- 1Key Laboratory of Crop Genetics and Physiology of Jiangsu Province, Jiangsu Key Laboratory of Crop Cultivation and Physiology, Co-innovation Center for Modern Production Technology of Grain Crops, Yangzhou University, Yangzhou, China
- 2Ministry of Agriculture Key Laboratory of Crop Ecophysiology and Farming System in the Middle Reaches of the Yangtze River, College of Plant Science and Technology, Huazhong Agricultural University, Wuhan, China
Six cultivated rice genotypes showing different stomatal conductance (gs) values were used to investigate the influence of leaf vein traits on leaf gas exchange and leaf hydraulics. The results showed that gs was the main determinant of the varietal difference in the net photosynthetic rate (PN), whereas the area-based leaf nitrogen content (Narea) and mesophyll conductance (gm) were not main factors. gs and PN were both positively correlated with leaf hydraulic conductance (Kleaf). A high density of leaf veins (vein length per leaf area, VLA), especially minor leaf veins (VLAminor), was of benefit for improving the Kleaf. The proportion of the minor leaf vein length to the total leaf vein length did not impact the leaf hydraulics or leaf gas exchange. Overall, these findings suggested that a high density of leaf veins, especially minor leaf veins, enhances Kleaf and promotes gs and PN in cultivated rice genotypes and a high VLA can be regarded as a high photosynthetic capacity trait in rice plants.
Introduction
Under the current ambient atmospheric conditions, CO2 diffusional conductance from air to carboxylation sites is regarded as one of the main limiting factors of net photosynthetic rate (PN) in C3 plants (Evans et al., 2009; Li et al., 2009; Yamori et al., 2011; Flexas et al., 2012; Adachi et al., 2013; Gago et al., 2019). To reach carboxylation sites, CO2 in the air must first overcome the air–leaf boundary resistance to reach the surroundings of the stomata, and it then enters the stomatal pores to reach the substomatal cavity, diffuses to the surroundings of the cell wall, and successively passes through the cell wall, cell membrane, cytoplasm, chloroplast envelope, and the stroma (Terashima et al., 2011; Tholen et al., 2012). The CO2 diffusional resistance from air to the surface of the leaf is called boundary layer resistance, the CO2 diffusional resistance from air to the substomatal cavity is called stomatal resistance, and the CO2 diffusional resistance from the substomatal cavity to the carboxylation sites is called mesophyll resistance. Mesophyll resistance can be as important as stomatal resistance under many conditions (Terashima et al., 2011), although it was ignored in earlier studies (Farquhar et al., 1980; Kodama et al., 2011). The reciprocals of stomatal resistance and mesophyll resistance are called stomatal conductance (gs) and mesophyll conductance (gm), respectively. Many previous studies have shown that PN is positively correlated with both gs and gm (Giuliani et al., 2013; Carriquí et al., 2015; Liu and Li, 2016).
Stomatal pores are the common pathway for CO2 entering the leaf and H2O evaporating from the leaf. gs is mainly determined by the stomatal size, stomatal density and distribution, and especially by the stomatal aperture (Xu and Zhou, 2008; Ocheltree et al., 2012; Ouyang et al., 2017). gs has been found to be closely linked to plant hydraulics (Hirasawa et al., 1992; Brodribb et al., 2007; Xiong and Nadal, 2020). Tabassum et al. (2016) reported that the PN, gs, and the transpiration rate (E) values in Oryza sativa L. plants were all positively correlated with whole-plant hydraulic conductance.
As leaf hydraulic conductance (Kleaf) is one of the key components of whole-plant hydraulic conductance (Sack et al., 2003; Sack and Holbrook, 2006), it is vitally important for determining leaf gas exchange parameters. Brodribb et al. (2007) found that PN was positively correlated with Kleaf across diverse terrestrial plants. Hirasawa et al. (1992) found that gs was positively correlated with Kleaf in rice plants across different light and chemical treatments. Taylaran et al. (2011) found that the PN of the high-yield rice cultivar Takanari was higher than that of the other common rice cultivars, which was partly due to its higher Kleaf. In higher plants, transpiration drives water from the stem into the petiole, and then, the water enters the midrib, flows in an orderly fashion to different vein branches, passes through the vascular bundle sheath, enters the mesophyll tissue, evaporates into the intercellular airspace, and finally diffuses out of the leaf from stomatal pores (Rockwell et al., 2014; Xiong et al., 2017). Thus, leaf hydraulic transport can be divided into xylem hydraulic transport and outside-xylem hydraulic transport.
According to the water transport route inside leaves, Kleaf is mainly determined by the leaf vein traits such as the leaf vein density, the size of the xylem conduits within the bundle sheath (Flexas et al., 2013), and the leaf anatomical traits such as the fraction of intercellular airspace (Xiong et al., 2017). Many recent studies have focused on the relationships between Kleaf and leaf vein density (vein length per leaf area, VLA) (Xiong et al., 2015; Tabassum et al., 2016). Although a few studies have demonstrated that Kleaf showed no or even negative relationships with VLA, most studies illustrated that a high VLA is beneficial for improving Kleaf and promoting gs and PN (Sack and Frole, 2006; Brodribb et al., 2007; Boyce et al., 2009; Brodribb and Field, 2010; McKown et al., 2010; Field et al., 2011; Walls, 2011; Nardini et al., 2012; Flexas et al., 2013). High VLA can improve the parallel transport pathways per leaf area, thus improving the xylem hydraulic conductance (Kx), and it can also shorten the distance from veins to evaporating sites, thus improving the outside-xylem hydraulic conductance (Kox) (Sack and Frole, 2006; Brodribb et al., 2007; Sack et al., 2013; Buckley et al., 2015).
Nevertheless, VLA was found to affect Kx but not Kox or Kleaf across Oryza species, including cultivated and wild genotypes (Xiong et al., 2015, 2017). The irrelevance of Kox and Kleaf with VLA may be due to the low ratio of xylem hydraulic resistance (Rx) to leaf hydraulic resistance (Rleaf), which is only 40% (Xiong et al., 2017). Thus, although a high VLA improved Kx, the improvement in Kx contributed to Kleaf very slightly. Although the average Rx/Rleaf in Oryza species was only 40%, the ratios in cultivated rice genotypes were much higher than 40% (Xiong et al., 2017), which may be due to the increase in VLA during domestication. Thus, although no correlations were found between Kleaf and VLA across Oryza species, these correlations may exist when only considering cultivated rice genotypes because cultivated genotypes have relatively high Rx/Rleaf (Xiong et al., 2017). Therefore, we hypothesized that in cultivated rice genotypes in which Rx/Rleaf is relatively high, high VLA can enhance Kleaf and promote leaf gas exchange.
In fact, the correlations among leaf gas exchange, leaf hydraulics, and leaf vein traits have already attracted a lot of attention. For example, Brodribb et al. (2007) reported that leaf maximum photosynthetic rate and venation are linked by hydraulics across 43 species. Brocious and Hacke (2016) found that stomatal conductance was positively correlated with the transport capacity of the petiole, estimated from the diameter and number of xylem vessels, whereas the variation in stomatal conductance and leaf hydraulic conductance was not linked to vein density or other anatomical lamina properties across different populus genotypes. However, most previous studies were done in wood species, and rare studies were done in cereal species, especially in rice.
In the present study, six rice genotypes that showed different gs values in a previous study (Ye et al., 2019) were selected to verify the hypothesis that high VLA can enhance Kleaf and promote leaf gas exchange in cultivated rice genotypes. The results may provide insights for high photosynthetic capacity rice breeding.
Materials and Methods
Plant Materials
A pot experiment was conducted at the Huazhong Agricultural University (114.37°E, 30.48°N), Wuhan, Hubei Province, China. Six cultivated rice genotypes (O. sativa L.) found worldwide were used, including Kirmizi Celtik, Huayou 675, Teqing, Huanghuazhan, Champa, and N22. The gs of these six genotypes varied greatly in the study by Ye et al. (2019), thus indicating feasibility for studying the underlying mechanisms for different gs and PN values in rice. Rice plants were grown from September to December 2014. After germination on moist filters, seeds were transferred to nursery plates. When the seedlings had developed an average of three leaves, they were transplanted to 11 L pots with a density of three hills per pot and two seedlings per hill. There were five pots per genotype, and each pot was filled with 10 kg of soil. The soil used for the experiment had a clay loam texture, with pH 6.63, organic matter 6.42 g/kg, total nitrogen (N) 0.07 mg/kg, available phosphorus (P) 8.21 mg/kg, and available potassium (K) 126.95 mg/kg. P and K were applied as basal fertilizers at an amount of 1.5 g/pot. N was applied at an amount of 2.0 g(N)/pot, with 40% applied as a basal fertilizer, and 60% applied at the mid-tillering stage. Plants were watered daily, and a minimum 2 cm water layer was maintained to avoid drought stress. Pests were intensively controlled using chemical pesticides.
Rice plants were grown outdoors. All genotypes were arranged in a random design with five replicates. The radiation intensity, average temperature, and relative humidity during rice growth were 12.6 ± 5.7 MJ/m2/d, 23.3 ± 3.4°C, and 74.4 ± 10.4%, respectively. Gas exchange measurements were conducted in a growth chamber (Conviron GR48, Controlled Environments Limited, Winnipeg, MB, Canada) [photosynthetic photon flux density (PPFD), 1,000 μmol(photo)/m2/s at the leaf level; temperature, 28°C; relative humidity, 60%; and CO2 concentration, 400 μmol/mol] to avoid the influence of a changing environment on gas exchange parameters. The vapor pressure deficit between leaf and air (VPDleaf−air) of the six rice genotypes during the measurements is shown in Supplementary Table 1. The measurements were conducted 2 weeks after mid-tillering fertilization, and all measurements were conducted on the newly expanded leaves from three different pots of each genotype.
Gas Exchange and Chlorophyll Fluorescence Measurements
A portable photosynthesis system (LI-6400XT, LI-CORInc., Lincoln, NE, United States) with an integrated fluorescence leaf chamber (Li-6400-40; Li-Cor) was used to measure gas exchange and chlorophyll fluorescence on leaves between 08:00 and 16:00. Measurements began after the plants had acclimatized to the chamber for ~2 h. In the LI-6400XT cuvette, the ambient CO2 concentration was controlled and set to 400 μmol/mol, the leaf temperature was maintained at 28°C, the PPFD was 1,500 μmol(photo)/m2/s, and the flow rate was 500 μmol/s. After reaching a steady state, which usually takes 25 min, the gas exchange parameters, steady-state fluorescence (Fs), and maximum fluorescence (Fm') were recorded with a light saturating pulse of 8,000 μmol(photo)/m2/s. The actual photochemical efficiency of photosystem II (ΦPSII) was calculated as follows:
The electron transport rate (J) was calculated as follows:
where α is the leaf absorptance and β is the partitioning of absorbed quanta between photosystem II and photosystem I. The product αβ was determined from the slope of the relationship between ΦPSII and the quantum efficiency of CO2 uptake (ΦCO2), which was obtained by varying the light intensity under non-photorespiratory conditions at <2% O2 (Valentini et al., 1995).
The variable J method described by Harley et al. (1992) was used to calculate the chloroplastic CO2 concentration (Cc) and gm. Cc and gm were calculated as follows:
where Γ* represents the CO2 compensation point in chloroplasts without day respiration. The day respiration (Rd) and the apparent CO2 photocompensation point () were determined using the Laisk method (Brooks and Farquhar, 1985). Briefly, A/Ci (A, net photosynthetic rate; Ci, intercellular CO2 concentration) curves were measured over the linear portion of the response curve (at 100, 80, 50, and 25 μmol CO2/mol air) over three PPFDs (150, 300, and 600 μmol/m2/s) with an LI 6400-02B chamber (Li-Cor), and then linear regressions to the responses for each PPFD were fitted for individual leaves. The intersection point of three A/Ci curves was considered as (x-axis) and Rd (y-axis) (von Caemmerer et al., 1994). Γ* was calculated as follows:
The Γ* and Rd of the six rice genotypes are shown in Supplementary Table 2.
Leaf Hydraulic Conductance
The evaporative flux method (EFM) was used to determine the Kleaf (Sack et al., 2002; Brodribb et al., 2007; Guyot et al., 2012; Sack and Scoffoni, 2012; Tabassum et al., 2016). A leaf of each genotype was excised in water, and the base of the leaf was placed in a test tube filled with distilled water under favorable conditions for transpiration (in a growth chamber under a PPFD of 1,000 μmol(photo)/m2/s and air temperature of 28°C). Immediately after placing the leaf in a test tube filled with distilled water, the leaf was attached to a Li-COR 6400XT portable infrared gas analyzer (IRGA) (LI-COR, NE, United States) to record the leaf transpiration rate (E). A Dewpoint Potential Meter WP4C (Decagon, Pullman, WA, United States) was used to measure the leaf water potential (Ψleaf) of the leaf that was used to measure E. Kleaf was calculated as follows (Taylaran et al., 2011):
Leaf Morphological Traits
Leaf width was measured in vivo, and leaves were excised to count the major veins (midrib vein and large veins) and minor veins separately under 40× magnification using a light microscope (SA3300, Beijing Tech Instrument Co., Ltd, Beijing, China). Four leaves were counted for each genotype. As rice leaf veins are parallel to each other, the major leaf vein density (VLAmajor), minor leaf vein density (VLAminor), and total leaf vein density (VLA) were calculated as follows:
Leaf Nitrogen Content
The leaf used for gas exchange measurements was detached, and another two leaves were also detached, then the leaf area of these three leaves was measured using a LI-Cor 3000C (LI-COR Inc., Lincoln, NE, United States) leaf area analyzer. Leaves were then oven-dried at 80°C until they achieved a constant weight. Afterward, the leaf dry mass was weighed and the leaf mass per area (LMA) was calculated as the ratio of the leaf dry mass to leaf area. The leaf N content based on leaf mass (Nmass, %) was measured with an Elementar Vario MAX CN analyzer (Elementar Analysesysteme GmbH, Hanau, Germany), and the area-based leaf N content (Narea) was calculated by multiplying Nmass with LMA.
Statistical Analysis
One-way analysis of variance (ANOVA) was used to assess the effects of the genotypes on each parameter using Statistix 9.0 software (Analytical Software, Tallahassee, FL, United States). Parameters were compared between genotypes based on the least significant difference (LSD) test level at the 0.05 probability level. Graphs were created, and a linear regression analysis was performed to test the correlations between the parameters using SigmaPlot 10.0 (Systat Software Inc., CA, United States).
Results
Varietal Differences in Leaf Gas Exchange Parameters and Narea
As shown in Table 1, PN, Narea, and gs showed significant varietal differences among the six rice genotypes. PN ranged from 21.2 to 36.2 μmol/m2/s, and the highest and the lowest values were found in Champa and N22, respectively. Kirmizi Celtik showed the highest Narea of 2.03 g/m2 and N22 showed the lowest Narea of 1.23 g/m2. gs ranged from 0.18 to 0.46 mol/m2/s and the highest and lowest values were found in Champa and N22, respectively. gm showed no significant varietal difference among the six rice genotypes. The highest gm was 0.33 mol/m2/s, which was found in Teqing, whereas the lowest gm was 0.23 mol/m2/s, which was found in Kirmizi Celtik. As shown in Figure 1, PN was not correlated with Narea or gm but was positively correlated with gs across the six rice genotypes.
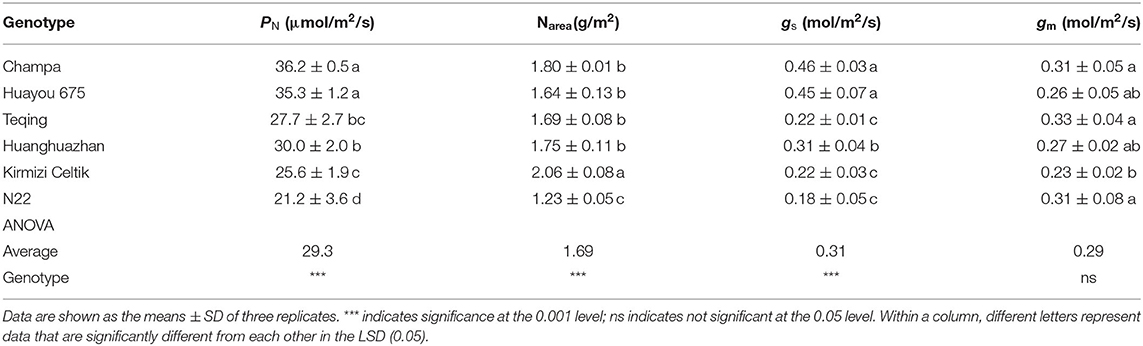
Table 1. Gas exchange parameters and area-based leaf nitrogen content (Narea) of the six rice genotypes.
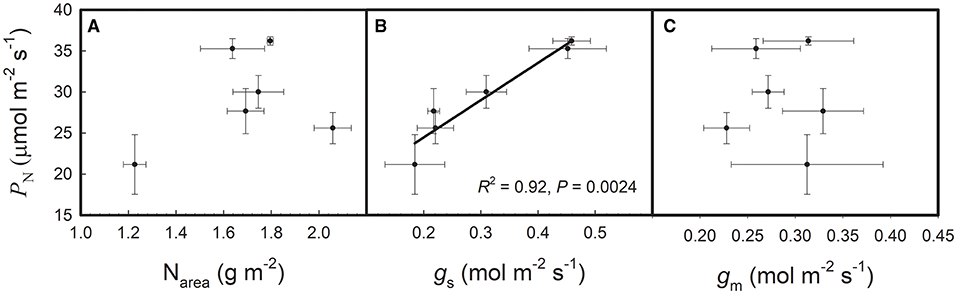
Figure 1. Relationships of net photosynthetic rate (PN) with: (A) area-based leaf nitrogen content (Narea), (B) stomatal conductance (gs), and (C) mesophyll conductance (gm) across the six rice genotypes. Data are shown as the means ± SD of three replicates.
Varietal Differences in the Leaf Morphological Traits and Leaf Hydraulics
As shown in Table 2, leaf width showed significant varietal differences. Champa possessed the widest leaf of 14.2 mm, whereas Kirmizi Celtik possessed the narrowest leaf of 9.4 mm. VLA, VLAminor, and the proportion of minor leaf vein length to the total leaf vein length all showed no significant varietal differences. VLAmajor showed significant varietal differences. Huayou 675 had the largest VLAmajor of 0.82 mm/mm2, and N22 had the smallest VLAmajor of 0.66 mm/mm2. E, Ψleaf, and Kleaf all showed no significant varietal differences.
Relationships Among the Leaf Gas Exchange Parameters, Leaf Hydraulics, and Leaf Morphological Traits
As shown in Figure 2, gs was positively correlated with both E and Kleaf across the six rice genotypes and PN was positively correlated with Kleaf but had no relationship with E across the six rice genotypes. As shown in Figure 3, E, Kleaf, gs, and PN were all positively correlated with VLA and VLAminor across the six rice genotypes; PN was positively correlated with VLAmajor, but E, Kleaf, and gs had no relationship with VLAmajor across the six rice genotypes.
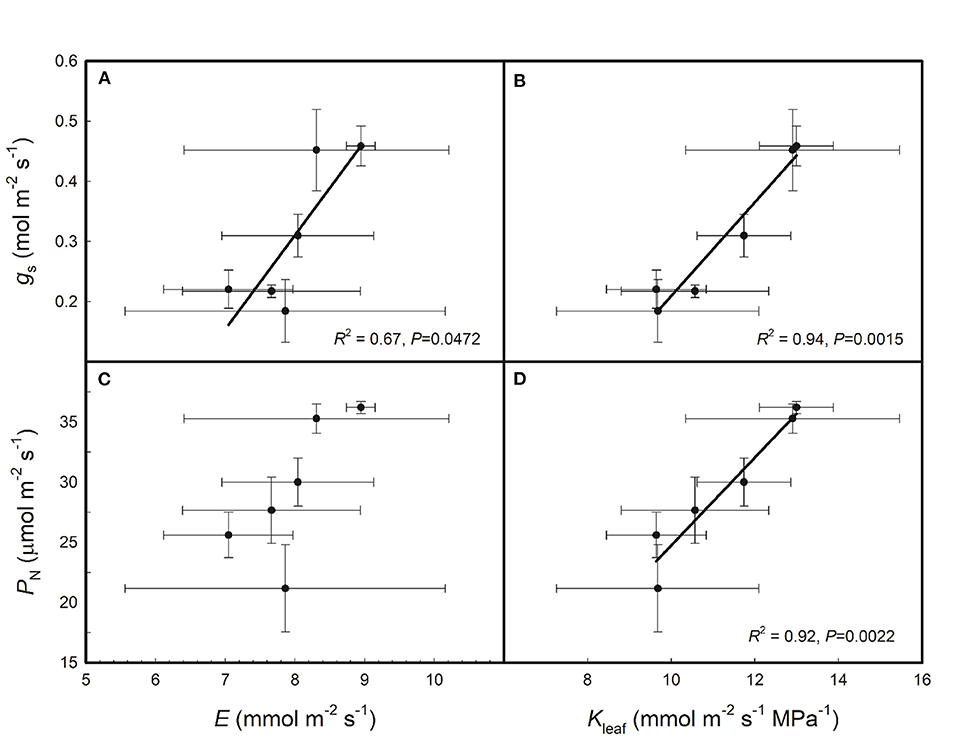
Figure 2. Relationships of stomatal conductance (gs) and net photosynthetic rate (PN) with leaf transpiration rate (E) (A,C) and leaf hydraulic conductance (Kleaf) (B,D) across the six rice genotypes. Data are shown as the means ± SD of three replicates.
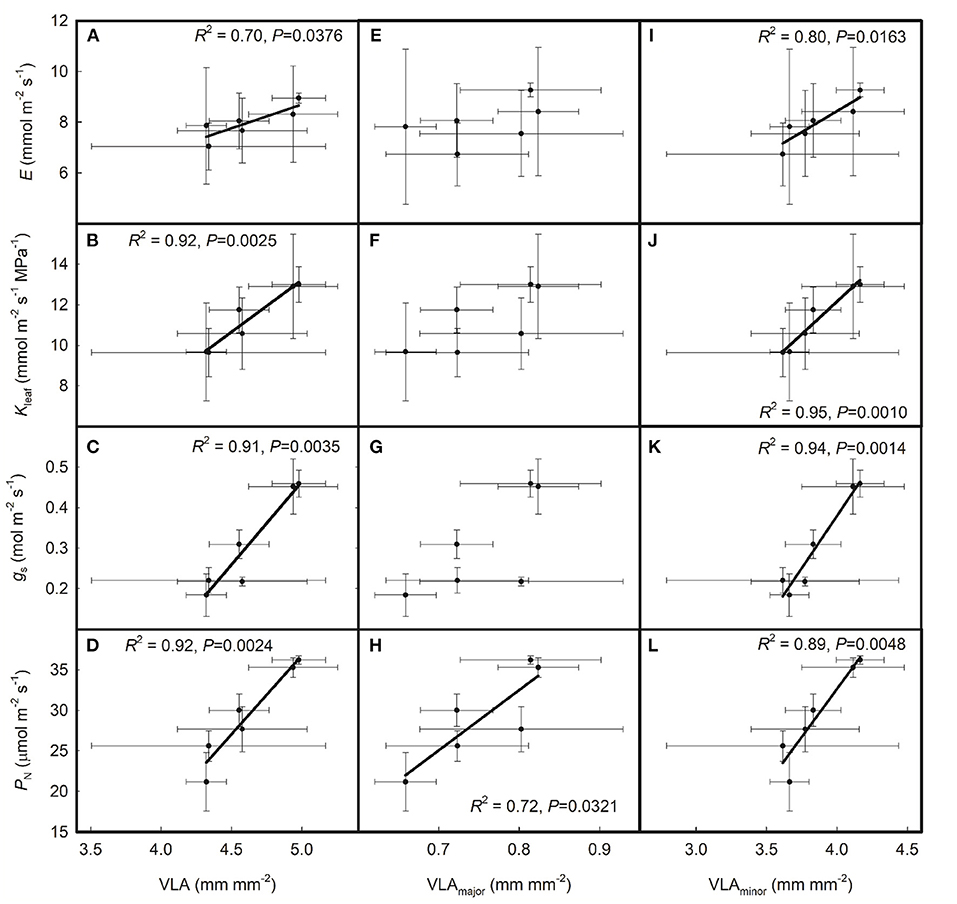
Figure 3. Relationships of the leaf hydraulics and leaf gas exchange parameters with (A–D) total leaf vein density (VLA), (E–H) major leaf vein density (VLAminor), and (I–L) minor leaf vein density (VLAminor) across the six rice genotypes. Data are shown as the means ± SD. There were four replicates for leaf vein densities and three replicates for PN, gs, Kleaf, and E.
Discussion
In the present study, PN was not correlated with Narea and gm but was correlated with gs and Kleaf. VLA and VLAminor showed no varietal differences in the present study, which was perhaps due to the few genotypes used, and their vein traits ranged very narrowly. Rice leaf veins are distributed in parallel, and the leaf vein density is strongly affected by the leaf width (Baird et al., 2021). For example, Tabassum et al. (2016) reported that polyethylene glycol–induced water deficit caused rice leaf narrowing and led to an increase in leaf density. Xiong et al. (2015) investigated the leaf morphological traits of 11 rice genotypes, including seven wild genotypes and four cultivated genotypes, and found that leaf morphological traits showed great varietal differences because the leaf area showed ~seven times the variation, ranging from 18.4 to 127.3 cm2, and leaf width showed ~six times the variation, ranging from 0.38 to 2.20 cm. The leaf width in the present study only showed 1.5 times the variation, ranging from 9.4 to 14.2 mm. Thus, leaf vein densities in the present study did not vary greatly. More genotypes and varying environmental conditions should be included in future studies to investigate the varietal differences in rice leaf vein traits and their impacts on leaf gas exchange.
In the present study, Kleaf, E, gs, and PN all substantially increased with VLA and VLAminor across the six cultivated rice genotypes, thus verifying the hypothesis that high VLA can enhance Kleaf and promote leaf gas exchange in cultivated rice genotypes in which Rx/Rleaf is relatively high. By sorting the correlations between leaf gas exchange parameters, leaf hydraulic parameters, and leaf vein densities, we drew the conclusion that leaf vein density affected leaf gas exchange parameters by regulating leaf water transport. However, we still lack the analyses of many other vein traits such as the diameter of xylem conduits and the outside xylem anatomical traits on leaf hydraulics and gas exchange. More studies should be done to explore the coordination between the physiological and structural traits of the leaf.
Furthermore, VLA and Kleaf data from other studies (Xiong et al., 2015, 2017) were extracted to verify our hypothesis. As shown in Figure 4, Kleaf was positively correlated with VLA and VLAminor across the cultivated rice genotypes, whereas these correlations disappeared across the wild rice genotypes. Therefore, we conclude that in cultivated rice genotypes, high VLA and high VLAminor can enhance Kleaf and promote leaf gas exchange, and a high VLA can be regarded as a feature of rice materials having high photosynthetic capacity. Variations in VLA drove the correlation between Kleaf and VLA, which was probably due to the determining role of VLA on Kleaf, as other features of hydraulic architecture may vary slightly in cultivated rice genotypes. However, this correlation may be obscured when other features of hydraulic architecture vary. For example, the positive effects of high VLA on Kleaf may be attenuated by the negative effects of small xylem conduits in a plant. Besides, as one of the few studies on cereal crops, the present study showed different results with previous studies on wood species, for example, populus plants in Brocious and Hacke's (Brocious and Hacke, 2016) study. A positive correlation was found between Kleaf and VLA in the present study but not in Brocious and Hacke (2016) may also be attributed to the different importance of VLA to Kleaf in the species. The comprehensive effects of hydraulic architecture on leaf hydraulics should be summarized.
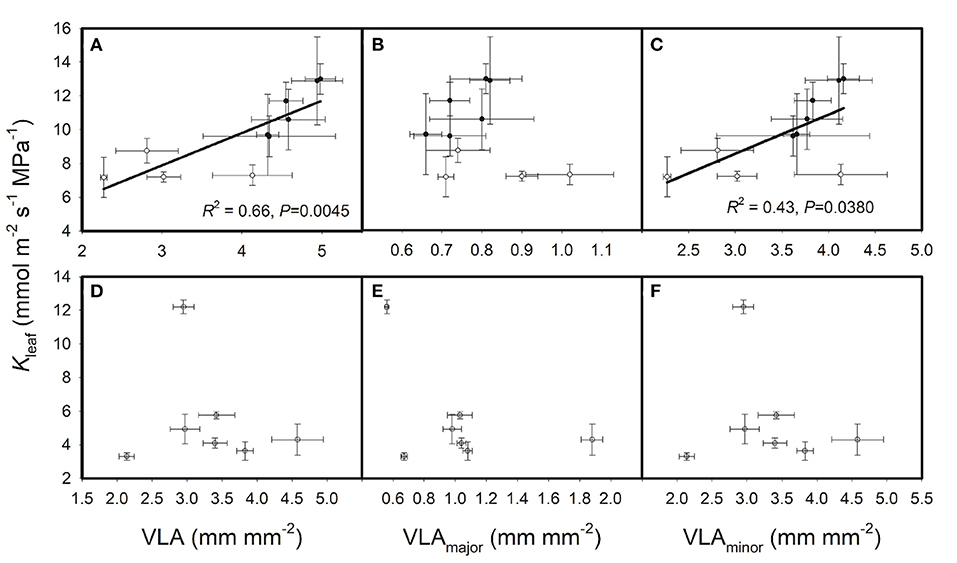
Figure 4. Relationships of leaf hydraulic conductance (Kleaf) with leaf vein densities across (A–C) cultivated rice genotypes and (D–F) wild rice genotypes. The solid circles are data for the six cultivated rice genotypes in the present study, and the empty circles are data from Xiong et al. (2015, 2017) studies.
Correlations between Kleaf and VLA existing in cultivated but not wild rice genotypes illustrate the importance of leaf vein traits during rice domestication or genetic improvement. For example, Wu et al. (2020) revealed that an increase in leaf vein dimensions during rice genetic improvement enhanced leaf gas exchange. In addition to leaf vein traits, leaf morphological traits associated with leaf gas exchange, such as stomatal density and stomatal size in rice, have also been improved during crop genetic improvement (Panda et al., 2018; Wu et al., 2020). There is a need for establishing the underlying morphological and physiological changes for crop improvement.
A previous study (Venturas et al., 2017) illustrated that minor leaf vein length accounts for more than 80% of the total leaf vein length in higher plants. In the present study, minor leaf vein length accounted for 83.5% of the total leaf vein length on average (Table 2). Xiong et al. (2015) reported that the proportion of minor leaf vein length to total leaf vein length but not leaf vein density is the critical factor determining Kleaf. However, in the present study, neither E, Kleaf, gs, nor PN showed significant correlations with the proportion of minor leaf vein length to total leaf vein length (data not shown).
Except for the leaf vein density, other vein traits also greatly impacted Kleaf. Previous studies documented that short distance from minor veins to stomata and large diameters of xylem conduit accompanied by thin xylem conduit walls were beneficial for improving Kleaf (Brodribb et al., 2007; Sack and Scoffoni, 2013; Venturas et al., 2017; Xiong and Nadal, 2020). Nardini et al. (2014) found that large leaves tend to have higher VLAmajor than small leaves and can promote Kleaf. Simonin et al. (2012) demonstrated that Kleaf increased with leaf area. However, a recent study exploring the development and biophysical determinations of grass leaf size worldwide revealed that small leaves have hydraulic benefits due to their higher VLAmajor (Baird et al., 2021). Optimal leaf morphological and anatomical traits for improving Kleaf and PN should be defined to provide theories for high photosynthetic capacity rice breeding.
Kx is mainly determined by leaf vein traits, whereas Kox is mainly determined by leaf anatomical traits. Xiong et al. (2017) found that Kox is positively correlated with the fraction of intercellular airspace, the mesophyll cell surface area exposed to intercellular airspace per leaf area, and the surface area of chloroplasts exposed to intercellular airspace per leaf area, whereas it is negatively correlated with cell wall thickness. gm has been reported to be positively correlated with gs in recent years (Flexas et al., 2013). One reason for the positive relationship between gs and gm is that they are both positively correlated with Kleaf (Xiong and Nadal, 2020). gs is positively correlated with Kleaf due to the close relationship between stomatal opening and leaf water transport; gm is positively correlated with Kleaf and Kox because gm and Kox are mediated by leaf anatomical traits (Xiong et al., 2017). However, Kx, Kox, and leaf anatomical traits were not measured in the present study, and more studies should be done to investigate and explain the coordination among leaf gas exchange, leaf hydraulics, and leaf anatomy.
In addition, the water transport pathways inside leaves, i.e., where the evaporating sites occur, are currently unclear. Rockwell et al. (2014) reported that water dominantly evaporated from the vascular bundle sheath and its surroundings to the intercellular airspace in Quercus rubra. Moreover, the conversion of water between the vapor and liquid phases is unknown. For example, Buckley et al. (2015) found that vapor phase transport contributed 39.25–44.00% to Kox when the temperature difference between the xylem and leaf epidermis reached 0.2 K, suggesting that temperature greatly affects leaf hydraulic status. Evaporating sites and leaf hydraulic status inside rice leaves need more investigation.
Conclusions
In the present study, VLAmajor showed great varietal differences among cultivated rice genotypes, whereas VLA, VLAminor, and the proportion of minor leaf vein length to total leaf vein length showed no varietal differences. E, Kleaf, gs, and PN were all positively correlated with VLA and VLAminor, and PN was positively correlated with VLAmajor. We concluded that high VLA and high VLAminor can enhance Kleaf and promote leaf gas exchange in the cultivated rice genotypes and high VLA can be regarded as a high photosynthetic capacity trait in rice plants.
Data Availability Statement
The original contributions presented in the study are included in the article/Supplementary Material, further inquiries can be directed to the corresponding author.
Author Contributions
MY designed the research and wrote the paper. MY and ZuoZ conducted the experiments. MY and MW analyzed the data. HZ and ZujZ commented and revised the paper. All authors contributed to the article and approved the submitted version.
Funding
This research was funded by the National Natural Science Foundation of China, grant nos. 31871532 and 31871559, the National Key Research and Development Program of China, grant nos. 2016YFD0300102 and 2016YFD0300502, and the Priority Academic Program Development of Jiangsu Higher Education Institutions.
Conflict of Interest
The authors declare that the research was conducted in the absence of any commercial or financial relationships that could be construed as a potential conflict of interest.
Publisher's Note
All claims expressed in this article are solely those of the authors and do not necessarily represent those of their affiliated organizations, or those of the publisher, the editors and the reviewers. Any product that may be evaluated in this article, or claim that may be made by its manufacturer, is not guaranteed or endorsed by the publisher.
Acknowledgments
We thank Ms. Xi Liu from Huazhong Agricultural University for helping to measure the leaf gas exchange parameters. We also thank Professor Yong Li from Huazhong Agricultural University for revising and commenting on this manuscript.
Supplementary Material
The Supplementary Material for this article can be found online at: https://www.frontiersin.org/articles/10.3389/fpls.2021.693815/full#supplementary-material
References
Adachi, S., Nakae, T., Uchida, M., Soda, K., Takai, T., Oi, T., et al. (2013). The mesophyll anatomy enhancing CO2 diffusion is a key trait for improving rice photosynthesis. J. Exp. Bot. 64, 1061–1072. doi: 10.1093/jxb/ers382
Baird, A. S., Taylor, S. H., Pasquet-Kok, J., Vuong, C., Zhang, Y., Watcharamongkol, T., et al. (2021). Developmental and biophysical determinants of grass leaf size worldwide. Nature 592, 242–247. doi: 10.1038/s41586-021-03370-0
Boyce, C. K., Brodribb, T. J., Field, T. S., and Zwieniecki, M. J. (2009). Angiosperm leaf vein evolution was physiologically and environmentally transformative. Proc. R. Soc. B. Biol. Sci. 276, 1771–1776. doi: 10.1098/rspb.2008.1919
Brocious, C. A., and Hacke, U. G. (2016). Stomatal conductance scales with petiole xylem traits in populus genotypes. Funct. Plant Biol. 43, 553–562. doi: 10.1071/FP15336
Brodribb, T. J., and Field, T. S. (2010). Leaf hydraulic evolution led a surge in leaf photosynthetic capacity during early angiosperm diversification. Ecol. Lett. 13, 175–183. doi: 10.1111/j.1461-0248.2009.01410.x
Brodribb, T. J., Field, T. S., and Jordan, G. J. (2007). Leaf maximum photosynthetic rate and venation are linked by hydraulics. Plant Physiol. 144, 1890–1898. doi: 10.1104/pp.107.101352
Brooks, A., and Farquhar, G. D. (1985). Effect of temperature on the CO2/O2 specificity of ribulose-1, 5-bisphosphate carboxylase/oxygenase and the rate of respiration in the light. Planta 165, 397–406. doi: 10.1007/BF00392238
Buckley, T. N., John, G. P., Scoffoni, C., and Sack, L. (2015). How does leaf anatomy influence water transport outside the xylem? Plant Physiol. 168, 1616–1635. doi: 10.1104/pp.15.00731
Carriquí, M., Cabrera, H. M., Conesa, M. À., Coopman, R. E., Douthe, C., Gago, J., et al. (2015). Diffusional limitations explain the lower photosynthetic capacity of ferns as compared with angiosperms in a common garden study. Plant Cell Environ. 38, 448–460. doi: 10.1111/pce.12402
Evans, J. R., Kaldenhoff, R., Genty, B., and Terashima, I. (2009). Resistances along the CO2 diffusion pathway inside leaves. J. Exp. Bot. 60, 2235–2248. doi: 10.1093/jxb/erp117
Farquhar, G. D., von Caemmerer, S., and Berry, J. A. (1980). A biochemical model of photosynthetic CO2 assimilation in leaves of C3 species. Planta 149, 78–90. doi: 10.1007/BF00386231
Field, T. S., Brodribb, T. J., Iglesias, A., Chatelet, D. S., Baresch, A., Upchurch, G. R. Jr., et al. (2011). Fossil evidence for Cretaceous escalation in angiosperm leaf vein evolution. Proc. Natl. Acad. Sci. U. S. A. 108, 8363–8366. doi: 10.1073/pnas.1014456108
Flexas, J., Barbour, M. M., Brendel, O., Cabrera, H. M., Carriqu,í, M., Díaz-Espejo, A., et al. (2012). Mesophyll diffusion conductance to CO2: an unappreciated central player in photosynthesis. Plant Sci. 193, 70–84. doi: 10.1016/j.plantsci.2012.05.009
Flexas, J., Scoffoni, C., Gago, J., and Sack, L. (2013). Leaf mesophyll conductance and leaf hydraulic conductance: an introduction to their measurement and coordination. J. Exp. Bot. 64, 3965–3981. doi: 10.1093/jxb/ert319
Gago, J., Carriquí, M., Nadal, M., Clemente-Moreno, M. J., Coopman, R. E., Fernie, A. R., et al. (2019). Photosynthesis optimized across land plant phylogeny. Trends Plant Sci. 24, 947–958. doi: 10.1016/j.tplants.2019.07.002
Giuliani, R., Koteyeva, N., Voznesenskaya, E., Evans, M. A., Cousins, A. B., and Edwards, G. E. (2013). Coordination of leaf photosynthesis, transpiration, and structural traits in rice and wild relatives (genus Oryza). Plant Physiol. 162, 1632–1651. doi: 10.1104/pp.113.217497
Guyot, G., Scoffoni, C., and Sack, L. (2012). Combined impacts of irradiance and dehydration on leaf hydraulic conductance: insights into vulnerability and stomatal control. Plant Cell Environ. 35, 857–871. doi: 10.1111/j.1365-3040.2011.02458.x
Harley, P. C., Loreto, F., Di Marco, G., and Sharkey, T. D. (1992). Theoretical considerations when estimating the mesophyll conductance to CO2 flux by analysis of the response of photosynthesis to CO2. Plant Physiol. 98, 1429–1436. doi: 10.1104/pp.98.4.1429
Hirasawa, T., Tsuchida, M., and Ishihara, K. (1992). Relationship between resistance to water transport and exudation rate and the effect of the resistance on the midday depression of stomatal aperture in rice plants. Jap. J. Crop Sci. 61, 145–152. doi: 10.1626/jcs.61.145
Kodama, N., Wada, R., Nakayama, T., Takemura, K., and Matsumi, Y. (2011). “High precision and continuous measurements of mesophyll and stomatal conductance to CO2 diffusion during photosynthesis using QCL,” in AGU Fall Meeting Abstracts (San Francisco, CA).
Li, Y., Gao, Y., Xu, X., Shen, Q., and Guo, S. (2009). Light-saturated photosynthetic rate in high-nitrogen rice (Oryza sativa L.) leaves is related to chloroplastic CO2 concentration. J. Exp. Bot. 60, 2351–2360. doi: 10.1093/jxb/erp127
Liu, X., and Li, Y. (2016). Varietal difference in the correlation between leaf nitrogen content and photosynthesis in rice (Oryza sativa L.) plants is related to specific leaf weight. J. Integr. Agr. 15, 2002–2011. doi: 10.1016/S2095-3119(15)61262-X
McKown, A. D., Cochard, H., and Sack, L. (2010). Decoding leaf hydraulics with a spatially explicit model: principles of venation architecture and implications for its evolution. Am. Nat. 175, 447–460. doi: 10.1086/650721
Nardini, A., Õunapuu-Pikas, E., and Savi, T. (2014). When smaller is better: leaf hydraulic conductance and drought vulnerability correlate to leaf size and venation density across four Coffea arabica genotypes. Funct. Plant Biol. 41, 972–982. doi: 10.1071/FP13302
Nardini, A., Pedà, G., and Rocca, N. L. (2012). Trade-offs between leaf hydraulic capacity and drought vulnerability: morpho-anatomical bases, carbon costs and ecological consequences. New Phytol. 196, 788–798. doi: 10.1111/j.1469-8137.2012.04294.x
Ocheltree, T. W., Nippert, J. B., and Prasad, P. V. V. (2012). Changes in stomatal conductance along grass blades reflect changes in leaf structure. Plant Cell Environ. 35, 1040–1049. doi: 10.1111/j.1365-3040.2011.02470.x
Ouyang, W., Struik, P. C., Yin, X., and Yang, J. (2017). Stomatal conductance, mesophyll conductance, and transpiration efficiency in relation to leaf anatomy in rice and wheat genotypes under drought. J. Exp. Bot. 68, 5191–5205. doi: 10.1093/jxb/erx314
Panda, D., Mahakhud, A., Mohanty, B., Mishra, S. S., and Barik, J. (2018). Genotypic variation of photosynthetic gas exchange and stomatal traits in some traditional rice (Oryza sativa L.) landraces from Koraput, India for crop improvement. Physiol. Mol. Biol. Plants 24, 973–983. doi: 10.1007/s12298-018-0542-3
Rockwell, F. E., Holbrook, N. M., and Stroock, A. D. (2014). The competition between liquid and vapor transport in transpiring leaves. Plant Physiol. 164, 1741–1758. doi: 10.1104/pp.114.236323
Sack, L., Cowan, P. D., Jaikumar, N., and Holbrook, N. M. (2003). The ‘hydrology’ of leaves: co-ordination of structure and function in temperate woody species. Plant Cell Environ. 26, 1343–1356. doi: 10.1046/j.0016-8025.2003.01058.x
Sack, L., and Frole, K. (2006). Leaf structural diversity is related to hydraulic capacity in tropical rain forest trees. Ecology 87, 483–491. doi: 10.1890/05-0710
Sack, L., and Holbrook, N. M. (2006). Leaf hydraulics. Annu. Rev. Plant Biol. 57, 361–381. doi: 10.1146/annurev.arplant.56.032604.144141
Sack, L., Melcher, P. J., Zwieniecki, M. A., and Holbrook, N. M. (2002). The hydraulic conductance of the angiosperm leaf lamina: a comparison of three measurement methods. J. Exp. Bot. 53, 2177–2184. doi: 10.1093/jxb/erf069
Sack, L., and Scoffoni, C. (2012). Measurement of leaf hydraulic conductance and stomatal conductance and their responses to irradiance and dehydration using the Evaporative Flux Method (EFM). J. Vis. Exp. 70:e4179. doi: 10.3791/4179
Sack, L., and Scoffoni, C. (2013). Leaf venation: structure, function, development, evolution, ecology and applications in the past, present and future. New Phytol. 198, 983–1000. doi: 10.1111/nph.12253
Sack, L., Scoffoni, C., John, G. P., Poorter, H., Mason, C. M., Mendez-Alonzo, R., et al. (2013). How do leaf veins influence the worldwide leaf economic spectrum? Review and synthesis. J. Exp. Bot. 64, 4053–4080. doi: 10.1093/jxb/ert316
Simonin, K. A., Limm, E. B., and Dawson, T. E. (2012). Hydraulic conductance of leaves correlate with leaf lifespan: implications for lifetime carbon gain. New Phytol. 193, 939–947. doi: 10.1111/j.1469-8137.2011.04014.x
Tabassum, M. A., Ye, Y., Yu, T., Zhu, G., Rizwan, M. S., Wahid, M. A., et al. (2016). Rice (Oryza sativa L.) hydraulic conductivity links to leaf venation architecture under well-watered condition rather than PEG-induced water deficit. Acta. Physiol. Plant. 38:92. doi: 10.1007/s11738-016-2109-7
Taylaran, R. D., Adachi, S., Ookawa, T., Usuda, H., and Hirasawa, T. (2011). Hydraulic conductance as well as nitrogen accumulation plays a role in the higher rate of leaf photosynthesis of the most productive variety of rice in Japan. J. Exp. Bot. 62, 4067–4077. doi: 10.1093/jxb/err126
Terashima, I., Hanba, Y. T., Tholen, D., and Niinemets, Ü. (2011). Leaf functional anatomy in relation to photosynthesis. Plant Physiol. 155, 108–116. doi: 10.1104/pp.110.165472
Tholen, D., Boom, C., and Zhu, X. G. (2012). Opinion: Prospects for improving photosynthesis by altering leaf anatomy. Plant Sci. 197, 92–101. doi: 10.1016/j.plantsci.2012.09.005
Valentini, R., Epron, D., De Angelis, P., Matteucci, G., and Dreyer, E. (1995). In situ estimation of net CO2 assimilation, photosynthetic electron flow and photorespiration in Turkey oak (Q. cerris L.) leaves: diurnal cycles under different levels of water supply. Plant Cell Environ. 18, 631–640. doi: 10.1111/j.1365-3040.1995.tb00564.x
Venturas, M. D., Sperry, J. S., and Hacke, U. G. (2017). Plant xylem hydraulics: what we understand, current research, and future challenges. J. Integr. Plant Biol. 59, 356–389. doi: 10.1111/jipb.12534
von Caemmerer, S., Evans, J. R., Hudson, G. S., and Andrews, T. J. (1994). The kinetics of ribulose-1, 5-bisphosphate carboxylase/oxygenase in vivo inferred from measurements of photosynthesis in leaves of transgenic tobacco. Planta 195, 88–97. doi: 10.1007/BF00206296
Walls, R. L. (2011). Angiosperm leaf vein patterns are linked to leaf functions in a global scale data set. Am. J. Bot. 98, 244–253. doi: 10.3732/ajb.1000154
Wu, L., Boer, H. J., Zhang, Z., Chen, X., Shi, Y., Peng, S., et al. (2020). The coordinated increase in stomatal density and vein dimensions during genetic improvement in rice. Agron. J. 112, 2791–2804. doi: 10.1002/agj2.20180
Xiong, D., Flexas, J., Yu, T., Peng, S., and Huang, J. (2017). Leaf anatomy mediates coordination of leaf hydraulic conductance and mesophyll conductance to CO2 in Oryza. New Phytol. 213, 572–583. doi: 10.1111/nph.14186
Xiong, D., and Nadal, M. (2020). Linking water relations and hydraulics with photosynthesis. Plant J. 101, 800–815. doi: 10.1111/tpj.14595
Xiong, D., Yu, T., Zhang, T., Li, Y., Peng, S., and Huang, J. L. (2015). Leaf hydraulic conductance is coordinated with leaf morpho-anatomical traits and nitrogen status in the genus Oryza. J. Exp. Bot. 66, 741–748. doi: 10.1093/jxb/eru434
Xu, Z., and Zhou, G. (2008). Responses of leaf stomatal density to water status and its relationship with photosynthesis in a grass. J. Exp. Bot. 59, 3317–3325. doi: 10.1093/jxb/ern185
Yamori, W., Nagai, T., and Makino, A. (2011). The rate-limiting step for CO2 assimilation at different temperatures is influenced by the leaf nitrogen content in several C3 crop species. Plant Cell Environ. 34, 764–777. doi: 10.1111/j.1365-3040.2011.02280.x
Keywords: cultivated rice (Oryza sativa L.), leaf gas exchange, stomatal conductance, leaf hydraulics, leaf vein density
Citation: Ye M, Wu M, Zhang H, Zhang Z and Zhang Z (2021) High Leaf Vein Density Promotes Leaf Gas Exchange by Enhancing Leaf Hydraulic Conductance in Oryza sativa L. Plants. Front. Plant Sci. 12:693815. doi: 10.3389/fpls.2021.693815
Received: 12 April 2021; Accepted: 20 September 2021;
Published: 25 October 2021.
Edited by:
Joan Laur, Montreal Botanical Garden, CanadaReviewed by:
Elizabeth Van Volkenburgh, University of Washington, United StatesDebabrata Panda, Central University of Orissa, Koraput, India
Yusuke Mizokami, Tokyo University of Pharmacy and Life Sciences, Japan
Copyright © 2021 Ye, Wu, Zhang, Zhang and Zhang. This is an open-access article distributed under the terms of the Creative Commons Attribution License (CC BY). The use, distribution or reproduction in other forums is permitted, provided the original author(s) and the copyright owner(s) are credited and that the original publication in this journal is cited, in accordance with accepted academic practice. No use, distribution or reproduction is permitted which does not comply with these terms.
*Correspondence: Zujian Zhang, enpqQHl6dS5lZHUuY24=