- 1School of Ecology and Environment, Northwestern Polytechnical University, Xi'an, China
- 2Shenzhen Research Institute of Northwestern Polytechnic University, Shenzhen, China
- 3Institute of Ecological Agriculture, Sichuan Agricultural University, Chengdu, China
Introduction
Hypoxia is one of the major abiotic stresses, primarily caused by numerous flooding events such as waterlogging and submergence (Zhou et al., 2020a; Xie et al., 2021), with deleterious effects on plant growth and development (Bailey-Serres et al., 2012; Voesenek and Bailey-Serres, 2015; Xie et al., 2021; Zhou et al., 2021). Due to the excessive water absorption, hypoxia mechanically damages seed germination, seedling establishment, and finally crop yield (Nakayama et al., 2004; Arguello et al., 2016; Yanjun et al., 2016; Striker and Colmer, 2017; Wang et al., 2017; Shen et al., 2020; Lee et al., 2021; Tian et al., 2021). Further, flooding decreases the seed quality of cotton and soybean by altering the accumulation and distribution of carbohydrates, oil, and protein (Wang et al., 2018; Xu et al., 2021). Collectively, hypoxia stress negatively regulates numerous aspects of plant development.
Abscisic acid (ABA) is an essential phytohormone that regulates plant growth and development, such as seed germination, seed dormancy, seed longevity, and seedling establishment (Zhu, 2016; Khan et al., 2020; Umashankar et al., 2020; Zhou et al., 2020b). It is worth noting that ABA also responds to abiotic stresses such as drought, salt, and water stresses (Zhu, 2016; Shu et al., 2018). ABA induces stomata formation on underwater leaves (Iida et al., 2016) and controls stomatal movement by regulating the size of guard cells, thus mediating water potential in plants (Zhu, 2016; He et al., 2018). During hypoxia stress, ABA biosynthesis is inhibited, while the catabolism cascade is enhanced, and thus, exogenous ABA can increase the tolerance of plants to hypoxia stress (Dawood et al., 2016; De Ollas et al., 2021). Under flooding conditions, pretreatment with ABA increases the abundance of protein through glycolysis, fermentation, and tricarboxylic acid cycle (TCA), thereby enhancing hypoxic properties and improving survival rate in soybean (Komatsu et al., 2013; Yin et al., 2016; Wang et al., 2018). Moreover, the application of ABA positively regulates the net assimilation rate (NAR), relative growth rate (RGR), and chlorophyll content of rice under flooding (Saha et al., 2021). However, these reports did not provide a detailed molecular mechanism of hormone regulation under hypoxia. Therefore, considering the response of ABA to hypoxia stress, we need to understand the detailed molecular mechanisms, especially the underlying mechanisms of ABA biosynthesis, catabolism, and signal transduction under hypoxia stress. Here, this opinion intends to highlight some critical unanswered questions, which need to be addressed in future exploration.
Abscisic Acid, Ethylene, and Gibberellin (GA) Cross Talk in Response of Plants to Hypoxia Stress
Because of its gaseous nature, it is difficult for ethylene to leave the plant under flooding conditions, so it rapidly accumulates inside the plant and reflects the predicament of plants (Hattori et al., 2009; Alpuerto et al., 2016). Ethylene is the primary signal for adaptation of a plant to flooding (Loreti et al., 2016), which regulates ABA, GA, and auxin, affecting plant growth and development under hypoxia stress (Steffens et al., 2006; Vidoz et al., 2010; Dawood et al., 2016; Yang et al., 2018). Ethylene involves in stimulating bud elongation, aerenchyma development, and adventitious root (AR) formation under flooding conditions (Voesenek et al., 2003; Rajhi et al., 2010; Dawood et al., 2016; Nguyen et al., 2018). As such, ethylene has become a hot topic in hypoxia research like flooding.
Ethylene accumulated under flooding stress induces elongation by inhibiting the biosynthesis of ABA in Rumex palustris (Benschop et al., 2005). Thus, with the increased ethylene level under waterlogging conditions, the ABA concentration decreases (Figure 1) and endogenous GA increases. The reduction in ABA is necessary for the submergence-induced GA response, which promotes internode or petiole elongation (Kende et al., 1998; Benschop et al., 2006). Mechanistically, the accumulated ethylene inhibits the expression of 9-cis-epoxycarotenoid dioxygenase encoding genes (NCEDs), which also leads to the breakdown of ABA into phaseic acid (PA), thereby reducing ABA content (Benschop et al., 2005; Saika et al., 2007). A reduction in ABA content interferes with the GA pathway, leading to rapid shoot elongation under submergence, as seen in marsh docks (Benschop et al., 2006) and rice (Kende et al., 1998). Similarly, flooding increases stem elongation in deepwater rice varieties, partially by reducing endogenous ABA content and increasing GA concentration (Yang and Choi, 2006).
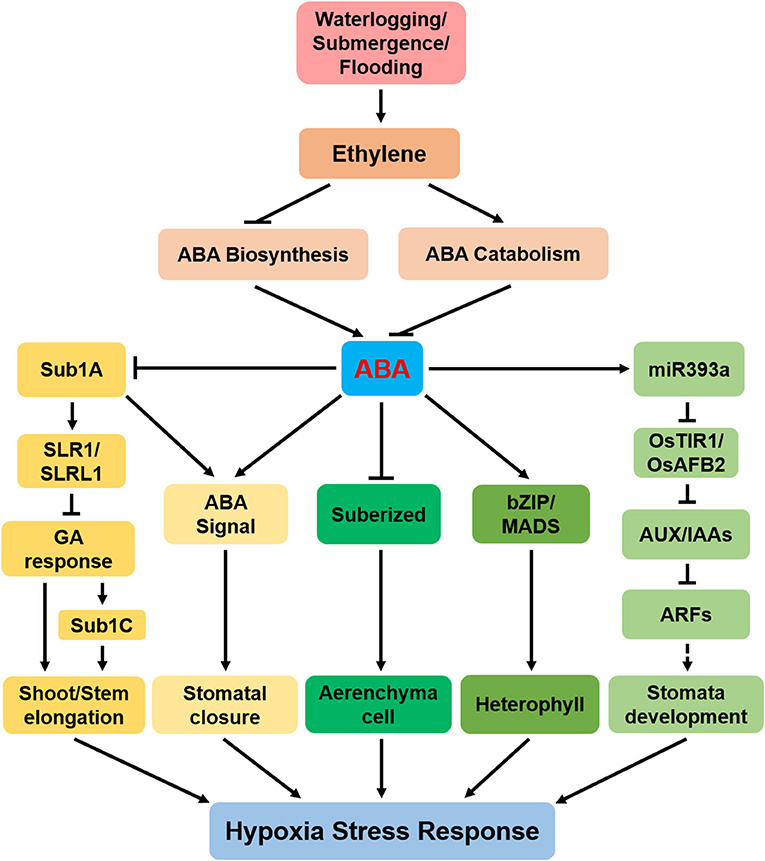
Figure 1. A model showing that abscisic acid (ABA) regulates hypoxia stress response. Submergence, waterlogging, and flooding cause hypoxia, which leads to ethylene accumulation. Ethylene positively regulates ABA catabolism and negatively regulates ABA biosynthesis, thereby affecting ABA content. ABA affects tolerance of plant hypoxia through the following pathways: (1) ABA negatively regulates submergence1 A (Sub1A) expression. Sub1A promotes the accumulation of two gibberellin (GA)-negative regulators [SLENDER RICE 1 (SLR1) and SLENDER RICE-LIKE 1 (SLRL1)] and then directly promotes shoot elongation; (2) ABA signal induces stomata to close; (3) ABA positively regulates the formation of aerenchyma cell by negatively regulating root cell suberization; (4) ABA induces the expression of the basic region-leucine zipper (bZIP/MADS) to promote the formation of special-shaped leaves of submerged plants and semiaquatic plants; (5) ABA positively regulates miR393a, then negatively regulates auxin signaling cascade, and finally positively regulates stomatal development. The arrows indicate the promotion effect, and the flat lines indicate the inhibition effect. Dotted lines indicate indirect interactions.
Ethylene and its precursor 1-aminocyclopropane-1-carboxylic acid (ACC) induce rapidly the expression of ABA 8′-hydroxylase 1 (OsABA8ox1), and pretreatment with the ethylene receptor inhibitor 1-methylcyclopropene (1-MCP) inhibits its expression (Saika et al., 2007). These results suggest that under flooding conditions, the rapid decline of ABA in deepwater rice varieties is partly controlled by the ethylene-induced expression of OsABA8ox1 (Saika et al., 2007; Pan et al., 2021). Degradation of ABA is enhanced by submergence in submergence1 A (Sub1A)-independent manner (Figure 1) (Fukao and Bailey-Serres, 2008). At the same time, the exogenous ABA decreases the abundance of the Sub1 gene, suggesting that during submergence, reduction in ABA content may be a prerequisite for the increased accumulation of Sub1 transcript (Fukao and Bailey-Serres, 2008). Thus, to determine the cross talk between ethylene and ABA under hypoxia stress, it is necessary to clarify the molecular mechanisms by which ethylene regulates ABA biosynthesis and/or signaling.
Abscisic Acid Biosynthesis Under Hypoxia Stress
The abscisic acid level in internode meristem and cell elongation zone of submerged plants decreased by 75% in deepwater rice after flooding (Kende, 1992). Similarly, endogenous ABA content in Rumex palustris decreases in petioles after submergence (Cox et al., 2004). In addition, a recent study also showed that flooding leads to the decline in ABA level in tomato roots (De Ollas et al., 2021). What is the reason for the decrease in ABA content under hypoxia stress? In rice, the expressions of OsNCED1, OsNCED2, and OsNCED3 decrease rapidly after submergence (Saika et al., 2007). AtNCED3 expression is also downregulated in roots under submergence, and endogenous ABA level of root decreases significantly (Hsu et al., 2011). Meanwhile, the upregulation of AtNCED4 expression in shoots under submergence is also documented (Hsu et al., 2011). These results suggest that regulation of ABA biosynthesis in the aboveground and underground parts is distinct and needs further exploration.
In Solanum dulcamara, transcription of two NCED genes is significantly downregulated under flooding; thus, ABA content in ARs and stems reduces substantially (Dawood et al., 2016). Further studies found that the partial submersion and complete submersion both have no difference in AR growth, and both of them attenuate the expression of NCEDs, thereby reducing ABA content (Yang et al., 2018). With the decrease in expressions of TaNCEDs (TaNCED1 and TaNCED2) and ABA content in stem nodes, ARs appeared on stem nodes after waterlogging in Triticum aestivum L. (Nguyen et al., 2018). All the available evidence supports the fact that ABA is a negative regulator of AR formation and shoot elongation under hypoxia stress (Figure 1).
Abscisic Acid Catabolism Under Hypoxia Stress
In plants, the catabolism of ABA has two pathways: One is the direct inactivation to form PA, which is the oxidative inactivation pathway. The other is combined with glucose to produce ABA-glucose ester (ABA-GE) and is named as binding inactivation pathway. In Rumex species and rice, the high expression of OsABA8ox1 after submergence accelerates the ABA degradation and forms a large amount of PA (Benschop et al., 2005; Saika et al., 2007). Studies have found that ABA-GE is involved in regulating the response of plants to drought, salt, and saline–alkali stresses (Dietz et al., 2000; Xu et al., 2012; Gong et al., 2014; Dong et al., 2015; Wang et al., 2020). However, existing reports have found that ABA-GE level under submergence has not changed in rice (Saika et al., 2007; Fukao and Bailey-Serres, 2008). These analyses confirmed that during submersion, the hydroxylation of ABA to PA is the primary pathway of ABA catabolism.
In deepwater rice, the rapid decrease in ABA content is a prerequisite for the increase in bud elongation (Kende et al., 1998; Steffens et al., 2006). Further research found that transcription of cytochrome P450 A5 (CYP707A5) gene is significantly upregulated under submergence in deepwater rice, thus promoting ABA catabolism (Yang and Choi, 2006). SdABA8ox is upregulated in the AR primordia of Solanum dulcamara after flooding, and the ABA level gets significantly reduced (Dawood et al., 2016). In Nasturtium officinale, CYP707A1, and CYP707A2 are induced under submergence, showing a decline in ABA level (Müller et al., 2019). Similarly, CYP707A1-1 expression is induced and the mRNA level of CYP707A1-2 is downregulated in both partial submersion and complete submersion in Solanum dulcamara (Yang et al., 2018). In a nutshell, hypoxia promotes ABA catabolism (Figure 1). However, the specific molecular mechanism of enhanced ABA catabolism under hypoxia needs to be elucidated.
Abscisic Acid Signaling Under Hypoxia Stress
It has been reported that the expressions of ABA receptor genes pyrabactin resistance (PYR) and pyrabactin resistance-like (PYL) increase after flooding (Arbona et al., 2017; De Ollas et al., 2021). This may be feedback for maintaining a certain level of ABA signal under flooded soil. Further, the expressions of late embryogenesis abundant proteins 5 (LEA5), LEA14-1, LEA14-2, and ABA-insensitive 5 (ABI5) also reduce under submergence (Yang et al., 2018).
Abscisic acid regulates heterophylly initiation through basic region-leucine zipper (bZIP) class genes and AGAMOUS-like 11 (AGL11) gene in Marsilea quadrifolia (Hsu et al., 2001; Shan et al., 2009). OE-SUB1A enhances the sensitivity to ABA, which is consistent with the inhibition of ABA on seed germination and bud elongation (Fukao et al., 2011). Under waterlogging stress, RELATED TO APETALA2.6-LIKE (RAP2.6L) inhibits ABI1 transcription, and the abi1-1/OE-RAP2.6L double mutant showed increased sensitivity to ABA, which suggests that the overexpression of RAP2.6L modifies the ABA-insensitive phenotype of abi1-1 mutant (Liu et al., 2012). In particular, the zinc-containing finger/BTB (bric-a-brac, tramtrack, and broad complex) domain-containing protein 47, glycine-rich protein, and rRNA-processing protein Rrp5 associated with ABA response are significantly phosphorylated under flooding stress (Yin and Komatsu, 2015). Meanwhile, ABA inhibits the elongation of the coleoptile by upregulating miR393a transcription (Guo et al., 2016). Although there are some studies on the above aspects, the specific mechanism still needs further explanation and clarification.
Conclusions and Perspectives
The regulation of plant growth and development under waterlogging stress is very complex, and a single hormone might not fully reflect the adaptation strategy of plants to hypoxia. ABA, as the downstream of ethylene, regulates plant response to hypoxia stress (Figure 1).
All the current studies mainly focus on the vegetative growth stage of plants under flooding stress. The following aspects need more attention in future studies: (i) The regulatory mechanism of ABA in seed germination and early seedling morphogenesis under waterlogging stress is a worthy subject. (ii) How does ABA regulate plant reproductive growth under submergence? (iii) Because exogenous ABA can alleviate flooding stress, thus, the development of an anti-waterlogging regulator by modifying ABA is a novel interesting project.
Furthermore, are there unknown genetic factors that control ABA-mediated cascade under waterlogging conditions? Does the kinase involved in the ABA signaling pathway to regulate seed germination, stomatal movement, and reproductive growth in hypoxia environments? These questions are essential to fully understand the hypoxia response of plants and are especially important for crops. Together, a better understanding of ABA biosynthesis and signaling during flooding can further dissect the metabolic and genetic pathways that adapt to flooding pressures and will ultimately help us to develop more resilient crop varieties.
Author Contributions
QW and KS designed the opinion. LW, XL, and CZ helped in providing the inputs. QW, LW, UC, and KS wrote the manuscript. All authors contributed to the article and approved the submitted version.
Funding
Our research team was currently supported by funding from the National Natural Science Foundation of China (31872804 and 31701064), the Science, Technology and Innovation Commission of Shenzhen Municipality (JCYJ20190806154009040), and the Talents Team Construction Fund of Northwestern Polytechnical University (31020190QD007).
Conflict of Interest
The authors declare that the research was conducted in the absence of any commercial or financial relationships that could be construed as a potential conflict of interest.
Acknowledgments
We thank all the laboratory members for their valuable discussions during the preparation of this study.
References
Alpuerto, J. B., Hussain, R. M. F., and Fukao, T. (2016). The key regulator of submergence tolerance, SUB1A, promotes photosynthetic and metabolic recovery from submergence damage in rice leaves. Plant Cell Environ. 39, 672–684. doi: 10.1111/pce.12661
Arbona, V., Zandalinas, S. I., Manzi, M., González-Guzmán, M., Rodriguez, P. L., and Gómez-Cadenas, A. (2017). Depletion of abscisic acid levels in roots of flooded Carrizo citrange (Poncirus trifoliata L. Raf. × Citrus sinensis L. Osb.) plants is a stress-specific response associated to the differential expression of PYR/PYL/RCAR receptors. Plant Mol. Biol. 93, 623–640. doi: 10.1007/s11103-017-0587-7
Arguello, M. N., Mason, R. E., Roberts, T. L., Subramanian, N., Acuña, A., Addison, C. K., et al. (2016). Performance of soft red winter wheat subjected to field soil waterlogging: grain yield and yield components. Field Crops Res. 194, 57–64. doi: 10.1016/j.fcr.2016.04.040
Bailey-Serres, J., Fukao, T., Gibbs, D. J., Holdsworth, M. J., Lee, S. C., Licausi, F., et al. (2012). Making sense of low oxygen sensing. Trends Plant Sci. 17, 129–138. doi: 10.1016/j.tplants.2011.12.004
Benschop, J. J., Bou, J., Peeters, A. J. M., Wagemaker, N., Gühl, K., Ward, D., et al. (2006). Long-term submergence-induced elongation in Rumex palustris requires abscisic acid-dependent biosynthesis of gibberellin. Plant Physiol. 141, 1644–1652. doi: 10.1104/pp.106.082636
Benschop, J. J., Jackson, M. B., Gühl, K., Vreeburg, R. A. M., Croker, S. J., Peeters, A. J. M., et al. (2005). Contrasting interactions between ethylene and abscisic acid in Rumex species differing in submergence tolerance. Plant J. 44, 756–768. doi: 10.1111/j.1365-313X.2005.02563.x
Cox, M. C. H., Benschop, J. J., Vreeburg, R. A. M., Wagemaker, C. A. M., Moritz, T., Peeters, A. J. M., et al. (2004). The roles of ethylene, auxin, abscisic acid, and gibberellin in the hyponastic growth of submerged rumex palustris petioles. Plant Physiol. 136, 2948–2960. doi: 10.1104/pp.104.049197
Dawood, T., Yang, X., Visser, E. J. W., Beek, T. A. H. T., Kensche, P. R., Cristescu, S. M., et al. (2016). A co-opted hormonal cascade activates dormant adventitious root primordia upon flooding in Solanum dulcamara. Plant Physiol. 170, 2351–2364. doi: 10.1104/pp.15.00773
De Ollas, C., González-Guzmán, M., Pitarch, Z., Matus, J. T., Candela, H., Rambla, J. L., et al. (2021). Identification of ABA-mediated genetic and metabolic responses to soil flooding in tomato (Solanum lycopersicum L. Mill). Front. Plant Sci. 12:613059. doi: 10.3389/fpls.2021.613059
Dietz, K. J., Sauter, A., Wichert, K., Messdaghi, D., and Hartung, W. (2000). Extracellular β-glucosidase activity in barley involved in the hydrolysis of ABA glucose conjugate in leaves. J. Exp. Bot. 51, 937–944. doi: 10.1093/jexbot/51.346.937
Dong, T., Park, Y., and Hwang, I. (2015). Abscisic acid: biosynthesis, inactivation, homoeostasis and signalling. Essays Biochem. 58, 29–48. doi: 10.1042/bse0580029
Fukao, T., and Bailey-Serres, J. (2008). Submergence tolerance conferred by Sub1A is mediated by SLR1 and SLRL1 restriction of gibberellin responses in rice. Proc. Natl. Acad. Sci. U.S.A. 105, 16814–16819. doi: 10.1073/pnas.0807821105
Fukao, T., Yeung, E., and Bailey-Serres, J. (2011). The submergence tolerance regulator SUB1A mediates crosstalk between submergence and drought tolerance in rice. Plant Cell. 23, 412–427. doi: 10.1105/tpc.110.080325
Gong, B., Zhang, C. J., Li, X., Wen, D., Wang, S. S., Shi, Q. H., et al. (2014). Identification of NaCl and NaHCO3 stress responsive proteins in tomato roots using iTRAQ-based analysis. Biochem. Biophys. Res. Commun. 446, 417–422. doi: 10.1016/j.bbrc.2014.03.005
Guo, F., Han, N., Xie, Y., Fang, K., Yang, Y., Zhu, M., et al. (2016). The miR393a/target module regulates seed germination and seedling establishment under submergence in rice (Oryza sativa L.). Plant Cell Environ. 39, 2288–2302. doi: 10.1111/pce.12781
Hattori, Y., Nagai, K., Furukawa, S., Song, X.-J., Kawano, R., Sakakibara, H., et al. (2009). The ethylene response factors SNORKEL1 and SNORKEL2 allow rice to adapt to deep water. Nature 460, 1026–1030. doi: 10.1038/nature08258
He, F., Wang, H.-L., Li, H.-G., Su, Y., Li, S., Yang, Y., et al. (2018). PeCHYR1, a ubiquitin E3 ligase from Populus euphratica, enhances drought tolerance via ABA-induced stomatal closure by ROS production in Populus. Plant Biotechnol. J. 16, 1514–1528. doi: 10.1111/pbi.12893
Hsu, F.-C., Chou, M.-Y., Peng, H.-P., Chou, S.-J., and Shih, M.-C. (2011). Insights into hypoxic systemic responses based on analyses of transcriptional regulation in Arabidopsis. PLoS ONE 6:e28888. doi: 10.1371/journal.pone.0028888
Hsu, T.-C., Liu, H.-C., Wang, J.-S., Chen, R.-W., Wang, Y.-C., and Lin, B.-L. (2001). Early genes responsive to abscisic acid during heterophyllous induction in Marsilea quadrifolia. Plant Mol. Biol. 47, 703–715. doi: 10.1023/A:1013612331583
Iida, S., Ikeda, M., Amano, M., Sakayama, H., Kadono, Y., and Kosuge, K. (2016). Loss of heterophylly in aquatic plants: not ABA-mediated stress but exogenous ABA treatment induces stomatal leaves in Potamogeton perfoliatus. J. Plant Res. 129, 853–862. doi: 10.1007/s10265-016-0844-x
Kende, H., Knaap, E., and Cho, H. (1998). Deepwater rice: a model plant to study stem elongation. Plant Physiol. 118, 1105–1110. doi: 10.1104/pp.118.4.1105
Kende, S. H.-B. H. (1992). On the role of abscisic acid and gibberellin in the regulation of growth in rice. Plant Physiol. 99, 1156–1161. doi: 10.1104/pp.99.3.1156
Khan, I. U., Ali, A., Khan, H. A., Baek, D., and Yun, D. J. (2020). PWR/HDA9/ABI4 complex epigenetically regulates ABA dependent drought stress tolerance in Arabidopsis. Front. Plant Sci. 11:623. doi: 10.3389/fpls.2020.00623
Komatsu, S., Han, C., Nanjo, Y., Altaf-Un-Nahar, M., Wang, K., He, D., et al. (2013). Label-free quantitative proteomic analysis of abscisic acid effect in early-stage soybean under flooding. J. Proteome Res. 12, 4769–4784. doi: 10.1021/pr4001898
Lee, E.-J., Kim, K. Y., Zhang, J., Yamaoka, Y., Gao, P., Kim, H., et al. (2021). Arabidopsis seedling establishment under waterlogging requires ABCG5-mediated formation of a dense cuticle layer. New Phytol. 229, 156–172. doi: 10.1111/nph.16816
Liu, P., Sun, F., Gao, R., and Dong, H. (2012). RAP2.6L overexpression delays waterlogging induced premature senescence by increasing stomatal closure more than antioxidant enzyme activity. Plant Mol. Biol. 79, 609–622. doi: 10.1007/s11103-012-9936-8
Loreti, E., Veen, H., and Perata, P. (2016). Plant responses to flooding stress. Curr. Opin. Plant Biol. 33, 64–71. doi: 10.1016/j.pbi.2016.06.005
Müller, J. T., Veen, H., Bartylla, M. M., Akman, M., Pedersen, O., Sun, P., et al. (2019). Keeping the shoot above water – submergence triggers antithetical growth responses in stems and petioles of watercress (Nasturtium officinale). New Phytol. 229, 140–155. doi: 10.1111/nph.16350
Nakayama, N., Hashimoto, S., Shimada, S., Takahashi, M., Kim, Y.-H., Oya, T., et al. (2004). The effect of flooding stress at the germination stage on the growth of soybean in relation to initial seed moisture content. Japan. J. Crop Sci. 73, 323–329. doi: 10.1626/jcs.73.323
Nguyen, T.-N., Tuan, P. A., Mukherjee, S., Son, S., and Ayele, B. T. (2018). Hormonal regulation in adventitious roots and during their emergence under waterlogged conditions in wheat (Triticum aestivum L.). J. Exp. Bot. 69, 4065–4082. doi: 10.1093/jxb/ery190
Pan, J., Sharif, R., Xu, X., and Chen, X. (2021). Mechanisms of waterlogging tolerance in plants: research progress and prospects. Front. Plant Sci. 11, 627331–627331. doi: 10.3389/fpls.2020.627331
Rajhi, I., Yamauchi, T., Takahashi, H., Nishiuchi, S., Shiono, K., Watanabe, R., et al. (2010). Identification of genes expressed in maize root cortical cells during lysigenous aerenchyma formation using laser microdissection and microarray analyses. New Phytol. 190, 351–368. doi: 10.1111/j.1469-8137.2010.03535.x
Saha, I., Hasanuzzaman, M., Dolui, D., Sikdar, D., Debnath, S. C., and Adak, M. K. (2021). Silver-nanoparticle and abscisic acid modulate sub1A quantitative trait loci functioning towards submergence tolerance in rice (Oryza sativa L.). Environ. Exp. Bot. 181:104276. doi: 10.1016/j.envexpbot.2020.104276
Saika, H., Okamoto, M., Miyoshi, K., Kushiro, T., Shinoda, S., Jikumaru, Y., et al. (2007). Ethylene promotes submergence-induced expression of OsABA8ox1, a gene that encodes ABA 8'-hydroxylase in rice. Plant Cell Physiol. 48, 287–298. doi: 10.1093/pcp/pcm003
Shan, H., Zahn, L., Guindon, S., Wall, P. K., Kong, H., Ma, H., et al. (2009). Evolution of plant MADS box transcription factors: evidence for shifts in selection associated with early angiosperm diversification and concerted gene duplications. Mol. Biol. Evol. 26, 2229–2244. doi: 10.1093/molbev/msp129
Shen, C., Yuan, J., Qiao, H., Wang, Z., Liu, Y., Ren, X., et al. (2020). Transcriptomic and anatomic profiling reveal the germination process of different wheat varieties in response to waterlogging stress. BMC Genetics 21:93. doi: 10.1186/s12863-020-00901-y
Shu, K., Zhou, W., Chen, F., Luo, X., and Yang, W. (2018). Abscisic acid and gibberellins antagonistically mediate plant development and abiotic stress responses. Front. Plant Sci. 9:416. doi: 10.3389/fpls.2018.00416
Steffens, B., Wang, J., and Sauter, M. (2006). Interactions between ethylene, gibberellin and abscisic acid regulate emergence and growth rate of adventitious roots in deepwater rice. Planta 223, 604–612. doi: 10.1007/s00425-005-0111-1
Striker, G. G., and Colmer, T. D. (2017). Flooding tolerance of forage legumes. J. Exp. Bot. 68, 1851–1872. doi: 10.1093/jxb/erw239
Tian, L.-X., Zhang, Y.-C., Chen, P.-L., Zhang, F.-F., Li, J., Yan, F., et al. (2021). How does the waterlogging regime affect crop yield? A global meta-analysis. Front. Plant Sci. 12, 634898–634898. doi: 10.3389/fpls.2021.634898
Umashankar, C., Xiaofeng, L., Wenguan, Z., and Kai, S. (2020). Multifaceted signaling networks mediated by abscisic acid insensitive 4. Plant Commun. 1:100040. doi: 10.1016/j.xplc.2020.100040
Vidoz, M. L., Loreti, E., Mensuali, A., Alpi, A., and Perata, P. (2010). Hormonal interplay during adventitious root formation in flooded tomato plants. Plant J. 63, 551–562. doi: 10.1111/j.1365-313X.2010.04262.x
Voesenek, L. A. C. J., and Bailey-Serres, J. (2015). Flood adaptive traits and processes: an overview. New Phytol. 206, 57–73. doi: 10.1111/nph.13209
Voesenek, L. A. C. J., Benschop, J. J. J., Bou, M. C. H. C., Groeneveld, H. W., Millenaar, F. F., Vreeburg, R. A. M., et al. (2003). Interactions between plant hormones regulate submergence-induced shoot elongation in the flooding-tolerant dicot Rumex palustris. Ann. Bot. 91, 205–211. doi: 10.1093/aob/mcf116
Wang, C., Chen, S., Dong, Y., Ren, R., Chen, D., and Chen, X. (2020). Chloroplastic Os3BGlu6 contributes significantly to cellular ABA pools and impacts drought tolerance and photosynthesis in rice. New Phytol. 226, 1042–1054. doi: 10.1111/nph.16416
Wang, X., Deng, Z., Zhang, W., Meng, Z., Chang, X., and Lv, M. (2017). Effect of waterlogging duration at different growth stages on the growth, yield and quality of cotton. PLoS ONE 12:e0169029. doi: 10.1371/journal.pone.0169029
Wang, X., Sakata, K., and Komatsu, S. (2018). An integrated approach of proteomics and computational genetic modification effectiveness analysis to uncover the mechanisms of flood tolerance in soybeans. Int. J. Mol. Ences. 19:1301. doi: 10.3390/ijms19051301
Xie, L.-J., Zhou, Y., Chen, Q.-F., and Xiao, S. (2021). New insights into the role of lipids in plant hypoxia responses. Prog. Lipid Res. 81:101072. doi: 10.1016/j.plipres.2020.101072
Xu, B., Chen, Y., Wang, H., Zhao, W., and Zhou, Z. (2021). Elevated temperature and waterlogging decrease cottonseed quality by altering the accumulation and distribution of carbohydrates, oil and protein. Physiol. Plantarum. 171, 108–124. doi: 10.1111/ppl.13213
Xu, Z. Y., Lee, K. H., Dong, T., Jeong, J. C., Jin, J. B., Kanno, Y., et al. (2012). A vacuolar β-glucosidase homolog that possesses glucose-conjugated abscisic acid hydrolyzing activity plays an important role in osmotic stress responses in Arabidopsis. Plant Cell. 24, 2184–2199. doi: 10.1105/tpc.112.095935
Yang, S.-H., and Choi, D. (2006). Characterization of genes encoding ABA 8'-hydroxylase in ethylene-induced stem growth of deepwater rice (Oryza sativa L.). Biochem. Biophys. Res. Commun. 350, 685–690. doi: 10.1016/j.bbrc.2006.09.098
Yang, X., Jansen, M. J., Zhang, Q., Sergeeva, L., Ligterink, W., Mariani, C., et al. (2018). A disturbed auxin signaling affects adventitious root outgrowth in Solanum dulcamara under complete submergence. J. Plant Physiol. 224–225, 11–18. doi: 10.1016/j.jplph.2018.03.006
Yanjun, Z., Yizhen, C., Hequan, L., Xiangqiang, K., Jianlong, D., Zhenhuai, L., et al. (2016). Growth, lint yield and changes in physiological attributes of cotton under temporal waterlogging. Field Crops Res. 194, 83–93. doi: 10.1016/j.fcr.2016.05.006
Yin, X., and Komatsu, S. (2015). Quantitative proteomics of nuclear phosphoproteins in the root tip of soybean during the initial stages of flooding stress. J. Proteom. 119, 183–195. doi: 10.1016/j.jprot.2015.02.004
Yin, X., Nishimura, M., Hajika, M., and Komatsu, S. (2016). Quantitative proteomics reveals the flooding-tolerance mechanism in mutant and abscisic acid-treated soybean. J. Proteome Res. 15, 2008–2025. doi: 10.1021/acs.jproteome.6b00196
Zhou, W., Chen, F., Luo, X., Dai, Y., Yang, Y., Zheng, C., et al. (2020a). A matter of life and death: Molecular, physiological, and environmental regulation of seed longevity. Plant Cell Environ. 43, 293–302. doi: 10.1111/pce.13666
Zhou, W., Chen, F., Meng, Y., Chandrasekaran, U., Luo, X., Yang, W., et al. (2020b). Plant waterlogging/flooding stress responses: From seed germination to maturation. Plant Physiol. Biochem. 148, 228–236. doi: 10.1016/j.plaphy.2020.01.020
Zhou, W. G., Yang, Y. Z., Zheng, C., Luo, X. F., Chandrasekaran, U., Yin, H., et al. (2021). Flooding represses soybean seed germination by mediating anaerobic respiration, glycometabolism and phytohormones biosynthesis. Environ. Exp. Bot. 188:104491. doi: 10.1016/j.envexpbot.2021.104491
Keywords: ABA, hypoxia, submergence, waterlogging, flooding, ethylene
Citation: Wang Q, Wang L, Chandrasekaran U, Luo X, Zheng C and Shu K (2021) ABA Biosynthesis and Signaling Cascades Under Hypoxia Stress. Front. Plant Sci. 12:661228. doi: 10.3389/fpls.2021.661228
Received: 30 January 2021; Accepted: 28 May 2021;
Published: 24 June 2021.
Edited by:
Miguel Gonzalez-Guzman, University of Jaume I, SpainReviewed by:
Akhtar Ali, Konkuk University, South KoreaJunhua Zhang, Chinese Academy of Agricultural Sciences, China
Copyright © 2021 Wang, Wang, Chandrasekaran, Luo, Zheng and Shu. This is an open-access article distributed under the terms of the Creative Commons Attribution License (CC BY). The use, distribution or reproduction in other forums is permitted, provided the original author(s) and the copyright owner(s) are credited and that the original publication in this journal is cited, in accordance with accepted academic practice. No use, distribution or reproduction is permitted which does not comply with these terms.
*Correspondence: Kai Shu, a3NodUBud3B1LmVkdS5jbg==
†These authors have contributed equally to this work