- 1Department of Agriculture, Ryukoku University, Otsu, Japan
- 2Center for Ecological Research, Kyoto University, Otsu, Japan
- 3Department Agrobiological Resources, Faculty of Agriculture, Meijo University, Nagoya, Japan
It is known that undamaged plants that have been exposed to volatiles from damaged con- or heterospecific plants become more resistant against herbivores. This is one of the plants’ induced resistant responses against herbivores. To test whether this response can be used for rice production, we conducted the following experiments over 2 years (2012 and 2013). Rice seedlings were first planted in the rice seedling bed for 2 weeks in early May. There, half of the rice seedlings were exposed to artificially damaged weed volatiles three times for 12 days (treated plants). Weeds were randomly collected from the areas that were >100 m away from the seedling bed and the rice paddy fields. The remaining seedlings were not exposed (control plants). In the middle of May, bunches (ca. three seedlings per bunch) were transplanted to the rice paddy field. In July, leaf damage was observed. The total number of leaves in the treated and control plants was not significantly different. In contrast, the total number of damaged leaves in the treated plants was significantly lower than that in the control plants. In September, rice grains were harvested. The average weight of a rice grain from the treated and control plants was not significantly different. However, the weight of grains per bunch of treated plants was significantly higher than that of control plants; this indicated a significant increase of the number of grains by 23% in 2012 and by 18% in 2013 in the treated plants compared to that in the control plants. The volatiles emitted from the weeds included monoterpenoids (40.4% in total), green leaf volatiles (46.5%), short-chain alcohols (5.3%), short-chain ketone (5.4%), short-chain acetate (0.5%), short-chain aldehyde (1.1%), and hydrocarbon (0.7%). These results suggest that exposure of volatiles from artificially damaged weeds to rice seedlings has the potential to increase rice production.
Introduction
In response to damages caused by herbivorous arthropods, plants start emitting herbivory-induced plant volatiles (HIPVs) (Takabayashi and Shiojiri, 2019). When uninfested conspecific plants received HIPVs, they become more defensive against herbivores [for review see Karban et al. (2014) and Yoneya and Takabayashi (2016)]. Besides responding to HIPVs, plants also respond to volatiles from artificially damaged plants. These phenomena are called “priming of plant resistant by plant volatiles.” Under laboratory conditions, green leaf volatiles (GLVs), which are one of the common volatiles emitted from herbivore-damaged and artificially damaged plants, have been shown to induce resistant responses in Arabidopsis thaliana, Citrus jambhiri (rough lemon), Zea mays (corn), and Nicotiana attenuata (wild tobacco) (Bate and Rothstein, 1998; Gomi et al., 2003; Engelberth et al., 2004; Farag et al., 2005; Kishimoto et al., 2005, 2006; Paschold et al., 2006; Yamauchi et al., 2018). When undamaged pyrethrum plants (Chrysanthemum cinerariifolium) were exposed to a blend of volatiles (GLVs and a sesquiterpene) emitted from artificially damaged conspecific leaves, the amount of pyrethrin in the exposed plants increased significantly compared to that in plants that were not exposed (Kikuta et al., 2011). Under field conditions, sagebrush plants (Artemisia tridentata) exposed to volatiles from artificially damaged conspecific plants suffered less damage compared to unexposed conspecifics (Karban et al., 2006). The priming is also observed between heterospecific plants (Farmer and Ryan, 1990; Oudejans and Bruin, 1995; Karban et al., 2003). Under laboratory conditions, exposure of undamaged cucumber plants (Cucumis sativus) to HIPVs from spider mite-infested Lima bean (Phaseolus lunatus) plants resulted in the attraction of predatory mites that preyed on spider mites (Oudejans and Bruin, 1995). Under field conditions, Karban et al. (2003) showed an induced resistance in tobacco when sagebrush neighbors were clipped either with scissors or damaged with herbivores.
Weeding is a common practice in agricultural fields. During this practice, a large amount of artificially damaged weed volatiles is spread over the surrounding areas, including agricultural fields, thus exposing the surrounding crops to artificially damaged weed volatiles. After weeding, the exposed crops are expected to be more resistant than non-exposed crops to herbivores (priming of plant resistance by volatiles from heterospecific plants). Recently, this possibility was demonstrated in field-grown black soybeans and yellow soybeans (Glycine max); when young soybean plants were exposed to artificially damaged goldenrod volatiles, both plants and their grains became more resistant against herbivores (Shiojiri et al., 2017, 2020).
Sticky rice (Oryza sativa subsp. japonica) is an important food source worldwide. In Japan, weeding around rice paddy fields is conducted in spring to remove Gramineae weeds, which host many insect pests of rice plants. This weeding results in the rice seedlings being exposed to weeding-related volatiles. In the present study, we investigated whether such exposure affected rice grain production in pesticide-free rice paddy fields. We discussed the potential of using weeding-related volatiles in yield control in rice production.
Materials and Methods
Experimental Conditions
Field experiments were carried out during 2012 and 2013 in pesticide-free commercial rice paddy fields owned by a farmer in Makino, Shiga, Japan. Rice plants (O. sativa subsp. japonica var. Koshihikari) were cultivated in eight paddy fields. Approximately 15,000 rice bunches (2–3 plants per bunch) were planted in each paddy field (20 m × 50 m). Four paddy fields (A, B, C, and D) were set together, while the other four (E, F, G, and H) were set at another site; the two sites were located ca. 200 m away from each other. At each site, we established treatment and control fields. A seedling bed (1 m × 15 m) was used to grow rice seedlings before transplanting them to the paddy fields.
Odor Sources
Several species of weeds growing in areas that were >100 m away from the rice paddy fields and from the rice seedling bed were used as odor sources. In both years, we observed weeds belonging to more than six families (including Asteraceae, Equisetaceae, Caryophyllaceae, Fabaceae, Poaceae, and others) and identified the following four major species: Picris hieracioides var. glabrescens (Asteraceae; Cichorieae), Artemisia indica Willd. var. maximowiczii (Asteraceae), Equisetum arvense (Equisetaceae), and Trifolium repens (Fabaceae; Trifolieae). We randomly collected weeds (5 kg) in the areas and divided them equally into ten portions. Each portion was placed in a mesh bag (45 cm × 35 cm). The composition of weed species used for the experiments was the same during the 2-year experiments. The used weeds were perennial plants and no herbicides were used in two consecutive years.
Experimental Procedures
To facilitate the exposure experiments, we conducted experiments in a rice seedling bed, because plant defenses for future growth are often developed at the seedling stage (Barton and Koricheva, 2010). We divided the seedling bed into two parts (the control area and the exposed area) with a polycarbonate board (ca. 1 m high and 2 m long) to prevent weed volatiles from reaching the untreated seedlings. When the seedlings in the bed were ca. 3–5 cm high, half of them (ca. 150,000 seedlings) were exposed to weeding-related volatiles. Ten mesh bags containing cut weeds (ca. 500 g per bag) were hung equidistantly in the exposed area of the seedling bed. The cut weeds were replaced with new ones every 4 days for 12 days. In 2012, after the exposure, the treated and control rice seedlings (ca. 10 cm high) were transplanted into rice paddy fields: the exposed seedlings were planted in A and H fields, and the control seedlings were placed in D and E fields. B, C, F, and G fields were used for other experiments. In 2013, the exposed seedlings were placed in B, D, E, and G fields, and the control seedlings were placed in A, C, F, and H fields.
Approximately 50 days after the transplantation, we collected the leaves from 15 treated and 15 control rice bunches randomly selected [except for field B (treated) in 2013, N = 14] to evaluate the number of damaged leaves on each paddy field.
In the rice paddy field used in this study, we observed commonly found herbivorous arthropods, such as rice leaf beetles (Oulema oryzae), rice leaf folders (Cnaphalocrocis medinalis), rice green caterpillars (Naranga aenescens), and Japanese grasshoppers (Oxya yezoensis). Rice leaf beetles and rice leaf folders made similar fed-edge leaving leaf veins after herbivory. Rice green caterpillars and Japanese grasshoppers made different fed-edge because they did not leave leaf veins. In the field observation, we could not identify the herbivore species based on the fed-edges on damaged leaves. The two types of fed-edges were evaluated together as damaged leaves.
After harvest, we measured the weight of rice grains from 15 treated, and 15 control rice bunches randomly selected. We weighted 100 grains per bunch. Because each bunch had approximately 8,000–10,000 grains, we were unable to count the number of grains per bunches on site. The detailed procedure of the experiments is presented in Supplementary Table 1.
Chemical Analysis
On May 12, 2013, in order to analyze the chemical structure of weeding-related volatiles, we used ca. 100 g of cut weeds per mesh bag from five randomly selected mesh bags that were used for the exposure experiments. After volatile collection, we returned them to the mesh bag. We placed the samples in polyethylene terephthalate (PET) bags (180 mm × 250 mm) (Mitsubishi Gas Chemical Company, Inc., Tokyo, Japan). To collect volatiles, we sent air (100 mL/min airflow) into each PET bag containing weeds. At the outlet of each bag, we set a tube containing Tenax-TA (3.0 mm internal diameter, 160 mm long with 100 mg, GL Sciences Inc., Tokyo, Japan) to collect volatiles for 1 h. We analyzed the collected volatiles by gas chromatography/mass spectrometry (GC/MS) (GC: Agilent 6890 with an HP-5MS capillary column: 30 m long, 0.25-mm i.d., and 0.25-μm film thickness; MS: Agilent 5973 mass selective detector, 70 eV with helium as the carrier gas; Agilent, Santa Clara, CA, United States) equipped with a thermal desorption cold trap injector (TCT; CP4010; Chrompack, Middelburg, Netherlands). We programmed the oven temperature of the gas chromatograph to increase from 40°C (5 min hold) to 280°C at 15°C/min. We identified the compounds by comparing their mass spectra with those of compounds from the Wiley7N database (John Wiley & Sons Inc., Hoboken, NJ, United States) and their retention times and mass spectra with those of synthetic compounds.
Statistical Analyses
The effects of treatment, year, and their interaction on the number of leaves and grain weight per bunch were analyzed with the least square mixed model after Box-Cox transformation in JMP version 14.2.0 (SAS Institute, 2018). The rice paddy field was a random effect in all models. The weight of 100 grains was analyzed with a t-test in JMP version 14.2.0.
Results
Leaf Damage
We found that the treatment and the interaction of treatment × year did not significantly influence the total number of leaves per bunch (treatment: df = 1, 159.6, F = 1.0171, P = 0.3147; interaction: df = 1, 7.138, F = 0.0777, P = 0.7884), whereas the year had highly significant influence on the total number of leaves per bunch (df = 1, 159.6, F = 15.2639, P = 0.0001) (Figure 1A). In contrast, we found that the treatment and year had significant influence on the number of damaged leaves per bunch (treatment: df = 1, 173.2, F = 4.008, P = 0.0468, year: df = 1, 173.2, F = 13.3238, P = 0.0003), whereas their interaction did not (df = 1, 6.763, F = 0.0354, P = 0.8564). These results indicated that over 2 years, the number of damaged leaves was significantly lower in the exposed rice plants than in the control rice plants (Figure 1). The relative decrease of the damaged leaves in the exposed plants compared to that in control plants of respective year was 11.1% in 2012 and 9.8% in 2013.
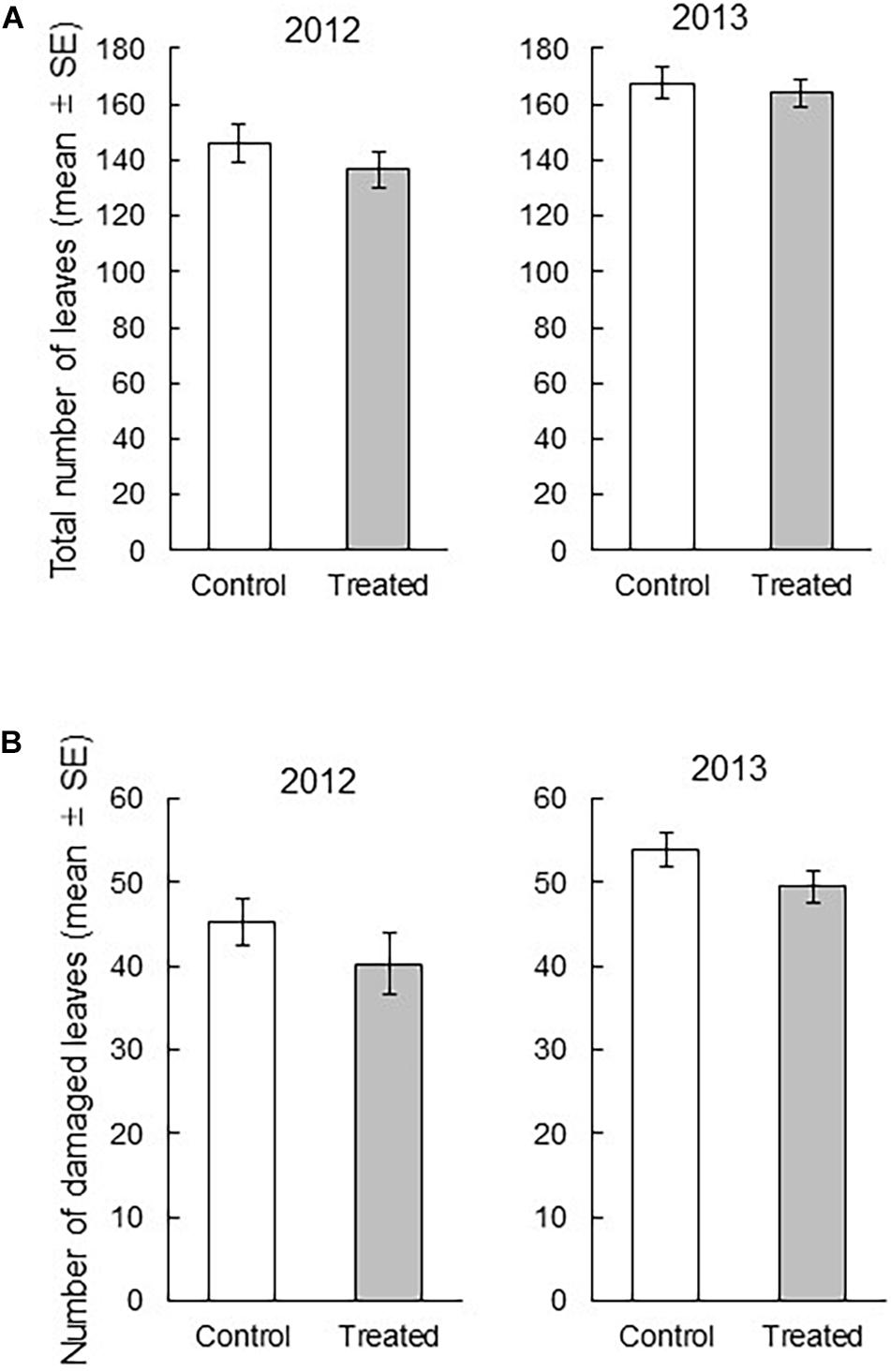
Figure 1. (A) Comparison between the total number of leaves in a treated bunch and that in a control bunch (treatment P = 0.3147, year P = 0.0001, treatment × year P = 0.7884), and (B) the comparison between the total number of damaged leaves in a treated bunch and that in a control bunch (treatment P = 0.0468, year P = 0.0003, treatment × year P = 0.8564). The effects of treatment, year, and their interaction on the number of leaves were analyzed with the least square mixed model after Box-Cox transformation. N = 15, except for the data of one field in 2013 (N = 14).
Grain Weight
We found that the treatment and year had significant influence on the grain weight per bunch (treatment: df = 1, 174, F = 7.3799, P = 0.0073, year: df = 1, 174, F = 16.2277, P < 0.0001), whereas their interaction did not (df = 1, 5.838, F = 0.0131, P = 0.9126) (Figure 2). In addition, we measured the weight of 100 grains to obtain the weight of one rice grain. In both years, the weight of a grain from treated bunches (mean ± SE) was not significantly different from that from the control bunches (2012: the control bunch = 2.683 ± 0.026; the treated bunch = 2.659 ± 0.028, P = 0.476; 2013: the control bunch = 2.684 ± 0.024; the treated bunch = 2.633 ± 0.033, P = 0.251). These results indicated that over 2 years, the weight of rice grains per bunch was significantly higher in the treated rice plants than in the control rice plants. The increase in grain weight per bunch was explained by the increase of the number of grains. The relative increase of the number of grains in the exposed plants were 23% in 2012 and 15.9% in 2013 compared to that in control rice plants of respective year.
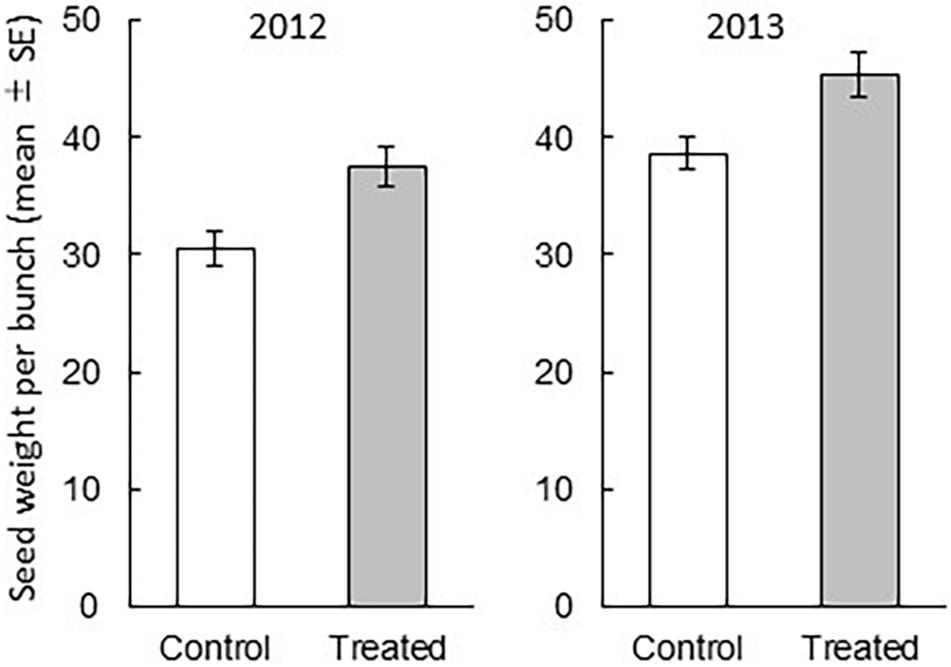
Figure 2. Comparison between the rice grain weight (mean ± SE) from a treated bunch and that from a control bunch (treatment P = 0.0073, year P < 0.0001, treatment × year P = 0.9126). The effects of treatment, year, and their interaction on grain weight per bunch were analyzed with the least square mixed model after Box-Cox transformation. N = 15, except for the data of one field in 2013 (N = 14).
Chemical Analyses
The headspace of volatiles emitted from the five odor sources was analyzed separately. We found 26 compounds in all the odor sources (Table 1). The compounds were classified into monoterpenoids (12 compounds, 40.4% in total), green leaf volatiles (eight compounds, 46.5%), short-chain (C5) alcohols (two compounds, 5.3%), short-chain (C5) ketone (5.4%), short-chain (C5) acetate (0.5%), short-chain (C9) aldehyde (1.1%), and hydrocarbon (0.7%). The major compounds were (Z)-3-hexen-1-ol, (Z)-3-hexenyl acetate, α-pinene, camphene, β-pinene, β-myrcene, 1,8-cyneole, and pentene-3-one.
Discussion
Artificially damaged weeds set in the seedling bed emitted 26 volatile compounds, including green leaf volatiles (GLVs), monoterpenoids, short-chain (C5) alcohol/ketone/acetate, a short-chain (C9) aldehyde, and a hydrocarbon (Table 1). The exposure of rice seedlings to these volatiles resulted in a significant increase in the number of rice grains (23% increase in 2012 and 18% increase in 2013 compared to that in control rice plants). It is important to note that the exposure was only three times with an interval of 4 days at the seedling stage (from ca. 5 cm to ca. 10 cm plant height), indicating that the exposure at the rice seedling stage affected the rice grain production.
One of the possible reasons for the increase would be the reduction of the leaf damages in the exposed plants. Although the damaged leaves of the exposed rice plants were significantly lower than that of the control rice plants, the differences were relatively small (11.1% reduction in 2012 and 9.8% reduction in 2013). In this study, we did not evaluate the behavior of the herbivorous insects observed in the rice paddy fields. The mechanisms involved in the reduction of the damage made by herbivores insects remains necessary.
The differences in the rice grain production between the control and treated plants might also be explained by other factors, such as effects of fungi/viruses, growth, nutrition intake, photosynthesis, which might be affected by the volatiles either positively or negatively. As we did not find disease symptoms on leaves, and the farmer did not mention to us disease problems during the experimental periods, the involvement of fungi and viruses was unlikely. Whether other factors were involved in the increase of performance in rice plants is worth evaluating, as previous studies showed that volatiles affect plant physiology. (E)-2-Hexenal acts as signal chemicals that strongly induce the gene expression of abiotic-related transcription factors in Arabidopsis (Yamauchi et al., 2015). Inhibitory effects on the growth of volatile compounds have been reported in Arabidopsis, for example, on root length in Arabidopsis (Bate and Rothstein, 1998; Mirabella et al., 2008; Scala et al., 2017). Horiuchi et al. (2007) also reported that monoterpenoids, borneol, and bornyl acetate reduced the root length of Arabidopsis.
The origins and the principal compounds involved in the increased rice grain production remained unknown. Among them, GLVs are commonly found in green plants whose leaves underwent mechanical damage. GLVs have been reported as signaling molecules in the elicitation of priming in several plant species (Bate and Rothstein, 1998; Gomi et al., 2003; Engelberth et al., 2004; Farag et al., 2005; Kishimoto et al., 2005, 2006; Paschold et al., 2006; Yamauchi et al., 2018). Volatile terpenoids are also involved in the priming. Soybean plants previously exposed to a blend of α-pinene, β-myrcene, and limonene became more defensive against common cutworms (Spodoptera litura larvae) (Shiojiri et al., 2017). 1.8-Cineol, constitutively released from mint plants (Mentha × piperita), was suggested to be one of the signaling molecules that induce resistant responses in soybean plants (Sukegawa et al., 2018). Riedlmeier et al. (2017) reported that a mixture of α-pinene and β-pinene induced resistant responses in Arabidopsis.
Detailed studies on the relationship between the effects of the volatile exposure to rice seedlings and the increased production of rice grains, especially focusing on the mechanism involved in the long-lasting effects, are needed. The effects of the timing of the exposure (i.e., which growth stages are most effective) should also be considered. Further, for achieving an effective exposure-related increase of rice grain production, it is worthwhile to evaluate whether specific weed groups (specific families or genera) are needed for the exposure, or if any weeds in the areas surrounding rice paddy fields can be used for the exposure. Thus, our findings suggest that exposure of volatiles from mechanically damaged weeds to rice seedlings has the potential to increase rice production.
Data Availability Statement
The original contributions presented in the study are included in the article/Supplementary Material, further inquiries can be directed to the corresponding author/s.
Author Contributions
KS designed and conducted the field experiments. RO conducted the field experiments and the chemical analyses. MU conducted the statistical analyses. KS, RO, MU, and JT analyzed the data and wrote the manuscript. All authors gave final approval of the manuscript for publication.
Funding
This study was in part supported by grants for the scientific research (A) (grant number 18H03952) from the Ministry of Education, Culture, Sports, Science and Technology, and in part by the Research Institute for Food and Agriculture of Ryukoku University (grant number FA1906).
Conflict of Interest
The authors declare that the research was conducted in the absence of any commercial or financial relationships that could be construed as a potential conflict of interest.
Acknowledgments
We would like to thank Asato Fukui who kindly allowed us to use his rice paddy fields for experiments, and Yoshitaka Nakashima, Kaoru Tsuji, Eizi Yano for their comments on the data. We would also like to thank Kikumi Katami and Ayako Masaoka for helping to collect data. This study was partly supported by Center for Ecological Research, Kyoto University, a Joint Usage/Research Center.
Supplementary Material
The Supplementary Material for this article can be found online at: https://www.frontiersin.org/articles/10.3389/fpls.2021.692924/full#supplementary-material
References
Barton, K. E., and Koricheva, J. (2010). The ontogeny of plant defense and herbivory: characterizing general patterns using meta-analysis. Am. Nat. 175, 481–493. doi: 10.1086/650722
Bate, N. J., and Rothstein, S. J. (1998). C6-volatiles derived from the lipoxygenase pathway induce a subset of defense-related genes. Plant J. 16, 561–569. doi: 10.1046/j.1365-313x.1998.00324.x
Engelberth, J., Alborn, H. T., Schmelz, E. A., and Tumlinson, J. H. (2004). Airborne signals prime plants against insect herbivore attack. Proc. Natl. Acad. Sci. U. S. A. 101, 1781–1785. doi: 10.1073/pnas.0308037100
Farag, M. A., Fokar, M., Abd, H., Zhang, H., Allen, R. D., and Pareì, P. W. (2005). (Z)-3-Hexenol induces defense genes and downstream metabolites in maize. Planta 220, 900–909. doi: 10.1007/s00425-004-1404-5
Farmer, E. E., and Ryan, C. A. (1990). Interplant communication: airborne methyl jasmonate induces synthesis of proteinase inhibitors in plant leaves. Proc. Natl Acad. Sci. U. S. A. 87, 7713–7716. doi: 10.1073/pnas.87.19.7713
Gomi, K., Yamasaki, Y., Yamamoto, H., and Akimitsu, K. (2003). Characterization of a hydroperoxide lyase gene and effect of C6-volatiles on expression of genes of the oxylipin metabolism in Citrus. J. Plant Physiol. 160, 1219–1231. doi: 10.1078/0176-1617-01177
Horiuchi, J., Muroi, A., Takabayashi, J., and Nishioka, T. (2007). Exposing Arabidopsis seedlings to borneol and bornyl acetate affects root growth: specificity due to the chemical and optical structures of the compounds. J. Plant Interact. 2, 101–104. doi: 10.1080/17429140701575624
Karban, K., Shiojiri, K., Huntzinger, M., and Mccall, A. C. (2006). Damage-induced resistance in sagebrush: volatiles are key to intra- and interplant communication. Ecology 87, 922–930. doi: 10.1890/0012-9658(2006)87[922:drisva]2.0.co;2
Karban, R., Maron, J., Felton, G. W., Ervin, G., and Eichenseer, H. (2003). Herbivore damage to sagebrush induces resistance in wild tobacco: evidence for eavesdropping between plants. Oikos 100, 325–332. doi: 10.1034/j.1600-0706.2003.12075.x
Karban, R., Yang, L. H., and Edwards, K. F. (2014). Volatile communication between plants that affects herbivory: a meta-analysis. Ecol. Lett. 17, 44–52. doi: 10.1111/ele.12205
Kikuta, Y., Ueda, H., Nakayama, K., Katsuda, Y., Ozawa, R., Takabayashi, J., et al. (2011). Specific regulation of pyrethrin biosynthesis in Chrysanthemum cinerariaefolium by a blend of volatiles emitted from artificially damaged conspecific plants. Plant Cell Physiol. 52, 588–596. doi: 10.1093/pcp/pcr017
Kishimoto, K., Matsui, K., Ozawa, R., and Takabayashi, J. (2005). Volatile C6-aldehydes and allo-ocimene activate defense genes and induce resistance against Botrytis cinerea in Arabidopsis thaliana. Plant Cell Physiol. 46, 1093–1102. doi: 10.1093/pcp/pci122
Kishimoto, K., Matsui, K., Ozawa, R., and Takabayashi, J. (2006). Components of C6-aldehyde-induced resistance in Arabidopsis thaliana against a necrotrophic fungal pathogen, Botrysis cinerea. Plant Sci. 170, 715–723. doi: 10.1016/j.plantsci.2005.11.002
Mirabella, R., Rauwerda, H., Struys, E. A., Jakobs, C., Triantaphylides, C., Haring, M. A., et al. (2008). The Arabidopsis her1 mutant implicates GABA in E-2-hexenal responsiveness. Plant J. 53, 197–213. doi: 10.1111/j.1365-313X.2007.03323.x
Oudejans, A. M. C., and Bruin, J. (1995). Does spider-mite damage induce information transfer between plants of different species? Med. Fac. Landbouww. Univ. Gent. 59, 733–739.
Paschold, A., Halitschke, R., and Baldwin, I. T. (2006). Using ‘mute’ plants to translate volatile signals. Plant J. 45, 275–291. doi: 10.1111/j.1365-313X.2005.02623.x
Riedlmeier, M., Ghirardo, A., Wenig, M., Knappe, C., Kerstin, K., Georgii, E., et al. (2017). Monoterpenes support systemic acquired resistance within and between plants. Plant Cell 29, 1440–1459. doi: 10.1105/tpc.16.00898
Scala, A., Mirabella, R., Goedhart, J., de Vries, M., Haring, M. A., and Schuurink, R. C. (2017). Forward genetic screens identify a role for the mitochondrial HER2 in E-2-hexenal responsiveness. Plant Mol. Biol. 95, 399–409. doi: 10.1007/s11103-017-0659-8
Shiojiri, K., Ozawa, R., Yamashita, K., Uefune, M., Matsui, K., Tsukamoto, C., et al. (2017). Weeding volatiles reduce leaf and seed damage to field-grown soybeans and increase seed isoflavones. Sci. Rep. 7:41508. doi: 10.1038/srep41508
Shiojiri, K., Ozawa, R., Yamashita, K., Uefune, M., Matsui, K., Tsukamoto, C., et al. (2020). Exposure to artificially damaged goldenrod volatiles increases saponins in seeds of field-grown soybean plants. Phytochem. Lett. 36, 7–10. doi: 10.1016/j.phytol.2020.01.014
Sukegawa, S., Shiojiri, K., Higami, T., Suzuki, S., and Arimura, G. (2018). Pest management using mit volatiles to elicit resistance in soy: mechanism and application potential. Plant J. 96, 910–920. doi: 10.1111/tpj.14077
Takabayashi, J., and Shiojiri, K. (2019). Multifunctionality of herbivory-induced plant volatiles in chemical communication in tritrophic interactions. Curr. Opin. Insect Sci. 32, 110–117.
Yamauchi, Y., Kunishima, M., Mizutani, M., and Sugimoto, Y. (2015). Reactive short-chain leaf volatiles act as powerful inducers of abiotic stress-related gene expression. Sci. Rep. 5:8030. doi: 10.1038/srep08030
Yamauchi, Y., Matsuda, A., Matuura, N., Mizutani, M., and Sugimoto, Y. (2018). Transcriptome analysis of Arabidopsis thaliana treated with green leaf volatiles: possible role of green leaf volatiles as self-made damage-associated molecular patterns. J. Pestic. Sci. 43, 207–213. doi: 10.1584/jpestics.D18-020
Keywords: rice, artificially damaged plant volatiles, weeding, green leaf volatiles, terpenoids, Oryza sativa subsp. japonica
Citation: Shiojiri K, Ozawa R, Uefune M and Takabayashi J (2021) Field-Grown Rice Plants Become More Productive When Exposed to Artificially Damaged Weed Volatiles at the Seedling Stage. Front. Plant Sci. 12:692924. doi: 10.3389/fpls.2021.692924
Received: 09 April 2021; Accepted: 18 June 2021;
Published: 12 July 2021.
Edited by:
Islam S. Sobhy, Keele University, United KingdomReviewed by:
Velemir Ninkovic, Swedish University of Agricultural Sciences, SwedenJurgen Engelberth, University of Texas at San Antonio, United States
Foteini Paschalidou, Institut National de la Recherche Agronomique (INRA), France
Copyright © 2021 Shiojiri, Ozawa, Uefune and Takabayashi. This is an open-access article distributed under the terms of the Creative Commons Attribution License (CC BY). The use, distribution or reproduction in other forums is permitted, provided the original author(s) and the copyright owner(s) are credited and that the original publication in this journal is cited, in accordance with accepted academic practice. No use, distribution or reproduction is permitted which does not comply with these terms.
*Correspondence: Kaori Shiojiri, a2Fvcmkuc2hpb2ppcmlAYWdyLnJ5dWtva3UuYWMuanA=; Junji Takabayashi, dGFrYWJheWFzaGkuanVuamkuNGFAa3lvdG8tdS5hYy5qcA==