- Department of Botany, Leopold-Franzens-University of Innsbruck, Innsbruck, Austria
A bimodal radial growth (RG) pattern, i.e., growth peaks in spring and autumn, was repeatedly found in trees in the Mediterranean regions, where summer drought causes reduction or cessation of cambial activity. In a dry inner Alpine valley of the Eastern Alps (Tyrol, Austria, 750 m asl), Pinus sylvestris shows unimodal RG with onset and cessation of cambial activity in early April and late June, respectively. A resumption of cambial activity after intense summer rainfall was not observed in this region. In a field experiment, we tested the hypothesis that early cessation of cambial activity at this drought-prone site is an adaptation to limited water availability leading to an early and irreversible switch of carbon (C) allocation to belowground. To accomplish this, the C status of young P. sylvestris trees was manipulated by physical blockage of phloem transport (girdling) 6 weeks after cessation of cambial cell division. Influence of manipulated C availability on RG was recorded by stem dendrometers, which were mounted above the girdling zone. In response to blockage of phloem flow, resumption of cambial activity was detected above girdling after about 2 weeks. Although the experimentally induced second growth surge lasted for the same period as in spring (c. 2 months), the increment was more than twice as large due to doubling of daily maximum RG rate. After girdling, wood anatomical traits above girdling no longer showed any significant differences between earlywood and latewood tracheids indicating pronounced effects of C availability on cell differentiation. Below girdling, no reactivation of cambial activity occurred, but cell wall thickness of last formed latewood cell was reduced due to lack of C supply after girdling. Intense RG resumption after girdling indicates that cessation of cambial activity can be reversed by manipulating C status of the stem. Hence, our girdling study yielded strong support for the hypothesis that belowground organs exert high C sink strengths on the drought-prone study site. Furthermore, this work highlights the need of in-depth experimental studies in order to understand the interactions between endogenous and exogenous factors on cambial activity and xylem cell differentiation more clearly.
Introduction
Drought stress is a common trigger of growth reduction or premature cessation of cambial activity and cell differentiation in trees (e.g., van der Werf et al., 2007; Camarero et al., 2010; De Luis et al., 2011; Balducci et al., 2013), because cambial activity and cell differentiation are highly responsive to water availability (Sterck et al., 2008; Muller et al., 2011; Deslauriers et al., 2016). In several Mediterranean tree species (Pinus spp., Juniperus thurifera, Quercus ilex, and Arbutus unedo), cambial activity resumes, i.e., is reactivated in autumn if soil water availability increases again after prolonged summer drought (De Luis et al., 2007; Battipaglia et al., 2010; Camarero et al., 2010; Gutiérrez et al., 2011; Pacheco et al., 2016; Campelo et al., 2018). This bimodal pattern of cambial activity leads to the formation of intra-annual density fluctuations (IADFs; e.g., Campelo et al., 2007; Novak et al., 2013; Battipaglia et al., 2016; De Micco et al., 2016; Pacheco et al., 2018). The formation of earlywood-like tracheids with wide lumen and thin cell walls in latewood (also called L-IADF; Campelo et al., 2013) reflects the ability of some tree species to respond to favorable environmental conditions at the end of the growing season. However, IADF formation is not obligatorily linked to bimodal growth, because short-term fluctuations in cambial activity and cell enlargement during the growing period can also induce formation of IADFs in trees of temperate climate zone (Wimmer et al., 2000; Rigling et al., 2002; Vieira et al., 2009; Rozas et al., 2011).
Several dendroecological studies conducted at a drought-prone inner Alpine site revealed that limited soil water availability in spring and early summer constrains radial stem growth (RG) in coniferous species (e.g., Rigling et al., 2002; Zweifel et al., 2006; Schuster and Oberhuber, 2013). Analyses of intra-annual dynamics of RG and wood formation by dendrometers and microcoring, respectively, revealed that the maximum RG rate of co-occurring conifers peaked early in the growing season in late May through early June (Gruber et al., 2010; Oberhuber et al., 2014), although higher precipitation in summer would provide more favorable environmental conditions for tree growth. Gruber et al. (2010) suggested that the early decrease in RG might be due to an early shift of carbon (C) allocation to belowground organs as an adaptation to ameliorate drought stress. This view is corroborated by several authors who reported that (i) plant growth is limited by competition between sinks rather than directly by C resources (e.g., Körner, 2003; Simard et al., 2013; Guillemot et al., 2017) and (ii) that the mycorrhiza-associated root system is a strong sink for C in plants experiencing water shortage during the growing period (Shipley and Meziane, 2002; Brunner et al., 2015; Hagedorn et al., 2016; Rainer-Lethaus and Oberhuber, 2018; Hartmann et al., 2020).
Wood formation is a highly C-demanding process (Koch, 2004; Simard et al., 2013; Cuny et al., 2015; Deslauriers et al., 2016). Therefore, physical blockage of phloem transport (girdling) allows evaluation of the influence of changes in tree C status on RG (Maier et al., 2010; Maunoury-Danger et al., 2010; De Schepper and Steppe, 2011; Rademacher et al., 2019). Previously, we found that in potted Norway spruce (Picea abies) saplings exposed to drought, cambial reactivation and intense RG occurred above girdling (Oberhuber et al., 2017). However, a field study determining RG response and effects on xylem cell differentiation of drought-stressed Pinus sylvestris to phloem blockage after cessation of RG is still lacking. Due to higher sensitivity of growth processes compared to photosynthesis to drought (Muller et al., 2011), carbohydrates accumulate during periods of water shortage and C accumulation during drought could modulate wood formation and growth dynamics during resumption of cambial activity. By applying automatic dendrometers, intra-annual dynamics of RG can be followed at high-resolution pre- and post-girdling, i.e., during the regular and induced growing period, respectively.
Aims of this study therefore were (i) to assess whether phloem blockage after cessation of RG and shoot growth induces reactivation of cambial activity in young P. sylvestris trees at a xeric site in the field, (ii) to analyze differences in dynamics (growth rate, duration, and intensity) of RG before and after phloem blockage, and (iii) to compare wood anatomical traits [cell lumen diameter (CLD), cell area (CA), and cell wall thickness] between regular spring growth and the induced growth phase after girdling. We hypothesized that cambial reactivation after girdling occurs and due to increase in C availability above girdling, induced RG in summer shows different kinetics, i.e., increase in duration, growth rate, and total increment, compared with regular spring RG. Furthermore, we expected a crucial role of higher C availability after phloem blockage on wood anatomical traits, especially an increase in cell wall thickness.
Materials and Methods
Study Site
The field study was conducted at a xeric site at 750 m asl in a dry inner Alpine valley of the Eastern Alps in Austria (47° 13′ 53″ N, 10° 50′ 51″ E). Based on >100 years of climate records at Ötz (812 m asl, 5 km from the study area) mean annual air temperature and total precipitation amount to 7.3°C and 724 mm, respectively (long-term means during 1911–2017). According to the FAO classification system (FAO, 2006) soils of the protorendzina type, i.e., rendzic and lithic leptosols, are primarily developed. As a result of low soil depth and the coarse-textured structure, soils have a low water holding capacity. In this drought-prone environment, Scots pine (Pinus sylvestris L.) dominates and forms poorly growing open stands (Erico-Pinetum typicum, Ellenberg and Leuschner, 2010). We selected a south-west facing steep slope (c. 30°), where P. sylvestris rejuvenates naturally under open canopy. In order to minimize the impact on the protected forest area, we used young trees for the girdling experiment instead of mature trees. Stem height and diameter of selected trees (n = 7) amounted to 1.5 m and 2.9 cm, respectively. Age of trees at 30 cm stem height was 35 ± 5 years (Table 1).
Phloem Blockage
Girdling was applied to block phloem C transport from aboveground to belowground sinks. Trees (n = 3) were girdled after cessation of shoot and RG in mid-July 2019 (day of the year (doy 199) at a stem height of 25 cm above the soil surface by carefully detaching a 2–3-cm-wide band of bark including periderm, living phloem, and cambium. Exposed wood was treated with a fat-containing cream to prevent dehydration. At the time of girdling, cambial cell division of trees has already stopped for about 6 weeks (cf. Figure 2).
Dendrometer Records
Temperature compensated electronic diameter dendrometers with resolutions of <3 μm (DD-S, Ecomatik, Munich, Germany) were installed on trees (controls: n = 4; girdled trees: n = 3) at 5–7 cm above girdling to record intra-annual dynamics of RG. The temperature coefficient of the sensor was <0.2 μm/K (unverified information of the producer). The dead outermost loose layer of the bark (periderm) was slightly removed to allow proper mounting of the dendrometer and to ensure close contact with the stem. Data were recorded every 30 min with analog data loggers (HOBO UX120-006M, ONSET, Bourne, MA, United States).
Daily stem diameter variations (SDVs) were calculated by averaging all daily measurements (48 values per day), i.e., one value per day was extracted from the time series. The daily mean approach yields time series of daily SDVs, which consist of both water- and growth-induced diameter changes (Deslauriers et al., 2007). It has to be considered that irreversible growth-related diameter increments recorded by dendrometers include formation of xylem, phloem, and outer bark (Plomion et al., 2001). Time series of daily SDVs were set to zero on March 1, and we modeled short-term variations in intra-annual RG with the Gompertz function, which is commonly used to describe RG dynamics in trees (Zeide, 1993; Rossi et al., 2003; De Micco et al., 2019), by applying the Origin software package (OriginLab Corporation, Northampton, MA, United States).
Microclimate Records
At the study site, environmental conditions [air temperature, relative air humidity (RH), and daily precipitation] were continuously monitored during the study period (March through October 2019) at 2 m aboveground (ONSET, Pocasset, MA, United States). In addition, three soil moisture sensors (ThetaProbes Type ML2x, Delta-T, Cambridge, England) were installed at 5–10 cm soil depth to record changes in volumetric soil water content (SWC). In data loggers, measuring intervals for all sensors were programmed to 30 min. All measurements per day (i.e., 48 values) were used to calculate mean daily air temperature and relative air humidity (RH). The equation presented in Prenger and Ling (2000) was used to compute vapor pressure deficit of the air (VPD). Climate records and SWC during the study period are depicted in Figure 1. Student’s t test was applied to detect significant differences among climate and environmental variables during the regular (i.e., pre-girdling) and induced (i.e., post-girdling) growing period (cf. Figure 2).
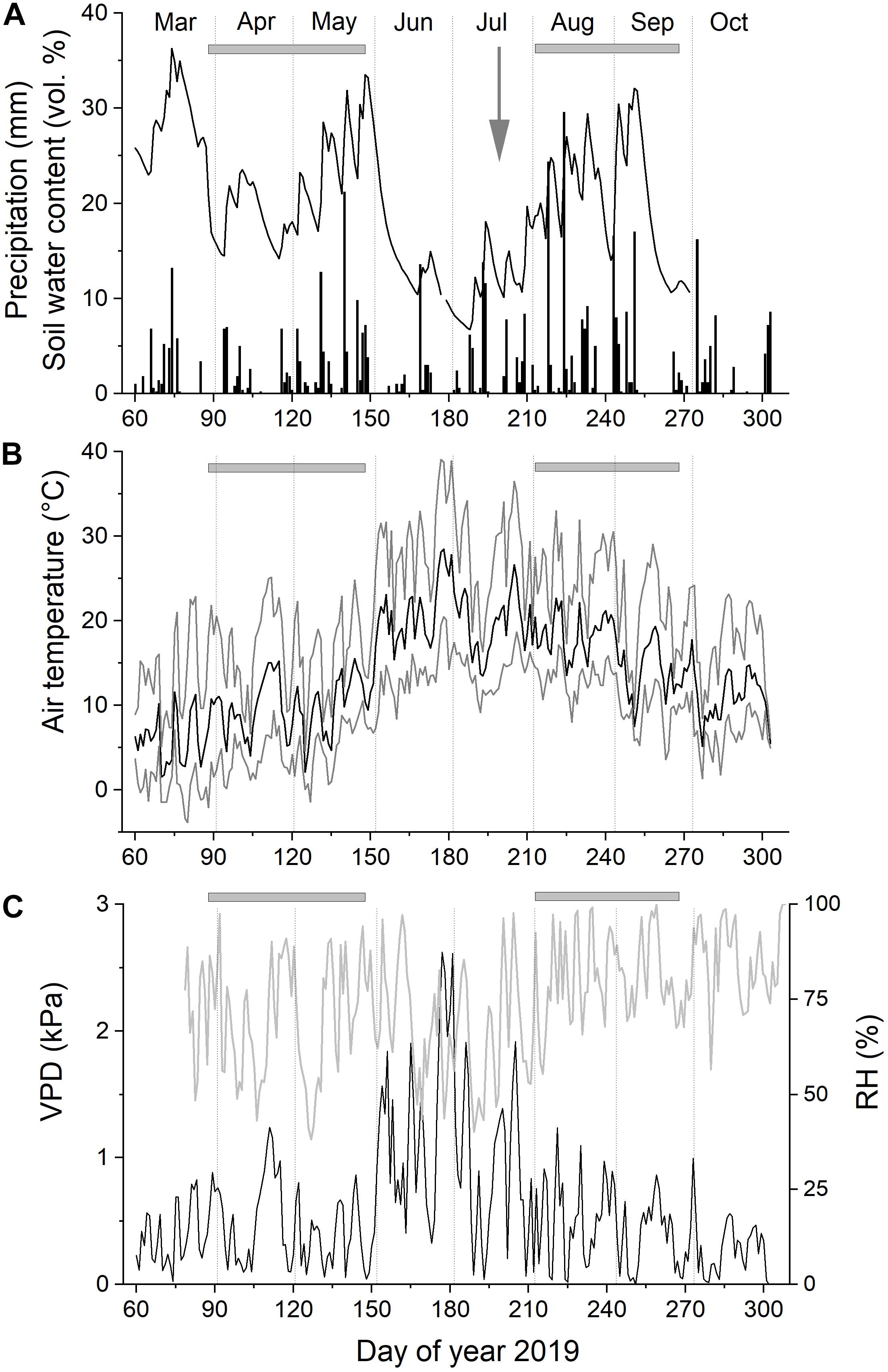
Figure 1. Climate variables and soil water content recorded at the study plot from March through October 2019. Daily precipitation and soil water content (A), mean daily air temperature (B), and relative air humidity (RH) and vapor pressure deficit of the air (VPD; C). Gray horizontal bars indicate regular (pre-girdling) and induced (post-girdling) growing periods in April–May and August–September, respectively (cf. Figure 2). Gray arrow in (A) indicates time of girdling at doy 199.
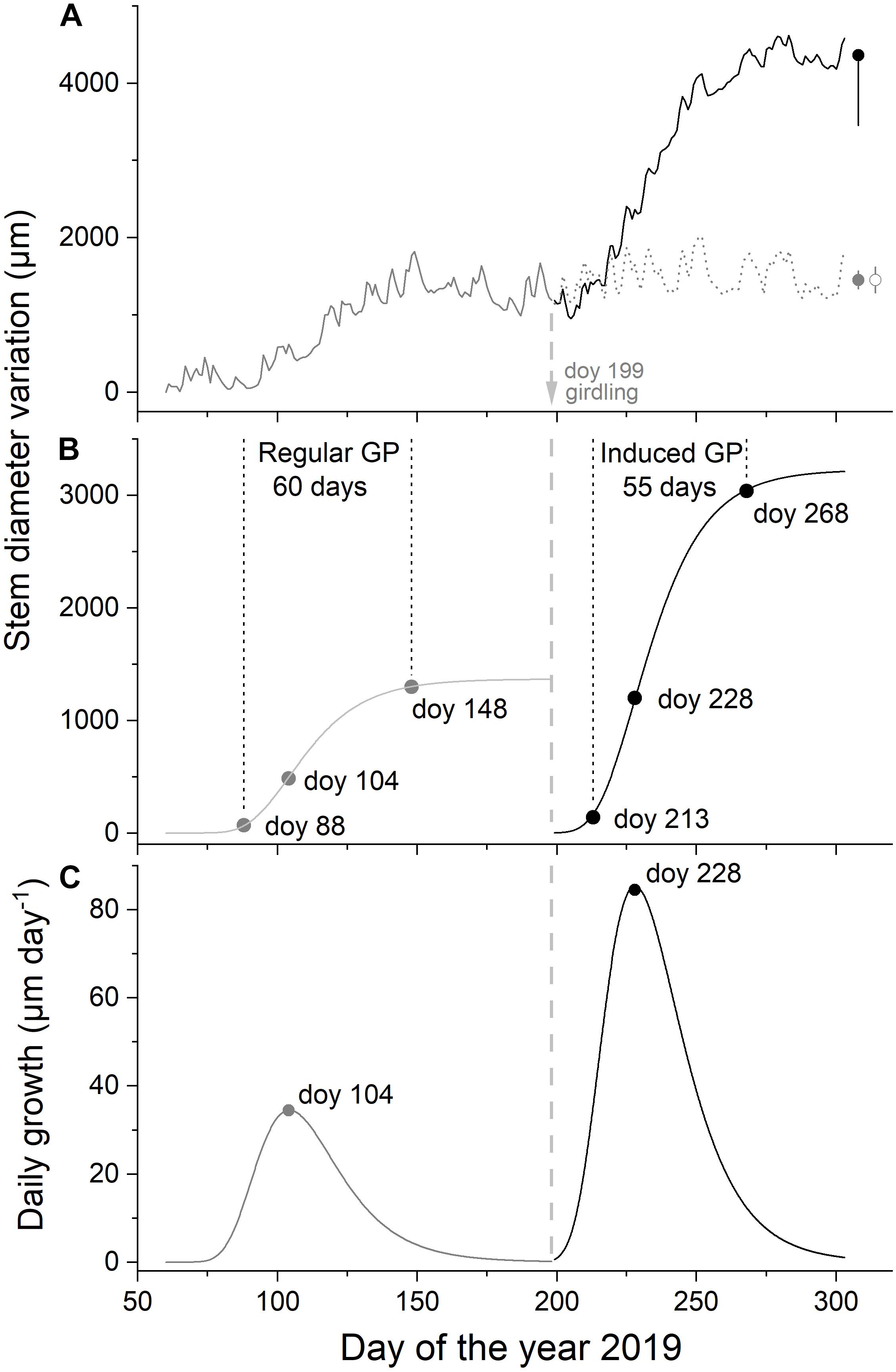
Figure 2. Time series of mean daily dendrometer records. Regular growth (pre-girdling, n = 7) and induced growth (post-girdling, n = 3) are given in gray and black lines, respectively. Dendrometer record of the non-girdled subset of trees (n = 4) is shown in dashed gray line (A), modeled intra-annual radial growth by applying the Gompertz function in (B; for parameters, see Table 2), and daily growth pre-girdling and post-girdling calculated from Gompertz function in (C; GP, growth period). Arrow indicates time of girdling. Bars in (A) depict standard errors of mean daily dendrometer records during the last 25 days.
Wood Anatomy
Changes in wood anatomy (radial CLD; CA; and radial cell wall thickness, CWT) in response to C manipulation were analyzed at the end of the growing season. Stem cross-sections were collected from all trees (controls and girdled trees) at the position of dendrometers. In girdled trees additional cross-sections were sampled 5 cm above and below the girdling zone. The chosen distance to the girdling zone ensured that influence of wound responses on wood formation was avoided. CWT, CA, and CLD were measured in earlywood and latewood on stem transverse sections of ∼20-μm thickness, which were cut using a sliding microtome. To distinguish between earlywood and latewood tracheids, the Mork’s index, i.e., the ratio between the double CWT and CLD (both measured in radial direction), was computed (Denne, 1988). In non-girdled controls (n = 4 trees) and in girdled trees (n = 3 trees), anatomical features of tracheids were determined in earlywood and latewood pre- and post-girdling. Additionally, continuous records of wood anatomical parameters were determined above and below girdling and in non-girdled controls along five radial cell rows per tree, and mean values were calculated. In these time series time of girdling was deduced from intra-annual wood formation dynamics determined in P. sylvestris within the study area (Gruber et al., 2010) and abrupt alterations in anatomical traits. Because separation of individual cell walls could not be unequivocally detected in all samples, CWT of adjacent cells was recorded (double radial CWT), and this value was then halved yielding radial CWT. Cell anatomical parameters were measured by applying the image analysis software PROGRES Gryphax (Version 2.0.0.68, Jenoptik Optical Systems GmbH, Jena, Germany) and were recorded throughout five earlywood and latewood cells along five cell rows (i.e., n = 25 cells per sample), and mean values and standard deviations were calculated. The proportion of cell wall material was calculated as the ratio between CLD and CWT. A decrease/increase in CLD:CWT indicates an increase/decrease in wood density. Student’s dependent sample t test was used to determine significant differences among cell anatomical traits pre- and post-girdling and non-girdled controls.
Results
At the start of the growing season in late March/early April, mean daily air temperature and SWC reached 10°C and >20 vol%, respectively (Figures 1A,B). After frequent rainfalls in May, SWC rose to >30 vol%. Only sporadic rainfall during June and July caused SWC to drop to c. 10 vol%. Mean daily air temperature and VPD reached highest values during late June (28°C and 2.6 kPa, respectively; Figures 1B,C). Starting with rainfall events in mid-July, SWC reached again >30 vol% in early September. The observed abrupt drops in SWC following precipitation events are caused by low water holding capacity of the shallow, stony soils prevailing at the study plot (Table 1). Mean SWC during the regular and induced RG period (see Figure 2) amounted to about 21% (Table 2). Mean air temperature during these periods was 9.8 ± 3.3°C (regular RG period) and 16.5 ± 3.5°C (induced RG period). Precipitation was 31% higher during the induced RG period compared with the regular RG period (Table 2). Other environmental variables recorded at the study plot (SWC, VPD, and RH) were not significantly different between RG periods.

Table 2. Climate variables and soil water content during the regular (i.e., pre-girdling) and induced (i.e., post-girdling) growing period (cf. Figure 2).
Dendrometer traces and Gompertz growth models pre- and post-girdling are depicted in Figure 2. Regular growth period started end of March (doy 88), reached a maximum in mid-April (doy 104), and ceased end of May (doy 148). Girdling in mid-July (doy 199) of a subset of trees induced a second growth surge after about 2 weeks (doy 213), which exceeded regular RG in spring more than twofold (Figure 2 and Table 3). During regular and induced RG, the inflection point of modeled growth was reached about 2 weeks after growth onset (Table 3). While growth duration amounted to about 2 months during both growing periods, growth intensity (i.e., increment) and growth rate (i.e., slope of modeled growth at the inflection point) were quite different during regular and induced RG period. These parameters more than doubled post-girdling (Table 3).

Table 3. Parameters of the Gompertz function for radial growth dynamics of trees during the regular (i.e., pre-girdling) and induced (i.e., post-girdling) growing period (cf. Figure 2) and R2 of the model (A, upper asymptote; Ip, inflection point; κ, rate of change parameter; and Growthmax, maximum daily growth at inflection point).
Measurement of wood anatomical parameters above the girdling zone revealed that after girdling CLD and CA significantly decreased in earlywood and increased in latewood, while CWT of tracheids developed after girdling significantly increased in earlywood (Figures 3, 4). After girdling, the ratio CLD:CWT decreased in earlywood (P = 0.019) and increased in latewood (P = 0.009). Wood anatomical parameters (CLD, CA, CWT, and CLD:CWT) were all significantly different between earlywood and latewood tracheids pre-girdling (P < 0.01), but not significantly different post-girdling (P > 0.05). Continuous measurements of cell anatomy revealed that below the girdling zone cambial activity did not resume after girdling (Figures 5A–F) and last latewood tracheid showed striking decrease in CWT (-52%) and slight increase in CLD leading to decrease in wood density (Figure 5F). Above the girdling zone CLD increased sharply after girdling (Figures 5G–I) and remained at about 50% of regular earlywood-CLD except for the last formed cells, which show decreasing CLD (Figure 5G). CWT stayed constant after girdling, i.e., it remained at the level of latewood-CWT again with the exception of last formed tracheids showing a decrease in CWT (Figure 5H).
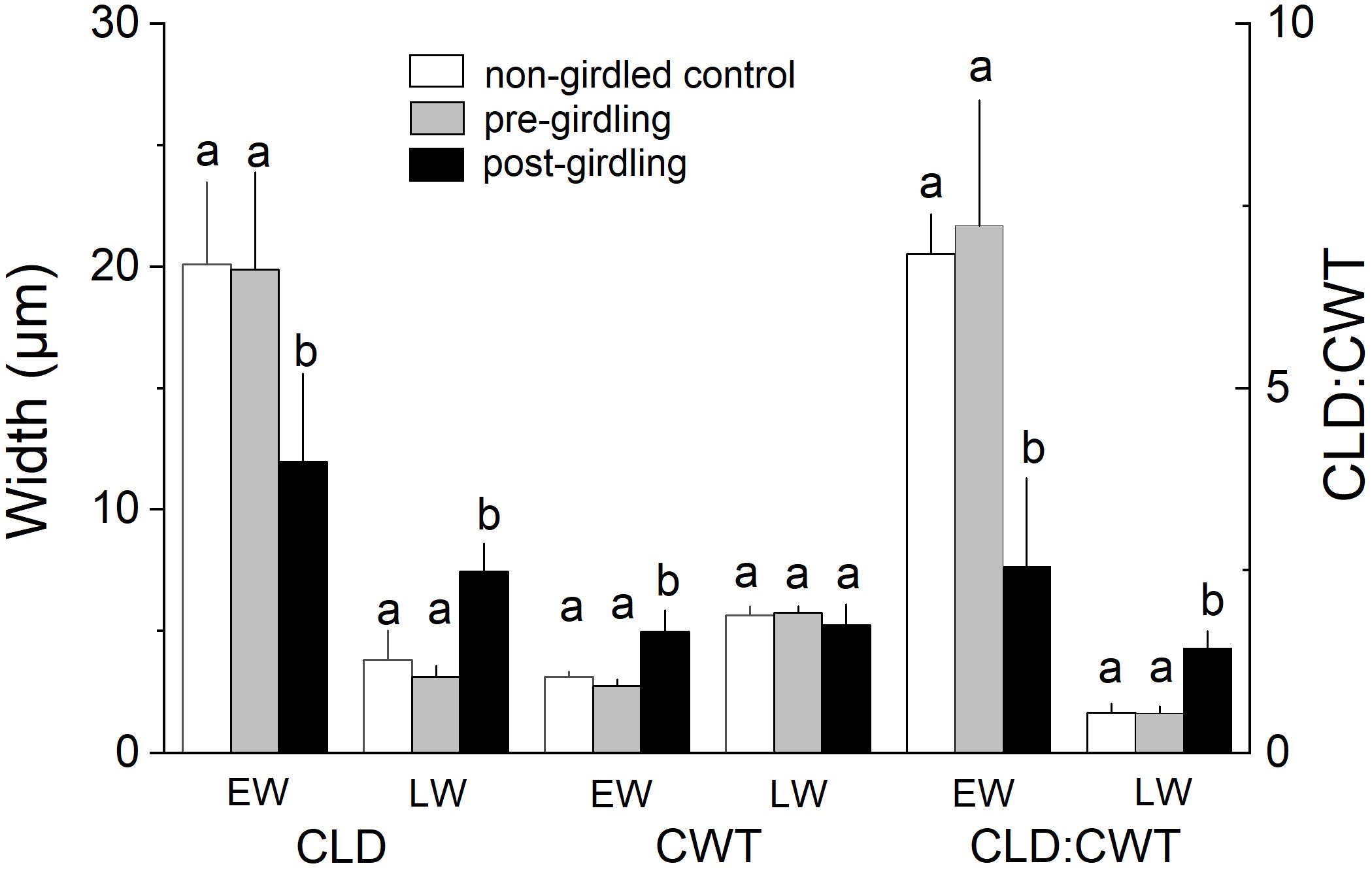
Figure 3. Radial cell lumen diameter (CLD), cell wall thickness (CWT), and ratio of CLD:CWT of earlywood (EW) and latewood (LW) tracheids in non-girdled controls, and above the girdling zone during the regular (i.e., pre-girdling) and induced growing period (i.e., post-girdling). Student’s t test was applied to determine statistically significant differences of anatomical parameters between non-girdled controls, and pre- and post-girdling. Different letters indicate significant difference at P < 0.05.
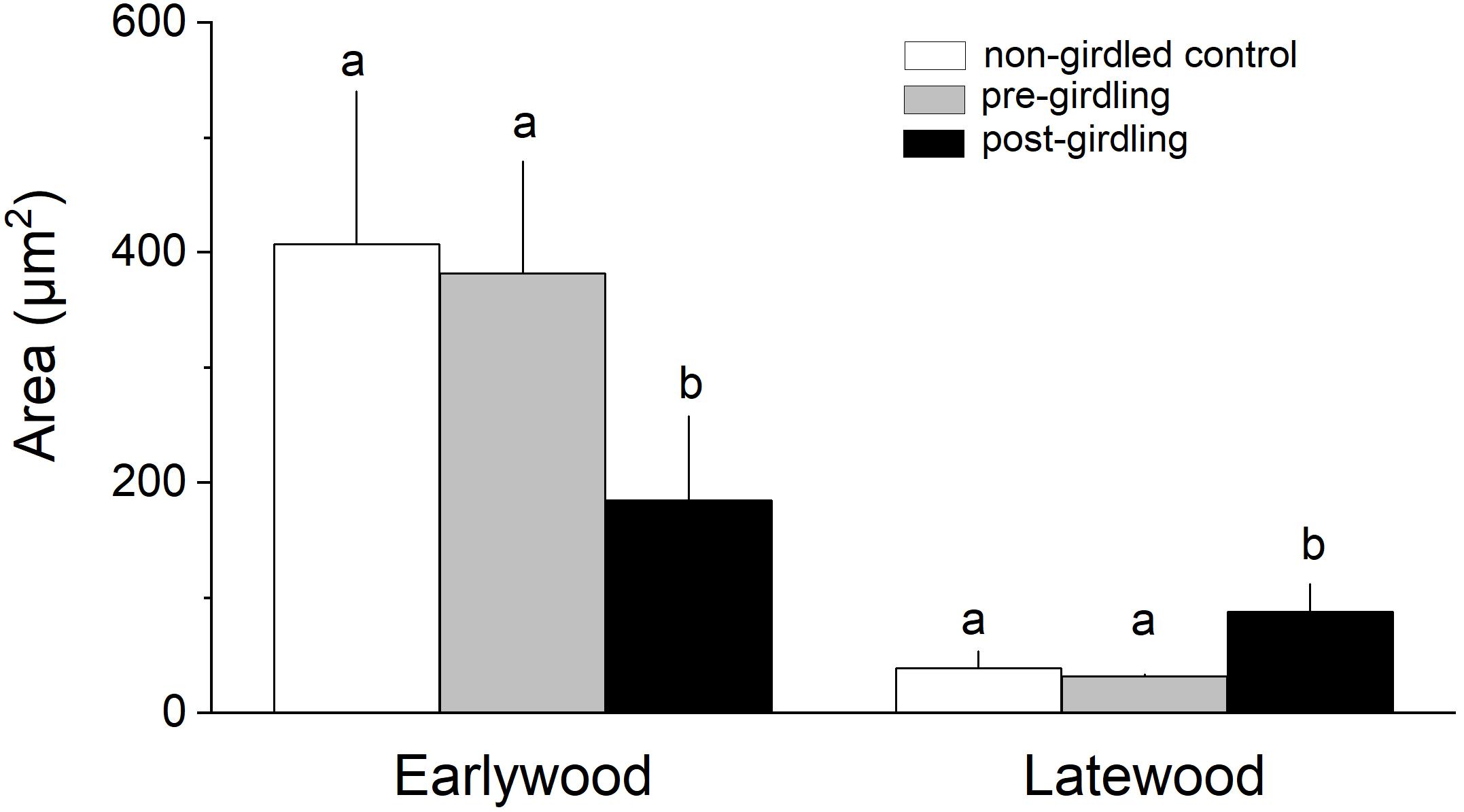
Figure 4. Cell area of earlywood (EW) and latewood (LW) tracheids in non-girdled controls, and above the girdling zone during the regular (i.e., pre-girdling) and induced growing period (i.e., post-girdling). Student’s t test was applied to determine statistically significant differences of anatomical parameters between non-girdled controls and pre- and post-girdling. Different letters indicate significant difference at P < 0.05.
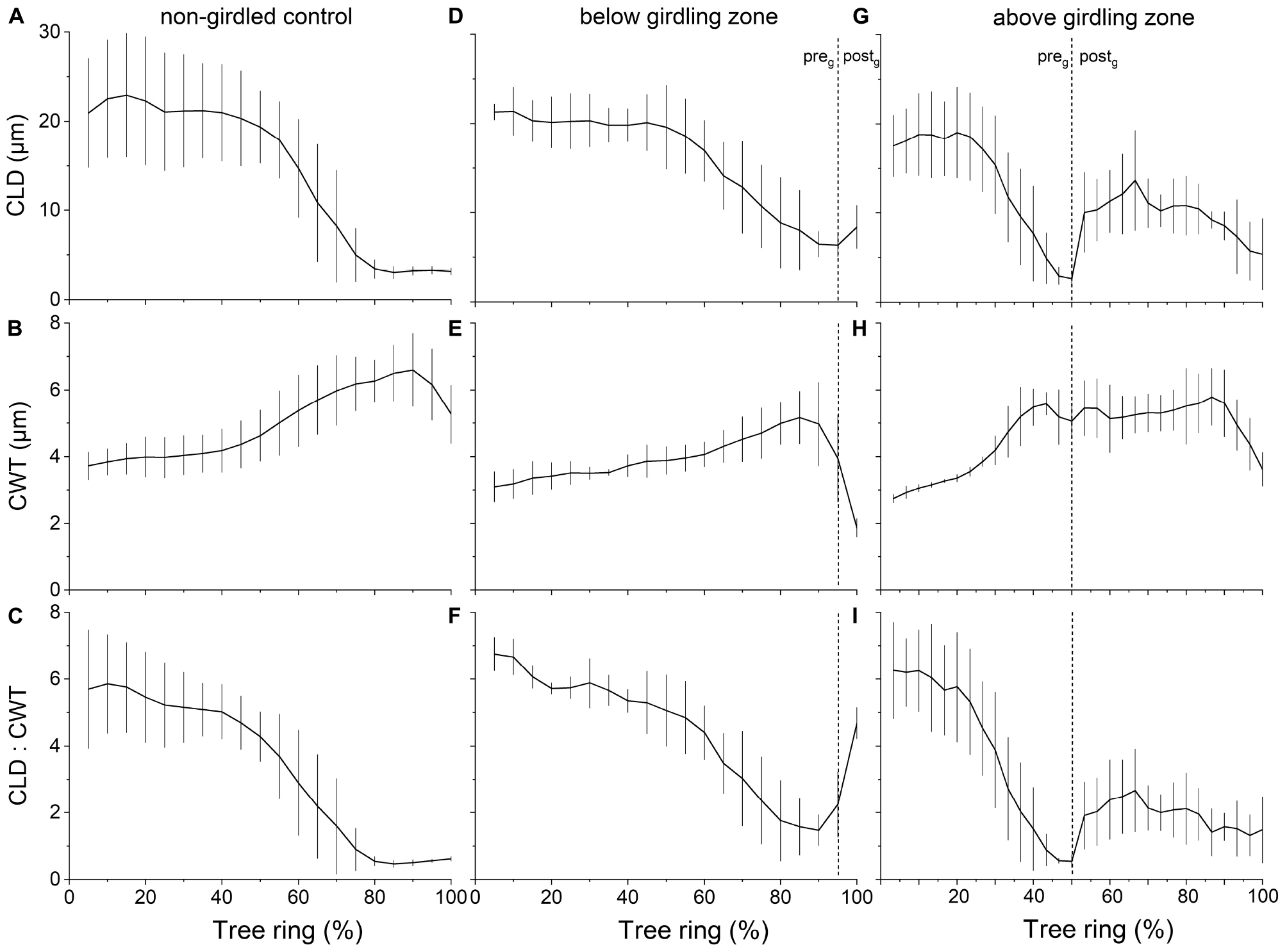
Figure 5. Change in wood anatomical parameters throughout growing period(s) in (A–C) non-girdled controls (n = 4) and (D–F) below and (G–I) above girdling (n = 3). In (D–I), the regular (i.e., pre-girdling = preg) and induced growing period (i.e., post-girdling = postg) are separated by dotted lines. Data were normalized to overall cell count of the individual tree in 2019. Mean values ± standard deviations are shown.
Discussion
Although frequently applied, girdling, i.e., removing surface tissues including outer bark, living phloem, and cambium around the stem, is a rather crude method to manipulate phloem transport compared with less-destructive methods like phloem compression or phloem chilling (Rademacher et al., 2019). Tangential rows of traumatic resin ducts and callus tissue are generally formed in conifers as a response to tissue damage (Schweingruber, 2007). Wood anatomical analyses of stem cross sections taken at the position of dendrometers, however, did not reveal presence of overgrowing callus tissue or abnormal formation of tangential rows of resin ducts after girdling, because we avoided possible wound reactions by analyzing growth response and wood anatomy 5–7 cm above the girdling zone. It has also been found that the less-destructive phloem chilling method leads to similar growth responses as girdling (De Schepper and Steppe, 2011), indicating that the applied method is a useful tool to infer growth response and changes in xylem cell differentiation provided the zone of direct wound response is avoided.
Unimodal Pattern of Stem Radial Growth of Pinus sylvestris in Dry Inner Alpine Valleys
In contrast to tree species from the Mediterranean regions (e.g., Camarero et al., 2010; Battipaglia et al., 2016; Pacheco et al., 2018), which use adequate water availability in spring and autumn for tree growth, but show strongly reduced or no growth during the dry and hot summer period, a comparable bimodal growth pattern does not occur in P. sylvestris in dry inner Alpine environments. Several authors (Gruber et al., 2010; Oberhuber et al., 2014; Swidrak et al., 2014) reported a unimodal growth pattern characterized by early peak of RG in mid-May to early June in coniferous species (P. sylvestris, P. abies, and Larix decidua) within the study area, although extended dry periods frequently occur in spring and higher precipitation during summer would provide more favorable environmental conditions for tree growth. It was suggested that extreme environmental conditions, i.e., drought stress and nutrient deficiency of the predominantly dolomite bedrock cause an early shift in C allocation from aboveground stem growth to belowground sinks to ensure adequate resource acquisition (Oberhuber and Gruber, 2010; Swidrak et al., 2013). Dendrometer records of root RG of mature P. sylvestris trees having a diameter of approximately 10 mm also revealed unimodal growth lasting from June through early August (Supplementary Figure 1).
Cambial Reactivation Induced by Girdling
Based on a previous experimental set-up using potted P. abies saplings (Oberhuber et al., 2017), we expected that girdling also triggers cambial reactivation of P. sylvestris under field conditions. Results of this study confirmed our hypothesis and revealed reactivation of cambial activity by phloem blockage 2 weeks after girdling at a drought-prone site. Girdling occurred in mid-July (doy 199), i.e., 6 weeks after cessation of the regular RG period excluding wall-thickening of latewood tracheids, which at xeric sites lasts until September (Gruber et al., 2010). Phloem blockage triggered cambial reactivation causing a bimodal RG pattern, i.e., a spring peak (doy 104) was followed by a secondary peak in summer (doy 228). While in Mediterranean areas bimodal growth is initiated by increase in water availability in autumn after intense summer drought, we could show that reactivation of cambial activity can be induced by an endogenous trigger, i.e., an increase in tree C status. In accordance with other studies (e.g., Daudet et al., 2005; De Schepper and Steppe, 2011; Oberhuber et al., 2017) results strongly suggest that interruption of C transport in the phloem to belowground sinks increased stem C availability above girdling inducing reactivation of cambial activity. This reasoning is corroborated by significant decrease in fine root mass of potted P. abies saplings in response to blockage of phloem C transport (Rainer-Lethaus and Oberhuber, 2018). Below girdling phloem blockage caused cessation of cell differentiation (evident in the form of reduced CWT of last latewood tracheids) and did not lead to a resumption of cambial activity. Hence, C availability in the stem, i.e., an endogenous factor is important for RG to occur under extreme environmental conditions. Based on findings of Smith and Stitt (2007) and Lastdrager et al. (2014), we suggest that sugar signaling—induced by interruption of phloem C transport—is involved in reactivation of cambial activity and triggering of bimodal RG in P. sylvestris trees.
Plant hormones direct growth and development and also responses to environmental stimuli (Davies, 2010). Accordingly, tree-ring formation, i.e., cambial activity and xylem cell differentiation, is induced and controlled by hormones (for a review see Buttò et al., 2020). Specifically, cambial activity is known to be highly responsive to the growth-promoting hormone auxin [indole-3-acetic acid (IAA); Uggla et al., 1998; Dünser and Kleine-Vehn, 2015; Bhalerao and Fischer, 2016] and Uggla et al. (1996) found a steep radial concentration gradient of IAA in Scots pine peaking in the cambium meristem. IAA is transported in the mature phloem in a basipetal flux from source tissues (leaf primordia and young leaves) to proximal regions (Muday and DeLong, 2001; Hacke et al., 2017). Several findings argue against the influence of accumulation of IAA above girdling on cambial reactivation: (i) heating of the stem can trigger cambial activity during dormancy (Oribe et al., 2003; Gričar et al., 2006; Begum et al., 2010), (ii) high levels of IAA are found in cambial tissues in the dormant period (Sundberg et al., 1990; Eklund et al., 1998; Funada et al., 2002), (iii) reduced responsiveness of cambial tissues to IAA during activity-dormancy transition (Baba et al., 2011), and (iv) sugar signaling can stimulate cambial cell division (e.g., Uggla et al., 2001; Lastdrager et al., 2014). The lag of about 2 weeks between girdling and onset of the induced RG period above girdling might be due to an extensive drought period at the time of girdling or as Oribe et al. (2003) suggested, that newly formed phloem cells are necessary for xylem formation to resume. The latter assumption is supported by results of Swidrak et al. (2014), who reported that phloem formation in P. sylvestris precedes xylem formation within the study area by about 3 weeks. In general, girdling induced cambial reactivation after regular spring growth was made possible because at the time of girdling the cambial meristem was not yet in endodormancy state (Lang et al., 1987; Chang et al., 2021), which can only be released by external triggers, i.e., chilling, photoperiod, and temperature (e.g., Badeck et al., 2004; Pallardy, 2008; Swidrak et al., 2011; Basler and Körner, 2014; Begum et al., 2018).
Comparison of Regular vs. Induced Radial Stem Growth
The increase of C availability above girdling strongly affected RG rate and intensity (increase by 154 and 136%, respectively, compared with regular RG), while RG duration remained quite constant amounting to about 2 months (excluding duration of cell wall thickening). Hence, more than a doubling of increment in the induced compared with regular RG period resulted from striking increase in RG rate. This finding is in accordance with Rathgeber et al. (2011), who reported that the extent of RG in silver fir (Abies alba) is more related to the rate of cell production than to its duration. Hence, phloem blockage of C transport to belowground organs provided a continuous supply of carbohydrates to sustain increased rate of cambial cell division and wood formation, processes that are considerable energy sinks (Oribe et al., 2003; Koch, 2004; Muller et al., 2011). Several authors (e.g., Simard et al., 2013; Delpierre et al., 2015; Guillemot et al., 2017) also reported that tree growth is limited by C competition between sinks rather than by C resources. During the induced RG period, which extended from August through September, C allocation was primarily restricted to cambial activity and wood formation, because within the study area shoot and needle growth of P. sylvestris ceases in June and July, respectively (Swidrak et al., 2013), and apical meristems remained in dormancy state after girdling. Similarly, internal shifts of C allocation were found to affect the second growth peak in Pinus pinaster, a species showing a bimodal RG pattern (Garcia-Forner et al., 2019). It has to be considered that water availability is the primary growth-limiting environmental factor within the study area (Pichler and Oberhuber, 2007; Oberhuber et al., 2011; Gruber et al., 2012) and therefore increase in precipitation by c. 30% during the induced compared with regular RG period might also be involved in RG increase at the xeric study site. However, air temperature was found to be inversely related to RG and stem water deficit of P. sylvestris within the study area (Oberhuber et al., 1998, 2015; Oberhuber, 2017). Therefore, strikingly higher temperatures during the induced compared with regular RG period (+6.7°C) increased evapotranspiration, which probably compensated the stimulating effect of higher precipitation on RG for the most part. Lack of increase in SWC despite higher precipitation is explained by increase in evapotranspiration together with surface run-off on the steep slope during high precipitation events in summer.
Although maximum daily RG in conifers from cold environments is related to photoperiodic growth constraint to allow xylem differentiation to be completed before early frosts occur (e.g., Rossi et al., 2006; Gruber et al., 2009), this does not apply for P. sylvestris on drought-prone sites (Swidrak et al., 2011). Because both RG periods are characterized by (i) the same duration (about 2 months), and (ii) the same length of time until the maximum daily RG rate was reached (about 2 weeks), an endogenous control of cambial activity and tracheid differentiation as an adaptation to extreme environmental conditions (drought stress, nutrient deficiency) can be assumed.
Wood Anatomical Changes After Phloem Blockage
Wood formation is a highly C demanding process (Koch, 2004; Simard et al., 2013; Cuny et al., 2015) and low sink priority of the cambium for C was frequently reported (Cannell and Dewar, 1994; Ericsson et al., 1996; Muller et al., 2011; Heinrich et al., 2015). Therefore, we expected a clear role of C accumulation above girdling on wood anatomical traits after interruption of basipetal C transport by girdling. This hypothesis was confirmed by missing significant differences (p > 0.05) of wood anatomical parameters (i.e., CLD, CA, CWT, and CLD:CWT) between earlywood and latewood tracheids after girdling, i.e., the characteristic wood anatomical pattern in conifers (thin-walled earlywood cells with large lumina vs. thick-walled latewood cells having narrow lumina) was not sustained as a result of increased C supply. Significant decrease in CLD and CA in earlywood after girdling can be explained by (i) decrease in xylem sap flow frequently reported to be a consequence of physically blocking phloem transport (e.g., Zwieniecki et al., 2004; Lopez et al., 2015; Oberhuber et al., 2017) and leading to decrease in turgor pressure and cell enlargement (Cabon et al., 2020), and/or (ii) high C availability resulting in faster cell wall deposition, which reduces cell enlargement time (Cartenì et al., 2018). That cell enlargement time primarily explains CLD in conifers was reported by Cuny et al. (2014). Increase in osmotically active C compounds after phloem blockage are required to produce adequate wall-yielding turgor pressure for cell expansion (Pantin et al., 2013; Steppe et al., 2015) and explain significant increase in CLD and CA and concomitant decrease in wood density (increase of CLD:CWT ratio) of latewood tracheids formed after girdling. Furthermore, significant increase in CWT and wood density (decrease in CLD:CWT ratio) in earlywood tracheids after girdling can also be regarded as an outcome of C accumulation above the girdling zone, because C availability is a constraint of cell wall formation (Cuny et al., 2015; Deslauriers et al., 2016; Winkler and Oberhuber, 2017; Cartenì et al., 2018).
Below the girdling zone cell differentiation ceased after interruption of C transport by phloem blockage causing a striking decrease in CWT in last few latewood tracheids (resulting in increase of CLD). Although a decrease in CWT in last latewood tracheids frequently occurs (Arzac et al., 2018; Pacheco et al., 2018; Castagneri et al., 2020), a direct link to decline in C availability by girdling can be deduced from observations that (i) cell wall thickening in P. sylvestris lasts until September within the study area (Gruber et al., 2010), (ii) reduction in C supply due to insect defoliation similarly reduces cell wall thickening in conifer species (e.g., Axelson et al., 2014; Castagneri et al., 2020; Peters et al., 2020), and (iii) cell wall thickening has a considerable C requirement (e.g., Cuny et al., 2015).
Conclusion
Our experimental study revealed that manipulation of stem C status in young P. sylvestris trees by phloem blockage triggered bimodal RG above the girdling zone and strongly affected xylem cell differentiation. Results indicate that endogenous control over C allocation, which is most likely hormonally mediated, is a key driver of RG and tracheid differentiation in addition to exogenous factors, i.e., water availability. It is reasonable to assume that a high C sink-strength of belowground root growth and/or storage organs develops early during the growing season as an adaptation to extreme site conditions, i.e., frequent drought stress and low nutrient availability of the substrate, prevailing within the study area. We conclude that tree species showing higher plasticity in RG than P. sylvestris will be at an advantage in the long term, because more variable environmental conditions are predicted under future climate change.
Data Availability Statement
The raw data supporting the conclusions of this article will be made available by the authors, without undue reservation.
Author Contributions
WO conceived and designed the experiments, and coordinated the research project. AL-W and DS measured and compiled wood anatomical data. All authors were involved in data analyses, and WO wrote the manuscript.
Funding
The research was funded by the Austrian Science Fund (FWF), P25643-B16.
Conflict of Interest
The authors declare that the research was conducted in the absence of any commercial or financial relationships that could be construed as a potential conflict of interest.
Supplementary Material
The Supplementary Material for this article can be found online at: https://www.frontiersin.org/articles/10.3389/fpls.2021.674438/full#supplementary-material
References
Arzac, A., Babushkina, E. A., Fonti, P., Slobodchikova, V., Sviderskaya, I. V., and Vaganov, E. A. (2018). Evidences of wider latewood in Pinus sylvestris from a forest-steppe of Southern Siberia. Dencrochronologia 49, 1–8. doi: 10.1016/j.dendro.2018.02.007
Axelson, J. N., Bast, A., Alfaro, R., Smith, D. J., and Gärtner, H. (2014). Variation in wood anatomical structure of Douglas-fir defoliated by the western spruce budworm, a case study in the coastal-transitional zone of British Columbia, Canada. Trees 28, 1837–1846. doi: 10.1007/s00468-014-1091-1
Baba, K., Karlberg, A., Schmidt, J., Schrader, J., Hvidsten, T. R., Bako, L., et al. (2011). Activity-dormancy transition in the cambial meristem involves stage-specific modulation of auxin response in hybrid aspen. Proc. Natl. Acad. Sci. U.S.A. 108, 3418–3424. doi: 10.1073/pnas.1011506108
Badeck, F.-W., Bondeau, A., Böttcher, K., Doktor, D., Lucht, W., Schaber, J., et al. (2004). Responses of spring phenology to climate change. New Phytol. 162, 295–309. doi: 10.1111/j.1469-8137.2004.01059.x
Balducci, L., Deslauriers, A., Giovannelli, A., Rossi, S., and Rathgeber, C. B. K. (2013). Effects of temperature and water deficit on cambial activity and woody ring features in Picea mariana saplings. Tree Physiol. 33, 1006–1017. doi: 10.1093/treephys/tpt073
Basler, D., and Körner, C. (2014). Photoperiod and temperature responses of bud swelling and bud burst in four temperate forest tree species. Tree Physiol. 34, 377–388. doi: 10.1093/treephys/tpu021
Battipaglia, G., Campelo, F., Vieira, J., Grabner, M., De Micco, V., Nabais, C., et al. (2016). Structure and function of intra–annual density fluctuations: mind the gaps. Front. Plant Sci. 7:595. doi: 10.3389/fpls.2016.00595
Battipaglia, G., De Micco, V., Brand, W. A., Linke, P., Aronne, G., Saurer, M., et al. (2010). Variations of vessel diameter and δ13C in false rings of Arbutus unedo L. reflect different environmental conditions. New Phytol. 188, 1099–1112. doi: 10.1111/j.1469-8137.2010.03443.x
Begum, S., Kudo, K., Rahman, M. D. H., Nakaba, S., Yamagishi, Y., Nabeshima, E., et al. (2018). Climate change and the regulation of wood formation in trees by temperature. Trees 32, 3–15. doi: 10.1007/s00468-017-1587-6
Begum, S., Nakaba, S., Oribe, Y., Kubo, T., and Funada, R. (2010). Cambial sensitivity to rising temperatures by natural condition and artificial heating from late winter to early spring in the evergreen conifer Cryptomeria japonica. Trees 24, 43–52. doi: 10.1007/s00468-009-0377-1
Bhalerao, R. P., and Fischer, U. (2016). Environmental and hormonal control of cambial stem cell dynamics. J. Exp. Bot. 68, 79–87. doi: 10.1093/jxb/erw466
Brunner, I., Herzog, C., Dawes, M. A., Arend, M., and Sperisen, C. (2015). How tree roots respond to drought. Front. Plant Sci. 6:547. doi: 10.3389/fpls.2015.00547
Buttò, V., Deslauriers, A., Rossi, S., Rozenberg, P., Shishov, V., and Morin, H. (2020). The role of plant hormones in tree-ring formation. Trees 34, 315–335. doi: 10.1007/s00468-019-01940-4
Cabon, A., Fernàndez-de-Uña, L., Gea-Izquierdo, G., Meinzer, F. C., Woodruff, D. R., Martìnez-Vilalta, J., et al. (2020). Water potential control of turgor-driven tracheid enlargement in Scots pine at its xeric distribution edge. New Phytol. 225, 209–221. doi: 10.1111/nph.16146
Camarero, J. J., Olano, J. M., and Parras, A. (2010). Plastic bimodal xylogenesis in conifers from continental Mediterranean climates. New Phytol. 185, 471–480. doi: 10.1111/j.1469-8137.2009.03073.x
Campelo, F., Gutiérrez, E., Ribas, M., Sánchez-Salguero, R., Nabais, C., and Camarero, J. J. (2018). The facultative bimodal growth pattern in Quercus ilex – a simple model to predict sub-seasonal and inter-annual growth. Dendrochronologia 49, 77–88. doi: 10.1016/j.dendro.2018.03.001
Campelo, F., Nabais, C., Freitas, H., and Gutiérrez, E. (2007). Climatic significance of tree-ring width and intra-annual density fluctuations in Pinus pinea from a dry Mediterranean area in Portugal. Ann. For. Sci. 64, 229–238. doi: 10.1051/forest:2006107
Campelo, F., Vieira, J., and Nabais, C. (2013). Tree-ring growth and intra-annual density fluctuations of Pinus pinaster responses to climate: does size matter? Trees 27, 763–772. doi: 10.1007/s00468-012-0831-3
Cannell, M. G. R., and Dewar, R. C. (1994). Carbon allocation in trees: a review of concepts for modeling. Adv. Ecol. Res. 25, 59–104. doi: 10.1016/s0065-2504(08)60213-5
Cartenì, F., Deslauriers, A., Rossi, S., Morin, H., de Micco, V., Mazzoleni, S., et al. (2018). The physiological mechanisms behind the earlywood-to-latewood transition: a process-based modeling approach. Front. Plant Sci. 9:1053. doi: 10.3389/fpls.2018.01053
Castagneri, D., Prendin, A. L., Peters, R. L., Carrer, M., von Arx, G., and Fonti, P. (2020). Long-term impacts of defoliator outbreaks on larch xylem structure and tree-ring biomass. Front. Plant Sci. 11:1078. doi: 10.3389/fpls.2020.01078
Chang, C. Y.-Y., Bräutigam, K., Hüner, N. P. A., and Ensminger, I. (2021). Champions of winter survival: cold acclimation and molecular regulation of cold hardiness in evergreen conifers. New Phytol. 229, 675–691. doi: 10.1111/nph.16904
Cuny, H. E., Rathgeber, C. B. K., Frank, D., Fonti, P., and Fournier, M. (2014). Kinetics of tracheid development explain conifer tree-ring structure. New Phytol. 203, 1231–1241. doi: 10.1111/nph.12871
Cuny, H. E., Rathgeber, C. B. K., Frank, D., Fonti, P., Mäkinen, H., Prislan, P., et al. (2015). Woody biomass production lags stem-girth increase by over one month in coniferous forests. Nat. Plants 1, 1–6. doi: 10.1038/NPLANTS.2015.16
Daudet, F. A., Ameglio, T., Cochard, H., Archilla, O., and Lacointe, A. (2005). Experimental analysis of the role of water and carbon in tree stem diameter variations. J. Exp. Bot. 56, 135–144.
Davies, P. J. (2010). Plant Hormones: Biosynthesis, Signal Transduction, Action!. Dordrecht: Springer Netherlands, 802.
De Luis, M., Gričar, J., Čufar, K., and Raventós, J. (2007). Seasonal dynamics of wood formation in Pinus halepensis from dry and semi-arid ecosystems in Spain. IAWA J. 28, 389–404. doi: 10.1163/22941932-90001651
De Luis, M., Novak, K., Raventós, J., Gričar, J., Prislan, P., and Čufar, K. (2011). Cambial activity, wood formation and sapling survival of Pinus halepensis exposed to different irrigation regimes. For. Ecol. Manage. 262, 1630–1638. doi: 10.1016/j.foreco.2011.07.013
De Micco, V., Campelo, F., de Luis, M., Bräuning, A., Grabner, M., and Cherubini, P. (2016). Intra-annual density fluctuations in tree rings: how, when, where, and why? IAWA J. 37, 232–259. doi: 10.1163/22941932-20160132
De Micco, V., Carrer, M., Rathgeber, C. B. K., Camarero, J. J., Voltas, J., Cherubini, P., et al. (2019). From xylogenesis to tree rings: wood traits to investigate tree response to environmental changes. IAWA J. 40, 155–182. doi: 10.1163/22941932-40190246
De Schepper, V., and Steppe, K. (2011). Tree girdling responses simulated by a water and carbon transport model. Ann. Bot. 108, 1147–1154. doi: 10.1093/aob/mcr068
Delpierre, N., Berveiller, D., Granda, E., and Dufrene, E. (2015). Wood phenology, not carbon input, controls the interannual variability of wood growth in a temperate oak forest. New Phytol. 210, 459–470. doi: 10.1111/nph.13771
Denne, M. P. (1988). Definition of latewood according to Mork (1928). IAWA Bull. 10, 59–62. doi: 10.1163/22941932-90001112
Deslauriers, A., Huang, J.-G., Balducci, L., Beaulieu, M., and Rossi, S. (2016). The contribution of carbon and water in modulating wood formation in black spruce saplings. Plant Physiol. 170, 2072–2084. doi: 10.1104/pp.15.01525
Deslauriers, A., Rossi, S., and Anfodillo, T. (2007). Dendrometer and intra-annual tree growth: what kind of information can be inferred? Dendrochronologia 25, 113–124. doi: 10.1016/j.dendro.2007.05.003
Dünser, K., and Kleine-Vehn, J. (2015). Differential growth regulation in plants–the acid growth balloon theory. Curr. Opin. Plant. Biol. 28, 55–59. doi: 10.1016/j.pbi.2015.08.009
Eklund, L., Little, C. H. A., and Riding, R. T. (1998). Concentrations of oxygen and indole-3- acetic acid in the cambial region during latewood formation and dormancy development in Picea abies stems. J. Exp. Bot. 49, 205–211. doi: 10.1093/jexbot/49.319.205
Ellenberg, H., and Leuschner, C. (2010). Vegetation Mitteleuropas Mit Den Alpen in Ökologischer, Dynamischer und Historischer Sicht. Stuttgart: Ulmer Velag.
Ericsson, T., Rytter, L., and Vapaavuori, E. (1996). Physiology of carbon allocation in trees. Biomass Bioenergy 11, 115–127. doi: 10.1016/0961-9534(96)00032-3
Funada, R., Kubo, T., Sugiyama, T., and Fushitani, M. (2002). Changes in levels of endogenous plant hormones in cambial regions of stems of Larix kaempferi at the onset of cambial activity in springtime. J. Wood Sci. 48, 75–80. doi: 10.1007/bf00766242
Garcia-Forner, N., Vieira, J., Nabais, C., Carvalho, A., Martínez-Vilalta, J., and Campelo, F. (2019). Climatic and physiological regulati on of the bimodal xylem formation pattern in Pinus pinaster saplings. Tree Physiol. 39, 2008–2018. doi: 10.1093/treephys/tpz099
Gričar, J., Zupančič, M., Čufar, K., Koch, G., Schmitt, U., and Oven, P. (2006). Effect of local heating and cooling on cambial activity and cell differentiation in the stem of Norway spruce (Picea abies). Ann. Bot. 97, 943–951. doi: 10.1093/aob/mcl050
Gruber, A., Baumgartner, D., Zimmermann, J., and Oberhuber, W. (2009). Temporal dynamic of wood formation in Pinus cembra along the alpine treeline ecotone and the effect of climate variables. Trees 23, 623–635. doi: 10.1007/s00468-008-0307-7
Gruber, A., Pirkebner, D., Florian, C., and Oberhuber, W. (2012). No evidence for depletion of carbohydrate pools in Scots pine (Pinus sylvestris L.) under drought stress. Plant Biol. 14, 142–148.
Gruber, A., Strobl, S., Veit, B., and Oberhuber, W. (2010). Impact of drought on the temporal dynamics of wood formation in Pinus sylvestris. Tree Physiol. 30, 490–501. doi: 10.1093/treephys/tpq003
Guillemot, J., Francois, C., Hmimina, G., Dufrene, E., Martin-StPaul, N. K., Soudani, K., et al. (2017). Environmental control of carbon allocation matters for modelling forest growth. New Phytol. 214, 180–193. doi: 10.1111/nph.14320
Gutiérrez, E., Campelo, F., Camarero, J. J., Ribas, M., Muntán, E., Nabais, C., et al. (2011). Climate controls act at different scales on the seasonal pattern of Quercus ilex L. stem radial increments in NE Spain. Trees 25, 637–646. doi: 10.1007/s00468-011-0540-3
Hacke, U. G., Spicer, R., Schreiber, S. G., and Plavcová, L. (2017). An ecophysiological and.developmental perspective on variation in vessel diameter. Plant Cell Environ. 40, 831–845 doi: 10.1111/pce.12777
Hagedorn, F., Joseph, J., Peter, M., Luster, J., Pritsch, K., Geppert, U., et al. (2016). Recovery of trees from drought depends on belowground sink control. Nat. Plants 2:16111. doi: 10.1038/NPLANTS.2016.111
Hartmann, H., Bahn, M., Carbone, M., and Richardson, A. D. (2020). Plant carbon allocation in a changing world–challenges and progress: introduction to a virtual issue on carbon allocation. New Phytol. 227, 981–988. doi: 10.1111/nph.16757
Heinrich, S., Dippold, M. A., Werner, C., Wiesenberg, G. L., Kuzyakov, Y., and Glaser, B. (2015). Allocation of freshly assimilated carbon into primary and secondary metabolites after in situ 13C pulse labelling of Norway spruce (Picea abies). Tree Physiol. 35, 1176–1191.
Koch, K. (2004). Sucrose metabolism: regulatory mechanisms and pivotal roles in sugar sensing and plant development. Curr. Opin. Plant Biol. 7, 235–246. doi: 10.1016/j.pbi.2004.03.014
Körner, C. (2003). Carbon limitation in trees. J. Ecol. 91, 4–17. doi: 10.1046/j.1365-2745.2003.00742.x
Lang, G. A., Early, J. D., Martin, G. C., and Darnell, R. L. (1987). Endo-, para-, and ecodormancy: physiological terminology and classification for dormancy research. Hort. Sci. 22, 371–377.
Lastdrager, J., Hanson, J., and Smeekens, S. (2014). Sugar signals and the control of plant growth and development. J. Exp. Bot. 65, 799–807. doi: 10.1093/jxb/ert474
Lopez, R., Brossa, R., Gil, L., and Pita, P. (2015). Stem girdling evidences a trade-off between cambial activity and sprouting and dramatically reduces plant transpiration due to feedback inhibition of photosynthesis and hormone signaling. Front. Plant Sci. 6:285. doi: 10.3389/fpls.2015.00285
Maier, C. A., Johnsen, K. H., Clinton, B. D., and Ludovici, K. H. (2010). Relationships between stem CO2 efflux, substrate supply, and growth in young loblolly pine trees. New Phytol. 185, 502–513. doi: 10.1111/j.1469-8137.2009.03063.x
Maunoury-Danger, F., Fresneau, C., Eglin, T., Berveiller, D., François, C., Lelarge- Trouverie, C., et al. (2010). Impact of carbohydrate supply on stem growth, wood and respired CO2 δ13C: assessment by experimental girdling. Tree Physiol. 30, 818–830. doi: 10.1093/treephys/tpq039
Muday, G. K., and DeLong, A. (2001). Polar auxin transport: controlling here and how much. Trends Plant Sci. 6, 535–542. doi: 10.1016/s1360-1385(01)02101-x
Muller, B., Pantin, F., Genard, M., Turc, O., Freixes, S., Piques, M., et al. (2011). Water deficits uncouple growth from photosynthesis, increase C content, and modify the relationships between C and growth in sink organs. J. Exp. Bot. 62, 1715–1729. doi: 10.1093/jxb/erq438
Novak, K., Saz Sánchez, M. A., Čufar, K., Raventós, J., and de Luis, M. (2013). Age, climate and intra-annual density fluctuations in Pinus halepensis in Spain. IAWA J. 34, 459–474. doi: 10.1163/22941932-00000037
Oberhuber, W. (2017). Soil water availability and evaporative demand affects seasonal growth dynamics and use of stored water in co-occurring saplings and mature conifers under drought. Trees 31, 467–478. doi: 10.1007/s00468-016-1468-4
Oberhuber, W., and Gruber, A. (2010). Climatic influences on intra-annual stem radial increment of Pinus sylvestris (L.) exposed to drought. Trees 24, 887–898. doi: 10.1007/s00468-010-0458-1
Oberhuber, W., Gruber, A., Kofler, W., and Swidrak, I. (2014). Radial stem growth in response to microclimate and soil moisture in a drought-prone mixed coniferous forest at an inner Alpine site. Eur. J. For. Res. 133, 467–479. doi: 10.1007/s10342-013-0777-z
Oberhuber, W., Gruber, A., Lethaus, G., Winkler, A., and Wieser, G. (2017). Stem girdling indicates prioritized carbon allocation to the root system at the expense of radial stem growth in Norway spruce under drought conditions. Environ. Exp. Bot. 138, 109–118. doi: 10.1016/j.envexpbot.2017.03.004
Oberhuber, W., Kofler, W., Schuster, R., and Wieser, G. (2015). Environmental effects on stem water deficit in co-occurring conifers exposed to soil dryness. Int. J. Biometeorol. 59, 417–426. doi: 10.1007/s00484-014-0853-1
Oberhuber, W., Stumböck, M., and Kofler, W. (1998). Climate-tree growth relationships of Scots pine stands (Pinus sylvestris L.) exposed to soil dryness. Trees 13, 19–27. doi: 10.1007/pl00009734
Oberhuber, W., Swidrak, I., Pirkebner, D., and Gruber, A. (2011). Temporal dynamics of non-structural carbohydrates and xylem growth in Pinus sylvestris exposed to drought. Can. J. For. Res. 41, 1590–1597. doi: 10.1139/x11-084
Oribe, Y., Funada, R., and Kubo, T. (2003). Relationships between cambial activity, cell differentiation and the localization of starch in storage tissues around the cambium in locally heated stems of Abies sachalinensis (Schmidt) Masters. Trees 17, 185–192. doi: 10.1007/s00468-002-0231-1
Pacheco, A., Camarero, J. J., and Carrer, M. (2016). Linking wood anatomy and xylogenesis allows pinpointing of climate and drought influences on growth of coexisting conifers in continental Mediterranean climate. Tree Physiol. 36, 502–512. doi: 10.1093/treephys/tpv125
Pacheco, A., Camarero, J. J., Ribas, M., Gazol, A., Gutierrez, E., and Carrer, M. (2018). Disentangling the climate-driven bimodal growth pattern in coastal and continental Mediterranean pine stands. Sci. Tot. Env. 615, 1518–1526. doi: 10.1016/j.scitotenv.2017.09.133
Pantin, F., Fanciullino, A. L., Massonnet, C., Dauzat, M., Simonneau, T., and Muller, B. (2013). Buffering growth variations against water deficits through timely carbon usage. Front. Plant Sci. 4:483. doi: 10.3389/fpls.2013.00483
Peters, R. L., Miranda, J. C., Schönbeck, L., Nievergelt, D., Fonti, M. V., Saurer, M., et al. (2020). Tree physiological monitoring of the 2018 larch budmoth outbreak: preference for leaf recovery and carbon storage over stem wood formation in Larix decidua. Tree Physiol. 40, 1697–1711. doi: 10.1093/treephys/tpaa087
Pichler, P., and Oberhuber, W. (2007). Radial growth response of coniferous forest trees in an inner Alpine environment to heat-wave in 2003. For. Ecol. Manage. 242, 688–699. doi: 10.1016/j.foreco.2007.02.007
Plomion, C., Leprovost, G., and Stokes, A. (2001). Wood formation in trees. Plant Physiol. 127, 1513–1523.
Prenger, J. J., and Ling, P. P. (2000). Greenhouse Condensation Control: Understanding and Using Vapour Pressure Deficit (VPD). Fact Sheet (Series) AEX-800. Columbus, OH: Ohio State University Extension.
Rademacher, T. T., Basler, D., Eckes-Shephard, A. H., Fonti, P., Friend, A. D., Moine, J. L., et al. (2019). Using direct phloem transport manipulation to advance understanding of carbon dynamics in forest trees. Front. For. Glob. Ch. 2:11. doi: 10.3389/ffgc.2019.00011
Rainer-Lethaus, G., and Oberhuber, W. (2018). Phloem girdling of Norway spruce alters quantity and quality of wood formation in roots particularly under drought. Front. Plant Sci. 9:392. doi: 10.3389/fpls.2018.00392
Rathgeber, C. B. K., Rossi, S., and Bontemps, J.-D. (2011). Cambial activity related to tree size in a mature silver-fir plantation. Ann. Bot. 108, 429–438. doi: 10.1093/aob/mcr168
Rigling, A., Bräker, O. U., Schneiter, G., and Schweingruber, F. H. (2002). Intra-annual tree-ring parameters indicating differences in drought stress of Pinus sylvestris forests within the Erico-Pinion in the Valais (Switzerland). Plant Ecol. 163, 105–121.
Rossi, S., Deslauriers, A., Anfodillo, T., Morin, H., Saracino, A., Motta, R., et al. (2006). Conifers in cold environments synchronize maximum growth rate of tree-ring formation with day length. New Phytol. 170, 301–310. doi: 10.1111/j.1469-8137.2006.01660.x
Rossi, S., Deslauriers, A., and Morin, H. (2003). Application of the Gompertz equation for the study of xylem cell development. Dendrochronologia 21, 33–39. doi: 10.1078/1125-7865-00034
Rozas, V., García-González, I., and Zas, R. (2011). Climatic control of intra-annual wood density fluctuations of Pinus pinaster in NW Spain. Trees 25, 443–453. doi: 10.1007/s00468-010-0519-5
Schuster, R., and Oberhuber, W. (2013). Drought sensitivity of three co-occurring conifers within a dry inner Alpine environment. Trees 27, 61–69. doi: 10.1007/s00468-012-0768-6
Shipley, B., and Meziane, D. (2002). The balanced-growth hypothesis and the allometry of leaf and roots biomass allocation. Funct. Ecol. 16, 326–331. doi: 10.1046/j.1365-2435.2002.00626.x
Simard, S., Giovannelli, A., Treydte, K., Traversi, M. L., King, G. M., Frank, D., et al. (2013). Intra-annual dynamics of non-structural carbohydrates in the cambium of mature conifer trees reflects radial growth demands. Tree Physiol. 33, 913–923. doi: 10.1093/treephys/tpt075
Smith, A. M., and Stitt, M. (2007). Coordination of carbon supply and plant growth. Plant Cell Environ. 30, 1126–1149. doi: 10.1111/j.1365-3040.2007.01708.x
Steppe, K., Sterck, F., and Deslauriers, A. (2015). Diel growth dynamics in tree stems: linking anatomy and ecophysiology. Trends Plant Sci. 20, 335–343. doi: 10.1016/j.tplants.2015.03.015
Sterck, F. J., Zweifel, R., Sass-Klaassen, U., and Chowdhury, Q. (2008). Persisting soil drought reduces leaf specific conductivity in Scots pine (Pinus sylvestris) and pubescent oak (Quercus pubescens). Tree Physiol. 28, 528–536.
Sundberg, B., Little, C. H. A., and Cui, K. (1990). Distribution of indole-3-acetic acid and the occurrence of its alkali-labile conjugates in the extraxylary region of Pinus sylvestris stems. Plant Physiol. 93, 1295–1302. doi: 10.1104/pp.93.4.1295
Swidrak, I., Gruber, A., Kofler, W., and Oberhuber, W. (2011). Effects of environmental conditions on onset of xylem growth in Pinus sylvestris under drought. Tree Physiol. 31, 483–493. doi: 10.1093/treephys/tpr034
Swidrak, I., Gruber, A., and Oberhuber, W. (2014). Xylem and phloem phenology in co-occurring conifers exposed to drought. Trees 28, 1161–1171. doi: 10.1007/s00468-014-1026-x
Swidrak, I., Schuster, R., and Oberhuber, W. (2013). Comparing growth phenology of co-occurring deciduous and evergreen conifers exposed to drought. Flora 208, 609–617. doi: 10.1016/j.flora.2013.09.004
Uggla, C., Magel, E., Moritz, T., and Sundberg, B. (2001). Function and dynamics of auxin and carbohydrates during early wood/latewood transition in Scots pine. Plant Physiol. 125, 2029–2039. doi: 10.1104/pp.125.4.2029
Uggla, C., Mellerowicz, E. J., and Sundberg, B. (1998). Indole-3-acetic acid controls cambial growth in Scots pine by positional signaling. Plant Physiol. 117, 113–121. doi: 10.1104/pp.117.1.113
Uggla, C., Moritz, T., Sandberg, G., and Sundberg, B. (1996). Auxin as a positional signal in pattern formation in plants. Proc. Natl. Acad. Sci. U.S.A. 93, 9282–9286. doi: 10.1073/pnas.93.17.9282
van der Werf, G. W., Sass-Klaassen, U. G. W., and Mohren, G. M. J. (2007). The impact of the 2003 summer drought on the intra-annual growth pattern of beech (Fagus sylvatica L.) and oak (Quercus robur L.) on a dry site in the Netherlands. Dendrochronologia 25, 103–112. doi: 10.1016/j.dendro.2007.03.004
Vieira, J., Campelo, F., and Nabais, C. (2009). Age-dependent responses of tree-ring growth and intra-annual density fluctuations of Pinus pinaster to Mediterranean climate. Trees 23, 257–265. doi: 10.1007/s00468-008-0273-0
Wimmer, R., Strumia, G., and Holawe, F. (2000). Use of false rings in Austrian pine to reconstruct early growing season precipitation. Can. J. For. Res. 30, 1691–1697. doi: 10.1139/x00-095
Winkler, A., and Oberhuber, W. (2017). Cambial response of Norway spruce to modified carbon availability by phloem girdling. Tree Physiol. 37, 1527–1535. doi: 10.1093/treephys/tpx077
Zeide, B. (1993). Analysis of growth equations. For. Sci. 39, 594–616. doi: 10.1093/forestscience/39.3.594
Zweifel, R., Zimmermann, L., Zeugin, F., and Newberry, D. M. (2006). Intra-annual radial growth and water relations of trees: implications towards a growth mechanism. J. Exp. Bot. 57, 1445–1459. doi: 10.1093/jxb/erj125
Keywords: bimodal growth, cambial activity, carbon availability, drought, girdling, radial growth, Scots pine, wood anatomy
Citation: Oberhuber W, Landlinger-Weilbold A and Schröter DM (2021) Triggering Bimodal Radial Stem Growth in Pinus sylvestris at a Drought-Prone Site by Manipulating Stem Carbon Availability. Front. Plant Sci. 12:674438. doi: 10.3389/fpls.2021.674438
Received: 01 March 2021; Accepted: 09 April 2021;
Published: 28 May 2021.
Edited by:
Sebastian Leuzinger, Auckland University of Technology, New ZealandReviewed by:
Paolo Cherubini, Snow and Landscape Research (WSL), SwitzerlandRoman Zweifel, Snow and Landscape Research (WSL), Switzerland
Copyright © 2021 Oberhuber, Landlinger-Weilbold and Schröter. This is an open-access article distributed under the terms of the Creative Commons Attribution License (CC BY). The use, distribution or reproduction in other forums is permitted, provided the original author(s) and the copyright owner(s) are credited and that the original publication in this journal is cited, in accordance with accepted academic practice. No use, distribution or reproduction is permitted which does not comply with these terms.
*Correspondence: Walter Oberhuber, V2FsdGVyLk9iZXJodWJlckB1aWJrLmFjLmF0
†These authors have contributed equally to this work