- 1State Key Lab of Crop Genetics and Germplasm Enhancement, Cytogenetics Institute, Nanjing Agricultural University/JiangSu Collaborative Innovation Center for Modern Crop Production, Nanjing, China
- 2The Laboratory of Seed Science and Technology, Guangdong Key Laboratory of Plant Molecular Breeding, Guangdong Laboratory of Lingnan Modern Agriculture, State Key Laboratory for Conservation and Utilization of Subtropical Agro-Bioresources, South China Agricultural University, Guangzhou, China
- 3State Key Laboratory for Managing Biotic and Chemical Threats to the Quality and Safety of Agro-products, Institute of Virology and Biotechnology, Zhejiang Academy of Agricultural Sciences, Hangzhou, China
EXO70 belongs to the exocyst complex subunit that plays a critical role in regulating plant cell polarity establishment and defense response. A previous study proved that the E3 ligase CMPG1-V from Haynaldia villosa, a diploid wheat relative, positively regulates the resistance to wheat powdery mildew (Pm), caused by fungus Blumeria graminis f.sp tritici (Bgt). In this study, a member of EXO70 superfamily named EXO70E1-V was isolated from H. villosa, and EXO70E1-V interacted with CMPG1-V were shown by yeast two-hybrid (Y2H), pull-down assay, bimolecular fluorescence complementation (BiFC) assay, and luciferase complementation imaging (LCI) assay. It is localized in various subcellular organs, i.e., plasma membrane (PM) and endoplasmic reticulum. Co-expression of EXO70E1-V and CMPG1-V showed dot-like structure fluorescence signals that were mainly in PM and nucleus. Expression of EXO70E1-V was relatively higher in leaf and was significantly induced by Bgt infection and exogenous application of hormones such as salicylic acid. Transient or stable overexpression of EXO70E1-V could not enhance/decrease the Pm resistance level, suggesting overexpression of EXO70E1-V alone has no impact on Pm resistance in wheat.
INTRODUCTION
The exocyst complex is a conserved octameric vesicle–tethering complex with principal roles in mediating secretory vesicle to the target membrane prior to SNARE-mediated fusion (Sabol et al., 2017; Kulich et al., 2018). EXO70, a key subunit of the exocyst complex, serves as a spatial landmark of the exocyst complex at the active exocytosis sites (Wu and Guo, 2015). There are multicopies of EXO70 in land plants (Ma et al., 2016). In coordination with other proteins, different members play a diverse role in the processes of cell polarity establishment, including a deposition of seed coat pectin (Kulich et al., 2010), xylem development and formation of Casparian strip (Li et al., 2013; Kalmbach et al., 2017), vascular bundle differentiation (Tu et al., 2015), pollen tube and trichome cell wall maturation (Fendrych et al., 2010; Synek et al., 2017; Kulich et al., 2018), leaf senescence and legume growth and development (Wang et al., 2016), and transport of PIN auxin carriers to specific parts of the plasma membrane (PM) (Drdova et al., 2013).
The important role of EXO70 subunits in plant defense responses against biotic stresses has been reported. AtEXO70B2 is a positive regulator in plant–pathogen interaction. It interacts with secreted defense-related protein SNAP33 and negative regulator PUB22 in PAMP-triggered responses, which prevents pathogens from infecting cells by increasing the cell wall thickness (Pecenkova et al., 2011; Stegmann et al., 2012). AtEXO70B1 is highly homologous with AtEXO70B2, which can be recruited to the cytoplasmic membrane by RIN (Sabol et al., 2017). AtEXO70B1 involves in plant defense responses by interacting with RIC7 and PUB18 to regulate the stomatal movement (Hong et al., 2016) or phosphorylated by CDPK5 and interacts with the atypical immune receptor TIR-NBS2 to block the growth of pathogens (Zhao et al., 2015; Liu et al., 2017). Moreover, AtEXO70E2 is essential for exocyst subunit recruitment to form a new organelle named EXPO. EXPO is a novel spherical double-membrane structure involved in unconventional protein secretion for cytosolic proteins (Wang et al., 2010; Ding et al., 2014; Robinson et al., 2016). OsEXO70E1 interacts with Bph6 to enhance the exocytosis and strengthen the cell wall, thereby hindering the feeding of the planthopper and as a result, improving the resistance (Guo et al., 2018).
Common wheat (Triticum aestivum L., 2n = 42, AABBDD) is one of the most important food crops, which is the staple food for at least one-third of the population of the world (Shi and Ling, 2018). The release of the draft genome sequence allows us to identify and analyze all the members of EXO70 superfamily (Zhao et al., 2018). In our previous study, a U-box/Arm-type E3 ligase gene CMPG1-V was cloned from Haynaldia villosa. The functional study demonstrated that CMPG1-V is a positive regulator in powdery mildew (Pm) resistance of common wheat (Zhu et al., 2015). However, the regulation mechanism remains unknown.
In this study, a yeast two-hybrid (Y2H) screening was performed by using CMPG1-V as a bait, and a subunit of the exocyst complex was identified and cloned from H. villosa, namely EXO70E1-V. The interaction between EXO70E1-V and CMPG1-V in vivo and in vitro was verified by pull down assay, luciferase complementation imaging (LCI) assay, and bimolecular fluorescence complementation (BiFC) assay. EXO70E1-V was significantly upregulated in response to Blumeria graminis f.sp tritici (Bgt) inoculation and exogenous salicylic acid (SA) treatments. Nevertheless, the functional analysis suggested that overexpression of EXO70E1-V alone has no impact on Pm resistance in wheat.
Materials and Methods
Plant Materials
Haynaldia villosa (2n = 14, VV, accession no. 91C43) was used for cloning and expression analysis; a set of T. aestivum–H. villosa addition lines (DA1V–DA7V), each contains one pair of chromosomes from H. villosa in Chinese Spring background used for chromosome location; wheat cultivar Yangmai158 (moderately susceptible to Bgt) was used for the subcellular localization analysis and as receptors for stable transformation; Sumai3 was used for propagation of the freshly mixed races of Bgt spores, and all of those materials were developed or preserved by Cytogenetic Institute, Nanjing Agricultural University (CINAU). Nicotiana benthamiana plants were grown in a controlled growth room at 24°C/20°C day/night with 12 h/day light and 70% humidity. The 5- to 6-week-old plants were used for Agrobacterium-mediated transient expression.
The Chemical Treatments of Plants
The H. villosa seedlings were grown in liquid with constant 14 h light/10 h dark (24°C/18°C, 70% humidity). At the three-leaf stages, the plants were inoculated with Bgt and treated with 100 μg/mL insoluble chitin (No. C7170, Sigma-Aldrich, United States) or 0.1 mmol/L flg22. Meanwhile, the treatments with exogenous hormone or signal molecules, including sprayed with 5 mmol/L SA, 0.1 mmol/L methyl jasmonate (MeJA), 0.1 mmol/L ethylene (ET), 0.2 mmol/L abscisic acid (ABA), and 7 mmol/L hydrogen peroxide (H2O2), respectively, were conducted. All samples were collected after 0, 1, 4, 8, 12, 24, 48 h treatments and rapidly frozen in liquid nitrogen and then stored in ultra-freezer (–80°C) until used.
Yeast Two-Hybrid Protein–Protein Interaction
The yeast strain AH109 was cultured on YPAD plates that were used to test protein to protein interaction between CMPG1-V and EXO70-V. In brief, the full-length CMPG1-V fused with the DNA-binding domain of GAL4 in pGBKT7, while the EXO70-V cDNA sequence was inserted into a pGADT7 vector harboring an activation domain (AD) (Faheem et al., 2016). The transformation mixtures were plated on SD-Leu-Trp medium incubation at 28°C for 2–3 days, and then the individual clones were spotted onto selection media SD-His/Leu/Trp with X-α-Gal (40 mg/ml). The primers are listed in Supplementary Table 1.
Firefly LCI and BiFC Assays
For firefly LCI assay, the coding regions of EXO70E1-V (without the stop codon) and CMPG1-V were ligated into the pCAMBIA-NLUC and pCAMBIA-CLUC vectors, respectively. For BiFC assays, the EXO70E1-V and CMPG1-V were fused with the N-terminus or C-terminus of the split-yellow fluorescent protein (YFP), respectively. The primers are listed in Supplementary Table 1. Different recombinant plasmids including NLUC-EXO70E1-V, CLUC-CMPG1-V, YN-EXO70E1-V, and YC-CMPG1-V with the control vector were introduced into Agrobacterium tumefaciens strain GV3101. Overnight agrobacterial cultures were resuspended with infiltration buffer (10 mM MgCl2, 0.1 mM acetosyringone, and 10 mM MES). Different experimental and control group agrobacterial suspensions were mixed and co-infiltrated into 5- to 6-week-old N. benthamiana leaves by using a needleless syringe and then weak light growth. Two days later, for LCI, 1 mmol/L luciferin was infiltrated into the leaves, and the plants were kept in the dark for 10 min. LCI images were captured using a low-light cooled CCD imaging apparatus, as described by Chen et al. (2008). For BiFC, fluorescence was observed by confocal microscopy.
In vitro Pull-Down Assay
The full-length open reading frame (ORF) of EXO70E1-V was individually ligated into pGEX6p-1 vector for protein expression, and the primers are listed in Supplementary Table 1. The recombinant glutathione S-transferase (GST)-EXO70E1-V plasmid and control vector were expressed in the Escherichia coli strain BL21 (DE3) and purified by anti-chromatography using glutathione sepharose beads. Generation of the Maltose-Binding Protein (MBP)-CMPG1-V construct and protein purification were performed as described by Zhu et al. (2015). For pull-down assay, the GST-EXO70E1-V and GST alone (control) proteins were incubated with glutathione sepharose beads at 4°C for 2 h with gentle shaking and then incubation with MBP-CMPG1-V and MBP proteins, respectively, for 1 h at room temperature. After incubation, the beads were harvested, washed once with PBS buffer containing 500 mM NaCl, and subsequently washed five times with the same buffer containing 135 mM of NaCl. The bound protein complex retained on the beads was extracted by boiling the beads in 10 μL of 2× SDS-PAGE loading buffer and finally analyzed by western blotting using GST and MBP antibodies.
Phylogenetic Analysis
The amino acid sequences of Arabidopsis EXO70 proteins were downloaded from the Ensembl Plants database1. All EXO70 protein sequences were aligned by ClustalW, and a phylogenetic tree was constructed by MEGA6 using the neighbor-joining method with the pairwise deletion option, Poisson correction, and bootstrap analysis with 1,000 replicates (Tamura et al., 2013).
RNA Extraction, cDNA Synthesis, and Gene Expression Profiling
Total RNA was extracted by using the Trizol Reagent Kit (Invitrogen, Carlsbad, CA, United States) and analyzed by gel electrophoresis. The first-strand cDNA was synthesized with random oligonucleotides using the HiScript® II Reverse Transcriptase system (Vazyme, Nanjing, China). Quantitative reverse transcription (qRT)-PCR was carried out in a total volume of 20 μL containing 2 μL of cDNA, 0.4 μL of gene-specific primers (10 μm), 10 μL of SYBR Green Mix, and 7.2 μL of RNase free ddH2O, using the Roche LightCycler 480 Real-time System (Roche, Basel, Swiss). The wheat tubulin gene was used as internal controls. The program and data analysis were carried out as described in the method suggested by Wang et al. (2018). Primers used for the qRT-PCR are designed by Primer5 listed in Supplementary Table 1. Three biological replications were performed. We obtained the in silico expression data of EXO70E1-V ortholog genes in wheat (TraesCS3A02G302600, TraesCS3B02G333800, and TraesCS3D02G299200) induced by Pm from the Triticeae Multi-omics Center wheat gene expression website2.
Vector Construction and Subcellular Localization of EXO70E1-V
The ORF of EXO70E1-V (without the stop codon) was amplified by using primers green fluorescent protein (GFP)-EXO70E1-V-F/GFP-EXO70E1-V-R and then inserted into pAN580 vector as C-terminal fusions to the GFP reporter gene driven by the double 35S promoter. The GFP-EXO70E1-V vector was transformed into Yangmai158 protoplasts. Plasmid DNA (1.5 μg/μL) for each construct was mixed with a red fluorescent protein (RFP)/mCherry-fused marker protein (1.5 μg/μL), and 20 μL of total DNA was used to transform 200 μL of protoplasts derived from 5- to 7-day-old plants. Vectors expressing the PM marker PIP2a-mCherry and the trans-Golgi network (TGN)/early endosome marker mCherry-SYP61 were provided by Yiqun Bao (College of Life Science, Nanjing Agricultural University, Nanjing, China), and vectors for the endoplasmic reticulum (ER) marker (RFP-ER) and the Golgi marker (GmMan49–RFP) were provided by Libo Shan (Department of Plant Pathology and Microbiology, Texas A&M University, TX, United States). The GFP/RFP/mCherry signals were assessed by confocal imaging, 16–20 h after transformation. For imaging, an LSM780 confocal microscope (Zeiss, Jena, Germany)3 was used as described by Zhu et al. (2015). The primers are listed in Supplementary Table 1.
Single-Cell Transient Overexpression Assay
EXO70E1-V was cloned into plant expression vector pBI220 to generate vectors pBI220-EXO70E1-V (Supplementary Table 1). Transient overexpression assay (TOA) was performed according to Shirasu et al. (1999) and Cao et al. (2011). The reporter plasmid pWMB002 containing the β-glucuronidase (GUS) gene and the expression plasmid were mixed before particle coating (molar ratio of 1:1; 1 μg of total DNA). The bombarded leaves were transferred to 1% agar plates supplemented with 85 μm benzimidazoles and incubated at 18°C for 8 h before inoculation of high density Bgt conidia spores. Leaves were stained at 48 hours after innoculation for identifying GUS-expressing cells, which is the indicator of cells transformed with EXO70E1-V. The average haustorium index (HI, percentage of GUS-stained cells with haustorium in the total GUS-staining cells attacked by Bgt) was computed based on the means of three independent experiments, each based on at least 100 independent interaction events.
Wheat Transformation
The EXO70E1-V was overexpressed (driven by the CAMV 35S promoter) in Yangmai158 by stable transformation using the bombardment method according to Xing et al. (2008). The pAHC25 containing the reporter gene GUS and the herbicide tolerance gene driven by the Ubi promoter was used as a selectable vector. The pBI220-EXO70E1-V and pAHC25 vectors were co-transformed into calli cultured from immature embryos of wheat variety Yangmai158 by particle bombardment. Regenerated plants were produced as previously described (Xing et al., 2008). For screening of the positive transgenic plants, a specific primer pair (OE-EXO70E1-V-F/R, the product was 304 bp), which amplifies the sequence cover in the CaMV 35S promoter and part of EXO70E1-V, was designed to detect the transgene in plants from the T0 and T1 generations. Then, a pair of specific primers (T-Q-EXOE1-F and T-Q-EXOE1-R) that only detect the expression of EXO70-V from H. villosa were designed to detect the expression of EXO70-V in transgenic plants. The primers are listed in Supplementary Table 1.
Results
EXO70E1-V Interacted With CMPG1-V in vivo and in vitro
To elucidate the resistance pathway mediated by CMPG1-V, a Y2H cDNA library of H. villosa was constructed and used for screening using CMPG1-V as bait (Faheem et al., 2016). One positive fragment clone contains the typical Pfam03081 domain, which is typical for EXO70 superfamily. Based on the homologous cloning, the 1,815 bp full length of the gene was isolated from H. villosa, named EXO70E1-V, by the phylogenetic analysis with Arabidopsis thaliana (Supplementary Figure 1). EXO70E1-V protein is comprised of 605 amino acid proteins with the predicted molecular weight of 68.93 kDa and an isoelectric point of 5.04.
The interaction of full-length EXO70E1-V and CMPG1-V were verified by Y2H (Figure 1A). To test the direct interaction between EXO70E1-V and CMPG1-V, a GST pull-down assay was performed. The expected about 100 kDa fusion protein could be visualized only when GST-EXO70E1-V and MBP-CMPG1-V were co-incubated but not MBP (Figure 1B).
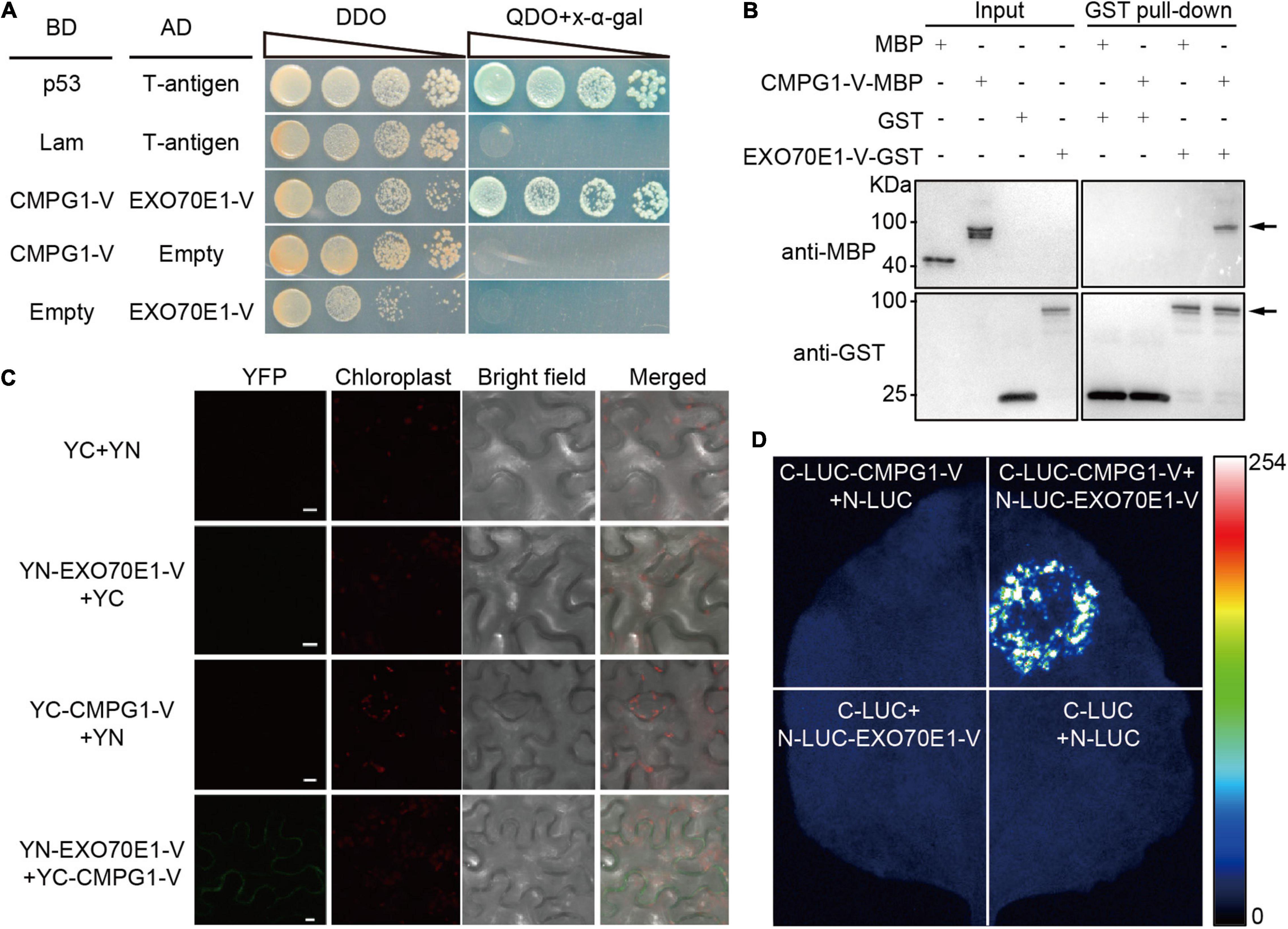
Figure 1. Interaction analysis between EXO70E1-V and CMPG1-V. (A) Analysis of EXO70E1-V and CMPG1-V interaction on double dropout or quadruple dropout medium by yeast two-hybrid (Y2H). Interaction of CMPG1-V (fused to BD, pGBKT7) with EXO70E1-V (fused to AD, pGADT7) by a Y2H experiment. p53 (pGBKT7-53)/T-antigen (pGADT7-T) and Lam (pGBKT7-Lam)/T-antigen were positive and negative controls, respectively. (B) In vitro EXO70E1-V/CMPG1-V interaction analysis using the GST pull-down assay. Anti-MBP antibody was used to detect the GST pull-down products, and the CMPG1-V-MBP could be detected only when co-incubated with EXO70E1-V-GST. Arrowheads indicate the CMPG1-V-MBP (upper panel) and the EXO70E1-V-GST (lower panel) proteins, respectively. (C) In vivo EXO70E1-V/CMPG1-V interaction analysis by BiFC assay in the Nicotiana benthamiana epidermal cells. The green channel shows the localization of the yellow fluorescent protein (YFP) and its fusion proteins, and the red channel shows the location of the chloroplast. YN and YC indicate N-terminal and C-terminal of YFP, respectively. Scale bar = 10 μm. (D) In vivo EXO70E1-V/CMPG1-V interaction analysis by LUI assay in the N. benthamiana epidermal cells. LUI image of N. benthamiana leaves co-infiltrated with the agrobacterial strains containing N-LUC-EXO70E1-V and C-LUC-CMPG1-V.
To confirm the interaction between EXO70E1-V and CMPG1-V in vivo, the BiFC and firefly LCI assays were operated. The results showed that only the co-expression of YN-EXO70E1-V and YC-CMPG1-V in tobacco leaves resulted in the complementation of fluorescence localized on the PM (Figure 1C). Meanwhile, the co-expression of CLUC-CMPG1-V and NLUC-EXO70E1-V in tobacco leaves could reconstitute a high luciferase activity, compared with the various negative controls (Figure 1D), and these results demonstrated that EXO70E1-V was able to interact with CMPG1-V both in vitro and in vivo (Figure 1).
The EXO70E1s Were Conversely Present on the Homologous Group 3
The chromosomal location of EXO70E1-V was determined by amplification using DNA from Triticum durum–H. villosa amphiploidy (genome AABBVV) and a complete set of Chinese Spring-H. villosa alien addition lines (DA1V–DA7V). A 285 bp product (YST-EXO70E1-V-F/R) was amplified in H. villosa, the amphiploid, and the addition line DA3V, but not in Chinese Spring, and the remaining tested addition lines. Thus, the EXO70E1-V was mapped to the chromosome 3V of H. villosa (Figure 2A).
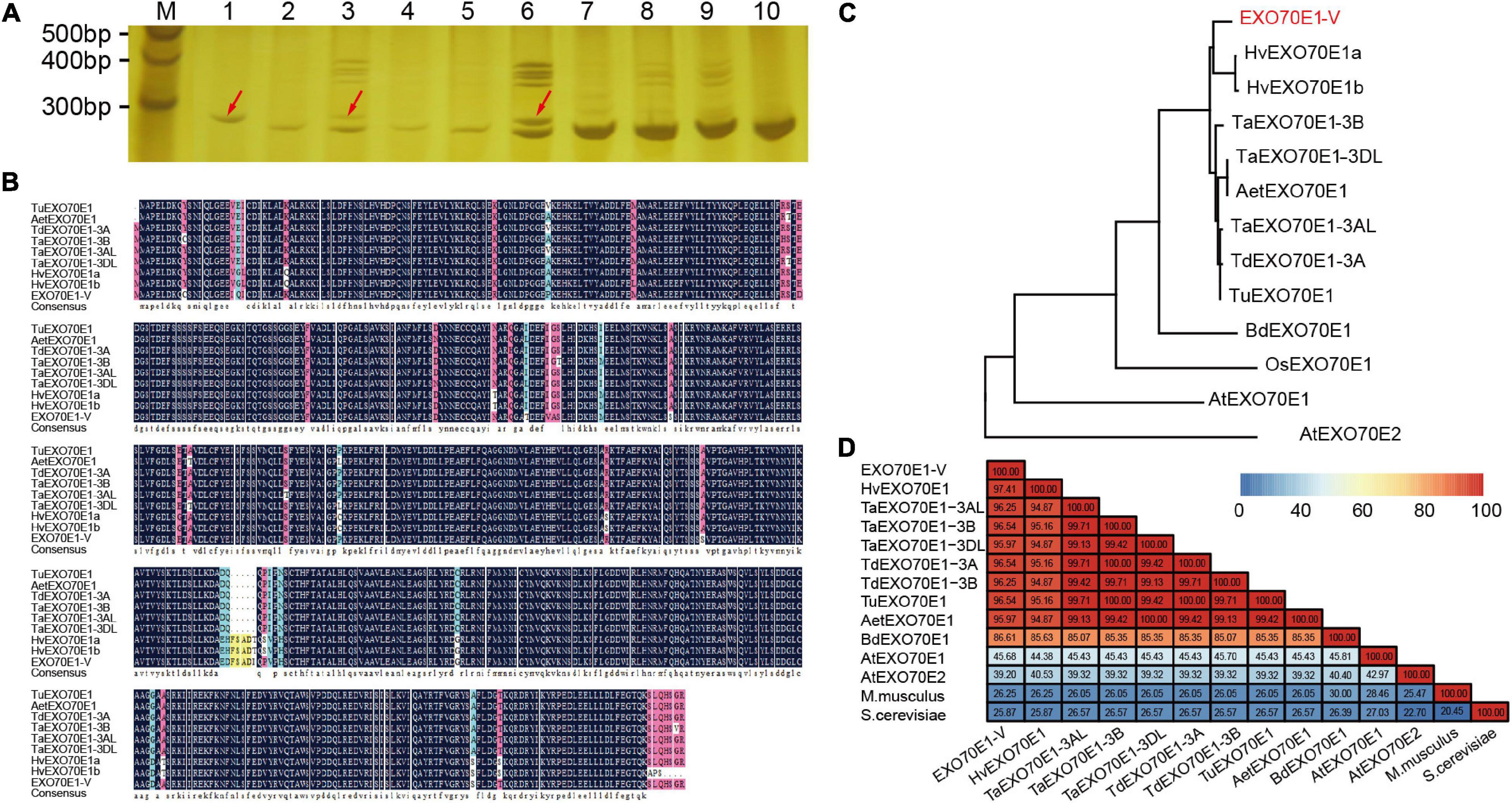
Figure 2. The sequence analysis of EXO70E1-V. (A) Chromosomal location of EXO70E1-V using a set of wheat-Haynaldia villosa addition lines. M, 20 bp DNA Ladder; 1, H. villosa; 2, Chinese Spring (CS); 3, Triticum durum–H. villosa amphiploid (AABBVV); 4–10, DA1V–DA7V. The red arrow indicates the target band. (B) Alignment of EXO70E1 ortholog proteins in different Triticeae species. (C) Phylogenetic analysis of EXO70E1-V and its orthologs in Triticeae species, Brachypodium distachyon, Oryza sativa, and Arabidopsis thaliana. The EX070 gene from H. villosa is marked in red. (D) Sequence similarity analysis of EXO70E1 ortholog proteins in Triticeae species, A. thaliana, M. musculus, and S. cerevisiae. Tu, Triticum urartu; Aet, Aegilops tauschii; Td, Triticum dicoccoides; Ta, Triticum aestivum; Hv, Hordeum vulgare; –V, Haynaldia villosa; Os, Oryza sativa; Bd, Brachypodium distachyon.
In addition, we obtained the EXO70E1 protein orthologs from five Triticeae species (T. aestivum, Triticum urartu, Aegilops tauschii, Triticum dicoccoides, and Hordeum vulgare) by using the bioinformatics analysis. The EXO70E1 is a single copy in all five species and in all locations in homologous group 3 chromosomes. The alignment of EXO70E1-V with its orthologs indicated that EXO70E1s are well-conserved (Figure 2B). The results of the phylogenetic analysis showed obviously the branching between Arabidopsis and gramineous crops, and EXO70E1-V was closest to EXO70E1 from H. vulgare (Figure 2C). Through the sequence similarity analysis, the similarity of EXO70E1 orthologous genes was more than 94%, and the similarity between EXO70E1-V and HvEXO70E1 is 97.41% (Figure 2D), which is consistent with the results of the phylogenetic analysis.
The Subcellular Localization of EXO70E1-V
The biological function of protein is closely related to its subcellular location. To determine the subcellular localization of EXO70E1-V, a co-expression vector containing EXO70E1-V and GFP-EXO70E1-V was constructed and transformed into wheat protoplasts. Compared with the ubiquity of GFP signals in the cell (Figure 3A), GFP-EXO70E1-V signals were mainly distributed in the nucleus and on the PM and also in some cytoplasmic signals (Figure 3B). To determine the specific location, the marker genes for PM (PIP2a-mCherry), ER (RFP-ER), TGN/early endosome vesicle (RFP-SYP61), and Golgi (GmMan49-RFP) were co-transformed with GFP-EXO70E1-V, and the GFP signal was found to overlap with PM, ER, and TGN but not Golgi (Figures 3B–E). These results indicate that EXO70E1-V is located in the nucleus, PM, ER, and TGN. When GFP-EXO70E1-V and RFP-CMPG1-V were co-transformed into wheat protoplasts, dot-like structure fluorescence signals were partly colocated in PM and nucleus (Figure 3F). This result indicated the possibility of participation of EXO70E1-V and CMPG1-V together in similar biological processes.
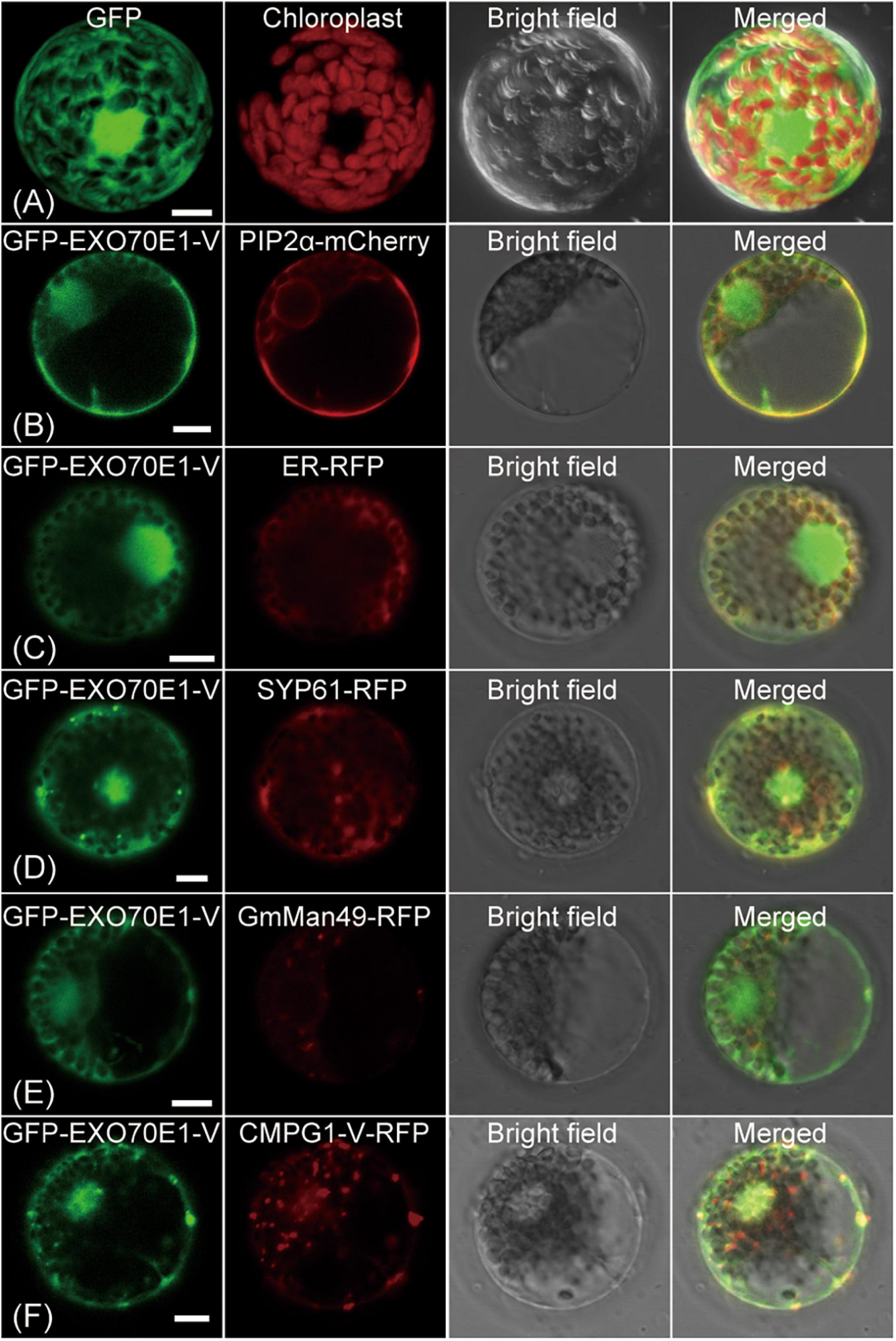
Figure 3. Subcellular localization of GFP-EXO70E1-V and interaction of GFP-EXO70E1-V with CMPG1-V-YFP in wheat protoplast. (A) GFP control. (B) Co-localization of the constructs GFP-EXO70E1-V (in green) and PIP2α–mCherry (in red, marker gene on plasma membrane). (C) Co-localization of the constructs GFP-EXO70E1-V (in green) and ER-RFP (in red, marker gene on endoplasmic reticulum). (D) Co-localization of the constructs GFP-EXO70E1-V (in green) and SYP61-RFP (in green, marker gene on trans-Golgi network/early endosome). (E) Co-localization of the constructs GFP-EXO70E1-V (in green) and GmMan49-RFP (in green, marker gene on Golgi). (F) Co-localization of GFP-EXO70E1-V (in green) and CMPG1-V-RFP (in red). Scale bar = 10 μm.
Expression Profiling of EXO70E1s
To explore the potential biological function of EXO70E1-V genes in different organs and in response to stress treatments, the qRT-PCR was performed. The expression of EXO70E1-V showed a high expression level in leaves than in stems and roots (Figure 4A). EXO70E1-V was upregulated and reached a peak at 48 h after Bgt inoculation, which was about 6-fold compared with non-inoculated H. villosa, but hardly induced by flg22 and chitin (Figure 4B). EXO70E1-V was quickly induced by SA, which showed the tendency of quickly increasing first and then decreasing rapidly, got the peak value after 1 h treatment, that was about 7.5-fold compared with control and then back to a relatively lower level (Figure 4C). The in silico expression analysis of EXO70E1-V ortholog genes (TraesCS3A02G302600, TraesCS3B02G333800, and TraesCS3D02G299200) in common wheat (Chinese Spring, susceptible) showed that they were not induced by Pm (Supplementary Figure 2).
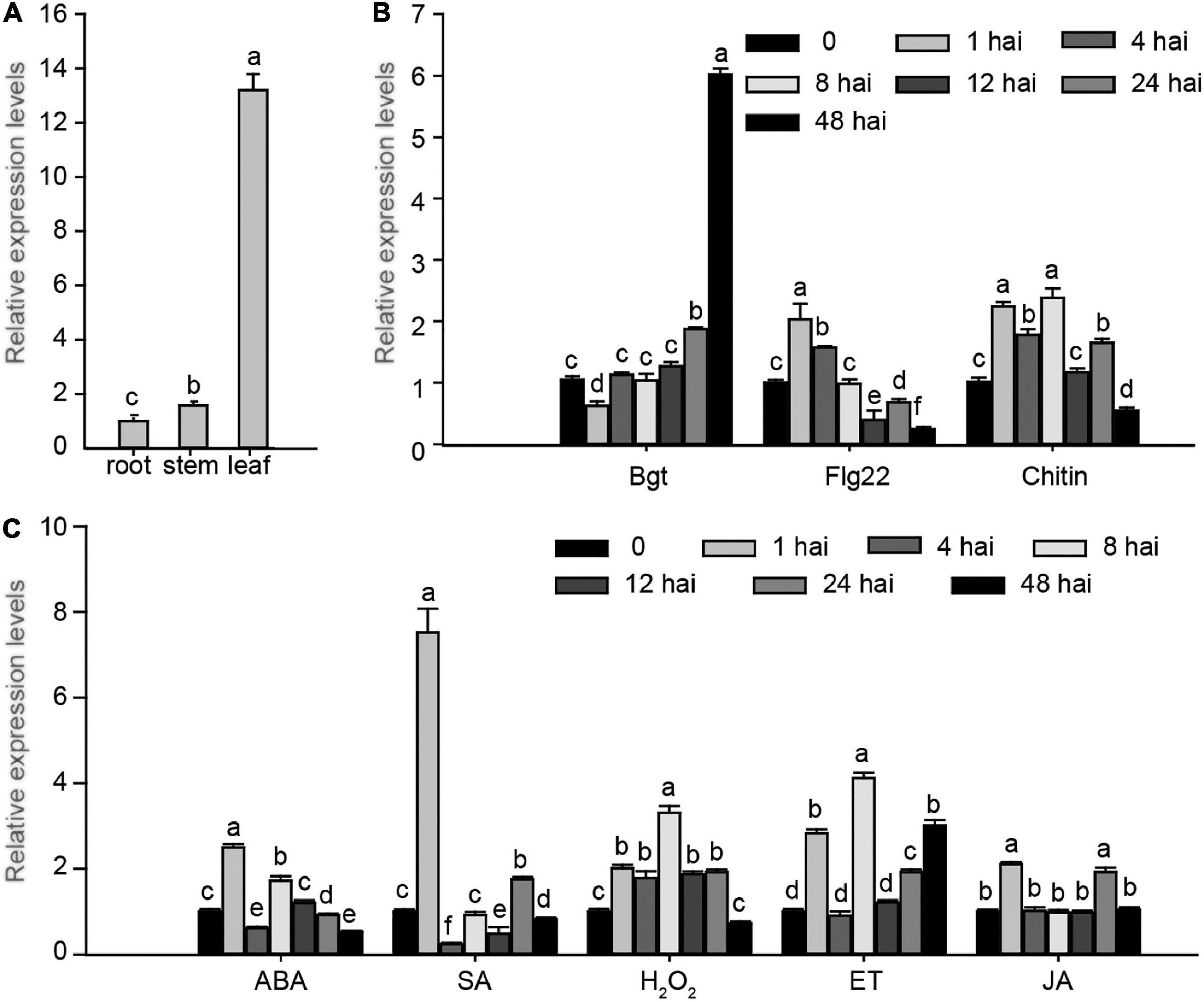
Figure 4. Expression profiling of EXO70E1-V. (A) The expression of EXO70E1-V in root, stem, and leaf of H. villosa. (B) Expression levels of EXO70E1-V in biotic stresses of H. villosa. (C) Expression profiling of EXO70E1-V under stress-related signal treatments. Bgt, Blumeria graminis f.sp tritici; SA, salicylic acid; MeJA, methyl jasmonate; ET, ethephon; ABA, abscisic acid; H2O2, hydrogen peroxide. Each column represents the means ± SD. Different lowercase letters represent significant differences at 5% level according to the least significant difference (LSD) test.
Functional Analysis of EXO70E1-V in Pm Resistance
The function of EXO70E1-V in Pm resistance was validated using single cell TOA and stable transformation. The formation of haustorium indicates successful penetration of Bgt into wheat epidermal cells (Figure 5A). A higher HI indicates increased Pm susceptibility. For TOA, GUS alone and GUS + EXO70E1-V constructs were bombarded into leaves of a moderate Pm susceptible wheat variety Yangmai158. The HI values in the GUS-expressing epidermal cells were compared. The average HI in the GUS-expressing epidermal cells showed no significant difference in plants transforming GUS alone (HI: 60.97%) and plants co-transforming GUS + EXO70E1-V (HI: 60.54%) (Figure 5B). This indicated that transient overexpression of EXO70E1-V in Yangmai158 could not change its HI.
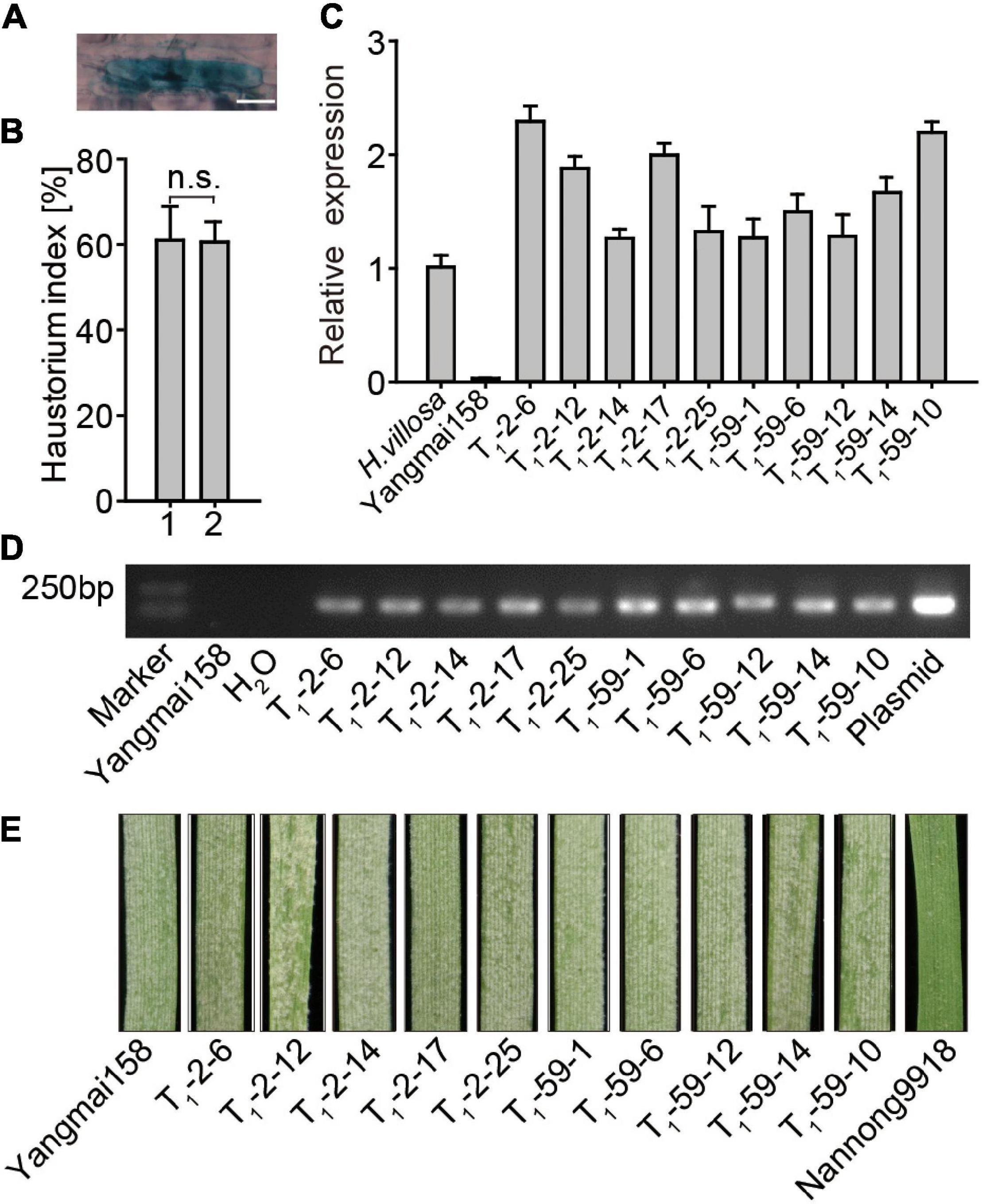
Figure 5. Functional analysis of EXO70E1-V in powdery mildew (Pm) resistance. (A) Examples of β-glucuronidase (GUS) staining and haustorium, scale bar = 20 μm. (B) Transient overexpression assay (TOA) of EXO70E1-V. 1 and 2 represent vectors GUS alone and GUS + EXO70E1-V, respectively. Haustorium index is the percentage of GUS-stained cells having haustorium in the total GUS-staining cells attacked by Bgt. Each column represents the means ± SD. n.s. represents not significant. (C) EXO70E1-V expression in H. villosa, receptor Yangmai158, and two T1 transgenic lines (T1-2 and T1-59). The expression levels were normalized to Tubulin, and H. villosa is set as “1.” (D) Amplification for EXO70E1-V in T1 transgenic lines. Yangmai158 and H2O were negative controls, and plasmid pBI220-EXO70E1-V was used as a positive control. Marker: DL2000 DNA ladder. (E) Observation of Pm spores on leaves. Yangmai158 and Nannong9918 were used as susceptible and resistant control, respectively.
The regeneration plant was obtained by co-transforming pBI220-EXO70E1-V and pAHC25 (carrying bar gene as a selection marker) vectors into 1,000 young embryo calluses of Yangmai158 by using the particle bombardment-mediated transformation approach. The T1 lines generated from EXO70E1-V-T0-2 and EXO70E1-V-T0-59 were selected for further analysis. The result of qRT-PCR showed that the expression level of EXO70E1-V varied in the T1 transgenic plants and was comparable with H. villosa (contains EXO70E1-V), but no expression of EXO70E1-V was detected in the Yangmai158 (does not contain EXO70E1-V) (Figure 5C). The agarose gel electrophoresis analysis showed that EXO70E1-V existed in each of these two transgenic lines (Figure 5D). However, T1 lines were showed no obvious effect on Pm resistance at the seedling when detached leaves were inoculated with a mixture of Bgt isolates (Figure 5E). The results further proved that the overexpression of EXO70E1-V in Yangmai158 could not change the resistance to mixed Bgt.
Discussion
EXO70 acts as a key member of the exocyst complex, formed by three distinct subfamilies (EXO70.1, EXO70.2, and EXO70.3) (Elias, 2003), which functions in cell and organ morphogenesis, stress responses, and hormone signaling (Synek et al., 2006; Zhao et al., 2015; Sabol et al., 2017; Guo et al., 2018). In this study, we identified an EXO70 isoform by Y2H screening and cloned from H. villosa, named EXO70E1-V. The results of the experiment showed that EXO70E1-V was able to interact with wheat Pm positive regulator CMPG1-V both in vitro and in vivo (Figure 1), and the transcript could be rapidly induced by Pm and exogenous SA (Figure 4), speculating that EXO70E1-V might play a role in wheat Pm resistance.
Subcellular localization is a key characteristic of protein functional research. In previous studies, AtEXO70E1 has mainly distributed a diffuse cytoplasmic signal in the protoplasts of A. thaliana (Ding et al., 2014). In this study, the GFP-EXO70E1-V fusion protein was transformed into wheat protoplasts, and the result revealed its main localization in the PM and nucleus. The incomplete consistent result possibly explained by different species has different evolutionary environments, and to better adapt to the change of the environment, the gene may evolve a new function based on the original function (Roulin et al., 2013). In addition, the co-localization fluorescence signals of GFP-EXO70E1-V and RFP-CMPG1-V proteins were mainly in PM and nucleus with the dot-like structure. There are a few reports about the ubiquitination of PM proteins in plants, including auxin efflux carrier PIN2, water channel aquaporin PIP2, flagellin receptor FLS2, and iron transporter IRT1 (Abas et al., 2006; Lee et al., 2009; Barberon et al., 2011; Lu et al., 2011). This result indicated the possibility of EXO70E1-V and CMPG1-V participation in some overlapping biological process, and the location of the PM may be related to its function. CMPG1-V is localized in the PM, nucleus, ER, and partially in TGN/early endosome vesicles (Zhu et al., 2015). Combined with the result of BiFC that showed fluorescence signal in PM, we speculated that after EXO70E1-V interacted with CMPG1-V, they mainly function on PM. When the Bgt infected, they enriched on PM to identify the infection of Bgt whether EXO70E1-V is modified by CMPG1-V ubiquitination on the PM and how its functions can be further studied.
The study showed that the members of EXO70A, EXO70C, and EXO70G isoforms are usually linked with the growth and development of plants (Lai, 2016; Synek et al., 2017; Du et al., 2018). Arabidopsis EXO70A1, the best characterized members of EXO70A isoform, involved in cytokinesis (Fendrych et al., 2010), root hair and cell growth (Synek et al., 2006; Wu et al., 2013; Cole et al., 2014), pollen–stigma interactions (Safavian et al., 2015), hypocotyl development (Hala et al., 2008; Jankova Drdova et al., 2019), and primary and secondary cell wall biogenesis (Li et al., 2013; Oda et al., 2015; Vukasinovic et al., 2017). Instead, EXO70B, E, F, and H isoforms are often related to plant biotic interactions and defense responses. Among them, two members of Arabidopsis EXO70B clade (i.e., EXO70B1 and EXO70B2) are the best representatives, which play an important role in autophagy-related transport, stomatal regulation, and plant immunity (Pecenkova et al., 2011; Stegmann et al., 2012; Kulich et al., 2013; Hong et al., 2016; Seo et al., 2016).
In our previous study, the phylogenetic analysis revealed that EXO70E1-V, together with AtEXO70E1/E2 and OsEXO70E1 fell into the E clade of EXO70 proteins (Zhao et al., 2018). AtEXO70E2 can recruit other subunits to form a new organelle function on an unconventional secretory pathway, but no functional studies have been reported so far, so does its closest homolog AtEXO70E1 (Wang et al., 2010; Ding et al., 2014; Lin et al., 2015). OsEXO70E1 is involved in defense against herbivorous planthoppers by interacting with Bph6, which increases the exocytosis and leads to cell wall thickening (Guo et al., 2018). In this study, transient and stable overexpression of EXO70E1-V had no obvious effect on the resistance of wheat when compared with the Yangmai158 (Figure 5). Consequently, we speculated that the function of EXO70E1-V was possibly more inclined to AtEXO70E2, which is only responsible for recruiting other subunits to the area of active secretion, rather than participating in the later biochemical process. The overexpression of EXO70E1-V does not increase the resistance of wheat to Pm, which indicated that it may require the participation of other proteins, such as its interaction protein CMPG1-V. Meanwhile, we also transiently silenced EXO70E1-V gene in H. villosa by virus-induced gene silencing using the barley stripe mosaic virus-mediated system; however, we did not change the Pm resistance level of H. villosa (data not shown). We suspected that it may be due to the presence of Pm21 that showed high resistance to Pm (Cao et al., 2011). The function of EXO70E1-V in Pm resistance needs to be further verified.
The phytohormone SA is closely related to disease resistance by inducing the expression of disease-related (PR) genes or as an early signal component (Vlot et al., 2009). H2O2 accumulates in plant mesophyll cells, it induces the hypersensitive reaction, and the oxidative burst (production of ROS, including H2O2) was related to the resistance of plants to the pathogen. In a previous study, the expression of CMPG1-V in H. villosa was increased when treated with SA, ABA, and H2O2, and the CMPG1-V transgenic plants show improved Pm resistance due to enhanced expression of SA-responsive genes and H2O2 accumulation (Zhu et al., 2015). Our results showed that EXO70E1-V was also quickly induced by SA treatments, and EXO70E1-V and CMPG1-V were interacting on the PM. This indicates that CMPG1-V and EXO70E1-V may participate in the same hormone pathway. We speculated that EXO70E1-V participates in the transmission of different hormone signals in the process of CMPG1-V disease resistance to help CMPG1-V resist the infection of Pm.
Data Availability Statement
The raw data supporting the conclusions of this article will be made available by the authors, without undue reservation, to any qualified researcher.
Author Contributions
JX, XW, JZ, and HZ conceived and designed the study and wrote the manuscript. JZ, HZ, XZ, ZW, YN, and YC analyzed the data. JZ, LS, HW, and HZ collected the plant materials. JZ, HZ, and XZ performed the experiments. All authors reviewed and edited the manuscript.
Funding
This study was supported by the National Key Research and Development Program (Nos. 2016YFD0101004 and 2016YFD0102001-004), the National Science Foundation of China (Nos. 31471490, 31661143005, and 31290213), the Important National Science & Technology Specific Projects in Transgenic Research (No. 2014ZX0800907B), the High Tech Program of China (No. 2011AA1001), the Program of Introducing Talents of Discipline to Universities (No. B08025), a project funded by the Priority Academic Program Development of Jiangsu Higher Education Institutions (PAPD), the 333 Talent Project of Jiangsu Province and Shanghai Agriculture Applied Technology Development Program, China (No. Z201502), and SAAS Program for Excellent Research Team.
Conflict of Interest
The authors declare that the research was conducted in the absence of any commercial or financial relationships that could be construed as a potential conflict of interest.
Supplementary Material
The Supplementary Material for this article can be found online at: https://www.frontiersin.org/articles/10.3389/fpls.2021.652337/full#supplementary-material
Footnotes
- ^ http://plants.ensembl.org/index.html
- ^ http://202.194.139.32/expression/index.html
- ^ http://www.zeiss.com
References
Abas, L., Benjamins, R., Malenica, N., Paciorek, T., Wisniewska, J., Moulinier-Anzola, J. C., et al. (2006). Intracellular trafficking and proteolysis of the Arabidopsis auxin-efflux facilitator PIN2 are involved in root gravitropism. Nat. Cell Biol. 8, 249–256. doi: 10.1038/ncb1369
Barberon, M., Zelazny, E., Robert, S., Conejero, G., Curie, C., Friml, J., et al. (2011). Monoubiquitin-dependent endocytosis of the iron-regulated transporter 1 (IRT1) transporter controls iron uptake in plants. Proc. Natl. Acad. Sci. U.S.A. 108, E450–E458.
Cao, A., Xing, L., Wang, X., Yang, X., Wang, W., Sun, Y., et al. (2011). Serine/threonine kinase gene Stpk-V, a key member of powdery mildew resistance gene Pm21, confers powdery mildew resistance in wheat. Proc. Natl. Acad. Sci. U.S.A. 108, 7727–7732. doi: 10.1073/pnas.1016981108
Chen, H., Zou, Y., Shang, Y., Lin, H., Wang, Y., Cai, R., et al. (2008). Firefly luciferase complementation imaging assay for protein-protein interactions in plants. Plant Physiol 146, 368–376.
Cole, R. A., Mcinally, S. A., and Fowler, J. E. (2014). Developmentally distinct activities of the exocyst enable rapid cell elongation and determine meristem size during primary root growth in Arabidopsis. BMC Plant Biol. 14:386. doi: 10.1186/s12870-014-0386-0
Ding, Y., Wang, J., Chun Lai, J. H., Ling Chan, V. H., Wang, X., Cai, Y., et al. (2014). Exo70E2 is essential for exocyst subunit recruitment and EXPO formation in both plants and animals. Mol. Biol. Cell 25, 412–426. doi: 10.1091/mbc.e13-10-0586
Drdova, E. J., Synek, L., Pecenkova, T., Hala, M., Kulich, I., Fowler, J. E., et al. (2013). The exocyst complex contributes to PIN auxin efflux carrier recycling and polar auxin transport in Arabidopsis. Plant J. 73, 709–719. doi: 10.1111/tpj.12074
Du, Y., Overdijk, E. J. R., Berg, J. A., Govers, F., and Bouwmeester, K. (2018). Solanaceous exocyst subunits are involved in immunity to diverse plant pathogens. J. Exp. Bot. 69, 655–666. doi: 10.1093/jxb/erx442
Elias, M. (2003). The exocyst complex in plants. Cell Biol. Int. 27, 199–201. doi: 10.1016/s1065-6995(02)00349-9
Faheem, M., Li, Y., Arshad, M., Jiangyue, C., Jia, Z., Wang, Z., et al. (2016). A disulphide isomerase gene (PDI-V) from Haynaldia villosa contributes to powdery mildew resistance in common wheat. Sci. Rep. 6:24227.
Fendrych, M., Synek, L., Pecenkova, T., Toupalova, H., Cole, R., Drdova, E., et al. (2010). The Arabidopsis exocyst complex is involved in cytokinesis and cell plate maturation. Plant Cell 22, 3053–3065. doi: 10.1105/tpc.110.074351
Guo, J., Xu, C., Wu, D., Zhao, Y., Qiu, Y., Wang, X., et al. (2018). Bph6 encodes an exocyst-localized protein and confers broad resistance to planthoppers in rice. Nat. Genet. 50:297–306. doi: 10.1038/s41588-018-0039-6
Hala, M., Cole, R., Synek, L., Drdova, E., Pecenkova, T., Nordheim, A., et al. (2008). An exocyst complex functions in plant cell growth in Arabidopsis and tobacco. Plant Cell 20, 1330–1345. doi: 10.1105/tpc.108.059105
Hong, D., Jeon, B. W., Kim, S. Y., Hwang, J. U., and Lee, Y. (2016). The ROP2-RIC7 pathway negatively regulates light-induced stomatal opening by inhibiting exocyst subunit Exo70B1 in Arabidopsis. New Phytol. 209, 624–635. doi: 10.1111/nph.13625
Jankova Drdova, E., Klejchova, M., Janko, K., Hala, M., Soukupova, H., Cvrckova, F., et al. (2019). Developmental plasticity of Arabidopsis hypocotyl is dependent on exocyst complex function. J. Exp. Bot. 70, 1255–1265. doi: 10.1093/jxb/erz005
Kalmbach, L., Hematy, K., De Bellis, D., Barberon, M., Fujita, S., Ursache, R., et al. (2017). Transient cell-specific EXO70A1 activity in the CASP domain and Casparian strip localization. Nat. Plants 3:17058.
Kulich, I., Cole, R., Drdova, E., Cvrckova, F., Soukup, A., Fowler, J., et al. (2010). Arabidopsis exocyst subunits SEC8 and EXO70A1 and exocyst interactor ROH1 are involved in the localized deposition of seed coat pectin. New Phytol. 188, 615–625. doi: 10.1111/j.1469-8137.2010.03372.x
Kulich, I., Pecenkova, T., Sekeres, J., Smetana, O., Fendrych, M., Foissner, I., et al. (2013). Arabidopsis exocyst subcomplex containing subunit EXO70B1 is involved in autophagy-related transport to the vacuole. Traffic 14, 1155–1165.
Kulich, I., Vojtikova, Z., Sabol, P., Ortmannova, J., Nedela, V., Tihlarikova, E., et al. (2018). Exocyst subunit EXO70H4 has a specific role in callose synthase secretion and silica accumulation. Plant Physiol. 176, 2040–2051. doi: 10.1104/pp.17.01693
Lai, K. S. (2016). Analysis of EXO70C2 expression revealed its specific association with late stages of pollen development. Plant Cell Tissue Organ Cult. 124, 209–215. doi: 10.1007/s11240-015-0881-5
Lee, H. K., Cho, S. K., Son, O., Xu, Z., Hwang, I., and Kim, W. T. (2009). Drought stress-induced Rma1H1, a RING membrane-anchor E3 ubiquitin ligase homolog, regulates aquaporin levels via ubiquitination in transgenic Arabidopsis plants. Plant Cell 21, 622–641. doi: 10.1105/tpc.108.061994
Li, S., Chen, M., Yu, D., Ren, S., Sun, S., Liu, L., et al. (2013). EXO70A1-mediated vesicle trafficking is critical for tracheary element development in Arabidopsis. Plant Cell 25, 1774–1786. doi: 10.1105/tpc.113.112144
Lin, Y., Ding, Y., Wang, J., Shen, J., Kung, C. H., Zhuang, X., et al. (2015). Exocyst-positive organelles and autophagosomes are distinct organelles in plants. Plant Physiol. 169, 1917–1932.
Liu, N., Hake, K., Wang, W., Zhao, T., Romeis, T., and Tang, D. (2017). CALCIUM-DEPENDENT PROTEIN KINASE5 associates with the truncated NLR Protein TIR-NBS2 to contribute to exo70B1-mediated immunity. Plant Cell 29, 746–759. doi: 10.1105/tpc.16.00822
Lu, D., Lin, W., Gao, X., Wu, S., Cheng, C., Avila, J., et al. (2011). Direct ubiquitination of pattern recognition receptor FLS2 attenuates plant innate immunity. Science 332, 1439–1442. doi: 10.1126/science.1204903
Ma, W., Wang, Y., Yao, X., Xu, Z., An, L., and Yin, M. (2016). The role of Exo70 in vascular smooth muscle cell migration. Cell Mol. Biol. Lett. 21:20.
Oda, Y., Iida, Y., Nagashima, Y., Sugiyama, Y., and Fukuda, H. (2015). Novel coiled-coil proteins regulate exocyst association with cortical microtubules in xylem cells via the conserved oligomeric golgi-complex 2 protein. Plant Cell Physiol. 56, 277–286. doi: 10.1093/pcp/pcu197
Pecenkova, T., Hala, M., Kulich, I., Kocourkova, D., Drdova, E., Fendrych, M., et al. (2011). The role for the exocyst complex subunits Exo70B2 and Exo70H1 in the plant-pathogen interaction. J. Exp. Bot. 62, 2107–2116. doi: 10.1093/jxb/erq402
Robinson, D. G., Ding, Y., and Jiang, L. (2016). Unconventional protein secretion in plants: a critical assessment. Protoplasma 253, 31–43. doi: 10.1007/s00709-015-0887-1
Roulin, A., Auer, P. L., Libault, M., Schlueter, J., Farmer, A., May, G., et al. (2013). The fate of duplicated genes in a polyploid plant genome. Plant J. 73, 143–153. doi: 10.1111/tpj.12026
Sabol, P., Kulich, I., and Zarsky, V. (2017). RIN4 recruits the exocyst subunit EXO70B1 to the plasma membrane. J. Exp. Bot. 68, 3253–3265. doi: 10.1093/jxb/erx007
Safavian, D., Zayed, Y., Indriolo, E., Chapman, L., Ahmed, A., and Goring, D. R. (2015). RNA silencing of exocyst genes in the stigma impairs the acceptance of compatible pollen in Arabidopsis. Plant Physiol. 169, 2526–2538.
Seo, D. H., Ahn, M. Y., Park, K. Y., Kim, E. Y., and Kim, W. T. (2016). The N-Terminal UND Motif of the Arabidopsis U-Box E3 Ligase PUB18 Is critical for the negative regulation of aba-mediated stomatal movement and determines its ubiquitination specificity for exocyst subunit Exo70B1. Plant Cell 28, 2952–2973. doi: 10.1105/tpc.16.00347
Shi, X., and Ling, H. Q. (2018). Current advances in genome sequencing of common wheat and its ancestral species. Crop J. 6, 17–23.
Shirasu, K., Nielsen, K., Piffanelli, P., Oliver, R., and Schulze-Lefert, P. (1999). Cell-autonomous complementation of mlo resistance using a biolistic transient expression system. Plant J. 17, 293–299. doi: 10.1046/j.1365-313X.1999.00376.x
Stegmann, M., Anderson, R. G., Ichimura, K., Pecenkova, T., Reuter, P., Zarsky, V., et al. (2012). The ubiquitin ligase PUB22 targets a subunit of the exocyst complex required for PAMP-triggered responses in Arabidopsis. Plant Cell 24, 4703–4716. doi: 10.1105/tpc.112.104463
Synek, L., Schlager, N., Elias, M., Quentin, M., Hauser, M. T., and Zarsky, V. (2006). AtEXO70A1, a member of a family of putative exocyst subunits specifically expanded in land plants, is important for polar growth and plant development. Plant J. 48, 54–72. doi: 10.1111/j.1365-313x.2006.02854.x
Synek, L., Vukasinovic, N., Kulich, I., Hala, M., Aldorfova, K., Fendrych, M., et al. (2017). EXO70C2 Is a key regulatory factor for optimal tip growth of pollen. Plant Physiol. 174, 223–240. doi: 10.1104/pp.16.01282
Tamura, K., Stecher, G., Peterson, D., Filipski, A., and Kumar, S. (2013). MEGA6: molecular evolutionary genetics analysis version 6.0. Mol. Biol. Evol. 30, 2725–2729. doi: 10.1093/molbev/mst197
Tu, B., Hu, L., Chen, W., Li, T., Hu, B., Zheng, L., et al. (2015). Disruption of OsEXO70A1 causes irregular vascular bundles and perturbs mineral nutrient assimilation in rice. Sci. Rep. 5:18609.
Vlot, A. C., Dempsey, D. A. and Klessig, D. F. (2009). Salicylic Acid, a multifaceted hormone to combat disease. Annu. Rev. Phytopathol. 47, 177–206. doi: 10.1146/annurev.phyto.050908.135202
Vukasinovic, N., Oda, Y., Pejchar, P., Synek, L., Pecenkova, T., Rawat, A., et al. (2017). Microtubule-dependent targeting of the exocyst complex is necessary for xylem development in Arabidopsis. New Phytol. 213, 1052–1067. doi: 10.1111/nph.14267
Wang, J., Ding, Y., Wang, J., Hillmer, S., Miao, Y., Lo, S. W., et al. (2010). EXPO, an exocyst-positive organelle distinct from multivesicular endosomes and autophagosomes, mediates cytosol to cell wall exocytosis in Arabidopsis and tobacco cells. Plant Cell 22, 4009–4030. doi: 10.1105/tpc.110.080697
Wang, Z., Cheng, J., Fan, A., Zhao, J., Yu, Z., Li, Y., et al. (2018). LecRK-V, an L-type lectin receptor kinase in Haynaldia villosa, plays positive role in resistance to wheat powdery mildew. Plant Biotechnol. J. 16, 50–62. doi: 10.1111/pbi.12748
Wang, Z., Li, P., Yang, Y., Chi, Y., Fan, B., and Chen, Z. (2016). Expression and functional analysis of a novel group of legume-specific WRKY and Exo70 protein variants from soybean. Sci. Rep. 6:32090.
Wu, J., Tan, X., Wu, C., Cao, K., Li, Y., and Bao, Y. (2013). Regulation of cytokinesis by exocyst subunit SEC6 and KEULE in Arabidopsis thaliana. Mol. Plant 6, 1863–1876. doi: 10.1093/mp/sst082
Xing, L., Wang H., Jiang Z., Ni J., Cao A., Yu L., et al. (2008). Transformation of wheat thaumatin-like protein gene and diseases resistance analysis of transgenic plants. Acta Agron. Sin. 34, 349–354.
Zhao, J., Zhang, X., Wan, W., Zhang, H., Liu, J., Li, M., et al. (2018). Identification and characterization of the EXO70 gene family in polyploid wheat and related species. Int. J. Mol. Sci. 20:60. doi: 10.3390/ijms20010060
Zhao, T., Rui, L., Li, J., Nishimura, M. T., Vogel, J. P., Liu, N., et al. (2015). A truncated NLR protein, TIR-NBS2, is required for activated defense responses in the exo70B1 mutant. PLoS Genet. 11:e1004945. doi: 10.1371/journal.pgen.1004945
Keywords: EXO70E1-V, CMPG1-V, Haynaldia villosa, interaction protein, powdery mildew
Citation: Zhao J, Zhang H, Zhang X, Wang Z, Niu Y, Chen Y, Sun L, Wang H, Wang X and Xiao J (2021) The Exocyst Complex Subunit EXO70E1-V From Haynaldia villosa Interacts With Wheat Powdery Mildew Resistance Gene CMPG1-V. Front. Plant Sci. 12:652337. doi: 10.3389/fpls.2021.652337
Received: 12 January 2021; Accepted: 08 June 2021;
Published: 08 July 2021.
Edited by:
Zuhua He, Center for Excellence in Molecular Plant Sciences, Chinese Academy of Sciences (CAS), ChinaReviewed by:
Zhiyong Liu, Institute of Genetics and Developmental Biology, Chinese Academy of Sciences (CAS), ChinaQian-Hua Shen, Institute of Genetics and Developmental Biology, Chinese Academy of Sciences (CAS), China
Copyright © 2021 Zhao, Zhang, Zhang, Wang, Niu, Chen, Sun, Wang, Wang and Xiao. This is an open-access article distributed under the terms of the Creative Commons Attribution License (CC BY). The use, distribution or reproduction in other forums is permitted, provided the original author(s) and the copyright owner(s) are credited and that the original publication in this journal is cited, in accordance with accepted academic practice. No use, distribution or reproduction is permitted which does not comply with these terms.
*Correspondence: Jin Xiao, eGlhb2ppbkBuamF1LmVkdS5jbg==
†These authors have contributed equally to this work