- 1College of Horticulture, Shanxi Agricultural University, Jinzhong, China
- 2Collaborative Innovation Center for Improving Quality and Increase of Protected Vegetables in Shanxi Province, Jinzhong, China
- 3Datong Daylily Industial Development Research Institute, Datong, China
The Hemerocallis accessions is widely consumed as nutritious vegetable and traditional medicine in eastern Asia and used as an ornamental flower worldwide. Compared with most other horticultural products, its flower is richer in polyphenols, flavonoids, carotenoids, and anthocyanins. Therefore, the flower has strong antioxidant activity that inhibits cancer cell proliferation, which could used for health and pharmaceutical purposes. The flavonoids composition and distribution in the flowers, and the content varied between different accssions is still unclear. In this context, eight flavonols, two flavones, and two anthocyanins were determined in Hemerocallis flower by high-performance liquid chromatography (HPLC) coupled with photodiode array and mass spectrometric detectors. Rutin was the most abundant flavonols and cyanidin 3,5-glucoside and cyanidin 3-rutinoside were the major anthocyanins in Hemerocallis tepals, resulting in flower petal coloration, and their content in the petal was higher than that of the sepal. Hierarchical cluster analysis grouped the 42 accessions into four groups, and they were significantly different (p < 0.05) from each other in the ten significant compounds by One-way ANOVA. Overall, the qualitative and quantitative analysis of flavonoid constituents in six floral parts of 42 Hemerocallis accessions were elucidated, which could be helpful for the food and pharmaceutical industries, and lay the foundation for the Hemerocallis flower color research.
Highlights
- First report on systematic identification and quantification of flavonoid in Hemerocallis floral organs.
- Eight flavonols, two flavones, and two anthocyanins in Hemerocallis flower, and Rutin was the dominant flavonol.
- Our data are helpful for the Hemerocallis flower used for the food and pharmaceutical industries.
Introduction
Hemerocallis spp. are ornamental herbaceous perennials with more than 83,000 modern cultivars in the world (Wang and Gao, 2014). These Hemerocallis accessions have a cultivation history of more than 2,000 years in China, where is the distribution center of Hemerocallis in the world. Hemerocallis citrina is a traditional vegetable. According to our preliminary statistics, its cultivated area in China exceeds 73,000 hectares, with an annual production of 80,000 tons. Therefore, there is considerably economic value in H. citrina. Besides, Hemerocallis is mainly used for landscape beautification and is also an important cut flower material. These plants grow well in different soil types and can bloom normally under either full sun or light shade. Hence, from the cold temperate zone to the tropics can see a large number of applications of Hemerocallis.
Hemerocallis have been widely consumed as nutritious food and traditional medicine in eastern Asia (Rodriguez-Enriquez and Grant-Downton, 2013). The edible part of Hemerocallis is the flower bud growing on top of the floral axis and typically has more than 20 flowers per scape. Compared with most other vegetables, its flower is richer in polyphenols (Lin et al., 2011), flavonoids (Fu and Mao, 2006; Lin et al., 2011), carotenoids (Tai and Chen, 2000; Hsu et al., 2011), and anthocyanins (Tai and Chen, 2000; Deng et al., 2003; Fernandes et al., 2017). The flowers also have strong antioxidant activity that inhibits cancer cell proliferation (Cichewicz et al., 2004; Kao et al., 2015), hence it is used for health and pharmaceutical purposes. This perennial herb is projected to have substantial market prospects in the future (Wiseman et al., 1996; Zloch, 1996; Cos et al., 2004; Manach et al., 2004).
The variation of Hemerocallis flower color is abundant (Li et al., 2016), and the color of petals and sepals may also be different. Therefore, Hemerocallis is an ideal material in flower color research. The flower color is the result of metabolite accumulation in the vacuoles of flower epidermal cells (Wang et al., 2018). Flavonoid metabolism pathways play important roles in modulating plant color. The differences in the presence, quantity or type of flavonoid pigments is one of the main reasons for the yellow flower color (Deng et al., 2013). However, the differential accumulation of flavonols, flavones, and anthocyanins in different Hemerocallis germplasm remain unclear. In addition, there are few reports on the qualitative and quantitative analysis of the secondary metabolites especially flavonoids in Hemerocallis flower parts, which greatly slows down the process of its flower color breeding and restricts its economic and industrial development.
In this study, we collected 42 Hemerocallis accessions with different colors and origins. Our aim is to (1) assess the flavonoid composition and content in different floral parts of Hemerocallis and (2) evaluate variations in the flavonoid contents of the different accessions. These research results provide a theoretical basis for analyzing the accumulation of Hemerocallis flavonoids and a scientific reference for exploring its edible value by food and pharmaceutical industries.
Materials and Methods
Plant Materials
A total of 42 accessions were used in this study. These accessions were from different geographic regions around the world including commercial accessions and landraces. The details of these 42 accessions were presented in Supplementary Table 1 and Table 1. The sepals and petals were collected from each accession and divided into six parts (Figure 1), sepal throat (ST), sepal eye (SE), sepal limb (SL), petal throat (PT), petal eye (PE), and petal limb (PL), according to a previously described method (Cui et al., 2019). Subsequently, each part was placed in liquid nitrogen immediately after detaching, then preserved at −80°C until the flavonoids extraction.
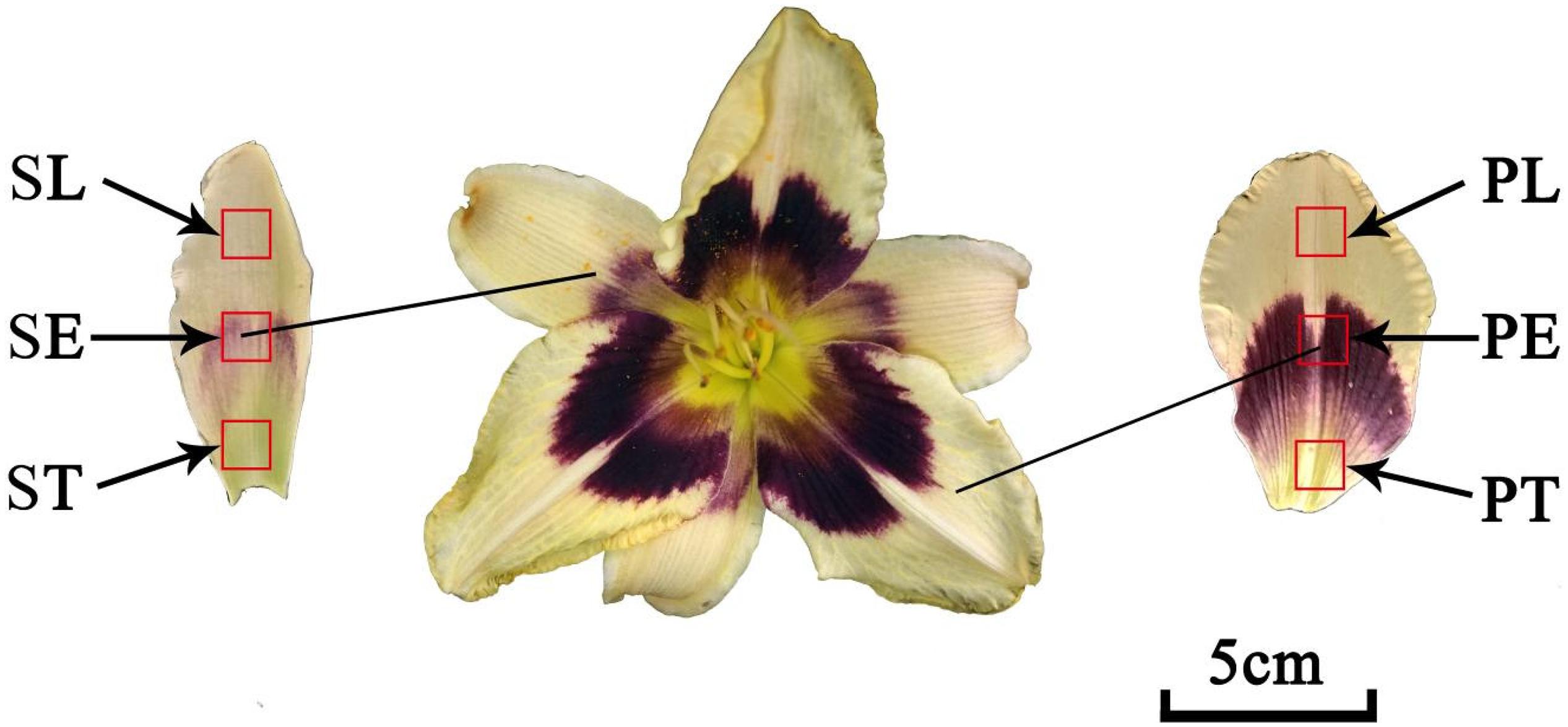
Figure 1. The flower organs of daylily. ST, sepal throat; SE, sepal eye; SL, sepal limb; PT, petal throat; PE, petal eye; PL, petal limb.
Reagents and Chemicals
High-performance liquid chromatography grade methanol, formic acid, trifluoroacetic acid (TFA), and acetonitrile were purchased from Fisher Scientific (Fair Lawn, NJ). Quercetin 3-glucoside (Qu3g), myricetin, kaempferol 3-glucorhamnoside, apigenin, and two cyanidin derivatives standards, cyanidin 3-glucoside (Cy3g), and cyanidin 3-rutinoside (Cy3r) were purchased from Sigma-Aldrich (St. Louis, MO). Kaempferol (Km), quercetin (Qu), and rutin (Rt) were purchased from Solarbio (Solarbio, China). Ultrapure water from PureLab Ultra Water System (ELGA LabWater, United Kingdom) was used in this experiment. All other reagents used were of analytical grade.
Extraction and HPLC Analysis of Flavonoids and Anthocyanins
Samples (0.1 g fresh weight, FW) were fully ground in liquid nitrogen, extracted in 2 mL solvent mixture (methanol/water/formic acid/TFA, 70:27:2:1, v/v/v/v), and allowed to settle for 24 h without light. The extracts were then centrifuged (12,000 rpm, 20 min), and the supernatant was filtered (0.22 μm) into vials. The HPLC analyses were carried out on a Thermo Fisher HPLC system connected with a 996 photodiode array detector (UltiMate 3000, ThermoFisher, United States), which was set in the range of 190–600 nm. Data collection and processing was accomplished by the Chameleon software version 2.0. The chromatographic separation was performed on a Venusil ASB C18 column (Agela Technologies, China) with 4.6 mm × 250 mm, 5 μm. The mobile phases comprised 2% aqueous formic acid (A) and acetonitrile (B). The gradients were programed as follows: 0 min, 8% B; 3 min, 8% B; 23 min, 20% B; 33 min, 40% B; 43 min, 40% B; 45 min, 8% B. The column temperature, injection volume, and flow rate were set at 35°C, 10 μL, and 0.8 mL/min, respectively. The flavonoids and anthocyanin chromatograms were extracted at 350 nm and 520 nm. All samples were extracted in triplicate.
LC–MS Analysis of Flavonoids and Anthocyanins
Liquid chromatography–mass spectrometry (LC–MS) analysis was performed on the HPLC instrument described above, interfaced with a microOTOF Q quadrupole time-of-flight mass spectrometer (Thermo Fisher, United States) connected to either electrospray ionization (ESI) or an atmospheric pressure chemical ionization source. The HPLC analysis conditions were similar to those described above. The mass signal range was m/z 50–1,100. The ionization of flavonoids was achieved with an ESI source in both positive and negative modes, and the parameters were set as follows: capillary voltage, 3,500 V; endplate offset, 500 V; drying gas (nitrogen) flow, 8.0 L/min; drying gas temperature, 180°(C; collision rf, 200 Vpp; nebulizer pressure, 0.8 bar; prepulse storage, 8.0 (s; transfer time, 80.0 μs; and collision energy, 10.0 eV.
Quantitation of Flavonoids and Anthocyanins
The quantitation was conducted by external calibration of the corresponding standards from the areas of the chromatographic peaks at 350 nm for flavonoids and 520 nm for anthocyanins. All standards were dissolved in methanol. The following equations were used: cyanidin-3-glucoside (y = 274.1046 x + 0.1328, R2 = 0.99987); quercetin (y = 392.9441 x + 0.5815, R2 = 0.99865); quercetin 3-glucoside (y = 339.3973 x − 0.3364, R2 = 0.99997); rutin (y = 214.2924 x − 0.1913, R2 = 0.98327); apigenin (y = 516.2105 x + 0.0001, R2 = 0.99666); myricitrin (y = 1040.74 x − 2.1097, R2 = 0.99798). The content of compounds that did not have corresponding standards was calculated from the most suitable standard calibration curve.
Statistical Analysis
The mean value of each sample was obtained from three replications and used for further analysis. The statistical analysis was conducted by the R (x64 3.5.1) software. The variations in the contents of ten major flavonoids among different floral parts were determined by the Mann–Whitney U test at p < 0.001. The hierarchical cluster analysis was conducted by the Ward D method, and the flavonoids difference between clusters were determined by one-way ANOVA at p < 0.05.
Results and Discussion
Identification of Flavonoids
The flavonoids were identified according to HPLC retention times, UV λmax spectrum, and MS data (in both NI and PI modes), as well as previous reports (Markham, 1989). In total, 15 flavonoids were detected in the 42 accessions. The HPLC-DAD and HPLC-ESI(±)-MS2 analyses results, such as molecular ion, aglycone ion, and main fragments of MS2, are summarized in Table 2. In this study, eight flavonols, two flavones, and two anthocyanins were identified from the flowers of Hemerocallis, and three compositions were unknown. The PE of Hemerocallis ‘Little Bumble Bee’ was of these 15 compositions (Figures 2, 3).
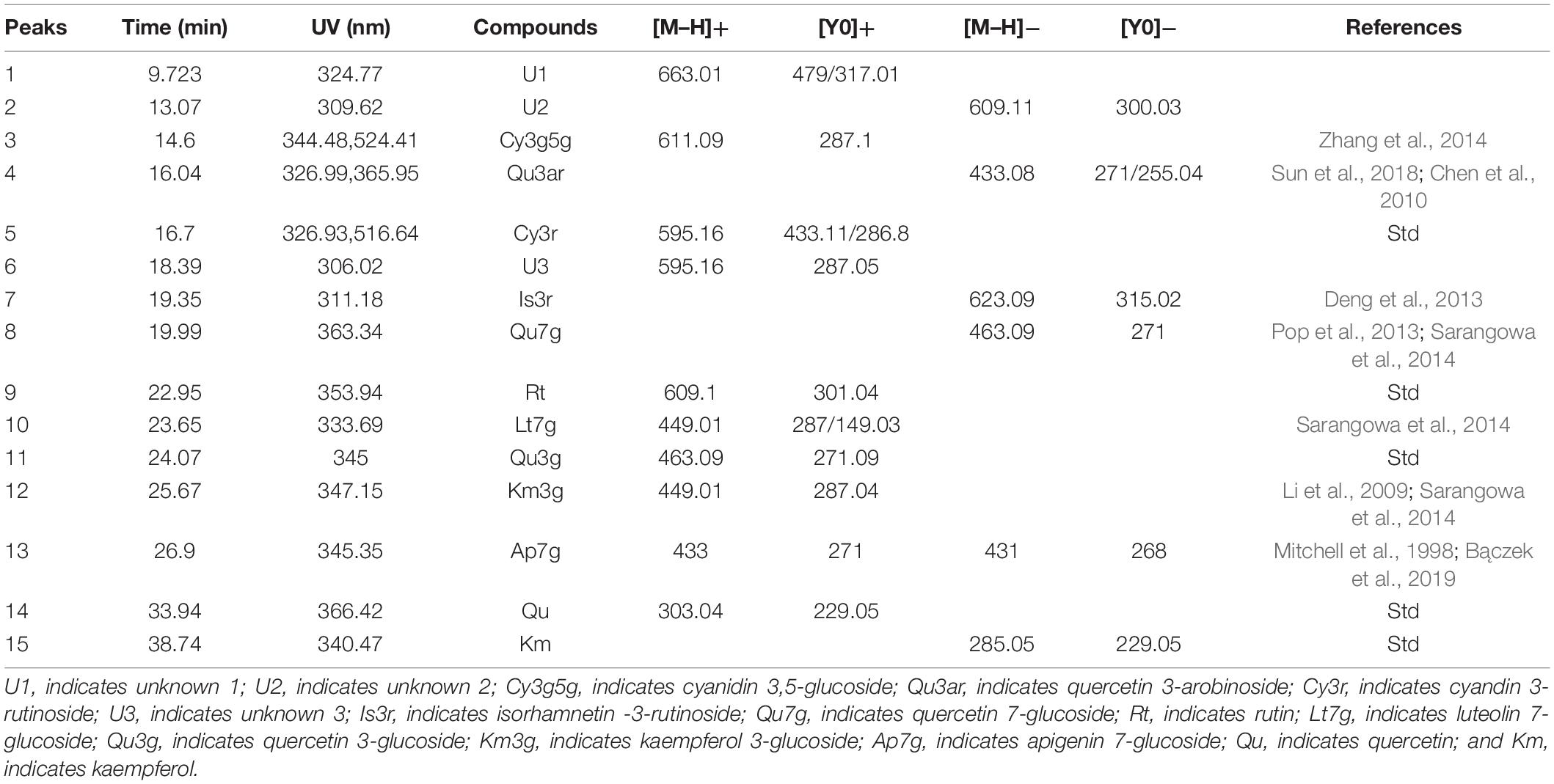
Table 2. Chromatographic, spectroscopic, and mass spectrometric features of flavonoids detected in this study.
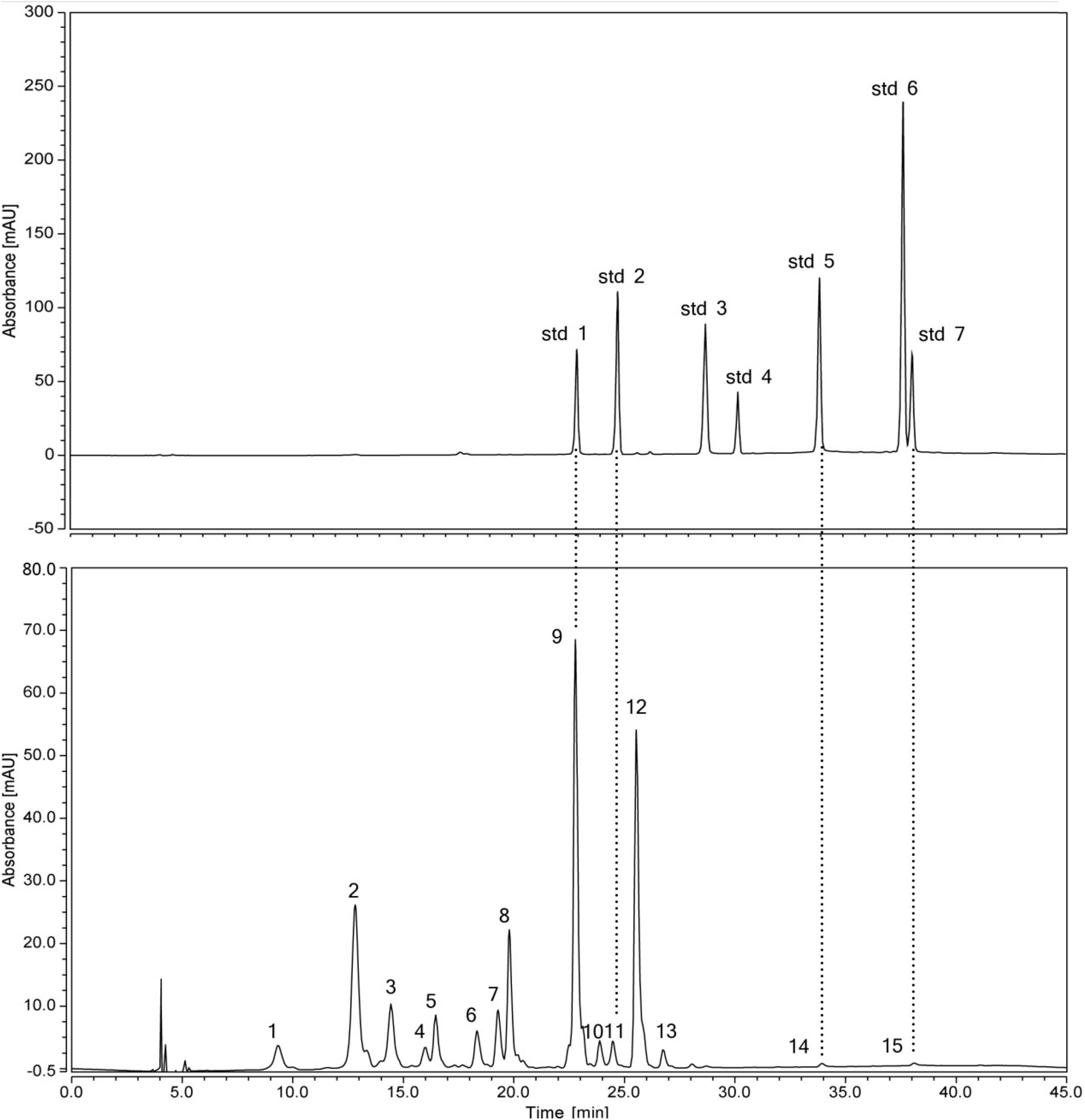
Figure 2. HPLC chromatogram of a mix of standard flavonoids and flavonoids extractd from the petal eye of ‘Little Bumble Bee’ (detection at 350 nm). Std1, rutin; std2, quercetin 3-glucoside; std3, myricetin; std4, kaempferol 3-glucorhamnoside; std5, quercetin; std6, apigenin; std7, kaempferol. Peaks numbers were shown in Table 2.
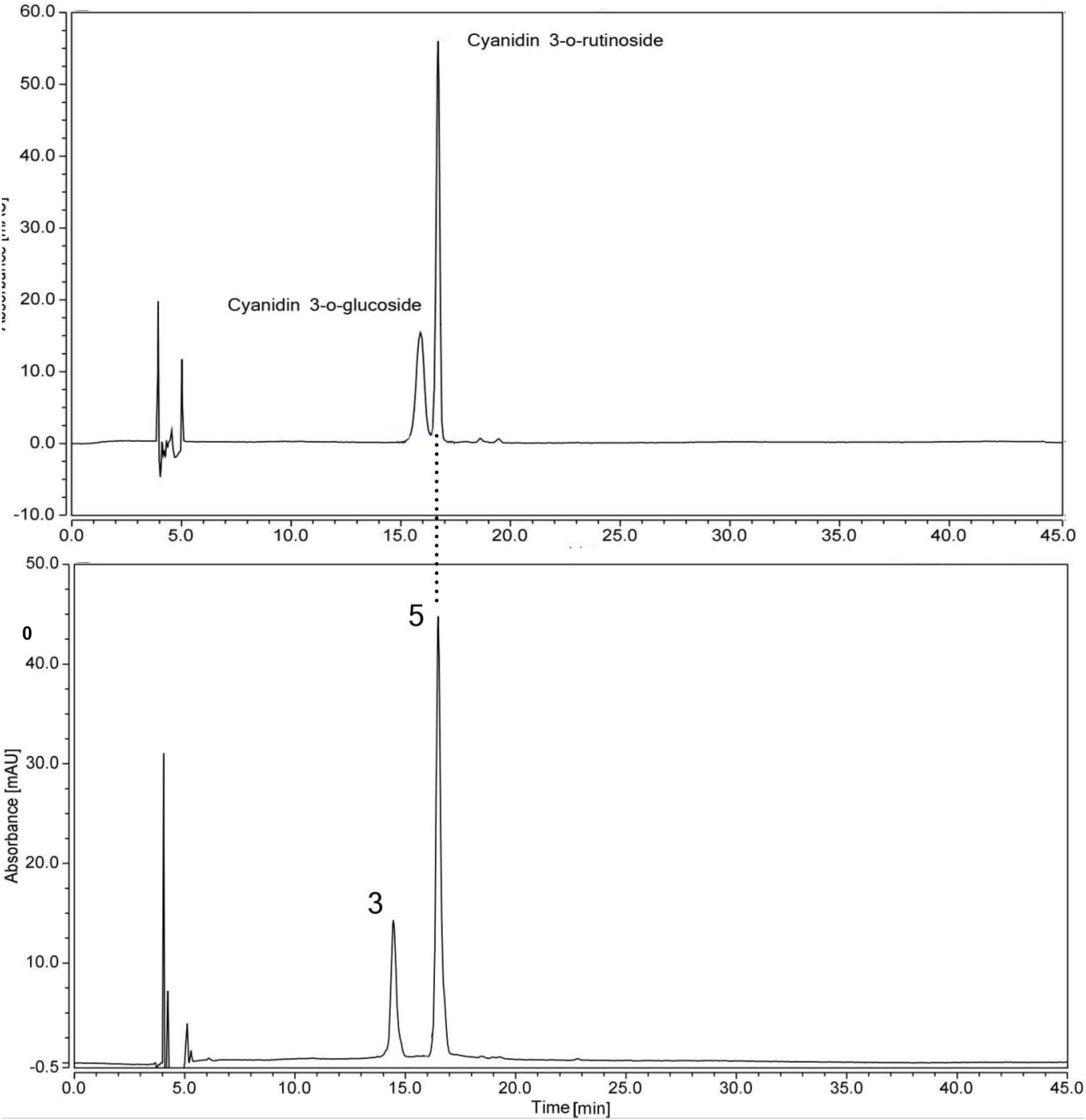
Figure 3. HPLC chromatogram of a mix of standard anthocyanins and flavonoids extracted from the petal eye of ‘Little Bumble Bee’ (detection at 520 nm). Peak numbers were the same as shown in Table 2.
Thirteen of the fifteen separated peaks showed λmax at 350 nm, indicating they were flavonols and flavones. According to four standard retention times (Figure 2) and MS data, the peaks 9, 11, 14, and 15 were speculated as rutin (Rt), quercetin 3-glucoside (Qu3g), quercetin (Qu), and kaempferol (Km), respectively. Rt, Qu, and Km were previously published in the alabastrum of H. fulva (Lin et al., 2011; Chen et al., 2012; Kao et al., 2015). Also, Qu3g is found in H. fulva fresh leaves (Yanjun et al., 2004) and ‘Stella de Oro’ flowers (Cichewicz and Nair, 2002). Leaves of different amaranth species also have abundant Rt, Qu, Qu3g, and Km (Sarker and Oba, 2018, 2019, 2020c,d).
In methanol, kaempferol exhibits λmax spectrum at approximately 266 nm (band II) and 367 nm (band I), while the λmax of quercetin was approximately 255 and 370 nm (Markham, 1989). Furthermore, the glycosylation of the 3-hydroxyls could cause a hypsochromic shift of band I by about 12–17 nm, whereas the 7-hydroxyls would not change the λmax (Singh et al., 2010). Based on these principles, peak 8 was indicated as quercetin 7-glucoside (Qu7g) with λmax spectrum (band I) at 363.3 nm, MS data 463.09 ([M–H]+), and fragment m/z 271 ([Y0]+), with a glucoside (162 Da) loss in molecular weight. Peak 12 showed λmax at 345 nm (band I hypsochromic shift of nearly 21 nm), which was assigned to kaempferol 3-glucoside (Km3g) with the MS data 449.01 ([M–H]+) and fragment m/z 287.04 ([Y0]+). As widely distributed flavonols in many plants (Li et al., 2009; Sarangowa et al., 2014), Qu7g and Km3g are also present in other daylily accessions (Cichewicz and Nair, 2002; Lin et al., 2011; Sun et al., 2018). Peak 4 was characterized as quercetin 3-arabinoside (Qu3ar) because it had fragments at m/z 433.08 ([M–H]–) and 271 ([Y0]–), indicating the loss of an arabinoside (150 Da) (Chen et al., 2010). A similar finding was previously reported in the daylily accession ‘Baihua’ (Sun et al., 2018).
Peak 7 had λmax at 311.18 nm, the MS was 623.09 ([M–H]–) and fragment m/z 315.02 (losing 309 Da), pointing out to isorhamnetin 3-rutinoside (Is3r) (Deng et al., 2013). The Is3r was previously reported in H. fulva (Yanjun et al., 2004) and other plant resources, such as lotus (Deng et al., 2013) and sea buckthorn (Pop et al., 2013). Peaks 10 and 13 were speculated as flavones, luteolin 7-glucoside (Lt7g), and apigenin 7-glucoside (Ap7g), respectively. Lt7g was previously reported in yellow color tree peony (Li et al., 2009) and olive leaves (Lama-Muñoz et al., 2019), while Ap7g has been published in chamomile (Bączek et al., 2019). However, this study is the first to report the two flavones in Hemerocallis.
Unfortunately, peaks 1, 2, and 6 were not inferred. Peak 1 had MS 663 ([M–H]+), and fragment m/z 479 ([Y0]+), indicating the tentative compound was isorhamnetin 3-neohesperidoside. However, the isorhamnetin 3-neohesperidoside showed λmax at around 254 nm in previous reports (Deng et al., 2013; Pop et al., 2013), which was not confirmed (λmax = 324.77 nm) in our study. Similarly, peaks 2 and 6 were also confirmed according to λmax spectrum and MS data.
In our study, peaks 3 and 5 showed λmax at 520 nm with more peak area, indicating they were anthocyanins (Mitchell et al., 1998). Two anthocyanin standards, cyanidin 3-rutinoside (Cy3r) and cyanidin 3-glucoside (Cy3g) were used to identify the different compounds by co-elution, and the retention times were 15.40 min and 16.7 min, respectively (Figure 3). Peak 5 showed the same retention time by the two standards, and further MS data indicated it was Cy3r. However, the retention time of peak 3 was earlier than the Cy3g standard with MS 611.09 ([M–H]+) and m/z 287.1 ([Y0]+). Previous studies proved that the polarity of di-glucosides is greater than that of mono-glucosides. Therefore, the elution time is always earlier than mono-glucosides (Yongcheng, 2018), indicating cyanidin 3,5-glucoside (Cy3g5g) was the speculated compound. Cy3r and Cy3g5g are common anthocyanin glycosides and are widely distributed in many plants, such as wild bananas (Kitdamrongsont et al., 2008), tree peony (Zhang et al., 2014), and rose (Zhang et al., 2015).
Composition and Content of Flavonoids in Different Parts of Floral Organ
The flavonoid composition and contents varied dramatically among the different accessions and parts (Supplementary Table 2), ranging from 0.00 to 321.99 μg g–1 FW. Thus, Rt, Qu3ar, and U1 were dominant flavonoids in Hemerocallis floral organ, comprising 31.58, 20.66, and 15.61% of relative content, respectively. Our study found that most of the flavonoids we detected were higher in the petal than the sepal (Figure 4). The coefficient of variation (CV) ranged from 25.58 to 186.92% (Table 3). For ST, the flavonol content of Qu had the most considerable CV value (149.57%), followed by U2 (135.21%) and Qu7g (128.16%). For SE, the most significant CV was Qu3g with 163.47%, followed by Cy3r with 143.83% and Km with 133.24%, respectively. For SL, the first three compounds with the most extensive variation range were Rt, Is3r, and Cy3r, with CV values of 141.31, 127.52, and 112.80%, respectively. For PT, the largest variation compound was Km (CV = 158.50%), followed by Qu3g and U2 (CV = 151.81% and 147.89%, respectively). For PE, Qu3g had the largest CV value (178.05%), followed by Cy3r (146.04%), and Is3r (138.68%). For PL, the first three compounds with the largest variation range were Is3r, Cy3g5g, and U2, with CV values of 186.92, 136.45, and 121.40%, respectively.
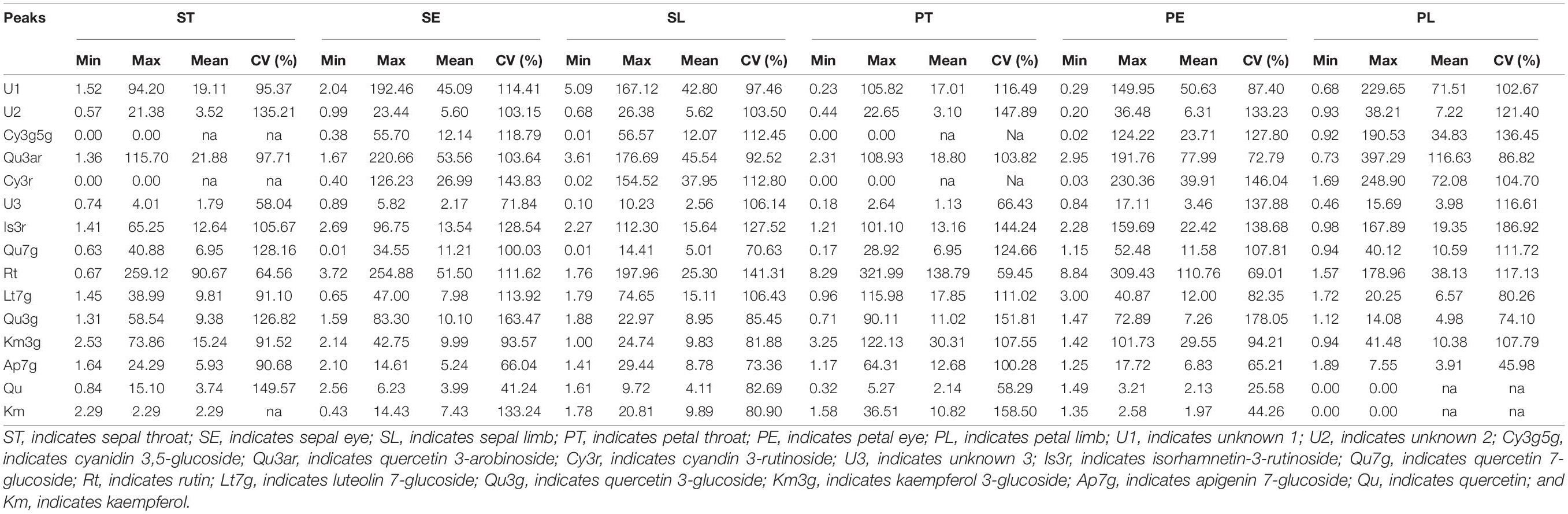
Table 3. The difference of flavonoids among different floral parts in the 42 accessions (μg g–1 FW).
The different flavonoids showed different distribution patterns in the six floral parts. In the three parts of the sepal, including ST, SE, and SL, three compounds, Rt, Lt7g, and Km3g, could be detected in the ST of all the 42 accessions. Moreover, we did not detect Rt in SE of three accessions, including ‘Nakai’, ‘Green Mystique’, and ‘Little Wine Cup.’ Meanwhile, six accessions detected no Rt in SL, including ‘Canadian Border patrol 2’, ‘Green Mystique’, ‘Bonanza’, ‘Dahuaxuancao’, ‘Frans Hals’, and H. fulva var. kwanso var. reasata. In the three parts of the petal, Rt could be detected in PT of all the 42 accessions, but PE was not detected in ‘Canadian Border Patrol’ and ‘Little Wine Cup.’ Thirteen accessions did not detect Rt in PL.
Hence, Rt was the dominant compound (Figure 4), similar to the findings of previous reports (Sun et al., 2018), and mainly distributed in PT. In addition, we discovered that Rt was more widely distributed in the sepal than the petal, but the average content was higher in the petal than the sepal. Moreover, the Rt contents were higher in the eye (SE and PE) than limb (SL and PL). Qu7g, and Km3g also showed the same distribution pattern. Ap7g nearly had the same distribution pattern, except the limb, that SL (8.78 μg g–1 FW) higher than PL (3.91 μg g–1 FW).
In this study, the two cyanidin derivatives (Cy3g5g and Cy3r) could not be detected in nightlilies and daylilies with yellow flowers, while only other 20 colored accessions detected cyanidin derivatives. Moreover, the anthocyanin was nearly not detected in the throat (ST and PT) of the 42 accessions (Supplementary Table 2), indicating no anthocyanin contributed to throat pigment coloration. Throat only had three color phenotypes, yellow, yellow-green, and light yellow, similar to our previous investigation (Cui et al., 2019). As shown in Table 3, the average value of Cy3r ranged from 26.99 to 72.08 μg g–1 FW, which is higher than Cy3g5g (12.07∼34.83 μg g–1 FW). For the Cy3r content, the average SE (26.99 μg g–1 FW) was lower than PE (39.91 μg g–1 FW). Similarly, the SL also showed a lower average value (37.95 μg g–1 FW) than PL (72.08 μg g–1 FW). For Cy3g5g content, SE and SL showed lower average values (12.14 μg g–1 FW and 12.07 μg g–1 FW, respectively) than PE and PL (23.71 μg g–1 FW and 34.83 μg g–1 FW, respectively). Thus, petals had higher anthocyanin contents than sepals in the 20 colored accessions.
The Mann–Whitney U Test Analysis
The calyx of the Hemerocallis flower organ is specialized into a bright colored sepal, which becomes the main ornamental part of the corolla together with petals. However, we revealed that the flavonoid composition and content showed a considerable difference between sepals and petals. Obviously, the flavonoids compositions were different among the various floral parts. This study used the Mann–Whitney U non-parametric test via the R language to analyze the difference in ten major compounds among the six floral parts (Table 4). Generally, the diversity in petal compositions was more than the sepal. Eight compounds showed a significant difference (p < 0.05) between PT and PL, while only three compounds showed a significant difference (p < 0.05) between ST and PT.
For sepals, the ST was significantly different from the other two parts. In the Mann–Whitney U non-parametric test results between ST and SE, six compounds showed significant differences. For instance, Cy3g5g and Rt showed a significant difference at p < 0.001, Lt7g and Km3g at p < 0.01, and two compounds (U1 and Qu3ar) at p < 0.05. At the same time, ST showed a significant difference with SL in Cy3g5g, Rt, and Km3g at p < 0.001, U1 and Qu3ar at p < 0.01, and Qu3g at p < 0.05. However, only Rt showed a significant difference (p < 0.01) between SE and SL.
For petals, the Mann–Whitney U non-parametric test results between PT and PE showed five compounds had significant differences, such as one compound (Cy3g5g) at p < 0.001 level, one compound (Is3r) at p < 0.01 level, and three combinations (Qu3ar, Lt7g, and Ap7g) at p < 0.05. Eight compounds showed significant differences between PT and PL, such as Cy3g5g, Rt, Lt7g, Qu3g, Km3g, and Ap7g at p < 0.001, U1 at p < 0.01, and Qu3ar at p < 0.05. The difference between PE and PL was also significant, which was not similar to sepals; five compounds (Rt, Lt7g, Qu3g, Km3g, and Ap7g) were significantly different at p < 0.001, and one compound, Is3r, was significantly different at p < 0.05.
However, the difference between the same parts in the sepal and petal was not substantial. Only three compounds showed a significant difference between ST and PT, including U1 (p < 0.01), Rt (p < 0.01), and Qu3ar (p < 0.05). The significantly different compounds between SE and PE were Rt and Km3g, p < 0.001, and Is3r at p < 0.05. Between SL and PL, two compounds (Lt7g and Ap7g) showed significant differences at p < 0.001, and two compounds (Qu3g and Km3g) had a considerable difference at p < 0.05.
Hierarchical Cluster Analysis
Hierarchical cluster analysis was conducted by Ward’s D method to accurately describe the characteristics among the 42 accessions. Depending on the variations of ten identified flavonoids and anthocyanin components of the accessions, finally, these accessions were grouped into four clusters (Figure 5). Flavonoid compounds, including flavonols, flavones, etc. in leaves were also varied across the amaranth accessions (Sarker and Oba, 2020a,b; Sarker et al., 2020). One-way ANOVA showed a significant difference in the ten significant compounds between four clusters (p < 0.05). The variations in ten major compounds between the four groups are shown in Figure 6.
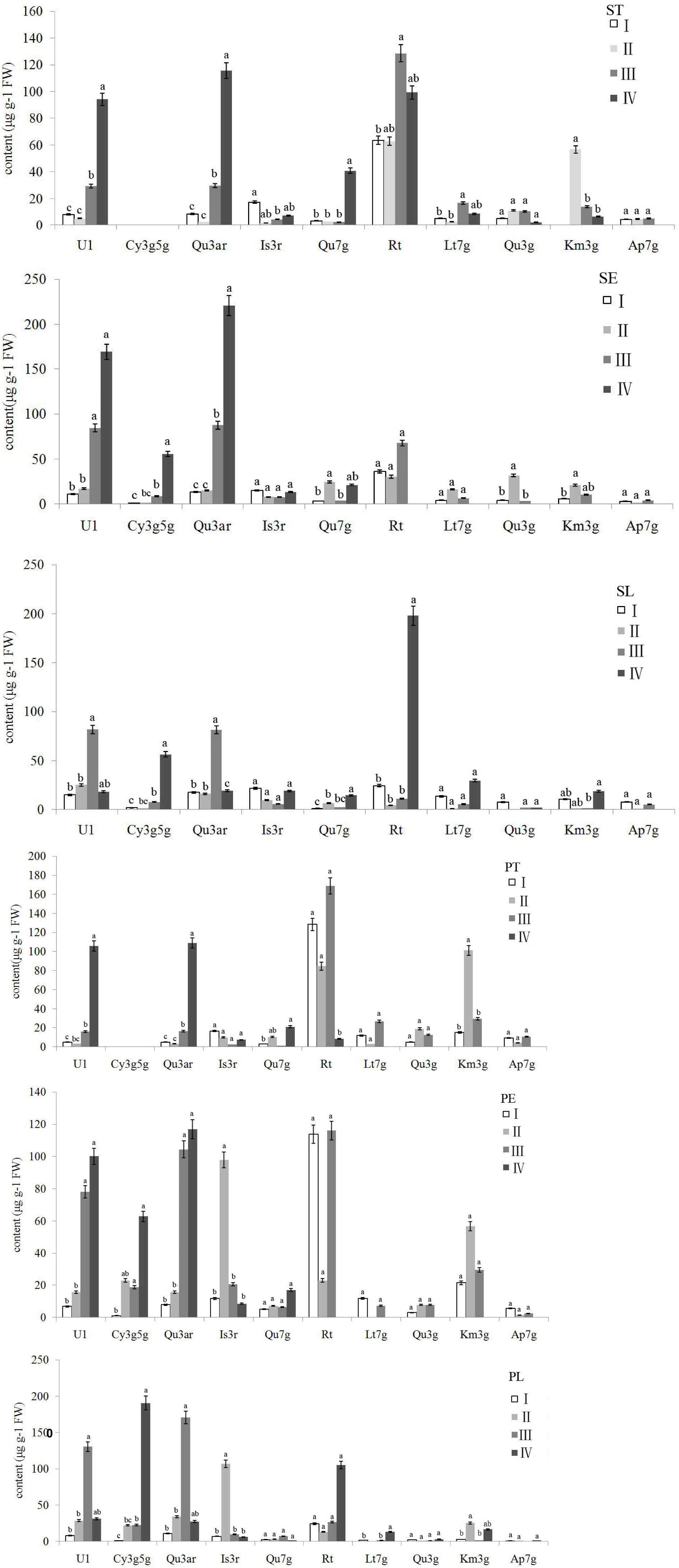
Figure 6. Variations in ten major flavonoids between four clusters. Letters in common are significantly different (p < 0.05). ST, sepal throat; SE, sepal eye; SL, sepal limb; PT, petal throat; PE, petal eye; PL, petal limb.
The accessions falling in clusters I and II had lower U1 and Qu3ar relative to other clusters in the six floral parts. The cluster I was composed of 21 accessions, which could be divided into three subgroups. The landraces of nightlilies clustered in the same subgroup, which included two branches. For instance, ‘Datonghuanghua’, ‘Malinhuanghua’, ‘Yanchihuanghua’, and ‘Qiaotouhuanghua’ fell into the same branch; ‘Dongzhuanghuanghua’, ‘Dalihuanghua’, and H. minor clustered in another branch of this subgroup. The five compounds of Rt, Lt7g, Qu3g, Km3g, and Ap7g were not detected in PL, but the orange ‘Childrens Festival’ clustered into the same branch, which was contrary to our expectation. Meanwhile, the three accessions, H. citrine, H. thunbergii, and H. altissima, and two landraces, ‘Huohuanghua’, and ‘Qiezi’, clustered in another subgroup. The third subgroup included one night lily accession, one purple daylily, two yellow daylily, and two pink daylily accessions.
The cluster II was composed of three pink accessions, ‘Canadian Border Patrol 2’, ‘Always Afternoon’, and ‘Canadian Border Patrol.’ The pink accessions showed the highest average content of Is3r in PE and PL (97.8 and 107 μg g–1 FW, respectively) than other clusters; One-way ANOVA also supported these results (p < 0.05). The Km3g content of ST and PT was also significantly higher (p < 0.05) than other clusters, with average values 56.78 and 101.25 μg g–1 FW, respectively. Although the Km3g content in SE and PE was the highest, the differences were not significant (p < 0.05).
A total of 17 accessions, including most orange and red accessions, fell in cluster III; their PE showed higher contents in Qu3ar and Rt than cluster II. The average Qu3ar was 104.4 μg g–1 FW, ranging from 11.76 to 191.76 μg g–1 FW, while the average Rt was 116 μg g–1 FW, varying from 8.84 to 309.43 μg g–1 FW. The cluster IV was composed of a single ‘Little wine cup’, and it was the only accession that was not detected in Rt, Lt7g, Qu3g, Km3g, and Ap7g, in SE and PE. Thus, ‘Little wine cup’ could be thought of as a natural mutant to study the loss of Rt metabolism in Hemerocallis.
Conclusion
In conclusion, this is the first report on systematic identification and quantification of flavonols, flavones, and anthocyanins in Hemerocallis floral organs. A total of eight flavonols, two flavones, and two anthocyanins were identified. Rutin was the most abundant flavonols in the Hemerocallis tepals, followed by quercetin 3-arabinoside, kaempferol 3-glucoside, isorhamnetin 3-rutinoside. Two flavones, luteolin 7-glucoside and apigenin 7-glucoside, were reported for the first time in Hemerocallis flowers. The flavonoid composition and content varied dramatically among the different accessions. The different flavonoids showed different distribution patterns in the six floral parts. According to the type and content of flavonoids we detected, the 42 accessions were further divided into 4 groups through hierarchical cluster analysis, and each group had similar flower color phenotypes. These results increased our understanding on the diversity of germplasm resources caused by the differential accumulation and distribution of flavonoid in Hemerocallis; and these results is helpful for further studies on the physiol-ecological and molecular mechanisms of flavonoid metabolism pathways in Hemerocallis.
Data Availability Statement
The original contributions presented in the study are included in the article/Supplementary Material, further inquiries can be directed to the corresponding author/s.
Author Contributions
SL collected the germplasm and designed the project. HC and XX ran the HPLC. FH and JW collected and manage the germplasm. XK and GX advised the manuscript and supported the whole project. All authors contributed to the article and approved the submitted version.
Funding
This work was supported by the Key Research and Development Project of Shanxi Province (201903D211011 and 201703D211001-04-05) and the Innovative Talents Plan of Shanxi Agricultural University (BJRC201601).
Conflict of Interest
The authors declare that the research was conducted in the absence of any commercial or financial relationships that could be construed as a potential conflict of interest.
Supplementary Material
The Supplementary Material for this article can be found online at: https://www.frontiersin.org/articles/10.3389/fpls.2021.670506/full#supplementary-material
References
Bączek, K. B., Wiśniewska, M., Przybył, J. L., Kosakowska, O., and Wêglarz, Z. (2019). Arbuscular mycorrhizal fungi in chamomile (Matricaria recutita L.) organic cultivation. Ind. Crops Prod. 140:111562.
Chen, H., Huang, Y., and Qu, J. (2012). Dertermination of rutin, quercetin and kaempferol in alabastrum Hemerocallis fulva from different origin in Gansu province. J. Pharm. Anal. 09, 1574–1577. doi: 10.16155/j.0254-1793.2012.09.013
Chen, H., Zhou, X., Cao, G., and Gan, X. (2010). Determination of quercetin 3-O-α-L-arabinopyranoside in Periploca forrestii by RP-HPLC. J. Chin. Mater. Med. 35, 1284–1286. doi: 10.4268/cjcmm20101014
Cichewicz, R. H., and Nair, M. G. (2002). Isolation and characterization of stelladerol, a new antioxidant naphthalene glycoside, and other antioxidant glycosides from edible daylily (Hemerocallis) flowers. J. Agric. Food Chem. 50, 87–91. doi: 10.1021/jf010914k
Cichewicz, R. H., Zhang, Y., Seeram, N. P., and Nair, M. G. (2004). Inhibition of human tumor cell proliferation by novel anthraquinones from daylilies. Life Sci. 74, 1791–1799. doi: 10.1016/j.lfs.2003.08.034
Cos, P., De Bruyne, T., Hermans, N., Apers, S., Berghe, D. V., and Vlietinck, A. J. (2004). Proanthocyanidins in health care: current and new trends. Curr. Med. Chem. 11, 1345–1359. doi: 10.2174/0929867043365288
Cui, H., Zhang, Y., Shi, X., Gong, F., Xiong, X., Kang, X., et al. (2019). The numerical classification and grading standards of daylily (Hemerocallis) flower color. PLoS One 14:e216460. doi: 10.1371/journal.pone.0216460
Deng, F., Yin, H., Li, J., and Hong, Y. (2003). On latest application and countermeasure for industrialization exploitation of daylily flower. J. Hum. Agric. Univ. 29, 529–532. doi: 10.13331/j.cnki.jhau.2003.06.019
Deng, J., Chen, S., Yin, X., Wang, K., Liu, Y., Li, S., et al. (2013). Systematic qualitative and quantitative assessment of anthocyanins, flavones and flavonols in the petals of 108 lotus (Nelumbo nucifera) cultivars. Food Chem. 139, 307–312. doi: 10.1016/j.foodchem.2013.02.010
Fernandes, L., Casal, S., Pereira, J. A., Saraiva, J. A., and Ramalhosa, E. (2017). Edible flowers: A review of the nutritional, antioxidant, antimicrobial properties and effects on human health. J. Food Compost. Anal. 60, 38–50. doi: 10.1016/j.jfca.2017.03.017
Fu, M., and Mao, L. (2006). A review of the research on the health efficacy and chemical constituents of daylily (Hemerocallis fulva). Food Ferment. Ind. 10, 108–112.
Hsu, Y., Tsai, C., Chen, W., Ho, Y., and Lu, F. (2011). Determination of lutein and zeaxanthin and antioxidant capacity of supercritical carbon dioxide extract from daylily (Hemerocallis disticha). Food Chem. 129, 1813–1818. doi: 10.1016/j.foodchem.2011.05.116
Kao, F. J., Chiang, W. D., and Liu, H. M. (2015). Inhibitory effect of daylily buds at various stages of maturity on nitric oxide production and the involved phenolic compounds. LWT 61, 130–137. doi: 10.1016/j.lwt.2014.11.023
Kitdamrongsont, K., Pothavorn, P., Swangpol, S., Wongniam, S., Atawongsa, K., Svasti, J., et al. (2008). Anthocyanin Composition of Wild Bananas in Thailand. J. Agric. Food Chem. 56, 10853–10857. doi: 10.1021/jf8018529
Lama-Muñoz, A., Contreras, M. D. M., Espínola, F., Moya, M., Romero, I., and Castro, E. (2019). Optimization of Oleuropein and Luteolin-7-O-Glucoside Extraction from Olive Leaves by Ultrasound-Assisted Technology. Energies 12:2486. doi: 10.3390/en12132486
Li, C., Du, H., Wang, L., Shu, Q., Zheng, Y., Xu, Y., et al. (2009). Flavonoid Composition and Antioxidant Activity of Tree Peony (Paeonia Section Moutan) Yellow Flowers. J. Agric. Food Chem. 57, 8496–8503. doi: 10.1021/jf902103b
Li, S., Shi, Q., Hou, F., Chen, Z., Ji, F., Dang, H., et al. (2016). Diversity of the main ornamental traits for Hemerocallis spp. accessions. J. Shanxi Agric. Univ. 09, 619–627. doi: 10.13842/j.cnki.issn1671-8151.2016.09.003
Lin, Y., Lu, C., Huang, Y., and Chen, H. (2011). Antioxidative Caffeoylquinic Acids and Flavonoids from Hemerocallis fulva Flowers. J. Agric. Food Chem. 59, 8789–8795. doi: 10.1021/jf201166b
Manach, C., Scalbert, A., Morand, C., Remesy, C., and Jimenez, L. (2004). Polyphenols: food sources and bioavailability. Am. J. Clin. Nutr. 79, 727–747. doi: 10.1093/ajcn/79.5.727
Markham, K. R. (1989). “Flavones, flavonols and their glycosides,” in Methods Plant Biochemistry, ed. J. B. Harborne (Cambridge: Academic Press), 197–235.
Mitchell, K., Markham, K. R., and Boase, M. R. (1998). Pigment chemistry and colour of Pelargonium flowers. Phytochemistry 47, 355–361. doi: 10.1016/s0031-9422(97)00595-5
Pop, R. M., Socaciu, C., Pintea, A., Buzoianu, A. D., and Sanders, M. G. (2013). UHPLC/PDA-ESI/MS Analysis of the Main Berry and Leaf Flavonol Glycosides from Different Carpathian Hippophaë rhamnoides L. Varieties. Phytochem. Anal. 24, 484–492. doi: 10.1002/pca.2460
Rodriguez-Enriquez, M. J., and Grant-Downton, R. T. (2013). A new day dawning: Hemerocallis (daylily) as a future model organism. AoB Plants 5:s55. doi: 10.1093/aobpla/pls055
Sarangowa, O., Kanazawa, T., Nishizawa, M., Myoda, T., Bai, C., and Yamagishi, T. (2014). Flavonol glycosides in the petal of Rosa species as chemotaxonomic markers. Phytochemistry 107, 61–68. doi: 10.1016/j.phytochem.2014.08.013
Sarker, U., and Oba, S. (2018). Drought stress enhances nutritional and bioactive compounds, phenolic acids and antioxidant capacity of Amaranthus leafy vegetable. BMC Plant Biol. 18:258. doi: 10.1186/s12870-018-1484-1
Sarker, U., and Oba, S. (2019). Antioxidant constituents of three selected red and green color Amaranthus leafy vegetable. Sci. Rep. 9:18233. doi: 10.1007/s11295-011-0447-6
Sarker, U., and Oba, S. (2020c). Polyphenol and flavonoid profiles and radical scavenging activity in leafy vegetable Amaranthus gangeticus. BMC Plant Biol. 20:499. doi: 10.1186/s12870-020-02700-0
Sarker, U., and Oba, S. (2020d). The Response of Salinity Stress-Induced A. tricolor to Growth, Anatomy, Physiology, Non-Enzymatic and Enzymatic Antioxidants. Front. Plant Sci. 11:559876. doi: 10.3389/fpls.2020.559876
Sarker, U., and Oba, S. (2020b). Phenolic profiles and antioxidant activities in selected drought-tolerant leafy vegetable amaranth. Sci. Rep. 10:18287. doi: 10.1038/s41598-020-71727-y
Sarker, U., and Oba, S. (2020a). Nutraceuticals, phytochemicals, and radical quenching ability of selected drought-tolerant advance lines of vegetable amaranth. BMC Plant Biol. 20:564. doi: 10.1186/s12870-020-02780-y
Sarker, U., Hossain, M. N., Iqbal, M. A., and Oba, S. (2020). Bioactive Components and Radical Scavenging Activity in Selected Advance Lines of Salt-Tolerant Vegetable Amaranth. Front. Nutr. 7:587257. doi: 10.3389/fnut.2020.587257
Singh, R., Wu, B., Tang, L., Liu, Z., and Hu, M. (2010). Identification of the Position of Mono-O -glucuronide of Flavones and Flavonols by Analyzing Shift in Online UV Spectrum (λmax) Generated from an Online Diode Array Detector. J. Agric. Food Chem. 58, 9384–9395. doi: 10.1021/jf904561e
Sun, J., Liu, W., Zhang, M., Geng, P., Shan, Y., Li, G., et al. (2018). The analysis of phenolic compounds in daylily using UHPLC-HRMSn and evaluation of drying processing method by fingerprinting and metabolomic approaches. J. Food Process Pres. 42:e13325. doi: 10.1111/jfpp.13325
Tai, C., and Chen, B. (2000). Analysis and stability of carotenoids in the flowers of daylily (Hemerocallis disticha) as affected by various treatments. J. Agric. Food Chem. 48, 5962–5968. doi: 10.1021/jf000956t
Wang, A., Li, R., Ren, L., Gao, X., Zhang, Y., Ma, Z., et al. (2018). A comparative metabolomics study of flavonoids in sweet potato with different flesh colors (Ipomoea batatas (L.) Lam). Food Chem. 260, 124–134. doi: 10.1016/j.foodchem.2018.03.125
Wiseman, S. A., Mathot, J. N., de Fouw, N. J., and Tijburg, L. B. (1996). Dietary non-tocopherol antioxidants present in extra virgin olive oil increase the resistance of low density lipoproteins to oxidation in rabbits. Atherosclerosis 120, 15–23. doi: 10.1016/0021-9150(95)05656-4
Yanjun, Z., Chichewicz, R. H., and Nair, M. G. (2004). Lipid peroxidation inhibitory compounds from daylily (Hemerocallis fulva) leaves. Life Sci. 75, 753–763. doi: 10.1016/j.lfs.2004.07.001
Yongcheng, N. (2018). Structural Identification of Organic Compounds with Spectroscopic Techniques, 4th Edn. Beijing: Science Press.
Zhang, C., Wang, W., Wang, Y., Gao, S., Du, D., Fu, J., et al. (2014). Anthocyanin biosynthesis and accumulation in developing flowers of tree peony (Paeonia suffruticosa) ‘Luoyang Hong’. Postharvest. Biol. Technol. 97, 11–22. doi: 10.1016/j.postharvbio.2014.05.019
Zhang, L., Xu, Z., Tang, T., Zhang, H., and Zhao, L. (2015). Analysis of anthocyanins related compounds and their biosynthesis pathways in Rosa rugosa ‘Zi Zhi’ at blooming stages. Sci. Agri. Sin. 13, 2600–2611. doi: 10.3864/j.issn.0578-1752.2015.13.012
Keywords: Hemerocallis, flavonoids, floral organ, HPLC, qualitative, quantitative
Citation: Li S, Cui H, Wang J, Hou F, Xiong X, Kang X and Xing G (2021) Qualitative and Quantitative Analysis on Flavonoid Distribution in Different Floral Parts of 42 Hemerocallis Accessions. Front. Plant Sci. 12:670506. doi: 10.3389/fpls.2021.670506
Received: 21 February 2021; Accepted: 29 March 2021;
Published: 07 May 2021.
Edited by:
Deyu Xie, North Carolina State University, United StatesReviewed by:
Umakanta Sarker, Bangabandhu Sheikh Mujibur Rahman Agricultural University, BangladeshMarcelo J. Pena Ferreira, University of São Paulo, Brazil
Copyright © 2021 Li, Cui, Wang, Hou, Xiong, Kang and Xing. This is an open-access article distributed under the terms of the Creative Commons Attribution License (CC BY). The use, distribution or reproduction in other forums is permitted, provided the original author(s) and the copyright owner(s) are credited and that the original publication in this journal is cited, in accordance with accepted academic practice. No use, distribution or reproduction is permitted which does not comply with these terms.
*Correspondence: Sen Li, saulisen@163.com; Guoming Xing, xingguoming@163.com