, Bainong4199, Zhoumai27, and 4446, and a semi-winter red wheat cultivar, Chuanmai64. Bainong418 and Bainong4199 were developed by Henan Institute of Science and Technology; Zhoumai27 and Chuanmai64 were released by Zhoukou Academy of Agricultural Sciences and Crop Research Institute of the Sichuan Academy of Agricultural Sciences, respectively.
Genotyping
Total genomic DNA was extracted from young leaves according to Ma and Sorrells (1995). PCR was performed in Applied BiosystemsTM ProFlexTM 96-Well PCR System (ThermoFisher Scientific, MA, USA) following the procedure of Ma et al. (1996). Each 12.5 μl of PCR reaction consisted of 10–30 ng of DNA template, 1 × PCR buffer, 2.5 nmol dNTP, 2 pmol of each primer, 18.75 nmol MgCl2, and 0.4 U Taq DNA polymerase.
Marker WGRB619, designed according to the Fhb1 sequence, was used in Fhb1 detection (Li et al., 2019b). GWM149 and GWM513 were used in Fhb4 detection (Xue et al., 2010b). Three markers, including WMC752, BARC180, and MAG9482 (5′-CATGATTGATTCGATGACTATAATATCTT-3′, 5′TCTTTCTCCCGTTGCAATGT-3′), were used for Fhb5 identification. Xmag9482 and Xwmc752 are distal and proximal to Fhb5 (unpublished data). Xbarc180 is also proximal to, but further from, Fhb5 (Xue et al., 2011). The PCR profile was as follows: 94°C for 5 min, followed by 36 cycles of 94°C for 30 s, 52–60°C for 30 s (WGRB619, GWM513, and WMC752 at 60°C; GWM149 at 54°C; MAG9482 and BARC180 at 52°C), and 72°C for 40 s or 2 min (WGRB619), then 72°C for 5 min. WGRB619 PCR products were separated into 1% agarose gels and visualized by ethidium bromide staining. The other PCR products were separated in 8% non-denaturing polyacrylamide gels with acrylamide and bis-acrylamide in 29:1 and visualized by silver staining (Bassam et al., 1991).
Field Trials
Field trials were conducted in the wheat-growing seasons at Huaiyin Institute of Agricultural Sciences, Huai'an, China, from 2018–2020, using the randomized complete block design with commonly undertaken cultivation practices in wheat production. Two trials, one for type I resistance evaluation and one for type II resistance evaluation, were set up in 2018–2019. Each of the trials consisted of two blocks in which each plot had two 1.5-m rows spaced by 0.25 m. About 25 seeds were planted per row. In 2019–2020, three trials were set up. One trial for type I resistance evaluation and one trial for type II resistance evaluation had two blocks, and one trial for agronomic trait evaluation had three blocks. In the blocks, each plot had 60 seeds planted in a 3-m row and the row spacing was 0.5 m.
FHB Resistance Evaluation
Type I resistance was evaluated by spraying at anthesis, the mixed conidial suspension (one spore per microliter) of four local virulent strains of F. graminearum. About 14 days after the inoculation, 82–100 spikes were selected randomly in each plot and the number of spikes with visible FHB symptoms in at least one of their florets was scored. Percentage of infected spikes (PIS) was used to represent the type I resistance.
Type II resistance was evaluated by single floret inoculation at anthesis. About 10 μl mixed conidial suspension of F. graminearum containing 1,000 spores was injected into a flowering floret near the middle of a spike. Twenty spikes were inoculated in each plot, and 10 spikes with the most serious symptom were investigated for the number of diseased spikelets (NDS) and the length of diseased rachides (LDR) at about 18 days after the inoculation to represent the type II resistance.
Agronomic Trait Evaluation
Anthesis was the time from sowing to more than half of the plants flowering in the plot. Plant height, number of kernels per spike, and number of productive tillers of five plants randomly chosen from the middle of each plot were investigated at physiological maturity and the plot means were used in the analysis. The plant height was the total length of the aboveground part excluding the awn. The number of kernels per spike was counted from the main spikes. The plants located in the middle 1 m of a plot and without inoculations were harvested at maturity for yield and thousand kernel weight (TKW) measurements. TKW was measured after oven-drying.
Statistical Analysis
A one-way ANOVA was carried out using SPSS Statistics version 25 (IBM, USA). The Tukey test was used in multiple comparisons.
Results
Parental Examination With Foreground-Selection Markers
To obtain markers suitable for selection of Fhb1, Fhb4, or Fhb5 and to find out whether the recipients carry these three QTLs, the five recipient lines for the QTL introgression were examined with markers WGRB619 for Fhb1, GWM149 and GWM513 flanking Fhb4, and MAG9482 and BARC180 flanking Fhb5. None of these lines possess the expected marker alleles (Figure 1), indicating that the five recipients do not carry Fhb1, Fhb4, or Fhb5. WMC752 detected polymorphism between NMAS022 and among all the recipients but Chuanmai 64 (Figure 1F). It was, therefore, used in the detection of Fhb5 in introgression into these four cultivars, since Xwmc752 was located on the same side of Fhb5 as Xbarc180 and closer to the QTL peak.
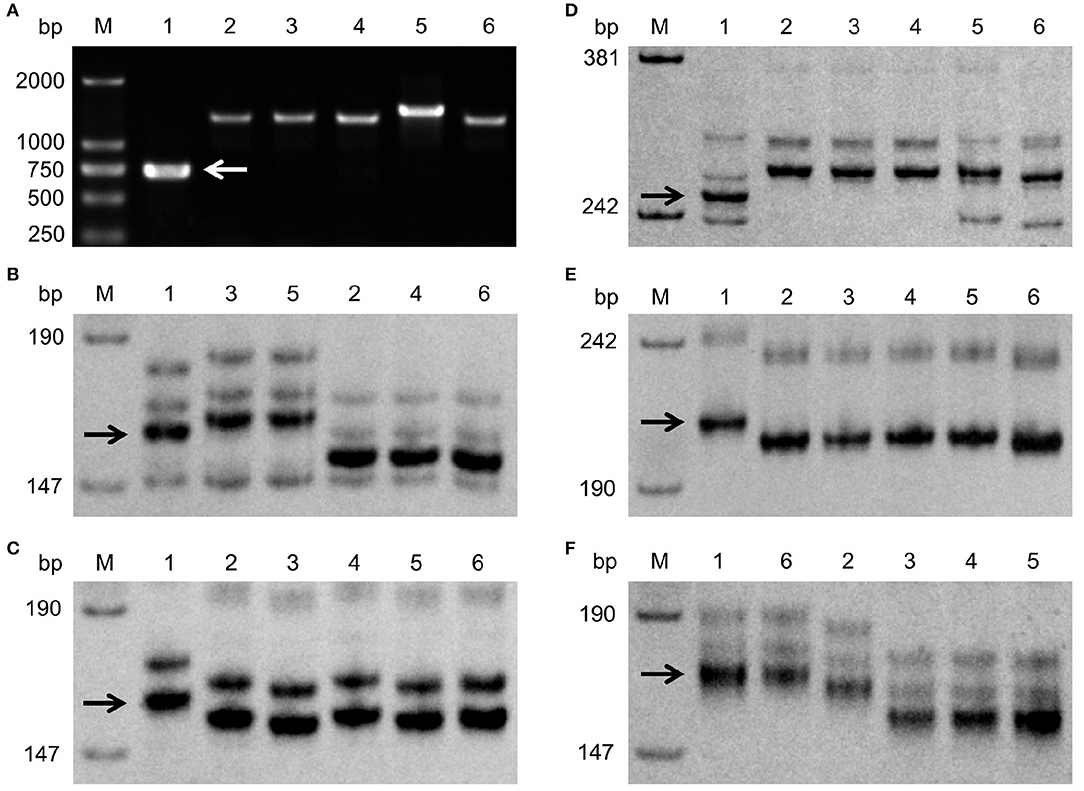
Figure 1. Detection of Fhb1 by WGRB619 (A), Fhb4 by GWM149 (B) and GWM513 (C), and Fhb5 by MAG9482 (D), BARC180 (E), and WMC752 (F). The target bands were indicated by arrows. M: the DNA size standard (in bp). 1, 2, 3, 4, 5, and 6: NMAS022, Bainong418, Bainong4199, Zhoumai27, 4446, and Chuanmai 64, respectively.
Fhb1, Fhb4, and Fhb5 Pyramiding
Fhb1, Fhb4, and Fhb5 were introduced from NMAS022 to Bainong418, Bainong4199, Zhoumai27, 4446, and Chuanmai 64 through three generations of marker-assisted backcross using the recipient lines as recurrent parents (Figure 2). To identify plants carrying the target QTL, an average of 37 plants were examined per generation per cross with the foreground-selection markers (Table 1). In each backcross generation, plants carrying all three target QTLs accounted for 9.4~16.1%, which was in accordance with the expected 1:7 ratio ( = 0~0.37 < = 3.841). To obtain plants homozygous at Fhb1, Fhb4, and Fhb5, 109–140 BC3F2 plants from each combination were surveyed with the foreground markers (Table 1). Usually, the plants more similar to the respective recipient parents were chosen for backcrossing or self-seed harvest.
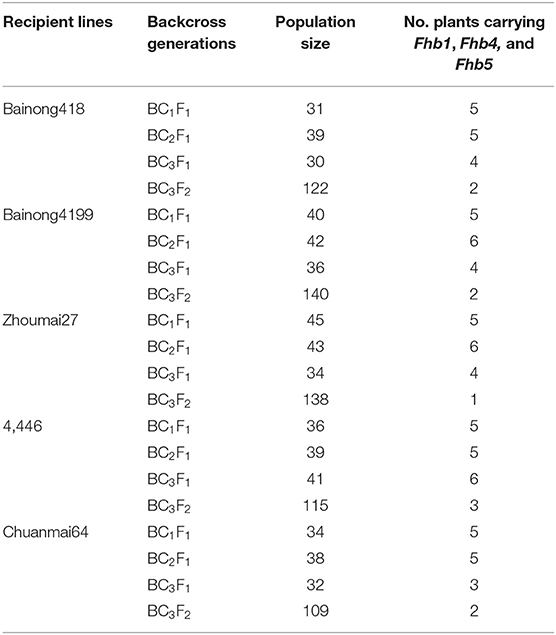
Table 1. Population size and the number of plants carrying Wangshuibai (WSB) Fhb1, Fhb4, and Fhb5 in the backcross and F2 generations derived from each recipient line.
FHB Resistance of Fhb1, Fhb4, and Fhb5 Introgression Lines
The five introgression lines obtained from the BC3F2 survey and their parents were subjected to type I and type II resistance evaluations in the 2018–2019 season. For both resistance types, all the introgression lines performed significantly better than their recipient parents (Table 2, Figure 3). About 14 days after the spraying inoculation, PIS of the introgression lines was <17%, while that of the recipient parents was higher than 48%. About 18 days after single floret inoculation, the introgression lines had only one diseased spikelet and about 1 cm of LDR, much lower than the recipient parents, which had, on average, 6.1 diseased spikelets and 4.2 cm LDR.
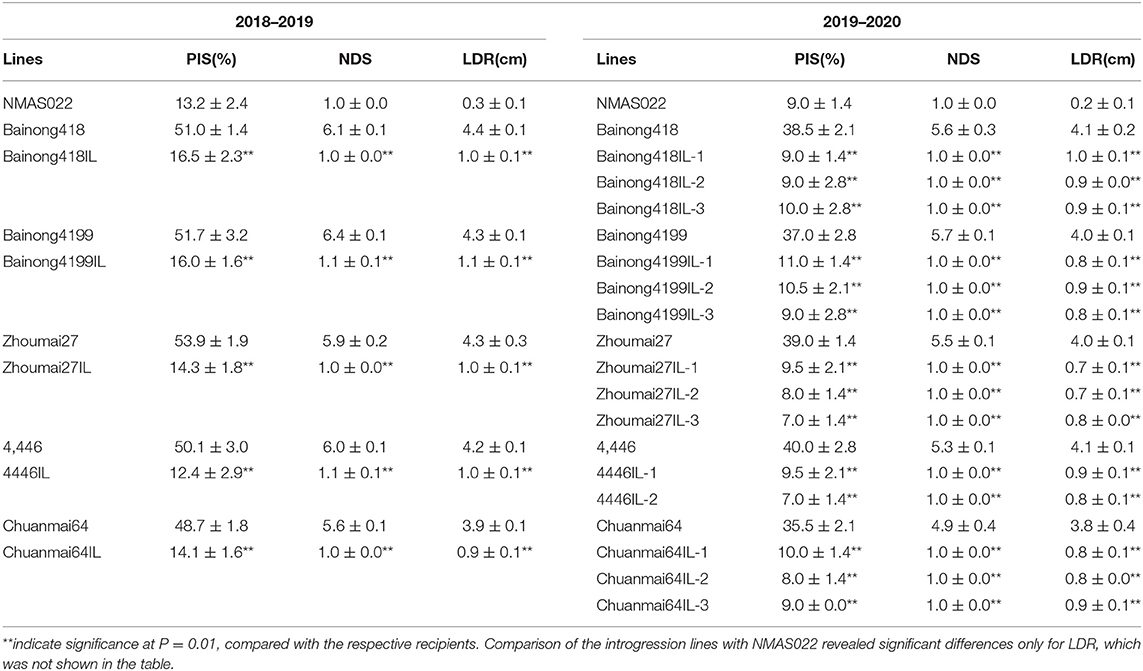
Table 2. Percentage of infected spikes (PIS), number of diseased spikelets (NDS), and length of diseased rachides (LDR) (represented as mean ± SD) of the WSB Fhb1, Fhb4, and Fhb5 introgression lines compared with donor parent NMAS022 and the recipient lines.
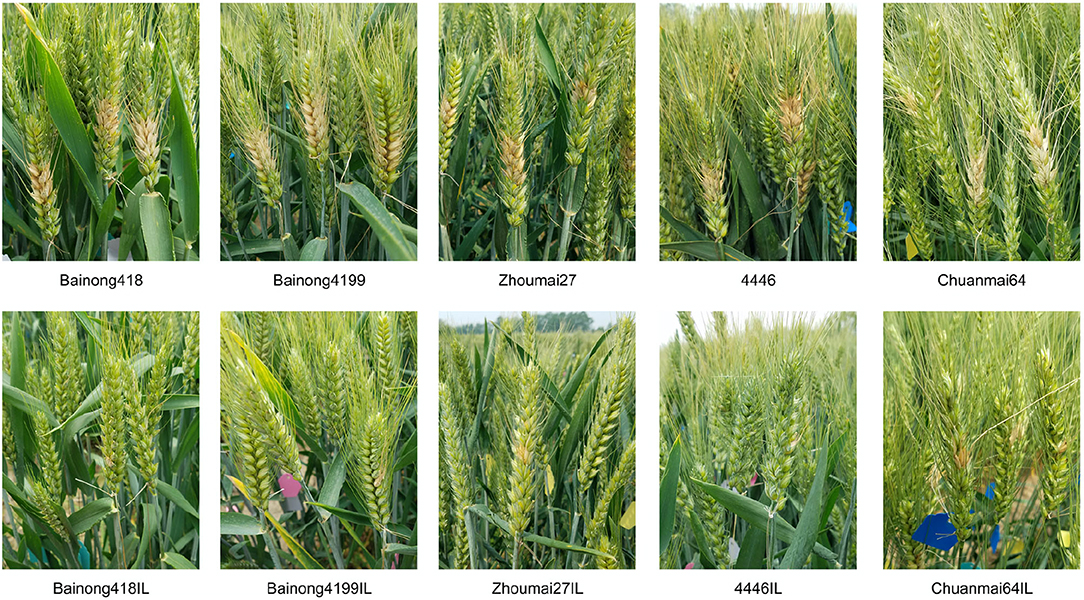
Figure 3. Fusarium head blight (FHB) symptom illustration of the recipients (top) and Fhb1, Fhb4, and Fhb5 introgression lines (bottom). Photos were taken 18 days after single floret inoculation.
It was noted in the field that the overall morphology of these introgression lines was similar to their recipient parents, but variations in some traits, such as plant height and spike shape, still existed. Thus, two to three plants were selected from each line with these variations in mind for further evaluation of both FHB resistance and agronomic traits in the 2019–2020 season. It was shown that all 14 selected lines still had significantly less NDS and LDR than the recipient parents (Table 2), indicating the stability of the resistance conferred by the three QTLs. All the introgression lines were similar to NMAS022 in terms of PIS and NDS but had longer diseased rachides (Table 2). These results indicated that the introgression of Fhb1, Fhb4, and Fhb5 led to a type I resistance level comparative to the QTL donor parent and a significantly improved type II resistance.
Agronomic Performance of the Fhb1, Fhb4, and Fhb5 Introgression Lines
To determine the effects of Fhb1, Fhb4, and Fhb5 pyramiding on agronomic performance, six traits, namely, anthesis, plant height, number of kernels per spike, number of productive tillers, TKW, and 0.5-m2 yield, were investigated. NMAS022 was different from all the recipient lines in most of the investigated traits (Table 3). In comparison with the respective recipient parents, the introgression lines were similar in anthesis, and the introgression line 4446IL-1 was the only one showing significant variation in the number of productive tillers. As to the remaining four traits, some introgression lines were similar to the recipient parents, some showed positive changes, and some varied negatively (Table 3). It was, therefore, concluded that it was not the Fhb1, Fhb4, and Fhb5 pyramiding but the variations of genetic composition that conditioned the agronomic trait variations. Interestingly, the 0.5-m2 yield of all the introgression lines was similar to or even significantly higher than the respective recipient parents.
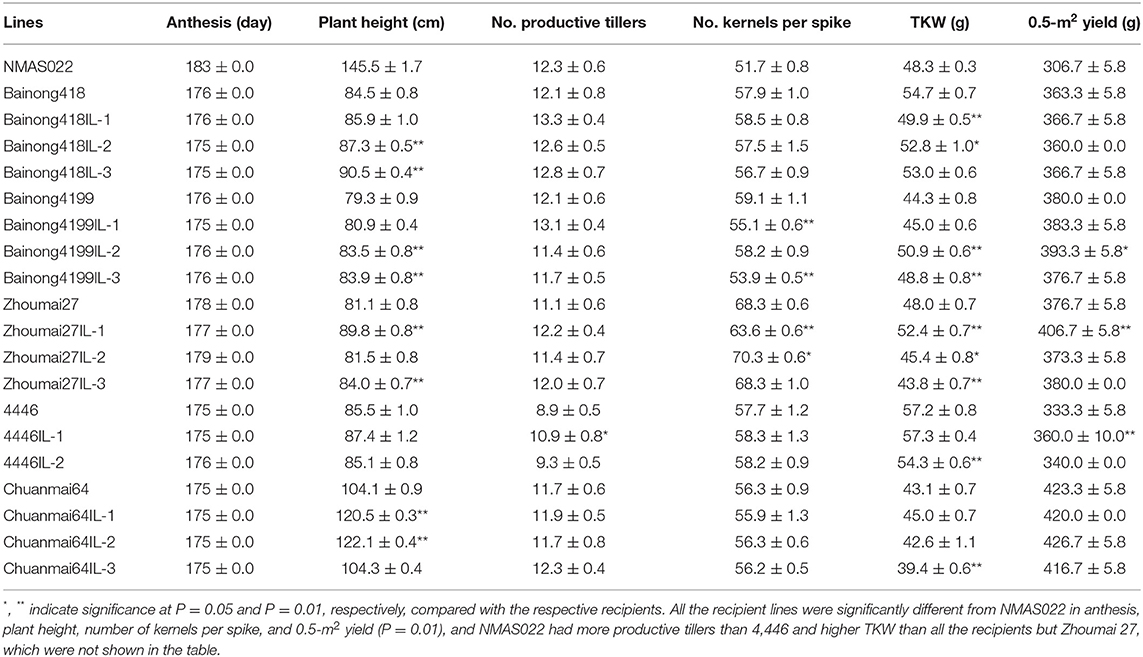
Table 3. Agronomic traits (represented as mean ± SD) of the WSB Fhb1, Fhb4, and Fhb5 introgression lines and the parents.
Discussion
In this study, the FHB resistance QTLs, Fhb1, Fhb4, and Fhb5, from WSB were simultaneously introduced into five modern wheat cultivars or lines adapting to different wheat-growing areas using a marker-assisted backcross strategy. A 2-year FHB resistance evaluation indicated that Fhb1, Fhb4, and Fhb5 pyramiding significantly improved both type I and type II resistances in all backgrounds without exception. Due to the significant correlation of Fhb1, Fhb4, and Fhb5 intervals with mycotoxin DON accumulation (Somers et al., 2003; Jiang et al., 2007a,b; Bonin and Kolb, 2009; Jayatilake et al., 2011; Szabó-Hevér et al., 2014), the developed introgression lines are expected to reduce the kernel DON level too.
Fhb1 improves only type II resistance, and Fhb4 and Fhb5 enhance only type I resistance (Lin et al., 2004, 2006; Ma et al., 2008; Xue et al., 2010a). Compared with the introduction of a single QTL, pyramiding of QTLs for different types of FHB resistance is more effective against the disease, as illustrated in FHB resistance improvement of AK58 by Xu et al. (2017), and should be promoted in breeding programs due to the lack of genes conferring immunity to FHB. It was noted that the introgression lines had longer diseased rachides after point inoculation than NMAS022 that carries Fhb2 as well as Fhb1, Fhb4, and Fhb5, implying that the introgression of Fhb2 could further improve the FHB resistance.
The total disease index was not investigated in this study because of the limitation of the experiments; however, the obtained results were still telling since the local pathogen pressure imposed by artificial inoculation in the resistance evaluation was far greater than that imposed by natural inoculation. Based on the NDS obtained after single floret inoculation and the PIS obtained after spraying inoculation, the Fhb1, Fhb4, and Fhb5 pyramiding raised the FHB resistance level by 95% and made the introgression lines highly resistant to FHB. The QTL pyramiding effects are, however, still in dispute, as shown by Brar et al. (2019a), who introduced Fhb1, Fhb2, and Fhb5 from Sumai 3 into two hard red spring wheat cultivars from Canada, and by Salameh et al. (2011), who made a similar attempt in European winter wheat. We reasoned that the discrepancy could be due to the small effect of Fhb2 (unpublished data), different trial conditions and resistance evaluation methods, and the genetic backgrounds.
Wheat breeders often find it difficult to obtain plants with satisfied agronomic performance and a high level of FHB resistance in conventional breeding using Sumai 3 as a parent, which prompts deliberation on whether the FHB resistance genes have deleterious effects on agronomic traits. Indeed, the Fhb4 interval showed association with plant height (Jia et al., 2013), and the Fhb5 interval was related to plant height and grain weight (Huang et al., 2004, 2015; Jia et al., 2013; Steiner et al., 2019). In a few studies, the introduction of Fhb4 interval resulted in plant height increase (McCartney et al., 2007; Xue et al., 2010a), and the introduction of Fhb5 interval led to lower TKW and a slight increase in plant height (Brar et al., 2019b). We demonstrated, using multiple parental combinations, that these associations can be broken through selection, particularly with the help of suitable markers. In terms of yield performance, the Fhb1, Fhb4, and Fhb5 introgression lines were as good as the recipient parents. The yield of introgression line 4446IL-1 even increased up to 8%. These results suggested that the Fhb1, Fhb4, and Fhb5 pyramiding was not in conflict with agronomic trait improvement.
Marker-assisted selection has displayed the potential in improving FHB resistance breeding efficiency. In addition to breaking up unfavorable linkage drags, MAS can also speed up the breeding process (Xue et al., 2010a; Brar et al., 2019a). Taking Fhb1, Fhb4, and Fhb5, which are located on different chromosomes, as an example, the plants carrying all three QTLs theoretically account for one-eighth in each backcross. Therefore, the probability of obtaining such a plant is more than 98% when more than 30 BCF1 plants are surveyed. Enlarging the backcross population size manageably, together with marker-assisted background selection, could greatly accelerate the QTL introgression (Xue et al., 2010a; Huang et al., 2015). This study showed again the usefulness and effectiveness of the Fhb1 functional marker and the closely-linked Fhb4 and Fhb5 flanking markers.
The recipient parents used in this study were all newly bred cultivars or lines and represented different ecological types. The obtained introgression lines could not only be used as breeding parents but also have the potential to be directly deployed in production.
Data Availability Statement
The original contributions presented in the study are included in the article/supplementary material, further inquiries can be directed to the corresponding author/s.
Author Contributions
YZ conducted experiments, data analysis, and prepared the draft. HM, LH, FD, and CR participated in genotyping and material development. ZY, YD, and ZG contributed to phenotyping. HJ, GL, and ZK contributed to project implementation. ZM designed the project and reviewed the article. All the authors have read and approved the final manuscript.
Funding
This study was partially supported by grants from the National Natural Science Foundation of China (31930081 and 30430440), the Ministry of Science and Technology of China (2016YFD0101004 and 2016YFD0101802), and Jiangsu collaborative innovation initiative for modern crop production.
Conflict of Interest
The authors declare that the research was conducted in the absence of any commercial or financial relationships that could be construed as a potential conflict of interest.
Acknowledgments
The authors are grateful to Professors Wuyun Yang of Sichuan Academy of Agricultural Sciences, Guihong Ying of Henan Agricultural University, Zhenggang Ru of Henan Institute of Science and Technology, and Yinhuai Zhao for providing the recipient lines. The authors thank all the staff and students of Crop Genomics and Bioinformatics Center and the Wheat Breeding Group of Huaiyin Institute of Agriculture Sciences for their help in the project implementation.
References
Anderson, J. A., Wiersma, J. J., Linkert, G. L., Kolmer, J. A., Jin, Y., Dill-Macky, R., et al. (2012). Registration of ‘Sabin' wheat. J. Plant Regist. 6, 174–179. doi: 10.3198/jpr2011.06.0344crc
Anderson, J. A., Wiersma, J. J., Reynolds, S. K., Caspers, R., Linkert, G. L., Kolmer, J. A., et al. (2019). Registration of ‘Shelly' hard red spring wheat. J. Plant Regist.13, 199–206. doi: 10.3198/jpr2018.07.0049crc
Badea, A., Eudes, F., Graf, R. J., Laroche, A., Gaudet, D. A., and Sadasivaiah, R. S. (2008). Phenotypic and marker-assisted evaluation of spring and winter wheat germplasm for resistance to Fusarium head blight. Euphytica 164, 803–819. doi: 10.1007/s10681-008-9735-0
Ban, T., and Suenaga, K. (2000). Genetic analysis of resistance to Fusarium head blight caused by Fusarium graminearum in Chinese wheat cultivar Sumai 3 and the Japanese cultivar Saikai 165. Euphytica 113, 87–99. doi: 10.1023/a:1003951509797
Bassam, B. J., Caetano-Anollés, G., and Gresshoff, P. M. (1991). Fast and sensitive silver staining of DNA in polyacrylamide gels. Anal. Biochem. 196, 80–83. doi: 10.1016/0003-2697(91)90120-i
Bernardo, A., Bai, G., Yu, J., Kolb, F., Bockus, W., and Dong, Y. (2013). Registration of near-isogenic winter wheat germplasm contrasting in Fhb1 for Fusarium head blight resistance. J. Plant Regist. 8, 106–108. doi: 10.3198/jpr2013.05.0021crgs
Bonin, C. M., and Kolb, F. L. (2009). Resistance to Fusarium head blight and kernel damage in a winter wheat recombinant inbred line population. Crop Sci. 49, 1304–1312. doi: 10.2135/cropsci2008.08.0459
Brar, G. S., Brûlé-Babel, A. L., Ruan, Y., Henriquez, M. A., Pozniak, C. J., Kutcher, H. R., et al. (2019a). Genetic factors affecting Fusarium head blight resistance improvement from introgression of exotic Sumai 3 alleles: (including Fhb1, Fhb2, and Fhb5) in hard red spring wheat. BMC Plant Biol. 19:179. doi: 10.1186/s12870-019-1782-2
Brar, G. S., Pozniak, C. J., Kutcher, H. R., and Hucl, P. J. (2019b). Evaluation of Fusarium head blight resistance genes Fhb1, Fhb2, and Fhb5 introgressed into elite Canadian hard red spring wheats: effect on agronomic and end-use quality traits and implications for breeding. Mol. Breed. 39:44. doi: 10.1007/s11032-019-0957-8
Buerstmayr, H., Ban, T., and Anderson, J. A. (2009). QTL mapping and marker-assisted selection for Fusarium head blight resistance in wheat: a review. Plant Breed. 128, 1–26. doi: 10.1111/j.1439-0523.2008.01550.x
Buerstmayr, H., Steiner, B., Hartl, L., Griesser, M., Angerer, N., Lengauer, D., et al. (2003). Molecular mapping of QTLs for Fusarium head blight resistance in spring wheat. II. Resistance to fungal penetration and spread. Theor. Appl. Genet. 107, 503–508. doi: 10.1007/s00122-003-1272-6
Chen, Y., Kistler, H. C., and Ma, Z. (2019). Fusarium graminearum trichothecene mycotoxins: biosynthesis, regulation, and management. Annu. Rev. Phytopathol. 57, 15–39. doi: 10.1146/annurev-phyto-082718-100318
Dudley, J. W. (1993). Molecular markers in plant improvement: manipulation of genes affecting quantitative traits. Crop Sci. 33, 660–668. doi: 10.2135/cropsci1993.0011183X003300040003x
Frohberg, R. C., Stack, R. W., Olson, T., Miller, J. D., and Mergoum, M. (2006). Registration of ‘Alsen' wheat. Crop Sci. 46, 2311–2312. doi: 10.2135/cropsci2005.12.0501
Gilbert, J., and Tekauz, A. (2000). Review: recent developments in research on Fusarium head blight of wheat in Canada. Can. J. Plant Pathol. 22, 1–8. doi: 10.1080/07060660009501155
Huang, X. Q., Kempf, H., Ganal, M. W., and Röder, M. S. (2004). Advanced backcross QTL analysis in progenies derived from a cross between a German elite winter wheat variety and a synthetic wheat: (Triticum aestivum L.). Theor. Appl. Genet. 109, 933–943. doi: 10.1007/s00122-004-1708-7
Huang, Y., Kong, Z., Wu, X., Cheng, R., Yu, D., and Ma, Z. (2015). Characterization of three wheat grain weight QTLs that differentially affect kernel dimensions. Theor. Appl. Genet. 128, 2437–2445. doi: 10.1007/s00122-015-2598-6
Jayatilake, D. V., Bai, G. H., and Dong, Y. H. (2011). A novel quantitative trait locus for Fusarium head blight resistance in chromosome 7A of wheat. Theor. Appl. Genet. 122, 1189–1198. doi: 10.1007/s00122-010-1523-2
Jia, H., Wan, H., Yang, S., Zhang, Z., Kong, Z., Xue, S., et al. (2013). Genetic dissection of yield-related traits in a recombinant inbred line population created using a key breeding parent in China's wheat breeding. Theor. Appl. Genet. 126, 2123–2139. doi: 10.1007/s00122-013-2123-8
Jia, H., Zhou, J., Xue, S., Li, G., Yan, H., Ran, C., et al. (2018). A journey to understand wheat Fusarium head blight resistance in the Chinese wheat landrace Wangshuibai. Crop J. 6, 48–59. doi: 10.1016/j.cj.2017.09.006
Jiang, G., Dong, Y., Shi, J., and Ward, R. W. (2007a). QTL analysis of resistance to Fusarium head blight in the novel wheat germplasm CJ9306. II. Resistance to deoxynivalenol accumulation and grain yield loss. Theor. Appl. Genet. 115, 1043–1052. doi: 10.1007/s00122-007-0630-1
Jiang, G., Shi, J., and Ward, R. W. (2007b). QTL analysis of resistance to Fusarium head blight in the novel wheat germplasm CJ 9306. I. Resistance to fungal spread. Theor. Appl. Genet. 116, 3–13. doi: 10.1007/s00122-007-0641-y
Lee, M. (1995). DNA markers and plant breeding programs. Adv. Agron. 55, 265–344. doi: 10.1016/S0065-2113(08)60542-8
Li, C., Zhu, H., Zhang, C., Lin, F., Xue, S., Cao, Y., et al. (2008). Mapping QTLs associated with Fusarium-damaged kernels in the Nanda 2419 × Wangshuibai population. Euphytica 163, 185–191. doi: 10.1007/s10681-007-9626-9
Li, G., Jia, L., Zhou, J., Fan, J., Yan, H., Shi, J., et al. (2019a). Evaluation and precise mapping of Qfhb.nau-2B conferring resistance against Fusarium infection and spread within spikes in wheat: (Triticum aestivum L.). Mol. Breed. 39:62. doi: 10.1007/s11032-019-0969-4
Li, G., Zhou, J., Jia, H., Gao, Z., Fan, M., Luo, Y., et al. (2019b). Mutation of a histidine-rich calcium-binding-protein gene in wheat confers resistance to Fusarium head blight. Nat. Genet. 51, 1106–1112. doi: 10.1038/s41588-019-0426-7
Li, T., Zhang, H., Huang, Y., Su, Z., Deng, Y., Liu, H., et al. (2019c). Effects of the Fhb1 gene on Fusarium head blight resistance and agronomic traits of winter wheat. Crop. J. 7, 799–808. doi: 10.1016/j.cj.2019.03.005
Lin, F., Kong, Z. X., Zhu, H. L., Xue, S. L., Wu, J. Z., Tian, D. G., et al. (2004). Mapping QTL associated with resistance to Fusarium head blight in the Nanda2419 × Wangshuibai population. I. Type II resistance. Theor. Appl. Genet. 109, 1504–1511. doi: 10.1007/s00122-004-1772-z
Lin, F., Xue, S. L., Zhang, Z. Z., Zhang, C. Q., Kong, Z. X., Yao, G. Q., et al. (2006). Mapping QTL associated with resistance to Fusarium head blight in the Nanda2419 × Wangshuibai population. II: type I resistance. Theor. Appl. Genet. 112, 528–535. doi: 10.1007/s00122-005-0156-3
Ma, Z., Xie, Q., Li, G., Jia, H., Zhou, J., Kong, Z., et al. (2020). Germplasms, genetics and genomics for better control of disastrous wheat Fusarium head blight. Theor. Appl. Genet. 133, 1541–1568. doi: 10.1007/s00122-019-03525-8
Ma, Z., Xue, S., Lin, F., Yang, S., Li, G., Tang, M., et al. (2008). Mapping and validation of scab resistance QTLs in the Nanda2419 × Wangshuibai population. Cereal Res. Commun. 36, 245–251. doi: 10.1556/crc.36.2008.suppl.b.22
Ma, Z. Q., Röder, M., and Sorrells, M. E. (1996). Frequencies and sequence characteristics of di-, tri-, and tetra-nucleotide microsatellites in wheat. Genome 39, 123–130. doi: 10.1139/g96-017
Ma, Z. Q., and Sorrells, M. E. (1995). Genetic analysis of fertility restoration in wheat using restriction fragment length polymorphisms. Crop Sci. 35:1137. doi: 10.2135/cropsci1995.0011183X003500040037x
Mardi, M., Buerstmayr, H., Ghareyazie, B., Lemmens, M., Mohammadi, S. A., Nolz, R., et al. (2005). QTL analysis of resistance to Fusarium head blight in wheat using a ‘Wangshuibai'-derived population. Plant Breed. 124, 329–333. doi: 10.1111/j.1439-0523.2005.01103.x
Marza, F., Bai, G. H., Carver, B. F., and Zhou, W. C. (2006). Quantitative trait loci for yield and related traits in the wheat population Ning7840 × Clark. Theor. Appl. Genet. 112, 688–698. doi: 10.1007/s00122-005-0172-3
McCartney, C. A., Somers, D. J., Fedak, G., DePauw, R. M., Thomas, J., Fox, S. L., et al. (2007). The evaluation of FHB resistance QTLs introgressed into elite Canadian spring wheat germplasm. Mol. Breed. 20, 209–221. doi: 10.1007/s11032-007-9084-z
Mesterházy, A. (1995). Types and components of resistance to Fusarium head blight of wheat. Plant Breed. 114, 377–386. doi: 10.1111/j.1439-0523.1995.tb00816.x
Miedaner, T., Wilde, F., Steiner, B., Buerstmayr, H., Korzun, V., and Ebmeyer, E. (2006). Stacking quantitative trait loci: (QTL) for Fusarium head blight resistance from non-adapted sources in an European elite spring wheat background and assessing their effects on deoxynivalenol (DON) content and disease severity. Theor. Appl. Genet. 112, 562–569. doi: 10.1007/s00122-005-0163-4
Miller, J. D., Young, J. C., and Sampson, D. R. (1985). Deoxynivalenol and Fusarium head blight resistance in spring cereals. J. Phytopathol. 113, 359–367. doi: 10.1111/j.1439-0434.1985.tb04837.x
Nayak, S. N., Singh, V. K., and Varshney, R. K. (2017). “Marker-assisted selection,” in Encyclopedia of Applied Plant Sciences, eds B. Thomas, B. G. Murray, and D. J. Murphy (Waltham, MA: Academic Press), 183–197.
Salameh, A., Buerstmayr, M., Steiner, B., Neumayer, A., Lemmens, M., and Buerstmayr, H. (2011). Effects of introgression of two QTL for Fusarium head blight resistance from Asian spring wheat by marker-assisted backcrossing into European winter wheat on Fusarium head blight resistance, yield and quality traits. Mol. Breed. 28, 485–494. doi: 10.1007/s11032-010-9498-x
Schroeder, H. W., and Christensen, J. J. (1963). Factors affecting resistance of wheat to scab caused by Gibberella zeae. Phytopathology 53, 831–838.
Somers, D. J., Fedak, G., and Savard, M. (2003). Molecular mapping of novel genes controlling Fusarium head blight resistance and deoxynivalenol accumulation in spring wheat. Genome. 46, 555–564. doi: 10.1139/g03-033
Steiner, B., Buerstmayr, M., Wagner, C., Danler, A., Eshonkulov, B., Ehn, M., et al. (2019). Fine-mapping of the Fusarium head blight resistance QTL Qfhs.ifa-5A identifies two resistance QTL associated with anther extrusion. Theor. Appl. Genet. 132, 2039–2053. doi: 10.1007/s00122-019-03336-x
Szabó-Hevér, Á., Lehoczki-Krsjak, S., Varga, M., Purnhauser, L., Pauk, J., Lantos, C., et al. (2014). Differential influence of QTL linked to Fusarium head blight, Fusarium-damaged kernel, deoxynivalenol contents and associated morphological traits in a Frontana-derived wheat populatiom. Euphytica. 200, 9–26. doi: 10.1007/s10681-014-1124-2
Xu, F., Li, W., Yan, S., Zhang, C., Zheng, J., Du, J., et al. (2017). Analysis of pyramiding effect of major QTLs for resistance to scab in wheat (in Chinese with English abstract). J. Triticeae Crops. 37, 585–593.
Xue, S., Li, G., Jia, H., Lin, F., Cao, Y., Xu, F., et al. (2010a). Marker-assisted development and evaluation of near-isogenic lines for scab resistance QTLs of wheat. Mol. Breed. 25, 397–405. doi: 10.1007/s11032-009-9339-y
Xue, S., Li, G., Jia, H., Xu, F., Lin, F., Tang, M., et al. (2010b). Fine mapping Fhb4, a major QTL conditioning resistance to Fusarium infection in bread wheat: (Triticum aestivum L.). Theor. Appl. Genet. 121, 147–156. doi: 10.1007/s00122-010-1298-5
Xue, S., Xu, F., Tang, M., Zhou, Y., Li, G., An, X., et al. (2011). Precise mapping Fhb5, a major QTL conditioning resistance to Fusarium infection in bread wheat: (Triticum aestivum L.). Theor. Appl. Genet. 123, 1055–1063. doi: 10.1007/s00122-011-1647-z
Yu, J. B., Bai, G. H., Zhou, W. C., Dong, Y. H., and Kolb, F. L. (2007). Quantitative trait loci for Fusarium head blight resistance in a recombinant inbred population of Wangshuibai/Wheaton. Phytopathology 98, 87–94. doi: 10.1094/phyto-98-1-0087
Zhang, X., Rouse, M. N., Nava, I. C., Jin, Y., and Anderson, J. A. (2016). Development and verification of wheat germplasm containing both Sr2 and Fhb1. Mol. Breed. 36:85. doi: 10.1007/s11032-016-0502-y
Keywords: wheat, Fusarium head blight, marker-assisted selection, gene pyramiding, Fhb1, Fhb4, Fhb5
Citation: Zhang Y, Yang Z, Ma H, Huang L, Ding F, Du Y, Jia H, Li G, Kong Z, Ran C, Gu Z and Ma Z (2021) Pyramiding of Fusarium Head Blight Resistance Quantitative Trait Loci, Fhb1, Fhb4, and Fhb5, in Modern Chinese Wheat Cultivars. Front. Plant Sci. 12:694023. doi: 10.3389/fpls.2021.694023
Received: 12 April 2021; Accepted: 10 June 2021;
Published: 14 July 2021.
Edited by:
Feng Chen, Henan Agricultural University, ChinaReviewed by:
David Anthony Van Sanford, University of Kentucky, United StatesFrancis Chuks Ogbonnaya, Grains Research and Development Corporation, Australia
Copyright © 2021 Zhang, Yang, Ma, Huang, Ding, Du, Jia, Li, Kong, Ran, Gu and Ma. This is an open-access article distributed under the terms of the Creative Commons Attribution License (CC BY). The use, distribution or reproduction in other forums is permitted, provided the original author(s) and the copyright owner(s) are credited and that the original publication in this journal is cited, in accordance with accepted academic practice. No use, distribution or reproduction is permitted which does not comply with these terms.
*Correspondence: Zhengqiang Ma, zqm2@njau.edu.cn