- 1Laboratory of Pollinator, Pest and Vector Arthropods, Department of Agriculture, Society and Environment, El Colegio de la Frontera Sur, Tapachula, Mexico
- 2Consejo Nacional de Ciencia y Tecnología, Mexico City, Mexico
- 3Centro de Investigación y de Estudios Avanzados del Instituto Politécnico Nacional, Irapuato, Mexico
- 4Departamento de Botánica y Zoología, Instituto de Botánica, Centro Universitario de Ciencias Biológicas y Agropecuarias, Universidad de Guadalajara, Zapopan, Mexico
Domestication is an evolutionary process with an impact on plant reproduction. Many domesticated plants are self-compatible (i.e., they lack mechanisms to reject their own pollen), but few domesticated plants are fully or partially self-incompatible. We used the husk tomato, Physalis philadelphica, as a study model to investigate changes in the reproductive strategy of an annual partially self-incompatible plant during the process of domestication. Wild and cultivated populations of this species coexist in close proximity. These different populations present a high level of morphological and genetic variation associated with different degrees of domestication. We hypothesized that artificial selection favors self-compatibility in cultivated plants through changes in their reproductive strategy and some reproductive parameters associated with domestication. To test this hypothesis, we characterized the floral morphology and some reproductive parameters of weedy plants (wild plants), landraces (semi-domesticated plant), and commercial plants (domesticated plants). We conducted an artificial crossing experiment, germinated the seeds, and recorded seedling growth. Commercial plants had the largest flowers and the highest number of ovules. Yet, they did not differ in other reproductive parameters (e.g., herkogamy, size of pollen grains, stigmatic area, and pollen:ovule ratio) from landraces and weedy plants. Physalis philadelphica produced fruits by autonomous autogamy in the artificial crossing experiment. These fruits were the smallest and lightest fruits at all degrees of domestication; however, fruit set of autonomous autogamy was higher in weedy plants. In addition, fruit production was higher when weedy plants donated pollen to commercial plants. Although seeds produced by autonomous autogamy of weedy plants had a low germination percentage, their cotyledons and the embryonic foliage leaves appeared earlier than in landraces and commercial plants. In conclusion, the domestication syndrome in this plant was manifested as increments in flower size and ovule production. Contrary to expectations, there was higher fruit production by autonomous autogamy in weedy plants than in cultivated plants. It seems that artificial selection in P. philadelphica favors self-incompatibility in cultivated plants. Nonetheless, spontaneous self-pollination seems to be advantageous in weedy populations because they produced viable seeds from which cotyledons and the embryonic foliage leaves emerged earlier than in cultivated plants.
Introduction
Plant domestication is an evolutionary process mediated by artificial human selection and evolutionary forces, which continues to act on managed or cultivated plant populations (Casas and Barbera, 2002). This process can modify not only reproduction and survival of domesticated plants, but also genetic composition and ecological behavior (Bye and Linares, 2000; Pickersgill, 2007). That is why it is possible to find different degrees of plant domestication, which vary phenotypically and genotypically in the same species (Purugganan and Fuller, 2009).
Populations of a plant species can present a gradient of modifications. (1) Domesticated populations are crops that vary in terms of morphology and genetics from wild relatives. They depend on humans to survive as they have lost their ecological adaptations. (2) Despite being cultivated, semi-domesticated populations are able to survive in the wild without human intervention, but their selective traits are different from those observed in wild populations. (3) Incipiently domesticated populations, for example, are cultivars whose selected traits do not differ from those found in wild populations. (4) Adapted to human-disturbed environments, incidentally co-evolved populations display no human selection trait. (5) And, wild- or non-domesticated populations are natural populations, whose genotype and phenotype have not been modified by humans (Clement, 1999; Meyer et al., 2012). When a plant species becomes the targeted of artificial selection, crops are classified in different evolutionary stages regarding their diversification and phenotypic characteristics (Meyer and Purugganan, 2013). In the Pre-stage 1, deliberate planting or attendance of wild plants that have favorable traits occurs. In the Stage 1, after attempts to develop or establish cultivation, crop selection occurs in an agroecosystem, which leads to the onset domestication. In Stage 2 there is an in situ increase of frequencies of desirable alleles in the population that leads to initial increase in yield, and selection favors crop phenotypes increasing trait variation. In Stage 3, the domesticated crop extends its geographic ranges and adapts to new environments. Finally, in Stage 4 deliberate breeding of crop varieties aims to maximize yield, ease farming effort, and increases uniformity and quality.
During domestication, artificial selection decreases fitness in natural conditions, but increases fitness to human exploitation (Meyer and Purugganan, 2013). Therefore, traits selection affects reproductive success or plant defenses (Rosenthal and Dirzo, 1997; Meyer et al., 2012). Early plant breeders have observed some characteristics exhibited by plants or populations that facilitate domestication, such as self-pollination, vegetative propagation, and delayed seed dispersal (Cox, 2009). For this reason, most crops (75%) are self-compatible (SC) (lack of mechanisms to reject their own pollen). Only a few crops have self-incompatibility (SI) systems, and a few others have partial or complete loss of the SI system (Dempewolf et al., 2012). Another group of crops have changes in their reproductive strategy due to their level of polyploidy (Meyer et al., 2012), which leads to partial sterility and promotes agamospermy (Richards, 1997). In the case of SI species, artificial selection favors SC in order to select rapidly and achieve cultivar uniformity of a desirable phenotype is associated with the costs of inbreeding (Rick, 1988; Bixby and Levin, 1996). This change in the mating system is sometimes accompanied by changes in floral morphology that favor self-pollination such as reduction in floral size and in stigma exertion (Rick, 1988).
The Solanaceae is one of the main plant families with many domesticated species. Its large family comprises 2,700–3000 species widely distributed in tropical and subtropical regions around the world, with most of the species concentrated in America (Nee, 1993; Olmstead et al., 2008; Särkinen et al., 2013). Many solanaceous species belonging to the genera Capsicum and Solanum have been subjected to artificial selection for food production on a global or regional scale. For example, other Solanaceae genus, as Physalis, possesses attractive characteristics in its edible fruit to local consumption. Also, it has been reported that the Solanaceae display an RNase-based SI gametophytic system (i.e., RNases as the mechanism of self-pollen recognition and rejection), which was the ancestral state in most eudicots (Igic and Kohn, 2001). In the Solanaceae SI has been lost a minimum of 60 times (Igic et al., 2006). Once the SI system is lost, it is irreversible (Igic et al., 2003, 2006). For example, in Solanum sect. Lycopersicon a recent transition to SC has been documented (Igic et al., 2008). The cultivated tomato, Solanum lycopersicum, is considered SC but its wild relatives are SI, or have a mixed mating system (Igic et al., 2008; Bedinger et al., 2010).
Nowadays, it is estimated that 39% of Solanaceae species are SI (Igic et al., 2006), the rest of the species are SC (e.g., Capsicum: Pickersgill, 1997) or exhibit a transition to SC with diverse mating systems (e.g., Solanum sect. Lycopersicon; Bedinger et al., 2010). The Solanaceae, thus, provides an excellent opportunity to study the transition from SI to SC as related to artificial selection. In particular, one interesting study model is Physalis philadelphica Lam. (Solanaceae). This husk tomato is a partially self-incompatible plant that was domesticated in Mexico, where it is important to both economy and culture (e.g., in Mexican gastronomy; Montes-Hernández and Aguirre-Rivera, 1994). This plant possesses a gametophytic self-incompatibility system (GSI) (i.e., phenotypic recognition determined by a haploid gametophyte; Pandey, 1957) controlled by an S-locus (Mulato-Brito et al., 2007). The GSI system is characterized by late recognition of self-pollen tubes at the pistil. Pollen grains placed on stigmas by self-pollination germinate but the growth of pollen tubes is limited to the first third of pistil (Richards, 1997; Carvalho et al., 2020). It is known that the common ancestor of Physalis and Witheringia suffered a historical bottleneck that reduced S-allele diversity in three S-lineages, and its subsequent rapid diversification resulted in current species with a number of alleles similar to other Solanaceae genera (Igic et al., 2003).
Although P. philadelphica has been categorized as a self-incompatible species, it is possible to find some self-compatible individuals in husk tomato crops and wild populations (Pandey, 1957; Labate and Robertson, 2015). This may be attributed to a mutation in the S locus (Mulato-Brito et al., 2007). Thus, P. philadelphica is considered a partially self-incompatible species, and it has wide morphological variation in flowers and fruits between wild and cultivated plants (Hudson, 1986; Montes-Hernández and Aguirre-Rivera, 1994). This species presents an evolutionary gradient from wild plants, landraces, and crops (Zamora-Tavares et al., 2015) as well as different reproductive strategies. Consequently, P. philadelphica makes a suitable model for the study of changes in reproductive strategies associated with different degrees of domestication.
This study aimed to investigate changes in the reproductive system and parameters in wild (weedy plants), semi-domesticated (landraces), and domesticated plants (commercial plants) of P. philadelphica. We hypothesized that artificial selection favors self-compatibility in cultivated plants by changing their reproductive strategy and some reproductive parameters associated with domestication. To test this hypothesis, we first characterized their floral morphology and reproductive parameters of weeds, landraces, and commercial plants. Next, we conducted an artificial crossing experiment among plants with different degrees of domestication. Then, the seeds obtained from this experiment were germinated and seedling growth was recorded.
Materials and Methods
Biological Material
In this study, we classified plants of different degrees of domestication considering the habitat in which they grow, namely natural or crop areas, as well as morphometric and genetic characteristics as classification criteria. We classified plants as weedy plants, landraces, and commercial plants. Weedy wild plants are those which grow spontaneously in forest or milpa agroecosystems (i.e., agroecosystems where corn, beans and squash planted in association). They produce small fruits (<2 cm) and flowers (1.2–1.9 cm of corolla diameter). Natural populations of weedy plants are not subjected to artificial selection (non-domesticated populations), but they sometimes grow in association with humans in disturbed habitats (i.e., ruderals). People usually pick their fruits to sell them in local markets or for home consumption (Pre-Stage 1) (Zamora-Tavares et al., 2015). In the context of our research, we referred to them as weedy plants (code OVP337, OVP274, PZ275B, and SN). Landraces are cultivated plants with a historical origin (tradition varieties), which have been locally adapted and associated with traditional farming. They have a distinct identity and are genetically diverse, but lack formal crop improvement (Camacho-Villa et al., 2005). These traditional plants are artificially selected and belong to semi-domesticated populations (Stage 2). We recorded the fruits of landraces as medium size (2.5–3.5 cm) and with different colors (yellow, green, and purple). They are referred to in our study as landraces (code OVP2007 and JS522). Commercial plants were also used as biological materials; their seeds were obtained from commercial companies (code JS1, JS7, JS35, and JS347). The fruits of commercial plants were the largest (>3.5 cm). The green fruits are usually the most popular ones and are marketed on a larger scale in the country. The purple or green ones with purple hues are rather commercialized in the western region of Mexico (domesticated populations or Stage 3). Only JS347, which is a hybrid obtained by the Universidad Autónoma Chapingo, was considered to be in Stage 4. Herbarium specimens were deposited in ECO-TAP (Table 1).
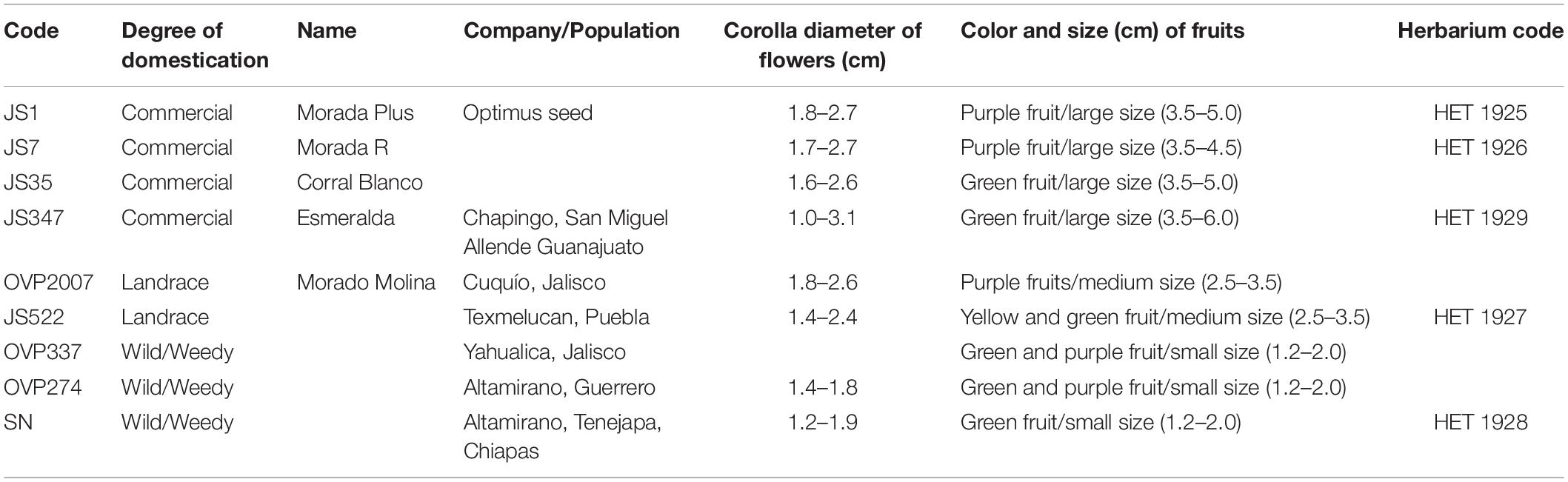
Table 1. Characteristics of flowers (corolla diameter range) and fruits (color and size), and origin of seeds germinated in greenhouse for description of reproductive systems.
Physalis philadelphica seeds were collected at different places from 2009 to 2012 or were bought from different companies in 2009 (Table 1). When we collected weedy plants, depending on population size, we picked some fruits from 20 to 50 individuals to produce a homogenous and representative sample. All fruits collected in one site were placed in a paper bag to transport to the laboratory. Later seeds were obtained and mixed to make a homogenous sample. In the case of the landraces, we sampled 30 plants of each traditional crop, taking care that they were in different rows and separated from each other. From each plant, we harvested one fruit and mixed them to make up the representative sample of each site. Finally, seeds from commercial plants were bought from different companies as described in Table 1. Seeds were stored at 4°C until planting.
Plant Growth
To obtain plants to describe the modification in reproductive systems during the domestication process, we germinated seeds of plants with different degrees of domestication: commercial plants (JS1, JS7, JS35, and JS347), landraces (OVP2007 and JS522), and weedy plants (OVP337, OVP274, PZ275B, and SN). We sowed 10 to 20 seeds per section at three times: January 2016, August 2016, and December 2017. The seeds were previously soaked in tap water for 2 h and, subsequently, sowed in germination trays in the greenhouse at El Colegio de la Frontera Sur (ECOSUR; 14° 53′ 194″ N, 92° 17′ 18″ W, 152 masl), Tapachula, Chiapas, Mexico. After 2 weeks, plants were transplanted into pots (5 cm diameter) and, after four more weeks, they were transplanted into larger pots (15 cm of diameter) with slow-release fertilizer (NUTRI-TABS, Miracle-Gro, Netherland) until they began to bloom. We conducted the experiments described below and measured floral traits during three periods: February to April 2016, September to November 2016, and January to March 2017.
Floral Morphology Variation Between Weedy and Cultivated Plants
This experiment was performed to determine the variation in floral morphology during the domestication process. We measured 2–3 flowers from 5 to 7 individuals per accession (a total of 134 flowers from 46 individuals) from September to November 2016. We measured the following 15 traits using a digital caliper (Steren) when flowers were completely open: (1) length (LS) and (2) width of one sepal (WS); (3) corolla diameter (CD); (4) length (LA) and (5) width of one anther (WA); (6) length (LF) and (7) width of the anther filament (WF); (8) length (LS) and (9) width of style (WS); (10) stigmatic area (STA); (11) length (LO) and (12) width of ovary (WO); (13) length (LN) and (14) width of nectary guides (WN); and (15) distance between anthers and stigma (herkogamy). In addition, we measured the size of 5 pollen grains per flower.
Pollen: Ovule Ratio Between Weedy and Cultivated Plants
In this experiment, we calculated the pollen: ovule ratio (i.e., the ratio of the amount of pollen to the number of ovules in the flower; Erbar and Langlotz, 2005). We counted the number of ovules from the same flowers used in the floral morphology section and the number of pollen grains from anthers previously collected to dehiscence during the second and third season (6–11 flowers per accession). We placed one anther per flower in a 1 ml tube with a pinch of powdered detergent (Salvo, Mexico). This was then macerated, and the anther tissue was eliminated (Márquez-Guzmán et al., 2016). We counted the number of pollen grains with a Newbauer chamber using a microscope (AxioLab A1, Zeiss, Germany). Counting was performed four times per sample. We obtained an average per sample to extrapolate for the initial volume. Later, we multiplied this number by five anthers to obtain the total amount of pollen per flower.
Artificial Crossing Experiment
We conducted experimental manipulation in a greenhouse, which excluded plants from pollinators, to investigate change in the P. philadelphica reproductive system. As flowers opened (i.e., corolla was completely expanded usually within 2 days after anthesis) and the style extended its wet stigma, the following treatments were applied from 9:00 to 14:00 h following Eckert et al. (2010). Some modifications were made as further described. (1) Emasculation (E). The anthers were removed before dehiscence at the floral-bud stage or on the first day of anthesis. This was used to determine whether different accessions of P. philadelphica set seed without pollination (i.e., through agamospermy). (2) Pollinator exclusion (PE). Flowers were left intact until senescence. The purpose of this treatment was to assess the plant’s ability to self-fertilize in the absence of pollinators (i.e., autonomous autogamy). To quantify the pollen deposited autonomously on the stigma of the flowers previously used for morphological measurements, the terminal end of the style, together with the stigma, was cut and placed on a slide with fuchsine-stained gel (Kearns and Inouye, 1993). (3) Hand-mediated self-pollination (S). The pollen, from the same flower, was deposited, on its stigma. The purpose of doing this was to assess the plant’s ability to accept or reject its own pollen grains (i.e., self-compatibility system). (4) Hand-mediated outcross pollination (CP). Pollen used in this treatment was gathered from three individuals from a different accession. The flowers that received this pollen were previously emasculated. In hand-mediated pollination, pollen was deposited on receptive stigmas to maximize grain deposition. Specifically, the white pollen was added until they were visible to the naked eye. Commercial, landrace, and weedy accessions were inter-crossed so individuals of each accession were pollen donors (male parent) and recipients (female parent). Each treatment was carried out in duplicate on 15 individuals per accession in each season.
After the artificial crossing experiment had been carried out, we quantified the fruit set. Subsequently, we weighed and measured (length and width) the fruits. Later, we quantified the seed sets by distinguishing between viable and aborted seeds. We weighed only the viable seeds, and sowed 50 seeds per treatment (in total 550 seeds) following methods previously described.
Seeds Germination and Seedling Growth
We recorded the day the seeds germinated and calculated the germination rate during 15 days of observation. Moreover, we recorded the day after sowing (DAS) that cotyledons and the embryonic foliage leaves emerged (Valdivia-Mares et al., 2016). In addition, we measured seedling height when cotyledons emerged (i.e., from base of seedling to the point of cotyledons emergence) and one week after germination. We measured the length of one cotyledon a week after germination and the length of one embryonic foliage leaf five days after their emergence (i.e., from cotyledon or leaf base to apex). Finally, we calculated the proportion of seedlings that survived 23 days after sowing.
Statistical Analysis
For the analyses, we used R statistical software, ver. 3.6.0 (R Core Development Team, 2019). We conducted a principal components analysis (PCA) with floral trait measurements to establish whether flower size differed in P. philadelphica according to domestication degree. We used seven separate linear mixed models (LMM) to analyze the difference between the reproductive parameters measured in the three degrees of domestication commercial, landrace, and weedy plants as shown in Supplementary Table 1. Each model used the degree of domestication as a fixed effect, with plant identity and population origin as random effects. Random effects that were not significant were eliminated from each model. The variable used as a fixed effect was log-transformed. For each model, we conducted an analysis of variance (ANOVA) to determine statistical differences and the Tukey test using the glht function from the multcomp library (Hothorn et al., 2008). Mixed models were developed using the lmerTest package (Zeileis and Hothorn, 2002). The variance and covariance of the random effects were obtained using the ranef function (package lme4).
In addition, we conducted 17 separate generalized linear models (GLM) to analyze the difference in fruit set, seed set, and percentage of germination among treatments. We fit fruit set and percentage of germination with binomial error and seed set with Poisson error. Treatments without fruit production were excluded. In addition, we calculated the confidence intervals using the Hmisc package to compare the fruit sets between the emasculation and the self-pollination treatments (Harrell and Dupont, 2019). We conducted 12 separate GLM analyses fitted with Gaussian error to analyze the difference in fruit weight, length, width, and viable seed weight among treatments of artificial crosses. Afterward, we conducted multiple comparisons of means (Tukey contrasts) using the glht function from multcomp library. We conducted a survival analysis (Kaplan-Meier method) using the survival package (Therneau and Grambsch, 2000) in order to analyze whether the probability of germination differed among treatments over 15 days of observation as well as the probability of seedling survival among treatments over 23 days of observation. To graph, we used the survival probability using the survminer package (Alboukadel and Kosinski, 2019). In the case of the germination graph, we used the inverse of survival probability. We used 10 separate GLM fit with Poisson error and Tukey test to compare time of emergence of cotyledons and the embryonic foliage leaves. Finally, we used six separate GLM fit with Gaussian error to analyze the difference in plant height at the time cotyledons emerged and one week after germination. We used another six separate GLM fit with Gaussian error to analyze the difference in cotyledon and embryonic foliage leaf size.
Results
Floral Morphology Variation Between Weedy and Cultivated Plants
The PCA showed that the first component (PC1) could explain 57.3% of the variance in floral morphology. Size was represented by this component as all eigenvalues have similar signs and values (Supplementary Table 2). The ANOVA with the PC1 scores showed that commercial individuals had the largest flowers, while weedy individuals had the smallest ones (F2,131 = 92.04, P < 0.001). Landraces had intermediate size flowers compared with the other two degrees of domestication (Figure 1). No differences in other reproductive parameters were found (Table 2), except for the number of ovules, which was found to be higher in commercial plants than in landraces. Weedy plants had the lowest number of ovules (Table 2; Supplementary Table 1).
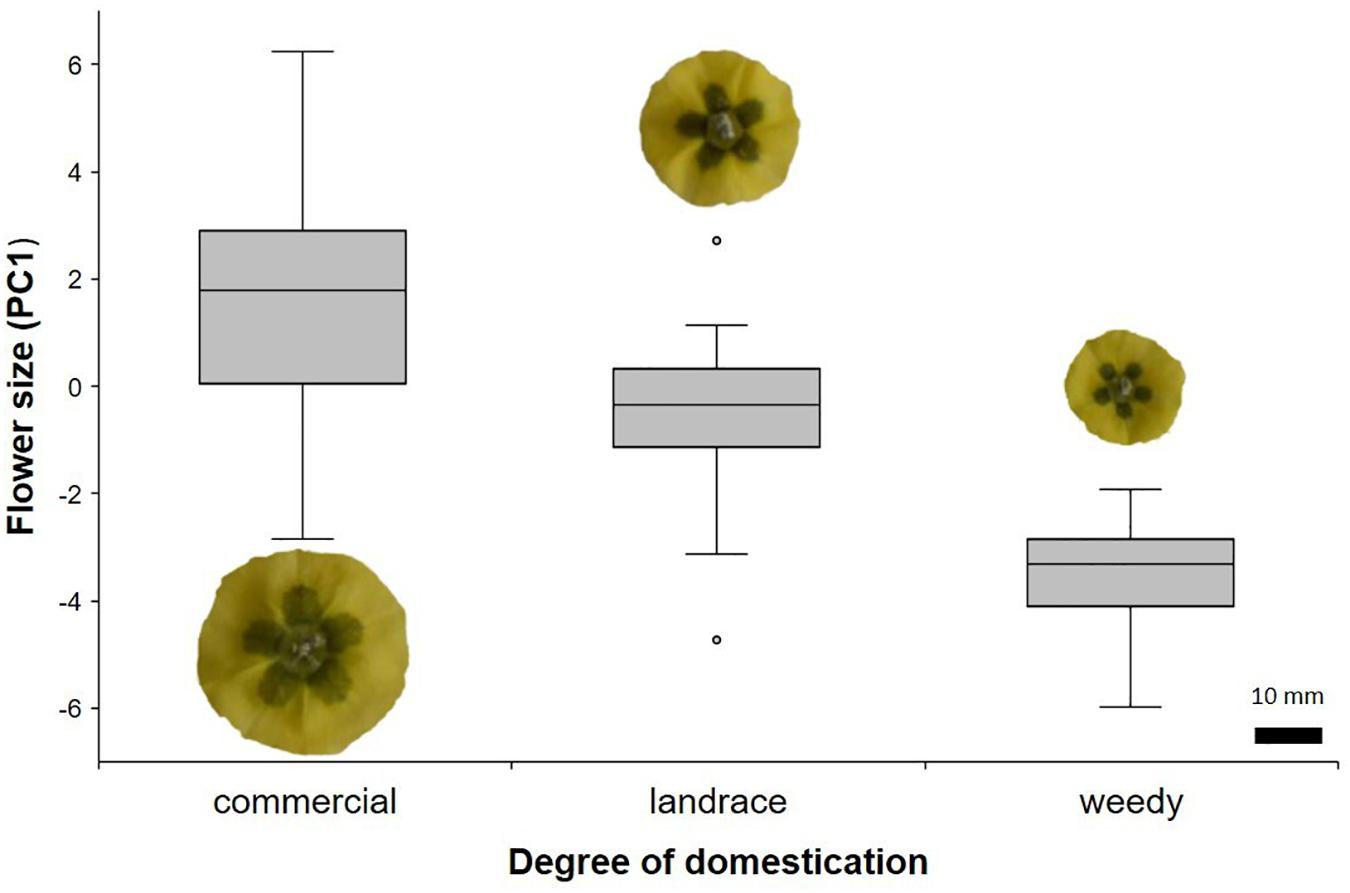
Figure 1. Flower size among different degrees of domestication in Physalis philadelphica represented by scores of the first principal component.
Artificial Crossing Experiment
Physalis philadelphica did not produce fruits through agamospermy (Table 3). Landraces of this species did not develop fruits from hand-mediated self-pollinated flowers. In the same treatment, weedy and commercial plants produced very few fruit sets (0.8% each treatment). These results were not different from those obtained in the emasculation treatment CI commercial plants (0–0.06) and in hand-mediated self-pollination (0.008–0.003). They did not differ from the emasculation treatment CI weedy plants (0–0.28) and hand-mediated self-pollination (0.0004–0.04). Conversely, we found that all degrees of domestication produced fruits when they were excluded from pollinators. Weedy plants produced the highest fruit set (2.4:1, ratio of SC to SI individuals), followed by landraces (1:1.3) and commercial plants (1: 8.2). These fruits, which represent all degrees of domestication, were the smallest and the lightest (Table 4). The outcross pollination treatments differed significantly when commercial plants functioned as pollen receptors and landraces or weedy plants functioned as pollen donors. The highest percentage of fructification was obtained when weedy plants donated pollen to commercial plants (Table 3).
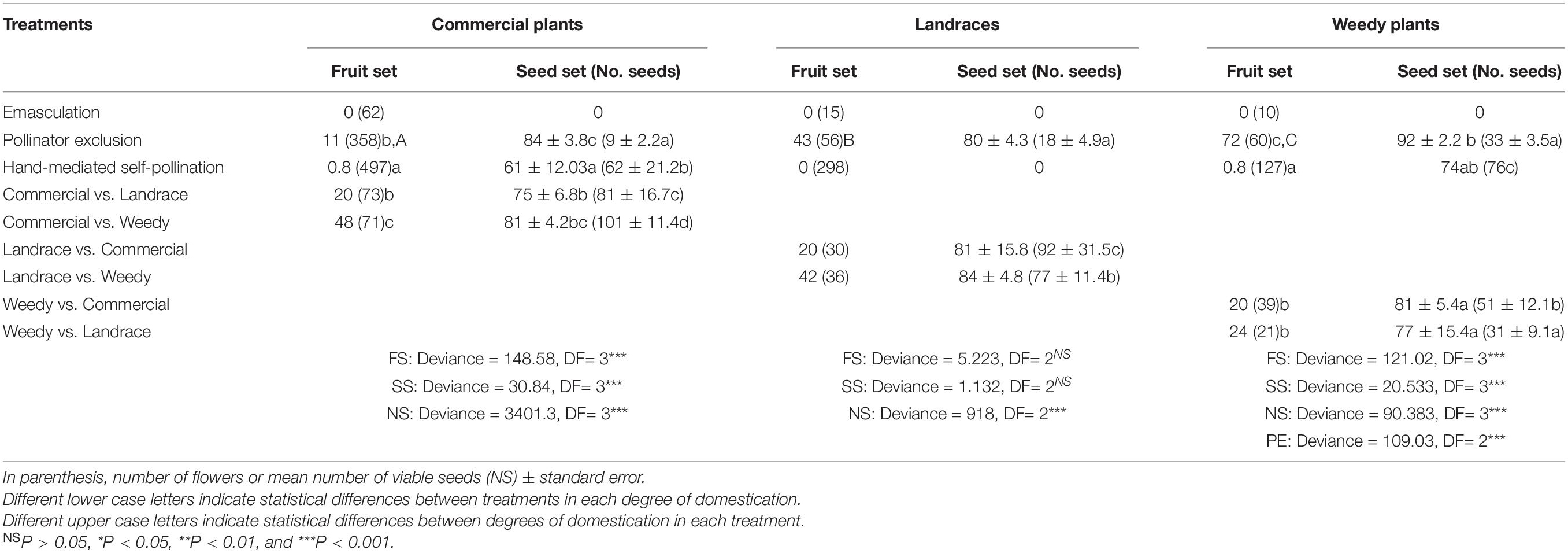
Table 3. Fruit set (FS; percentage of flowers maturing into fruits) and mean seed set (SS; percentage of viable seeds) of artificial crosses in Physalis philadelphica with different degrees of domestication.
In commercial plants, a significant difference in seed set was found between the seed sets from the pollinator exclusion treatment and those from the outcross pollination treatment when landraces donated pollen. These treatments resulted in the highest and the lowest values, respectively (Table 3). As we expected, the lowest numbers of viable seeds were produced in the pollination exclusion and hand-mediated self-pollination treatments. In contrast, the highest number of seeds was found in the outcross treatment when weedy plants donated pollen (n = 101 viable seeds). With regard to landraces, there were no differences in seed sets among treatments (Table 3). The highest number of viable seeds was obtained between landraces and commercial plants (92) in the outcrossed treatment. The lowest number was obtained in the pollination exclusion treatment (18). This treatment produced the heaviest seeds. Finally, in weedy plants the seed set did not differ between outcross pollination treatments, but it was higher in the pollination exclusion treatment. The lowest number of viable seeds was found in the pollination exclusion (33) and outcross treatments (31) when landraces functioned as pollen donors. The highest number of viable seeds was found in the self-pollination treatment (76).
Germination
The seeds resulting from outcrossing between landraces and commercial plants showed the highest germination percentage, followed by the hand mediated self-pollination treatment in commercial plants and outcrossing between landraces and weedy plants (Table 5). In these three treatments, most of the seeds germinated between day 4 and 5 (Figure 2). Conversely, seeds produced by spontaneous self-pollination in weedy plants had the lowest germination percentage, followed by outcrossing between commercial and weedy plants and the reciprocal cross. Seeds of spontaneous self-pollination and commercial and weedy crosses germinated on day 3 and 7 after sowing (Figure 2).
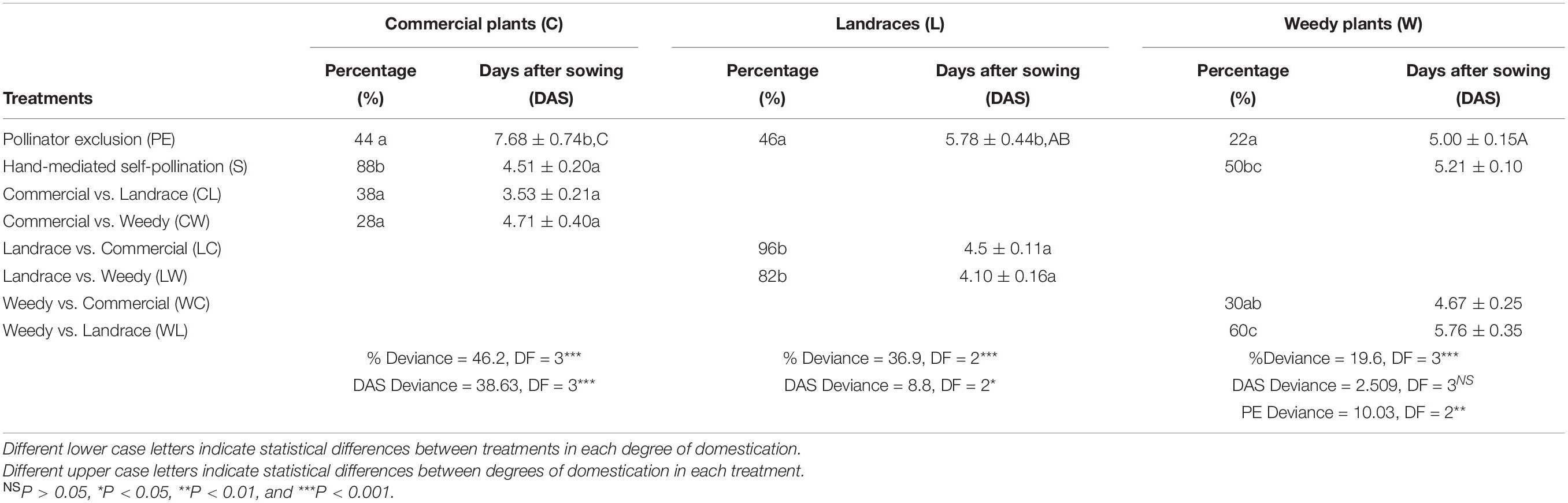
Table 5. Percentage of germination of artificial crosses in Physalis philadelphica with different degrees of domestication.
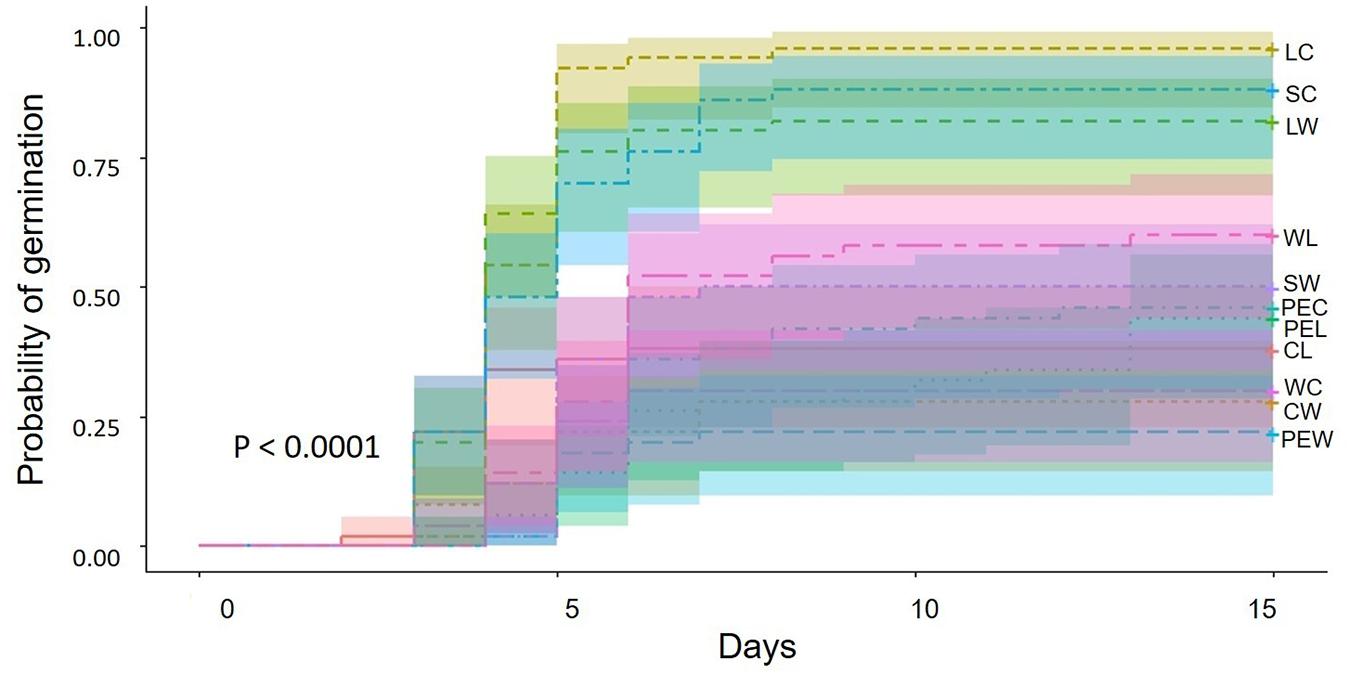
Figure 2. Germination probability and intervals of confidence of seeds produced by artificial crossing of Physalis philadelphica during 15 days of observation. Abbreviations: LC (landraces vs. commercial plants), SC (self-pollination in commercial plants), LW (landraces vs. weedy plants), WL (weedy plants vs. landraces), SW (self-pollination in weedy plants), PEC (pollinator exclusion in commercial plants), PEL (pollinator exclusion in landraces), CL (commercial plants vs. landraces), WC (weedy vs. commercial plants), CW (commercial vs. weedy plants), PEW (pollinator exclusion in weedy plants).
Emergence of Cotyledons and Embryonic Foliage Leaves
In plants resulting from the commercial plant pollinator exclusion treatment, the cotyledons and the embryonic foliage leaves emerged later than those in weedy plants (Table 6). However, cotyledon emergence in landraces was not different from that in commercial and weedy plants. Emergence of the embryonic foliage leaves differed only in commercial plants.
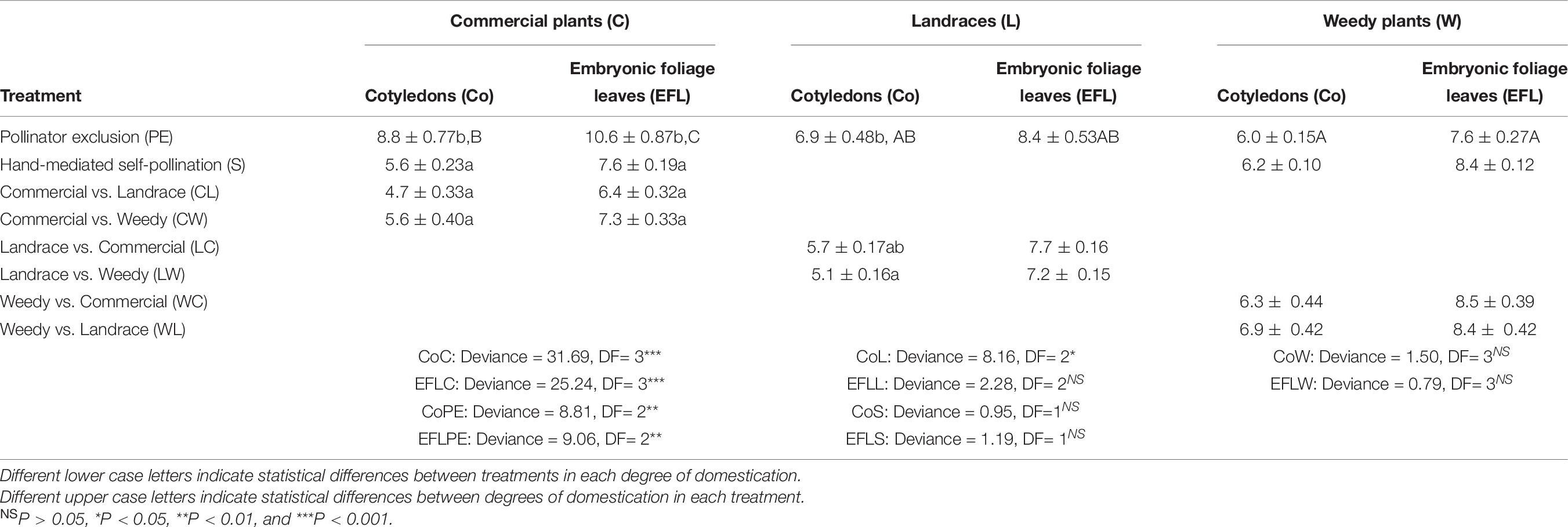
Table 6. Mean days after sowing (DAS) of cotyledon emergence and mean DAS of appearance of embryonic foliage leaves (±standard error) of artificial crosses in Physalis philadelphica with different degrees of domestication.
When comparing all treatments in the same degree of domestication, it was found that, in commercial plants, the cotyledons and the embryonic foliage leaves of the pollinator exclusion treatment emerged later than those of the other treatments. With regard to landraces, there were differences in cotyledon emergence. Cotyledons of plants resulting from the pollinator exclusion treatment emerged later than those from outcrossing between landraces and weedy plants. However, they did not differ in the crosses between landraces and commercial plants treatments. Finally, emergence of cotyledons and embryonic foliage leaves did not differ among other treatments in weedy plants.
Seedling Growth
When cotyledons emerged, seedling height did not differ among treatments in commercial plants (Table 7), but differences in seedling height were observed in landraces and weedy plants. In landraces, the seedlings resulting from the pollen exclusion treatment and those from the cross between landraces and weedy plants were taller than those of the cross between landraces and commercial plants. Weedy seedlings resulting from the hand-mediated self-pollination treatment were shorter than those in all of the other treatments.
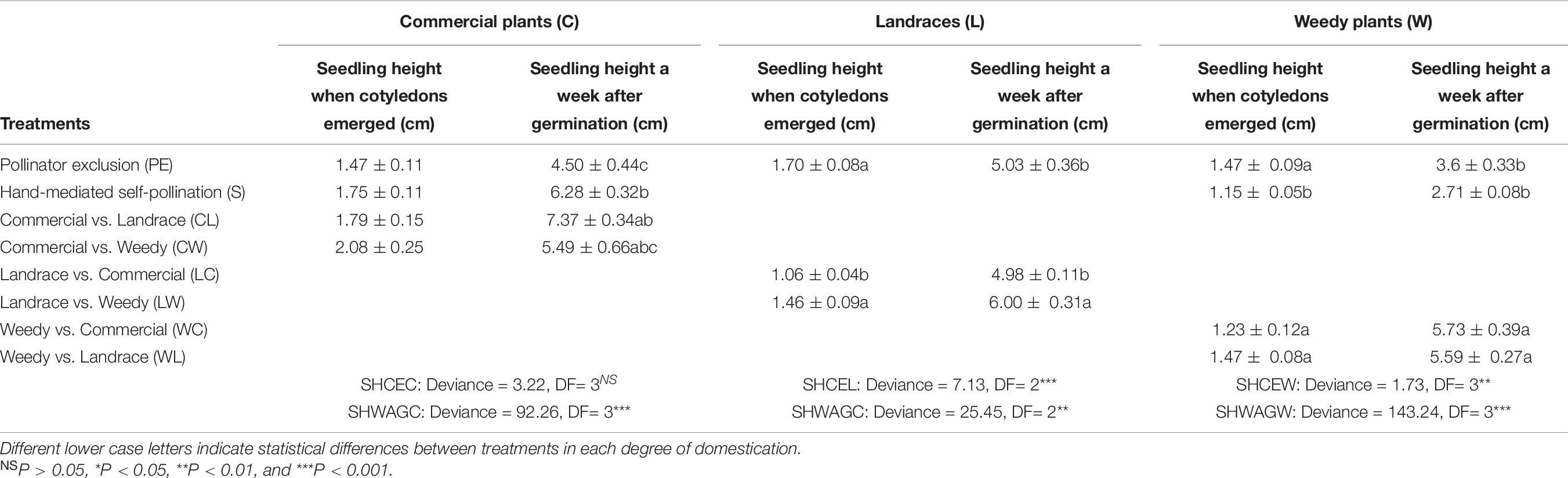
Table 7. Mean seedlings height when cotyledons emerged (SHCE) and a week after germination (SHWAG) (±standard error) resulting from different treatments of artificial crosses experiment.
Although this pattern changed, when we measured seedling height one week after germination, seedlings resulting from autonomous autogamy in commercial plants were the shortest. They did not differ from those plants resulting from crosses commercial and weedy plants (Table 7). The seedlings resulting from crosses landraces and weedy plants were taller than landraces used in the other treatments. Finally, seedlings resulting from the crosses between weedy vs. commercial plants and weedy plants vs. landraces were almost double in size compared to seedlings from the autogamy treatments (pollination exclusion and hand-mediated self-pollination).
Cotyledon and embryonic foliage leaf size (length) of seedlings resulting from autogamy were smaller than those of outcrosses between commercial plants and landraces and their reciprocal cross (Table 8). However, in both variables weedy seedlings resulting from autogamy were not different from those in outcrosses. Finally, there was no significant difference in the probability of seedling survival (P = 0.57) among treatments over 23 days of observation (93–100% of the seedlings survived; Table 8).
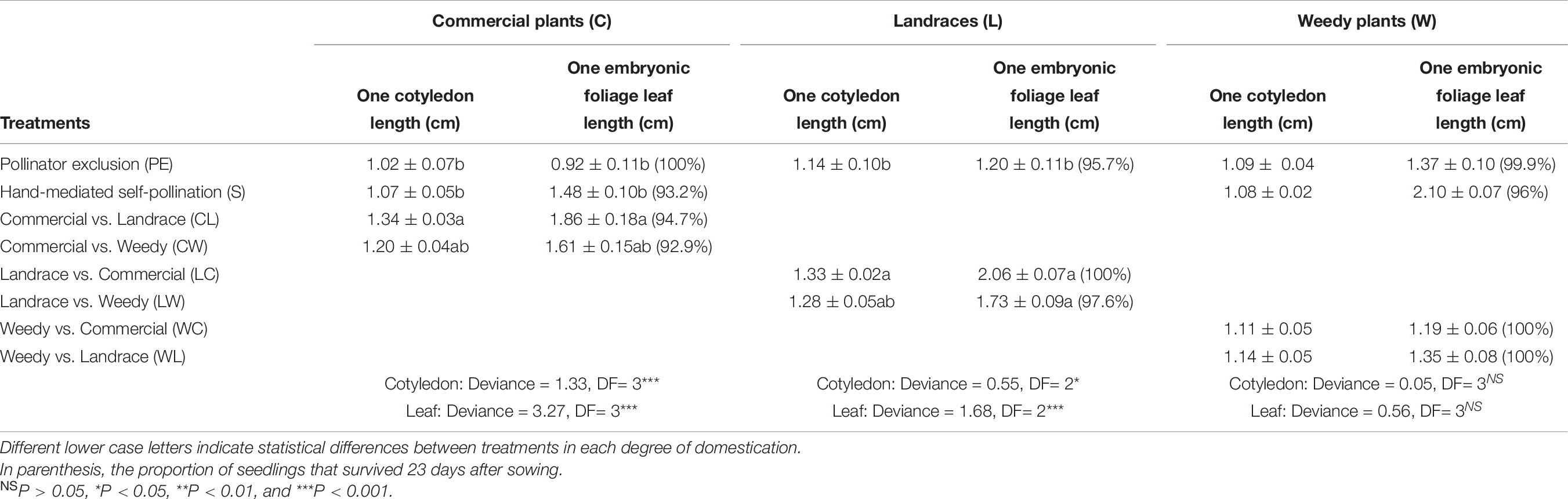
Table 8. Mean left cotyledon length a week after germination and left embryonic foliage leaf length 5 days after emergence (±standard error) resulting from different treatments of the artificial crossing experiment.
Discussion
Since the domestication process prefers self- to cross-fertilization, artificial selection usually suppresses or removes self-incompatibility and outcrossing traits (Dempewolf et al., 2012). Contrary to our expectations, by comparing other domesticated species in the Solanaceae, we found that in P. philadelphica artificial selection favors SI over SC. Weedy plants presented higher fruit set produced by autonomous autogamy compared to cultivated plants. Spontaneous self-pollination seems to be advantageous in weedy populations because it produces viable seeds, with cotyledons and embryonic foliage leaves emerging before those of cultivated plants. These changes in the reproductive strategy are also associated with increments in flower size and ovule production indicative to the domesticated syndrome.
Changes in Reproductive Strategy During Domestication
The domestication syndrome is defined as crops having traits that diverge from wild relatives, including those associated with changes in reproductive strategies (Meyer et al., 2012). In this study, we found that cultivated plants of P. philadelphica differ from weedy plants in flower size and ovule numbers. Reduction in floral size is related to a reduction in distance between anthers and stigma, usually associated with an increase in self-fertilization. For example, in six SC species of Solanum section Androceras transition from large to small flowers and lower P:O ratios suggest a transition toward self-fertilization (Vallejo-Marín et al., 2014). It seems that the breakdown of SI is followed by a rapid evolution of selfing attributes, such as a reduction in flower size (Igic et al., 2008) and floral longevity (Broz et al., 2017). For example, it has been reported that some SC species of Solanum sect. Lycopersicon show decreased flower size and stigma exertion (Rick, 1988; Bedinger et al., 2010). Regarding the number of ovules, commercial P. philadelphica plants produce three times more ovules than weedy plants. However, weedy plants are proportionally more efficient in seed production considering the lower number of ovules. Similar results have been reported in cotton, Gossypium hirsutum, since commercial plants present twice as many ovules as wild plants despite producing a proportionally smaller number of seeds under natural pollination conditions (Velázquez-López et al., 2018).
In the artificial crossing experiment, our results suggest that P. philadelphica is a partially self-incompatible species and that domestication selects for SI in cultivars. Previously, Mulato-Brito et al. (2007) suggested that the self-incompatibility (SI) system is the common condition in cultivated plants, while the self-compatible (SC) system is common in wild plants of P. philadelphica. In this study, we confirmed this assumption. We found that P. philadelphica, in all degrees of domestication, could produce fruits autonomously when excluded from pollinators. In weedy plants, the proportion of self-compatible individuals was most responsible for producing the highest fruit set in all treatments. It has been reported that self-compatible individuals in cultivated plants of P. philadelphica (Pandey, 1957; Labate and Robertson, 2015) result from the S locus mutation (Mulato-Brito et al., 2007) causing an irreversible loss of the SI system (Igic et al., 2008). Instead, P. philadelphica has a labile reproductive system (self- and cross-fertilization) and may present a mixed system strategy, allowing fruit production by autogamy and allogamy (Carvalho et al., 2020). In small weedy populations of P. philadelphica, self-pollination would provide an advantage assuring some seed reproduction but under crop domestication artificial selection could favor SI individuals in the production of larger flowers and fruits.
Why Is It More Common to Find SI Individuals in Cultivated Plants of P. philadelphica Than in Weedy Plants?
Self-incompatibility in domesticated plants of P. philadelphica is atypical compared to the trend in SC in most domesticated species (Dempewolf et al., 2012). Solanum lycopersicum and Capsicum annum, for example, are SC species under domestication, but their wild populations are SI (Pickersgill, 1997; Bai and Lindhout, 2007; Bedinger et al., 2010). Conversely, it is more common to find SI individuals in cultivars of P. philadelphica than in weedy populations. One possible explanation for this is the loss of genetic diversity in domesticated plants compared with their wild relatives due to a bottleneck during domestication (Doebley, 1989; Cox, 2009). For example, the domesticated S. lycopersicum genetically depauperate while wild populations are rich genetic reservoirs (Bai and Lindhout, 2007). However, it has been found that genetic diversity of P. philadelphica is slightly higher in cultivated plants than in weedy and wild plants (Zamora-Tavares et al., 2015). This apparently contradictory evidence might be due to the higher proportion of self-incompatible individuals found in crops, which favors outcrossing and increases genetic diversity, compared with that of landraces and weedy plants. An example of this can be found in Arabidopsis lyrata as research has shown that the SI predominant populations present higher outcrossing rates than those observed in the predominantly SC populations. This shift in the mating system is also associated with a loss of genetic diversity (Mable et al., 2005). Future studies should characterize the mating system of P. philadelphica using molecular markers and their relationship to the degree of domestication.
Another possible explanation for this unexpected shift toward SI in cultivated plants of P. philadelphica is that SI individuals were artificially selected during the domestication process. Seeds and fruits with more desirable traits were collected by people (Doebley et al., 2006). In the case of SI plants, fruits are usually larger compared with those from SC plants (see above). It is probable that large fruits were artificially selected from SI individuals, inheriting incompatibility via the nucleus (Mulato-Brito et al., 2007). Self-compatible individuals in crops are most likely to appear later due to random mutations of the S locus retained by artificial selection.
Ecological Advantages/Disadvantages of Changes in P. philadelphica Reproductive Strategies
Variation in SI strength could allow SC individuals to reproduce when compatible mates or pollinators are limited (Mable et al., 2005). However, SC has been associated with costs such as inbreeding depression, pollen, ovule and seed discounting, and low genetic variation (Lloyd, 1992; Barrett, 2002). As spontaneous self-pollination ensures reproduction (Eckert et al., 2006), we suggest that SC individuals of P. philadelphica, which accept their own pollen by autonomous autogamy, can ensure some fruit production in all degrees of domestication if pollen is limited. This is to be anticipated in small wild populations that are usually ruderal with few individuals (Zamora-Tavares et al., 2015) and when compatible mates are probably limited. In other SI taxa in the Solanaceae, it is common to find SC individuals in small populations (Stone et al., 2006) where S-allele diversity is probably low, and the cost of SI is high (Vallejo-Marín and Uyenoyama, 2004).
Furthermore, we found a high number of self-deposited pollen grains on stigmas (Range = 54–191 pollen grains) in all degrees of domestication, confirming autonomous pollen deposition. This might be associated with the cost of pollen discounting (i.e., the reduction of the outcross pollen pool due to a higher contribution by self-pollinating to seed set) or to seed discounting (i.e., relative changes in the number of seeds set by outcrossing and self-pollination; Lloyd, 1992; Vallejo-Marín and Uyenoyama, 2004). If most of the pollen deposited on the stigma is self-pollen compared to outcross pollen, the seed set will be severely reduced in SI individuals (Vallejo-Marín and Uyenoyama, 2004). Therefore, it is relevant to study pollen deposition and the pollination ecology of P. philadelphica undergoing different degrees of domestication.
Germination percentage in self-pollinated wild species of Physalis (e.g., Physalis acutifolia, Physalis chenopodifolia, and Physalis pubescens) is high. They also present a high percentage of plant establishment under greenhouse conditions (Valdivia-Mares et al., 2016). In P. philadelphica, fruit and seed production by spontaneous self-pollination is similar or higher compared to those in outcrossing treatments in all degrees of domestication, yet fewer seeds are produced. Furthermore, these surviving seeds germinate at a lower or similar percentage than outcrossed seeds, depending on the degree of domestication. Another study reported that Physalis seeds show a secondary or induced latency following cold storage, which is more marked in the wild (Sánchez-Martínez et al., 2018). Researchers suggest that this type of latency in wild species provides plants with a mechanism to survive temperature fluctuations, whereas cultivated species have lost this characteristic through domestication. Moreover, Ozaslan et al. (2017) have indicated that P. philadelphica seeds germinate under a wide range of environmental conditions including extremes of ambient temperature, pH, water levels and soil salinity. This helps explain the invasive potential of this plant in Turkey.
In weedy populations of P. philadelphica, cotyledons and embryonic foliage leaves resulting from spontaneous self-pollination emerged before those of landraces and commercial plants in the same treatment in this study. In addition, in early stages of seedling development (cotyledon emergence) they did present differences in height compared with outcrosses, but then they were surpassed by outcross seedlings a week following germination. The cotyledon and embryonic foliage leaf size in seedlings resulting from autonomous autogamy did not differ from outcrosses, nor did they differ in terms of survival probability and proportion. This suggests that in weedy plants low inbreeding depression in seeds, resulting from autonomous autogamy, is to be expected in partially self-fertilized species (Charlesworth and Charlesworth, 1987). Similar results have been obtained from self-compatible Physalis angulata, whose low inbreeding depression index (δ = 0.27) makes self-pollination favorable (Carvalho et al., 2020). Although early emergence of cotyledons and leaves could provide an advantage for plant establishment in weedy plants, seedling germination and growth, reported in this study, occurred in a greenhouse under controlled conditions. There are certain limitations in assessing plant competition under greenhouse conditions. Further research may consider these variables in field conditions taking into account plant density (low in weedy populations vs. high in crops) as well as water and nutrient availability (Gurevitch et al., 2006).
Within the Solanaceae some species in some genera such as Capsicum and Solanum present unilateral incompatibility (i.e., pollen of an SC population is rejected on flowers styles of an SI population but not in reciprocal crosses). This makes hybridization difficult (Pickersgill, 1997; Bedinger et al., 2010; Samuels, 2012). Unilateral incompatibility represents a barrier between SI and SC populations of the same or different species, and it evolved as a stage in which SI is lost (Bedinger et al., 2010). It seems that in P. philadelphica unilateral incompatibility was not present, or it was weak. When weedy, most SC plants donated pollen to commercial SI plants, and higher fruit production occurred (see above). Thus, artificial selection favors the production of larger but fewer fruits compared to their partially SI progenitors (Doebley et al., 2006). The finding in this study shows that crosses between commercial and weedy plants could increase crop productivity probably with desirable phenotypes, as it is the case of domesticated Helianthus. When domesticated Helianthus was crossed with wild relative, Helianthus mollis, their progeny tended to resemble the domesticated parent while progeny of the reciprocal cross favored H. mollis phenotype (Faure et al., 2002).
Possible Implications of Gene Flow Among Different Degrees of Domestication
Cultivated and wild husk tomato distributed mainly throughout Mexico (Montes-Hernández and Aguirre-Rivera, 1994; Zamora-Tavares et al., 2015; SIAP, 2018), and they are sympatric. This might increase the probability of crosses between weedy and domesticated plants, but gene flow has been found to be low (Zamora-Tavares et al., 2015). Gene flow between wild and semi-domesticated plants may ultimately be useful to traditional and commercial crops as it could increase a crop’s genetic diversity in the future. It could also expand its adaptive traits relevant to pest and/or disease resistance and environmental stress (Rick, 1988; Cox, 2009; Warschefsky et al., 2014; Purugganan, 2019). The SI system of P. philadelphica is a factor that limits crop improvement. Therefore, it is desirable to find self-compatible genes present in landraces (Mulato-Brito et al., 2007). As reported above, there is a high probability of finding compatible individuals in weedy and landrace populations. However, gene flow in the opposite direction (from crops to landraces and wild populations) might be dangerous in a context involving introduction of transgenic crops, evolution of aggressive weeds, or increased extinction of wild species (Ellstrand et al., 1999; Alavez-Gómez et al., 2009; Velázquez-López et al., 2018). From our findings, it is important to emphasize that fruit and seed set is higher when commercial plants receive pollen from weedy plants and landraces rather than when crossing occurs in the opposite direction. In the field, plants with different degrees of domestication are in sympatry, and thus it is necessary to study pollen flow among them and the survival and identification of their hybrids under field conditions.
Conservation of P. philadelphica
Physalis philadelphica could be a suitable model system to explore how artificial selection favors SI in some cultivated plants. It is of relevant importance to conserve the gradient of domestication found in Mexican territories. The conservation should be in situ (in ecosystems and crops) and ex situ (seed banks and botanical gardens) in order to preserve the genetic reservoir of this species due to its high genetic diversity as reported under different degrees of domestication (Alavez-Gómez et al., 2009; Meyer et al., 2012; Zamora-Tavares et al., 2015).
Data Availability Statement
The datasets presented in this study can be found in online repositories. The names of the repository/repositories and accession number(s) can be found in the article/Supplementary Material.
Author Contributions
LS-M: experiment design, data analysis and manuscript writing. LA-C: experiment design and conduction. MV-P: floral morphology characterization. OV-P: seed collection and manuscript reviewing. All authors contributed to the article and approved the submitted version.
Funding
LA-C was supported by a scholarship (Grant No. 12991) from the Consejo Nacional de Ciencia y Tecnología (CONACYT).
Conflict of Interest
The authors declare that the research was conducted in the absence of any commercial or financial relationships that could be construed as a potential conflict of interest.
Acknowledgments
We thank Danika Mateo, Fernando García, and Berenice Vázquez for their assistance in laboratory work, and Mario Vallejo-Marín and Julio Rojas for their comments and suggestions for early version of the manuscript. Also, we thank to Thomas Miedaner, Peter Bernhardt, and Michael Kantar, for their comments and suggestions in the review of manuscript. We are very grateful to Gillian Flint, Magdalena Escobar, and Diane Fumiko to English edition. In memory of Bulmaro Cadena, a husk tomato farmer from Puebla, Mexico.
Supplementary Material
The Supplementary Material for this article can be found online at: https://www.frontiersin.org/articles/10.3389/fpls.2021.658406/full#supplementary-material
References
Alavez-Gómez, V., Jardón-Barbolla, L. O., Moyers-Arévalo, L., Ortega del Vecchyo, D., Wegier-Briuolo, A. L., et al. (2009). Recopilación de Información Acerca de la Evolución del Género Physalis en México, del Origen y Diversidad de Physalis Philadelphica Lam. (Tomate Verde), Informe Final. Instituto de Ecología, UNAM y Universidad Autónoma de Querétaro. Dentro del Proyecto “Generación y Recopilación de Información de las Especies de las que México es Centro de Origen y Diversidad Genética”, Financiado por la Dirección General del Sector Primario y Recursos Naturales Renovables (DGSPRNR), Perteneciente a la SEMARNAT y Coordinado por la CONABIO. México: CONABIO, 98.
Alboukadel, K., and Kosinski, M. (2019). Survminer: Drawing Survival Curves using ‘ggplot2’. R package version 0.4.4.
Bai, Y., and Lindhout, P. (2007). Domestication and breeding of tomatoes: what have we gained and what can we gain in the future? Ann. Bot. 100, 1085–1094. doi: 10.1093/aob/mcm150
Barrett, S. C. H. (2002). The evolution of plant sexual diversity. Nat. Rev. Genet. 3, 274–284. doi: 10.1038/nrg776
Bedinger, P. A., Chetelat, R. T., McClure, B., Moyle, C. L., Rose, C. K. J., Stack, M. S., et al. (2010). Interspecific reproductive barriers in the tomato clade: opportunities to decipher mechanisms of reproductive insolation. Sex Plant Reprod. 24, 171–187. doi: 10.1007/s00497-010-0155-7
Bixby, P. J., and Levin, D. (1996). Response to selection on autogamy in Phlox. Evolution 50, 892–899. doi: 10.2307/2410860
Broz, A. K., Randle, A. M., Sianta, S. A., Tovar-Mendez, A., McCluere, B., and Bedinger, P. (2017). Mating system transitions in Solanum habrochaites impact interactions between populations and species. New Phytol. 213, 440–454. doi: 10.1111/nph.14130
Bye, R., and Linares, E. (2000). “Relationships between Mexican ethnobotanical diversity and indigenous people,” in Biodiversity and Native America, eds P. E. Minnis and W. J. Elisens (Norman: University of Oklahoma Press), 44–74.
Camacho-Villa, T. C., Maxted, N., Scholten, M., and Ford-Lloyd, B. (2005). Defining and identifying crop landraces. Plant Genet. Resour. 3, 373–384. doi: 10.1079/pgr200591
Carvalho, C. F. M., Rodrigues, P. A., Mendes, H. F., Souza, D. S. K., Leite, D. S. A., and Soares, T. L. (2020). Reproductive biology of Physalis angulata L. (Solanaceae). Sci. Hortic. 267:109307. doi: 10.1016/j.scienta.2020.109307
Casas, A., and Barbera, G. (2002). “Mesoamerican domestication and diffusion,” in Cacti: Biology and Uses, ed. P. S. Nobel (California: University of California Press), 143–162.
Charlesworth, D., and Charlesworth, B. (1987). Inbreeding depression and its evolutionary consequences. Annu. Rev. Ecol. Evol. Syst. 18, 237–268. doi: 10.1146/annurev.es.18.110187.001321
Clement, C. R. (1999). 1492 and the loss of Amazonian crop genetic resources. I. the relation between domestication and human population decline. Econ. Bot. 53, 188–202. doi: 10.1007/bf02866498
Cox, T. S. (2009). “Crop domestication and the first plant breeders,” in Plant Breeding and Farmer Participation, eds S. Ceccarelli, E. P. Guimarães, and E. Weltzien (Rome: Food and Agriculture Organization), 1–26.
Dempewolf, H., Hodgins, K. A., Rummell, S. E., Ellstrand, N. C., and Rieseberg, L. H. (2012). Reproductive insolation during domestication. Plant Cell 24, 2710–2717. doi: 10.1105/tpc.112.100115
Doebley, J. (1989). “Isozymic evidence and the evolution of crop plants,” in Isozymes in Plant Biology, eds D. Soltis and P. Soltis (Portland: Dioscorides Press), 165–191. doi: 10.1007/978-94-009-1840-5_9
Doebley, J. F., Gaut, B. S., and Smith, B. D. (2006). The molecular genetics of crop domestication. Cell 127, 1309–1321. doi: 10.1016/j.cell.2006.12.006
Eckert, C. G., Kalisz, S., Geber, M., Sargent, R., Elle, E., Cheptou, P. O., et al. (2010). Plant mating systems in a changing world. Trends Ecol. Evol. 25, 35–43. doi: 10.1016/j.tree.2009.06.013
Eckert, C. G., Samis, K. E., and Dart, S. (2006). “Reproductive assurance and the evolution of uniparental reproduction in flowering plants,” in Ecology and Evolution of Flowers, eds L. D. Harder and S. C. H. Barrett (Oxford: Oxford University Press), 183–203.
Ellstrand, N. C., Prentice, H. C., and Hancock, J. F. (1999). Gene flow and introgression from domesticated plants into their wild relatives. Annu. Rev. Ecol. Syst. 30, 539–563. doi: 10.1146/annurev.ecolsys.30.1.539
Erbar, C., and Langlotz, M. (2005). Pollen to ovule ratios: standard or variation-a compilation. Bot. Jahrb. Syst. 126, 71–132. doi: 10.1127/0006-8152/2005/0126-0071
Faure, H., Serieys, E., Cazaux, F., Kaan, F., and Bervillé, A. (2002). Partial hybridazation in wide crosses between cultivated sunflower and the perennial Helianthus species H. mollis and H. orgyalis. Ann. Bot. 89, 31–39. doi: 10.1093/aob/mcf003
Gurevitch, J., Scheiner, M. S., and Fox, A. G. (2006). Competition. in the Ecology of Plants, 2nd Edn. Sunderland: Sinauer Associates.
Hothorn, T., Bretz, F., and Westfall, P. (2008). Simultaneous inference in general parametric models. Biom. J. 50, 346–363. doi: 10.1002/bimj.200810425
Hudson, W. D. (1986). “Relationships of domesticated and wild physalis philadelphica,” in Solanaceae: Biology and Systematics, ed. W. G. D’Arcy (Nueva York: Columbia University Press), 416–432.
Igic, B., and Kohn, J. R. (2001). Evolutionary relationships among self-incompatibility RNases. PNAS 98, 13167–13171. doi: 10.1073/pnas.231386798
Igic, B., Bohs, L., and Kohn, J. R. (2003). Historical inferences from the self-incompatibility locus. New Phytol. 161, 97–105. doi: 10.1046/j.1469-8137.2003.00952.x
Igic, B., Bohs, L., and Kohn, J. R. (2006). Ancient polymorphism reveals unidirectional breeding system shifts. PNAS 103, 1359–1363. doi: 10.1073/pnas.0506283103
Igic, B., Lande, R., and Kohn, J. R. (2008). Loss of self-incompatibility and its evolutionary consequences. Int. J. Plant Sci. 169, 93–104. doi: 10.1086/523362
Kearns, C. A., and Inouye, D. W. (1993). Techniques for Pollination Biologists. Colorado: University Press of Colorado.
Labate, J., and Robertson, L. D. (2015). Nucleotide diversity estimates of tomatillo (Physalis philadelphica) accession including nine new inbred lines. Mol. Breed. 35:106.
Lloyd, D. G. (1992). Self- and cross-fertilization in plants. II. the selection of self- fertilization. Int. J. Plant Sci. 153, 370–380. doi: 10.1086/297041
Mable, B. K., Robertson, A. V., Dart, S., Di Berardo, C., and Witham, L. (2005). Breakdown of self-incompatibility in the perennial Arabidopsis lyrata (Brassicaceae) and its genetic consequences. Evolution 59, 1437–1448. doi: 10.1554/05-004
Márquez-Guzmán, J., Wong, R., Pérez, M., López, L., and Murguía, G. (2016). Técnicas de Laboratorio para el Estudio del Desarrollo en Angiospermas. Coordinación en Servicios Editoriales, Facultad de Ciencias. México: UNAM.
Meyer, R. S., and Purugganan, M. D. (2013). Evolution of crop species: genetics of domestication and diversification. Nat. Rev. Genet. 14, 840–852. doi: 10.1038/nrg3605
Meyer, R. S., DuVal, A. E., and Jensen, H. R. (2012). Patterns and processes in crop domestication: an historical review and quantitative analysis of 203 global food crops. New Phytol. 196, 29–48. doi: 10.1111/j.1469-8137.2012.04253.x
Montes-Hernández, S., and Aguirre-Rivera, A. R. (1994). Etnobotánica del tomate mexicano (Physalis philadelphica Lam.). Revista de Geografía Agrícola. 20, 163–172.
Mulato-Brito, J., Peña-Lomelí, A., Sahagún-Castellanos, J., Villanueva-Verduzco, C., and López-Reynoso, J. J. (2007). Self-compatibility inheritance in tomatillo (Physalis ixocarpa Brot.). Veg. Crop. Res. Bull. 67, 17–24. doi: 10.2478/v10032-007-0026-4
Nee, M. (1993). “Solanaceae II,” in Flora de Veracruz, Vol 72, ed. V. Sosa (Xalapa: Instituto de Ecología AC & University of California).
Olmstead, R. G., Bohs, L., Migid, H. A., Santiago-Valentin, E., Garcia, V. F., and Collier, S. M. (2008). A molecular phylogeny of the Solanaceae. Taxon 57, 1159–1181. doi: 10.1002/tax.574010
Ozaslan, C., Faraooq, S., Onen, H., Ozcan, S., Bukun, B., and Gunal, H. (2017). Germination Biology of two invasive physalis species and implications for their management in arid and semi-arid regions. Sci. Rep. 7:16960.
Pandey, K. K. (1957). Genetics of self-incompatibility in Physalis ixocarpa Brot. a new system. Am. J. Bot. 44, 879–887. doi: 10.1002/j.1537-2197.1957.tb08275.x
Pickersgill, B. (2007). Domestication of plant in the Americas: insights from mendelian and molecular genetics. Ann. Bot. 100, 925–940. doi: 10.1093/aob/mcm193
Purugganan, M. D. (2019). Evolutionary insights into the nature of plant domestication. Curr. Biol. 29, R705–R714.
Purugganan, M. D., and Fuller, D. Q. (2009). The nature of selection during plant domestication. Nature 457, 843–848. doi: 10.1038/nature07895
R Core Development Team (2019). A Language and Environment for Statistical Computing R Version 3.6.0. Vienna: The R Foundation for Statistical Computing.
Rick, C. M. (1988). “Evolution of mating systems in cultivated plants,” in Plant Evolutionary Biology, eds L. D. Gottlieb and S. K. Jain (Dordrecht: Springer), 133–147. doi: 10.1007/978-94-009-1207-6_6
Rosenthal, J. P., and Dirzo, R. (1997). Effects of life history, domestication and agronomic selection on plant defence against insects: evidence from maizes and wild relatives. Evol. Ecol. 11, 337–355. doi: 10.1023/a:1018420504439
Samuels, J. (2012). Solanum incanum s.l. (Solanaceae): taxonomic relationships between S. incanum, S. campylacanthum, S. panduriforme and S. lichtensteinii. Kew Bull. 67, 401–411. doi: 10.1007/s12225-012-9373-5
Sánchez-Martínez, J., Avendaño López, A. N., Vargas-Ponce, O., and Arellano Rodríguez, J. L. (2018). Latencia natural e inducida por almacenaje en variedades cultivadas y especies silvestres de Physalis. Revista de Innovación Sistemática 2, 21–24
Särkinen, T., Bohs, L., Olmstead, R. G., and Knapp, S. (2013). A phylogenetic framework for evolutionary study of the nightshades (Solanaceae): a dated 1000 tip tree. BMC Evol. Biol. 13:214. doi: 10.1186/1471-2148-13-214
SIAP (2018). Anuario Estadístico de la Producción Agrícola. Sist. Inf. Agroalimentaria Pesq. Available online at: http://infosiap.siap.gob.mx/aagricola_siap_gb/ientidad/index.jsp (accessed January 7, 2019).
Stone, J. L., Sasuclark, M. A., and Blomberg, C. P. (2006). Variation in the self-incompatibility response within and among populations of the tropical shrub Witheringia solanacea (Solanaceae). Am. J. Bot. 93, 592–598. doi: 10.3732/ajb.93.4.592
Therneau, T. M., and Grambsch, P. M. (2000). Modeling Survival Data: Extending the Cox Model. New York: Springer.
Valdivia-Mares, L. E., Zaragoza, F. A., Sánchez, J. J., and Vargas-Ponce, O. (2016). Phenology, agronomic and nutritional potential of three wild husk tomato species (Physalis, Solanaceae) from Mexico. Sci. Hortic. 200, 83–94. doi: 10.1016/j.scienta.2016.01.005
Vallejo-Marín, M., and Uyenoyama, M. K. (2004). On the evolutionary costs of self-incompatibility: incomplete reproductive compensation due to pollen limitation. Evolution 58, 1924–1935. doi: 10.1111/j.0014-3820.2004.tb00480.x
Vallejo-Marín, M., Walker, C., Friston-Reilly, P., Solís-Montero, L., and Igic, B. (2014). Recurrent modification of floral morphology in heterantherous Solanum reveals a parallel shift in reproductive strategy. Philos. Trans. R. Soc. B Biol. Sci. 369:20130256. doi: 10.1098/rstb.2013.0256
Velázquez-López, R., Wegier, A., Alavez, V., Pérez-López, J., Vázquez-Barrios, V., Arroyo-Lambaer, D., et al. (2018). The mating system of the wild-to-domesticated complex of Gossypium hirsutum L. is mixed. Front. Plant Sci. 9:574. doi: 10.3389/fpls.2018.00574
Warschefsky, E., Penmetsa, R. V., Cook, D. R., and von Wettberg, E. J. B. (2014). Back to the wilds: tapping evolutionary adaptations for resilient crops through systematic hybridization with crop wild relatives. Am. J. Bot. 101, 1791–1800. doi: 10.3732/ajb.1400116
Zamora-Tavares, P., Vargas-Ponce, O., Sánchez-Martínez, J., and Cabrera-Toledo, D. (2015). Diversity and genetic structure of the husk tomato (Physalis philadelphica Lam.) in Western Mexico. Genet. Resour. Crop Evol. 62, 141–153. doi: 10.1007/s10722-014-0163-9
Keywords: autonomous autogamy, domestication, commercial-plants, husk tomato, landraces, plant reproduction, Physalis philadelphica, weedy populations
Citation: Solís-Montero L, Aceves-Chong L, Vega-Polanco M and Vargas-Ponce O (2021) Changes in Reproductive Traits in Physalis philadelphica; An Unexpected Shift Toward Self-Incompatibility in a Domesticated Annual Fruit Crop. Front. Plant Sci. 12:658406. doi: 10.3389/fpls.2021.658406
Received: 25 January 2021; Accepted: 14 April 2021;
Published: 21 May 2021.
Edited by:
Thomas Miedaner, University of Hohenheim, GermanyReviewed by:
Peter Bernhardt, Saint Louis University, United StatesMichael Kantar, University of Hawai‘i at Mānoa, United States
Copyright © 2021 Solís-Montero, Aceves-Chong, Vega-Polanco and Vargas-Ponce. This is an open-access article distributed under the terms of the Creative Commons Attribution License (CC BY). The use, distribution or reproduction in other forums is permitted, provided the original author(s) and the copyright owner(s) are credited and that the original publication in this journal is cited, in accordance with accepted academic practice. No use, distribution or reproduction is permitted which does not comply with these terms.
*Correspondence: Lislie Solís-Montero, bHNvbGlzQGVjb3N1ci5teA==