- College of Life Sciences, National Key Laboratory of Wheat and Maize Crop Science, Henan Agricultural University, Zhengzhou, China
Plant peptides secreted as signal molecular to trigger cell-to-cell signaling are indispensable for plant growth and development. Successful sexual reproduction in plants requires extensive communication between male and female gametophytes, their gametes, and with the surrounding sporophytic tissues. In the past decade, it has been well-documented that small peptides participate in many important reproductive processes such as self-incompatibility, pollen tube growth, pollen tube guidance, and gamete interaction. Here, we provide a comprehensive overview of the peptides regulating the processes of male-female crosstalk in plant, aiming at systematizing the knowledge on the sexual reproduction, and signaling of plant peptides in future.
Introduction
Small peptides refer to proteins that are less than 100 amino acids broadly (Hsu and Benfey, 2018), which can be divided into three categories from the source: processed from the original precursor protein; directly translated from an independent small open reading frame (ORF); and encoded by a small ORF in the 5' or 3' untranslated region (UTR) within a normal size protein. Small peptides used as signals are usually secreted proteins which are mainly divided into post-translationally modified small peptides and small cysteine-rich peptides (CRPs; Matsubayashi, 2003). High-throughput sequencing has predicted a large number of small peptide-encoding genes in a variety of plant genomes, and their functions have gradually attracted attention. It is well-established that small peptides are involved in many growth and development processes such as cell proliferation (Imin et al., 2013; Djordjevic et al., 2015), root development (Delay et al., 2013a,b; Mohd-Radzman et al., 2015; Taleski et al., 2016, 2018; Patel et al., 2018), pollen fertility (Okuda et al., 2009; Takeuchi and Higashiyama, 2012, 2016; Higashiyama and Takeuchi, 2015), stomata opening (Takahashi et al., 2018; Qu et al., 2019), absorption and regulation of mineral elements (Taleski et al., 2018), resistance to pests and diseases (Stotz et al., 2009a,b; Ziemann et al., 2018), and environmental adaptation.
Fertilization is a process in which male-female cells interact and fuse with each other in plants. Pollen grains fall onto the stigma through pollination and germinate to form a pollen tube which transports sperm cells through the stigma and grows into the embryo sac along the transmitting tract (Figure 1). A sperm cell fuses with the egg cell to form a zygote which develops into an embryo; the other sperm cell fuses with the central cell to form a fertilized polar nucleus which develops into an endosperm (Hamamura et al., 2012). The successful completion of fertilization relies on the continuous recognition and interaction between female and male cells. The basis for completing these processes is signal communication.
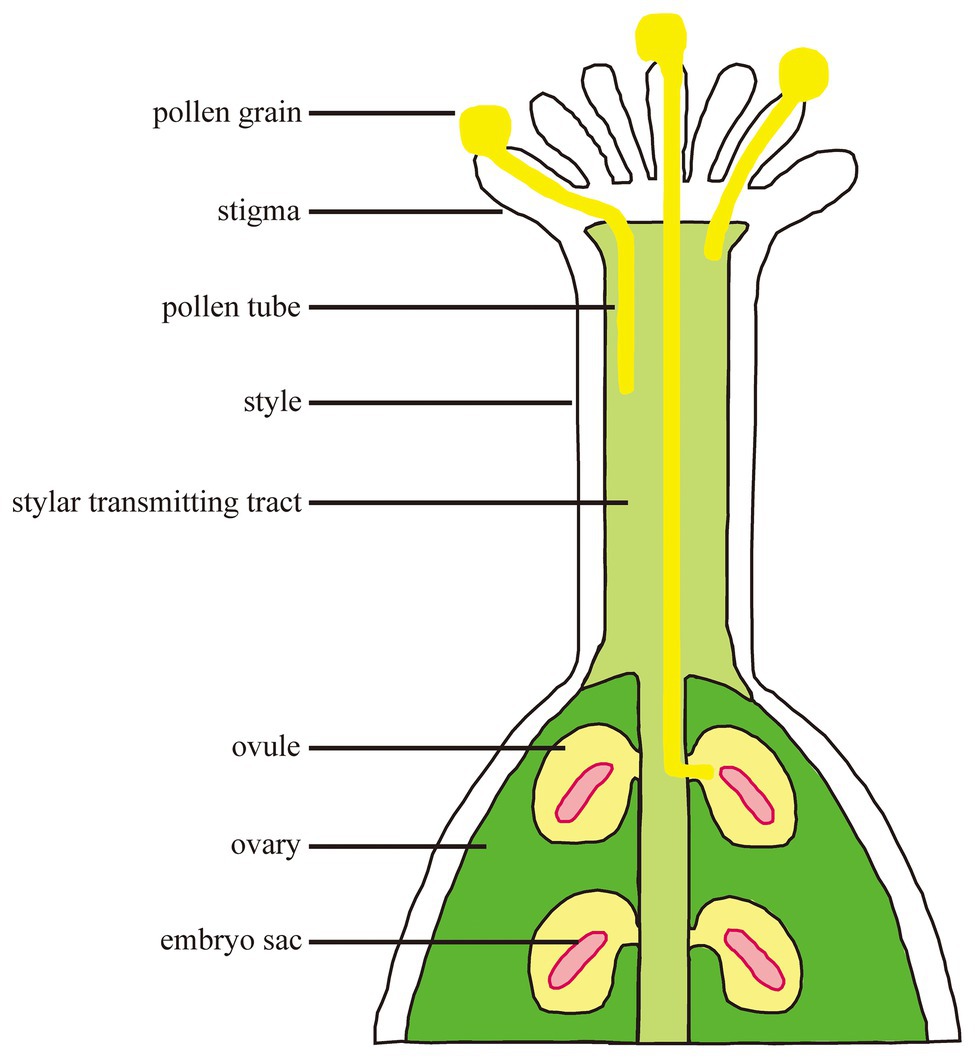
Figure 1. The processes from pollination to fertilization. Pollen grains interact with stigma and germinate. Pollen tube polar growth along the transmitting tract. The pollen tube enters the female gametophyte for fertilization.
In recent years, a good many of studies have demonstrated the important role of small peptides in male-female crosstalk in plants (Kim et al., 2021). Different small peptides involved in pollen grains-stigma recognition, pollen tube germination, polar growth and reception, ovule attraction, gamete activation, and other processes have been identified. The identification and functional analysis of small peptide during male-female crosstalks are helpful to reveal the formation mechanism of species in plants. Peptide-receptor interaction is the reason for the formation of inter-species isolation. Therefore, researches in this field have great significance for overcoming the reproductive barriers between different species.
Small Peptides Involved in Important Processes of Plant Reproduction
Self-Incompatibility
Self-incompatibility is the pre-fertilization reproductive barrier of many plants. The pollen grains fall on the stigma and recognize with the papilla cells quickly before germination, causing interspecific incompatibility and self-incompatibility, preventing different species from crossing and selfing decline (Takayama and Isogai, 2005). Self-incompatibility includes gametophyte self-incompatibility and sporophyte self-incompatibility. The sporophyte self-incompatibility reaction is controlled by the male and female substances encoded by the S locus gene, and the interaction of the S locus encoded protein of the same haplotype inhibits the growth of pollen (tube). Pollen-expressed small peptide ligand S-locus Cys-rich/S-locus protein 11 (SCR/SP11) and small peptide receptor kinase (SRK) on the stigma (Stein et al., 1991; Schopfer et al., 1999; Takayama et al., 2000) play an important role in the determination of sporophyte self-incompatibility in Brassica napus (Figure 2A). SCR/SP11 is a small CRP that is secreted into the pollen sac after translation, then transferred and adhered to the surface of the pollen, and interacted with SRK expressed in the stigma papillary cells after being pollinated (Table 1). After SCR/SP11 binds to SRK, the phosphorylation process of multiple factors recruits ubiquitin ligase to degrade the protein Exo70A1 involved in water absorption and hydration, and prevents the germination of pollen tubes (Samuel et al., 2009).
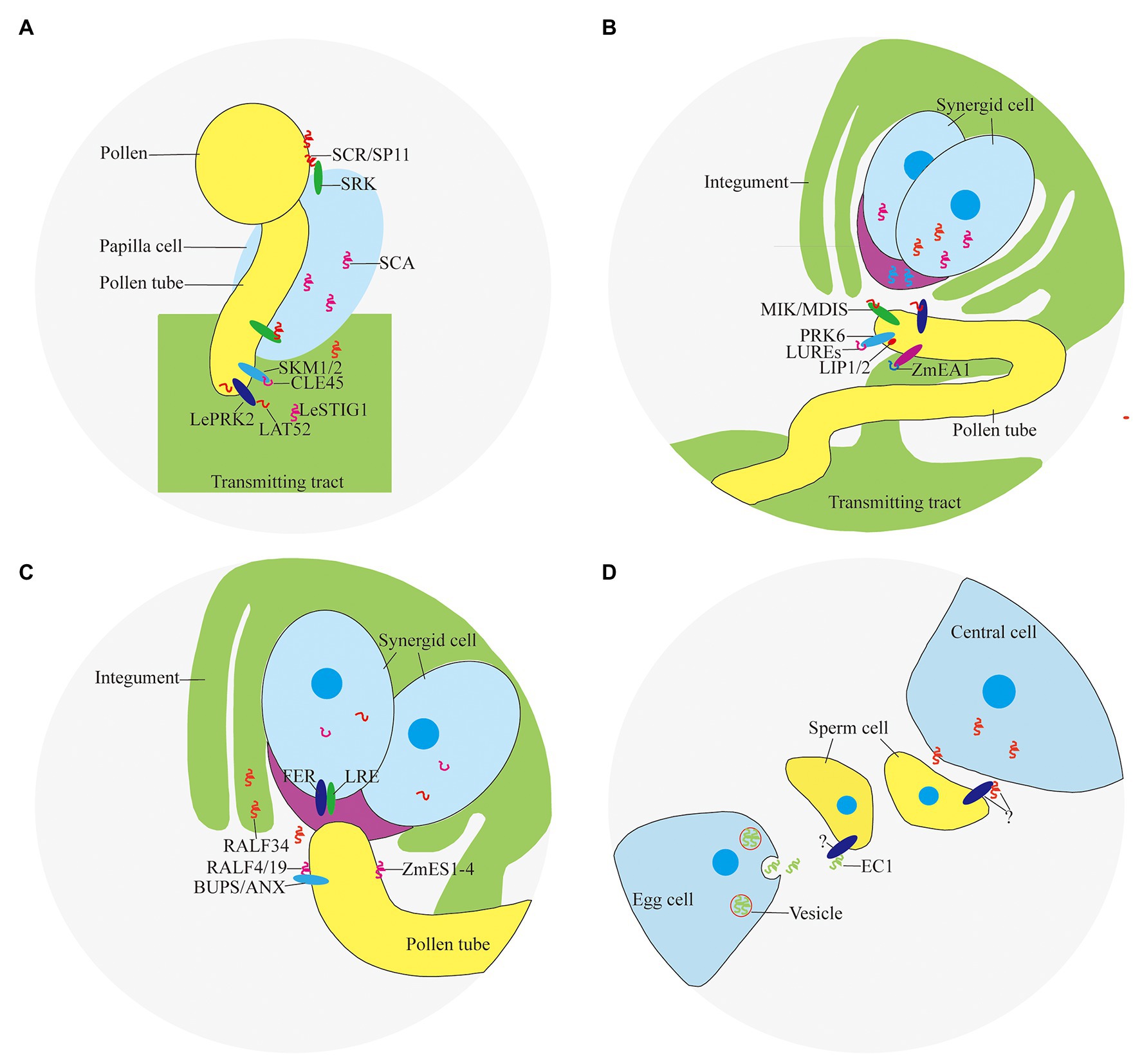
Figure 2. Peptides and receptors involved in double fertilization. (A) Pollen-stigma interaction; (B) ovule attraction; (C) pollen tube reception; and (D) gamete activation.
Pollen Germination
The nonmotile sperm cells must rely on the polar growth of the pollen tube to reach the embryo sac. Pollen tube germination and polar growth need the support and guidance of pistil tissue. The signal from the pollen or carpel is received by the receptor on the pollen tube and transmitted to the cell, changing the dynamic nature of the cytoskeleton and forming a pattern of polar growth (Guan et al., 2013).
It was found that PCP-Bα/β/γ/δ located in pollen coat are important for pollen germination because the pollen of pcp-bα/β/γ/δ displayed defects in pollen adhesion, pollen hydration, and pollen tube growth in vivo (Wang et al., 2017). In tomato, a small CRP LAT52 secreted by pollen is involved in pollen germination (Figure 2A). LAT52 can bind to the pollen tube receptor kinase LePRK2 specifically (Tang et al., 2002). This binding effect is strongest when the pollen tube germinates, and gradually weakens with the extension of the pollen tube. After the pollen tube germinates, substances from the carpel are needed to promote the growth of the pollen tube.
The small CRP LeSTIG1 expressed on stigma of tomato is involved in the regulation of pollen tube growth (Figure 2A). Application of LeSTIG1 can promote pollen tube elongation in vitro (Tang et al., 2004). After processing and maturation, LeSTIG1 is secreted out of the cell, combined with the receptor LePRK2, and enriched in the pollen tube. The intracellular domain of LePRK2 interacts with the plant-specific Rop GTPase guanylate exchange factor (GEF) family member KPP, and may regulate pollen tube growth through downstream ROP (Zhang et al., 2008). It has been discovered that the small peptide LeSTIG1 not only binds to receptors, but its C-terminal cysteine-rich domain can also bind to phospholipid molecules such as PI(3)P and participate in intracellular redox state regulation (Huang et al., 2014). Homologous genes of LeSTIG1 in other species may have other functions, such as regulating the secretion of petunia and tobacco stigma cells (Table 1; Verhoeven et al., 2005).
Pollen Tube Polar Growth
The polar growth of pollen tube is essential for the transportation of sperm cells to embryo sac to complete double fertilization. Small peptides can guide the pollen tube to grow in the transmitting tract of the style. Several CRPs expressed in pistil, such as stigma-style cysteine rich adhesin (SCA; Figure 2A), act as an adhesin binding the pollen tubes to the transmitting tract of the style (Park et al., 2000). The combination of SCA and pectic polysaccharide is necessary to induce pollen tube adhesion to other pollen tubes and to an in vitro style matrix (Lord, 2000). SCA is endocytosed into the pollen tube starting at the tip and subsequently moves through an endocytic route. This may be a process triggered by the ligand-receptor binding, but its receptor and the downstream events of the signal have not been clarified (Kim et al., 2006).
In addition, there are gradients formed by plantacyanin and other protein in pistil affect pollen tube elongation. Plantacyanins are secreted proteins with a size of about 10 kDa, with a distinctive gradient from the stigma to the ovule. The distribution may be regulated by the miRNA pathway (Maunoury and Vaucheret, 2011). The pollen tube will elongate at random in the papilla cells and the polar growth will be disrupted if overexpression of the plantacyanin gene to disrupt the gradient distribution pattern. Plantacyanin has properties of copper ion binding, which gives it a higher redox potential, and may participate in the metabolism of reactive oxygen species (ROS). Chemocyanin, the homologous protein of plantacyanin in Lily, is a similar chemotactic factor, which affects the polar growth of pollen tube (Kim et al., 2006).
Flowering plants in the breeding period are particularly susceptible to temperature. CLV3/ESR-related 45 (CLE45), a small modified peptide post-translationally, is involved in the process of maintaining seed yield under high temperature conditions. CLE45 is expressed in the stigma mainly at 22°C, but its expression expands to the transmitting tract upon temperature rise to 30°C. The synthetic CLE domain of CLE45 promotes pollen tube elongation in vitro at 30°C. In vivo, CLE45 cannot promote elongation, but can prolong the time of pollen tube growth. CLE45 binds the leucine-rich repeat receptor-like kinase STERILITY-REGULATING KINASE MEMBER1 (SKM1) and STERILITY-REGULATING KINASE MEMBER2 (SKM2; Figure 2A). The activity of pollen is maintained through the CLE45-SKM1/SKM2 signaling pathway under high temperature to ensure successful double fertilization (Table 1; Endo et al., 2013).
Attraction to Ovules
In recent years, it has been confirmed that pollen tubes are attracted by guidance signals from the embryo sac (Palanivelu and Preuss, 2000; Hepler et al., 2001). With the help of laser ablation, Higashiyama et al. observed that a single pollen tube penetrated a synergid cell and discharged its two gametes into the embryo sac as the synergid cell ruptured in Torenia fournieri. At the same time, it was found that the effective attracting distance of the synergid cells was 100–200 μm. It implies that the attracting substance has a short diffusible distance and may be secreted small peptides (Higashiyama et al., 2001). LURE1 and LURE2 expressed in the synergid cell abundantly and predominantly are secreted to the surface of the egg apparatus (Figure 2B). LUREs contain six conserved cysteines and are about 65 amino acids in length (~9 KDa). Injection of morpholino antisense oligomers against the LUREs impaired pollen tube attraction, demonstrating that LUREs are the attractants derived from the synergid cells of T. fournieri (Okuda et al., 2009).
Studies found that there are more than 300 defensin-like (DEFL) genes involving in cell-to-cell communication during male-female gametes interactions in Arabidopsis. AtLURE1 peptides, expressed in egg-accompanying synergid cells specifically, and secreted toward the funicular surface through the micropyle, are pollen tube attractants guiding pollen tubes to the ovular micropyle (Takeuchi and Higashiyama, 2012). In addition, there are still a certain percentage of pollen tubes can be fertilized normally in AtLURE1 RNAi transgenic plants, suggesting that there are other substances involved in pollen tube guidance. Lost In Pollen tube guidance 1 (LIP1) and 2 (LIP2) expressed in the membrane of pollen tube, interacted with PRK6 (Figure 2B), perceive the female signal AtLURE1 for micropylar pollen tube guidance (Liu et al., 2013). MALE DISCOVERER1-MDIS1 INTERACTING RECEPTOR LIKE KINASE1 (MDIS1-MIK; Figure 2B), a cell-surface receptor heteromer, was identified to perceive AtLURE1 in Arabidopsis (Wang et al., 2016).
In the monocotyledonous maize, Zea mays EGG APPARATUS1 (ZmEA1; Figure 2B) expressed in the egg cell and two synergids, is required for pollen tube attraction by the female gametophyte. Transgenic downregulation of the ZmEA1 gene led to ovule sterility caused by loss of close-range pollen tube guidance to the micropyle (Marton et al., 2005). ZmEA1 is recognized specifically by the pollen tube after being secreted by egg apparatus and degraded subsequently (Table 1; Marton et al., 2012).
Pollen Tube Reception
The synergid cells not only are required for pollen tube guidance, but also regulate the reception of the pollen tube. The pollen tube enters the female gametophyte by growing into one of the synergid cell which undergoes programmed cell death to burst and release sperm cells (Weterings and Russell, 2004).
The receptor-like serine-threonine kinase FERONIA/SIRENE (FER/SRN) is located on the cell membrane of the synergid cell (Figure 2C). In feronia (Huck et al., 2003) and sirene (Rotman et al., 2003), pollen tubes of wild-type can enter the embryo sac but fail to cease growth, rupture, and release their contents. Similar pollen tube overgrowths occur in interspecific crosses of Rhododendron and in the in vitro Torenia system (Higashiyama et al., 1998). It was found that ANXUR1 (ANX1) and ANXUR2 (ANX2; Figure 2C), the pollen-expressed homologs most closely related to FER, function redundantly to control the timing of pollen tube discharge. The pollen tubes of the double-mutant anx1 anx2 cease growth and burst in vitro and fail to reach the embryo sac in vivo (Boisson-Dernier et al., 2009; Miyazaki et al., 2009).
Rapid alkalinization factor (RALF), a secreted peptide, suppresses cell elongation of the primary root by activating the cell surface receptor FER in Arabidopsis (Haruta et al., 2014). It was found that RALF can induce the signal of Ca2+, suggesting an important role in the reception of pollen tubes (Pearce et al., 2001; Haruta and Constabel, 2003; Haruta et al., 2008). BUDDHA’S PAPER SEAL1 and 2 (BUPS1/2) and their peptide ligands RALF4/19 (Figure 2C), are pollen tube-expressed and are required to maintain pollen tube integrity since ralf4 ralf19 double mutants show pollen tube precocious rupture similar to anx1 anx2 mutants. Exogenous application of RALF34 peptide induced pollen tube burst (Ge et al., 2017).
Zea mays embryo sac (ZmES1-4; Figure 2C), four defensin-like peptides, expressed in synergid cells exclusively, is involved in pollen tube growth arrest, burst, and explosive sperm release. Application of ZmES1-4 results in pollen tube plasma membrane depolarization and sperm cells discharge in maize. The pollen tube-expressed K+ channel KZM1 as a target of ZmES1-4, which opens after ZmES1-4 treatment and probably leads to K+ influx and sperm release after osmotic burst (Table 1; Amien et al., 2010).
Gamete Activation
Two sperm cells are released after the pollen tube ruptures. One sperm cell fuses with the egg cell, the other sperm cell fuses with the central cell. This process involved in a large amount of signal transduction, is the last step in establishing species isolation, including cell migration, gamete recognition, cytoplasmic fusion, and nuclear fusion. EGG CELL 1 (EC1; Figure 2D), a small CRP, accumulated in storage vesicles of the egg cell, plays an important role in gamete activation (Table 1). Upon sperm arrival, EC1-containing vesicles are exocytosed. The sperm endomembrane system responds to exogenously applied EC1 peptides by redistributing the potential gamete fusogen HAPLESS 2/GENERATIVE CELL SPECIFIC 1 (HAP2/GCS1) to the cell surface (Sprunck et al., 2012). HAP2/GCS1 located in the inner membrane system of sperm cells is a critical fertilization factor involving in gamete fusion in Arabidopsis. Sperm cells of hap2/gcs1 mutants can be released normally, but cannot fuse with the female gametes, resulting in sterility (Mori et al., 2006; von Besser et al., 2006).
Is there the same mechanism in the central cell? The Arabidopsis female gametophytic mutant glauce (glc) exhibit one sperm cell fuses with the egg cell successfully but the second sperm cell fails to fuse with the central cell, resulting in single fertilization (Leshem et al., 2012). The BAHD transferase involved in secondary metabolism can rescue the fertilization defect of glc mutant, implying there may be signals that function similar to EC1 in central cell.
Conclusion and Perspectives
During the past decade, it has been established that small peptides play an essential role in many developmental processes in plants, such as cell proliferation, maintenance of stem cells, nodule formation, and male-female interaction during plant reproduction (Katsir et al., 2011). Here, we provide a comprehensive overview of the small peptides regulating the processes of male-female crosstalk, including self-incompatibility, pollen tube germination, polar growth and reception, attraction to ovules, and gamete activation (Table 1). Precise interaction between small peptides and the corresponding receptors is essential for reproduction, such as SCR/SP11-SRK regulate self-incompatibility in B. napus, LAT52-LePRK2 regulate pollen germination in tomato, CLE45-SKM1/2 regulate pollen tube polar growth, and LUREs-MDIS1/MIK/LIP regulate attraction to ovules.
At present, there are many similar family members of small peptides involved in the process of male-female crosstalk. All or some of them have the same expression pattern, and each of them can bind to the corresponding receptor and function redundantly. However, it is difficult to identify small peptides through traditional genetic and biochemical methods due to the small molecular weight, low content, and high redundancy. More small peptides will be identified with the development of high-throughput sequencing technologies, such as genomics, transcriptomics, and peptidomics.
Small peptides are usually used as ligands and perceived by receptors in male-female communication. The binding of receptor and ligand may cause autophosphorylation of receptor and intracellular phosphorylation cascades. However, many receptors have not been identified yet. It is difficult to identify the corresponding receptors because the ligand is small and the interaction with the receptor is transient. With the great progress of gene editing technology such as CRISPR in recent years, it can be predicted that the research of small peptide will be developed rapidly. In addition, researchers are also interested in how to transfer the signal to the cell after the small peptide and receptor binds.
Fertilization is the premise of seed production. Improving the success rate of fertilization may help to improve crop yield. Understanding the mechanism of male-female crosstalk in the process of reproduction can help solve the problem of self-incompatibility and cross-incompatibility and provide strong support for breeding. The concentration of small peptides to exert their physiological functions is very low. Unlike traditional plant hormones, small peptides are composed of amino acids essentially, and exogenous application will not pose a risk to the environment. The application of small peptides in agricultural production can reduce the amount of pesticides and fertilizers to protect the ecological environment, serving modern green agriculture.
Author Contributions
JZ and LY wrote the manuscript. XW, HL, and WW provided ideas and revised the manuscript. All authors contributed to the article and approved the submitted version.
Funding
This work was supported by grants from the National Natural Science Foundation of China (grant no. 32000244), the Research Start Up Fund of Henan Agricultural University (grant no. 30500484), the Natural Science Foundation of Guangdong (grant no. 2018A030313872), and the Key Project and Special Foundation of Research, Development and Promotion in Henan Province, China (grant no. 192102110014).
Conflict of Interest
The authors declare that the research was conducted in the absence of any commercial or financial relationships that could be construed as a potential conflict of interest.
Acknowledgments
We thank Yanpei Liu for modifying the grammar.
References
Amien, S., Kliwer, I., Marton, M. L., Debener, T., Geiger, D., Becker, D., et al. (2010). Defensin-like ZmES4 mediates pollen tube burst in maize via opening of the potassium channel KZM1. PLoS Biol. 8:e1000388. doi: 10.1371/journal.pbio.1000388
Boisson-Dernier, A., Roy, S., Kritsas, K., Grobei, M. A., Jaciubek, M., Schroeder, J. I., et al. (2009). Disruption of the pollen-expressed FERONIA homologs ANXUR1 and ANXUR2 triggers pollen tube discharge. Development 136, 3279–3288. doi: 10.1242/dev.040071
Chae, K., Gonong, B. J., Kim, S. C., Kieslich, C. A., Morikis, D., Balasubramanian, S., et al. (2010). A multifaceted study of stigma/style cysteine-rich adhesin (SCA)-like Arabidopsis lipid transfer proteins (LTPs) suggests diversified roles for these LTPs in plant growth and reproduction. J. Exp. Bot. 61, 4277–4290. doi: 10.1093/jxb/erq228
Delay, C., Imin, N., and Djordjevic, M. A. (2013a). CEP genes regulate root and shoot development in response to environmental cues and are specific to seed plants. J. Exp. Bot. 64, 5383–5394. doi: 10.1093/jxb/ert332
Delay, C., Imin, N., and Djordjevic, M. A. (2013b). Regulation of Arabidopsis root development by small signaling peptides. Front. Plant Sci. 4:352. doi: 10.3389/fpls.2013.00352
Djordjevic, M. A., Mohd-Radzman, N. A., and Imin, N. (2015). Small-peptide signals that control root nodule number, development, and symbiosis. J. Exp. Bot. 66, 5171–5181. doi: 10.1093/jxb/erv357
Endo, S., Shinohara, H., Matsubayashi, Y., and Fukuda, H. (2013). A novel pollen-pistil interaction conferring high-temperature tolerance during reproduction via CLE45 signaling. Curr. Biol. 23, 1670–1676. doi: 10.1016/j.cub.2013.06.060
Ge, Z., Bergonci, T., Zhao, Y., Zou, Y., Du, S., Liu, M. C., et al. (2017). Arabidopsis pollen tube integrity and sperm release are regulated by RALF-mediated signaling. Science 358, 1596–1600. doi: 10.1126/science.aao3642
Guan, Y., Guo, J., Li, H., and Yang, Z. (2013). Signaling in pollen tube growth: crosstalk, feedback, and missing links. Mol. Plant 6, 1053–1064. doi: 10.1093/mp/sst070
Hamamura, Y., Nagahara, S., and Higashiyama, T. (2012). Double fertilization on the move. Curr. Opin. Plant Biol. 15, 70–77. doi: 10.1016/j.pbi.2011.11.001
Haruta, M., and Constabel, C. P. (2003). Rapid alkalinization factors in poplar cell cultures. Peptide isolation, cDNA cloning, and differential expression in leaves and methyl jasmonate-treated cells. Plant Physiol. 131, 814–823. doi: 10.1104/pp.014597
Haruta, M., Monshausen, G., Gilroy, S., and Sussman, M. R. (2008). A cytoplasmic Ca2+ functional assay for identifying and purifying endogenous cell signaling peptides in Arabidopsis seedlings: identification of AtRALF1 peptide. Biochemistry 47, 6311–6321. doi: 10.1021/bi8001488
Haruta, M., Sabat, G., Stecker, K., Minkoff, B. B., and Sussman, M. R. (2014). A peptide hormone and its receptor protein kinase regulate plant cell expansion. Science 343, 408–411. doi: 10.1126/science.1244454
Hepler, P. K., Vidali, L., and Cheung, A. Y. (2001). Polarized cell growth in higher plants. Annu. Rev. Cell Dev. Biol. 17, 159–187. doi: 10.1146/annurev.cellbio.17.1.159
Higashiyama, T., Kuroiwa, H., Kawano, S., and Kuroiwa, T. (1998). Guidance in vitro of the pollen tube to the naked embryo sac of Torenia fournieri. Plant Cell 10, 2019–2032. doi: 10.1105/tpc.10.12.2019
Higashiyama, T., and Takeuchi, H. (2015). The mechanism and key molecules involved in pollen tube guidance. Annu. Rev. Plant Biol. 66, 393–413. doi: 10.1146/annurev-arplant-043014-115635
Higashiyama, T., Yabe, S., Sasaki, N., Nishimura, Y., Miyagishima, S., Kuroiwa, H., et al. (2001). Pollen tube attraction by the synergid cell. Science 293, 1480–1483. doi: 10.1126/science.1062429
Hsu, P. Y., and Benfey, P. N. (2018). Small but mighty: functional peptides encoded by small ORFs in plants. Proteomics 18:e1700038. doi: 10.1002/pmic.201700038
Huang, W. J., Liu, H. K., McCormick, S., and Tang, W. H. (2014). Tomato pistil factor STIG1 promotes in vivo pollen tube growth by binding to phosphatidylinositol 3-phosphate and the extracellular domain of the pollen receptor kinase LePRK2. Plant Cell 26, 2505–2523. doi: 10.1105/tpc.114.123281
Huck, N., Moore, J. M., Federer, M., and Grossniklaus, U. (2003). The Arabidopsis mutant feronia disrupts the female gametophytic control of pollen tube reception. Development 130, 2149–2159. doi: 10.1242/dev.00458
Imin, N., Mohd-Radzman, N. A., Ogilvie, H. A., and Djordjevic, M. A. (2013). The peptide-encoding CEP1 gene modulates lateral root and nodule numbers in Medicago truncatula. J. Exp. Bot. 64, 5395–5409. doi: 10.1093/jxb/ert369
Katsir, L., Davies, K. A., Bergmann, D. C., and Laux, T. (2011). Peptide signaling in plant development. Curr. Biol. 21, R356–R364. doi: 10.1016/j.cub.2011.03.012
Kim, M. J., Jeon, B. W., Oh, E., Seo, P. J., and Kim, J. (2021). Peptide signaling during plant reproduction. Trends Plant Sci. doi: 10.1016/j.tplants.2021.02.008 [Ebup ahead of print]
Kim, S. T., Zhang, K., Dong, J., and Lord, E. M. (2006). Exogenous free ubiquitin enhances lily pollen tube adhesion to an in vitro stylar matrix and may facilitate endocytosis of SCA. Plant Physiol. 142, 1397–1411. doi: 10.1104/pp.106.086801
Leshem, Y., Johnson, C., Wuest, S. E., Song, X., Ngo, Q. A., Grossniklaus, U., et al. (2012). Molecular characterization of the glauce mutant: a central cell-specific function is required for double fertilization in Arabidopsis. Plant Cell 24, 3264–3277. doi: 10.1105/tpc.112.096420
Liu, J., Zhong, S., Guo, X., Hao, L., Wei, X., Huang, Q., et al. (2013). Membrane-bound RLCKs LIP1 and LIP2 are essential male factors controlling male-female attraction in Arabidopsis. Curr. Biol. 23, 993–998. doi: 10.1016/j.cub.2013.04.043
Lord, E. (2000). Adhesion and cell movement during pollination: cherchez la femme. Trends Plant Sci. 5, 368–373. doi: 10.1016/S1360-1385(00)01744-1
Marton, M. L., Cordts, S., Broadhvest, J., and Dresselhaus, T. (2005). Micropylar pollen tube guidance by egg apparatus 1 of maize. Science 307, 573–576. doi: 10.1126/science.1104954
Marton, M. L., Fastner, A., Uebler, S., and Dresselhaus, T. (2012). Overcoming hybridization barriers by the secretion of the maize pollen tube attractant ZmEA1 from Arabidopsis ovules. Curr. Biol. 22, 1194–1198. doi: 10.1016/j.cub.2012.04.061
Matsubayashi, Y. (2003). Ligand-receptor pairs in plant peptide signaling. J. Cell Sci. 116, 3863–3870. doi: 10.1242/jcs.00733
Maunoury, N., and Vaucheret, H. (2011). AGO1 and AGO2 act redundantly in miR408-mediated Plantacyanin regulation. PLoS One 6:e28729. doi: 10.1371/journal.pone.0028729
Miyazaki, S., Murata, T., Sakurai-Ozato, N., Kubo, M., Demura, T., Fukuda, H., et al. (2009). ANXUR1 and 2, sister genes to FERONIA/SIRENE, are male factors for coordinated fertilization. Curr. Biol. 19, 1327–1331. doi: 10.1016/j.cub.2009.06.064
Mohd-Radzman, N. A., Binos, S., Truong, T. T., Imin, N., Mariani, M., and Djordjevic, M. A. (2015). Novel MtCEP1 peptides produced in vivo differentially regulate root development in Medicago truncatula. J. Exp. Bot. 66, 5289–5300. doi: 10.1093/jxb/erv008
Mori, T., Kuroiwa, H., Higashiyama, T., and Kuroiwa, T. (2006). GENERATIVE CELL SPECIFIC 1 is essential for angiosperm fertilization. Nat. Cell Biol. 8, 64–71. doi: 10.1038/ncb1345
Okuda, S., Tsutsui, H., Shiina, K., Sprunck, S., Takeuchi, H., Yui, R., et al. (2009). Defensin-like polypeptide LUREs are pollen tube attractants secreted from synergid cells. Nature 458, 357–361. doi: 10.1038/nature07882
Palanivelu, R., and Preuss, D. (2000). Pollen tube targeting and axon guidance: parallels in tip growth mechanisms. Trends Cell Biol. 10, 517–524. doi: 10.1016/S0962-8924(00)01849-3
Park, S. Y., Jauh, G. Y., Mollet, J. C., Eckard, K. J., Nothnagel, E. A., Walling, L. L., et al. (2000). A lipid transfer-like protein is necessary for lily pollen tube adhesion to an in vitro stylar matrix. Plant Cell 12, 151–164. doi: 10.1105/tpc.12.1.151
Patel, N., Mohd-Radzman, N. A., Corcilius, L., Crossett, B., Connolly, A., Cordwell, S. J., et al. (2018). Diverse peptide hormones affecting root growth identified in the Medicago truncatula secreted Peptidome. Mol. Cell. Proteomics 17, 160–174. doi: 10.1074/mcp.RA117.000168
Pearce, G., Moura, D. S., Stratmann, J., and Ryan, C. A. Jr. (2001). RALF, a 5-kDa ubiquitous polypeptide in plants, arrests root growth and development. Proc. Natl. Acad. Sci. U. S. A. 98, 12843–12847. doi: 10.1073/pnas.201416998
Qu, X., Cao, B., Kang, J., Wang, X., Han, X., Jiang, W., et al. (2019). Fine-tuning stomatal movement through small Signaling peptides. Front. Plant Sci. 10:69. doi: 10.3389/fpls.2019.00069
Rotman, N., Rozier, F., Boavida, L., Dumas, C., Berger, F., and Faure, J. E. (2003). Female control of male gamete delivery during fertilization in Arabidopsis thaliana. Curr. Biol. 13, 432–436. doi: 10.1016/S0960-9822(03)00093-9
Samuel, M. A., Chong, Y. T., Haasen, K. E., Aldea-Brydges, M. G., Stone, S. L., and Goring, D. R. (2009). Cellular pathways regulating responses to compatible and self-incompatible pollen in Brassica and Arabidopsis stigmas intersect at Exo70A1, a putative component of the exocyst complex. Plant Cell 21, 2655–2671. doi: 10.1105/tpc.109.069740
Schopfer, C. R., Nasrallah, M. E., and Nasrallah, J. B. (1999). The male determinant of self-incompatibility in Brassica. Science 286, 1697–1700. doi: 10.1126/science.286.5445.1697
Sprunck, S., Rademacher, S., Vogler, F., Gheyselinck, J., Grossniklaus, U., and Dresselhaus, T. (2012). Egg cell-secreted EC1 triggers sperm cell activation during double fertilization. Science 338, 1093–1097. doi: 10.1126/science.1223944
Stein, J. C., Howlett, B., Boyes, D. C., Nasrallah, M. E., and Nasrallah, J. B. (1991). Molecular cloning of a putative receptor protein kinase gene encoded at the self-incompatibility locus of Brassica oleracea. Proc. Natl. Acad. Sci. U. S. A. 88, 8816–8820. doi: 10.1073/pnas.88.19.8816
Stotz, H. U., Spence, B., and Wang, Y. (2009a). A defensin from tomato with dual function in defense and development. Plant Mol. Biol. 71, 131–143. doi: 10.1007/s11103-009-9512-z
Stotz, H. U., Thomson, J. G., and Wang, Y. (2009b). Plant defensins: defense, development and application. Plant Signal. Behav. 4, 1010–1012. doi: 10.4161/psb.4.11.9755
Takahashi, F., Suzuki, T., Osakabe, Y., Betsuyaku, S., Kondo, Y., Dohmae, N., et al. (2018). A small peptide modulates stomatal control via abscisic acid in long-distance signalling. Nature 556, 235–238. doi: 10.1038/s41586-018-0009-2
Takayama, S., and Isogai, A. (2005). Self-incompatibility in plants. Annu. Rev. Plant Biol. 56, 467–489. doi: 10.1146/annurev.arplant.56.032604.144249
Takayama, S., Shiba, H., Iwano, M., Shimosato, H., Che, F. S., Kai, N., et al. (2000). The pollen determinant of self-incompatibility in Brassica campestris. Proc. Natl. Acad. Sci. U. S. A. 97, 1920–1925. doi: 10.1073/pnas.040556397
Takeuchi, H., and Higashiyama, T. (2012). A species-specific cluster of defensin-like genes encodes diffusible pollen tube attractants in Arabidopsis. PLoS Biol. 10:e1001449. doi: 10.1371/journal.pbio.1001449
Takeuchi, H., and Higashiyama, T. (2016). Tip-localized receptors control pollen tube growth and LURE sensing in Arabidopsis. Nature 531, 245–248. doi: 10.1038/nature17413
Taleski, M., Imin, N., and Djordjevic, M. A. (2016). New role for a CEP peptide and its receptor: complex control of lateral roots. J. Exp. Bot. 67, 4797–4799. doi: 10.1093/jxb/erw306
Taleski, M., Imin, N., and Djordjevic, M. A. (2018). CEP peptide hormones: key players in orchestrating nitrogen-demand signalling, root nodulation, and lateral root development. J. Exp. Bot. 69, 1829–1836. doi: 10.1093/jxb/ery037
Tang, W., Ezcurra, I., Muschietti, J., and McCormick, S. (2002). A cysteine-rich extracellular protein, LAT52, interacts with the extracellular domain of the pollen receptor kinase LePRK2. Plant Cell 14, 2277–2287. doi: 10.1105/tpc.003103
Tang, W., Kelley, D., Ezcurra, I., Cotter, R., and McCormick, S. (2004). LeSTIG1, an extracellular binding partner for the pollen receptor kinases LePRK1 and LePRK2, promotes pollen tube growth in vitro. Plant J. 39, 343–353. doi: 10.1111/j.1365-313X.2004.02139.x
Verhoeven, T., Feron, R., Wolters-Arts, M., Edqvist, J., Gerats, T., Derksen, J., et al. (2005). STIG1 controls exudate secretion in the pistil of petunia and tobacco. Plant Physiol. 138, 153–160. doi: 10.1104/pp.104.054809
von Besser, K., Frank, A. C., Johnson, M. A., and Preuss, D. (2006). Arabidopsis HAP2 (GCS1) is a sperm-specific gene required for pollen tube guidance and fertilization. Development 133, 4761–4769. doi: 10.1242/dev.02683
Wang, L., Clarke, L. A., Eason, R. J., Parker, C. C., Qi, B., Scott, R. J., et al. (2017). PCP-B class pollen coat proteins are key regulators of the hydration checkpoint in Arabidopsis thaliana pollen-stigma interactions. New Phytol. 213, 764–777. doi: 10.1111/nph.14162
Wang, T., Liang, L., Xue, Y., Jia, P. F., Chen, W., Zhang, M. X., et al. (2016). A receptor heteromer mediates the male perception of female attractants in plants. Nature 531, 241–244. doi: 10.1038/nature16975
Weterings, K., and Russell, S. D. (2004). Experimental analysis of the fertilization process. Plant Cell 16(Suppl), S107–S118. doi: 10.1105/tpc.016873
Zhang, D., Wengier, D., Shuai, B., Gui, C. P., Muschietti, J., McCormick, S., et al. (2008). The pollen receptor kinase LePRK2 mediates growth-promoting signals and positively regulates pollen germination and tube growth. Plant Physiol. 148, 1368–1379. doi: 10.1104/pp.108.124420
Keywords: small peptides, self-incompatibility, pollen germination, polar growth, male-female crosstalk
Citation: Zhang J, Yue L, Wu X, Liu H and Wang W (2021) Function of Small Peptides During Male-Female Crosstalk in Plants. Front. Plant Sci. 12:671196. doi: 10.3389/fpls.2021.671196
Edited by:
Ning Li, Hong Kong University of Science and Technology, Hong KongReviewed by:
Rui Malhó, University of Lisbon, PortugalToshiyuki Mori, Osaka University, Japan
Pingfang Yang, Hubei University, China
Copyright © 2021 Zhang, Yue, Wu, Liu and Wang. This is an open-access article distributed under the terms of the Creative Commons Attribution License (CC BY). The use, distribution or reproduction in other forums is permitted, provided the original author(s) and the copyright owner(s) are credited and that the original publication in this journal is cited, in accordance with accepted academic practice. No use, distribution or reproduction is permitted which does not comply with these terms.
*Correspondence: Wei Wang, wangwei@henau.edu.cn; Hui Liu, liuhuisw@henau.edu.cn