- 1College of Agriculture, Shanxi Agricultural University, Taiyuan, China
- 2Department of Plant Science, College of Agronomy, Northwest Agriculture & Forestry University, Yangling, China
- 3School of Life Science and Technology, University of Electronic Science and Technology of China, Chengdu, China
- 4School of Life and Environmental Sciences, Plant Breeding Institute, The University of Sydney, Cobbitty, NSW, Australia
- 5Department of Botany, College of Life Science, Shanxi University, Taiyuan, China
- 6School of Life and Environmental Sciences, Plant Breeding Institute, The University of Sydney, Sydney, NSW, Australia
Thinopyrum intermedium (2n = 6x = 42, JJJSJSStSt) is one of the important resources for the wheat improvement. So far, a few Th. intermedium (Thi)-specific molecular markers have been reported, but the number is far from enough to meet the need of identifying alien fragments in wheat-Th. intermedium hybrids. In this study, 5,877,409 contigs were assembled using the Th. intermedium genotyping-by-sequencing (GBS) data. We obtained 5,452 non-redundant contigs containing mapped Thi-GBS markers with less than 20% similarity to the wheat genome and developed 2,019 sequence-tagged site (STS) molecular markers. Among the markers designed, 745 Thi-specific markers with amplification products in Th. intermedium but not in eight wheat landraces were further selected. The distribution of these markers in different homologous groups of Th. intermedium varied from 47 (7/12/28 on 6J/6St/6JS) to 183 (54/62/67 on 7J/7St/7JS). Furthermore, the effectiveness of these Thi-specific markers was verified using wheat-Th. intermedium partial amphidiploids, addition lines, substitution lines, and translocation lines. Markers developed in this study provide a convenient, rapid, reliable, and economical method for identifying Th. intermedium chromosomes in wheat. In addition, this set of Thi-specific markers can also be used to estimate genetic and physical locations of Th. intermedium chromatin in the introgression lines, thus providing valuable information for follow-up studies such as alien gene mining.
Introduction
Thinopyrum intermedium (Host) Barkworth & D.R. Dewey (2n = 6x = 42, JJJSJSStSt) belongs to the tribe Triticeae, which is a perennial cross-pollinated species and cultivated as a forage grass worldwide (Vogel and Jensen, 2001). It is also an ideal species for water and soil conservation and saline–alkali land improvement (Li and Wang, 2009). It is generally believed that the Th. intermedium J sub-genome is partially homologous to the genomes of Th. bessarabicum (2n = 2x = 14, JbJb) and Th. elongatum (2n = 2x = 14, JeJe), the St sub-genome is contributed by Pseudoroegneria spicata (2n = 2x = 14, StSt), whereas the JS sub-genome is derived from the J sub-genome partially recombined with the St genome (Chen et al., 1998; Mahelka et al., 2013).
Th. intermedium can be readily hybridized with common wheat (Triticum aestivum L., 2n = 6x = 42, AABBDD) (Peto, 1936; Stebbins and Pun, 1953; Dewey, 1984; Li et al., 2015). It has excellent quality, stress tolerance, and disease resistance, especially against powdery mildew, rusts, barley yellow dwarf virus, and wheat streak mosaic virus (Chang et al., 2010; Bao et al., 2014; Salina et al., 2015; Li et al., 2016; Zhang et al., 2020), making it an important wild resource for the wheat improvement. In the 1960s, Tsitsin (1965) obtained the wheat-Th. intermedium octoploid for the first time through distant hybridization, which initiated the exploration and utilization of Th. intermedium. Thereafter, Th. intermedium chromosomes have been introgressed into wheat, resulting in the production of wheat-Th. intermedium addition, substitution, and translocation lines (Forster et al., 1987; Chen et al., 1999; Yang et al., 2006; Zhan et al., 2015; Li et al., 2017). It is critical in germplasm enhancement to identify alien chromatin by genomic in situ hybridization (GISH) (Chen et al., 1998, 1999; Chen, 2005) or fluorescence in situ hybridization (FISH) (Chang et al., 2010; Li et al., 2015, 2016; Salina et al., 2015; Zhang et al., 2020). At present, several FISH probes, such as Oligo-pDb12H derived from Dasypyrum villosum (Yu et al., 2019) and Oligo-B11 and Oligo-pThp3.93 from Th. ponticum (Xi et al., 2019), are used to differentiate Th. intermedium chromosomes from wheat chromosomes. Recently, a set of pooled oligo probes Synt1~7 was developed to distinguish the seven homologous groups (HGs) of Triticeae species including Th. intermedium (Li et al., 2021). However, for very small alien fragments in the later generation of hybrids, it is difficult to determine their positions in the Th. intermedium genome cytologically; therefore, the identification based on molecular markers is crucial.
Markers from wheat or rice (Oryza sativa L.), such as simple sequence repeat (SSR) markers, PCR-based landmark unique gene (PLUG) markers, and single-nucleotide polymorphism (SNP) markers, were used as complementary means of cytological identification to detect alien fragments in wheat genome (Chen, 2005; Bao et al., 2010; Li et al., 2017, 2021; Xi et al., 2019; Yu et al., 2019). The SNP genotyping array (Cseh et al., 2019) and kompetitive allele-specific PCR (KASP) genotyping assay (Grewal et al., 2020) can also be used for characterizing wheat-Th. intermedium introgression lines. However, the position of the introgressed alien fragments in the Th. intermedium genome cannot be determined.
It is particularly important to develop specific markers directly based on the Th. intermedium sequences. In 2016, Kantarski et al. (2017) explored genotyping-by-sequencing (GBS) markers in Th. intermedium and constructed the first consensus genetic map containing all Th. intermedium linkage groups (Thi-LG1~21) using seven genetic populations. However, the sub-genome information corresponding to each Thi-LG remains unknown. Subsequently, Wang R. R. C. et al. (2020) compared the GBS sequences of Ps. spicata with the previously released Thi-GBS sequences and identified Thi-LG2, 4, 8, 11, 13, 17, and 21 as the St sub-genome. In this study, the above-mentioned Thi-GBS sequences were compared with the annotated coding sequence (CDS) data of Th. elongatum published recently (Wang H. et al., 2020) to distinguish the J and JS sub-genomes in Thi-LGs. Then, contigs assembled with the original Thi-GBS sequences were selected to develop sequence-tagged site (STS) markers. The Thi-specific markers that have amplification products in Th. intermedium but not in common wheat were identified, thereby providing an economical and convenient tool for identifying Th. intermedium fragments in wheat.
Materials and Methods
Plant Materials
Six independent plants from the same Th. intermedium accession (in order to avoid the individual differences caused by cross-pollination) and eight wheat landraces (in order to avoid the possibility that wheat cultivars may contain alien species fragments such as 1B/1R, which will affect the screening results) from different ecological regions in China were used to screen the Thi-specific markers. Th. elongatum, Th. bessarabicum, Ps. Spicata, and D. villosum were used as the related species of Th. intermedium to detect the amplification of these Thi-specific markers. Wheat-Th. intermedium partial amphidiploids, addition lines, substitution lines, and translocation lines were used to test the effectiveness of the Thi-specific markers. Materials used in this study and their relevant information including name, genome composition, and providers are listed in Table 1.
Informatics Analysis of Thi-GBSs
The method used to distinguish sub-genomes in Thi-LGs was described by Wang R. R. C. et al. (2020). The 10,029 Thi-GBS sequences mapped to Thi-LG1~21 (Kantarski et al., 2017) were aligned with the annotated CDSs of Th. elongatum (accession number GWHABKY00000000, version 1.0) (Wang H. et al., 2020) obtained from the National Geophysical Data Center database (NGDC, https://bigd.big.ac.cn/) with BLAST tool (version 2.6.0+), setting E ≤ 1.0 ×10−25. For the Thi-GBS sequences with multiple hits, the hit with the lowest e-value was selected for further analysis. In the same HG of Th. intermedium, the Thi-LG with the most matched The-CDSs was presumed to be the J sub-genome. For the number of significant hits, a Chi-squared test was performed with the Bonferroni adjustment for multiple tests to determine if observed values were significantly different.
Sequence Assembly and Primer Design
The original Thi-GBS data (accession number SRX3008333) downloaded from the Sequence Read Archive database (https://www.ncbi.nlm.nih.gov/sra/) was assembled as contigs using the SOAPdenovo2 software1 (Luo et al., 2012). After removing redundancy, contigs containing the mapped Thi-GBS marker (Kantarski et al., 2017) were used to blast the wheat genome (cv. Chinese Spring, version 1.0) downloaded from the International Wheat Genome Sequencing Consortium database (IWGSC, https://urgi.versailles.inra.fr/) (Lukaszewski et al., 2014). Then, the contigs with sequence similarity of less than 20% were obtained for developing STS markers. A Primer 3.0 software-based2 script written by the Perl language (Han et al., 2015) was used for a large-scale primer design, and the parameters were set as following: primer length was 18–22 bp, and the product length was 100–400 bp.
Screening and Validation of the Thi-Specific Markers
The developed STS markers were tested on six Th. intermedium individuals and eight wheat landraces, and those that can amplify in Th. intermedium but not in wheat were selected as the Thi-specific markers. These markers were then used on wheat-Th. intermedium partial amphiploids, addition lines, and substitution lines to verify their effectiveness. In addition, the amplification results of the Thi-specific markers in Th. bessarabicum, Th. elongatum, Ps. spicata, and D. villosum were visualized by the Venn diagram (http://bioinformatics.psb.ugent.be/webtools/Venn/) and were subjected to the phylogenetic analysis using MEGA6.03 (Tamura et al., 2013) with the neighbor-joining method and 1,000 bootstraps. A physical location of Thi-specific markers was obtained by blasting against the genome data of Th. intermedium (version 2.1, http://phytozome.jgi.doe.gov/).
PCR was performed in 10 μl reaction using PCR Mix (B532061, Sangon Biotech, Shanghai, China). Amplified products were electrophoresed in 8% non-denaturing polyacrylamide gels and then stained in a 0.1% silver nitrate solution.
Fluorescence in situ Hybridization and GISH Analyses
Mitotic metaphase chromosomes were obtained from root tips and were spread according to the procedures as described in Lang et al. (2018). Four oligo-nucleotide probes, such as Oligo-pSc119.2, Oligo-pTa535 (Tang et al., 2014), Oligo-k288 (Wang et al., 2019), and Oligo-B11 (Kantarski et al., 2017), were used to identify wheat and Th. intermedium chromosomes. They were 5′-end labeled with either 6-carboxyfluorescein (6-FAM) for green signals or 6-carboxytetramethylrhodamine (Tamra) for red signals (Supplementary Table 1). The protocol of non-denaturing FISH (ND-FISH) using oligo probes was according to Fu et al. (2015). The FISH images were captured with an Olympus BX-51 Microscope equipped with a DP-70 CCD Camera (Shinjuku, Tokyo, Japan) or a Zeiss Axio Imager Microscope (Oberkochen, Germany) equipped with a Retiga EXi CCD Camera (QImaging, Surrey, BC, Canada).
After stripping off the oligo probes, the same slides were analyzed by GISH as described in Zhang et al. (2001). Total genomic DNA from Th. intermedium (Cytogenetic stock accession C05.05, University of Sydney) was labeled with biotin-16-dUTP (Roche Diagnostics Australia, Castle Hill, NSW, Australia) using nick translation. Unlabeled total genomic DNA of wheat was used as a blocker. The probe to blocker ratio was ~1:80. Signals were detected with Fluorescein Avidin DN (Vector Laboratories, Burlingame, CA, USA). Chromosomes were counterstained with DAPI and pseudo-colored red.
Results
Determination of Sub-genomes for Thi-GBS Sequences
Using blastn of homology analysis, 284 The-CDSs were matched at a minimum e-value of 1.0 ×10−25 with those in 10,029 Thi-GBS sequences reported previously (Kantarski et al., 2017) (Table 2). Because the JS genome incorporated part of the St genome (Chen et al., 1998; Mahelka et al., 2013), the similarity between the The-Je genome and the Thi-J sub-genome is higher than that between the The-Je genome and the Thi-JS sub-genome. Therefore, Thi-LGs 1, 6, 7, 10, 14, 18, and 20 can be confidently assigned to Thi-HGs 1, 2, 3, 4, 5, 6, and 7 of the J sub-genome, respectively (Table 2). Because Thi-LGs 2, 4, 8, 11, 13, 17, and 21 were reported as Thi-HGs 1, 2, 3, 4, 5, 6, and 7 of the St sub-genome (Wang R. R. C. et al., 2020), respectively, the remaining seven Thi-LGs 3, 5, 9, 12, 15, 16, and 19 were presumed to be the 1JS−7JS sub-genomes.
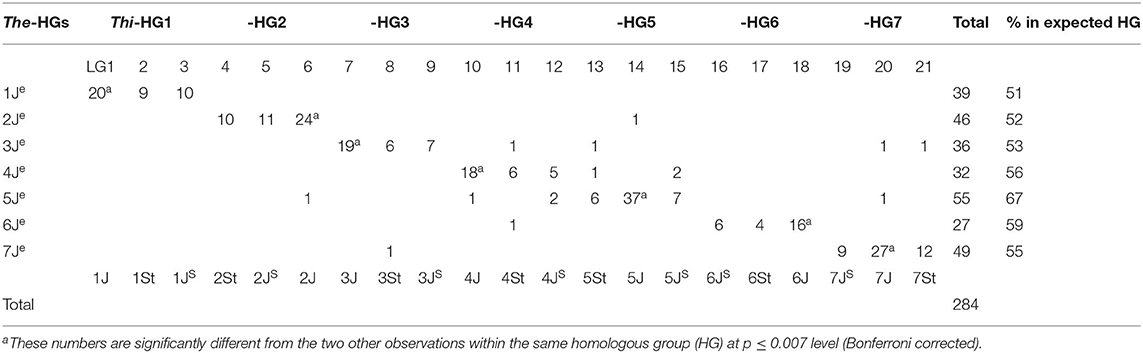
Table 2. Locations of 284 The-CDSs matched with Thi-GBS sequences reported in Kantarski et al. (2017).
Development of Thi-Specific Markers
A total of 5,877,409 contigs were assembled using the original Thi-GBS sequences, ranging in length from 100 to 3,094 bp, with a total length of 915,311,073 bp (Supplementary Table 2). After removing the redundancy, 5,452 contigs containing the mapped Thi-GBS markers (Kantarski et al., 2017) were identified. In total, 2,019 STS markers were developed for the 5,452 non-redundant contigs, with 250, 215, 323, 253, 323, 253, and 402 markers distributed in the Thi-HG1 to HG7, respectively (Figure 1).
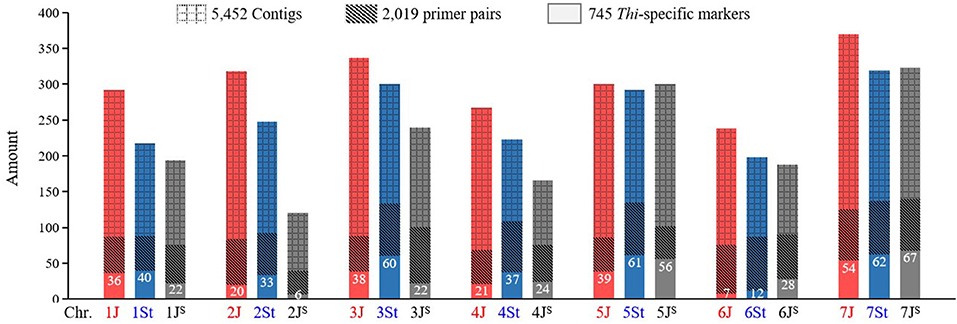
Figure 1. Amount and distribution of the GBS-contigs and markers developed in Th. intermedium. The number of Thi-specific markers on each chromosome is listed.
Out of the 2,019 STS markers, 745 amplified only in Th. intermedium but failed in the eight wheat landraces were considered as the Thi-specific markers (Supplementary Figure 1 and Supplementary Table 3). Linkage maps are shown in Figure 2, and the marker distribution in Thi-HG1-7 was 98 (36/40/22, 1J/1St/1JS), 59 (20/33/6, 2J/2St/2JS), 120 (38/60/22, 3J/3St/3JS), 82 (21/37/24, 4J/4St/4JS), 156 (39/61/56, 5J/5St/5JS), 47 (7/12/28, 6J/6St/6JS), and 183(54/62/67, 7J/7St/7JS). Among them, 224, 306, and 233 markers were located respectively in the J, St, and JS sub-genome.
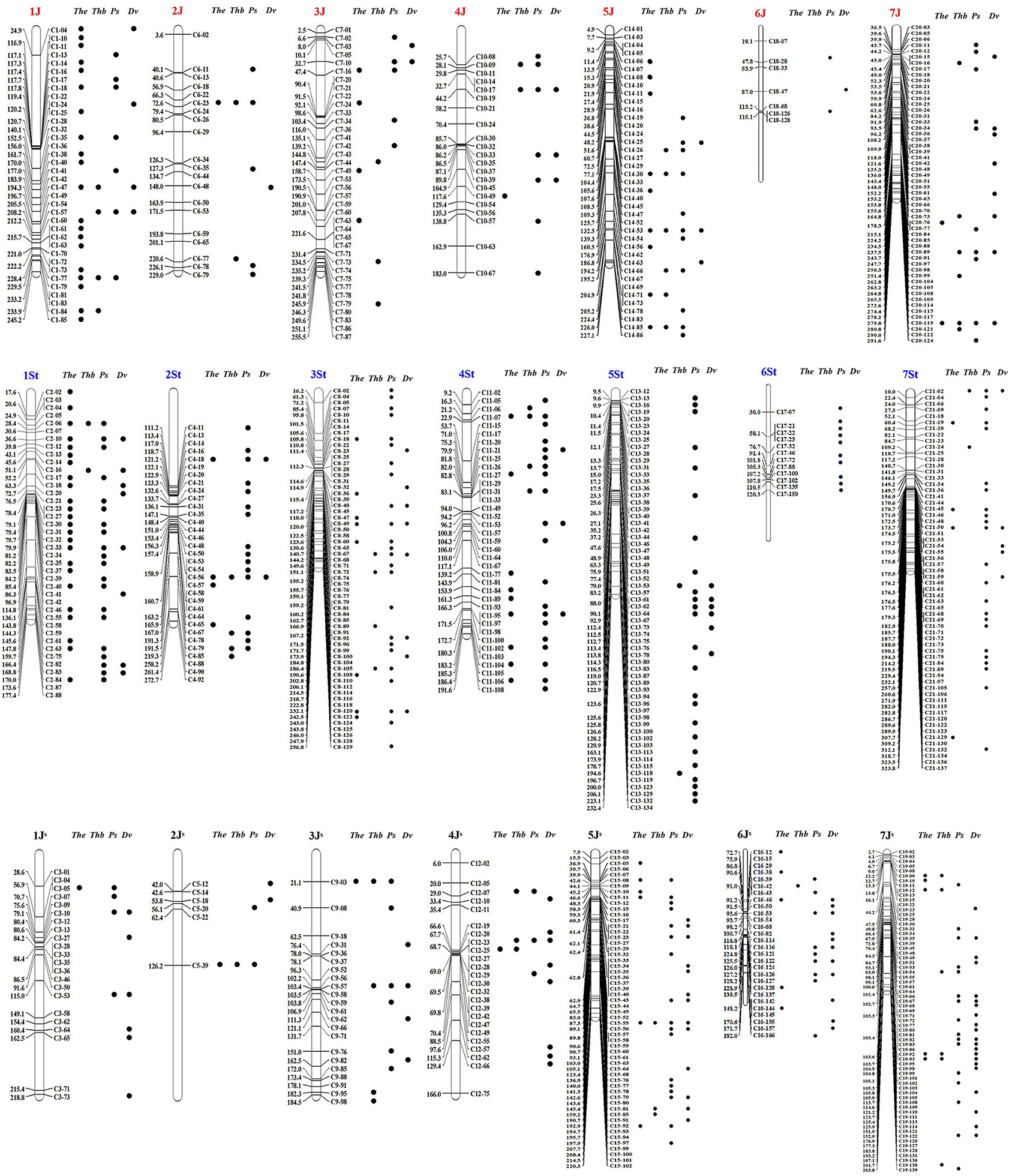
Figure 2. A linkage map of 745 Thi-specific STS markers derived from the Thi-GBS linkage map reported in Kantarski et al. (2017). Black dots next to the markers indicate that these markers have amplification products in Th. elongatum (The), Th. bessarabicum (Thb), Ps. spicata (Ps), and D. villosum (Dv).
Evaluation of Thi-Specific Markers Using Wheat-Th. intermedium Lines
The Thi-specific markers were used to amplify two wheat-Th. intermedium partial amphiploids TAF46 (ABD+1J+2St+3J+4St+5J+6St+7J) and TE-3 (ABD+1St+2Js+3J+4J+4Js+5Js+6St+7St). The detectable rate of Thi-specific markers in 1J (61%), 2St (58%), 3J (47%), 4St (57%), 5J (36%), 6St (77%), and 7J (54%) was higher than that of other sub-genomes in the corresponding HG in TAF46 (Figure 3A). In TE-3 (Figure 3B), the sub-genomes with high detectable rate were 1St (53%), 2JS (100%), 3J (66%), 4J (71%), 4Js (79%), 5Js (73%), 6St (69%), and 7St (61%).
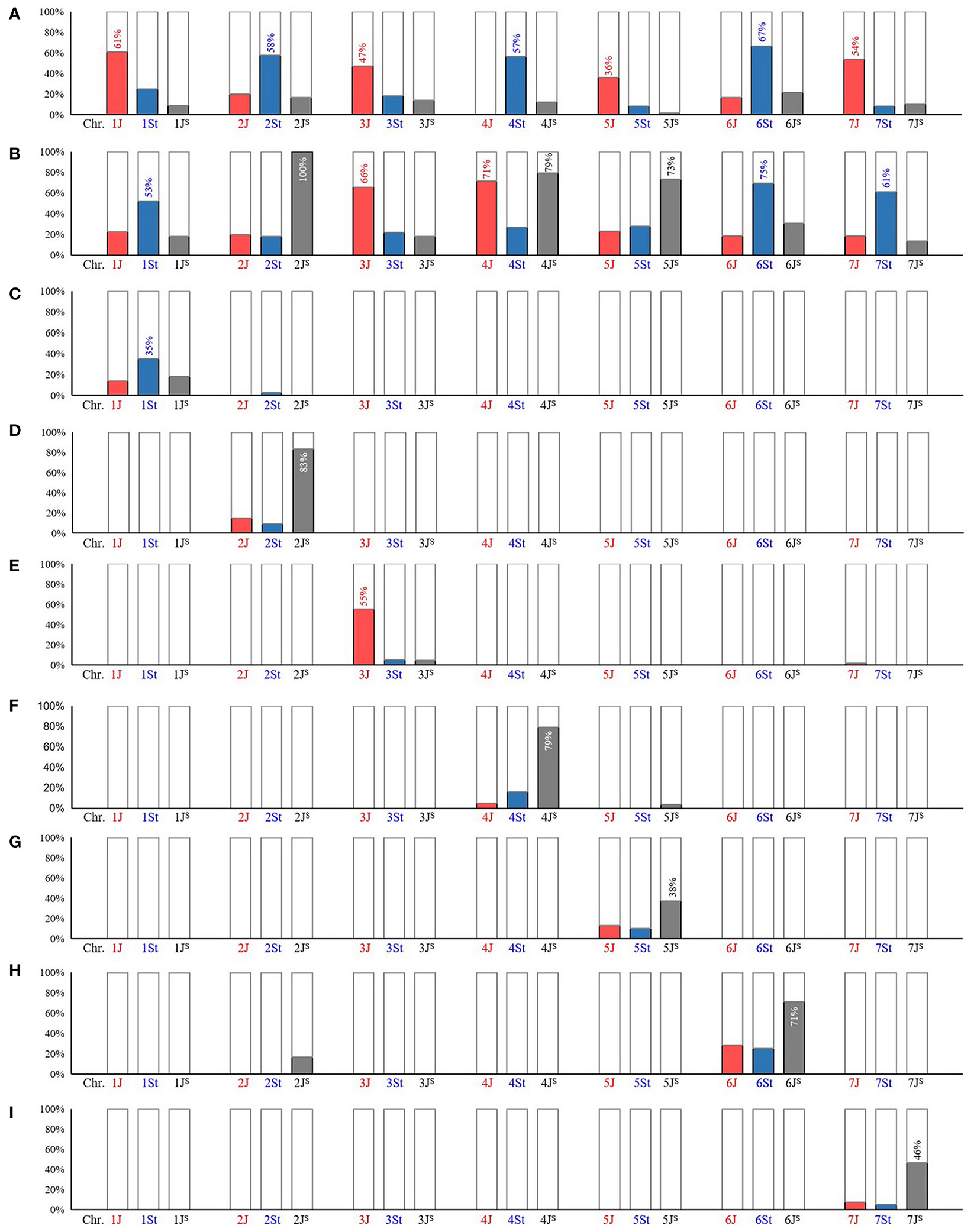
Figure 3. Amplification of Thi-specific markers in wheat-Th. intermedium introgression lines. (A) partial amphiploid TAF46 (ABD+1J+2St+3J+4St+5J+6St+7J); (B) partial amphiploid TE-3 (ABD+1St+2Js+3J+4J+4Js+5Js+6St+7St); (C) substitution line AS1677 [ABD+1St(1D)]; (D) addition line X24C14 (ABD+2Js); (E) addition line A1082 (ABD+3J); (F) substitution line A1125 [ABD+4Js(4B)]; (G) translocation line A39 (ABD+T4BS.5JsL); (H) substitution line XM-4 [ABD+6Js(6B)]; (I) translocation line Z4 (ABD+T7JsS-3AS.3AL+T7JsS.7JsL-3AL). The sub-genomes with the largest proportion of positive markers were labeled, and the numbers on the column were significantly different from the two other observations within the same homologous group (HG) at p ≤ 0.0125 level (Bonferroni corrected).
Furthermore, six wheat-Th. intermedium introgressions with single alien sub-genome from different Thi-HGs were characterized, namely AS1677 [ABD+1St(1D)], X24C14 (ABD+2JS), A1082 (ABD+3J), A1125 [ABD+4JS(4B)], A39 (ABD+T4BS.5JSL), and XM-4 [ABD+6JS(6B)] (Figure 4). These six introgressions and Z4 (ABD+T7JSS-3AS.3AL+T7JSS.7JSL-3AL) (Lang et al., 2018) were further used to evaluate the Thi-specific markers, which were able to detect the introgressed Thi-chromosomes in these lines correctly (Figures 3C–I), exhibiting good specificity among Thi-HGs. However, there was a certain degree of non-specificity in distinguishing the three sub-genomes within the same Thi-HG.
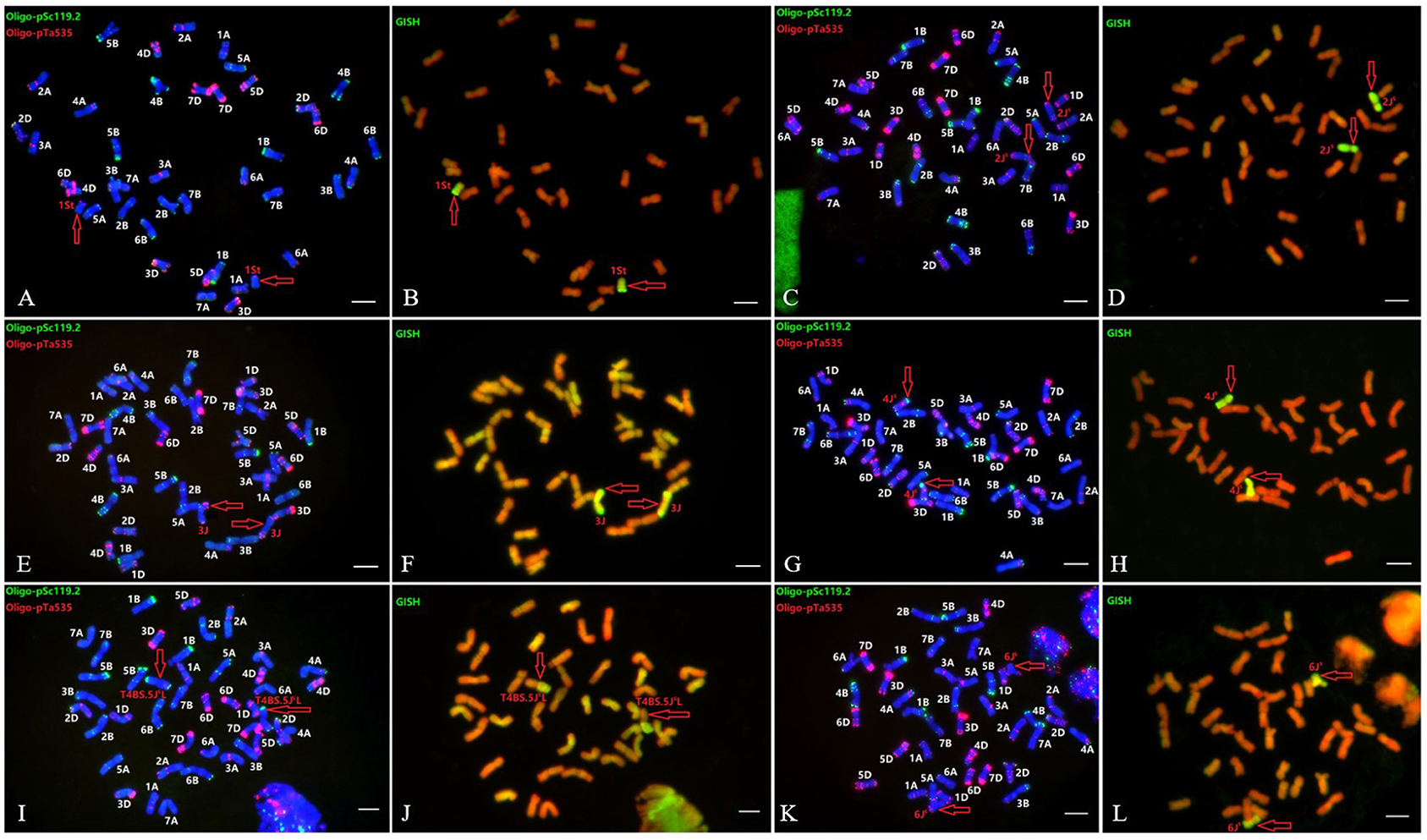
Figure 4. Sequential FISH and GISH patterns of six wheat-Th. intermedium introgressions with single alien sub-genome from Thi-HG1-6, respectively. (A,B) substitution line AS1677 [ABD+1St(1D)]; (C,D) addition line X24C14 (ABD+2Js); (E,F) addition line A1082 (ABD+3J); (G,H) substitution line A1125 [ABD+4Js(4B)]; (I,J) translocation line A39 (ABD+T4BS.5JsL); and (K,L) substitution line XM-4 [ABD+6Js(6B)]. The probes for FISH were Oligo-pSc119.2 (green) + Oligo-pTa535 (red) (A,C,E,G,I,K). The probe (yellow-green) for GISH analysis was Th. intermedium total genomic DNA (B,D,F,H,J,L). Bars, 10 μm.
Prediction of the Positions in of Alien Segments Th. intermedium by Thi-Specific Markers
The Thi-specific markers were used to predict the positions of Th. intermedium chromatin in T1332, a translocation line introduced segment of the long arm of Thi-chromosome 4J (Figures 5A–C). In order to improve the chromosome specificity of markers, 82 Thi-specific markers of Thi-HG4 were used on the substitution line X24C10 with Thi-chromosome 4J (4B) (Li et al., 2017) and the 4St addition line L4 (Forster et al., 1987; Chen et al., 1999). Combined with the previous identification results in the substitution line A1125 4JS (4B) (Figure 3F) and two partial amphiploids TAF46 and TE-3 (Figures 3A,B), 58 (71%) Thi-chromosome-specific markers were identified, of which 15 were 4J-specific, 27 were 4St-specific, and 16 were 4JS-specific (Figure 5D). Among the 58 Thi-chromosome specific markers in T1332 (ABD+T4BS/4JL) showed that four 4J-specific markers C10-32, C10-49, C10-54, and C10-63 amplified target products. According to the physical location of these markers, it could be inferred that the introduced fragment contained the chromosome interval 4J:351604953-480594047Mb of Th. intermedium (Figure 5E).
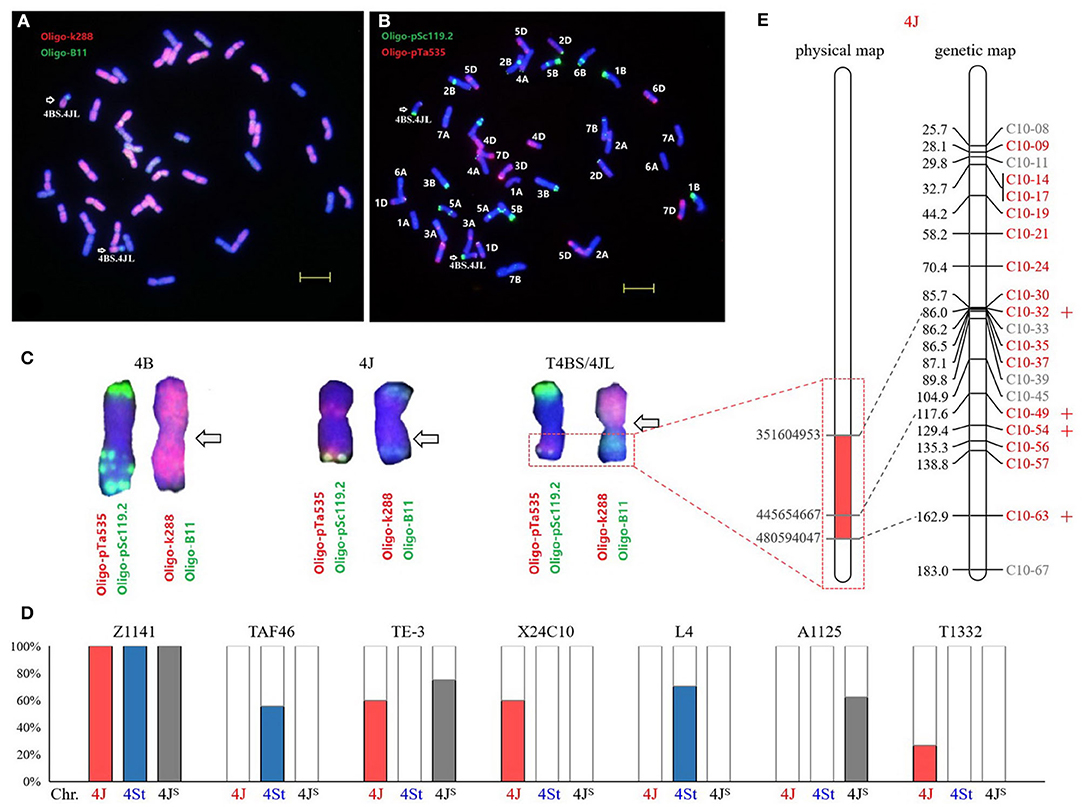
Figure 5. Identification of wheat-Th. intermedium translocation line T1332. The probes were Oligo-k288 (red) + Oligo-B11 (green) (A) and Oligo-pSc119.2 (green) + Oligo-pTa535 (red) (B). Bars, 10 μm. The chromosomes in the karyotype (C) were 4B, 4J, and T4BS/4JL, respectively, and the arrows pointed to the translocation breakpoints. (D) Amplification of 4J-, 4St-, and 4Js-specific markers in Th. intermedium and six wheat-Thi introgression lines. (E) The position of 21 Thi-specific markers on 4J physical and linkage maps. Red markers are 4J-specific, four of which with amplification products in translocation line T1332 are marked with plus sign.
Amplification of Thi-Specific Markers in the Je/Jb/St/V Genomes
The amplification results of Thi-specific markers showed that 107 (14%), 62 (8%), 233 (31%), and 116 (16%) markers could be amplified in Th. elongatum, Th. bessarabicum, Ps. spicata, and D. villosum, respectively. Among them, the markers located in the J and St sub-genomes were amplified the most in Ps. spicata, 22 and 44%, respectively, whereas markers in the JS sub-genome were amplified the most in D. villosum (29%) (Figure 6A). Similarly, the phylogenetic analysis showed that the J and St sub-genomes were closely related to Ps. spicata, whereas the JS sub-genome is relatively close to D. villosum (Figure 6A). The number of Thi-specific markers that specifically amplify in Th. elongatum, Th. bessarabicum, Ps. spicata, and D. villosum were 50, 20, 141, and 59, respectively, whereas 366 markers were not amplified in the above species (Figure 6B).
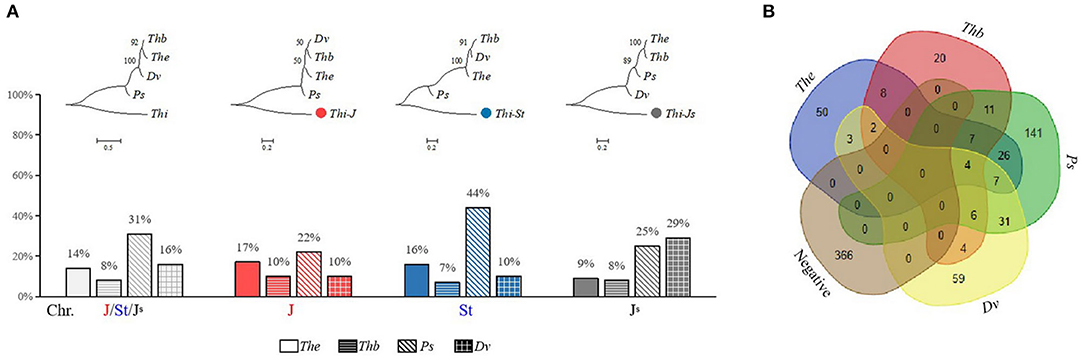
Figure 6. Amplification of Thi-specific markers in the Je/Jb/St/V genomes. (A) Phylogenetic analysis and proportion of the positive Thi-specific markers in Th. elongatum (The), Th. bessarabicum (Thb), Ps. spicata (Ps), and D. villosum (Dv). (B) Number of the negative and positive Thi-specific markers in Th. elongatum, Th. bessarabicum, Ps. spicata, and D. villosum.
Discussion
Thinopyrum intermedium is one of the important resources for the wheat improvement. In this study, 2,019 STS markers distributed on 21 Thi-chromosomes were developed based on the Thi-GBS sequences and used to amplify from Th. intermedium and eight wheat landraces from different ecological regions in China. Many species polymorphisms, including the presence or absence or the length difference of amplicons, were obtained. In order to identify the Thi-specific fragments in the wheat background more accurately, 745 Thi-specific markers with amplicons in Th. intermedium but not in wheat were screened. Due to the homology among the three sub-genomes J/JS/St of Th. intermedium, the developed Thi-specific markers are not exclusively specific to the corresponding sub-genome within the same HG. Using wheat-Th. intermedium introgression lines, 58 out of 82 (71%) Thi-specific markers in Thi-HG4 were identified. However, due to the lack of materials with single Thi-sub-genome introgressed, the Thi-specific markers in the remaining Thi-HGs were not identified.
We used this set of markers to accurately identify the alien chromosomes derived from different Thi-HGs in the wheat-Th. intermedium addition, substitution, and translocation lines. Chromosome 1St, 2JS, 3J, 4J, and 7J have been reported to carry genes for stripe rust resistance (Hu et al., 2011; Li et al., 2017, 2019; Lang et al., 2018). In addition, chromosome 4J also carries genes related to dwarf, tillering, and blue grain (Li et al., 2017). We will then identify whether the introgression lines have obtained beneficial agronomic traits from Th. intermedium and use them to develop small-fragment translocation lines. The Thi-specific markers will be used to track alien fragments and determine the approximate chromosomal location of the target alien gene.
The set of Thi-specific markers developed in this study can be used to identify not only Th. intermedium chromosomes in the wheat background, but also the alien chromosomes from other Triticeae species with J and St genomes, such as Th. ponticum (2n = 10x = 70, JJJJSJS/EeEbExStSt) (Zhang et al., 1996, 2001; Chen et al., 1998). Some Thi-specific markers can amplify species-specific bands in Th. elongatum, Th. bessarabicum, Ps. spicata, and D. villosum. Therefore, these markers can also be suitable for the identification of the alien chromosomes from the above species in the wheat background.
There are several advantages of this set of markers identified in this study. First, they are PCR-based markers, which are easy to use and cost-effective. Second, this set of markers, covering all Th. intermedium chromosomes, are developed based on the GBS markers from the published Th. intermedium genetic map, so each Thi-specific marker has a corresponding map location. Third, they can be used for the chromosome identification after further screening, whereas the current SNP chip and KASP chip cannot accurately identify the J and JS sub-genomes, which is due to the high similarity between the J and JS sub-genomes and the characteristic duality of SNP (Cseh et al., 2019; Grewal et al., 2020). Fourth, the physical positions of Thi-specific markers in Th. intermedium can be determined according to their contigs, so the sequence of small alien fragment in wheat-Th. intermedium translocations can be inferred, which can provide valuable information for further identification of small alien fragments, and even for the cloning of alien genes.
However, this set of markers also has some limitations. For substitution lines and translocation lines, it is impossible to identify which wheat chromosomes have been replaced or translocated onto. Therefore, cytological techniques or wheat chromosome-specific markers are needed for the identification. In addition, the distributions of Thi-specific markers on certain chromosomes are insufficient (such as chromosomes 2JS, 6J, and 6St, Figure 2) or uneven (such as chromosomes 1J and 2St, Figure 2). Thus, the alien Thi-segments that are not covered by markers cannot be detected. Furthermore, for wheat varieties with complex genetic backgrounds, especially containing multiple alien fragments, the accuracy of this set of markers will be affected.
Due to cross-pollination, genetic exchange between Th. intermedium and other species may occur, resulting in a complex evolutionary process and genome composition of Th. intermedium. Since 1936, several genome constitutions of Th. intermedium, such as AXY (Peto, 1936), BEF (Stebbins and Pun, 1953), B2X1X2 (Dewey, 1984), JrJvsSt (Cseh et al., 2019), have been proposed. At present, it is generally believed that the genome constitution of Th. intermedium should be JJSSt based on the GISH results with the St, J, and E genomic DNA probes, among which the St sub-genome is thought to derive from Ps. spicata, whereas the origins of J and JS sub-genomes are still uncertain (Chen et al., 1998; Mahelka et al., 2013). Studies have shown that these sub-genomes are partial homology with Th. elongatum, Th. bessarabicum, and D. villosum, in which the JS sub-genome is also partially recombined with the St genome (Chen et al., 1998; Mahelka et al., 2011, 2013). In this study, 44% of the markers located in the St sub-genome were positive in Ps. spicata, which was much higher than that in Th. elongatum (16%), Th. bessarabicum (7%), and D. villosum (10%), indicating that Thi-St genome has good homology with Ps. spicata genome. The markers of JS sub-genome have a high amplification percentage in D. villosum (29%) and Ps. spicata (25%), which is consistent with the reported GISH results (Mahelka et al., 2011). However, there were also many positive markers from the J sub-genome in Ps. spicata (22%), indicating the complexity of the origin of J sub-genome. In Th. elongatum, the percentage of positive Thi-J markers (17%) was higher than that of the positive Thi-JS markers (9%), which also confirms the feasibility of using The-CDSs to distinguish the J sub-genome from the 21 Thi-LGs.
As many as 366 (49%) of Thi-specific markers failed to amplify in Th. elongatum, Th. bessarabicum, Ps. spicata, and D. villosum, indicating that the Th. intermedium genome has undergone extensive recombination and gradually evolved into a new species after polyploidization by natural hybridization, which is a common phenomenon in nature (Hegarty and Hiscock, 2005). Moreover, there is a possibility that except for the above four species, there may be other species involved in the evolution of Th. intermedium, such as Aegilops tauschii (D genome) and Taeniatherum (Ta genome) (Mahelka et al., 2011). Therefore, the negative Thi-specific markers can be applied to other Triticeae species, which may be able to discover species close to the Th. intermedium genome or involved in the evolution of Th. intermedium.
Data Availability Statement
The original contributions presented in the study are included in the article/Supplementary Material, further inquiries can be directed to the corresponding author/s.
Author Contributions
ZYa and LQ designed the experiments. LQ developed the STS markers. ZYa and PZ provided the wheat-Th. intermedium introgression lines. SLiu, SLi, and JLiu performed the PCR experiments. JLi, ZYu, and PZ conducted the GISH and FISH experiments. CL, XL, and YR helped with data analysis. LQ and JLi wrote the manuscript. PZ, ZYa, XZ, and ZC revised the manuscript. All authors contributed to the article and approved the submitted version.
Funding
This work was funded by the National Key Research & Development Project (2016YFD0102004-07), the Key Science & Technology Project in Shanxi Province (201903D211003-2), the National Natural Science Foundation of China (31971886), the Grains Research and Development Corporation (GRDC) of Australia (9176057), the Shanxi Academy of Agricultural Sciences Project (YCX2018D2YS01), and the Taishan Scholars Project (tsqn201812123).
Conflict of Interest
The authors declare that the research was conducted in the absence of any commercial or financial relationships that could be construed as a potential conflict of interest.
Acknowledgments
We are grateful to Dr. Hongjie Li of Chinese Academy of Agricultural Sciences for reviewing the manuscript, Dr. Jian Ma of Sichuan Agricultural University for providing seeds of Th. elongatum, and Changbiao Wang and Jinlong Yan of Shanxi Agricultural University for providing Perl script for designing primers and seeds of wheat landraces, respectively.
Supplementary Material
The Supplementary Material for this article can be found online at: https://www.frontiersin.org/articles/10.3389/fpls.2021.685216/full#supplementary-material
Footnotes
References
Bao, Y., Li, X., Liu, S., Cui, F., and Wang, H. (2010). Molecular cytogenetic characterization of a new wheat-Thinopyrum intermedium partial amphiploid resistant to powdery mildew and stripe rust. Cytogenet. Genome Res. 126, 390–395. doi: 10.1159/000266169
Bao, Y., Wu, X., Zhang, C., Li, X., He, F., Qi, X., et al. (2014). Chromosomal constitutions and reactions to powdery mildew and stripe rust of four novel wheat-Thinopyrum intermedium partial amphiploids. J. Genet. Genomics 41, 663–666. doi: 10.1016/j.jgg.2014.11.003
Chang, Z. J., Zhang, X. J., Yang, Z. J., Zhan, H. X., Li, X., Liu, C., et al. (2010). Characterization of a partial wheat-Thinopyrum intermedium amphiploid and its reaction to fungal diseases of wheat. Hereditas 147, 304–312. doi: 10.1111/j.1601-5223.2010.02156.x
Chen, Q. (2005). Detection of alien chromatin introgression from Thinopyrum into wheat using S genomic DNA as a probe-A landmark approach for Thinopyrum genome research. Cytogenet. Genome Res. 109, 350–359. doi: 10.1159/000082419
Chen, Q., Conner, R. L., Laroche, A., Ji, W. Q., Armstrong, K. C., and Fedak, G. (1999). Genomic in situ hybridization analysis of Thinopyrum chromatin in a wheat-Th. intermedium partial amphiploid and six derived chromosome addition lines. Genome 42, 1217–1223. doi: 10.1139/g99-090
Chen, Q., Conner, R. L., Laroche, A., and Thomas, J. B. (1998). Genome analysis of Thinopyrum intermedium and Thinopyrum ponticum using genomic in situ hybridization. Genome 41, 580–586. doi: 10.1139/g98-055
Cseh, A., Yang, C., Hubbart-Edwards, S., Scholefield, D., Ashling, S. S., Burridge, A. J., et al. (2019). Development and validation of an exome-based SNP marker set for identification of the St, Jr and Jvs genomes of Thinopyrym intermedium in a wheat background. Theor. Appl. Genet. 132, 1555–1570. doi: 10.1007/s00122-019-03300-9
Dewey, D. R. (1984). “The genomics system of classification as a guide to intergeneric hybridization with perennial Triticeae,” in Gene Manipulation in Plant Improvement. Stadler Genetics Symposia Series, ed J. P. Gustafson (Boston, MA: Springer), 209–279.
Forster, B. P., Reader, S. M., Forsyth, S. A., Koebner, R. M. D., Miller, T. E., Gale, M. D., et al. (1987). An assessment of the homoeology of six Agropyron intermedium chromosomes added to wheat. Genet. Res. 50, 91–97. doi: 10.1017/S001667230002348X
Friebe, B., Mukai, Y., Gill, B. S., and Cauderon, Y. (1992). C-banding and in-situ hybridization analyses of Agropyron intermedium, a partial wheat × Ag. intermedium amphiploid, and six derived chromosome addition lines. Theor. Appl. Genet. 84, 899–905. doi: 10.1007/BF00227402
Fu, S., Chen, L., Wang, Y., Li, M., Yang, Z., Qiu, L., et al. (2015). Oligonucleotide probes for ND-FISH analysis to identify rye and wheat chromosomes. Sci. Rep. 5:10552. doi: 10.1038/srep10552
Grewal, S., Hubbart-Edwards, S., Yang, C., Devi, U., Baker, L., Heath, J., et al. (2020). Rapid identification of homozygosity and site of wild relative introgressions in wheat through chromosome-specific KASP genotyping assays. Plant Biotechnol. J. 18, 743–755. doi: 10.1111/pbi.13241
Han, B., Wang, C., Tang, Z., Ren, Y., Li, Y., Zhang, D., et al. (2015). Genome-wide analysis of microsatellite markers based on sequenced database in Chinese spring wheat (Triticum aestivum L.). PLoS ONE 10:e0141540. doi: 10.1371/journal.pone.0141540
Hegarty, M. J., and Hiscock, S. J. (2005). Hybrid speciation in plants: new insights from molecular studies. New Phytol. 165, 411–423. doi: 10.1111/j.1469-8137.2004.01253.x
Hu, L. J., Li, G. R., Zeng, Z. X., Chang, Z. J., Liu, C., Zhou, J. P., et al. (2011). Molecular cytogenetic identification of a new wheat-Thinopyrum substitution line with stripe rust resistance. Euphytica 177, 169–177. doi: 10.1007/s10681-010-0216-x
Kantarski, T., Larson, S., Zhang, X., DeHaan, L., Borevitz, J., Anderson, J., et al. (2017). Development of the first consensus genetic map of intermediate wheatgrass (Thinopyrum intermedium) using genotyping-by-sequencing. Theor. Appl. Genet. 130, 1–14. doi: 10.1007/s00122-016-2799-7
Lang, T., La, S., Li, B., Yu, Z., Chen, Q., Li, J., et al. (2018). Precise identification of wheat- Thinopyrum intermedium translocation chromosomes carrying resistance to wheat stripe rust in line Z4 and its derived progenies. Genome 61, 177–185. doi: 10.1139/gen-2017-0229
Li, G., Lang, T., Dai, G., Li, D., Li, C., Song, X., et al. (2015). Precise identification of two wheat-Thinopyrum intermedium substitutions reveals the compensation and rearrangement between wheat and Thinopyrum chromosomes. Mol. Breed. 35:1. doi: 10.1007/s11032-015-0202-z
Li, G., Wang, H., Lang, T., Li, J., La, S., Yang, E., et al. (2016). New molecular markers and cytogenetic probes enable chromosome identification of wheat-Thinopyrum intermedium introgression lines for improving protein and gluten contents. Plant 244, 865–876. doi: 10.1007/s00425-016-2554-y
Li, G., Zhang, T., Yu, Z., Wang, H., Yang, E., and Yang, Z. (2021). An efficient oligo-FISH painting system for revealing chromosome rearrangements and polyploidization in Triticeae. Plant J. 105, 978–993. doi: 10.1111/tpj.15081
Li, H., and Wang, X. (2009). Thinopyrum ponticum and Th. intermedium: the promising source of resistance to fungal and viral diseases of wheat. J. Genet. Genomics 36, 557–565. doi: 10.1016/S1673-8527(08)60147-2
Li, J., Chen, Q., Zhang, P., Lang, T., Hoxha, S., Li, G., et al. (2019). Comparative FISH and molecular identification of new stripe rust resistant wheat-Thinopyrum intermedium ssp. trichophorum introgression lines. Crop J. 7, 819–829. doi: 10.1016/j.cj.2019.06.001
Li, J., Lang, T., Li, B., Yu, Z., Wang, H., Li, G., et al. (2017). Introduction of Thinopyrum intermedium ssp. trichophorum chromosomes to wheat by trigeneric hybridization involving Triticum, Secale and Thinopyrum genera. Planta 245, 1121–1135. doi: 10.1007/s00425-017-2669-9
Lukaszewski, A. J., Alberti, A., Sharpe, A., Kilian, A., Stanca, A. M., Keller, B., et al. (2014). A chromosome-based draft sequence of the hexaploid bread wheat (Triticum aestivum) genome. Science 345:1251788. doi: 10.1126/science.1251788
Luo, R., Liu, B., Xie, Y., Li, Z., Huang, W., Yuan, J., et al. (2012). SOAPdenovo2: an empirically improved memory-efficient short-read de novo assembler. Gigascience 1:18. doi: 10.1186/2047-217X-1-18
Mahelka, V., Kopeck, D., and Patová, L. (2011). On the genome constitution and evolution of intermediate wheatgrass (Thinopyrum intermedium: Poaceae, Triticeae). BMC Evol. Biol. 11:127. doi: 10.1186/1471-2148-11-127
Mahelka, V., Kopecký, D., and Baum, B. R. (2013). Contrasting patterns of evolution of 45S and 5S rDNA families uncover new aspects in the genome constitution of the agronomically important grass Thinopyrum intermedium (Triticeae). Mol. Biol. Evol. 30, 2065–2086. doi: 10.1093/molbev/mst106
Peto, F. H. (1936). Hybridization of Triticum and Agropyron: II. cytology of the male parents and F1 generation. Can. J. Res. 14, 203–214. doi: 10.1139/cjr36c-017
Salina, E. A., Adonina, I. G., Badaeva, E. D., Kroupin, P. Y., Stasyuk, A. I., Leonova, I. N., et al. (2015). A Thinopyrum intermedium chromosome in bread wheat cultivars as a source of genes conferring resistance to fungal diseases. Euphytica 204, 91–101. doi: 10.1007/s10681-014-1344-5
Song, X., Li, G., Zhan, H., Liu, C., and Yang, Z. (2013). Molecular identification of a new wheat-Thinopyrum intermedium ssp. trichophorum addition line for resistance to stripe rust. Cereal Res. Commun. 41, 211–220. doi: 10.1556/CRC.2013.0009
Stebbins, G. L., and Pun, F. T. (1953). Artificial and natural hybrids in the gramineae, tribe hordeae. VI. chromosome pairing in Secale cereale × Agropyron intermedium and the problem of genome homologies in the Triticinae. Genetics 38, 600–608. doi: 10.1093/genetics/38.6.600
Tamura, K., Stecher, G., Peterson, D., Filipski, A., and Kumar, S. (2013). MEGA6: molecular evolutionary genetics analysis version 6.0. Mol. Biol. Evol. 30, 2725–2729. doi: 10.1093/molbev/mst197
Tang, Z., Yang, Z., and Fu, S. (2014). Oligonucleotides replacing the roles of repetitive sequences pAs1, pSc119.2, pTa-535, pTa71, CCS1, and pAWRC.1 for FISH analysis. J. Appl. Genet. 55, 313–318. doi: 10.1007/s13353-014-0215-z
Tsitsin, N. V. (1965). Remote hybridisation as a method of creating new species and varieties of plants. Euphytica 14, 326–330. doi: 10.1007/BF00149519
Vogel, K. P., and Jensen, K. J. (2001). Adaptation of perennial triticeae to the eastern Central Great Plains. J. Range Manage. 54, 674–679. doi: 10.2307/4003670
Wang, H., Sun, S., Ge, W., Zhao, L., Hou, B., Wang, K., et al. (2020). Horizontal gene transfer of Fhb7 from fungus underlies Fusarium head blight resistance in wheat. Science 368:5435. doi: 10.1126/science.aba5435
Wang, H., Yu, Z., Li, G., and Yang, Z. (2019). Diversified chromosome rearrangements detected in a wheat-Dasypyrum breviaristatum substitution line induced by Gamma-ray irradiation. Plants 8:175. doi: 10.3390/plants8060175
Wang, R. R. C., Li, X., Robbins, M. D., Larson, S. R., Bushman, S. B., Jones, T. A., et al. (2020). DNA sequence-based mapping and comparative genomics of the St genome of Pseudoroegneria spicata (Pursh) a. love versus wheat (Triticum aestivum L.) and barley (Hordeum vulgare L.). Genome 63, 445–457. doi: 10.1139/gen-2019-0152
Xi, W., Tang, Z., Tang, S., Yang, Z., Luo, J., and Fu, S. (2019). New ND-FISH-positive oligo probes for identifying Thinopyrum chromosomes in wheat backgrounds. Int. J. Mol. Sci. 20:2031. doi: 10.3390/ijms20082031
Yang, Z., Li, G., Chang, Z., Zhou, J, and Ren, Z. (2006). Characterization of a partial amphiploid between Triticum aestivum cv. Chinese Spring and Thinopyrum intermedium ssp. trichophorum. Euphytica 149, 11–17. doi: 10.1007/s10681-005-9010-6
Yu, Z., Wang, H., Xu, Y., Li, Y., Lang, T., Yang, Z., et al. (2019). Characterization of chromosomal rearrangement in new wheat-Thinopyrum intermedium addition lines carrying Thinopyrum-specific grain hardness genes. Agronomy 9:18. doi: 10.3390/agronomy9010018
Zhan, H., Zhang, X., Li, G., Pan, Z., Hu, J., Li, X., et al. (2015). Molecular characterization of a new wheat-Thinopyrum intermedium translocation line with resistance to powdery mildew and stripe rust. Int. J. Mol. Sci. 16, 2162–2173. doi: 10.3390/ijms16012162
Zhang, P., Friebe, B., Lukaszewski, A. J., and Gill, B. S. (2001). The centromere structure in Robertsonian wheat-rye translocation chromosomes indicates that centric breakage-fusion can occur at different positions within the primary constriction. Chromosoma 110, 335–344. doi: 10.1007/s004120100159
Zhang, X., Cui, C., Bao, Y., Wang, H., and Li, X. (2020). Molecular cytogenetic characterization of a novel wheat-Thinopyrum intermedium introgression line tolerant to phosphorus deficiency. Crop J. doi: 10.1016/j.cj.2020.08.014
Keywords: Thinopyrum intermedium, STS markers, specificity, chromosome identification, physical location
Citation: Qiao L, Liu S, Li J, Li S, Yu Z, Liu C, Li X, Liu J, Ren Y, Zhang P, Zhang X, Yang Z and Chang Z (2021) Development of Sequence-Tagged Site Marker Set for Identification of J, JS, and St Sub-genomes of Thinopyrum intermedium in Wheat Background. Front. Plant Sci. 12:685216. doi: 10.3389/fpls.2021.685216
Received: 24 March 2021; Accepted: 14 May 2021;
Published: 23 June 2021.
Edited by:
István Molnár, Hungarian Academy of Sciences (MTA), HungaryReviewed by:
Mahmoud Said, Academy of Sciences of the Czech Republic, CzechiaSurinder Banga, Punjab Agricultural University, India
Copyright © 2021 Qiao, Liu, Li, Li, Yu, Liu, Li, Liu, Ren, Zhang, Zhang, Yang and Chang. This is an open-access article distributed under the terms of the Creative Commons Attribution License (CC BY). The use, distribution or reproduction in other forums is permitted, provided the original author(s) and the copyright owner(s) are credited and that the original publication in this journal is cited, in accordance with accepted academic practice. No use, distribution or reproduction is permitted which does not comply with these terms.
*Correspondence: Zujun Yang, yangzujun@uestc.edu.cn; Xiaojun Zhang, zxjemail@163.com; Peng Zhang, peng.zhang@sydney.edu.au
†These authors have contributed equally to this work