- 1Institute of Grassland Research, Chinese Academy of Agricultural Sciences, Hohhot, China
- 2College of Grassland Science, Qingdao Agricultural University, Qingdao, China
- 3College of Grassland Science, Shanxi Agricultural University, Taigu, China
Shoot defoliation by grazers or mowing can affect root traits of grassland species, which may subsequently affect its aboveground traits and ecosystem functioning (e.g., aboveground primary production). However, experimental evidence for such reciprocal feedback between shoots and roots is limited. We grew the perennial grass Leymus chinensis–common across the eastern Eurasian steppe–as model species in a controlled-hydroponics experiment, and then removed half of its shoots, half of its roots, or a combination of both. We measured a range of plant aboveground and belowground traits (e.g., phenotypic characteristics, photosynthetic traits, root architecture) in response to the shoot and/or root removal treatments. We found the regenerated biomass was less than the lost biomass under both shoot defoliation and root severance, generating a under-compensatory growth. Root biomass was reduced by 60.11% in the defoliation treatment, while root severance indirectly reduced shoot biomass by 40.49%, indicating a feedback loop between shoot and root growth. This defoliation-induced shoot–root feedback was mediated by the disproportionate response and allometry of plant traits. Further, the effect of shoot defoliation and root severance on trait plasticity of L. chinensis was sub-additive. That is, the combined effects of the two treatments were less than the sum of their independent effects, resulting in a buffering effect on the existing negative influences on plant persistence by increased photosynthesis. Our results highlight the key role of trait plasticity in driving shoot–root reciprocal feedbacks and growth persistence in grassland plants, especially perennial species. This knowledge adds to earlier findings of legacy effects and can be used to determine the resilience of grasslands.
Introduction
Grasslands that are dominated by perennial species, which cover the largest terrestrial land area worldwide (Gibson, 2009), are commonly used by livestock grazing or mowing for haymaking and provide essential services for human (Dixon et al., 2014; Fetzel et al., 2017). In recent decades, a pressing challenge for ecologists is to understand the impacts of intensifying land use on biodiversity conservation and ecosystem dynamics, in particular, the overgrazing and frequent mowing of grasslands (Lü et al., 2012; Wang B. et al., 2020). With both grazing and mowing, the removal of plant shoot tissue (i.e., “defoliation”) is the main mechanism underlying the effects on plant performance and ecosystem function of grasslands (Liu et al., 2015; Lezama and Paruelo, 2016). Numerous studies have described the short-term responses to defoliation, these include individual growth (Ferraro and Oesterheld, 2002; Zhang Z. et al., 2020), physiological-biochemical characteristics (Loaiza et al., 2017; Liu et al., 2019), and biomolecular processes (Wan et al., 2015). However, knowledge on the legacy effects of defoliation (i.e., indirect responses) is limited, and is essential to improving plant persistence and grassland sustainability (Tsuzuki et al., 2020).
Legacy effect is defined as the persistence of impacts of a certain ecological event on ecosystem processes after the activity ceased (Cuddington, 2011; Kaisermann et al., 2017). Since the early 1990s, a growing number of studies have examined legacy effects in the field during ecosystem succession, plant invasion, ecosystem engineering, and human-induced land use change (Cuddington, 2011). Possible mechanisms of legacy effects on plant performance detected in previous studies may broadly involve either one or three major drivers: soil abiotic properties (Barthelemy et al., 2019), feedback of soil microbiome (Veen et al., 2014; Wang X. et al., 2020), and/or plant maternal effects (Ren et al., 2017; Yin et al., 2020). First, soil abiotic factors, such as nutrient availability, pH, and physical properties of soil substrates, can be influenced by plant species or ecological events and strongly affect plant performance (Chen Q. et al., 2018; Barthelemy et al., 2019). Second, the microbiome of plant tissues and soil are sensitive to external disturbance and can produce strong effects on plant performance via plant–soil and plant–phyllosphere feedback (Whitaker et al., 2017; Chen T. et al., 2018). Third, plant maternal effect mediated by epigenetic inheritance and changes in seed or bud quality is essential for subsequent plant growth and plant species resilience (Ren et al., 2017; Rendina González et al., 2018; Yin et al., 2019). In natural grasslands, plant communities are commonly dominated by perennial species (Benson and Hartnett, 2006). Therefore, in addition to the three major drivers described above, the feedback loop between shoot and root plasticity may be essential for determining the growth and persistence of perennial plant species.
Defoliation-related trait plasticity plays a central regulatory role in plant fitness, population development, and ecosystem function (Cruz et al., 2010; Leuschner et al., 2013; Li et al., 2015). The mechanism underlying the trait plasticity is the so-called compensation effect, in which plants can accelerate their growth to compensate the losses in performance induced by defoliation (Anten et al., 2003; van Staalduinen and Anten, 2005). However, in response to defoliation, plants can have under-, equal-, and over-compensatory growth according to the quantitative relationship between regenerated and removed biomass (van der Graaf et al., 2005; Ma et al., 2020). In consequence, the magnitude of phenotypic plasticity is dependent on defoliation intensity, and shifts in these traits may alter photosynthetic function and the regeneration capacity of tissue (Shen et al., 2019; Wang et al., 2019; Yuan et al., 2020). Additionally, defoliation may also influence the root architecture and biomass production mediated by photosynthate reallocation (Liu et al., 2018). It is well known that high-intensity defoliation would be more detrimental for root growth (Dawson et al., 2004). Meanwhile, some studies suggested that the responses of leaf and root traits related to defoliation are uncoupled (Kirkegaard et al., 2015; Erktan et al., 2018). These uncertainties in the root response may be due to the variations in species and the kind of functional traits measured (Husáková et al., 2018). Considering the feedback loop between shoot and root, our understanding on how shoot performance is affected by defoliation-induced root plasticity remains limited.
Plant traits can reflect the general response and trade−offs (or coordination) of the ecological function of environmental fluctuations (Abalos et al., 2018; van der Merwe et al., 2021). Thus, a trait-based approach allows a better understanding of how plants respond to external disturbance (Garnier and Navas, 2012). We conducted a controlled-hydroponics experiment to investigate the interactions between shoot defoliation and root severance of Leymus chinensis and their effects on its shoot and root traits. The perennial grass L. chinensis was selected as the focal species because it is a common forage species and has widely distributed across the eastern Eurasian temperate steppe (Liu et al., 2018). Specifically, to investigate defoliation-induced legacy effects on plant performance due to the changes in root trait, we asked three questions: (1) How do functional traits of shoots and roots differentially respond to defoliation? (2) How do shoot performances feedback to root plasticity? (3) Is the effect of shoot defoliation and root severing on trait variation additive or non-additive? Answering these questions can improve our understanding of the grazing stress tolerance and interannual stability of this important forage species.
Materials and Methods
Focal Species
Leymus chinensis is a perennial species in the family Gramineae possessing a rhizomatous root system and largely reproduces via clonal buds, leading to its extensive spread, through which it often forms large monocultures (Bai et al., 2009). It is abundant in many important grazing ecosystems across the eastern Eurasian steppe (Li et al., 2015), which includes the outer Baikal area of Russia, the People’s Republic of Mongolia, and the northern grasslands of China. Owing to its excellent stress tolerance, L. chinensis is found across a broad temperature and precipitation gradient. In addition, it is highly palatable to grazing livestock and is frequently used to make hay. L. chinensis is also a desirable species for reseeding in degraded grassland because of its rapid rhizomatous propagation (Liu and Han, 2008).
Experimental Design
The experiment was conducted in a growth chamber, and L. chinensis was grown via the hydroponics. This method allows us to remove roots easily and to detect how simulated root plasticity influence the plant performance. The following four treatments were used in our experiment (Supplementary Figure 1): (i) control (CK); (ii) shoot defoliation (SD1/2cut, 50% leaf removal); (iii) root severance (RS1/2cut, removal of 50% of the roots from the base of the root system); and (iv) shoot defoliation and root severance (SR1/2cut removal of 50% of leaves and roots).
For our experiment, L. chinensis seeds were from the same genotype and collected from a well-watered common garden at the Chinese Academy of Agricultural Sciences’ Shaerqin Research Station (40°34′N, 111°56′E, 1,040 m a.s.l.) at Hohhot, Inner Mongolia of China, to ensure that their maternal environment was consistent. Plump seeds were surface-sterilized by soaking in 2% sodium hypochlorite (w/v) for 25 min. L. chinensis seeds germinated approximately 10 days after sowing each seed into 9 cm deep soil plugs. To ensure consistency, we selected healthy, 20-day-old individuals with the same initial growth and development status for the hydroponics experiment with Hoagland nutrient solution (Ren et al., 2017). In each hydroponics container (10-cm diameter × 20-cm height), we transplanted four L. chinensis individuals and arranged them symmetrically along the edge of the containers. The hydroponics experiments were conducted in a plant growth chamber set to a photoperiod of 14 h/10 h (light/dark), temperatures of 25°C in the light and 15°C in the dark phases, and a light intensity of 500 μmol m–2 s–1. The nutrient solution was replaced every 3 days throughout the duration of the experiment.
In total, we conducted two cycles’ of shoot defoliation and root severance treatments. Specifically, the first and second treatment cycles were conducted on the 15th and 30th day after transplanting L. chinensis seedlings, respectively, for the SD1/2cut, RS1/2cut, and SR1/2cut treatments. For the shoot defoliation treatment, half of each leaf was cut off along the middle of the leaf (i.e., removing half of the leaf widthwise) using a pair of scissors (Supplementary Figure 1). For the root severance treatment, the fibrous roots of each L. chinensis individual were divided into two symmetrical halves and randomly removed a selected half (Supplementary Figure 1). During the experiment, the removed leaves and roots of L. chinensis were collected and oven-dried at 70°C for 72 h for the calculation of biomass accumulation.
Measurements
During the second treatment cycle, we measured the vertical height, relative chlorophyll value, and photosynthetic traits (e.g., net photosynthetic rate and leaf respiration rate) of leaves every 5 days; that is, in total, these parameters were measured four times. At the end of the second treatment cycle (45 days after transplanting), we measured leaf and root phenotypic traits (e.g., total root length, total root surface area) of L. chinensis. Roots and shoots of 65-day-old experimental L. chinensis (20 days after germination in soil plugs and 45 days in hydroponic containers) were separately harvested and oven-dried at 70°C for 72 h for subsequent measurement of biomass.
To measure the net photosynthetic rate, a LI–6400XT portable photosynthesis system (LI-COR, Lincoln, NE, United States) was used at light saturation (1,000 μmol m–2 s–1) (Ren et al., 2017). The respiration rates of leaves were measured at 0 μmol m–2 s–1 after 30 min of zero irradiance on the same leaf (Ayub et al., 2014). We calculated the gross photosynthetic rate as the difference between the value of the net photosynthetic rate and the respiration rate. The relative chlorophyll values were measured using a SPAD-502 Plus portable chlorophyll meter (Konica Minolta Inc., Japan).
Using the portable leaf area meter CI-202 (CID, Walz, Camas, WA, United States), leaf phenotypic traits, such as leaf area, leaf length, leaf width, and leaf perimeter, were measured. Each measured leaf was precisely weighted to 0.1 mg accuracy (Shimadzu Inc., Japan). Additionally, root morphology, including total root length, total root surface area, total root volume, average root diameter, root tip number, root tip forks, and root tip crossings were measured using a WinRhizoTM scanner-based system (Regent Instruments Inc., Quebec, ON, Canada). The samples showed no signs of fragmentation because the use of hydroponic containers enabled the harvest of intact root systems.
Calculation and Statistics
In the CK, SD1/2cut, RS1/2cut, and SR1/2cut treatments, the growth rates were represented by the average slopes of plant height along a time series using a linear regression analysis. The accumulated biomass of shoots and roots were the sums of the remaining biomass and the corresponding mass of removed leaves and roots. We calculated specific leaf area using the recorded single leaf mass and leaf area. Similarly, the specific root length was calculated using root biomass and total root length. In addition, respiration consumption was the proportion of the respiration rate in the gross photosynthetic rate of leaves.
The extent of trait variation within each treatment was represented by the coefficient of variation of trait values in each of the shoot and root traits (Zhang B. et al., 2020). In addition, to compare the sensitivity (i.e., trait plasticity) of various shoot and root traits to SD1/2cut, RS1/2cut, and SR1/2cut, we calculated all the parameters using Log response ratios (LRR). The LRR was calculated as follows:
where Tcontrol represented the trait values in the control and Ttreatment represented the trait values in the other three treatments.
To detect the additive effect of SD1/2cut and RS1/2cut, moreover, we calculated the predicted LRRs (i.e., LRRpredicted) of SR1/2cut by summing the corresponding LRRs in the treatments of SD1/2cut and RS1/2cut. They were additive effects when there were significant differences (P < 0.05) between the observed (LRRs in treatment of SR1/2cut, LRRobserved) and predicted (LRRpredicted) values. Meanwhile, the relative change ratios (RCs) of LRRs between LRRobserved and LRRpredicted were calculated as follows:
The allometric scaling among some key phenotypic traits of L. chinensis shoots and roots was assessed using the package “Standardized Major Axis Tests and Routines [(S)MATR].” Model Type II regression was used to determine the slope (a = scaling exponent) and y-intercept (log10b, where b is the allometric constant) of the log-log linear relationship. When the regressions under comparison had common slopes but different y-intercepts, the difference in the y-intercepts was inferred to underlie significant differences between the common slopes obtained under different treatments of CK, SD1/2cut, RS1/2cut, and SR1/2cut.
Kolmogorov–Smirnov tests were used to assess the assumption of normality of the data such as remaining and accumulated biomass, leaf and root phenotypic traits, and photosynthetic characteristics. Using IBM SPSS Statistics 25 (IBM Corporation, Armonk, NY, United States), differences in these indicators under different treatments were evaluated by a one-way analysis of variance (ANOVA). Meanwhile, the interactive effects of treatments and measurement time were evaluated by two-way ANOVA. Pearson’s correlation coefficient was used to evaluate the possible relationship between different indicators.
Results
Growth Rate and Shoot/Root Biomass Production
Both remaining and accumulated biomass were negatively influenced by SD1/2cut, RS1/2cut, and SR1/2cut (P < 0.05, Figure 1, Supplementary Figure 2, and Supplementary Table 1). The biomass accumulation was lower in RS1/2cut than SD1/2cut (P < 0.05; Figure 1). Compared to the roots, biomass accumulations of shoots had lower and higher reductions under SD1/2cut and RS1/2cut, respectively, leading to a slight decrease (P > 0.05) and a significant increase (P < 0.05) in the root-to-shoot ratios respectively, (Figure 1). The remaining biomass was largely dependent on the proportion of defoliated or severed biomass in shoots (P < 0.05), not roots (P > 0.05, Supplementary Figure 3). Individual growth rate significantly varied among the treatments (P < 0.05), especially resulting in a significant increase in SD1/2cut (P < 0.05, Figure 2 and Supplementary Figure 4). The growth rates, represented by slopes of plant height along a time series, were correlated with final (P < 0.05) rather than initial (P = 0.19) plant height (Supplementary Figure 5). In addition, a significant decrease in the major root and shoot phenotypic traits was observed for SD1/2cut, RS1/2cut, and SR1/2cut treatments (P < 0.05), except for specific leaf area and specific root length (Supplementary Figures 6, 7 and Supplementary Table 2). Notably, specific leaf area increased with increasing root severance rather than shoot defoliation (P < 0.05, Supplementary Figure 6), whereas specific root length increased with increasing shoot defoliation but not by root severance (P < 0.05, Supplementary Figure 7).
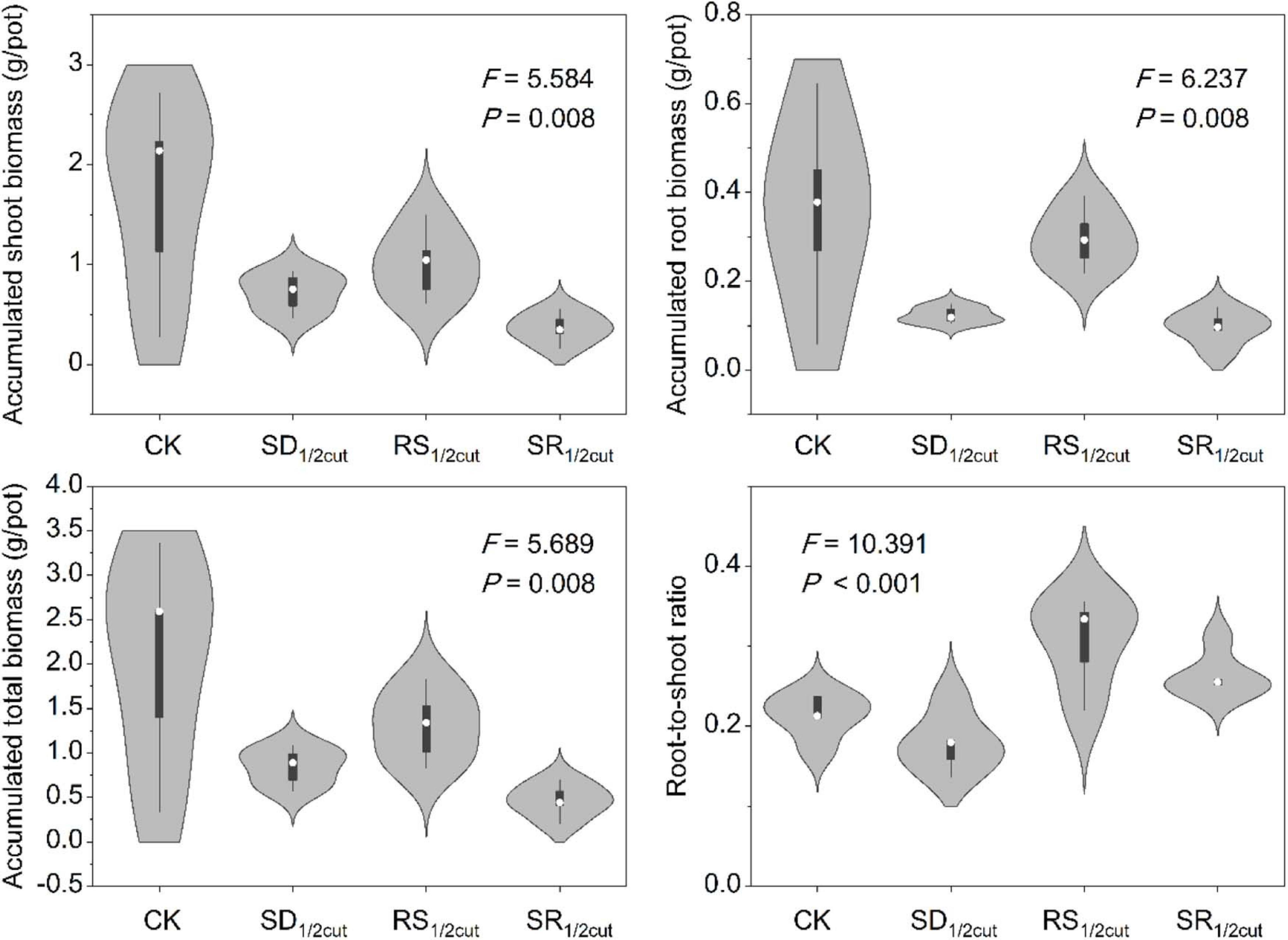
Figure 1. Effects of shoot defoliation and root severing on biomass accumulation and allocation of Leymus chinensis. CK, control; SD1/2cut, shoot defoliation; RS1/2cut, root severing; SR1/2cut, shoot defoliation and root severance.
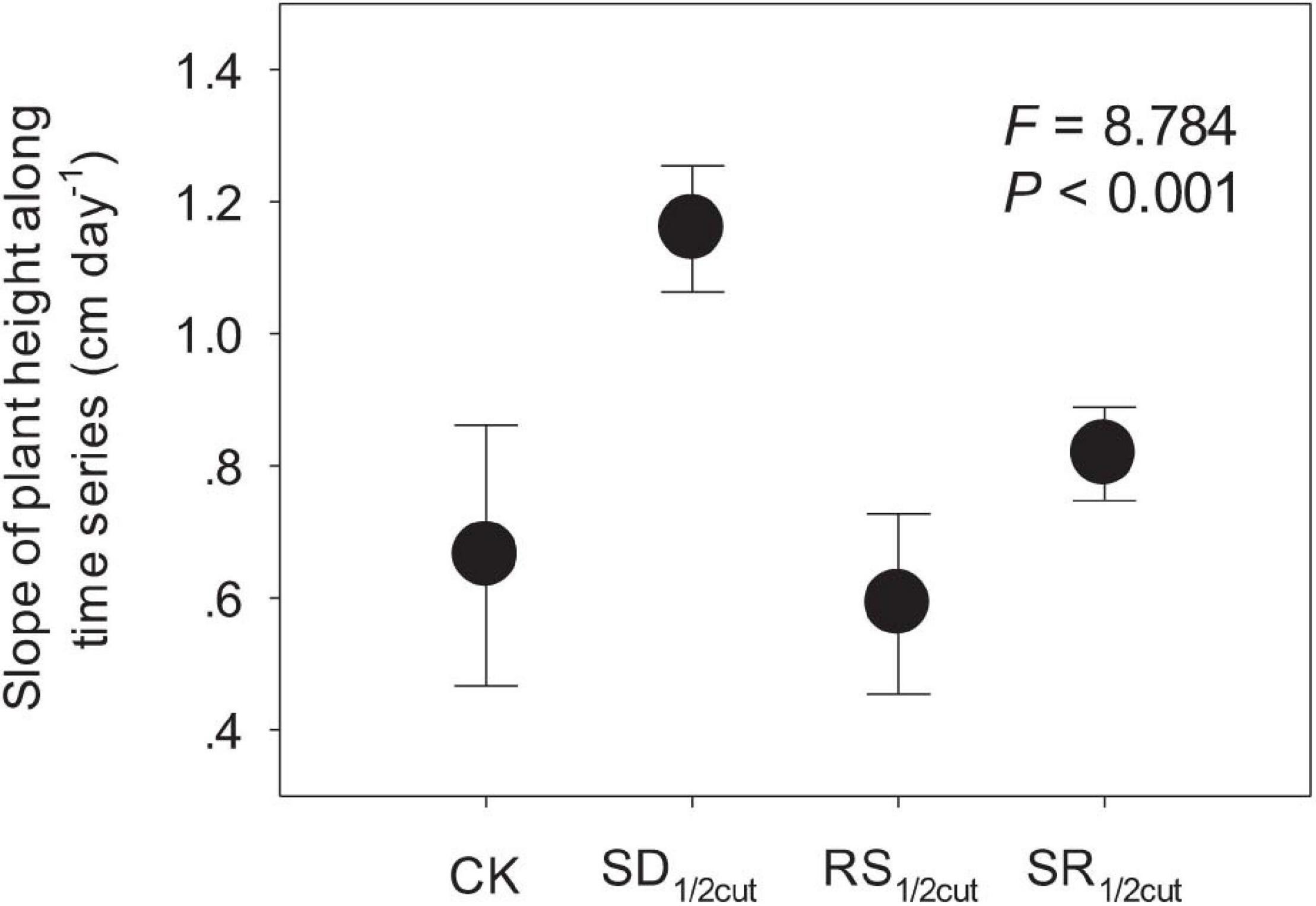
Figure 2. Effects of shoot defoliation and root severance on the growth rate (the slope of plant height along time series) of Leymus chinensis. CK, control; SD1/2cut, shoot defoliation; RS1/2cut, root severing; SR1/2cut, shoot defoliation and root severance.
LRR and Trait Variations
Biomass and phenotypic traits of L. chinensis were negatively influenced by SD1/2cut and RS1/2cut (P < 0.05, Supplementary Tables 1, 2) treatments, with differences in sensitivity ranging more than 10-fold (Figure 3). Biomass-related indicators and root traits had higher LRRs than shoot phenotypic in the SD1/2cut treatment compared to the control (Figure 3A). In contrast, negative LRRs were obtained for root traits in the RS1/2cut treatment, followed by biomass-related indicators and shoot phenotypic traits (Figure 3B). Moreover, the interactive effects of SD1/2cut and RS1/2cut were relatively weak (P > 0.05), except for specific root length (P = 0.003, Supplementary Tables 1, 2). Notably, SR1/2cut resulted in higher LRRs of all indicators than in both the SD1/2cut and RS1/2cut treatments (Figure 3C). In response to SR1/2cut, the sensitivities among L. chinensis indicators were as follows: root phenotypic traits > biomass-related indicators > shoot phenotypic traits (Figure 3C). The observed LRRs of SR1/2cut were significantly correlated with predicted LRRs (P < 0.05), which were calculated by determining the LRRs of SD1/2cut and RS1/2cut (Supplementary Figure 8). However, the relative change ratios between observed and predicted LRRs dramatically varied with plant trait and were less than 1.0 in the majority of measured indicators, except for the three root traits (Figure 4). Additionally, trait variations within each treatment were contrastingly different among various shoot and root traits (Supplementary Table 3) and were highly correlated with trait plasticity (P < 0.01, Figure 5).
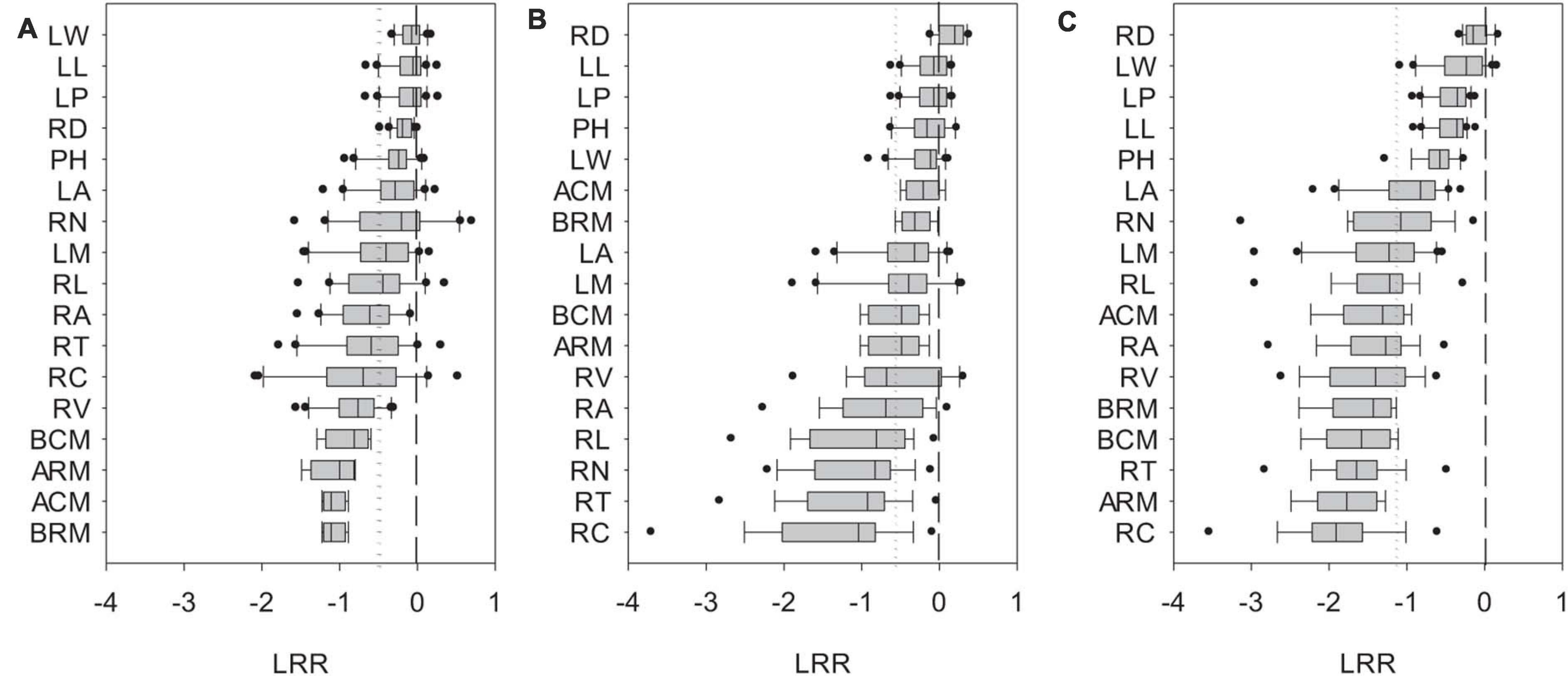
Figure 3. Ln-based response ratios (LRR) of shoot and root phenotypic traits of Leymus chinensis in response to (A) shoot defoliation, (B) root severing, and (C) shoot defoliation and root severance. Short and long dotted lines represent the mean value of response ratios of various phenotypic traits and the zero line. PH, plant height; LM, single leaf weight; LA, leaf area; LL, leaf length; LW, leaf width; LP, leaf perimeter; RL, total root length; RA, total root surface area; RV, total root volume; RD, average root diameter; RN, root tip number; RT, root tip forks; RC, root tip crossings; ARM, remaining aboveground biomass; BRM, remaining belowground biomass; BCM, aboveground biomass accumulation; ACM, belowground biomass accumulation.
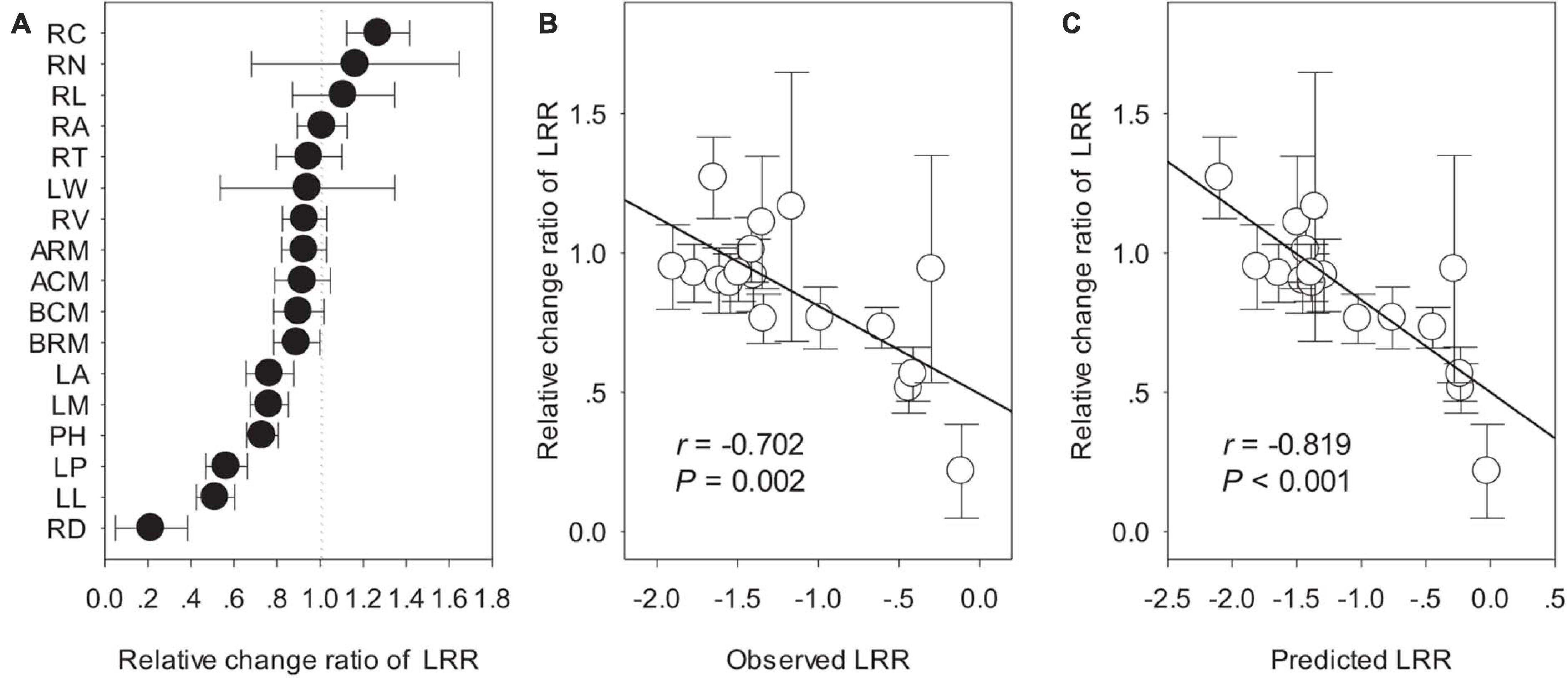
Figure 4. The relative change of ln response ratios (LRRs) between observed and predicted values: (A) ordering of relative change of LRRs; (B) linear relationship of observed values with the relative change of LRRs; (C) linear relationship of predicted values with the relative change of LRR. The observed values were the LRRs in the treatment of shoot defoliation and root severing (SR1/2cut), whereas predicted values were calculated by the LRRs in separate treatments of shoot defoliation (SD1/2cut) and root severing (RS1/2cut). PH, plant height; LM, single leaf weight; LA, leaf area; LL, leaf length; LW, leaf width; LP, leaf perimeter; RL, total root length; RA, total root surface area; RV, total root volume; RD, average root diameter; RN, root tip number; RT, root tip forks; RC, root tip crossings; ARM, remaining aboveground biomass; BRM, remaining belowground biomass; BCM, aboveground biomass accumulation; ACM, belowground biomass accumulation.
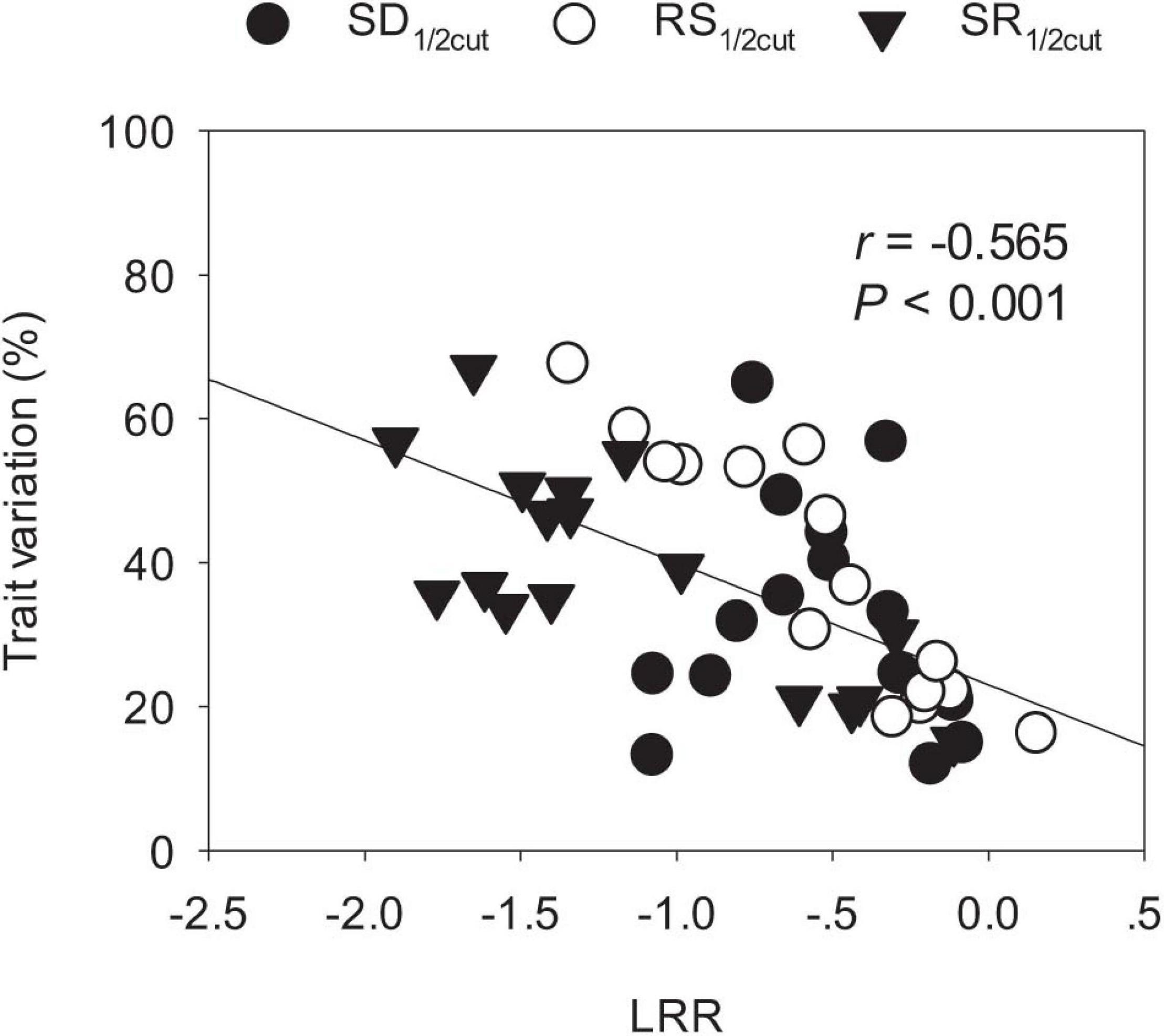
Figure 5. Linkages of ln response ratios (LRR) and trait variations among shoot and root phenotypic traits of Leymus chinensis. SD1/2cut, shoot defoliation; RS1/2cut, root severing; SR1/2cut, shoot defoliation and root severance.
Allometric Scaling Among Plant Traits
There was a significant positive relationship between log shoot traits and log root traits of L. chinensis within all pots across 15 of the SMA regressions (P < 0.05, Supplementary Figure 9). The allometric slopes for plant height with leaf perimeter (slope = 0.89, P = 0.03) and leaf mass per area (slope = 0.52, P < 0.01) were numerically less than 1.0 (Table 1). Among these measures, 13 of the 15 bivariate relationships had slopes significantly higher than 1.0 (P < 0.05, Table 1). Root traits generally had higher allometric slopes when plotted with plant height (2.06–2.23) than shoot phenotypic traits (Table 1). Similarly, the slopes of the SMA regressions between shoot traits (x-axis) and root traits (y-axis) were more than 1.0 (P < 0.05, Table 1). In addition, the shoot defoliation and root severing treatments resulted in heterogeneity of allometric slopes in the bivariate relationship between plant height and leaf area (P < 0.05, Supplementary Table 4). The SMA tests of the other 14 bivariate relationships showed that there were common slopes (P > 0.05) across the four treatments (Supplementary Table 4). However, there were significant shifts along the common slope of this bivariate relationships (P < 0.05, Supplementary Table 4).
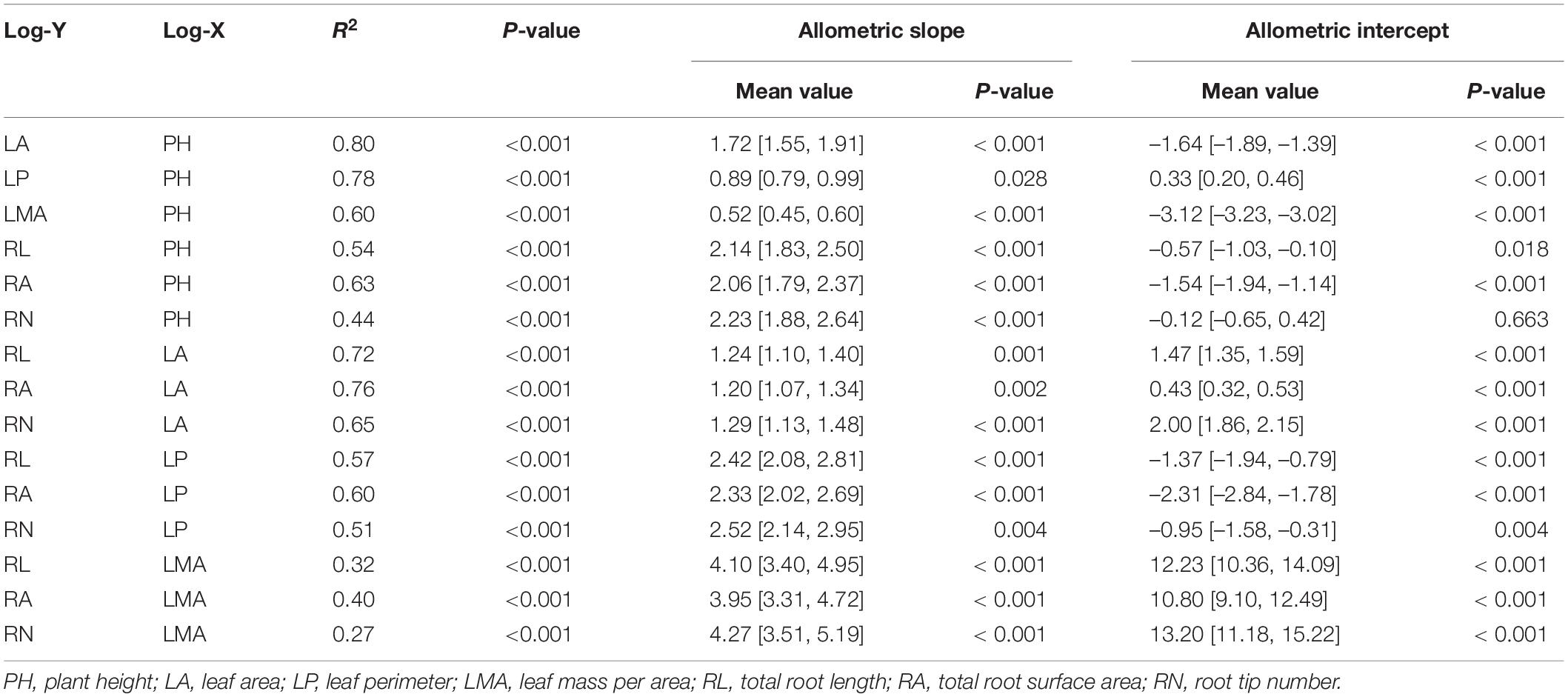
Table 1. Standardized major axis regression slopes and intercepts with 95% confidence intervals (shown in brackets) for log-log transformed relationships among some key phenotypic traits of Leymus chinensis shoots and roots.
Photosynthetic Responses to Treatments
Leaf photosynthetic rates were significantly influenced by the interactions between the experimental treatment and time after treatment application (P < 0.05, Figure 6 and Supplementary Figure 10). The SD1/2cut, RS1/2cut, and SR1/2cut treatments significantly decreased both net and gross photosynthetic rates on the first day of the treatment (P < 0.05, Figure 6 and Supplementary Figure 10). In contrast, L. chinensis had higher respiration rates when shoots were defoliated rather than when roots were severed (P < 0.05, Supplementary Figure 10). However, the photosynthetic and respiration rates gradually restored over time, with no difference between the four treatments on the 15th day after treatments were applied (P < 0.05, Figure 6 and Supplementary Figure 10). In consequence, the respiration consumption proportion was rapidly increased (P < 0.05) in the first day of the treatment and the consumption proportion gradually decreased with time from the date of treatment (Figure 6). In addition, the photosynthetic and respiration rates of leaves were highly correlated with plant height (P < 0.05, Supplementary Figure 11).
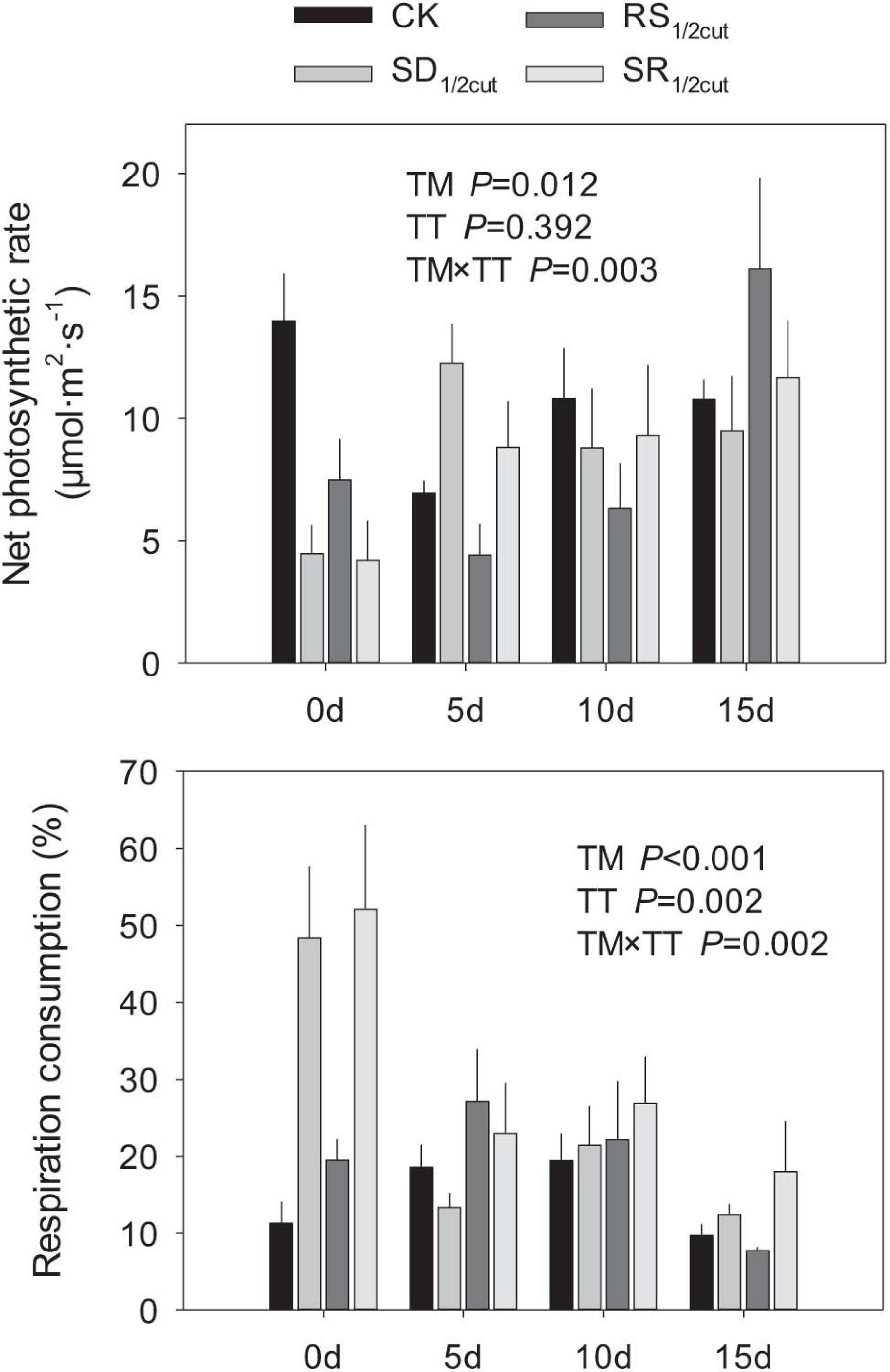
Figure 6. Effects of shoot defoliation and root severing on net photosynthetic rate and respiration consumption of Leymus chinensis after the second cycle of treatment. CK, control; SD1/2cut, shoot defoliation; RS1/2cut, root severing; SR1/2cut, shoot defoliation and root severing; TM, treatment; TT, time after treating.
Discussion
Using a trait-based approach, we experimentally tested defoliation-induced legacy effects on the growth and persistence of the perennial grass species L. chinensis. Our results show evidence for the shoot and root growth feedback loop (hereafter also shoot-root feedback loop) influenced by defoliation, which may occur via mowing and grazing (Liu et al., 2015; Yang et al., 2020). In addition, our results suggested that the shoot–root feedback loop induced by defoliation was mediated by disproportionate response and allometry of plant functional traits. We found that the effect of experimental defoliation and root severance on trait plasticity of L. chinensis was sub-additive, may resulting in a buffering effect on plant performances under grazing or mowing. Overall, our study established that feedback between different plant organs contributes toward defoliation legacy effects, and has implications on the growth and persistence of the perennial grass species under intensifying utilization.
Reciprocal Feedback Between Shoots and Roots
For perennial plant species, the interaction between above and belowground organs are critical to both individual growth in the current growing season and population persistence in the next year (Briske and Richards, 1995; Zong and Shi, 2019); thus, this may be the determining factor for the temporal stability of the whole ecosystem (Zhang et al., 2017; Xu et al., 2020). Two-rounds of shoot defoliation directly reduced the remaining and accumulated biomass by 64.66 and 57.51%, and indirectly reduced the root biomass by 60.11% (Figure 1 and Supplementary Figure 2). The reduction of root biomass may be due to the defoliation-induced reallocation of carbohydrate resources (Baptist et al., 2013; Wang et al., 2019). Our results suggested that root severance directly reduced 24.92 and 17.58% of the remaining and accumulated root biomass, and indirectly reduced 40.49% of shoot biomass (Figure 1 and Supplementary Figure 2). These finding clearly indicated that root severance generated a greater decrease in shoot biomass than root biomass. Together, these findings indicated that defoliation-induced reciprocal feedback between shoots and roots would generate legacy effects on future plant growth. Indeed, previous studies have shown that external disturbance can produce legacy effects via changes in soil abiotic properties and microorganisms in plant tissue and soil (Cuddington, 2011; Chen T. et al., 2018; Barthelemy et al., 2019). However, our finding of reciprocal feedback from different organs furthers our understanding of legacy effects independent from those generated by the fluctuations in the external environment.
In this study, both shoot defoliation and root severance generated under-compensatory growth, that is, the regenerated biomass was less than the lost biomass. The under-compensatory growth, rather than equal− or over-compensatory growth, might be caused by the high intensity of simulated defoliation and root severance. A previous study explicitly verified that over-and under-compensatory growth occurred in moderate and heavy treatments of grazing or mowing, respectively (Ma et al., 2020). In our experimental manipulation, half of the existing biomass was removed by twice, inducing strong adverse effects on plant growth capacity. Therefore, our results suggest that heavy, continuous grazing is detrimental to the plant growth not only via the direct effects of under-compensatory growth of shoot but also the indirect effects of root plasticity, finally impacting the sustainable utilization of grasslands.
Shoot–Root Interaction Determines Biomass Production
Although shoots are directly affected by defoliation (Liu et al., 2018), we found that the biomass of the root had a stronger response to defoliation. The decline in root biomass might be triggered by the tight coupling mechanism between different plant organs, which has been reported in previous studies (Wang et al., 2010; Ma and Wang, 2021). It is intriguing that roots rather than shoots had a greater decline in biomass when L. chinensis was subjected to shoot defoliation, leading to a much lower root-to-shoot ratio in defoliated plants than that in undisturbed plants. One possible explanation for this observation is that plants may enhance the translocation of assimilates to aboveground rather than belowground organs (Gao et al., 2008; Esmaeili et al., 2009; Wang et al., 2019). Our findings support this explanation since we found that defoliation significantly enhanced the growth rate (the slopes of plant growth along time series) of L. chinensis by approximately 75% (from 0.66 cm day–1 in undisturbed plants to 1.16 cm day–1 in defoliated plants. Following defoliation, this shoot tissue regeneration can be dramatically enhanced to compensate for biomass loss, whereas the root growth was greatly suppressed. Further, our photosynthetic measurements also supported this speculation. Immediately following defoliation, the gross photosynthetic rates were markedly suppressed, whereas the respiration rates were greatly improved. However, these trends were reversed during the restoration process, indicating that photosynthetic rates were greatly improved, while respiration was reduced. This could be ascribed to the regulation of the partitioning of new photosynthate carbon in response to plant tissue damage and biomass loss (Schierenbeck et al., 1994; Wang et al., 2019).
We found that root severance triggered a greater decrease in shoots than that in roots, resulting in a significant increase in the root-to-shoot ratio, which may be explained by the reallocation of photosynthate carbon to offset the lost root biomass. This finding suggests that root plasticity induced by shoot defoliation can generate a severe cascading reaction of plant growth in the following stage. Given that heavy grazing or frequent mowing tend to make the roots shallower (Kitchen et al., 2009), this finding that roots had more sensitive response than shoots to root severance (i.e., asymmetric response) can provide new insights for understanding the mechanism of grassland degradation. In general, root biomass highly relies on the changes in root traits (Roumet et al., 2008). Across the root traits, root volume, root surface area, and root diameter were tightly correlated with the changes in root biomass after severing treatment. Thus, an increased plasticity of root traits could lead to a decrease in the stability of root biomass.
Disproportionate Response and Allometry of Plant Traits
The linkage of functional traits with plant adaptation and ecological functioning have been addressed in previous studies (Garnier and Navas, 2012; van der Merwe et al., 2021). Results from the present study provided a new addition to this trait-based approach from the perspective of trait plasticity under defoliation. Although all traits negatively responded to shoot defoliation and root severance, the extent of trait plasticity dramatically (by approximately 10-fold) among the stable and sensitive traits. In general, across all plant traits, root traits were more sensitive than shoot traits in both the shoot defoliation and root severing treatments. Based on the SMA approach, we further showed that shoot/root asymmetry in plants with both shoot defoliation and root severing was allometric according to the regressions of log10-transformed traits. In agreement with studies on grassland species (Luo et al., 2013; Ma and Wang, 2021), the allometric slopes of shoots and roots were significantly different, indicating that L. chinensis traits did not meet the isometric prediction.
In general, the allometric slopes in bivariate relationships of aboveground traits (x-axis) versus belowground traits (y-axis), which varied from 1.20 to 4.27, were markedly higher than 1.0. In terms of the trait-specific allometry between above and belowground organs, shoot traits had relatively higher allometric slopes with root tip number and total root length. We surmise that this result is most likely related to the species growth phase and experimental methodology (Gedroc et al., 1996; Wang et al., 2010). First, plant age can potentially determine the allometric scaling between above and belowground organs. For example, Husáková et al. (2018) found that plant species generally allocated disproportionally more carbon photosynthate to aboveground organs as seedlings than as adults. In this study, the allometric scaling was assessed in 65-day-old plants, which corresponds to the rapid growth phase of L. chinensis; this may stimulate a higher biomass allocation in shoots than roots. Second, plants preferentially promoted the growth of aboveground than belowground organs because the water and nutrient resources were supplied in sufficient amounts in hydroponics with Hoagland’s nutrient solution (Ren et al., 2017).
Sub-Additive Effect of Shoot Defoliation and Root Severance
We demonstrated that the LRR of all shoot or root traits in RS1/2cut were greater than either SD1/2cut or RS1/2cut. However, the findings also showed that there was no significant interactive effect on plant performance for either defoliation or root biomass removal. Of particular interest is that the combined effects of shoot and root biomass removal were sub-additive, that is, the LRRs of plant traits in SR1/2cut was less than the sum of the independent effects of the two manipulations. This sub-additive effect can buffer the predicted negative feedback loop of non-adaptive plasticity in shoots and roots induced by defoliation (Arredondo and Johnson, 1999; Zhang et al., 2018), thereby preventing the worst−case outcomes of plant fitness. The observed sub-additive effects could arise from the enhancement of metabolic activity, such as photosynthesis, thus leading to a positive compensatory effect to cope with biomass loss (Richards and Caldwell, 1985; Iqbal et al., 2012). This is supported by our finding that photosynthetic and respiratory rates showed higher respiratory consumption and photosynthetic rates in SR1/2cut, which potentially stimulated key biological processes including biosynthesis, metabolism, and carbon remobilization.
In terms of trait-specific LRRs, our results showed that the relative change between observed and predicted LRRs, ranging from 0.22 to 1.27, meaning they varied greatly and were tight correlated with observed or predicted LRRs. This directly suggested that the sub-additive effects were dependent on plant traits. To our knowledge, our study is the first to report this. Comparatively, root traits had greater relative change between observed and predicted LRRs than shoot traits. We suspect the differences between shoots and roots can be partly explained by the trade-off and allometry of functional traits in response to disturbances (Li et al., 2016). For example, a recent study on Artemisia showed that an allometric strategy among leaves, stems, and roots was prevalent and was essential to optimize plant performance under environmental gradients (Liu et al., 2021). In the present study, considering that roots had higher plasticity than shoots, the stronger sub-additive effects of root traits may potentially buffer the negative effects of defoliation.
Conclusion
Overall, this study presents strong evidence that defoliation can produce a robust feedback loop for shoot and root growth, potentially generating observable legacy effects on plant performance at a later growth stage or even in following growing seasons. This shoot–root feedback is mediated by allometry among plant traits. Our findings showed that the combined effects of shoot defoliation and root severing were less than the sum of their independent effects but statistically greater than the effect of each of the two manipulations. In consequence, this sub-additive effect can buffer the negative influences of shoot–root feedback on plant performance induced by defoliation. Considering the existing knowledge of legacy effects due to land use, our findings provide new insight for this topic from the reciprocal feedback between shoots and roots of grassland species affected by a high-intensity of defoliation.
Data Availability Statement
The original contributions presented in the study are included in the article/Supplementary Material, further inquiries can be directed to the corresponding author/s.
Author Contributions
XL and JS designed the research and wrote first draft of the manuscript. XL, ZZ, FG, and JD performed the research. XL, ZZ, and FG analyzed the data. All authors contributed critically to revisions and gave final approval for publication.
Funding
Funding was supported by the National Natural Science Foundation of China (32071882 and 31702161), Science and Technology Project of Inner Mongolia (2021GG0055), Central Public-interest Scientific Institution Basal Research Fund (1610332020001), and National Agricultural Experimental Station for Soil Quality, Hohhot (NAES042SQ20).
Conflict of Interest
The authors declare that the research was conducted in the absence of any commercial or financial relationships that could be construed as a potential conflict of interest.
Publisher’s Note
All claims expressed in this article are solely those of the authors and do not necessarily represent those of their affiliated organizations, or those of the publisher, the editors and the reviewers. Any product that may be evaluated in this article, or claim that may be made by its manufacturer, is not guaranteed or endorsed by the publisher.
Acknowledgments
We thank Zhuo Bai and Fang Mu for their assistance in the experiments, and Kenny G. Png from NTU Singapore for comments on an earlier version of this manuscript.
Supplementary Material
The Supplementary Material for this article can be found online at: https://www.frontiersin.org/articles/10.3389/fpls.2021.684503/full#supplementary-material
Supplementary Figure 1 | Scheme of the experimental setup. Root or shoot removal was conducted in plants grown hydroponically. SD1/2cut, shoot defoliation; RS1/2cut, root severing; SR1/2cut, shoot defoliation and root severing.
Supplementary Figure 2 | Biomass composition of the remaining organ and the removed organ induced by shoot defoliation (A1–D1) or root severing (A2–D2) of Leymus chinensis. (A1,B1) Control; (A2,B2) shoot defoliation; (A3,B3) root severing; and (A4,B4) shoot defoliation and root severing. T1 and T2 mean the first and second cutting.
Supplementary Figure 3 | Relationships of the proportion of defoliated or severed biomass with the remaining biomass in the shoot (A1,A2), root (B1,B2) and overall (C1,C2) with (A1–C1) or without (A2–C2) a control treatment.
Supplementary Figure 4 | Plant height of Leymus chinensis across the four treatments along a time series. CK, control; SD1/2cut, shoot defoliation; RS1/2cut, root severing; SR1/2cut, shoot defoliation and root severing.
Supplementary Figure 5 | Relationship of the slope of plant height with the initial and final plant height of L. chinensis along a time series.
Supplementary Figure 6 | Effects of defoliation and root severing on L. chinensis leaf traits. CK, control; SD1/2cut, shoot defoliation; RS1/2cut, root severing; SR1/2cut, shoot defoliation and root severing.
Supplementary Figure 7 | Effects of defoliation and root severing on L. chinensis root traits. CK, control; SD1/2cut, shoot defoliation; RS1/2cut, root severing; SR1/2cut, shoot defoliation plus root severing.
Supplementary Figure 8 | The predicted ln-based response ratios (LRRs) (A) and their relationship with observed LRRs (B). The observed values were the LRRs in the shoot defoliation and root severing treatment (SR1/2cut), whereas the predicted values were calculated by the LRRs in separate treatments of shoot defoliation (SD1/2cut) and root severing (RS1/2cut). PH, plant height; LM, single leaf weight; LA, leaf area; LL, leaf length; LW, leaf width; LP, leaf perimeter; RL, total root length; RA, total root surface area; RV, total root volume; RD, average root diameter; RN, root tip number; RT, root tip forks; RC, root tip crossings; ARM, remaining aboveground biomass; BRM, remaining belowground biomass; BCM, aboveground biomass accumulation; ACM, belowground biomass accumulation.
Supplementary Figure 9 | Relationships of log-log transformed shoot and root traits of L. chinensis. PH, plant height; LA, leaf area; LP, leaf perimeter; LMA, leaf mass per area; RL, total root length; RA, total root surface area; RN, root tip number.
Supplementary Figure 10 | Effects of shoot defoliation and root severing on the gross photosynthetic rate and respiration rate of L. chinensis after the second cycle of treatment across the time series. CK, control; SD1/2cut, shoot defoliation; RS1/2cut, root severing; SR1/2cut, shoot defoliation and root severing; TM, treatment; TT, time after treating.
Supplementary Figure 11 | Linking plant size with the photosynthetic and respiration rates.
Supplementary Table 1 | Effects of shoot defoliation (SD1/2cut), root severing (RS1/2cut), and their interactions (SD1/2cut × RS1/2cut) on the biomass of L. chinensis. ARM, remaining aboveground biomass; BRM, remaining belowground biomass; TRM, remaining total biomass; RRS, root-to-shoot ratio of remaining biomass; BCM, aboveground biomass accumulation; ACM, belowground biomass accumulation; TCM, total biomass accumulation; CRS, root-to-shoot ratio of accumulated biomass.
Supplementary Table 2 | Effects of shoot defoliation (SD1/2cut), root severing (RS1/2cut), and their interactions (SD1/2cut × RS1/2cut) on phenotypic traits of L. chinensis shoots and roots. PH, plant height; LA, leaf area; LM, single leaf weight; SLA, specific leaf area; LL, leaf length; LW, leaf width; LP, leaf perimeter; RL, total root length; SRL, specific root length; RA, total root surface area; RD, average root diameter; RV, total root volume; RN, root tip number; RT, root tip forks; RC, root tip crossings.
Supplementary Table 3 | L. chinensis shoot and root trait variations (%) in the control (CK), shoot defoliation (SD1/2cut), root severing (RS1/2cut), and shoot defoliation and root severing (SR1/2cut) treatments. PH, plant height; LM, single leaf weight; LA, leaf area; LL, leaf length; LW, leaf width; LP, leaf perimeter; RL, total root length; RA, total root surface area; RV, total root volume; RD, average root diameter; RN, root tip number; RT, root tip forks; RC, root tip crossings; ARM, remaining aboveground biomass; BRM, remaining belowground biomass; BCM, aboveground biomass accumulation; ACM, belowground biomass accumulation.
Supplementary Table 4 | Standardized major axis regression (SMA) slopes for log-log transformed relationships among some key phenotypic traits of L. chinensis shoots and roots in the four treatments. In several of the bivariate cases, SMA tests for common slopes reveal no significant differences among the four treatment groups (i.e., P > 0.05). In such cases, common slopes for the bivariate relationships were shown. Where there was a common slope, significant shifts (P < 0.05; labeled “yes” in the table) along a common slope are also indicated. CK, control; SD1/2cut, shoot defoliation; RS1/2cut, root severing; SR1/2cut, shoot defoliation and root severing. PH, plant height; LA, leaf area; LP, leaf perimeter; LMA, leaf mass per area; RL, total root length; RA, total root surface area; RN, root tip number.
References
Abalos, D., van Groenigen, J. W., and De Deyn, G. B. (2018). What plant functional traits can reduce nitrous oxide emissions from intensively managed grasslands? Glob. Chang. Biol. 24, e248–e258.
Anten, N. P. R., Martinez-Ramos, M., and Ackerly, D. D. (2003). Defoliation and growth in an understory palm: quantifying the contributions of compensatory responses. Ecology 84, 2905–2918. doi: 10.1890/02-0454
Arredondo, J., and Johnson, D. (1999). Root architecture and biomass allocation of three range grasses in response to nonuniform supply of nutrients and shoot defoliation. New Phytol. 143, 373–385. doi: 10.1046/j.1469-8137.1999.00460.x
Ayub, G., Zaragoza-Castells, J., Griffin, K. L., and Atkin, O. K. (2014). Leaf respiration in darkness and in the light under pre-industrial, current and elevated atmospheric CO2 concentrations. Plant Sci. 226, 120–130. doi: 10.1016/j.plantsci.2014.05.001
Bai, W., Sun, X., Wang, Z., and Li, L. (2009). Nitrogen addition and rhizome severing modify clonal growth and reproductive modes of Leymus chinensis population. Plant Ecol. 205, 13–21. doi: 10.1007/s11258-009-9595-2
Baptist, F., Secher-Fromell, H., Viard-Cretat, F., Aranjuelo, I., Clement, J. C., Creme, A., et al. (2013). Carbohydrate and nitrogen stores in Festuca paniculata under mowing explain dominance in subalpine grasslands. Plant Biol. 15, 395–404. doi: 10.1111/j.1438-8677.2012.00652.x
Barthelemy, H., Dorrepaal, E., and Olofsson, J. (2019). Defoliation of a grass is mediated by the positive effect of dung deposition, moss removal and enhanced soil nutrient contents: results from a reindeer grazing simulation experiment. Oikos 128, 1515–1524. doi: 10.1111/oik.06310
Benson, E. J., and Hartnett, D. C. (2006). The role of seed and vegetative reproduction in plant recruitment and demography in tallgrass prairie. Plant Ecol. 187, 163–178. doi: 10.1007/s11258-005-0975-y
Briske, D. D., and Richards, J. H. (1995). “Plant responses to defoliation: a physiological, morphological and demographic evaluation,” in Wildland Plants: Physiological Ecology and Developmental Morphology, eds D. J. Bedunah and R. E. Sosebee (Denver, CO: Society for Range Management), 635–710.
Chen, Q., Wang, Z. L., Zou, C. B., Fan, Y., Dittert, K., and Lin, S. (2018). Legacy effects of historical grazing affect the response of vegetation dynamics to water and nitrogen addition in semi-arid steppe. Appl. Veg. Sci. 21, 229–239. doi: 10.1111/avsc.12364
Chen, T., Nan, Z., Kardol, P., Duan, T., Song, H., Wang, J., et al. (2018). Effects of interspecific competition on plant-soil feedbacks generated by long-term grazing. Soil Biol. Biochem. 126, 133–143. doi: 10.1016/j.soilbio.2018.08.029
Cruz, P., De Quadros, F. L. F., Theau, J. P., Frizzo, A., Jouany, C., Duru, M., et al. (2010). Leaf traits as functional descriptors of the intensity of continuous grazing in native grasslands in the south of Brazil. Rangel. Ecol. Manag. 63, 350–358. doi: 10.2111/08-016.1
Cuddington, K. (2011). Legacy effects: the persistent impact of ecological interactions. Biol. Theory 6, 203–210. doi: 10.1007/s13752-012-0027-5
Dawson, L., Thornton, B., Pratt, S., and Paterson, E. (2004). Morphological and topological responses of roots to defoliation and nitrogen supply in Lolium perenne and Festuca ovina. New Phytol. 161, 811–818. doi: 10.1111/j.1469-8137.2004.00979.x
Dixon, A., Faber-Langendoen, D., Josse, C., Morrison, J., and Loucks, C. (2014). Distribution mapping of world grassland types. J. Biogeogr. 41, 2003–2019. doi: 10.1111/jbi.12381
Erktan, A., Roumet, C., Bouchet, D., Stokes, A., Pailler, F., and Munoz, F. (2018). Two dimensions define the variation of fine root traits across plant communities under the joint influence of ecological succession and annual mowing. J. Ecol. 106, 2031–2042. doi: 10.1111/1365-2745.12953
Esmaeili, M. M., Bonis, A., Bouzillé, J.-B., Mony, C., and Benot, M.-L. (2009). Consequence of ramet defoliation on plant clonal propagation and biomass allocation: example of five rhizomatous grassland species. Flora Morphol. Distribut. Funct. Ecol. Plants 204, 25–33. doi: 10.1016/j.flora.2007.11.008
Ferraro, D. O., and Oesterheld, M. (2002). Effect of defoliation on grass growth. a quantitative review. Oikos 98, 125–133. doi: 10.1034/j.1600-0706.2002.980113.x
Fetzel, T., Havlik, P., Herrero, M., and Erb, K. H. (2017). Seasonality constraints to livestock grazing intensity. Glob. Chang. Biol. 23, 1636–1647. doi: 10.1111/gcb.13591
Gao, Y. Z., Giese, M., Lin, S., Sattelmacher, B., Zhao, Y., and Brueck, H. (2008). Belowground net primary productivity and biomass allocation of a grassland in inner Mongolia is affected by grazing intensity. Plant Soil 307, 41–50. doi: 10.1007/s11104-008-9579-3
Garnier, E., and Navas, M.-L. (2012). A trait-based approach to comparative functional plant ecology: concepts, methods and applications for agroecology. a review. Agron. Sustain. Dev. 32, 365–399. doi: 10.1007/s13593-011-0036-y
Gedroc, J., McConnaughay, K., and Coleman, J. (1996). Plasticity in root/shoot partitioning: optimal, ontogenetic, or both? Funct. Ecol. 10, 44–50. doi: 10.2307/2390260
Husáková, I., Weiner, J., and Münzbergová, Z. (2018). Species traits and shoot–root biomass allocation in 20 dry-grassland species. J. Plant Ecol. 11, 273–285.
Iqbal, N., Masood, A., and Khan, N. (2012). Analyzing the significance of defoliation in growth, photosynthetic compensation and source-sink relations. Photosynthetica 50, 161–170. doi: 10.1007/s11099-012-0029-3
Kaisermann, A., de Vries, F. T., Griffiths, R. I., and Bardgett, R. D. (2017). Legacy effects of drought on plant–soil feedbacks and plant–plant interactions. New Phytol. 215, 1413–1424. doi: 10.1111/nph.14661
Kirkegaard, J. A., Lilley, J. M., Hunt, J. R., Sprague, S. J., Ytting, N. K., Rasmussen, I. S., et al. (2015). Effect of defoliation by grazing or shoot removal on the root growth of field-grown wheat (Triticum aestivum L.). Crop Pasture Sci. 66, 249–259. doi: 10.1071/cp14241
Kitchen, D. J., Blair, J. M., and Callaham, M. A. (2009). Annual fire and mowing alter biomass, depth distribution, and C and N content of roots and soil in tallgrass prairie. Plant Soil 323, 235–247. doi: 10.1007/s11104-009-9931-2
Leuschner, C., Gebel, S., and Rose, L. (2013). Root trait responses of six temperate grassland species to intensive mowing and NPK fertilisation: a field study in a temperate grassland. Plant Soil 373, 687–698. doi: 10.1007/s11104-013-1836-4
Lezama, F., and Paruelo, J. M. (2016). Disentangling grazing effects: trampling, defoliation and urine deposition. Appl. Veg. Sci. 19, 557–566. doi: 10.1111/avsc.12250
Li, X., Hou, X., Ren, W., Baoyin, T., Liu, Z., Badgery, W., et al. (2016). Long-term effects of mowing on plasticity and allometry of Leymus chinensis in a temperate semi-arid grassland, China. J. Arid Land 8, 899–909. doi: 10.1007/s40333-016-0005-z
Li, X., Wu, Z., Liu, Z., Hou, X., Badgery, W., Guo, H., et al. (2015). Contrasting effects of long-term grazing and clipping on plant morphological plasticity: evidence from a rhizomatous grass. PLoS One 10:e0141055. doi: 10.1371/journal.pone.0141055
Liu, G., and Han, J. (2008). Seedling establishment of wild and cultivated Leymus chinensis (Trin.) Tzvel. under different seeding depths. J. Arid Environ. 72, 279–284. doi: 10.1016/j.jaridenv.2007.06.008
Liu, M., Gong, J., Li, Y., Li, X., Yang, B., Zhang, Z., et al. (2019). Growth–defense trade-off regulated by hormones in grass plants growing under different grazing intensities. Physiol. Plant. 166, 553–569. doi: 10.1111/ppl.12802
Liu, N., Kan, H., Yang, G., and Zhang, Y. (2015). Changes in plant, soil, and microbes in a typical steppe from simulated grazing: explaining potential change in soil C. Ecol. Monogr. 85, 269–286. doi: 10.1890/14-1368.1
Liu, R., Yang, X., Gao, R., Hou, X., Huo, L., Huang, Z., et al. (2021). Allometry rather than abiotic drivers explains biomass allocation among leaves, stems and roots of Artemisia across a large environmental gradient in China. J. Ecol. 109, 1026–1040. doi: 10.1111/1365-2745.13532
Liu, Y., Yang, X., Tian, D., Cong, R., Zhang, X., Pan, Q., et al. (2018). Resource reallocation of two grass species during regrowth after defoliation. Front. Plant Sci. 9:1767. doi: 10.3389/fpls.2018.01767
Loaiza, P., Balocchi, O., and Bertrand, A. (2017). Carbohydrate and crude protein fractions in perennial ryegrass as affected by defoliation frequency and nitrogen application rate. Grass Forage Sci. 72, 556–567. doi: 10.1111/gfs.12258
Lü, X.-T., Lü, F.-M., Zhou, L.-S., Han, X., and Han, X.-G. (2012). Stoichiometric response of dominant grasses to fire and mowing in a semi-arid grassland. J. Arid Environ. 78, 154–160. doi: 10.1016/j.jaridenv.2011.11.008
Luo, W., Jiang, Y., Lü, X., Wang, X., Li, M.-H., Bai, E., et al. (2013). Patterns of plant biomass allocation in temperate grasslands across a 2500-km transect in northern China. PLoS One 8:e71749. doi: 10.1371/journal.pone.0071749
Ma, H., Zheng, C., Gao, Y., Baskin, C. C., Sun, H., and Yang, H. (2020). Moderate clipping stimulates over-compensatory growth of Leymus chinensis under saline-alkali stress through high allocation of biomass and nitrogen to shoots. Plant Growth Regul. 92, 95–106. doi: 10.1007/s10725-020-00622-3
Ma, X. Z., and Wang, X. P. (2021). Aboveground and belowground biomass and its’ allometry for Salsola passerina shrub in degraded steppe desert in Northwestern China. Land Degrad. Dev. 32, 714–722. doi: 10.1002/ldr.3772
Ren, W., Hu, N., Hou, X., Zhang, J., Guo, H., Liu, Z., et al. (2017). Long-term overgrazing-induced memory decreases photosynthesis of clonal offspring in a perennial grassland plant. Front. Plant Sci. 8:419. doi: 10.3389/fpls.2017.00419
Rendina González, A. P., Preite, V., Verhoeven, K. J., and Latzel, V. (2018). Transgenerational effects and epigenetic memory in the clonal plant Trifolium repens. Front. Plant Sci. 9:1677. doi: 10.3389/fpls.2018.01677
Richards, J., and Caldwell, M. (1985). Soluble carbohydrates, concurrent photosynthesis and efficiency in regrowth following defoliation: a field study with Agropyron species. J. Appl. Ecol. 22, 907–920. doi: 10.2307/2403239
Roumet, C., Lafont, F., Sari, M., Warembourg, F., and Garnier, E. (2008). Root traits and taxonomic affiliation of nine herbaceous species grown in glasshouse conditions. Plant Soil 312, 69–83. doi: 10.1007/s11104-008-9635-z
Schierenbeck, K. A., Mack, R. N., and Sharitz, R. R. (1994). Effects of herbivory on growth and biomass allocation in native and introduced species of Lonicera. Ecology 75, 1661–1672. doi: 10.2307/1939626
Shen, H., Dong, S., Li, S., Xiao, J., Han, Y., Yang, M., et al. (2019). Grazing enhances plant photosynthetic capacity by altering soil nitrogen in alpine grasslands on the Qinghai-Tibetan plateau. Agric. Ecosyst. Environ. 280, 161–168. doi: 10.1016/j.agee.2019.04.029
Tsuzuki, Y., Koyanagi, T. F., and Miyashita, T. (2020). Plant community assembly in suburban vacant lots depends on earthmoving legacy, habitat connectivity, and current mowing frequency. Ecol. Evol. 10, 1311–1323. doi: 10.1002/ece3.5985
van der Graaf, A. J., Stahl, J., and Bakker, J. P. (2005). Compensatory growth of Festuca rubra after grazing: can migratory herbivores increase their own harvest during staging? Funct. Ecol. 19, 961–969. doi: 10.1111/j.1365-2435.2005.01056.x
van der Merwe, S., Greve, M., Olivier, B., and le Roux, P. C. (2021). Testing the role of functional trait expression in plant–plant facilitation. Funct. Ecol. 35, 255–265. doi: 10.1111/1365-2435.13681
van Staalduinen, M. A., and Anten, N. P. R. (2005). Differences in the compensatory growth of two co-occurring grass species in relation to water availability. Oecologia 146, 190–199. doi: 10.1007/s00442-005-0225-y
Veen, G., de Vries, S., Bakker, E. S., van der Putten, W. H., and Olff, H. (2014). Grazing-induced changes in plant–soil feedback alter plant biomass allocation. Oikos 123, 800–806. doi: 10.1111/j.1600-0706.2013.01077.x
Wan, D., Wan, Y., Hou, X., Ren, W., Ding, Y., and Sa, R. (2015). De novo assembly and transcriptomic profiling of the grazing response in Stipa grandis. PLoS One 10:e0122641. doi: 10.1371/journal.pone.0122641
Wang, B., Wu, L., Chen, D., Wu, Y., Hu, S., Li, L., et al. (2020). Grazing simplifies soil micro-food webs and decouples their relationships with ecosystem functions in grasslands. Glob. Chang. Biol. 26, 960–970. doi: 10.1111/gcb.14841
Wang, J., Abdullah, I., Xu, T., Zhu, W., Gao, Y., and Wang, L. (2019). Effects of mowing disturbance and competition on spatial expansion of the clonal plant Leymus chinensis into saline-alkali soil patches. Environ. Exp. Bot. 168:103890. doi: 10.1016/j.envexpbot.2019.103890
Wang, L., Niu, K., Yang, Y., and Zhou, P. (2010). Patterns of above-and belowground biomass allocation in China’s grasslands: evidence from individual-level observations. Sci. China Life Sci. 53, 851–857. doi: 10.1007/s11427-010-4027-z
Wang, X., Li, F. Y., Wang, Y., Liu, X., Cheng, J., Zhang, J., et al. (2020). High ecosystem multifunctionality under moderate grazing is associated with high plant but low bacterial diversity in a semi-arid steppe grassland. Plant Soil 448, 265–276. doi: 10.1007/s11104-020-04430-6
Whitaker, B. K., Bauer, J. T., Bever, J. D., and Clay, K. (2017). Negative plant-phyllosphere feedbacks in native Asteraceae hosts–a novel extension of the plant-soil feedback framework. Ecol. Lett. 20, 1064–1073. doi: 10.1111/ele.12805
Xu, B., Hugjiltu, M., Baoyin, T., Zhong, Y., Bao, Q., Zhou, Y., et al. (2020). Rapid loss of leguminous species in the semi-arid grasslands of northern China under climate change and mowing from 1982 to 2011. J. Arid Land 12, 752–765. doi: 10.1007/s40333-020-0022-9
Yang, Z., Minggagud, H., Baoyin, T., and Li, F. Y. (2020). Plant production decreases whereas nutrients concentration increases in response to the decrease of mowing stubble height. J. Environ. Manag. 253:109745. doi: 10.1016/j.jenvman.2019.109745
Yin, J., Li, X., Guo, H., Zhang, J., Kong, L., and Ren, W. (2020). Legacy effects of historical grazing alter leaf stomatal characteristics in progeny plants. PeerJ 8:e9266. doi: 10.7717/peerj.9266
Yin, J., Zhou, M., Lin, Z., Li, Q. Q., and Zhang, Y. Y. (2019). Transgenerational effects benefit offspring across diverse environments: a meta-analysis in plants and animals. Ecol. Lett. 22, 1976–1986. doi: 10.1111/ele.13373
Yuan, J., Li, H., and Yang, Y. (2020). The compensatory tillering in the forage grass Hordeum brevisubulatum after simulated grazing of different severity. Front. Plant Sci. 11:792. doi: 10.3389/fpls.2020.00792
Zhang, B., Hautier, Y., Tan, X., You, C., Cadotte, M. W., Chu, C., et al. (2020). Species responses to changing precipitation depend on trait plasticity rather than trait means and intraspecific variation. Funct. Ecol. 34, 2622–2633. doi: 10.1111/1365-2435.13675
Zhang, R., Schellenberg, M. P., Han, G., Wang, H., and Li, J. (2018). Drought weakens the positive effects of defoliation on native rhizomatous grasses but enhances the drought-tolerance traits of native caespitose grasses. Ecol. Evol. 8, 12126–12139. doi: 10.1002/ece3.4671
Zhang, Y., Loreau, M., He, N., Zhang, G., and Han, X. (2017). Mowing exacerbates the loss of ecosystem stability under nitrogen enrichment in a temperate grassland. Funct. Ecol. 31, 1637–1646. doi: 10.1111/1365-2435.12850
Zhang, Z., Gong, J., Wang, B., Li, X., Ding, Y., Yang, B., et al. (2020). Regrowth strategies of Leymus chinensis in response to different grazing intensities. Ecol. Appl. 30:e02113.
Keywords: phenotypic plasticity, plant functional trait, legacy effect, allometric scaling, shoot-root interaction, defoliation, grassland
Citation: Li X, Zhang Z, Guo F, Duan J and Sun J (2021) Shoot–Root Interplay Mediates Defoliation-Induced Plant Legacy Effect. Front. Plant Sci. 12:684503. doi: 10.3389/fpls.2021.684503
Received: 25 March 2021; Accepted: 13 July 2021;
Published: 05 August 2021.
Edited by:
John Paul Délano-Frier, Centro de Investigaciones y Estudios Avanzados, Instituto Politécnico Nacional de México (CINVESTAV), MexicoReviewed by:
Niels P. R. Anten, Wageningen University & Research, NetherlandsJianshuang Wu, Institute of Environment and Sustainable Development in Agriculture, Chinese Academy of Agricultural Sciences, China
Copyright © 2021 Li, Zhang, Guo, Duan and Sun. This is an open-access article distributed under the terms of the Creative Commons Attribution License (CC BY). The use, distribution or reproduction in other forums is permitted, provided the original author(s) and the copyright owner(s) are credited and that the original publication in this journal is cited, in accordance with accepted academic practice. No use, distribution or reproduction is permitted which does not comply with these terms.
*Correspondence: Juan Sun, sunjuan@qau.edu.cn