- 1State Key Laboratory of Tree Genetics and Breeding, Research Institute of Forestry, Chinese Academy of Forestry, Beijing, China
- 2Co-innovation Center for Sustainable Forestry in Southern China, Nanjing Forestry University, Nanjing, China
- 3Key Laboratory of Plant Molecular Physiology, Institute of Botany, Chinese Academy of Sciences, Beijing, China
Rab GTPases are the subfamily of the small guanosine triphosphate (GTP)-binding proteins which participated in the regulation of various biological processes. Recent studies have found that plant Rabs play some specific functions. However, the functions of Rabs in xylem development in trees remain unclear. In this study, functional identification of PagRabE1b in Populus was performed. Quantitative reverse transcription PCR (qRT-PCR) results showed that PagRabE1b was highly accumulated in stems, especially in phloem and xylem tissues. Overexpression of PagRabE1b in poplar enhanced programmed cell death (PCD) and increased the growth rate and the secondary cell wall (SCW) thickness. Quantitative analysis of monosaccharide content showed that various monosaccharides were significantly increased in secondary xylem tissues of the overexpressed lines. Flow cytometry analysis revealed that the number of apoptotic cells in PagRabE1b-OE lines is more than a wild type (WT), which indicated that PagRabE1b may play an important role in PCD. Further studies showed that overexpression of PagRabE1b increased the expression level of genes involved in SCW biosynthesis, PCD, and autophagy. Collectively, the results suggest that PagRabE1b plays a positive role in promoting the xylem development of poplar.
Introduction
Trees are the most abundant natural sources important for sustainable energy and the sinks of atmospheric carbon dioxide (Zhang et al., 2015; Zhong and Ye, 2015). Wood biomass is mainly composed of the secondary cell wall (SCW) and is widely used in many applications, such as house construction, biofuels, pulping, and paper-making. Because of the substantial economic value of wood, elucidating the molecular regulatory mechanism of wood formation will be useful for the manipulation of wood quality and quantity through molecular breeding.
The development of SCW is a complicated procedure requiring synchronization of several regulatory and metabolic pathways. Previous studies have revealed that the SCW biosynthesis is mainly regulated by transcriptional regulatory networks of NAC and MYB transcription factor (TF) families (Zhong and Ye, 2015). During SCW formation, several NAC TFs are considered as master switches and in the top layer of the SCW regulatory network (Xie et al., 2018). A series of additional TFs, such as MYB46 and MBY83, belong to the second layer of a transcriptional network of the SCW formation. In addition, several other MYB TFs in the third layer have been identified as direct targets of MYB46/83 (Zhang et al., 2018a); most of these MYB members positively regulate SCW biosynthesis. SCWs mainly consist of cellulose, hemicelluloses, and lignin. Cellulose taking about 40–50% of wood components is glucose polymers. Hemicelluloses constitute a major part of lignocellulosic biomass, mainly including xyloglucans, xylans, mannans, glucomannans, and β-(1→ 3, 1→ 4)-glucans (Scheller and Ulvskov, 2010). Lignin is a complex three-dimensional polyphenolic polymer of p-hydroxyphenyl (H), guaiacyl (G), and syringyl (S) lignins (Boerjan et al., 2003). Monolignol biosynthesis is carried out through the common phenylpropanoid pathway, and several central genes involved in lignin synthesis during secondary wall formation in Populus had been well-identified (Wang et al., 2018). Finally, the lignified vessel and fiber cells undergo programmed cell death (PCD) for complete lysis of cell content in the maturation of xylem cells (Courtois-Moreau et al., 2009).
Small GTPases also play important roles in the regulation of cell wall development by monitoring cytoskeletal arrangement and membrane trafficking (Oda and Fukuda, 2014). In the eukaryotic, the Ras subfamily of small GTPases comprises five families: Ras, Rab, Ran, Arf, and Rho (Hall, 1998). Rho and Rab of plants play critical roles in the development of primary and SCWs (Oda and Fukuda, 2014). ROPGEF4 and ROPGAP3 mediate Rho GTPase ROP11 to originate the fundamental patterning of SCWs in xylem cells, and then ROP11 interacts withMIDD1 to provoke local depolymerization of cortical microtubules (Oda and Fukuda, 2012). In Arabidopsis, a boundary of the ROP domain (BDR1) and Wallin (WAL) complex regulates cell wall development. BDR1 could recruit WAL to the plasma membrane and then regulate an ROP-act in a pathway to shape pit boundaries (Sugiyama et al., 2019). Rab GTPase has also been shown to play important roles in SCW deposition. Overexpression of a constitutively active mutant of RabG3b (RabG3bCA) stimulated both autophagy and tracheary element formation in Arabidopsis (Kwon et al., 2010) and increased xylem growth due to the stimulation of autophagy during xylem development in Populus (Kwon et al., 2011).
The Rab family in plants is categorized into eight subfamilies (RabA-RabH) (Vernoud et al., 2003). We found out that there was a total of 67 PtRab genes in Populus trichocarpa and were grouped into eight subfamilies (Zhang et al., 2018b). Most of the PtRab genes were preferentially expressed in phloem and xylem. During the development of the poplar stem, the majority of PtRabs were preferentially expressed in the transition region from primary growth to secondary growth. These results suggested that the PtRab genes might participate in the biological processes related to xylem development. In this study, we explored the function of PagRabE1b in xylem development using transgenics overexpressing constitutively active mutant and native PagRabE1b, which revealed that PagRabE1b influenced both SCW biosynthesis and final PCD during wood formation.
Materials and Methods
Plant Materials and Growth Conditions
Hybrid poplar (Populus alba × Populus glandulosa, Pag) clone 84 K was used for gene transformation. Plants were cultivated for 4 weeks under in vitro conditions and then transplanted to the soil and grown for 12 weeks in a greenhouse (16-h light: 8-h dark, 25°C: 20°C, with relative humidity in the range 50–60%).
Vector Construction and Plant Transformation
PagRabE1b and PagRabE1b (Q74L) (point mutation of Q74 to L in motif 2, a constitutively active form of PagRabE1b) genes were cloned into the pMDC32 plant expression vector and transformed into poplars previously (Zhang et al., 2018b). Two overexpression lines of PagRabE1b and PagRabE1b (Q74L) with high expression levels were selected and named as OE-1 and OE-9 for PagRabE1b and QL-8 and QL-13 for PtRabE1b (Q74L), respectively.
Histological Analyses
The basal stems of wild type (WT), OE-1, OE-9, QL-8, and QL-13 plants were sectioned for histology using a vibratome (VT1000S; Leica, Wetzlar, Germany) to a thickness of 50 μm. Then, the sections were stained with 0.05% toluidine blue (TBO) for 60 s at room temperature, rinsed three times in water, and photographed with an Olympus BX51 microscope (Du et al., 2020).
Wall Thickness Measurement
Secondary cell wall thickness was observed by using confocal laser scanning microscopy (CLSM) and transmission electron microscopy (TEM). The 15th internodes of 2-month-old WT and PagRabE1b transgenic plants were sectioned using a vibratome at a thickness of 50 μm. CLSM pictures were captured with an LSM880 microscopy (Zeiss) using 488-nm laser excitation (5% power). TEM observation was implemented as described by Zhao et al. (2020). The 20th internodes from 3-month-old WT and transgenic plants were cut into 2-mm pieces, which were fixed using 2.5% paraformaldehyde and 0.5% glutaraldehyde in PBS (0.1 M, pH 7.4) with vacuum infiltration. The pieces were then washed three times with 0.1 M PBS and further fixed in 1% osmic acid for 2 h at room temperature. Ultrathin sections were made and photographed using an HT-7700 microscope (Hitachi) and a Gatan ORIUSTM SC1000CCD Camera (Gatan Inc., USA). The cell wall thickness of at least 100 cells from three individual plants of each line was measured using ImageJ software and analyzed statistically using Student's t-test.
Analysis of SCW Composition
The 10th to 20th internodes of 3-month-old WT and PagRabE1b transgenic plants were collected and freeze-dried at −60°C on a vacuum freeze. The dried stems were powdered by ball milling and used to measure alcohol-insoluble residues according to Zhao et al. (2020). The monosaccharide content was analyzed through GC-MS (Agilent, Santa Clara, CA, USA). Three biological replicates were analyzed for each line.
Programmed Cell Death Analysis of Stem-Differentiating Xylem Protoplasts
The Populus protoplasts from SDX isolation were carried out as previously described by Lin et al. (2013) and Wang et al. (2020) with minor modifications. Briefly, the debarked stem segments of 3-month-old WT and PagRabE1b transgenic poplars were incubated in a cell wall digestion enzyme solution [1.5% (wt/vol) Cellulase R-10 and 0.4% (wt/vol) pectolyase Y-23 in 20-mM MES,0.6-M mannitol and 20-mM KCl solution, 10-mM CaCl2, and 0.1% (wt/vol) BSA, pH 5.7] for 40 min in the dark at room temperature. Protoplasts were filtered through a 70-μm cell strainer and spun down at 150 × g for 5 min. Protoplasts were resuspended in W5 solution (2-mM MES, pH 5.7, 125-mM CaCl2, 154-mM NaCl, 0.1-M glucose, and 5-mM KCl). The isolated protoplasts were resuspended in a 195-μl prediluted binding buffer, and 5-μl Annexin V-FITC solution (Solarbio, Beijing, China) was added, mixed, and incubated for 30 min at room temperature, and then added 1 μl of the 20-μg ml−1 propidium iodide (PI) storage solution. After the addition of another 300-μl binding buffer, the suspended protoplasts were analyzed using a BD Aria SORP cell sorter (BD Biosciences, USA) with 488-nm excitation for FITC and 530 nm for PI. Three independent sets of experiments were performed.
Gene Expression Analysis
The apex, stems, and roots were harvested from 8-week-old poplars and were sampled for quantitative reverse transcription PCR (qRT-PCR). For sampling, the xylem and phloem–cambium tissues from stems of 8-week-old poplar were debarked and scraped with a sterile double-edged razor blade from the outer of the debarked stem or the inner surface of the peeled bark. To examine the expression levels of marker genes involved in xylem development by qRT-PCR, 2-month-old WT and PagRabE1b transgenic plants were debarked, and developing xylems were scraped. Total RNA was extracted from all the samples using the RNeasy Plant Mini Kit (Qiagen, Hilden, Germany) and genomic DNA was eliminated with the on-column treatment of RNase-free DNase I (Qiagen, Hilden, Germany). First-strand complementary DNA (cDNA) was synthesized with about 1-μg RNA using the SuperScript III reverse transcription kit (Invitrogen) according to the instruction of the manufacturer. Primers with annealing temperatures of 58–60°C and amplification lengths of 100–250 bp were designed using Primer3 software (http://primer3.ut.ee/). All primers were listed in Supplementary Table 1. qRT-PCR was carried out using KAPA SYBR FAST qPCR master mixture on Roche LightCycler 480 (Roche Applied Science, Penzberg, Upper Bavaria, Germany) according to the instructions of the manufacturer. All experiments were performed in three biological replicates and three technical replicates. The PagActin gene (Potri.001G309500) was used as the internal control (Wang et al., 2016; Zhao et al., 2020).
Results
Expression Pattern of PagRabE1b
To investigate the expression pattern of PagRabE1b, qRT-PCR was used to analyze the gene expression in tissues collected from the apex, stems, and roots. The results revealed that PagRabE1b expression could be detected in all the tissues and was high in xylem and phloem–cambium (Figure 1A). A similar expression pattern was also observed by analysis of the AspWood database (http://aspwood.popgenie.org) (Sundell et al., 2017), which showed that PagRabE1b is highly expressed in expanding xylem (Figure 1B). These results suggested a potential role of PagRabE1b in xylem development in poplar.
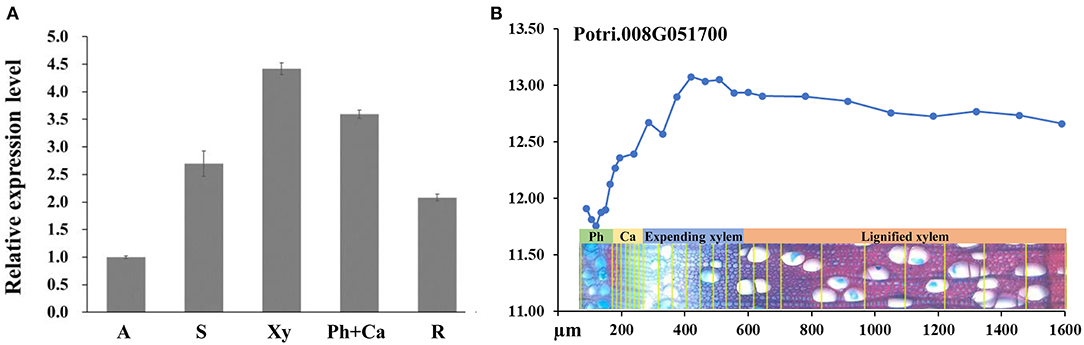
Figure 1. Expression patterns of PagRabE1b in poplar. (A) Quantitative reverse transcription PCR (qRT-PCR) was carried out to analyze the gene expression levels of PagRabE1b indifferent tissues of poplar. The actin gene was used as an internal control. (B) Expression profiles of PagRabE1b among developing phloem, cambium, and developing xylem. Data were derived from the AspWood database (http://aspwood.popgenie.org) (Sundell et al., 2017). A, apex; S, stem; Xy, xylem; Ph, phloem; Ca, cambium; R, root.
Overexpression of PagRabE1b Promotes Xylem Development in Transgenic Poplar
Mutation of particular residues of small GTPases can generate constitutively active forms, which can be used to explore Rab functions. Overexpression of constitutively active RabG3b stimulated autophagy and increased xylem development in Arabidopsis and Populus (Kwon et al., 2010, 2011). To elucidate the function of PagRabE1b in xylem development, transgenic poplars with PagRabE1b and PagRabE1b (Q74L) overexpression were generated under the control of the CaMV 35S promoter. Two independently transgenic lines (OE-1, OE-9) with higher expression of PagRabE1b and two lines with overexpressing a constitutively active form of PagRabE1b (Q74L) (QL-8, QL-13) (Zhang et al., 2018b) were selected for further analysis (Figures 2A,B). All WT and transgenic lines were grown in the greenhouse under the same environmental conditions. Compared with the WT, 2-month-old transgenic plants of PagRabE1b and PagRabE1b (Q74L) exhibited rapid growth and development (Figure 2A). Quantitative measurement of the growth rate and basal stem diameter showed that PagRabE1b transgenic plants had a 20.3–28.9% increase in the growth rate and an 11.8–26.5% increase in stem width compared with wild-type plants (Figures 2C,D). To examine whether the xylem development was altered in PagRabE1b transgenic poplars, cross-sections of basal stems from 3-month-old WT and PagRabE1b transgenic lines were analyzed. Compared with the WT, xylem width was significantly increased in all PagRabE1b overexpression lines (Figure 3A). Quantitative analysis showed that the xylem radial width was increased by 27–50% in PagRabE1b transgenic plants compared with WT plants (Figure 3B). All these results suggested that PagRabE1b may play critical roles in regulating poplar secondary growth and xylem development.
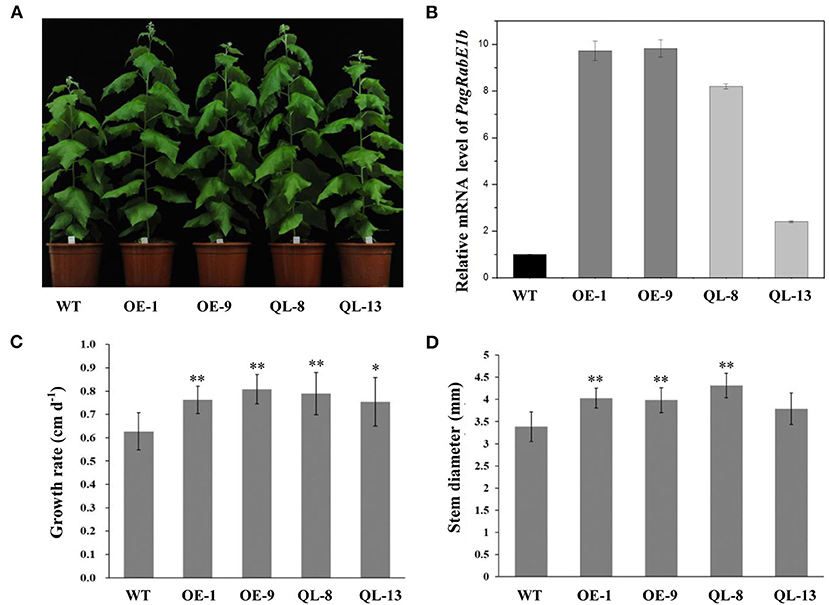
Figure 2. Growth phenotypes of PagRabE1b transgenic poplars compared with wild type (WT). (A) A photograph of WT and PagRabE1b overexpression lines. Bar = 10 cm; (B) qRT-PCR analyses of the transcript levels of PagRabE1b in WT, OE-1, OE-9, QL-8, and QL-13 lines. (C,D) The growth rate and basal diameter of 3-month-old WT, OE-1, OE-9, QL-8, and QL-13 lines. The growth rate and diameter: means ± SD of six clonally propagated plants. Student's t-test; *P < 0.05; **P < 0.01.
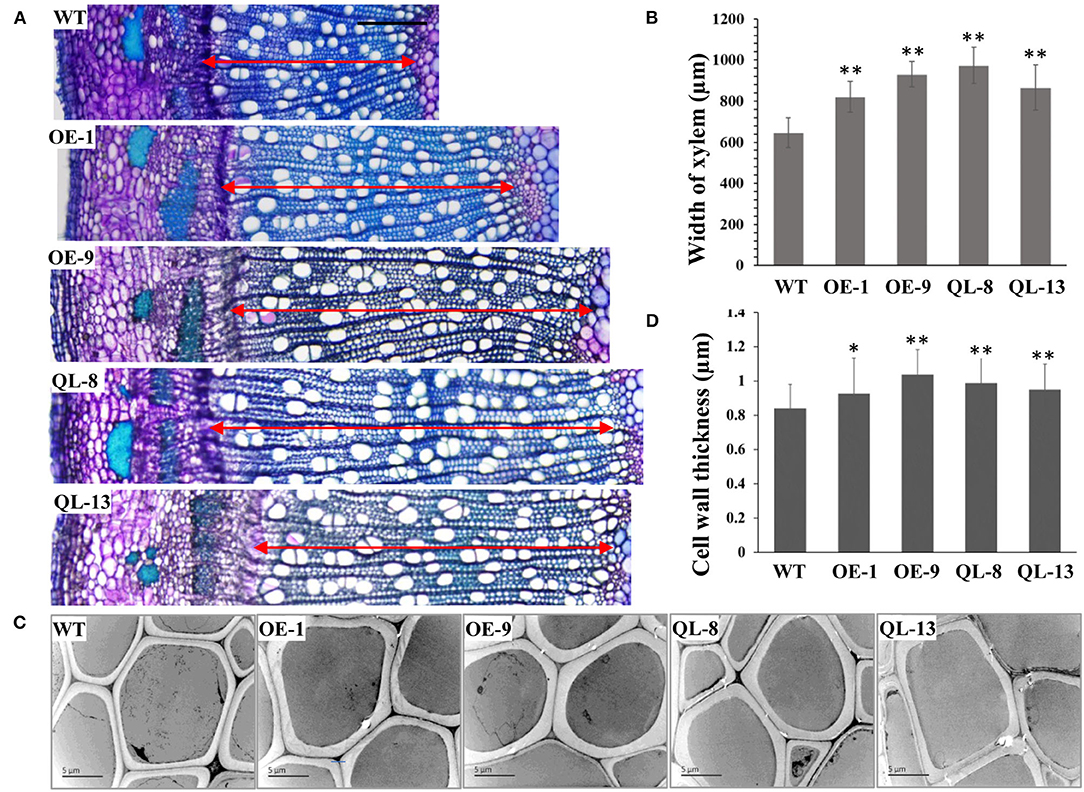
Figure 3. Xylem development was increased by PagRabE1b overexpression. (A) Transverse sections of the basal stems of 3-month-old WT, OE-1, OE-9, QL-8, and QL-13 plants. Bar = 200 μm; (B) Transmission electron microscopy (TEM) examination of the cell wall thickness of xylem fiber cells in 3-month-old WT, OE-1, OE-9, QL-8, and QL-13. Bar = 5 μm. (C) Statistical analysis of xylem width in the stems of WT, OE-1, OE-9, QL-8, and L-13 lines. (D) Statistical analysis of the cell wall thickness of WT, OE-1, OE-9, QL-8, and QL-13 lines. Student's t-test; *P < 0.05; **P < 0.01.
PagRabE1b Overexpression Affects SCW Deposition
Several studies have suggested that RabGTPase has positive roles in the controlling of SCW development. Therefore, we first examined the SCW structure by lignin autofluorescence using CLSM. The results showed that lignification and SCW deposition were increased in PagRabE1b transgenic plants than in WT (Supplementary Figure 1). Then, transverse-sections of the 20th internode of 3-month-old transgenic plants were used to examine the SCW structure using TEM. The results showed that the cell walls of xylem fiber cells were thicker in all PagRabE1b overexpression plants than in WT plants (Figure 3C). Statistical analysis showed that the SCW thickness of xylem fibers was increased by 10.2, 23.5, 17.65, and 13.1 in-OE-1, OE-9, QL-8, and QL-13 plants, respectively (Figure 3D).
The differences in SCW thickness may indicate changes in cell wall composition, and therefore, we determined the chemical components of the SCWs using the stem of wild-type and transgenic plants. Overexpression of PagRabE1b appeared to have a remarkable effect on monosaccharide contents. The contents of glucose and galactose were significantly increased in all transgenic plants compared with WT. Among these, the content of glucose was increased by 36.1–170% in PagRabE1b and PagRabE1b (Q74L) overexpression lines than in WT. In addition, the contents of fructose, arabinose, mannose, xylose, and galactose were significantly increased in the PagRabE1bor PagRabE1b (Q74L) transgenic lines compared with WT (Figure 4). These results suggest that PagRabE1b positively regulates secondary wall biosynthesis in Populus.
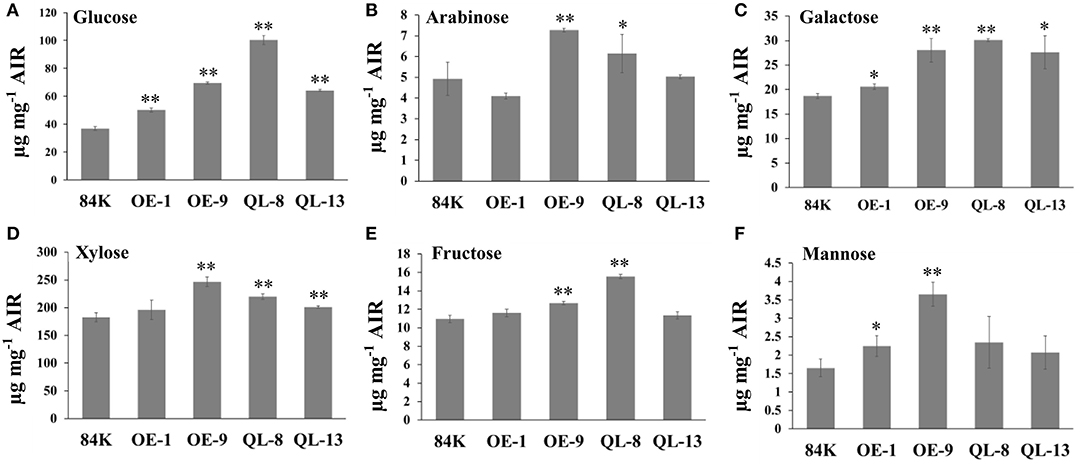
Figure 4. PagRabE1b promotes accumulation of monosaccharide content in secondary xylem tissues of transgenic plants. (A) Glucose; (B) arabinose; (C) galactose; (D) xylose; (E) fructose; (F) mannose. Student's t-test; *P < 0.05; **P < 0.01.
PagRabE1b Promotes the PCD Process in Xylem Formation
Programmed cell death is a pivotal step in xylem formation and is required for the complete maturation of both TEs and fiber cells during xylem development. To investigate the differences of PCD between PagRabE1b transgenic and WT plants, protoplasts were isolated from the SDX and analyzed by flow cytometry. Loss of plasma membrane irregularity is one of the earliest characteristics in PCD with exposing phospholipid phosphatidylserine (PS) to the exterior cellular environment. FITC-conjugated Annexin V has a high affinity with PS and acts as a sensitive probe for flow cytometric analysis of the early stage of PCD (O'brien et al., 1998). PI is commonly used to detect dead cells in a population since it is not permeant to live cells. Flow cytometry assay showed that the proportion of apoptotic cells was significantly different in WT and PagRabE1b transgenic plants. WT plants contained the lowest percentage (34.3%) of FITC positive cells, while PagRabE1bOE-9 and PagRabE1bQL-8 plants had the higher percentage (56.0 and 45.9%, respectively), of FITC positive cells (Figure 5). Similar to the FITC staining, PagRabE1bOE-9, and PagRabE1bQL-8 plants displayed a significant increase in PI-positive cells (41.5 and 34.8%, respectively), compared with WT plants (20.5%) (Figure 5). This result indicates that PagRabE1b has a positive role in PCD during xylem development.
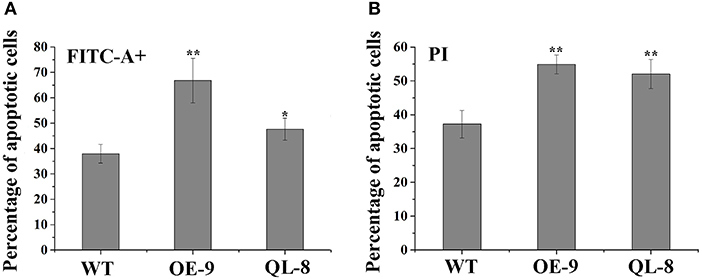
Figure 5. Flow cytometry analyses of the protoplasts from poplar stem-differentiating xylem (SDX) stained with (A) annexin V-FITC and (B) propidium iodide (PI). Results are means ± SD of three biological replicates. Student's t-test; *P < 0.05; **P < 0.01.
Expression Analysis of Xylem Development-Related Genes
Since manipulation of PagRabE1b expression resulted in changes in SCW thickness and PCD of xylem cells, we examined the expression of key genes involved in wood formation by qRT-PCR. As expected, xylem differentiation- and secondary wall regulation-related NAC and MYB genes, such as SND1-A1 (ortholog of AtSND1), VND6-C1 (AtVND6), MYB21 (AtMYB46 and AtMYB83), MYB031 (AtMYB69), MYB090 (AtMYB52 and AtMYB54), MYB127 (AtMYB67), and MYB128 (AtMYB103), were upregulated (Figure 6, Supplementary Figure 2). SCW synthesis-related genes containing homologs of Arabidopsis CESA4, CESA7, CESA8, LAC4, and LAC17 were also upregulated. Additionally, we also examined the expression levels of PCD-related genes (Peroxidase, VPE, MC9ATG8d1, ATG8f2, and ATG8i) (Kwon et al., 2011) (Figure 6, Supplementary Figure 2). All these genes were upregulated in OE-1, OE-9, QL-8, and QL-13 plants. These results suggest that PagRabE1b overexpression can activate the expression of wood formation-related genes in transgenic plants.
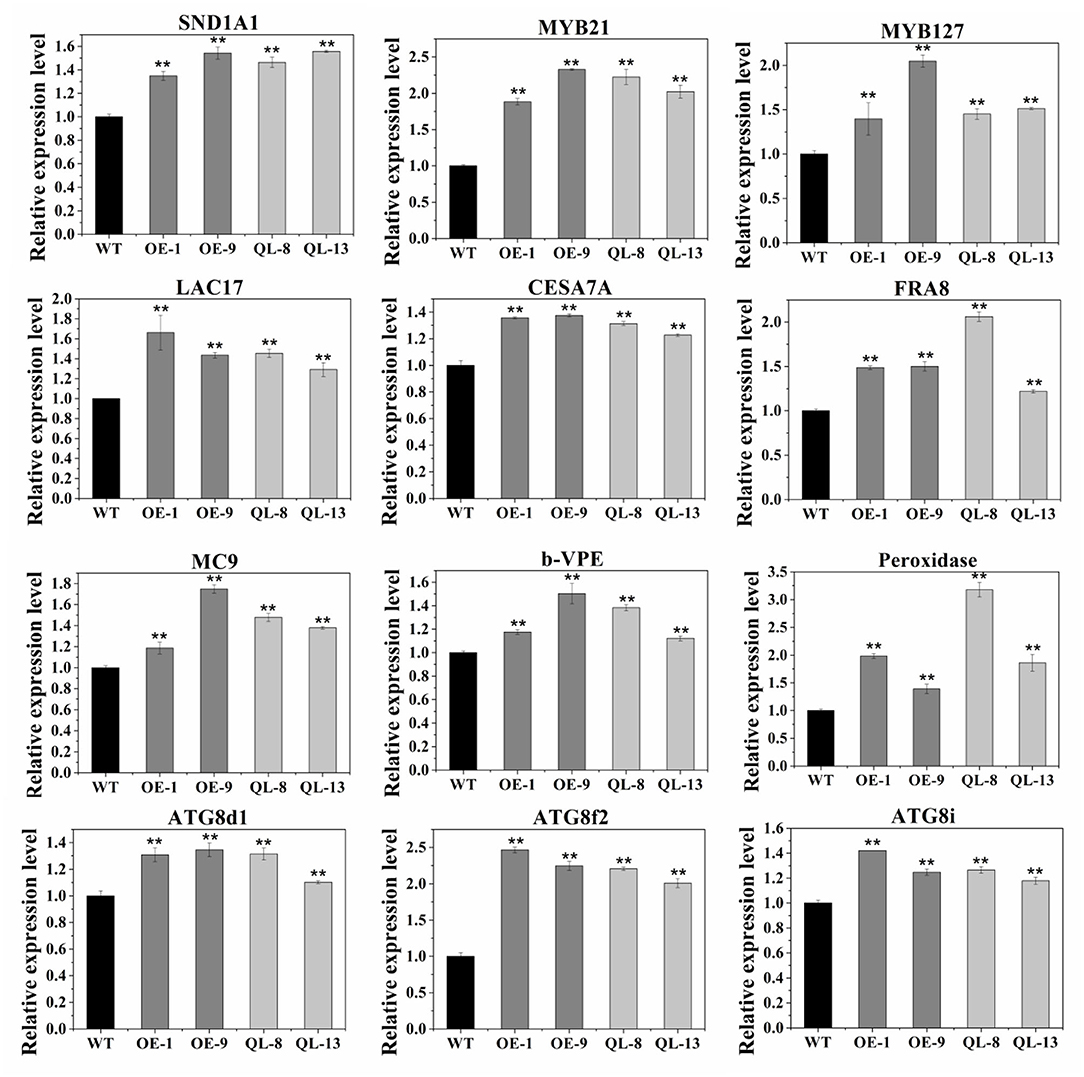
Figure 6. Expression analysis of xylem development-related genes in WT, OE-1, OE-9, QL-8, and QL-13 lines. The poplar actin gene was used as an internal control. Results are means ± SD of three biological replicates. Student's t-test; **P < 0.01.
Discussion
Wood formation is a complicated biological process, including the division and differentiation of the vascular cambium, cell elongation, SCW deposition, and PCD. Although the transcriptional regulatory network was outlined on the SCW synthesis of xylem cells (Zhang et al., 2018a), the precise regulatory mechanisms of xylem cell differentiation during xylem formation remain elusive. Previous research suggests that small GTPases may activate autophagy during wood formation (Kwon et al., 2011), other than regulate SCW development in the xylem of higher plants (Oda and Fukuda, 2014). Therefore, in this study, we further investigated the function of PagRabE1b in xylem development in poplar.
Eight of the 11 ROP genes in Arabidopsis were expressed in xylem tissues (Winter et al., 2007). In poplar, more than half of the PtRab genes were highly expressed in phloem and xylem (Zhang et al., 2018b). In this study, the qRT-PCR analysis showed that PagRabE1b was highly expressed in xylem and phloem–cambium (Figure 1). Similarly, the Eucalyptus ROP GTPase gene was found preferentially expressed in the cambium area and developing xylem (Foucart et al., 2009). Since cambium includes newly differentiating xylem cells (close to developing xylem) with higher expression than other cell types as shown in Figure 1, we propose that the involvement of PagRabE1b gene in the process of xylem development.
To investigate the role of RabE1b in woody plants, transgenic poplars overexpressing PagRabE1b were generated. The overexpression of PagRabE1b leads to an increase in both plant length and stem thickness in poplar (Figure 2). These phenotypic alterations are similar to that with Arabidopsis RabG3b overexpression (Kwon et al., 2011). In contrast, the reduced plant size and drastically altered leaf morphology were observed in the Arabidopsis RabE1d downregulated transgenic plants (Speth et al., 2009). In addition, virus-induced gene silencing of NbRabE1 caused various phenotypes, such as growth retardation, premature senescence, and aberrant leaf development (Ahn et al., 2013). These results prove that Rab GTPase plays a pivotal role in plant growth and development.
Phenotypic examination revealed that overexpression of PagRabE1b enhanced SCW thickness of fiber cells in poplar stems (Figure 3). Chemical components measurement showed an increase in cell wall constituents in the stems of transgenic plants (Figure 4). To better explain these phenotypes, we used qRT-PCR analyses and found that the expression of marker genes involved in xylem differentiation, cell wall synthesis, and PCD was upregulated in PagRabE1b OE plants (Figure 6). The NAC TFs, including SND1 and VND6, were considered as master switches (Zhang et al., 2018b) their orthologs PtrVND6 and PtrSND1 function together for reciprocal cross-regulation of xylem development in poplar (Lin et al., 2017). MYB46 and MYB83 were made up of the second layer of the regulatory network of SCW biosynthesis (Zhang et al., 2018a). Upregulation of which genes increased the biosynthesis of lignin, cellulose, and xylan, and led to the ectopic deposition of SCW (McCarthy et al., 2009; Chai et al., 2014). LAC17, CESA4A, CESA7A, and FRA8 were the enzymes that catalyzed the synthesis of lignin and cellulose. These data indicated that PagRabE1b plays a role in xylem differentiation at a higher level, rather than its function in mediating secretory vesicle transportation from the Golgi to the PM to provide substrates for SCW synthesis.
Programmed cell death occurs in the final stage during xylem cell development, which is essential to form empty, water-conducting tracheids or vessels through autolytic processes (Fukuda, 2000). In poplar, autophagosome- and autolysosome-like structures were observed in developing xylem cells in WT, and more accumulation was detected in RabG3bCA overexpression plants (Kwon et al., 2011). In this study, three autophagy-related genes, ATG8d1, ATG8f2, and ATG8i (Courtois-Moreau et al., 2009), and three PCD-related genes, peroxidase, VPE, and MC9, were highly expressed in growing stems of PagRabE1b OE plants (Figure 6). Flow cytometry analysis showed that the number of apoptotic cells in overexpression transgenic materials was more than that of WT. All these results suggest that PagRabE1b positively affects the whole xylem differentiation process, including the final cell lysisin poplar.
In conclusion, the study provided evidence that PagRabE1b acts as a positive factor for xylem differentiation and secondary wall formation. This finding provides new information on understanding the regulatory network of wood formation in woody plants.
Data Availability Statement
The original contributions presented in the study are included in the article/Supplementary Material, further inquiries can be directed to the corresponding author/s.
Author Contributions
S-TZ conceived and designed the experiments. Y-LL, L-JW, YL, Y-HG, and YC performed the experiments. Y-LL and S-TZ wrote the manuscript. All authors contributed to the article and approved the submitted version.
Funding
This work was supported by the National Nonprofit Institute Research Grant of CAF (CAFYBB2012039) and the National Key Program on Transgenic Research (2018ZX08020002).
Conflict of Interest
The authors declare that the research was conducted in the absence of any commercial or financial relationships that could be construed as a potential conflict of interest.
Acknowledgments
We thank Dr. Meng-Zhu Lu (Zhejiang Agriculture and Forestry University) and Dr. Jin Zhang (Zhejiang Agriculture and Forestry University) for the critical reading and revision of the manuscript. We thank the flow cytometry Core at National Center for Protein Sciences at Peking University for technical help.
Supplementary Material
The Supplementary Material for this article can be found online at: https://www.frontiersin.org/articles/10.3389/fpls.2021.686024/full#supplementary-material
Supplementary Figure 1. The microscopic images by confocal laser scanning microscopy (CLSM) of Rab transgenic poplars and wild type (WT). (A,B) WT; (C) OE-1; (D) OE-9; (E) QL-8; (F) QL-13.
Supplementary Figure 2. Quantitative reverse transcription PCR (qRT-PCR) analysis of xylem development-related genes in WT and transgenic lines. The poplar act in the gene was used as an internal control. Results are means ± SD of three biological replicates. Student's t-test; *P < 0.05; **P < 0.01.
Supplementary Table 1. List of primers used in this study.
References
Ahn, C. S., Han, J. A., and Pai, H. S. (2013). Characterization of in vivo functions of Nicotiana benthamianaRabE1. Planta 237, 161–172. doi: 10.1007/s00425-012-1760-5
Boerjan, W., Ralph, J., and Baucher, M. (2003). Lignin biosynthesis. Annu. Rev. Plant Biol. 54, 519–546. doi: 10.1146/annurev.arplant.54.031902.134938
Chai, G., Qi, G., Cao, Y., Wang, Z., Yu, L., Tang, X., et al. (2014). Poplar PdC3H17 and PdC3H18 are direct targets of PdMYB3 and PdMYB21, and positively regulate secondary wall formation in Arabidopsis and poplar. New Phytol. 203, 520–534. doi: 10.1111/nph.12825
Courtois-Moreau, C. L., Pesquet, E., Sjodin, A., Muniz, L., Bollhoner, B., Kaneda, M., et al. (2009). A unique program for cell death in xylem fibers of Populus stem. Plant J. 58, 260–274. doi: 10.1111/j.1365-313X.2008.03777.x
Du, J., Gerttula, S., Li, Z., Zhao, S. T., Liu, Y. L., Liu, Y., et al. (2020). Brassinosteroid regulation of wood formation in poplar. New Phytol. 225, 1516–1530. doi: 10.1111/nph.15936
Foucart, C., Jauneau, A., Gion, J. M., Amelot, N., Martinez, Y., Panegos, P., et al. (2009). Overexpression of EgROP1, a Eucalyptus vascular-expressed Rac-like small GTPase, affects secondary xylem formation in Arabidopsis thaliana. New Phytol. 183, 1014–1029. doi: 10.1111/j.1469-8137.2009.02910.x
Fukuda, H. (2000). Programmed cell death of tracheary elements as a paradigm in plants. Plant Mol. Biol. 44, 245–253. doi: 10.1007/978-94-010-0934-8_1
Hall, A. (1998). Rho GTPases and the actin cytoskeleton. Science 279, 509–514. doi: 10.1126/science.279.5350.509
Kwon, S. I., Cho, H. J., Jung, J. H., Yoshimoto, K., Shirasu, K., and Park, O. K. (2010). The Rab GTPase RabG3b functions in autophagy and contributes to tracheary element differentiation in Arabidopsis. Plant J. 64, 151–164. doi: 10.1111/j.1365-313X.2010.04315.x
Kwon, S. I., Cho, H. J., Lee, J. S., Jin, H., Shin, S. J., Kwon, M., et al. (2011). Overexpression of constitutively active Arabidopsis RabG3b promotes xylem development in transgenic poplars. Plant Cell Environ. 34, 2212–2224. doi: 10.1111/j.1365-3040.2011.02416.x
Lin, Y. C., Li, W., Sun, Y. H., Kumari, S., Wei, H., Li, Q., et al. (2013). SND1 transcription factor-directed quantitative functional hierarchical genetic regulatory network in wood formation in Populus trichocarpa. Plant Cell 25, 4324–4341. doi: 10.1105/tpc.113.117697
Lin, Y. J., Chen, H., Li, Q., Li, W., Wang, J. P., Shi, R., et al. (2017). Reciprocal cross-regulation of VND and SND multigene TF families for wood formation in Populus trichocarpa. Proc. Natl. Acad. Sci. U.S.A. 114, E9722–E9729. doi: 10.1073/pnas.1714422114
McCarthy, R. L., Zhong, R., and Ye, Z. H. (2009). MYB83 is a direct target of SND1 and acts redundantly with MYB46 in the regulation of secondary cell wall biosynthesis in Arabidopsis. Plant Cell Physiol. 50, 1950–1964. doi: 10.1093/pcp/pcp139
O'brien, I. E. W., Baguley, B. C., Murray, B. G., Morris, B. A. M., and Ferguson, I. B. (1998). Early stages of the apoptotic pathway in plant cells are reversible. Plant J. 13, 803–814. doi: 10.1046/j.1365-313X.1998.00087.x
Oda, Y., and Fukuda, H. (2012). Initiation of cell wall pattern by a Rho- and microtubule-driven symmetry breaking. Science 337, 1333–1336. doi: 10.1126/science.1222597
Oda, Y., and Fukuda, H. (2014). Emerging roles of small GTPases in secondary cell wall development. Front. Plant Sci. 5:428. doi: 10.3389/fpls.2014.00428
Scheller, H. V., and Ulvskov, P. (2010). Hemicelluloses. Annu. Rev. Plant Biol. 61, 263–289. doi: 10.1146/annurev-arplant-042809-112315
Speth, E. B., Imboden, L., Hauck, P., and He, S. Y. (2009). Subcellular localization and functional analysis of the Arabidopsis GTPase RabE. Plant Physiol. 149, 1824–1837. doi: 10.1104/pp.108.132092
Sugiyama, Y., Nagashima, Y., Wakazaki, M., Sato, M., Toyooka, K., Fukuda, H., et al. (2019). A Rho-actin signaling pathway shapes cell wall boundaries in Arabidopsis xylem vessels. Nat. Commun. 10:468. doi: 10.1038/s41467-019-08396-7
Sundell, D., Street, N. R., Kumar, M., Mellerowicz, E. J., Kucukoglu, M., Johnsson, C., et al. (2017). AspWood: high-spatial-resolution transcriptome profiles reveal uncharacterized modularity of wood formation in Populus tremula. Plant Cell 29, 1585–1604. doi: 10.1105/tpc.17.00153
Vernoud, V., Horton, A. C., Yang, Z., and Nielsen, E. (2003). Analysis of the small GTPase gene superfamily of Arabidopsis. Plant Physiol. 131, 1191–1208. doi: 10.1104/pp.013052
Wang, J. P., Matthews, M. L., Williams, C. M., Shi, R., Yang, C., Tunlaya-Anukit, S., et al. (2018). Improving wood properties for wood utilization through multi-omics integration in lignin biosynthesis. Nat. Commun. 9:1579. doi: 10.1038/s41467-018-03863-z
Wang, Y., Chen, Y., Ding, L., Zhang, J., Wei, J., and Wang, H. (2016). Validation of reference genes for gene expression by quantitative real-time RT-PCR in stem segments spanning srimary to secondary srowth in Populus tomentosa. PLoS ONE 11:e0157370. doi: 10.1371/journal.pone.0157370
Wang, Z., Mao, Y., Guo, Y., Gao, J., Liu, X., Li, S., et al. (2020). MYB transcription factor161 mediates feedback regulation of secondary wall-associated NAC-Domain1 family genes for wood formation. Plant Physiol. 184, 1389–1406. doi: 10.1104/pp.20.01033
Winter, D., Vinegar, B., Nahal, H., Ammar, R., Wilson, G. V., and Provart, N. J. (2007). An “Electronic Fluorescent Pictograph” browser for exploring and analyzing large-scale biological data sets. PLoS ONE 2:e718. doi: 10.1371/journal.pone.0000718
Xie, M., Zhang, J., Tschaplinski, T. J., Tuskan, G. A., Chen, J. G., and Muchero, W. (2018). Regulation of lignin biosynthesis and its role in growth-defense tradeoffs. Front. Plant. Sci. 9:1427. doi: 10.3389/fpls.2018.01427
Zhang, J., Li, Y., Liu, B., Wang, L., Zhang, L., Hu, J., et al. (2018b). Characterization of the PopulusRab family genes and the function of PtRabE1b in salt tolerance. BMC Plant Biol. 18:124. doi: 10.1186/s12870-018-1342-1
Zhang, J., Serra, J. A. A., and Helariutta, Y. (2015). Wood development: growth through knowledge. Nat. Plants 1:15060. doi: 10.1038/nplants.2015.60
Zhang, J., Xie, M., Tuskan, G. A., Muchero, W., and Chen, J. G. (2018a). Recent advances in the transcriptional regulation of secondary cell wall biosynthesis in the woody plants. Front. Plant Sci. 9:1535. doi: 10.3389/fpls.2018.01535
Zhao, Y., Song, X., Zhou, H., Wei, K., Jiang, C., Wang, J., et al. (2020). KNAT2/6b, a class I KNOX gene, impedes xylem differentiation by regulating NAC domain transcription factors in poplar. New Phytol. 225, 1531–1544. doi: 10.1111/nph.16036
Keywords: poplar, small gtp binding protein, PagRabE1b, cell wall, wood formation
Citation: Liu Y-L, Wang L-J, Li Y, Guo Y-H, Cao Y and Zhao S-T (2021) A Small Guanosine Triphosphate Binding Protein PagRabE1b Promotes Xylem Development in Poplar. Front. Plant Sci. 12:686024. doi: 10.3389/fpls.2021.686024
Received: 26 March 2021; Accepted: 14 May 2021;
Published: 04 June 2021.
Edited by:
Guohua Chai, Qingdao Agricultural University, ChinaReviewed by:
Yuxiang Cheng, Northeast Forestry University, ChinaBo Zheng, Huazhong Agricultural University, China
Copyright © 2021 Liu, Wang, Li, Guo, Cao and Zhao. This is an open-access article distributed under the terms of the Creative Commons Attribution License (CC BY). The use, distribution or reproduction in other forums is permitted, provided the original author(s) and the copyright owner(s) are credited and that the original publication in this journal is cited, in accordance with accepted academic practice. No use, distribution or reproduction is permitted which does not comply with these terms.
*Correspondence: Shu-Tang Zhao, zhaost318@163.com