- 1Department of Biology, University of Pisa, Pisa, Italy
- 2Department of Earth Science, University of Pisa, Pisa, Italy
- 3Centre for Climate Change Impact, University of Pisa, Pisa, Italy
Epiphytes colonizing adult seagrasses highly contribute to seagrass ecosystem functioning and plant growth. Yet, little information exists on epiphytic communities developing on seagrass seedlings. Moreover, for some species our knowledge about seedling performance is limited to early establishment phases, and the role of substrate type in affecting their growth is still unclear. These are considerable knowledge gaps, as seedlings play an important role in meadow expansion and recovery from disturbance. In this study, seedlings of Posidonia oceanica, a keystone species of the Mediterranean, were grown in a shallow (1.5 m deep) coastal area along the Tuscany coast (Italy). After five years of growth (July 2009), seedlings were collected and, through multivariate analysis, we examined whether the epiphytic communities of leaves (both internal and external side) and rhizomes, as well as the growth characteristics differed between rock and sand substrate. The epiphytic communities of seedlings largely reflected those found on adult shoots. Epiphyte cover was similar between the two leaf sides, and it was higher on seedlings grown on rock than on sand, with encrusting algae dominating the community. No differences in epiphyte cover and community structure on rhizomes were found between substrates. Seedling growth characteristics did not differ between substrates, apart from the number of standing leaves being higher on rock than on sand. No correlation was found among epiphyte communities and seedling growth variables (i.e., leaf area, maximum leaf length, number of leaves, total number of leaves produced, rhizome length, total biomass, and root to shoot biomass ratio). Results indicate that epiphytes successfully colonize P. oceanica seedlings, and the surrounding micro-environment (i.e., substrate type) can influence the leaf epiphytic community. This study provides new valuable insights on the biological interactions occurring in seagrass ecosystems and highlights the need for better understanding the effects of seedling epiphytes and substrate on the formation of new meadows.
Introduction
Seagrasses host a variety of epiphytic organisms, from cyanobacteria to macroalgae and invertebrates (Piazzi et al., 2007; Uku et al., 2007; Wilson, 2007), which strongly contribute to meadow productivity and biodiversity, and sustain sediment formation and food web (Boudouresque, 2004; Borowitzka et al., 2006; Michael et al., 2008; Piazzi et al., 2016). Epiphytes can also influence seagrass growth both negatively, competing for light and nutrients (Alcoverro et al., 2004; Borowitzka et al., 2006; Nelson, 2016), and positively, protecting leaves from photo-inhibition and desiccation, and providing nitrogen to plants (Harlin, 1975; Orth and Van Montfrans, 1984). Therefore, identifying the factors structuring epiphyte communities is fundamental for improving our understanding of seagrass ecosystems and establishing more efficient management strategies.
The epiphyte community that develops on adult seagrass plants arises from the combination of multiple biological and environmental factors, including plant growth cycle, light and nutrient availability, hydrodynamic regimes, temperature, and the nature of the substrate (Borowitzka et al., 2006; Michael et al., 2008; Ben Brahim et al., 2014b, 2020; Mabrouk et al., 2014). Since many algal epiphytes preferentially grow under high light availability conditions (Borowitzka et al., 2006), they are generally more abundant in plants growing in shallow than in deep meadows (Pinckney and Micheli, 1998; Rindi et al., 1999) and on the external leaf side of older leaves (Trautman and Borowitzka, 1999; Piazzi et al., 2016). Furthermore, species such as Posidonia oceanica L. Delile harbor higher epiphytic abundance and diversity in summer than in winter (Piazzi et al., 2016). Also, substrate type can strongly influence the epiphyte recruitment on seagrasses because rocky substrates provide a larger number of propagules (Van Elven et al., 2004; Borowitzka et al., 2006). Indeed, Thalassodendron ciliatum (Forsk.) den Hartog and P. oceanica harbor a higher epiphyte abundance on plants growing on hard substrates in comparison to soft bottoms (Bandeira, 2002; Piazzi et al., 2016), although for the latter this applies to rhizomes (Piazzi et al., 2016) but not leaves (Giovannetti et al., 2008).
Surprisingly, our knowledge of seagrass epiphytes is based on adult plants, but very little is known about the structure of epiphyte communities on seedlings. Available data are currently limited to 6-month old P. oceanica seedlings and mainly concerned the effects of a species-specific bacterium on epiphyte recruitment (Celdran et al., 2012). Thus, it is largely unknown whether other factors structuring the epiphyte communities of adult plants (e.g., substrate type) are also acting at the seedling stage. This is a relevant gap in knowledge considering the critical role that sexual recruitment plays in the maintenance and expansion of seagrass meadows (Balestri and Lardicci, 2008; Kendrick et al., 2012; Tan et al., 2020; Vanderklift et al., 2020).
Posidonia oceanica is an endemic, keystone seagrass of the Mediterranean Sea that forms extensive meadows on a variety of substrates (e.g., sand and rock) up to 45 m depth (Procaccini et al., 2003). This species provides important ecosystem services (e.g., coastal protection and nursery for fish; Campagne et al., 2015), and support a highly diverse epiphytic community (more than 600 species; Mazzella et al., 1989, 1992; Piazzi et al., 2016). Unfortunately, P. oceanica meadows are declining in the entire Mediterranean basin (Telesca et al., 2015) and, therefore, there is a strong interest in improving the conservation status of existing meadows.
Recent studies have shown that recruitment by seeds play an important role in the colonization of new sites, maintenance of genetic diversity, and for meadows recovery after disturbance (Balestri and Vallerini, 2003; Balestri and Lardicci, 2008; Alagna et al., 2013; Balestri et al., 2017). Furthermore, seedlings are a promising plant material for restoration interventions (Balestri et al., 1998; Terrados et al., 2013). Naturally established seedlings have been found in sheltered, shallower areas (<3 m depth) on different substrate types, preferentially on rock and sand substrate (Balestri et al., 2017; Pereda-Briones et al., 2020). Substrate type could influence seedlings development, as suggested by experimental studies where seedlings preferentially invested on shoots or roots depending if they grew on hard or unconsolidated substrate (i.e., rock and sand; Alagna et al., 2013, 2015). However, all available data on seedling performance are restricted to their first three years of life (Balestri et al., 1998; Balestri and Bertini, 2003; Alagna et al., 2013) and no data are available about their epiphytic community at this stage.
Here, we investigated the epiphytic community of leaves and rhizomes of P. oceanica seedlings grown for five years in a shallow site on two substrate types (rock vs. sand). We also examined seedling growth rate and biomass allocation. We hypothesized that seedlings grown on rock and sand differed in both total epiphyte cover and community structure, as well as in growth pattern. Furthermore, previous studies conducted on P. oceanica adult shoots have shown differences in epiphyte cover between the internal and the external leaf sides (Montefalcone et al., 2006), therefore, we hypothesized that the same epiphyte distribution pattern may occur on leaves of seedlings.
Materials and Methods
Experimental Procedure
Seedlings of P. oceanica were harvested five years after their transplanting at 1.5 m depth in a sheltered site in the Ligurian Sea (Livorno, Italy, 43° 29′ N, 10°19′ E; Supplementary Figure 1), near to a Cymodocea nodosa bed; no adult plants of P. oceanica were present close to transplanted seedlings (the closest P. oceanica meadow was >5 m deep). The substrate consisted of rock and patches of medium-fine calcareous sand (Balestri et al., 2015). The transplantation procedure has been described in a previous study (Balestri et al., 2015). Briefly, P. oceanica fruits were collected on a beach near Livorno (Italy) in June 2004, and seeds were extracted from fruits and placed in plastic boxes floating in an aquaculture tank equipped for seagrass culture (Balestri and Lardicci, 2012; Balestri et al., 2017). After one month, seedlings were transferred to the transplanting site where they were individually planted in rock fissures and sand patches; rock and sand patches were approximately 1–5 m apart, while the distance between seedlings was at least 50 cm. Rocks were colonized by turf species mainly consisted in filamentous algae, while sand patches were bare (Balestri et al., 2015). In July 2009, five seedlings (Figure 1) grown on rock and five seedlings grown on sand were carefully collected by hand and transported to the laboratory for plant phenology and epiphyte community analyses.
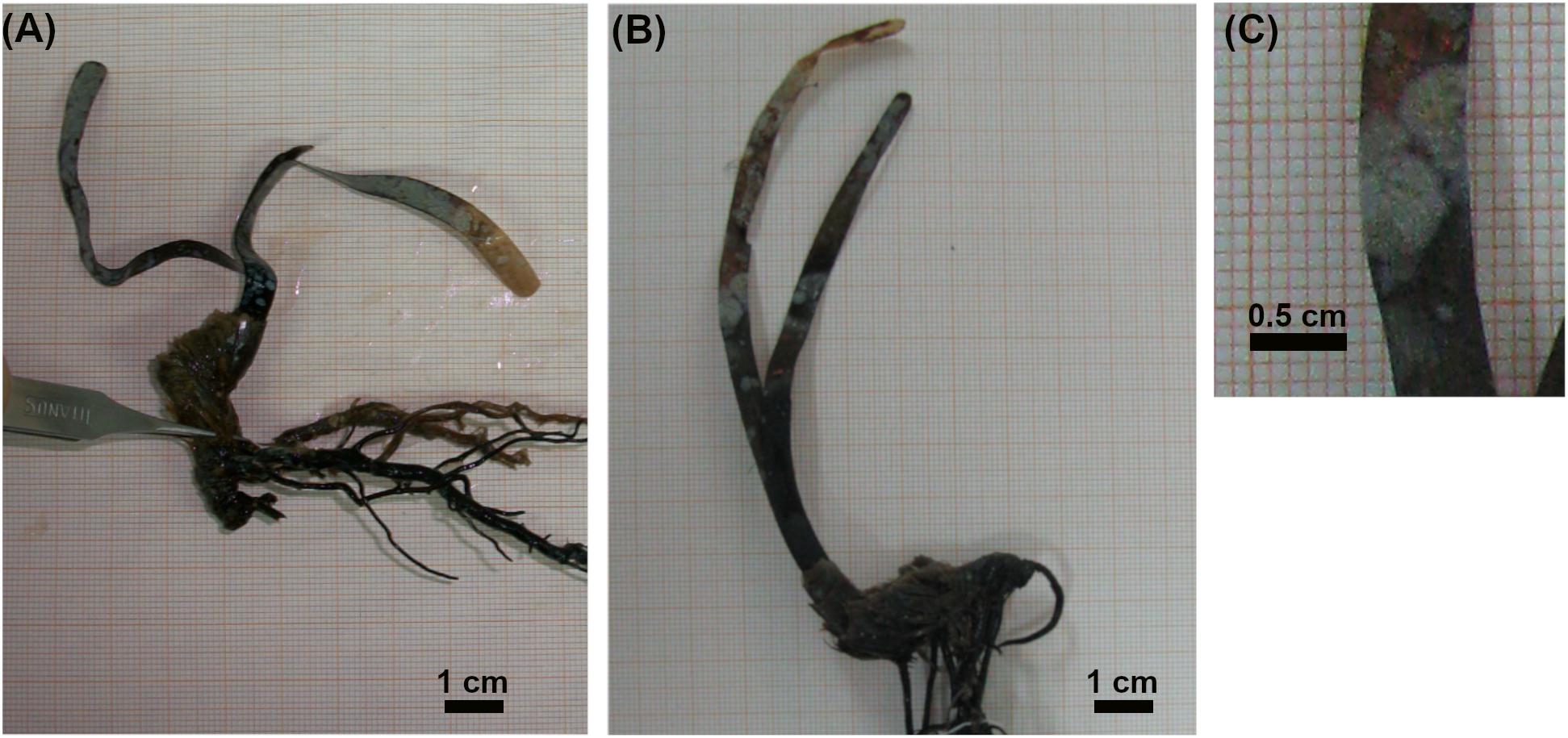
Figure 1. Images of five-year old Posidonia oceanica seedlings grown on rock (A) or sand (B) substrate, and of epiphytes grown on leaves (C).
Seedling Growth Variables and Epiphyte Characterization
Collected seedlings were washed from sediment and separated into aboveground structures (leaves, leaf sheaths, and rhizome) and belowground structure (roots). The two oldest leaves of each seedling were used for leaf epiphyte community characterization. Both the internal and external sides of each leaf were visually examined, in entirety, under a stereomicroscope equipped with a micrometer (Balata et al., 2007; Giovannetti et al., 2010; Mabrouk et al., 2017). Organisms (invertebrates and algae) were identified at species level when possible, otherwise higher taxonomical categories were used. To evaluate the abundance of each organism, the surface area (cm2) covered by each organism in orthogonal projection on the leaf was recorded (Pardi et al., 2006). Afterward, for each organism we calculated the percentage area covered with respect to total leaf area (Balata et al., 2010; Mabrouk et al., 2017). Similarly, the epiphyte community present on the rhizome of each seedling was examined. Total epiphyte percentage cover on rhizome was calculated by summing the percentage cover of each organism found on each of the two sides of the rhizome. Furthermore, for both leaves and rhizomes algae were grouped into morphological groups according to the classification of Steneck and Dethier (1994).
Then, for each seedling we counted the number of standing leaves, the length of the longest leaf, and the number of rhizome internodes. The total number of leaves produced by each seedling during its life span was obtained by summing the number of internodes and the number of standing leaves. For each seedling, the length and the width of standing leaves were measured using a ruler; leaf area was calculated multiplying leaf length by width and summing the areas of all leaves (Drew and Jupp, 1976). Rhizome length of each seedling was measured using a binocular stereomicroscope equipped with a micrometer. All separated seedling portions were oven-dried at 70°C to constant weight and weighted. Total plant biomass was determined by summing the biomass of above- and belowground portions, and the root to shoot biomass ratio was also calculated.
Statistical Analysis
A mixed-effect model (lme4 package; Bates et al., 2015) was used to test for differences in total leaf epiphyte cover between substrate types and leaf surface sides. In this model, total epiphyte cover was included as the response variable and substrate type (two levels: rock and sand) and leaf side (two levels: internal or external) as the predictor variables; shoot was considered as a random factor nested within substrate type to account for the non-independence of the two sides of the individual leaf. A two-sided t-test was used to investigate whether total epiphyte percentage cover on rhizomes differed between substrate types, i.e., rock and sand.
We used the multivariate analysis of variance based on permutations (PERMANOVA; Anderson, 2001) to test for differences between substrates in the epiphyte percentage cover on rhizomes and leaves, as well as in the percentage cover of algal morphological groups on leaves. Data from both sides of leaves were averaged since no differences were found in total epiphyte cover between the two leaves. PERMANOVAs was performed using the adonis function in the vegan package (Oksanen et al., 2019), setting 999 permutations for the test at an α-level of 0.05.
To visualize the epiphyte community composition on leaves and rhizomes of P. oceanica grown on the two substrates we used a two-dimensional n-MDS ordination (non-metric multidimensional scaling; Clarke, 1993). SIMPER analysis was used for identifying which organism or morphological group primarily accounted for observed differences in epiphytic assemblages between substrate types.
Separate two-sided t-tests were used to test for differences in growth characteristics of seedlings grown on the two substrate types. We used two-sided t-tests because of the scarcity of information on epiphytes growing on seedlings. Lastly, the RELATE procedure (PRIMER v.7) was used to assess possible correlations between seedling growth variables and leaf epiphyte cover. Separate analyses were performed for seedlings grown on sand and rock substrate. In this procedure, the matrix of similarities between epiphytic species abundance (based on the Bray Curtis coefficient from four root transformed data) was compared with a matrix of the similarities between morphological seedling variables (based on Euclidean distance from normalized data), and the significance of any correlation between the matrices was computed with a randomization test.
Before performing the analyses, Shapiro–Wilk and Levene’s test were used to test for assumptions of normality and homogeneity of variance. In the mixed-effect model, the response variable was square root transformed to meet model assumption. In PERMANOVA, a Bray–Curtis dissimilarity matrix (Bray and Curtis, 1957) calculated from fourth root transformed data was used to meet the assumption of homogeneity of variance. The permutest function (vegan package) was used to check for between groups dispersion. For morphological groups in rhizomes, a constant of 1 was added to avoid NAs generation. All plots were made using the ggplot2 package (Wickham, 2016) and all analysis were performed in R (R Core Team, 2018) except for the RELATE procedure that was performed in PRIMER (Anderson et al., 2008; Clarke and Gorley, 2015).
Results
In total, 13 species of macroalgae (eight Rhodophyta, three Heterokontophyta, and two Chlorophyta) and five taxa of invertebrates (two Hydroidea, one Polychaeta, one Bryozoan, and one Foraminifera; Table 1) were identified on leaves and rhizomes of P. oceanica seedlings. Seedlings from the two substrates shared only few taxa or species: encrusting algae, Chondria dasyphylla, Sphacelaria cirrosa, foraminifera, and Spirorbis spp. (Table 1). Encrusting algae comprised species belonging to the Corallinaceae family such as Pneophyllum fragile, Hydrolithon farinosum, Hydrolithon cruciatum, and Hydrolithon boreale. Exclusives of seedlings from rock were Chondria mairei, Dictyota fasciola, Herposiphonia tenella, Vertebrata subulifera, and Obelia caniculata, whereas Ceramium sp., Herposiphonia secunda, Polysiphonia sp., Dictyota mediterranea, and Orthopyxis caniculata were present only on seedlings from sand (Table 1).
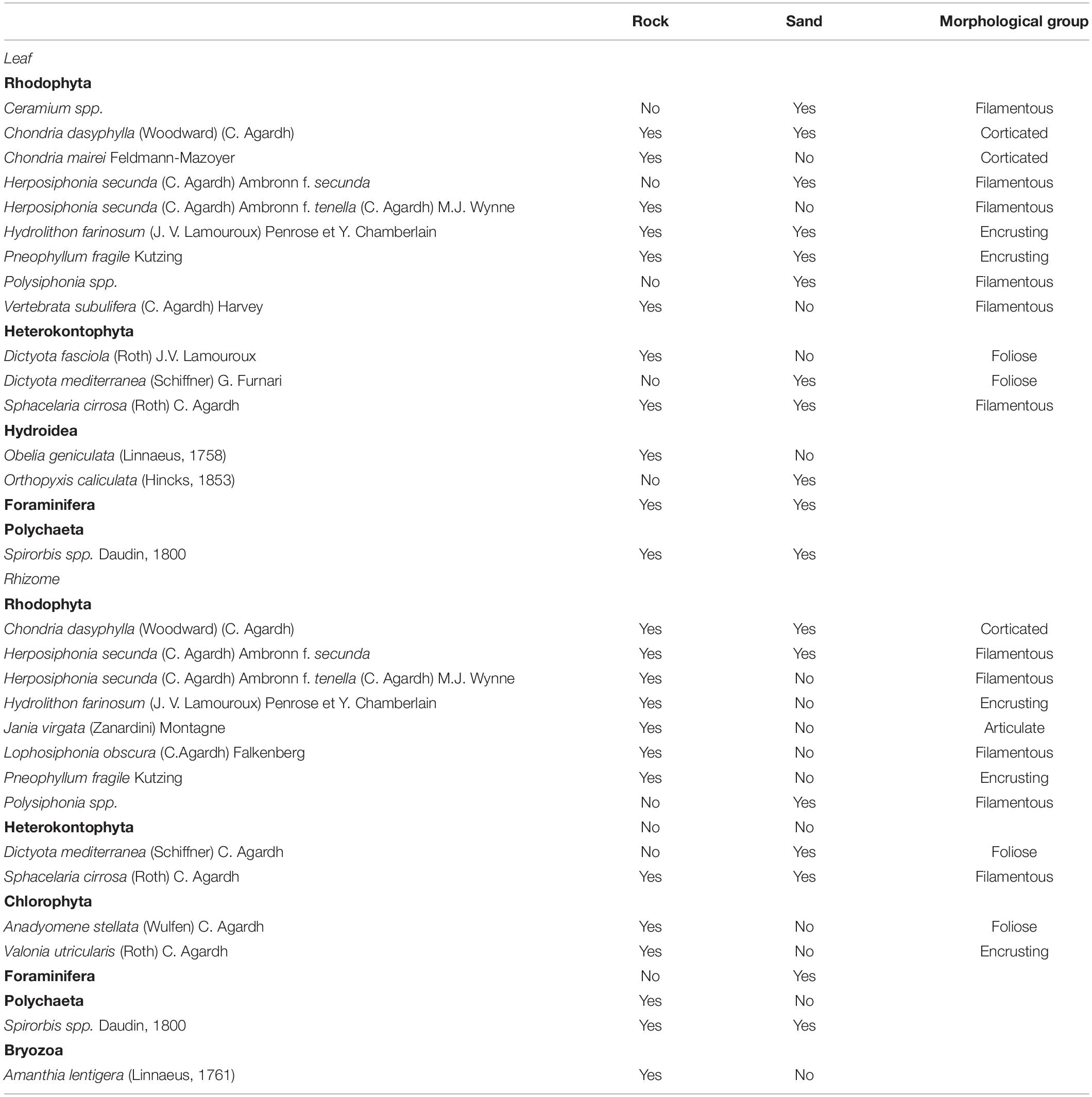
Table 1. Species and taxa found on leaves and rhizomes of five-year old Posidonia oceanica seedlings growing on rock or sand substrate.
No significant differences in the total leaf epiphyte cover between the internal and external side of leaves were found (Table 2). Substrate type significantly affected leaf epiphyte cover (Table 2 and Figure 2A); mean percentage cover was higher on rock (57.2% ± 8.5, mean ± SE) than on sand (13.4% ± 2.6 SE). Encrusting algae dominated the epiphyte assemblages on leaves in both substrates type, followed by V. subulifera and C. dasyphylla (Figure 2C).

Table 2. Mixed-effect model results of the effect of substrate type (two levels, rock and sand) and leaf side (two levels, internal and external) on total leaf epiphyte cover (expressed in percentage) on five-year old seedlings of Posidonia oceanica.
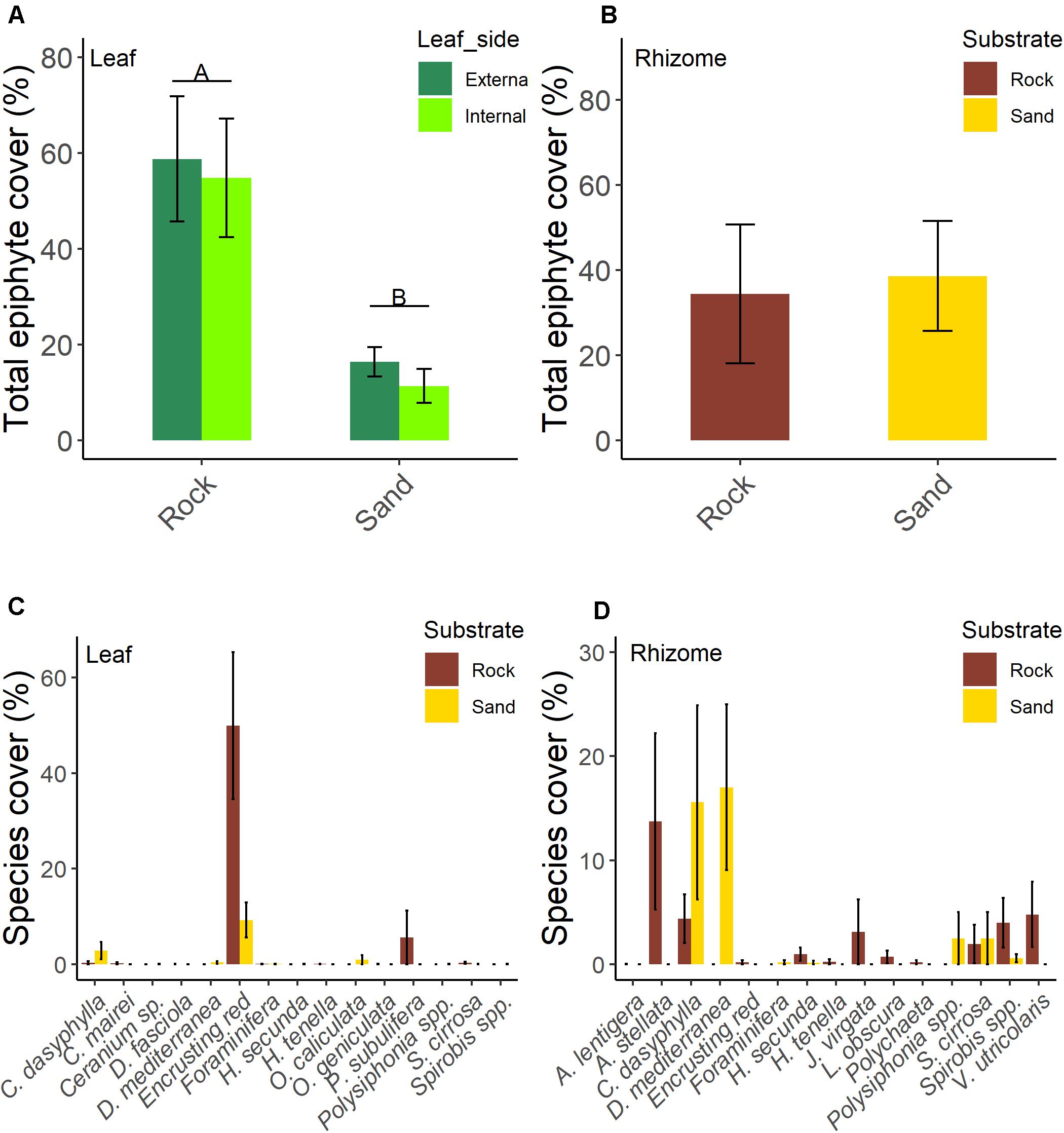
Figure 2. Total epiphytic percentage cover of the two sides of leaves (A) and rhizomes (B) and epiphytic percentage cover of organisms of leaves [data are averaged for leaf sides; (C)] and rhizomes (D) of five-year old seedlings of Posidonia oceanica grown on rock and sand substrate. Letters above bars denote significant differences between treatments at α < 0.01. Data are means ± SE; n = 5.
For rhizomes, the total cover of epiphytes was similar (t = −0.201, df = 8, p = 0.846; Figure 2B) between rock (34.4% ± 16.4 SE) and sand (38.6% ± 12.9 SE). The most represented species or taxa on rhizomes (Figure 2D) were Anadyomene stellata, C. dasyphylla, D. mediterranea, Spirobis spp., Polysiphonia spp., S. cirrosa, and Valonia utricolaris.
Multivariate analysis detected a significant effect of substrate type on the leaf epiphyte community (data were averaged for leaf sides; Table 3) which was also shown by the n-MDS configuration of the two communities (Figure 3A). SIMPER analysis showed that encrusting algae contributed the most to differences in communities of leaves between substrates (74%), followed by V. subulifera (14%), and C. dasyphylla (6%; Table 4). However, no significant difference in total epiphyte percentage cover on rhizomes between the two substrates was found (Table 3). Indeed, n-MDS configuration shows an overlap between the epiphyte communities of rhizomes from the two substrate types (Figure 3B).
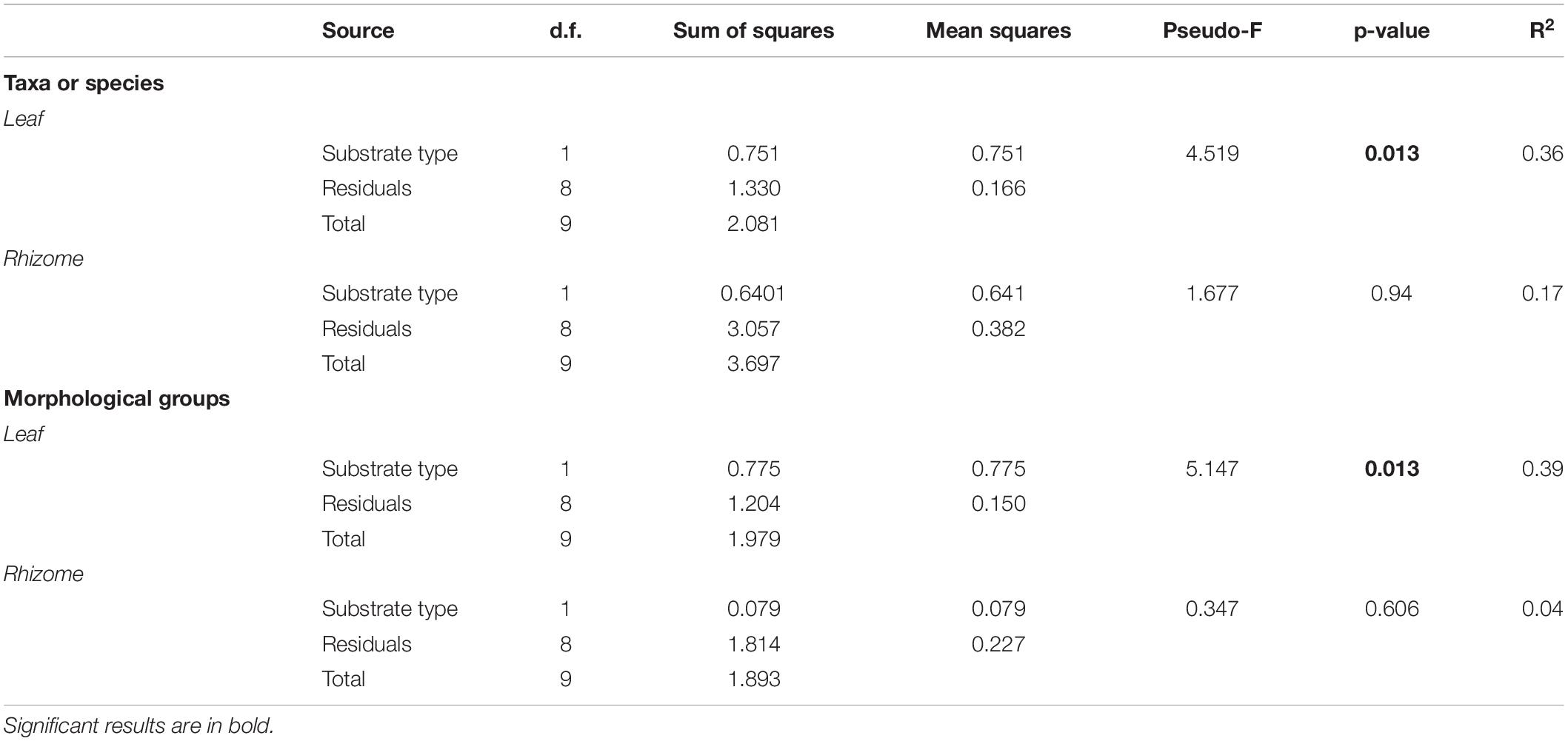
Table 3. Results of multivariate analysis of the epiphyte community (at the level of taxa and algal morphological groups) on leaves and rhizomes of five-year old Posidonia oceanica seedlings grown on rock and sand substrates.
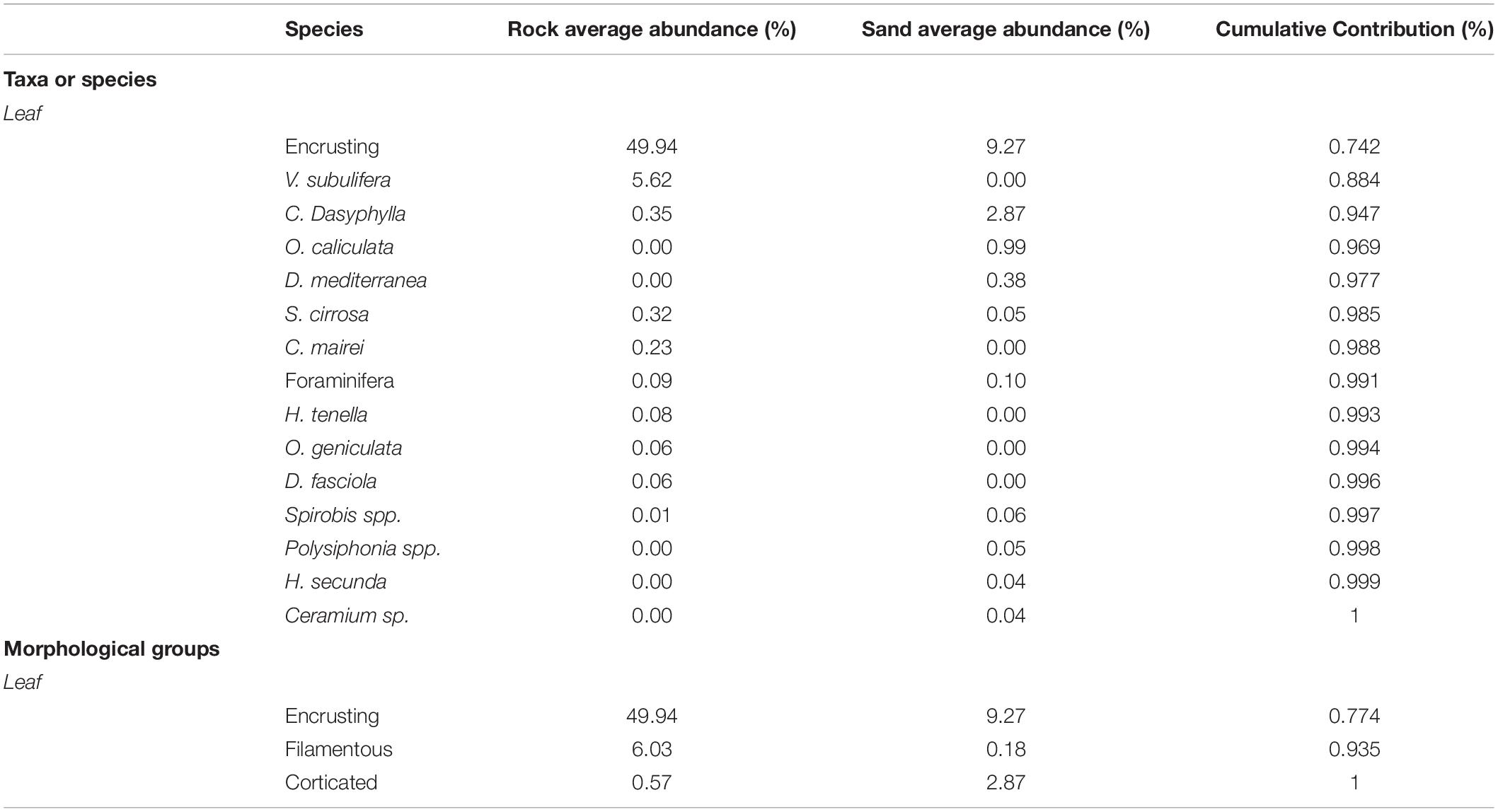
Table 4. Results of Simper analysis showing the taxa and species, and algal morphological groups most contributing to the multivariate pattern of the percentage cover of epiphytes on leaves of five-year old Posidonia oceanica seedlings grown on rock and sand substrate.
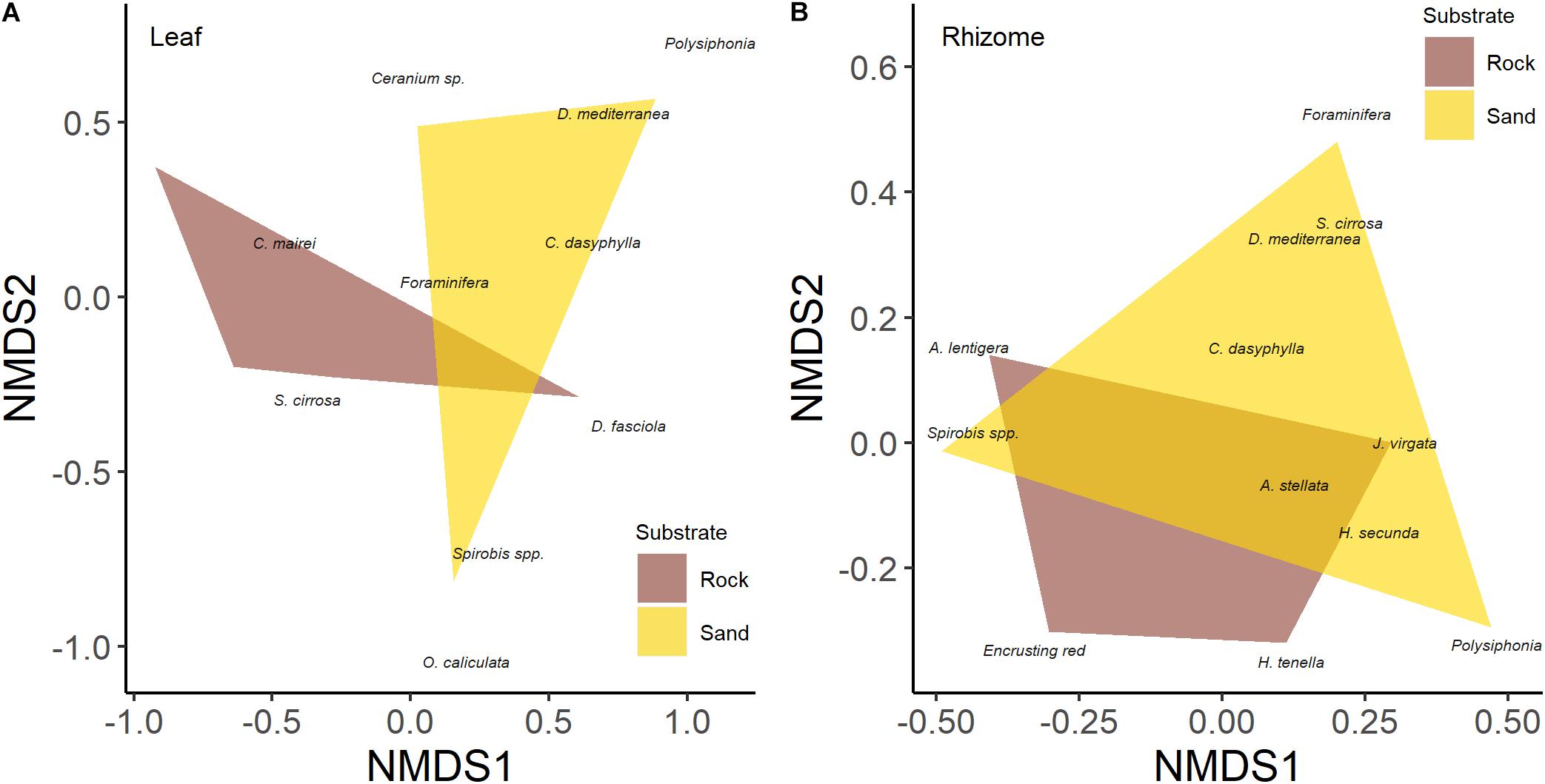
Figure 3. Differences in epiphyte community of leaves (A) and rhizomes (B) of five-year-old seedlings of Posidonia oceanica grown on rock and sand substrate type based on n-MDS classification.
Multivariate analysis performed on the percentage cover of algal morphological groups confirmed that the structure of the leaf epiphyte community on rock was statistically different from that on sand (Table 3). Simper analysis showed that encrusting algae accounted for most of the differences observed between the two communities (76%; Table 4).
Seedlings showed a rhizome with plagiotropic growth orientation bearing generally one shoot. However, up to three and two aborted shoots were observed on seedlings grown on rock and sand respectively. For most variables (Figure 4), no significant differences were detected among seedlings grown on rock and sand (leaf length, t = −0.234, df = 8, p = 0.814; leaf area, t = −1.801, df = 8, p = 0.109; total number of leaves produced during the life span, t = −0.476, df = 8, p = 0.647; rhizome length, t = 0.119, df = 8, p = 0.908; total plant biomass, t = −1.205, df = 8, p = 0.263; root to shoot biomass ratio, t = 0.412, df = 8, p = 0.691). However, seedlings on rock had more standing leaves than those on sand (t = −2.333, df = 8, p = 0.048): this difference was mainly due to a higher number of intermediate leaves. No correlation between epiphytic leaf cover and seedling growth variables was detected by RELATE procedure (Rock: Rho = −0.418, p = 0.843; Sand: Rho = −0.152 p = 0.686).
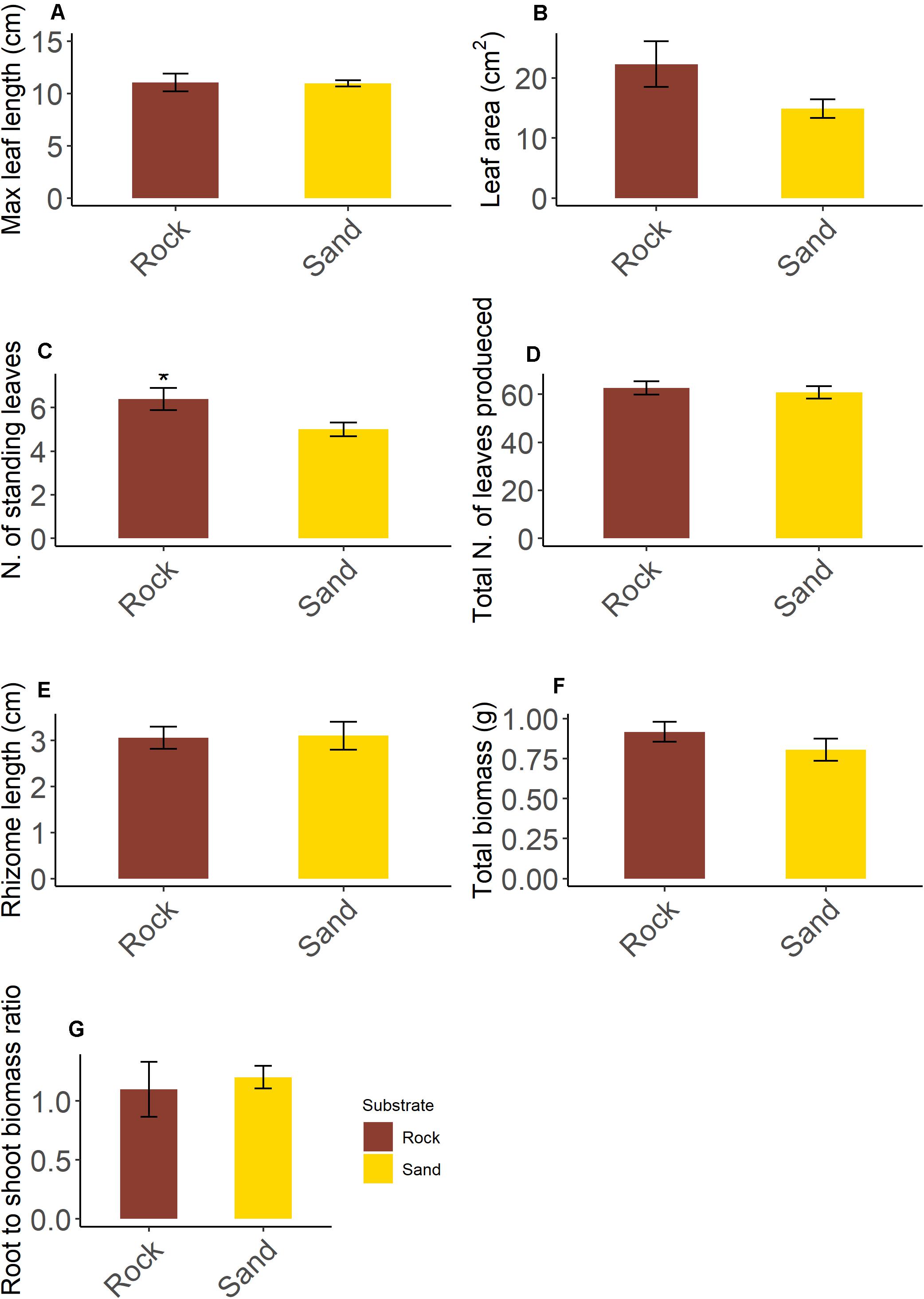
Figure 4. Morphological and growth variables of five-year old Posidonia oceanica seedlings grown on rock and sand substrate (A–G). Asterisk indicates a significant statistical difference at α < 0.05. Data are means ± SE; n = 5.
Discussion
Our results support the hypothesis that seedlings grown on rock and sand differed in epiphyte cover and community structure, but only for leaves and not for rhizomes. Contrary to expectations, we did not observe differences in epiphyte abundance between the two sides of leaves. Also, no substantial differences in growth characteristics were found among seedlings grown on rock and sand, apart from the number of standing leaves. No significant correlation was detected among plant variables and epiphyte communities.
Epiphyte Community of Leaves and Rhizomes of Seedlings
In total, we found a number of epiphytic organisms growing on P. oceanica seedlings within the range of that reported in previous studies (range: 17–71; Supplementary Table 1 and references therein) on adult shoots collected in the same season (summer) and at similar depths (<5 m; Supplementary Table 1) to that of our study. Encrusting algae were the most abundant group present on the leaves of seedlings (contributing with more than 70% in cover on average), with P. fragile and H. farinosum as the main species belonging to this group. This is in accordance with previous studies on leaves of P. oceanica shoots (Supplementary Table 1) showing that encrusting algae was the most abundant group regardless of geographical area (e.g., Western and Eastern Mediterranean; Pardi et al., 2006; Ben Brahim et al., 2014b; Piazzi et al., 2016), plant age (e.g., seedling and adult plants; Celdran et al., 2012; Piazzi et al., 2016), and depth (e.g., shallow and deep meadows; Tsirika et al., 2007; Ben Brahim et al., 2014a; Piazzi et al., 2016). Encrusting algae have been also observed in one-year old P. oceanica seedlings (Celdran et al., 2012). It seems that the recruitment and growth of these algae on P. oceanica seedlings may be facilitated by the colonization of leaves by Marinomonas posidonica, a bacterium that exclusively grows on this species (Celdran et al., 2012).
We also found that epiphytes colonizing rhizomes of seedlings had an overall lower cover with respect to leaves, but no single taxon dominated the community as in leaves. Indeed, Clorophyta, Heterokontophyta, and Bryozoans were well represented on rhizomes while encrusting algae, Porifera, and Tunicata were absent. This finding is partially in accordance with that of previous studies on rhizomes of adult P. oceanica (Piazzi et al., 2016; Supplementary Table 1). The higher species diversity on rhizomes of adult plants (Piazzi et al., 2016) has been related to a more stable and sheltered environment offered by rhizomes to epiphytes that could allow the development of slow-growing but longer-living organisms.
In adult plants, epiphytes collected in summer at shallow depths (4–5 m) preferentially grow either on the external leaf side (Montefalcone et al., 2006) or the internal side (Peirano et al., 2011). These differences in epiphyte composition and abundance between the two leaf sides have been related to the shape and orientation of leaves in adult shoots (Van der Ben, 1971; Alcoverro et al., 2004; Peirano et al., 2011) and to the different light incidence and degree of exposure of each leaf side to hydrodynamics (Casola and Scardi, 1989; Tursi et al., 2001; Ben Brahim et al., 2014b; Piazzi et al., 2016). In our study, similarly to adult plants leaves of five-years-old seedlings showed the internal side concave and the external side convex. Yet, in contrast to adult plants we found a lack of preferential distribution of epiphytes between leaf sides. This contrasting result might be explained by the curvilinear shape and reduced length of leaves of seedlings with respect to adult leaves (i.e., tens of centimeters vs. 1 m, respectively; Boudouresque and Meinesz, 1982), which could lead to a similar degree of exposure of external and internal leaf sides to light intensity and hydrodynamics. Moreover, adult P. oceanica plants form densely packed shoots in which the internal leaf side can be less exposed to light and nutrients than the external one (Montefalcone et al., 2006; Piazzi et al., 2016). Thus, since in our study seedlings grew as a single plant bearing only one shoot, the external and internal leaf sides were probably similarly exposed to light and nutrients possibly explaining the lack of differences in epiphytes growth between the two leaf sides. Furthermore, in adult plants Briozoan, and Electra posidoniae especially, which account for a large portion of leaf epiphyte cover (Peirano et al., 2011; Lepoint et al., 2014a), are more abundant on the internal leaf side (Lepoint et al., 2014b). Surprisingly, however, in our study Bryozoan were absent on the leaves of seedlings, and only one species was found on rhizomes (Amanthia lentigera). This might further explain the similarity in epiphyte cover between leaf sides observed here in seedlings.
Bryozoan is one of the most represented epiphyte taxa found in adult plants, accounting for up to 15% of all epiphyte biomass (Piazzi et al., 2016). In particular, the bryozoan E. posidoniae grows exclusively on P. oceanica, and it is by far the most common species colonizing leaves of adult plants (up to 60% of all Bryozoan; Lepoint et al., 2014a,b). Thus, the absence of this species on leaves of seedlings found in our study is strikingly. However, the presence of Bryozoan on P. oceanica leaves is tightly associated with seasonal blooms of microphytoplancton (e.g., diatoms) in the water column, on which Bryozoans feed (Lepoint et al., 2014a,b). Both Bryozoan abundance and diversity increase in late winter–early spring, when food sources are abundant, and they strongly decline in summer due to food shortage (Lepoint et al., 2014a,b). In addition, Bryozoan follow a depth distribution gradient, with higher abundance and diversity on plants at deeper meadows (more than 10 m; Lepoint et al., 2014a,b) than the seedlings used in our study. Therefore, in this study the lack of Bryozoan on seedlings could be related to both the time of sampling (July) and habitat depth (less than 2 m).
Effects of Substrate Type on the Epiphyte Community of Seedlings
The total leaf epiphyte cover we found on seedlings is in accordance with that of adult plants (60–80%; Pardi et al., 2006). Yet, we found higher total epiphyte cover on seedlings grown on rock (60%) than on sand (13%) substrate, which disagrees with studies on adult plants (Giovannetti et al., 2008). Thus, our findings support the hypothesis that the nature of substrate or microhabitat conditions can drive the development of the seedling epiphyte community, as shown for the seagrasses Thalassodendrum ciliatum and Posidonia sinuosa (Cambridge and Kuo) which harbored a higher leaf epiphyte cover on plants growing on rock or closer to reef banks (Bandeira, 2002; Van Elven et al., 2004). Furthermore, in our study the difference observed in the leaf epiphyte community between seedlings that grew on rock and sand was mainly related to the higher presence of encrusting algae on rock. An experimental study on six-months old P. oceanica seedlings showed that the bacterium M. posidonica, which exclusively grows on P. oceanica, facilitated the recruitment and growth of encrusting algae (Celdran et al., 2012). Therefore, it is possible that the rock substrate not only provides for more propagules for algae recruitment, but it might also facilitate the development of specific bacteria capable to shape the epiphyte community.
In rhizomes, however, we did not detect any difference in epiphyte cover and community structure on seedlings between substrate types. This finding contrasts with studies which indicate that hard substrates enhance epiphyte richness on plants both for seagrass in general (Bandeira, 2002; Van Elven et al., 2004) and in particular for P. oceanica adult rhizomes (Eugene, 1979; Pessani et al., 1989). Rhizomes of seedlings were much smaller than those of adult plants and thus, the less space available for colonization might have prevented the development of different communities between substrates. More studies are needed to fully elucidate the mechanisms by which rocky substrates influence epiphyte growth on P. oceanica seedlings.
Growth Characteristics of Seedlings
Our study shows that seedlings had produced approximately 60 leaves in five years. This corresponds to an average leaf production rate of about 12 leaves per year. This finding is in accordance with the leaf production rate previously observed in one-year old seedlings grown in natural environment (15 leaves per year; Balestri and Lardicci, 2008) and in aquarium (12–14 leaves per year; Alagna et al., 2015). Interestingly, the number of standing leaves seems not to depend on the period of observation or the growing conditions (Balestri and Bertini, 2003; Balestri and Lardicci, 2008; Balestri et al., 2009; Alagna et al., 2013, 2015) as it remains fairly constant at least up to five years (in this study). The higher number of standing leaves of seedlings grown on rock suggests that, at least during the period of observation, the leaf turnover could differ due to variations in micro-habitat conditions. Yet, the number of standing leaves were lower than that observed in adult shoots at 5 m depth, ranging from 6 to 10 per shoot (Buia et al., 1992).
In this study, seedling rhizomes slowly grew in five years, reaching approximately 3 cm in length which corresponds to an average elongation rate of 0.6 cm per year. This is in contrast with previous studies on P. oceanica seedlings reporting a rhizome length of 3 cm after the first year and three years of growth (Balestri et al., 1998, 2009). In adult P. oceanica plants rhizomes can grow up to 6 cm per year (Marbá and Duarte, 1998), which is an order of magnitude higher than what we found in this study. This is because adult plants can benefit from clonal integration which provides them the necessary resources for sustaining such a high growth rate (Ruocco et al., 2021).
Contrary to expectations, seedlings grown on rock and sand showed similar growth characteristics, except for the number of standing leaves. This finding contrasts with previous studies showing both higher leaf and rhizome length in one-year old P. oceanica seedlings growing on rock than on sand (Alagna et al., 2015, 2020). However, these studies were conducted in aquaria and on very short temporal scales. It is plausible that during their first year, seedlings on sand invest more biomass in roots at the expense of shoots to avoid uprooting (Infantes et al., 2011; Alagna et al., 2015). Instead, in our study the root to shoot biomass ratio of seedlings had approximately a value of one, indicating that they had equally invested on shoots and roots regardless of substrate type. In addition, in natural habitats other factors (e.g., light and nutrients) might have interacted with substrate in influencing seedling development.
Conclusion
Seagrass epiphytes have been widely studied but virtually nothing is known about the factors driving the recruitment and structure of epiphyte communities on seedlings. The present study provides valuable insights on the epiphytic community on five-year old of seedlings of P. oceanica grown on different types of micro-habitat (rock fissures and sand patches). It also provides new data on the growth potential of P. oceanica seedlings. Specifically, we showed that despite their small size, P. oceanica seedlings hosted an epiphyte community relatively well-structured and largely reflecting that of adult plants, although with some notable differences, i.e., the absence of Bryozoans, Porifera, and Tunicata, and lack of a preferential distribution on the external leaf side. Interestingly, our study suggests that micro-habitat (i.e., substrate type) can influence the structure and cover of leaf epiphyte communities on seedlings, but it does not substantially affect seedling growth and biomass allocation. Furthermore, the lack of correlation between plant growth variables and epiphyte communities across substrates emerged from this study suggests that plants characteristics are not strongly involved in shaping the epiphyte communities. Overall, these findings increase our understanding of the biological interactions occurring during the most critical life history stage of this seagrass. Considering the importance that seagrass seedlings may play in colonization, recovery after disturbances, as well as in restoration efforts, further studies are needed to elucidate possible positive or negative effects of epiphyte cover on seedling growth; for instance, through the transfer of or competition for nutrients or the increase in drag forces on seedlings leading to higher dislodgement.
Data Availability Statement
The raw data supporting the conclusions of this article will be made available by the authors, without undue reservation.
Author Contributions
DDB performed seedling morphological characterization and statistical analyses, interpreted results, and wrote the manuscript. EB designed the study, supervised the experiment, performed seedling morphological characterization and statistical analyses, interpreted the results, and wrote the manuscript. GP performed seedling epiphyte characterization and contributed to wrote the manuscript. VM contributed to statistical analyses and to wrote the manuscript. CL designed the study, supervised the experiment, and revised the manuscript. All authors read and approved the final version of the manuscript.
Funding
This research was supported by Fondi di Ateneo (FA) and Progetti di Ricerca di Ateneo (PRA) of University of Pisa (Italy).
Conflict of Interest
The authors declare that the research was conducted in the absence of any commercial or financial relationships that could be construed as a potential conflict of interest.
Acknowledgments
We sincerely thank Flavia Vallerini, Ph.D. for her valuable help in collecting P. oceanica seedlings in the field.
Supplementary Material
The Supplementary Material for this article can be found online at: https://www.frontiersin.org/articles/10.3389/fpls.2021.660658/full#supplementary-material
References
Alagna, A., Fernández, T. V., Anna, G. D., Magliola, C., Mazzola, S., and Badalamenti, F. (2015). Assessing Posidonia oceanica seedling substrate preference: an experimental determination of seedling anchorage success in rocky vs. sandy substrates. PLoS One 10:e0125321. doi: 10.1371/journal.pone.0125321
Alagna, A., Fernández, T. V., Terlizzi, A., and Badalamenti, F. (2013). Influence of microhabitat on seedling survival and growth of the mediterranean seagrass posidonia oceanica (l.) Delile. Estuar. Coast. Shelf Sci. 119, 119–125. doi: 10.1016/j.ecss.2013.01.009
Alagna, A., Zenone, A., and Badalamenti, F. (2020). The perfect microsite: how to maximize Posidonia oceanica seedling settlement success for restoration purposes using ecological knowledge. Mar. Environ. Res. 161:104846. doi: 10.1016/j.marenvres.2019.104846
Alcoverro, T., Pérez, M., and Romero, J. (2004). Importance of within-shoot epiphyte distribution for the carbon budget of seagrasses: the example of Posidonia oceanica. Bot. Mar. 47, 307–312. doi: 10.1515/BOT.2004.036
Anderson, M. J. (2001). A new method for non-parametric multivariate analysis of variance. Austral. Ecol. 26, 32–46. doi: 10.1111/j.1442-9993.2001.01070.pp.x
Anderson, M. J., Gorley, R. N., and Clarke, K. R. (2008). Permanova for Primer: Guide to Software and Statistical Methods. Plymouth, UK: PRIMER-E.
Balata, D., Nesti, U., Piazzi, L., and Cinelli, F. (2007). Patterns of spatial variability of seagrass epiphytes in the north-west Mediterranean Sea. Mar. Biol. 151, 2025–2035. doi: 10.1007/s00227-006-0559-y
Balata, D., Piazzi, L., Nesti, U., Bulleri, F., and Bertocci, I. (2010). Effects of enhanced loads of nutrients on epiphytes on leaves and rhizomes of Posidonia oceanica. J. Sea Res. 63, 173–179. doi: 10.1016/j.seares.2009.12.001
Balestri, E., and Bertini, S. (2003). Growth and development of Posidonia oceanica seedlings treated with plant growth regulators: possible implications for meadow restoration. Aquat. Bot. 76, 291–297. doi: 10.1016/S0304-3770(03)00074-3
Balestri, E., de Battisti, D., Vallerini, F., and Lardicci, C. (2015). First evidence of root morphological and architectural variations in young Posidonia oceanica plants colonizing different substrate typologies. Estuar. Coast. Shelf Sci. 154, 205–213. doi: 10.1016/j.ecss.2015.01.002
Balestri, E., Gobert, S., Lepoint, G., and Lardicci, C. (2009). Seed nutrient content and nutritional status of Posidonia oceanica seedlings in the northwestern Mediterranean Sea. Mar. Ecol. Prog. Ser. 388, 99–109. doi: 10.3354/meps08104
Balestri, E., and Lardicci, C. (2008). First evidence of a massive recruitment event in Posidonia oceanica: spatial variation in first-year seedling abundance on a heterogeneous substrate. Estuar. Coast. Shelf Sci. 76, 634–641. doi: 10.1016/j.ecss.2007.07.048
Balestri, E., and Lardicci, C. (2012). Nursery-propagated plants from seed: a novel tool to improve the effectiveness and sustainability of seagrass restoration. J. Appl. Ecol. 49, 1426–1435. doi: 10.1111/j.1365-2664.2012.02197.x
Balestri, E., Piazzi, L., and Cinelli, F. (1998). Survival and growth of transplanted and natural seedlings of Posidonia oceanica (L.) Delile in a damaged coastal area. J. Exp. Mar. Biol. Ecol. 228, 209–225. doi: 10.1016/S0022-0981(98)00027-6
Balestri, E., and Vallerini, F. (2003). Interannual variability in flowering of Posidonia oceanica in the North-Western Mediterranean Sea, and relationships among shoot, age and flowering. Bot. Mar. 46, 525–530. doi: 10.1515/BOT.2003.054
Balestri, E., Vallerini, F., and Lardicci, C. (2017). Recruitment and patch establishment by seed in the seagrass Posidonia oceanica: importance and conservation implications. Front. Plant Sci. 8:1067. doi: 10.3389/fpls.2017.01067
Bandeira, S. O. (2002). Leaf production rates of Thalassodendron ciliatum from rocky and sandy habitats. Aquat. Bot. 72, 13–24. doi: 10.1016/S0304-3770(01)00192-9
Bates, D., Mächler, M., Bolker, B., and Walker, S. (2015). Fitting linear mixed-effects models using lme4. J. Stat. Softw. 67, 1–48. doi: 10.18637/jss.v067.i01
Ben Brahim, M., Mabrouk, L., Hamza, A., and Jribi, I. (2020). Comparison of spatial scale variability of shoot density and epiphytic leaf assemblages of Halophila stipulacea and Cymodocea nodosa on the Eastern Coast of Tunisia. Plant Biosyst. 3, 413–426. doi: 10.1080/11263504.2019.1674399
Ben Brahim, M., Mabrouk, L., Hamza, A., Mahfoudhi, M., Bouain, A., and Aleya, L. (2014b). Spatial scale variability in shoot density and epiphytic leaves of Posidonia oceanica on Kerkennah Island (Tunisia) in relation to current tide effects. Mar. Ecol. 36, 1311–1331. doi: 10.1111/maec.12231
Ben Brahim, M., Mabrouk, L., Hamza, A., Mahfoudi, M., Bouain, A., and Aleya, L. (2014a). Bathymetric variation of epiphytic assemblages on Posidonia oceanica (L.) Delile leaves in relation to anthropogenic disturbance in the southeastern Mediterranean. Environ. Sci. Pollut. Res. 21, 13588–13601. doi: 10.1007/s11356-014-3315-8
Borowitzka, M. A., Lavery, P. S., and Van Keulen, M. (2006). “Epiphytes of seagrasses,” in Seagrasses: Biology, Ecology and Conservation, eds A. W. D. Larkum, R. J. Orth, and C. M. Duarte (Dordrecht: Springer), 463–501.
Boudouresque, C.-F. (2004). Marine biodiversity in the Mediterranean: status of species, populations and communities. Sci. Rep. Port Cros Natl. Park Fr. 20, 97–146.
Boudouresque, C. F., and Meinesz, A. (1982). Découverte de l’herbier de Posidonies. Cahier du Parc Natl. de Port Cros 4, 1–79.
Bray, J. R., and Curtis, J. T. (1957). An ordination of the upland forest communities of Southern Wisconsin. Ecol. Monogr. 27, 325–349. doi: 10.2307/1942268
Buia, M. C., Zupo, V., and Mazzella, L. (1992). Primary production and growth dynamics in Posidonia oceanica. Mar. Ecol. 13, 2–16. doi: 10.1111/j.1439-0485.1992.tb00336.x
Campagne, C. S., Salles, J.-M., Boissery, P., and Deteret, J. (2015). The seagrass Posidonia oceanica: ecosystem services identification and economic evaluation of goods and benefits. Mar. Pollut. Bull. 97, 391–400. doi: 10.1016/j.marpolbul.2015.05.061
Casola, E., and Scardi, M. (1989). Variabilità della comunità epifita sulle due facce delle lamine fogliari di Posidonia oceanica (L.) Delile. Oebalia 15, 89–97.
Celdran, D., Espinosa, E., Sánchez-Amat, A., and Marín, A. (2012). Effects of epibiotic bacteria on leaf growth and epiphytes of the seagrass Posidonia oceanica. Mar. Ecol. Prog. Ser. 456, 21–27. doi: 10.3354/meps09672
Clarke, K. R. (1993). Non−parametric multivariate analyses of changes in community structure. Aust. J. Ecol. 18, 117–143. doi: 10.1111/j.1442-9993.1993.tb00438.x
Drew, E. A., and Jupp, B. P. (1976). “Some aspects of the growth of Posidonia oceanica in Malta,” in Underwater Research, eds E. A. Drew and W. Lythgoe (London: Academic Press publ), 357–367.
Eugene, C. (1979). Epifaune des herbiers de Posidonies du littoral provençal (France) dans des secteurs pollués et non pollués. Rapports et Procès verbaux de réunion de la Commission Internationale pour l’Exploration Scientifique de la Méditerranée 25–26, 213–214.
Giovannetti, E., Lasagna, R., Montefalcone, M., Bianchi, C. N., Albertelli, G., and Morri, C. (2008). Inconsistent responses to substratum nature in Posidonia oceanica meadows: an integration through complexity levels? Chem. Ecol. 24, 83–91. doi: 10.1080/02757540801966439
Giovannetti, E., Montefalcone, M., Morri, C., Bianchi, C. N., and Albertelli, G. (2010). Early warning response of Posidonia oceanica epiphyte community to environmental alterations (Ligurian Sea, NW Mediterranean). Mar. Pollut. Bull. 60, 1031–1039. doi: 10.1016/j.marpolbul.2010.01.024
Harlin, M. M. (1975). Epiphyte—host relations in seagrass communities. Aquat. Bot. 1, 125–131. doi: 10.1016/0304-3770(75)90017-0
Infantes, E., Orfila, A., Bouma, T. J., Simarro, G., and Terrados, J. (2011). Posidonia oceanica and Cymodocea nodosa seedling tolerance to wave exposure. Limnol. Oceanogr. 56, 2223–2232. doi: 10.4319/lo.2011.56.6.2223
Kendrick, G. A., Waycott, M., Carruthers, T. J. B., Cambridge, M. L., Hovey, R., Krauss, S. L., et al. (2012). The central role of dispersal in the maintenance and persistence of seagrass populations. BioScience 62, 56–65. doi: 10.1525/bio.2012.62.1.10
Lepoint, G., Balancier, B., and Gobert, S. (2014a). Seasonal and depth-related biodiversity of leaf epiphytic Cheilostome Bryozoa in a Mediterranean Posidonia oceanica meadow. Cah. Biol. Mar. 55, 57–67. doi: 10.21411/CBM.A.E65A6F13
Lepoint, G., Mouchette, O., Pelaprat, C., and Gobert, S. (2014b). An ecological study of Electra posidoniae Gautier, 1954 (Cheilostomata, Anasca), a bryozoan epiphyte found solely on the seagrass Posidonia oceanica (L.) Delile, 1813. Belg. J. Zool. 144, 51–63. doi: 10.26496/bjz.2014.65
Mabrouk, L., Asma, H., and Bradai, M. N. (2017). Temporal and bathymetric variation of epiphyte cover and leaf biomass in a southern Posidonia oceanica (L.) meadow: the case of Mahdia coast. Tunisia. Mar. Ecol. 38:e12394. doi: 10.1111/maec.12394
Mabrouk, L., Ben Brahim, M., Hamza, A., and Bradai, M. N. (2014). Diversity and temporal fluctuations of epiphytes and sessile invertebrates on the rhizomes Posidonia oceanica in a seagrass meadow off Tunisia. Mar. Ecol. 35, 212–220. doi: 10.1111/maec.12072
Marbá, N., and Duarte, C. M. (1998). Rhizome elongation and seagrass clonal growth. Mar. Ecol. Prog. Ser. 174, 269–280.
Mazzella, L., Buia, M. C., Gambi, M. C., Lorenti, M., Russo, G. F., Scipione, M. B., et al. (1992). “Plant-animal trophic relationships in the Posidonia oceanica ecosystem of the Mediterranean Sea, a review,” in Plant-animal interactions in the marine benthos, eds D. M. John, S. J. Hawkins, and J. H. Price (Oxford: Clarendon Press), 165–187.
Mazzella, L., Scipione, M. B., and Buia, M. C. (1989). Spatio-temporal distribution of algal and animal communities in a Posidonia oceanica (L.) Delile meadow. PSZNI Mar. Ecol. 10, 107–131.
Michael, T. S., Shin, H. W., Hanna, R., and Spafford, D. C. (2008). A review of epiphyte community development: surface interactions and settlement on seagrass. J. Environ. Biol. 29, 629–638.
Montefalcone, M., Giovannetti, E., Bianchi, C. N., Morri, C., and Albertelli, G. (2006). Variazione temporale del ricoprimento epifita sulle due facce delle lamine fogliari di Posidonia oceanica. Biol. Mar. Medit. 13, 606–609.
Nelson, W. G. (2016). Development of an epiphyte indicator of nutrient enrichment: threshold values for seagrass epiphyte load. Ecol. Indic. 74, 343–356. doi: 10.1016/j.ecolind.2016.11.035
Oksanen, J., Guillaume Blanchet, F., Friendly, M., Kindt, R., Legendre, P., McGlinn, D., et al. (2019). “vegan: Community Ecology”. R package version 2.5-6.
Orth, R. J., and Van Montfrans, J. (1984). Epiphyte-seagrass relationships with an emphasis on the role of micrograzing: a review. Aquat. Bot. 18, 43–69. doi: 10.1016/0304-3770(84)90080-9
Pardi, G., Piazzi, L., Balata, D., Papi, I., Cinelli, F., and Benedetti-Cecchi, L. (2006). Spatial variability of Posidonia oceanica (L.) Delile epiphytes around the mainland and the islands of Sicily (Mediterranean Sea). Mar. Ecol. 27, 397–403. doi: 10.1111/j.1439-0485.2006.00099.x
Peirano, A., Cocito, S., Banfi, V., Cupido, R., Damasso, V., Farina, G., et al. (2011). Phenology of the Mediterranean seagrass Posidonia oceanica (L.) Delile: medium and long-term cycles and climate inferences. Aquat. Bot. 94, 77–92. doi: 10.1016/j.aquabot.2010.11.007
Pereda-Briones, L., Terrados, J., Agulles, M., and Tomas, F. (2020). Influence of biotic and abiotic factors of seagrass Posidonia oceanica recruitment: identifying suitable microsites. Mar. Environ. Res. 162:105076. doi: 10.1016/j.marenvres.2020.105076
Pessani, D., Caltagirone, A., Poncini, F., and Vetere, M. (1989). “Confronto tra due praterie di Posidonia oceanica della riviera ligure di levante e di ponente. 2. La fauna epifita,” in First International Workshop on Posidonia oceanica beds, eds C. F. Boudouresque, A. Meinesz, E. Fresi, and V. Gravez (Marseille: GIS Posidonie), 161–173.
Piazzi, L., Balata, D., and Ceccherelli, G. (2016). Epiphyte assemblages of the Mediterranean seagrass Posidonia oceanica: an overview. Mar. Ecol. 37, 3–41. doi: 10.1111/maec.12331
Piazzi, L., De Biasi, A. M., Balata, D., Pardi, G., Boddi, S., Acunto, S., et al. (2007). Species composition and spatial variability patterns of morphological forms in macroalgal epiphytic assemblages of the seagrass Posidonia oceanica. Vie Milieu 57, 171–179.
Pinckney, J. L., and Micheli, F. (1998). Microalgae on seagrass mimics: does epiphyte community structure differ from live seagrasses? J. Exp. Mar. Biol. Ecol. 221, 59–70. doi: 10.1016/S0022-0981(97)00115-9
Procaccini, G., Buia, M. C., Gambi, M. C., Perez, M., Pergent, G., Pergent-Martini, C., et al. (2003). “The seagrasses of the Western Mediterranean,” in World atlas of seagrasses, eds E. P. Green and F. T. Short (Berkeley, CA: University of California Press), 48–58.
R Core Team (2018). “R: A language and environment for statistical computing”. Austria: R Foundation for Statistical Computing.
Rindi, F., Maltagliati, F., Rossi, F., Acunto, S., and Cinelli, F. (1999). Algal flora associated with a Halophila stipulacea (Forsskal) Ascherson (Hydrocharitaceae, Helobiae) stand in the Western Mediterranean. Oceanol. Acta 4, 421–429. doi: 10.1016/S0399-1784(00)88725-3
Ruocco, M., Entrambasaguas, L., Dattolo, E., Milito, A., Marín-Guirao, L., and Procaccini, G. (2021). A king and vassals’ tale: molecular signatures of clonal integration in Posidonia oceanica under chronic light shortage. J. Ecol. 109, 294–312. doi: 10.1111/1365-2745.13479
Steneck, R. S., and Dethier, M. N. (1994). A functional group approach to the structure of algal dominated communities. Oikos 69, 476–498. doi: 10.2307/3545860
Tan, Y. M., Dalby, O., Kendrick, G. A., Statton, J., Sinclair, E. A., Fraser, M. W., et al. (2020). Seagrass restoration is possible: insights and lessons from Australia and New Zealand. Front. Mar. Sci. 7:610. doi: 10.3389/fmars.2020.00617
Telesca, L., Belluscio, A., Criscoli, A., Ardizzone, G., Apostolaki, E. T., Fraschetti, S., et al. (2015). Seagrass meadows (Posidonia oceanica) distribution and trajectories of change. Sci. Rep. 5:12505. doi: 10.1038/srep12505
Terrados, J., Marín, A., and Celdrán, D. (2013). Use of Posidonia oceanica seedlings from beachcast fruits for seagrass planting. Bot. Mar. 56, 185–195. doi: 10.1515/bot-2012-0200
Trautman, D., and Borowitzka, M. (1999). Distribution of epiphytic organisms on Posidonia australis and P. sinuosa, two seagrasses with differing leaf morphology. Mar. Ecol. Progr. Ser. 179, 213–229. doi: 10.3354/meps179215
Tsirika, A., Skoufas, G., and Haritonidis, S. (2007). Seasonal and bathymetric variations of epiphytic macroflora on Posidonia oceanica (L.) Delile leaves in the National Marine Park of Zakynthos (Greece). Mar. Ecol. 28, 146–153. doi: 10.1111/j.1439-0485.2007.00170.x
Tursi, A., Costantino, G., Mastrototaro, F., and Scardi, M. (2001). Variazioni del grado di epifitismo in due praterie di Posidonia oceanica della riserva marina delle Isole Tremiti (Mar Adriatico). Biol. Mar. Mediterr. 8, 636–640.
Uku, J., Björk, M., Bergman, B., and Díez, B. (2007). Characterization and comparison of prokaryotic epiphytes associated with three east African seagrasses. J. Phycol. 43, 768–779. doi: 10.1111/j.1529-8817.2007.00371.x
Van der Ben, D. (1971). Les épiphytes des feuilles de Posidonia oceanica Delile sur les côtes françaises de la Méditerranée. Vautierstraat: Institut Royal des Sciences Naturelles de Belgique, 1–101.
Van Elven, B. R., and Lavery, and Kendrick, G. A. (2004). Reefs as contributors to diversity of epiphyticmacroalgae assemblages in seagrass meadows. Mar. Ecol. Prog. Ser. 276, 71–83. doi: 10.3354/meps276071
Vanderklift, M. A., Doropoulos, C., Gorman, D., Leal, I., Minne, A. J. P., Statton, J., et al. (2020). Using propagules to restore coastal marine ecosystems. Front. Mar. Sci. 7:724. doi: 10.3389/fmars.2020.00724
Keywords: seagrass, recruitment, seedling, substrate, epiphyte, biological interaction
Citation: De Battisti D, Balestri E, Pardi G, Menicagli V and Lardicci C (2021) Substrate Type Influences the Structure of Epiphyte Communities and the Growth of Posidonia oceanica Seedlings. Front. Plant Sci. 12:660658. doi: 10.3389/fpls.2021.660658
Received: 29 January 2021; Accepted: 15 April 2021;
Published: 07 May 2021.
Edited by:
Miroslav Obornik, Institute of Parasitology, Academy of Sciences of the Czech Republic (ASCR), CzechiaReviewed by:
Lotfi Mabrouk, University of Gafsa, TunisiaJorge Terrados, Mediterranean Institute for Advanced Studies (IMEDEA), Spain
Asma Hamza, Institut National des Sciences et Technologies de la Mer (Tunisia), Tunisia
Copyright © 2021 De Battisti, Balestri, Pardi, Menicagli and Lardicci. This is an open-access article distributed under the terms of the Creative Commons Attribution License (CC BY). The use, distribution or reproduction in other forums is permitted, provided the original author(s) and the copyright owner(s) are credited and that the original publication in this journal is cited, in accordance with accepted academic practice. No use, distribution or reproduction is permitted which does not comply with these terms.
*Correspondence: Elena Balestri, elena.balestri@unipi.it