- 1Unit for Environmental Sciences and Management, North-West University, Potchefstroom, South Africa
- 2Julius Kühn-Institut, Federal Research Centre for Cultivated Plants, Institute for Epidemiology and Pathogen Diagnostics, Braunschweig, Germany
- 3Julius Kühn-Institut, Federal Research Centre for Cultivated Plants, Institute for Crop and Soil Science, Braunschweig, Germany
Root-knot nematodes (RKNs) are one of the most important plant-parasitic nematodes of cereal crops in sub-Saharan Africa. This study was designed to evaluate the rotation effects of different cultivars of sainfoin (Esparsette, Perly, Taja and Visnovsky), soybean (DM-5953-RSF) and alfalfa (BAR 7) with maize (P-2432-R), on a Meloidogyne enterolobii population, compared to monoculture maize. The results showed that sainfoin (Perly and Esparsette) and alfalfa had significantly (P ≤ 0.05) lower numbers of M. enterolobii eggs and second stage juveniles (J2) compared to the monoculture maize in the first experiment. However, in the repeat experiment all treatments had significantly (P ≤ 0.05) lower numbers of eggs and J2 compared to monoculture maize. Rotation of sainfoin Esparsette/maize resulted in the lowest numbers of eggs and J2 (91 and 202, respectively) in the first and repeat experiments. Rotation of sainfoin Esparsette/maize reduced M. enterolobii population density by 81 and 60% in the first and repeat experiments, respectively, followed by alfalfa (54 and 43%, respectively). Ultimately, substantial variation was evident in terms of the efficacy of different sainfoin cultivars with regards to their effect on nematode reduction when used in rotation with maize.
Introduction
Due to an increasing human population, more food has to be produced with cereal crops representing the staple food source for human and livestock consumption worldwide (FAO, 2017). Production of cereal crops in Africa, however, declined by 4.6% in 2019 in comparison to 2018 (FAO, 2020) and is a risk to food security in Africa. In South Africa, maize (Zea mays) is the most important cereal crop, but production of the crop is adversely affected due to various diseases and pests. Among others, plant-parasitic nematodes (PPNs) represent one of the major limiting biotic factors in maize cropping systems (Fourie et al., 2017; Mc Donald et al., 2017). The most economically important PPNs in maize production areas in South Africa are root-knot nematodes (Meloidogyne spp.) and root lesion nematodes (Pratylenchus spp.) (Degenkolb and Vilcinskas, 2016; Mc Donald et al., 2017). High population densities of Meloidogyne and/or Pratylenchus spp. have been reported recently for maize and other rotation crops (Fourie et al., 2017; Mc Donald et al., 2017). Earlier studies demonstrated that root-knot nematodes parasitism caused up to 60% yield losses to South African maize crops (Riekert and Henshaw, 1998). While M. incognita and M. javanica, followed by M. arenaria, are considered the predominant root-knot nematode species parasitizing local maize crops, M. enterolobii has also been discovered in a major maize producing area of the country (Pretorius, 2018). The latter species is known for its higher pathogenicity toward agricultural crops and especially for its virulence since it overcomes resistance in crops that are effective to its counterpart thermophilic species (Kiewnick et al., 2009).
To minimize damages caused by PPNs and increase crop production, combating destructive nematode pests should be considered a priority. Use of chemical nematicides was one of the most effective approaches in nematode management in the last decades (Fernández et al., 2001). Nevertheless, increasing awareness of the toxicity of pesticides (to animals, humans and the environment) and the emergence of resistance against synthetic nematicides (Kishi et al., 1995; Kaplan, 2004) are some of the reasons for the use of more environmentally friendly nematode management strategies. Therefore, to maintain PPN populations under a certain threshold, biological and cultural management strategies would play a major role in the future. In this context, the well-planned use of crop rotation is one of the possible management practices that can reduce the PPN populations in maize-based agriculture systems. However, most of the crops, especially legumes, which are used in rotation with maize in sub-Saharan Africa (SSA) and other parts of the world are parasitized by the same nematode pests of maize (Fourie et al., 2017; Coyne et al., 2018; Dababat and Fourie, 2018). Ultimately, it is crucial to find suitable crops that can be used as a rotation or cover crop in maize-based cropping systems, and that can suppress PPN densities. Sainfoin (Onobrychis viciifolia) can be an appropriate alternative, particularly due to its chemical properties, which amongst others include the production of condensed tannins with anthelmintic properties (Novobilský et al., 2013). Sainfoin was used for animal feeding in the past but after the green revolution it was replaced by alfalfa (Medicago sativa) especially because of alfalfa’s potential to produce higher yields. The yield range of sainfoin is 20–30% lower than that of alfalfa in the long-term, however, in the first cut, sainfoin yields are higher than that of alfalfa (Baldridge and Lohmiller, 1990). Sainfoin is a perennial, non-bloating forage legume, which grows up to 20–90 cm tall. It is highly palatable as a fodder source to ruminants when compared to alfalfa (Hume and Withers, 1985). Sainfoin is tolerant to drought and harsh environmental conditions due to the large and deep root system. A major trait, especially in regard to the topic of this study is that this plant is not subjected to major pests of alfalfa (Kaldy et al., 1979; Lance, 1980). The nematicidal activity of condensed tannins of sainfoin on the gastrointestinal parasitic nematodes of ruminants has been well documented (Novobilský et al., 2013; Mueller-Harvey et al., 2019). Consumption of low or moderate concentrations of condensed tannins is also associated with positive effects e.g., the increase in milk, growth and wool production in herbivore animals (Hoste et al., 2006) and a decrease in greenhouse gas emissions (Mueller-Harvey, 2006).
Both root-knot (Meloidogyne spp.) and stem nematodes (Ditylenchus dipsaci) have been reported to parasitize sainfoin in the US (Mathre, 1968). Most sainfoin cultivars used in the US, for example, showed high susceptibility when evaluated against M. hapla populations (Wofford and Gray, 1987; Wofford et al., 1989). By contrast, another study showed tolerance of sainfoin “Shoshone” to M. hapla (Gray et al., 2006b). In the last decades, research on sainfoin from a plant nematology point of view has ceased. Currently no information about the effect of sainfoin on PPN populations when grown in rotation with maize is available. This research aimed to elucidate the effect of four different sainfoin cultivars to Meloidogyne enterolobii population densities when used in a glasshouse set-up in a maize-based rotation system and compared the nematode susceptibility of one maize, one soybean (Glycine max) (a common rotation crop for maize) and one alfalfa cultivar (a closely-related plant to sainfoin).
Materials and Methods
Nematode Material
An original population of M. enterolobii was obtained from the Mbombela area where it infected roots of tomato (Solanum lycopersicon). A pure population was derived from single egg masses, and used for the experiments after confirmation of its identity using the SCAR-PCR method (Rashidifard et al., 2019b). The nematode was reared on roots of seedlings of the nematode susceptible tomato cultivar of “Moneymaker” (Fourie et al., 2012) and kept in a glasshouse at an ambient temperature range of 19–28°C and a photoperiod of 14L:10D. The main reason why M. enterolobii was selected for this study was the concerns raised regarding the widespread occurrence and pathogenicity of this species on various crops in SSA (Collett et al., 2021). Nematode infected tomato plants were uprooted 30 days after egg mass inoculation, and processed for nematode extraction using an adapted NaOCl extraction method (Riekert, 1995). The total numbers of eggs and second-stage juveniles (J2) for inoculation purposes were counted in a water suspension using a De Grisse counting dish (De Grisse, 1963) and Nikon SMZ 1 500 dissection microscope. Aliquots of ±500 eggs and J2 per pot were used to inoculate the plant roots.
Plant Material and Nematode Infection
This experiment was designed to evaluate the rotation of crops in a maize-based cultivation system with the inclusion of sainfoin as a cover crop. The seven treatments (sequences) consisted of the following: monoculture maize (maize/maize), soybean/maize, sainfoin (Visnovsky)/maize, sainfoin (Perly)/maize, sainfoin (Taja)/maize, sainfoin (Esparsette)/maize, alfalfa/maize, and tomato/tomato (positive control). Seeds of these cultivars were obtained from the respective owner companies. The effect of each plant rotation in terms of the reduction of the population densities of M. enterolobii was compared to the treatment of monoculture maize.
One week before the experiment commenced, 0.5-L capacity, white plastic pots were filled with a sandy loamy soil [5.3% clay, 93.6% sand, 1.1% silt, 0.47% organic matter; pH (H2O) of 7.47)] that has been fumigated with Telone II (a.s. 1,3 dichloropropene @ a dosage rate of 120 l/ha) on June 16, 2020 and was kept under plastic cover in the sun for 3 weeks until 07 July when the first experiment started. The second experiment was conducted on October 07, 2020 using the same batch of soil. For all treatments, pots were sown with four seeds of the respective crop cultivars and maintained in a glasshouse at the temperature range of 20–28 ± 1.6°C and a photoperiod of 14L:10D. Two weeks after germination when the seedlings were at the two-leave stage, they were thinned to contain one seedling per pot and each plant root system was inoculated with ± 500 eggs and J2 of the in vivo reared M. enterolobii population (1 egg/J2 per cc) as this was reported as damage threshold for Meloidogyne (Bowen et al., 2008; Tiwari et al., 2019). Five weeks after nematode inoculation –required time for M. enterolobii to have at least one generation (Collett, 2020), all plants were uprooted, the aerial parts and roots cut off into small pieces (2 cm) and incorporated into the soil (4–8 cm deep). One week after the incorporation of the roots and aerial plant parts, all pots were sown with one maize seed, except for those designated to contain tomato, to which seedlings of Moneymaker were replanted. The pots were maintained in the greenhouse for 5 weeks under the same conditions described above for the preceding trial. The plants were watered four times a week or as necessary and each plant was also provided with 50 ml (2 g/L) Starke Ayres Nutrifeed (nitrogen 6.5%; phosphorous 2.7%; potassium 13.0%; calcium 7.0%; magnesium 2.2%; sulfur 7.5%) every second week.
At termination of the experiments, maize plants were removed from the pots and the areal parts discarded after measuring shoot lengths. The root system of each plant was weighed and then rinsed with running tap water. Eggs and J2 of M. enterolobii were extracted using the adapted NaOCl method of Riekert (1995) and counted as explained above. The reproduction factor (Rf) [final population (Pf) / initial population (Pi)] of the nematode population was determined for each treatment according to Oostenbrink’s reproduction factor (Windham and Williams, 1987). The experiment was repeated once (at a different time interval) using the same protocols (for nematodes, plant material, inoculation, maintenance) and glasshouse conditions as described above for the first study.
Data Analysis
A randomized complete block design (RCBD) with six replicates (one plant per pot; six pots for each treatment) was chosen for the experimental layout for both experiments. Nematode data (eggs and J2 numbers per root system), were log(x) transformed, this also was applied for plant height in the repeat experiment. Data from each experiment was first subjected to an ANOVA (Statistica, Version 13.3) individually. Subsequently, Tukey’s HSD Test (P ≤ 0.05) was conducted to separate the means of the eight treatments of the two experiments. Combined data of both experiments was then subjected to a Factorial Analysis of Variance (ANOVA) (Statistica, Version 13.3) with time (representing the two experiments) as the main factor and treatments as the sub-factor. The effect of different treatments on control of nematode was calculated based on the following formula:
where “X” is the final number of eggs and J2 and “Y” is the initial nematode population (ca. 500 eggs and J2). The graphs showing the results of both experiments independently were generated using GraphPad Prism version 8.021.
Results
Plant height, as well as the number of nematode eggs and J2 and the Rf values per root system of each crop cultivar were significantly different (P ≤ 0.05) among the treatments for both experiments. There were no significant differences for the root mass among the treatments of each experiment. Significant interaction was observed for all parameters for experiment x treatments, showing that the maize plants (treatments) reacted differently with regard to the M. enterolobii population densities representing the different crop treatments during the two experiments.
Numbers of Eggs and J2s per Plant
A significant interaction (P = 0.000; F = 21.9) was observed for the numbers of eggs and J2 of M. enterolobii per root system for experiment × treatments (Supplementary Table 1) being significantly different (P ≤ 0.05) for the sainfoin (Taja)/maize rotation and the tomato standard for the two experiments.
The rotation of sainfoin (Esparsette)/maize and soil amendment with the roots and aerial parts of this cultivar resulted in the lowest numbers of M. enterolobii eggs and J2 per root system (91 and 202, respectively) in the first and repeat experiments. Among the treatments (excluding tomato), soil amendment and rotation of the sainfoin Taja/maize had the highest number (2581) of eggs and J2 in the first experiment, whilst the monoculture maize showed the highest number of eggs and J2 (1541) in the repeat experiment. In the first experiment two cultivars of sainfoin (Perly and Esparsette), and alfalfa had significantly (P ≤ 0.05) lower number of eggs and J2 compared to monoculture maize (Figure 1). However, in this experiment the results showed that soil amendment and rotation of sainfoin (Taja) with maize increased the nematode population significantly (P ≤ 0.05). No significant differences (P ≤ 0.05) were observed when soybean used for soil amendment and rotated with maize compared to monoculture maize in the first experiment. However, the repeat experiment indicated that rotation and amendment of all treatments resulted in significantly (P ≤ 0.05) lower number of eggs and J2 per root system compared to monoculture maize.
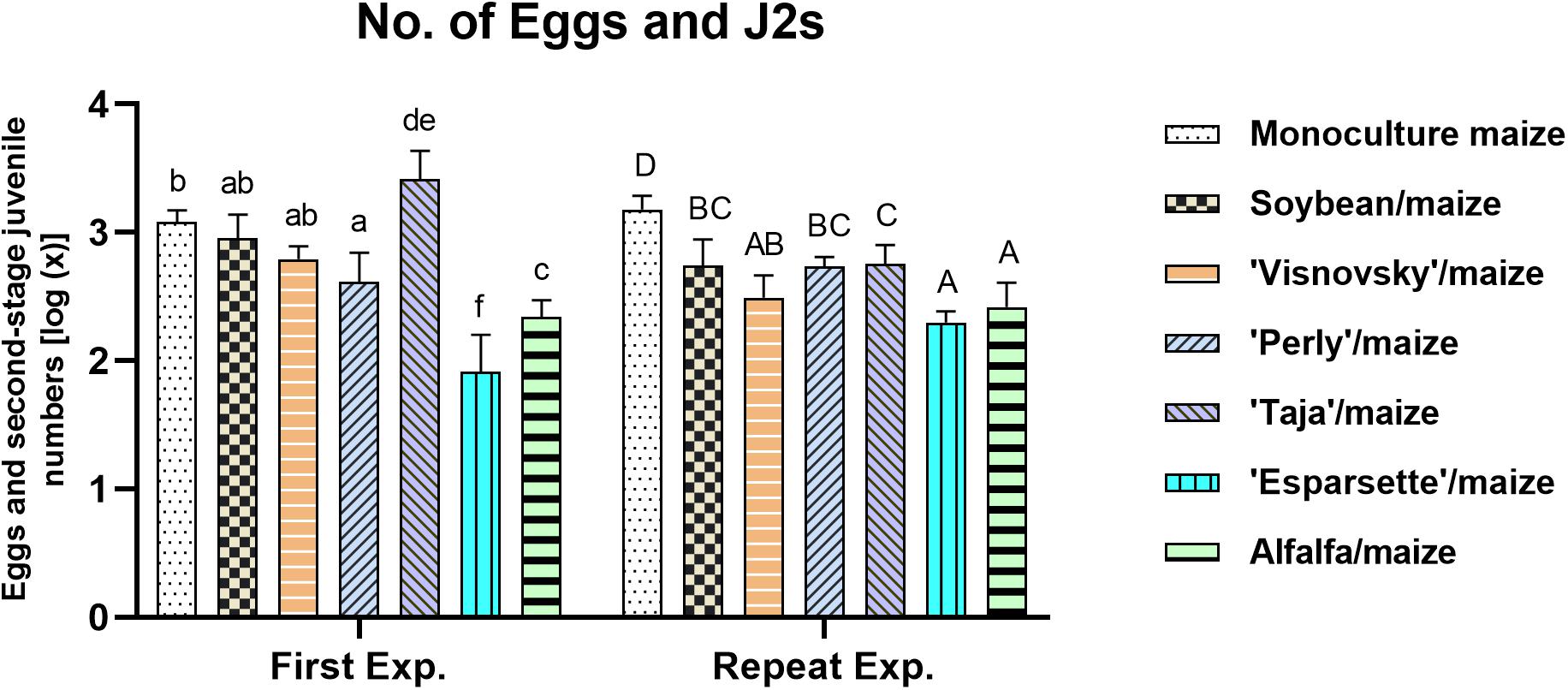
Figure 1. Population densities of Meloidogyne enterolobii in roots of maize, 12 weeks post-inoculation with ± 500 eggs and second-stage juveniles and incorporation of aerial material of previously grown maize (P-2432-R), soybean (DM-5953-RSF), sainfoin (Esparsette, Taja, Perly, Visnovsky), and alfalfa (BAR 7) into the soiland rotation thereof. The y-axis shows the log(x) transformed data. Different letters indicate significant differences at P ≤ 0.05 based on Tukey’s test for each experiment separately.
Effect of Rotation on Nematode Population Densities
Results from the first experiment showed that monoculture maize, soybean/maize and sainfoin (Visnovsky and Taja)/maize treatments increased M. enterolobii population densities, with Taja/maize having the highest (470%) and monoculture maize the second highest (146%) values (Figure 2). Sainfoin Perly, alfalfa and sainfoin Esparsette used for soil amendment and rotated with maize reduced nematode population densities by 7, 54, and 81%, respectively (Figure 2). In the repeat experiment monoculture maize had the highest increase (208%) in the M. enterolobii density, followed by soybean/maize (27%). Similar to the first experiment, soil amendment and rotation of sainfoin (Esparsette)/maize had the highest reduction (60%) in the nematode population density followed by the alfalfa/maize (43%). In contrast to the first experiment, sainfoin Taja/maize this time increased the nematode population by 19% (Figure 2).
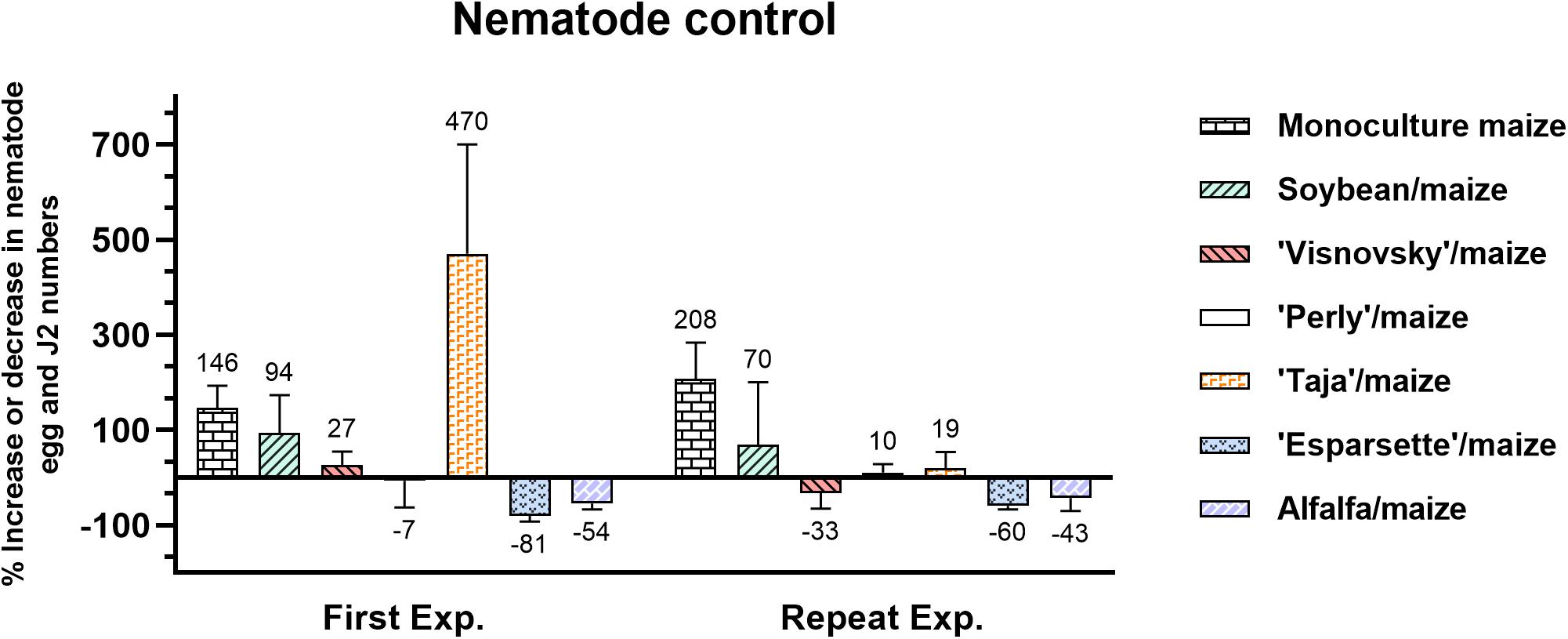
Figure 2. Variable Meloidogyne enterolobii density levels in roots of maize 12 weeks post-inoculation with ± 500 eggs and second-stage juveniles, and incorporation of aerial material of previously grown maize (P-2432-R), soybean (DM-5953-RSF), sainfoin (Esparsette, Taja, Perly, Visnovsky), and alfalfa (BAR 7) into the soil and rotation thereof of. The y-axis shows the percentage among which positive numbers show increase and negative numbers indicate reduction in nematode population.
Rf Value
There was a significant interaction (P = 0.00; F = 156.8) for Rf value for experiment x treatment, which was the result of a significant difference (P ≤ 0.05) for tomato as the positive control in the first (8.7) and repeat (58.8) experiments (Supplementary Table 1).
In the first experiment Rf values (excluding for tomato) ranged between 0.1 (sainfoin Esparsette/maize) and 2.4 (monoculture maize) with the former being significantly (P ≤ 0.05) lower, and sainfoin (Taja)/maize (5.7) significantly (P ≤ 0.05) higher than monoculture maize (Figure 3). In the repeat experiment Rf values ranged between 0.4 (sainfoin Esparsette/maize) and 3 (monoculture maize). In this experiment, all treatments had significantly (P ≤ 0.05) lower Rf values in comparison with monoculture maize (Figure 3).
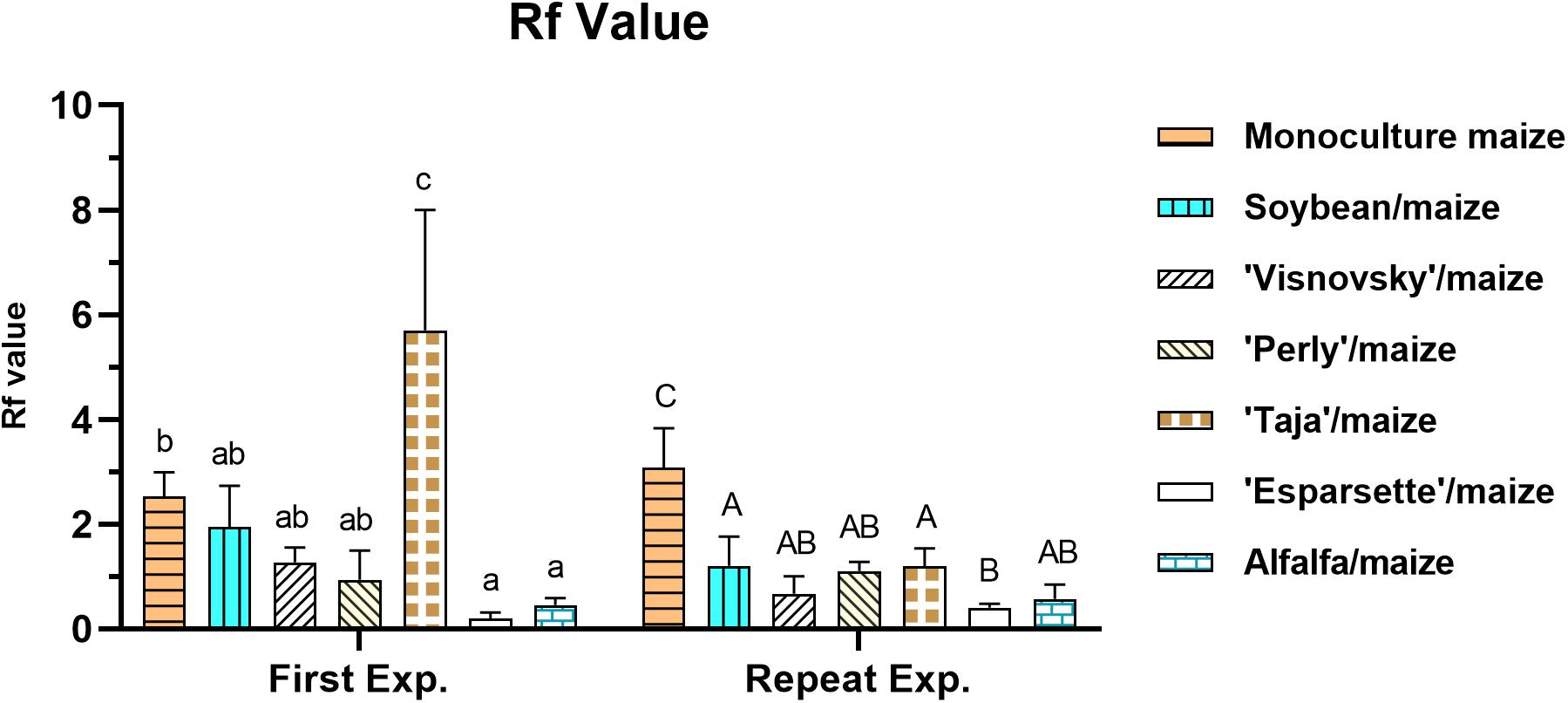
Figure 3. Reproduction factor (Rf) value of a Meloidogyne enterolobii population on maize, 12 weeks post-inoculation with ± 500 eggs and second-stage juveniles, and incorporation of aerial material of maize (P-2432-R), soybean (DM-5953-RSF), sainfoin (Esparsette, Taja, Perly, Visnovsky), and alfalfa (BAR 7) into the soil and rotation thereof. The y-axis shows Rf value. Different letters indicate significant differences at P ≤ 0.05 based on Tukey’s test for each experiment separately.
Plant Height
A significant interaction (P = 0.004; F = 3.53) for plant height for experiment x treatments was observed that can be ascribed by the significant difference (P ≤ 0.05) recorded for sainfoin (Taja)/maize 91.5 and 59 cm during the first and repeat experiments, respectively (Supplementary Table 2).
In the first experiment the plant height ranged from 63 cm (monoculture maize) to 92.1 cm (sainfoin Perly/maize). There were significant differences (P ≤ 0.05) between treatments in the first experiment, with soil amendment and rotation of sainfoin (Perly and Taja)/maize being significantly (P ≤ 0.05) taller than monoculture maize (Figure 4). Plant height values ranged between 56.1 cm (sainfoin Esparsette/maize) to 92.2 cm (soybean/maize) in the repeat experiment, with soybean/maize being significantly higher (P ≤ 0.05) than monoculture maize (Figure 4).
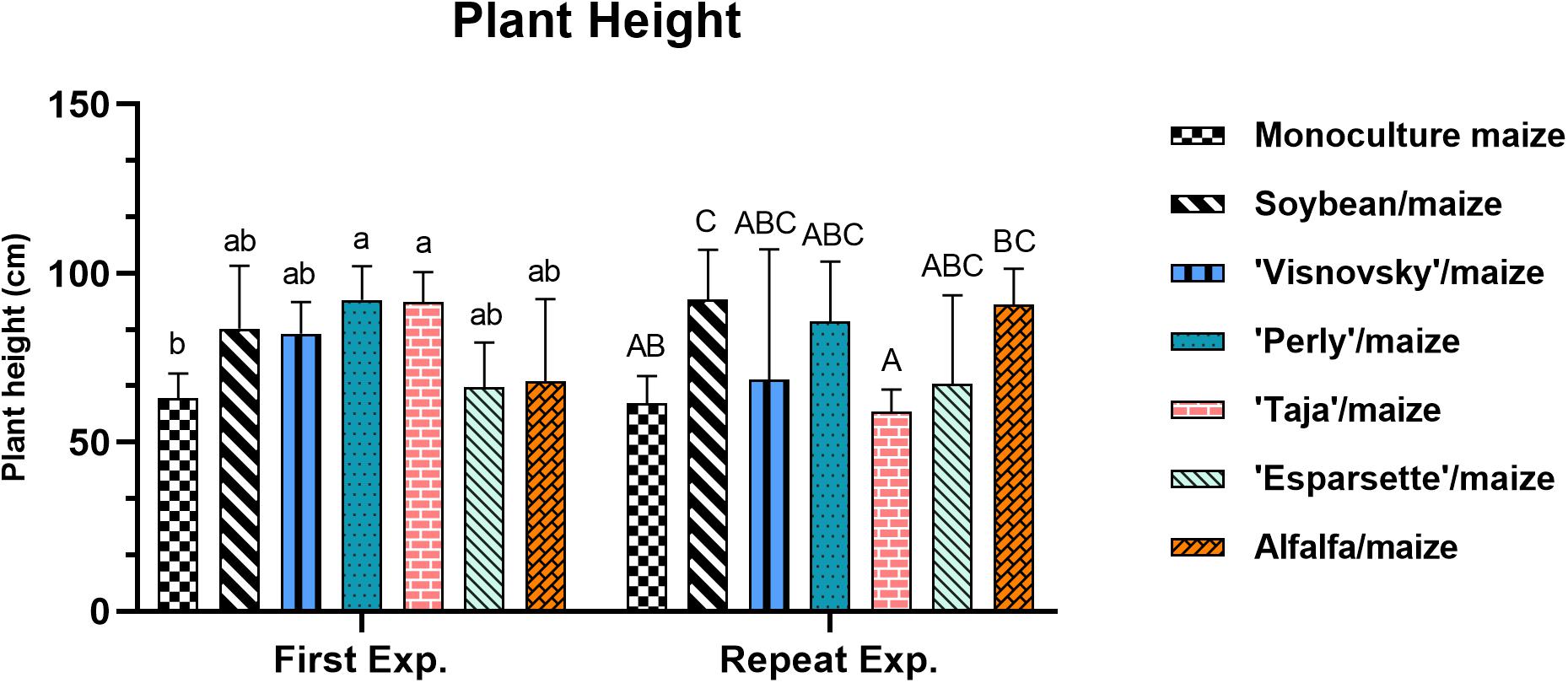
Figure 4. Plant height (cm) of maize plants, 12 weeks post-inoculation with ± 500 eggs and second-stage juveniles of Meloidogyne enterolobii, and incorporation of aerial material of maize (P-2432-R), soybean (DM-5953-RSF), sainfoin (Esparsette, Taja, Perly, Visnovsky), and alfalfa (BAR 7) into the soil and rotation thereof. The y-axis shows plant height value (cm). Different letters indicate significant differences at P ≤ 0.05 based on Tukey’s test for each experiment separately.
Root Mass
A significant interaction (P = 0.16; F = 6.045) for root weight for experiment × treatments was observed due to the significant differences (P ≤ 0.05) in root weight of the sainfoin: Taja/maize for the two experiments (Supplementary Table 2). No significant differences, however, existed among the treatments for the root weight in the first or repeat experiment (Supplementary Table 2). The root mass weight ranged between 16.8 g (sainfoin Taja/maize) to 21 g (soybean/maize and sainfoin (Esparsette)/maize) in the first experiment. In the repeat experiment, it ranged between 14.6 g (sainfoin Perly/maize) and 21.3 g (monoculture maize) and there was no significant difference (P ≤ 0.05) between different treatments (Figure 5).
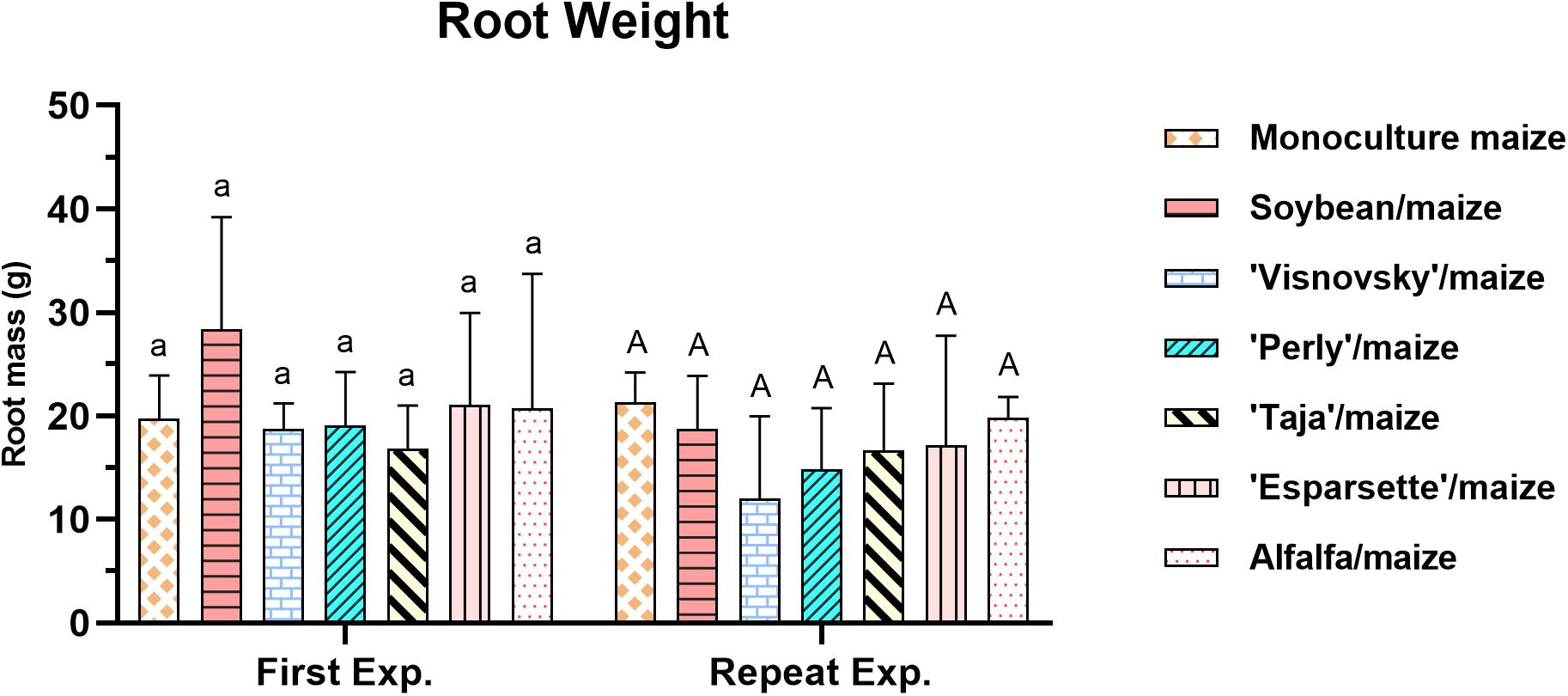
Figure 5. Root mass (g) of maize plants, 12 weeks post-inoculation with ± 500 eggs and second-stage juveniles of Meloidogyne enterolobii, and incorporation of aerial material of maize (P-2432-R), soybean (DM-5953-RSF), sainfoin (Esparsette, Taja, Perly, Visnovsky), and alfalfa (BAR 7) into the soil and rotation thereof. The y-axis shows plant weight (g). Different letters indicate significant differences at P ≤ 0.05 based on Tukey’s test for each experiment separately.
Discussion
In this study we observed significant reductions (compared to the initial/inoculated nematode population) of 81 and 60% of M. enterolobii population densities in the first and repeat experiments, respectively, when sainfoin cultivar Esparsette was rotated with maize and incorporated into the soil. This result highlights the positive role sainfoin can potentially play in grain-based sequences to minimize damage caused by M. enterolobii. Substantial reductions of 54 and 43% of M. enterolobii population densities (in the first and repeat experiments, respectively) when using the nematode resistant alfalfa cultivar BAR 7 in rotation with maize, further emphasizes the beneficial impact of using sainfoin for nematode control in cropping sequences. For sainfoin cultivar Taja contradicting results were observed with regard to the final M. enterolobii densities between the first and repeat experiment. Possible explanations that compromised these results could be that the inoculation effectivity in terms of numbers (although the same inoculation protocol was followed and the same M. enterolobii population was used) and/or hatching rate of J2 varied between the two experiments, and/or that potential differences in root growth of Taja existed in the respective experiments. These results, however, accentuated that growing Taja may lead to increased population densities of M. enterolobii. Similar experiences were recorded by Rashidifard et al. (2019a) and Agenbag (2016) in terms of differences recorded for root-knot final nematode population densities for initial and repeat experiments conducted with tomato.
The significant inhibiting effects of sainfoin cultivar Esparsette on M. enterolobii population densities suggests that it can be a potential alternative for alfalfa cultivar BAR 7 (reported as resistant to pest and disease)2 in areas where alfalfa pests, such as Sitona weevils, are a serious concern. In addition, it is important to take other advantages of sainfoin into consideration. Sainfoin was reported to be resistant to various pests that feed on alfalfa (Wallace, 1969; Lance, 1980). The condensed tannin present in sainfoin were reported to have non-bloating and anthelmintic effects (Novobilský et al., 2013).
The significantly lower numbers of M. enterolobii eggs and J2s on maize rotated with sainfoin cultivars Perly and Esparsette, and alfalfa (BAR 7) in the first experiment (compared to monoculture maize) opposed to similar population densities on maize rotated with soybean, demonstrate the potential use of these fodders as an alternative in traditional grain-based cropping systems. The high susceptibility of soybean to the same nematode pests that parasitize maize poses problems in SSA (Fourie et al., 2017) and other regions (Coyne et al., 2018; Dababat and Fourie, 2018) and could compromise the sustainable production of grain crops (Riekert and Henshaw, 1998; Miller et al., 2006).
The low Rf data obtained in both experiments, revealed that sequences with sainfoin cultivar Esparsette and alfalfa cultivar BAR 7 may be resistant to M. enterolobii. This should, however, be verified in host status experiments since this study was only investigating cropping sequences of the selected crops.
The current study showed high variation among sainfoin cultivars in terms of their effectiveness in reducing root-knot nematode population density levels when used in a crop rotation system. Additionally, the inhibiting effects of sainfoin on M. enterolobii recorded in our study were in contrast with previous reports that showed high susceptibility of sainfoin to M. hapla (Griffin, 1971; Wofford and Gray, 1987; Shigaki et al., 2008). This could be attributed to the high genetic diversity that was reported among different sainfoin cultivars (Mohajer et al., 2013; Zarrabian et al., 2013). It is speculated that the incorporation of leaves of sainfoin into soil substrate would result in the release of condensed tannins. The released tannins might then play a role in suppression of M. enterolobii in soil or in the plant tissues where this nematode pest feeds. Further studies will be needed to analyze the impact of condensed tannins in control of PPNs.
The value of a crop rotation and soil amendment with soybean, sainfoin and alfalfa reflected by maize height data was greater than monoculture maize in both experiments. This is a common phenomenon when crop rotation with legumes is practiced and it is in agreement with previous reports (Tanimu et al., 2007). High numbers of M. enterolobii population densities did not show a negative effect on plant height, showing that this cannot be used as an indication for nematode damage in these kind of experiments.
The root mass value for both experiments showed no significant differences among the treatments which is agreed with the results reported from a local study (Rashidifard et al., 2019a) and warrants no further discussion.
Besides this study, there has been only one report regarding a tolerant cultivar of sainfoin (Gray et al., 2006a), “Shoshone,” identified as tolerant to M. hapla. Other sainfoin cultivars that have been tested for their host status against M. hapla and Ditylenchus destructor, showed high susceptibility to these nematodes (Wofford and Gray, 1987; Wofford et al., 1989; Shigaki et al., 2008). Reporting the beneficial effects of sainfoin cultivar Esparsette, which is the only available cultivar in South Africa, is a novel addition to the limited, existing research on this crop. The fact that this cultivar was tested against M. enterolobii, known as an emerging threat for agriculture production and food security in SSA (Coyne et al., 2018; Visagie et al., 2018; Rashidifard et al., 2019b; Bello et al., 2020; Collett et al., 2021) could be an advantage for producers and crop industries.
Conclusion
In this paper, we showed that introducing sainfoin in a crop rotation system is a novel nematode management strategy with potential to be used by producers across the globe. Especially, sainfoin cultivar Esparsette showed a high efficacy in nematode reduction, making it a promising candidate to be used in rotation with maize and as cover crop for soil amendment in SSA where PPNs are a serious concern. More studies need to be conducted to elucidate the host status of different sainfoin cultivars against Meloidogyne and other economically important PPNs such as Pratylenchus species, with the role that condensed tannins play in this regard to be emphasized.
Data Availability Statement
The original contributions presented in the study are included in the article/Supplementary Material, further inquiries can be directed to the corresponding author/s.
Author Contributions
MR and SA developed the project and designed the experiments and drafted the manuscript. MR executed the experiments, collected, analyzed, and interpreted the data. SC, TT, and HF contributed to the design of the work. All authors reviewed and edited the manuscript.
Funding
Partial funding by the North-West University of South Africa and open access publication fees from the Julius Kühn-Institut, Federal Research Centre for Cultivated Plants, Germany are acknowledged.
Conflict of Interest
The authors declare that the research was conducted in the absence of any commercial or financial relationships that could be construed as a potential conflict of interest.
Supplementary Material
The Supplementary Material for this article can be found online at: https://www.frontiersin.org/articles/10.3389/fpls.2021.659322/full#supplementary-material
Footnotes
References
Agenbag, M. (2016). Identification and Reproduction Potential of South African Meloidogyne species. Master thesis, North-West Universit, Potchefstroom.
Baldridge, D. E., and Lohmiller, R. (1990). Montana interagency Plant maTerials Handbook for Forage Production, Conservation, Reclamation, and Wildlife. Bozeman: Montana State University, Extension Service.
Bello, T. T., Coyne, D. L., Rashidifard, M., and Fourie, H. (2020). Abundance and diversity of plant-parasitic nematodes associated with watermelon in Nigeria, with focus on Meloidogyne spp. Nematology 22, 781–797. doi: 10.1163/15685411-00003340
Bowen, K. L., Hagan, A. K., Campbell, H. L., and Nightengale, S. (2008). Effect of southern Root-knot nematode (Meloidogyne incognita race 3) on corn yield in Alabama. Plant Health Prog. 9, 1–9. doi: 10.1094/PHP-2008-0910-01-RS
Collett, R. L. (2020). A comparative Study of the Development and Reproduction of 388 Meloidogyne Enterolobii and Other Thermophilic South African Meloidogyne species. Master thesis, North-West University, Potchefstroom, 389.
Collett, R. L., Marais, M., Daneel, M. S., Rashidifard, M., and Fourie, H. (2021). Meloidogyne enterolobii, a threat to crop production with particular reference to sub-Saharan Africa: a critical and updated review. Nematology 23, 247–285. doi: 10.1163/15685411-bja10076
Coyne, D. L., Cortada, L., Dalzell, J. J., Claudius-Cole, A. O., Haukeland, S., Luambano, N., et al. (2018). Plant-parasitic nematodes and food security in Sub-Saharan Africa. Annu. Rev. Phytopathol. 56, 381–403. doi: 10.1146/annurev-phyto-080417-045833
Dababat, A. A., and Fourie, H. (2018). “Nematode parasites of cereals,” in Plant Parasitic Nematodes in Subtropical and Tropical Agriculture, 3rd Edn, eds R. Sikora, D. Coyne, and J. Hallmann (Wallingford: CAB International), 163–221. doi: 10.1079/9781786391247.0163
De Grisse, A. (1963). A counting dish for nematodes excluding border effect. Nematologica 9, 162–162. doi: 10.1163/187529263x00313
Degenkolb, T., and Vilcinskas, A. (2016). Metabolites from nematophagous fungi and nematicidal natural products from fungi as an alternative for biological control. Part I: metabolites from nematophagous ascomycetes. Appl. Microbiol. Biotechnol. 100, 3799–3812. doi: 10.1007/s00253-015-7233-6
Fernández, C., Rodrıguez-Kábana, R., Warrior, P., and Kloepper, J. W. (2001). Induced soil suppressiveness to a root-knot nematode species by a nematicide. Biol. Control 22, 103–114. doi: 10.1006/bcon.2001.0961
Fourie, H., Mc Donald, A. H., Steenkamp, S., and De Waele, D. (2017). “Nematode pests of leguminous and oilseed crops,” in Nematology in South Africa: a view from the 21st Century, eds H. Fourie, V. W. Spaull, R. K. Jones, M. S. Daneel, and D. De Waele (Cham: Springer International Publishing), 201–230. doi: 10.1007/978-3-319-44210-5_9
Fourie, H., Mothata, T., Ntidi, K. N., Mc Donald, A. H., and De Waele, D. G. M. A. (2012). Indications of variation in host suitability to root knot nematode populations in commercial tomato varieties. Afr. J. Agric. Res. 7, 2344–2355. doi: 10.5897/AJAR11.706
Gray, F. A., Koch, D. W., Delaney, R. H., and Gray, A. M. (2006b). Development and release of shoshone sainfoin (Onobrychus viciifolia) with some tolerance to the northern root knot nematode. Phytopathology 96:168.
Gray, F., Shigaki, T., Koch, D., Delaney, R., Hruby, F., Gray, A., et al. (2006a). Registration of ‘Shoshone’sainfoin. Crop Sci. 46, 988–988. doi: 10.2135/cropsci2005.07-0190
Griffin, G. (1971). Susceptibility of common sainfoin to Meloidogyne hapla. Plant Dis. Rep. 55, 1069–1072.
Hoste, H., Jackson, F., Athanasiadou, S., Thamsborg, S. M., and Hoskin, S. O. (2006). The effects of tannin-rich plants on parasitic nematodes in ruminants. Trends Parasitol. 22, 253–261. doi: 10.1016/j.pt.2006.04.004
Hume, L., and Withers, N. (1985). Nitrogen fixation in sainfoin (Onobrychis viciifolia) 1. Responses to changes in nitrogen nutrition. New Zeal. J. Agric. Res. 28, 325–335. doi: 10.1080/00288233.1985.10430435
Kaldy, M., Hanna, M., and Smoliak, S. (1979). Amino acid composition of sainfoin forage. Grass Forage Sci. 34, 145–148. doi: 10.1111/j.1365-2494.1979.tb01459.x
Kaplan, R. M. (2004). Drug resistance in nematodes of veterinary importance: a status report. Trends Parasitol. 20, 477–481. doi: 10.1016/j.pt.2004.08.001
Kiewnick, S., Dessimoz, M., and Franck, L. (2009). Effects of the Mi-1 and the N root-knot nematode-resistance gene on infection and reproduction of Meloidogyne enterolobii on tomato and pepper cultivars. J. Nematol. 41, 134–139.
Kishi, M., Hirschhorn, N., Djajadisastra, M., Satterlee, L. N., Strowman, S., and Dilts, R. (1995). Relationship of pesticide spraying to signs and symptoms in Indonesian farmers. Scand. J. Work Environ. Health 21, 124–133. doi: 10.5271/sjweh.19
Mathre, D. (1968). “Disease in sainfoin,” in Proceedings of the Sainfoin Symposium, eds C. S. Cooper and A. E. Carleton (Montana: Montana Agricultural Experiment Station Publications), 65–66.
Mc Donald, A. H., De Waele, D., and Fourie, H. (2017). “Nematode pests of maize and other cereal crops,” in Nematology in South Africa: a View from the 21st Century, eds H. Fourie, V. W. Spaull, R. K. Jones, M. S. Daneel, and D. De Waele (Cham: Springer International Publishing), 183–199. doi: 10.1007/978-3-319-44210-5_8
Miller, D., Chen, S., Porter, P., Johnson, G., Wyse, D., Stetina, S., et al. (2006). Rotation crop evaluation for management of the soybean cyst nematode in Minnesota. Agron. J. 98, 569–578. doi: 10.2134/agronj2005.0185
Mohajer, S., Jafari, A. A., Taha, R. M., Yaacob, J. S., and Saleh, A. (2013). Genetic diversity analysis of agro-morphological and quality traits in populations of sainfoin (Onobrychis sativa). Austr. J. Crop Sci. 7, 1024–1031.
Mueller-Harvey, I. (2006). Unravelling the conundrum of tannins in animal nutrition and health. J. Sci. Food Agric. 86, 2010–2037. doi: 10.1002/jsfa.2577
Mueller-Harvey, I., Bee, G., Dohme-Meier, F., Hoste, H., Karonen, M., Kölliker, R., et al. (2019). Benefits of condensed tannins in forage legumes fed to ruminants: importance of structure, concentration, and diet composition. Crop Sci. 59, 861–885. doi: 10.2135/cropsci2017.06.0369
Novobilský, A., Stringano, E., Carbonero, C. H., Smith, L., Enemark, H. L., Mueller-Harvey, I., et al. (2013). In vitro effects of extracts and purified tannins of sainfoin (Onobrychis viciifolia) against two cattle nematodes. Veter. Parasitol. 196, 532–537. doi: 10.1016/j.vetpar.2013.03.024
Pretorius, M. (2018). The Abundance, Identity and Population Dynamics of Meloidogyne spp. Associated with Maize in South Africa. Master thesis, North-West University, Potchefstroom.
Rashidifard, M., Marais, M., Daneel, M. S., and Fourie, H. (2019a). Reproductive potential of South African thermophilic Meloidogyne populations, with special reference to Meloidogyne enterolobii. Nematology 21, 913–921. doi: 10.1163/15685411-00003263
Rashidifard, M., Marais, M., Daneel, M. S., Mienie, C. M. S., and Fourie, H. (2019b). Molecular characterisation of Meloidogyne enterolobii and other Meloidogyne spp. from South Africa. Trop. Plant Pathol. 44, 213–224. doi: 10.1007/s40858-019-00281-4
Riekert, H. (1995). A modified sodium hypochlorite technique for the extraction of root-knot nematode eggs and larvae from maize root samples. Afr. Plant Protect. 1, 41–43.
Riekert, H., and Henshaw, G. (1998). Effect of soybean, cowpea and groundnut rotations on root-knot nematode build-up and infestation of dryland maize. Afr. Crop Sci. J. 6, 377–383.
Shigaki, T., Gray, F. A., Delaney, R. H., and Koch, D. W. (2008). Evaluation of host resistance and intercropping for management of the northern root-knot nematode in sainfoin, Onobrychis viciifolia. J. Sustain. Agric. 12, 23–39. doi: 10.1300/J064v12n01_04
Tanimu, J., Iwuafor, E., Odunze, A., and Tian, G. (2007). Effect of incorporation of leguminous cover crops on yield and yield components of maize. World J. Agric. Sci. 3, 243–249.
Tiwari, S., Eisenback, J. D., and Youngman, R. R. (2019). Root-knot Nematode in Field Corn. Petersburg, VA: Virginia Cooperative Extension, Virginia Tech, Virginia State University.
Visagie, M., Mienie, C. M., Marais, M., Daneel, M., Karssen, G., and Fourie, H. (2018). Identification of Meloidogyne spp. associated with agri-and horticultural crops in South Africa. Nematology 20, 397–401. doi: 10.1163/15685411-00003160
Wallace, L. (1969). “Current and potential insect problems of sainfoin in America,” in Proceedings of the Sainfoin Symposium, eds C. S. Cooper and A. E. Carleton (Bozeman: Montana State University).
Windham, G. L., and Williams, W. P. (1987). Host suitability of commercial corn hybrids to Meloidogyne arenaria and M. incognita. J. Nematol. 19, 13–16.
Wofford, D., and Gray, F. (1987). Evaluation of cultivars, experimental lines and plant introduction collection of Sainfoin for resistance to Meloidogyne hapla Chitwood. J. Nematol. 19, 30–37.
Wofford, D., Gray, F., and Eckert, J. (1989). Pathogenicity of two populations of Meloidogyne hapla Chitwood on alfalfa and sainfoin. J. Nematol. 21, 87–91.
Keywords: crop rotation, maize, management, Meloidogyne, Onobrychis viciifolia, plant-parasitic nematodes, sainfoin
Citation: Rashidifard M, Ashrafi S, Claassens S, Thünen T and Fourie H (2021) A Pilot Approach Investigating the Potential of Crop Rotation With Sainfoin to Reduce Meloidogyne enterolobii Infection of Maize Under Greenhouse Conditions. Front. Plant Sci. 12:659322. doi: 10.3389/fpls.2021.659322
Received: 27 January 2021; Accepted: 25 March 2021;
Published: 16 April 2021.
Edited by:
Juan Emilio Palomares-Rius, Institute for Sustainable Agriculture, Consejo Superior de Investigaciones Científicas (CSIC), SpainReviewed by:
Pablo Castillo, Institute for Sustainable Agriculture, Spanish National Research Council, SpainJohan Desaeger, University of Florida, United States
José Castro, Embrapa Semiárido, Brazil
Copyright © 2021 Rashidifard, Ashrafi, Claassens, Thünen and Fourie. This is an open-access article distributed under the terms of the Creative Commons Attribution License (CC BY). The use, distribution or reproduction in other forums is permitted, provided the original author(s) and the copyright owner(s) are credited and that the original publication in this journal is cited, in accordance with accepted academic practice. No use, distribution or reproduction is permitted which does not comply with these terms.
*Correspondence: Milad Rashidifard, bWlsYWQucmFzaGlkaWZhcmRAbnd1LmFjLnph
†These authors share last authorship