- 1Field and Horticultural Crops Research Department, Kurdistan Agricultural and Natural Resources Research and Education Center, Agricultural Research, Education and Extension Organization (AREEO), Sanandaj, Iran
- 2Department of Agronomy and Plant Breeding Science, College of Aburaihan, University of Tehran, Tehran, Iran
- 3Department of Plant Biotechnology, Faculty of Sciences & Biotechnology, Shahid Beheshti University, G.C., Tehran, Iran
- 4Department of Horticulture, Michigan State University, East Lansing, MI, United States
Abiotic stresses, mainly salinity and drought, are the most important environmental threats that constrain worldwide food security by hampering plant growth and productivity. Plants cope with the adverse effects of these stresses by implementing a series of morpho-physio-biochemical adaptation mechanisms. Accumulating effective osmo-protectants, such as proline and glycine betaine (GB), is one of the important plant stress tolerance strategies. These osmolytes can trigger plant stress tolerance mechanisms, which include stress signal transduction, activating resistance genes, increasing levels of enzymatic and non-enzymatic antioxidants, protecting cell osmotic pressure, enhancing cell membrane integrity, as well as protecting their photosynthetic apparatus, especially the photosystem II (PSII) complex. Genetic engineering, as one of the most important plant biotechnology methods, helps to expedite the development of stress-tolerant plants by introducing the key tolerance genes involved in the biosynthetic pathways of osmolytes into plants. Betaine aldehyde dehydrogenase (BADH) is one of the important genes involved in the biosynthetic pathway of GB, and its introduction has led to an increased tolerance to a variety of abiotic stresses in different plant species. Replacing down-regulated ferredoxin at the acceptor side of photosystem I (PSI) with its isofunctional counterpart electron carrier (flavodoxin) is another applicable strategy to strengthen the photosynthetic apparatus of plants under stressful conditions. Heterologous expression of microbially-sourced flavodoxin (Fld) in higher plants compensates for the deficiency of ferredoxin expression and enhances their stress tolerance. BADH and Fld are multifunctional transgenes that increase the stress tolerance of different plant species and maintain their production under stressful situations by protecting and enhancing their photosynthetic apparatus. In addition to increasing stress tolerance, both BADH and Fld genes can improve the productivity, symbiotic performance, and longevity of plants. Because of the multigenic and complex nature of abiotic stresses, the concomitant delivery of BADH and Fld transgenes can lead to more satisfying results in desired plants, as these two genes enhance plant stress tolerance through different mechanisms, and their cumulative effect can be much more beneficial than their individual ones. The importance of BADH and Fld genes in enhancing plant productivity under stress conditions has been discussed in detail in the present review.
Introduction
Drought and salinity are the most important abiotic stresses that are currently challenging global food security by reducing agricultural productivity and quality. The ever-increasing population of the world needs constant improvement in crop productivity to meet the growing food demands. Salinity stress is more pronounced in agricultural regions with water scarcity and inadequate irrigation (Isayenkov, 2019). Cellular dehydration is inevitable in salinity stress, which makes the mechanism of drought and salinity stress similar (Shailani et al., 2020; Wai et al., 2020). Therefore, increasing tolerance to salinity and drought stress can be done in similar ways. Drought and salinity impair the photosynthesis and transpiration of plants by decreasing levels of chlorophyll and carotenoids, distorting the chloroplast ultrastructure and photosystem II (PSII) complex, and reducing stomatal conductance (Pan et al., 2020). Photosynthesis is a pivotal physio-biochemical process of plants that is also highly sensitive to abiotic stresses. Abiotic stresses have adverse effects on PSII activity by disintegrating PSII reaction centers, oxygen-evolving complex (OEC), and limiting the activity of quinone acceptors. The photosynthetic electron transport chain (PETC) in plants, especially electron carriers at the acceptor side of photosystem I (PSI), are vulnerable to abiotic stresses (Arif et al., 2020; Chen et al., 2020). Cellular dehydration and toxicity along with high accumulation of reactive oxygen species (ROS) are other consequences of drought and salinity, which eventually lead to programmed cell death (PCD) (Latif et al., 2020). Although, reactive oxygen species oxidize and disrupt some cellular components compromising their original functions, at low levels, they can act as important signaling molecules and second messengers to regulate plant growth and stress responses. Therefore, maintaining the equilibrium of production and the scavenging of ROS is important; abiotic stresses disturb this balance, leading to increased intracellular ROS levels (Huang et al., 2019). Plants respond to harsh environments by applying an array of tolerance mechanisms. Cellular detoxification activation, osmoprotectants accumulation, antioxidant machinery activation (both enzymatic and non-enzymatic), signaling pathways activation/regulation, and ROS scavenging are involved in enhancing plant stress tolerance (Niazian and Shariatpanahi, 2020; Shailani et al., 2020). Cell osmotic pressure adjustment and the protection of cell membranes and photosynthesis machinery coupled with other functional macromolecules are vital measures to eliminate the harmful effects of abiotic stresses (Niazian et al., 2019). The activation of biosynthetic enzymes to produce higher levels of stress protectants and antioxidant compounds is one of the plant's strategies to quench the harmful oxidizing molecules (Wai et al., 2020).
Genetic engineering is one of the widely applied biotechnology-based breeding methods (BBBMs) to enhance a plant's resistance to stressful conditions. Key tolerance genes, explored from different sources, have been introduced into various plant species to improve abiotic stress tolerance. According to the functional analysis of gene products, the genes of interest for enhancing abiotic stresses tolerance are involved in (i) encoding ion transporters, (ii) osmolyte biosynthesis, (iii) antioxidant machinery, (iv) encoding stress-induced proteins, and (v) encoding transcription factors (Isayenkov, 2019). All of these genes try to mitigate the adverse effects of abiotic stresses and keep the photosynthesis efficiency and subsequent productivity of plants at an acceptable level. The introduction of K2-NhaD, a plasma membrane Na+/H+ antiporter encoding gene, to upland cotton (Gossypium hirsutum L.), led to a considerable increase of relative water content, chlorophyll, soluble sugar, proline levels, and superoxide dismutase (SOD), catalase (CAT), and peroxidase (POX) activity in transgenic plants more so than wild types under drought and salinity conditions (Guo et al., 2020). The overexpression of the IbCBF3 transcription factor enhanced low temperature and drought stress tolerance in transgenic sweet potatoes (Ipomoea batatas [L.] Lam) (Jin et al., 2017). Zhou et al. (2020a) transferred the Arabidopsis malonyl-CoA synthetase gene AAE13.1 (AtAAE13.1) to cell suspension cultures of rice (Oryza sativa L.), cotton (Gossypium hirsutum L.), and white pine (Pinus strobus L.) using the GV3101 strain of Agrobacterium tumefaciens. Reportedly, salinity stress tolerance was enhanced in transgenic cells with higher cell viability and growth rates and increased content levels of amino acids, glycolate, phosphoglycolate, sucrose, glucose, and fructose. It also showed lower lipid peroxidation and an increased oxidation rate (Zhou et al., 2020a). Overexpression of the NtabDOG1L gene showed drought tolerance improvement in tobacco (Nicotiana tabacum) by increasing antioxidant capacity and up-regulating the expression of drought defense genes (Zhang X. et al., 2019). There are some other key genes that can improve plant stress tolerance through a combination of the above-mentioned functions. In rice, overexpression of the OsRab7 gene enhanced drought and heat tolerance by modulating osmolytes, antioxidants, and abiotic stress-responsive gene expression (El-Esawi and Alayafi, 2019a). Overexpression of the StDREB2 transcription factor increased drought tolerance in cotton by improving gas-exchange performance, ROS scavenging, antioxidant enzyme activities, osmolytes accumulation, and expression of stress-related genes (El-Esawi and Alayafi, 2019b). The introduction of the codA gene from Arthrobacter globiformis into the potato (Solanum tuberosum) plastid genome, led to higher glycine betaine (GB) osmolyte accumulation, and higher relative water and chlorophyll content of the transformed plants grown under drought stress conditions (You et al., 2019).
Furthermore, genetic engineering increased the photosynthetic efficiency of plants under stressful conditions via the substitution of a stress-vulnerable key electron carrier at the acceptor side of PSI in the PETC (Zurbriggen et al., 2010). Ferredoxin (Fd)—the final electron acceptor of the PETC—is a multifunctional electron carrier iron-sulfur protein in photosynthetic organisms. Ferredoxin has critical roles in the CO2 fixation cycle, nitrogen/sulfur assimilation, amino acid synthesis, fatty acid desaturation, antioxidant regeneration, and different regulation, dissipation, and biosynthetic pathways (Zurbriggen et al., 2008; Gharechahi et al., 2015). Through interaction with Fd–NADP+ reductase (FNR), photoreduced Fd can generate the NADPH required for carbon assimilation and other biosynthetic and protective pathways (Figure 1). In addition, Fd can affect different metabolic regulatory and dissipative routes of the stroma by diverting reducing equivalents back to the cytochrome b6f complex in the PETC or to soluble electron acceptor enzymes involved in the pathways (Figure 1) (Giró et al., 2011). The significant role of Fd in plant stress responses has also been reported in several studies (Ma et al., 2008). However, environmental stresses can significantly down-regulate Fd expression and NADP+ deficiency and therefore compromise plant survival. Photosynthetic microorganisms like cyanobacteria and some algae have an alternative strategy in stressful environmental conditions. They compensate for a deficiency of Fd expression by activating the expression of flavodoxin (Fld)—an isofunctional mobile electron carrier protein that contains a prosthetic group of flavin mononucleotide—and keep their photosynthetic efficiency under stressful situations like iron deficiency, high illumination, salinity, and drought (Zurbriggen et al., 2007).
Increasing the accumulation of protective compatible solutes with compensation for a reduced level of Fd in PETC, through genetic engineering techniques, are two fast and applicable strategies to enhance stress tolerance of plants and to maintain their photosynthetic efficiency and productivity under stressful conditions. The principles of increasing plant stress tolerance using these two strategies are presented in the following sections of the present review.
Compatible Solutes and Osmotic Adjustment
Osmotic tolerance is one of the first plant responses to challenging environmental conditions, and it is achieved through rapid long-distance (root to shoot) signaling mechanisms (Isayenkov and Maathuis, 2019). Mobile molecules, such as small proteins, peptides, RNAs, metabolites, and second messengers, are involved in long-distance signaling in plant stress response (Takahashi and Shinozaki, 2019). Osmoprotectants/compatible solutes, including amino acids (proline), betaines (glycine betain), polyamines, and sugars are low-molecular-weight highly soluble non-toxic compounds. They act as ROS scavengers and are involved in a wide range of biological processes through interaction with antioxidative enzymes, stabilizing proteins, and plasma membranes (Chen and Murata, 2002). Despite their different biochemical groups, compatible solutes have similar roles in protecting plants against stresses (Surabhi and Rout, 2020). These organic osmolytes have significant roles in enzyme and membrane integrity and in the osmotic adjustment of plants under stress conditions (Ashraf and Foolad, 2007). A comparative analysis of 11 sugar beet cultivars under drought stress reported that drought-tolerant cultivars increased the accumulation of proline and GB osmolytes (Islam et al., 2020). Exogenous proline significantly enhanced cell membrane integrity, leaf water content, and photosynthetic efficiency and also up-regulated the osmoprotectant content of onions (Allium cepa L.) under drought stress growing conditions (Semida et al., 2020).
Osmolytes and related enzymes in their metabolism are important in plant survival under abiotic stress (Ozturk et al., 2020). Enhancing the endogenous accumulation of organic solutes responsible for cellular osmotic adjustment, such as mannitol, glycine, betaine, proline, myo-inositol, and sugars, by up- or down-regulation of genes involved in their synthetic pathway, can increase plants tolerance to major abiotic stresses, especially those of drought and salinity (Paschalidis et al., 2019). Glycine betaine and proline are the most common osmolytes under stress conditions (Ozturk et al., 2020). The exogenous application of proline can be more effective than GB in alleviating the adverse effect of abiotic stresses in some plants (Youssef et al., 2018; Bhuiyan et al., 2019). However, the introduction of GB biosynthetic genes is more effective than other osmoprotectant genes in enhancing plant abiotic stress tolerance (Surabhi and Rout, 2020). Recent studies indicated that the GB is the most important osmoprotectant in inducing stress tolerance (Hasan et al., 2020; Zhou et al., 2020b).
Glycine Betaine and Betaine Aldehyde Dehydrogenase (BADH) Gene
Glycine betaine is one of the main quaternary ammonium compounds (QAC) produced in cash crops. Among prominent betaines in plants, including glycine betaine, proline betaine, and beta alanine, GB is more significant and an extensively studied osmoprotectant. Glycine betaine enrichment is one of the common responses that higher plants have to drought and salinity stresses (Ashraf and Foolad, 2007). Glycine betaine can maintain osmotic adjustment by maintaining a high Na+ to K+ ratio and lowering the toxic effects of ions through accumulation in the cell (Arif et al., 2020). GB is chemically neutral and can interact with domains of both hydrophilic and hydrophobic macromolecules, such as enzymes and protein complexes, due to its molecular properties (Hasan et al., 2020). By providing osmotic adjustment, GB is able to protect the PSII complex, biomembrane system, and the quaternary structures of other pivotal enzymes and proteins (Golestan Hashemi et al., 2018; Surabhi and Rout, 2020). Betaine is a unique compatible solute, which exists semi-permanently or permanently in plant cells and has slower degradation when compared to other osmolytes (Chen and Murata, 2002). Glycine betaine is able to mitigate the detrimental effects of drought and salinity stresses through a wide range of physio-chemical processes. Through improving the Ca2+-ATPase and Hill reaction activities in the thylakoid membrane system, GB increases photosynthesis efficiency under drought stress conditions (Nawaz and Wang, 2020). The foliar application of GB induced the salinity tolerance in onions by up-regulating antioxidative defense systems, including non-enzymatic (glutathione and ascorbic acid), enzymatic [CAT, SOD, and ascorbate peroxidase (APX)] antioxidants, photosynthetic efficiency, and increasing the membrane stability index (Rady et al., 2018). Similarly, the exogenous application of GB enhanced the cold stress tolerance of perennial (Lolium perenne L.) and annual (L. multiflorum) ryegrass (Mickelbart and Boine, 2020) along with the drought tolerance of sweet potatoes (Ipomoea batatas) (Tisarum et al., 2020). A comparative analysis of the exogenous application of abscisic acid (ABA) and GB on enhancing the drought tolerance of Axonopus compressus revealed that GB was more effective than ABA. This study showed that exogenous GB application resulted in increasing levels of soluble sugars, proteins, proline, phenolics, and chlorophyll in addition to increasing activity of enzymatic antioxidants SOD, CAT, POX, and APX (Nawaz and Wang, 2020).
Betaine aldehyde dehydrogenase (BADH) is one of the genes that encode the enzymes involved in the synthesis of GB and it is responsible for the second oxidation reaction in the GB synthesis pathway. Both choline monooxygenase (CMO) and BADH genes are important in GB synthesis in higher plants. These two genes can be involved in the transcriptional regulation of different biological processes, such as stress and hormone responses, through their cis-effective regulatory elements (CREs) (Ozturk et al., 2020). Glycine betaine has an important role in manipulating gene expression, as GB-induced expression of specific genes (especially ROS-scavenging enzymes) and transcription factors (Chen and Murata, 2008, 2011; Xu et al., 2018). Yamada et al. (2015) reported that transgenic sugar beet plants with knocked out CMO, through antisense BvCMO, were more susceptible to salt stress when compared to wild-type plants. These results verified the importance of these two genes in GB synthesis and in enhancing tolerance to abiotic stresses. The BADH gene can be cloned from higher plants and other types of living organisms (Chen and Murata, 2002). Spinach (Spinacia oleracea), sugar beets (Beta vulgaris), barley (Hordeum vulgare), quinoa (Chenopodium quinoa), and rice are the main sources of BADH cloning (Yamada et al., 2015; Jiang et al., 2016). Another gene with BADH activity has been reported in Lycium ruthenicum, LrAMADH1, which is responsible for increasing GB accumulation under NaCl stress (Liu et al., 2018). Other than abiotic stress mechanisms, multifunctional BADH is involved in fragrance production (through polyamines oxidation pathway), and as a selectable marker in the antibiotic-free selection of transgenic plants. This gene is involved in a plant's abiotic stress response through the mitogen-activated protein kinase (MAPK) signaling pathway (Golestan Hashemi et al., 2018). Therefore, BADH is involved in a plant's tolerance to abiotic stresses through the MAPK signaling pathway and metabolic synthesis of GB. Introduction of the BADH gene can lead to enhanced abiotic stress tolerance in desired plant species. Enhanced tolerance to abiotic stresses in BADH-overexpressing transgenic plants has been reported in different plant species (Table 1).
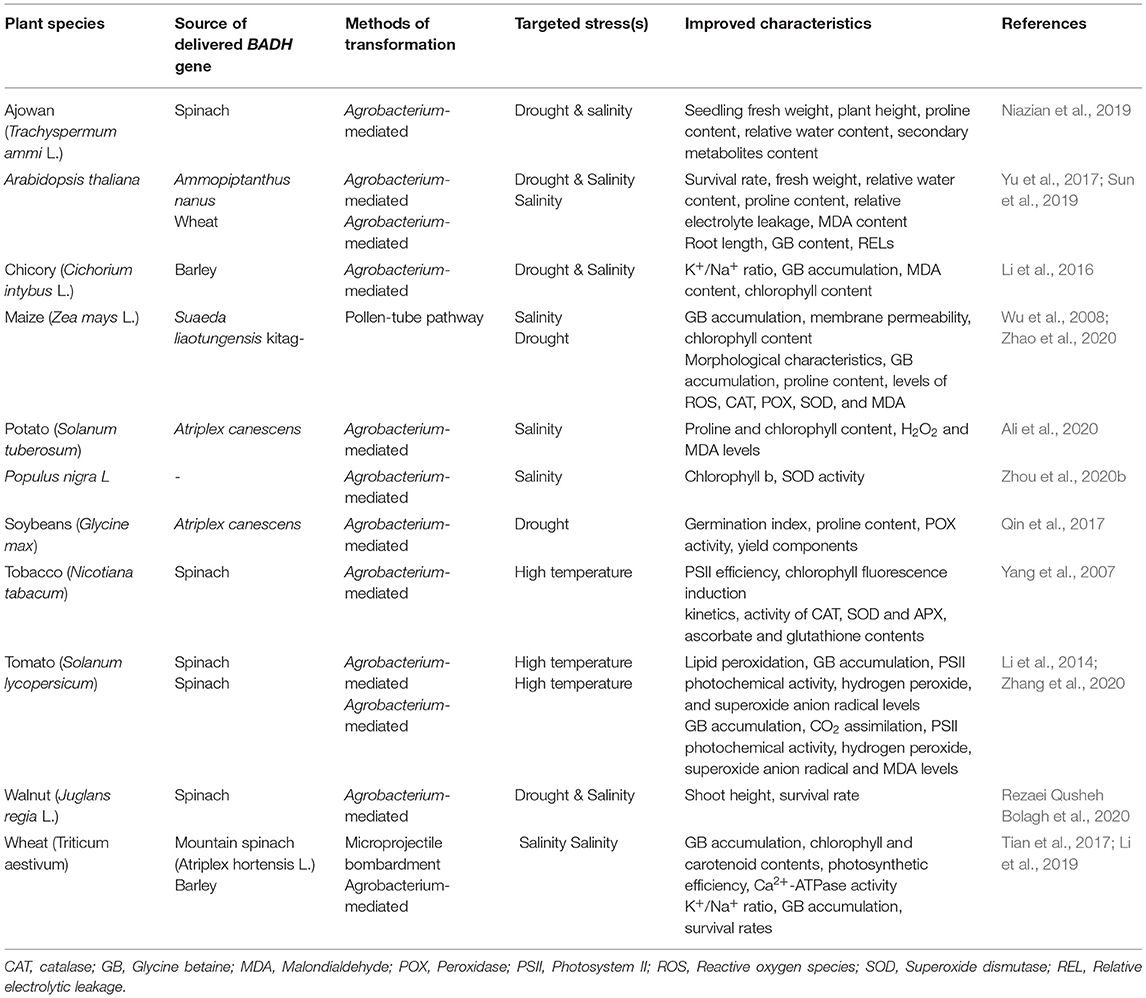
Table 1. Examples of transferred BADH genes to enhance abiotic stresses in different plants species.
The Agrobacterium-mediated transformation of soybeans by a BADH gene from Atriplex canescens increased the germination index of transgenic lines under osmotic stress compared with the control plants. In addition, the proline content, POX activity, and yield components of transgenic lines were higher than the control plants (Qin et al., 2017). The Agrobacterium-mediated transformation of Persian walnuts (Juglans regia L.) cv. Chandler using a spinach BADH gene led to enhanced tolerance to osmotic (PEG) and salinity (NaCl) stresses (Rezaei Qusheh Bolagh et al., 2020). The overexpression of the Ammopiptanthus nanus BADH gene, a xerophyte leguminous shrub, in Arabidopsis (Arabidopsis thaliana) led to a significantly increased tolerance of Arabidopsis transgenic plants to high salt and drought stresses. This was a result of an increased content of GB and proline, higher relative water content, and lower relative electrolyte leakage and malondialdehyde (MDA) content in relation to the untransformed plants (Yu et al., 2017). Li et al. (2019) transferred the HvBADH1 gene from hulless barley to bread wheat (Triticum aestivum) and reported a higher K+/Na+ ratio, greater accumulation of GB, and higher seedling survival rates of transgenic lines under severe salinity stress (150 mM NaCl). The heterologous expression of the hulless barley BADH (HvBADH1) gene enhanced drought and salinity stress in chicory (Cichorium intybus L.) (Li et al., 2016). Zhang et al. (2020) compared codA- and BADH-transgenic tomato (Solanum lycopersicum) plants under high-temperature stress and reported that GB level, CO2 assimilation, and photosystem II (PSII) photochemical activity in transgenic plants were more elevated than in wild types. However, hydrogen peroxide (H2O2), superoxide anion radical (), and MDA levels in wild-type plants were all increased than in transgenes ones. Authors also reported that codA tomato plants exhibited higher degrees of enhanced thermotolerance than BADH tomato plants (Zhang et al., 2020). BADH transgenic maize (Zea mays L.) plants showed better germination ability, morphological characteristics, higher levels of antioxidant enzymes (CAT, POX, and SOD), and osmotic regulatory substances, as well as a lower accumulation of MDA compared with wild type plants under drought stress conditions (Zhao et al., 2020). Delivery of the Atriplex canescens BADH gene to potatoes followed by increased proline and chlorophyll levels as well as decreased H2O2 and MDA levels in transgenic plants under salinity stress (Ali et al., 2020). Wheat plants transformed with a BADH gene from mountain spinach (Atriplex hortensis L.) showed higher chlorophyll and carotenoid levels and higher Hill reaction activities as well as higher Ca2+-ATPase activity under NaCl stress. The authors also reported that BADH transgenes over accumulated GB, which protected the components and functions of their thylakoid membranes followed by their enhanced photosynthesis under salinity stress (Tian et al., 2017). Sun et al. (2019) reported an enhanced GB accumulation and lower relative electrolytic leakage (REL) in Arabidopsis plants transformed by a BADH gene from salt-tolerant wheat variety DM1 (Dongnongdongmai 1) under salinity stress.
Metabolic engineering of GB, to enhance plant tolerance to abiotic stresses, can be performed by introducing genes from other types of living organisms, like the codA gene from Arthrobacter globiformis (You et al., 2019), glycine sarcosine methyltransferase (ApGSMT2), and dimethylglycine methyltransferase (ApDMT2) genes from Aphanothece halophytica (Song et al., 2018). A recent study reported overexpression of a novel GB1 gene that effectively increased the content of GB in transgenic maize and soybean plants (Castiglioni et al., 2018).
The other compatible solutes, besides GB, that accumulated through metabolic engineering include fructan, mannitol, D-Ononitol, proline, sorbitol, and trehalose (Chen and Murata, 2002).
Flavodoxin Electron Carriers and FLD Gene
Flavodoxins are small isofunctional electron carriers with the ubiquitous electron shuttle Fd which shuttles low potential electrons between donors and acceptors in redox-based metabolisms. Flavodoxins are specific to some bacteria and oceanic algae as opposite to ferredoxins, which prevalently distribute in all living organisms. Environmental stresses lead to down-regulation of ferredoxin in photosynthetic microorganisms. However, they make use of flavodoxins to perform Fds functions under challenging environmental conditions. Plants have lost the Fld gene and its adaptive advantages during their evolutionary period, somewhere between the green algal ancestor and the first terrestrial plants. Abundance and accessibility of iron in coastal regions and firm land, and the fact that Fd is more efficient than Fld as an electron carrier, are reasonable hypotheses for the loss of the Fld gene from their higher plant genetic pool despite its adaptive advantages (Zurbriggen et al., 2007; Pierella Karlusich et al., 2014). On the other hand, studies showed that flavodoxin can compensate for the down-regulation of PETC acceptor Fd. In Fd knocked-down transgenic tobacco plants, cyanobacterial Fld expression complemented Fd deficiency. Moreover, Fld expression restored growth, chlorophyll pigment content, and photosynthetic capacity, and it relieved electron pressure on the electron transport chain (Blanco et al., 2011). The replacement of down-regulated Fd electron acceptor with Fld helped to reconstruct electron delivery to productive routes rather than O2 and ROS production (Zurbriggen et al., 2008; Giró et al., 2011; Lodeyro et al., 2012), and subsequently led to a higher degree of stress tolerance. This was first demonstrated in the transgenic tobacco plants with an introduced Fld gene, which accumulated lower amounts of ROS under abiotic stress conditions (Tognetti et al., 2006).
Expressing microbially-sourced genes in transgenic plants is a popular strategy for improving plant desired phenotypes (Smith-Moore and Grunden, 2018). Fld is one of these microbial genes whose heterologous expression has enhanced plant abiotic stress tolerance. The overexpression of a cyanobacterial Fld in creeping bentgrass (Agrostis stolonifera L.) enhanced plant performance under oxidative, drought, heat, and nitrogen starvation stresses. It occurred by affecting the expression of heat-shock protein genes, up-regulating the expression of nitrite reductase, and N transporter genes. It also had an impact on increasing production of reduced thioredoxin, water retention, cell membrane integrity, N accumulation, and total chlorophyll content (Li et al., 2017). The comparative proteomic analysis of transgenic tobacco expressing cyanobacterial Fld and wild type plants under drought stress conditions, revealed that Fld expression enhanced drought tolerance by affecting an abundance of drought-responsive proteins (DRPs) such as remurin, ferredoxin-NADP reductase (FNR), chloroplast manganese stabilizing protein-II, phosphoglycerate mutase, and glutathione S-transferase. These DRPs were involved in carbohydrate and energy metabolism as well as oxidative stress responses (Gharechahi et al., 2015). The heterologous expression of a cyanobacterial Fld gene also improved drought and salinity stress tolerance in somatic embryos of Persian walnuts (Sheikh Beig Goharrizi et al., 2016). Transgenic ajowan plants, with an exogenous Fld gene introduced from cyanobacterial, showed greater drought tolerance than wild-type plants (Niazian et al., 2017). Transgenic potato plants with an introduced cyanobacterial Fld gene in their chloroplasts changed their transcriptional and metabolic profile under drought stress conditions and reported increased photosynthetic activity and consequent tuber yield in addition to a decreased ROS accumulation (Karlusich et al., 2020). A synthesis of Fld gene delivery to enhance stress tolerance in different plant species is presented in Table 2.
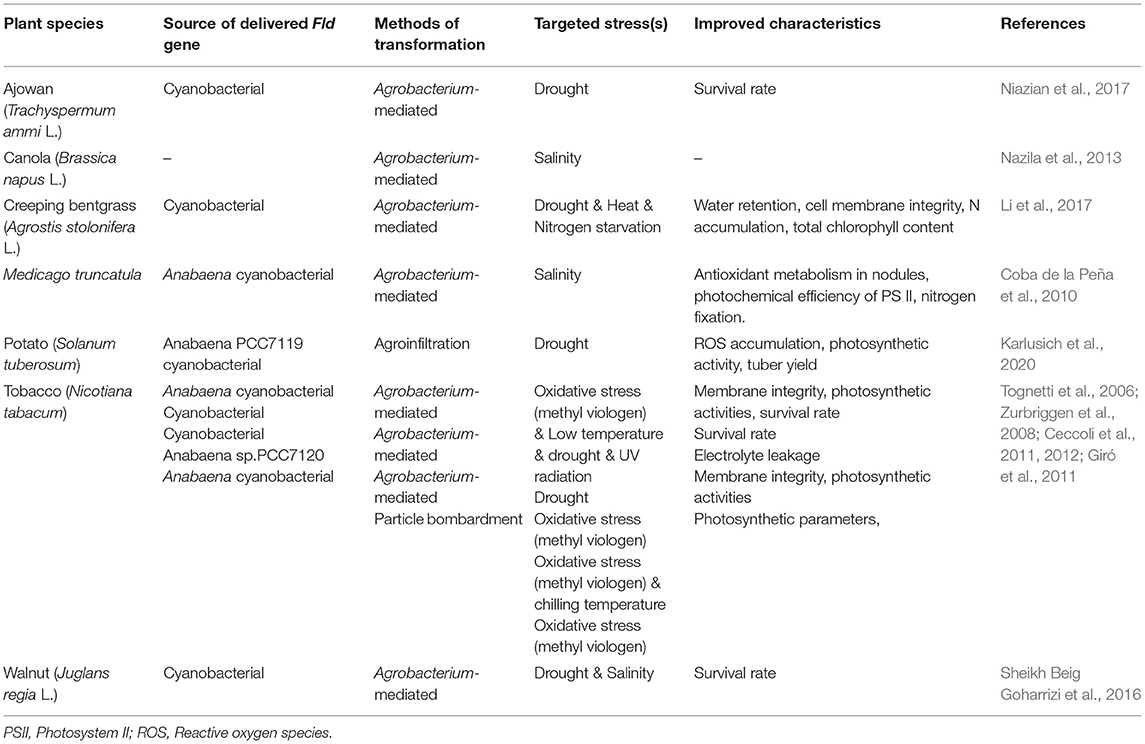
Table 2. Heterologous expression of Fld gene to enhance abiotic stresses in different plant species.
Intermediate overexpression of the Fld gene can also change plant physiological characteristics. Inoculation of alfalfa (Medicago sativa) plants with transformed bacterial cells of Sinorhizobium meliloti with the Anabaena variabili Fld gene led to a modified antioxidant metabolism, delayed nodule senescence, and improved starch accumulation in nodules (Redondo et al., 2009). Alfalfa plants nodulated by the flavodoxin-overexpressing rhizobia showed more tolerance to cadmium than plants nodulated by wild-type bacteria (Shvaleva et al., 2010).
In addition to Fld, other modifications of the acceptor end of PSI can affect plant stress tolerance (Gómez et al., 2019). Plant ferrodoxin-like protein (PFLP) and FNR as well as cyanobacterial flavodiiron (Flv) are applicable in this regard. Overexpression of PFLP-1 and PFLP-2 genes led to enhanced salinity tolerance in transgenic rice plants through higher antioxidant enzyme activities, higher ABA accumulation, up-regulated expression of stress-related genes (OsRBOHa, Cu/Zn SOD, OsAPX, OsNCED2, OsSOS1, OsCIPK24, OsCBL4, and OsNHX2), and lower leaf sodium ion content (Huang et al., 2020). Enhanced heat stress tolerance has been reported in Arabidopsis thaliana with the PFLP transgene (Lin et al., 2015). The chloroplastic overexpression of a pea (Pisum sativum) FNR led to increased oxidative stress tolerance in transgenic tobacco plants (Rodriguez et al., 2007). The introduction of cyanobacterial Flv1/Flv3 into the tobacco chloroplasts triggered a faster recovery of plant photosynthetic performance coupled with efficient electron transport and non-photochemical quenching during dark–light transitions in transgenic plants under light intensities. These results indicate the protective role of Flvs (Gómez et al., 2018). Shahinnia et al. (2020) introduced cyanobacterium Flv1 and Flv3 genes to barley, using the Agrobacterium-mediated gene transformation method. They observed accelerated days to heading, increased biomass, promotion of the number of spikes and grains per plant, and improved grain yield of the transgenic barley plants under drought stress. Using reducing equivalents of the PETC, Flv proteins are able to direct the reduction of O2 to H2O and decrease O2 concentration in chloroplasts (Gómez et al., 2019).
Roles Other Than Enhancing Stress Tolerance for BADH and FLD Transgenes
In addition to stress tolerance, BADH introduction has been applied for changing the fruit size and productivity of plants. Zhang T. et al. (2019) studied the effect of GB on tomato fruit development using codA and BADH transgenic lines and reported that codA and BADH transgenes led to the formation of enlarged flowers and fruits in comparison with wild-type plants. The observed fruit size enlargement was related to the contents of phytohormones, such as auxin, brassinolide, gibberellin, and cytokinin. In addition, the expression level of certain genes related to fruit growth and development was increased in transgenic lines. Transgenic and wild-type plants were mainly different in the expression level of the genes involved in photosynthesis, DNA replication, plant hormone signal transduction, and biosynthesis pathways. These results indicated that GB promoted tomato fruit development through multiple pathways, and, therefore, genetic engineering of GB synthesis can be used as a novel method to increase the productivity of tomatoes and other important plants (Zhang T. et al., 2019). Agrobacterium-mediated delivery of a spinach BADH gene changed the phytochemical profile of the ajowan medicinal plant and led to a significant increase in the thymol content of BADH-transformed plants compared with wild-type plants under drought stress conditions (Niazian et al., 2019). This report demonstrated the effective role of the BADH gene in changing the phytochemical profile of plants and its potential to improve the commercial value of medicinal and aromatic plants. Fld introduction can also change the plant's size and productivity as well as other biochemical processes. Flavodoxin expression increased the nitrogen fixation of the legume Medicago truncatula and therefore can be used to improve legume symbiotic performance under stressful conditions (Coba de la Peña et al., 2010). Transgenic tobacco lines expressing a plastid-targeted cyanobacterial Fld gene showed a decreased accumulation of ROS in their chloroplasts. Fld expression also delayed senescence in aging leaves by improving the maintenance of chlorophyll-protein complexes, photosynthetic electron flow, CO2 assimilation, central metabolic routes, and levels of bioactive cytokinins and auxins (Mayta et al., 2018). Expression of a chloroplast-targeted cyanobacterial Fld led to an increased harvest index in tomatoes by increasing plant density (a higher fruit number per plant in smaller plants) (Mayta et al., 2019). Thus, this report showed that Fld introduction had a great potential to extend flower longevity and shelf life and subsequently increase the commercial value of ornamental plants. These results implied that both BADH and Fld genes transformation had promising potential to improve major economically important traits of targeted plants in addition to enhancing stress tolerance.
Conclusion
A rising worldwide population and food shortages due to the impact of climate change and the adverse effects of several environmental stresses on crop productivity, particularly drought and salinity, remark the importance of the rapid development of stress-tolerant plants, able to maintain sustainable productions in harsh environments. Plants employ different adaptation mechanisms, at morphological physiological, biochemical, and molecular levels, over their evolutionary course in response to challenging environmental situations. By developing transgenic plants, plant breeders are able to efficiently combat abiotic stresses. Biotechnology can enhance the tolerance mechanisms of plants by using the up and down-regulation of genes involved in plant stress responses. Genetic engineering of important compatible solutes such as GB, through overexpressing of key genes involved in their biosynthesis pathway, is a creative biotechnological approach that enhances the stress tolerance of several plant species. BADH is one of the key genes in the biosynthesis pathway of GB, and its introduction led to an increased accumulation of GB and subsequently an improved photosynthetic rate and performance of transgenic plants under different abiotic stresses. The introduction of effective tolerance genes from other types of living organisms is another solution to enhance plant stress tolerance through genetic engineering methods. Cyanobacterial Fld is one of these genes whose delivery led to increased stress tolerance in different plant species. Both BADH and Fld genes are involved in strengthening photosynthetic apparatus under hostile environmental conditions by affecting PSII and PSI, respectively. Recent studies have shown that both BADH and Fld transgenes are involved in productivity and other commercially important traits, such as larger fruit size, delayed senescence in aging leaves, as well as the content of beneficial secondary metabolites. These findings indicate the important roles of BADH and Fld transgenes and their potentials to enhance economically important characteristics of different plant species in stressful environments. The cumulative effect of BADH and Fld transgenes would be much more valuable in this regard. In conclusion, biosafety and biosecurity concerns around genetically modified organisms (GMO), and the biological safety of transgenic plants is pivotal. Therefore, the need to carefully assess the effect of GM plants on the environment, in particular on the potential issues of persistence and invasiveness, the compatibility of GM plants with related plants, gene transfer between GM and non-GM plants and microorganisms, the effects on biogeochemical processes and the effects on human and animal health, should be considered in gene transformation studies. New targeted genome editing methods can solve some public concerns about GM plants such as the integration of DNA constructs in transgenic plants, integration of two or more T-DNA copies into the plant genome, and the presence of selectable marker genes in GM plants.
Data Availability Statement
The raw data supporting the conclusions of this article will be made available by the authors, without undue reservation.
Author Contributions
MN conceived the idea and wrote the whole body of the manuscript. SS-N and MT supervised the project. SM was the advisor of the project. PS reviewed the manuscript. All authors contributed to the article and approved the submitted version.
Conflict of Interest
The authors declare that the research was conducted in the absence of any commercial or financial relationships that could be construed as a potential conflict of interest.
Acknowledgments
The authors are thankful to Piama Svoboda and Rahil Ashtari Mahini for their kind assistance in proofreading the written English of the manuscript.
Abbreviations
ABA, Abscisic acid; APX, Ascorbate peroxidase; BADH, Betaine aldehyde dehydrogenase; CAT, Catalase; CMO, Choline monooxygenase; Fd, Ferredoxin; Fld, Flavodoxin; Flv, Flavodiiron; GB, Glycine betaine; GM, genetically modified; MDA, Malondialdehyde; MS, Murashige and Skoog medium; PETC, Photosynthetic electron transport chain; PFLP, Ferrodoxin-like protein; POX, Peroxidase; PSI, Photosystem I; PSII, Photosystem II; ROS, Reactive oxygen species; SOD, Superoxide dismutase.
References
Ali, A., Ali, Q., Iqbal, M. S., Nasir, I. A., and Wang, X. (2020). Salt tolerance of potato genetically engineered with the Atriplex canescens BADH gene. Biol. Plant 64, 271–279. doi: 10.32615/bp.2019.080
Arif, Y., Singh, P., Siddiqui, H., Bajguz, A., and Hayat, S. (2020). Salinity induced physiological and biochemical changes in plants: an omic approach towards salt stress tolerance. Plant Physiol. Biochem. 156, 64–77. doi: 10.1016/j.plaphy.2020.08.042
Ashraf, M. F. M. R., and Foolad, M. R. (2007). Roles of glycine betaine and proline in improving plant abiotic stress resistance. Environ. Exp. Bot. 59, 206–216. doi: 10.1016/j.envexpbot.2005.12.006
Bhuiyan, T. F., Ahamed, K. U., Nahar, K., Al Mahmud, J., Bhuyan, M. B., Anee, T. I., et al. (2019). Mitigation of PEG-induced drought stress in rapeseed (Brassica rapa L.) by exogenous application of osmolytes. Biocatal. Agric. Biotechnol. 20:101197. doi: 10.1016/j.bcab.2019.101197
Blanco, N. E., Ceccoli, R. D., Segretin, M. E., Poli, H. O., Voss, I., Melzer, M., et al. (2011). Cyanobacterial flavodoxin complements ferredoxin deficiency in knocked-down transgenic tobacco plants. Plant J. 65, 922–935. doi: 10.1111/j.1365-313X.2010.04479.x
Castiglioni, P., Bell, E., Lund, A., Rosenberg, A. F., Galligan, M., Hinchey, B. S., et al. (2018). Identification of GB1, a gene whose constitutive overexpression increases glycinebetaine content in maize and soybean. Plant Direct 2:e00040. doi: 10.1002/pld3.40
Ceccoli, R. D., Blanco, N. E., Medina, M., and Carrillo, N. (2011). Stress response of transgenic tobacco plants expressing a cyanobacterial ferredoxin in chloroplasts. Plant Mol. Biol. 76, 535–544. doi: 10.1007/s11103-011-9786-9
Ceccoli, R. D., Blanco, N. E., Segretin, M. E., Melzer, M., Hanke, G. T., Scheibe, R., et al. (2012). Flavodoxin displays dose-dependent effects on photosynthesis and stress tolerance when expressed in transgenic tobacco plants. Planta 236, 1447–1458. doi: 10.1007/s00425-012-1695-x
Chen, T. H., and Murata, N. (2002). Enhancement of tolerance of abiotic stress by metabolic engineering of betaines and other compatible solutes. Curr. Opin. Plant Biol. 5, 250–257. doi: 10.1016/S1369-5266(02)00255-8
Chen, T. H., and Murata, N. (2008). Glycinebetaine: an effective protectant against abiotic stress in plants. Trends Plant Sci. 13, 499–505. doi: 10.1016/j.tplants.2008.06.007
Chen, T. H., and Murata, N. (2011). Glycinebetaine protects plants against abiotic stress: mechanisms and biotechnological applications. Plant Cell Environ. 34, 1–20. doi: 10.1111/j.1365-3040.2010.02232.x
Chen, Y. E., Mao, H. T., Wu, N., Mohi Ud Din, A., Khan, A., Zhang, H. Y., et al. (2020). Salicylic acid protects photosystem II by alleviating photoinhibition in Arabidopsis thaliana under high light. Int. J. Mol. Sci. 21:1229. doi: 10.3390/ijms21041229
Coba de la Peña, T., Redondo, F. J., Manrique, E., Lucas, M. M., and Pueyo, J. J. (2010). Nitrogen fixation persists under conditions of salt stress in transgenic Medicago truncatula plants expressing a cyanobacterial flavodoxin. Plant Biotechnol. J. 8, 954–965. doi: 10.1111/j.1467-7652.2010.00519.x
El-Esawi, M. A., and Alayafi, A. A. (2019a). Overexpression of rice Rab7 gene improves drought and heat tolerance and increases grain yield in rice (Oryza sativa L.). Genes 10:56. doi: 10.3390/genes10010056
El-Esawi, M. A., and Alayafi, A. A. (2019b). Overexpression of StDREB2 transcription factor enhances drought stress tolerance in cotton (Gossypium barbadense L.). Genes 10:142. doi: 10.3390/genes10020142
Gharechahi, J., Hajirezaei, M. R., and Salekdeh, G. H. (2015). Comparative proteomic analysis of tobacco expressing cyanobacterial flavodoxin and its wild type under drought stress. J. Plant Physiol. 175, 48–58. doi: 10.1016/j.jplph.2014.11.001
Giró, M., Ceccoli, R. D., Poli, H. O., Carrillo, N., and Lodeyro, A. F. (2011). An in vivo system involving co-expression of cyanobacterial flavodoxin and ferredoxin–NADP+ reductase confers increased tolerance to oxidative stress in plants. FEBS Open Bio. 1, 7–13. doi: 10.1016/j.fob.2011.10.004
Golestan Hashemi, F. S., Ismail, M. R., Rafii, M. Y., Aslani, F., Miah, G., and Muharam, F. M. (2018). Critical multifunctional role of the betaine aldehyde dehydrogenase gene in plants. Biotechnol. Biotechnol. Equip. 32, 815–829. doi: 10.1080/13102818.2018.1478748
Gómez, R., Carrillo, N., Morelli, M. P., Tula, S., Shahinnia, F., Hajirezaei, M. R., et al. (2018). Faster photosynthetic induction in tobacco by expressing cyanobacterial flavodiiron proteins in chloroplasts. Photosyn. Res. 136, 129–138. doi: 10.1007/s11120-017-0449-9
Gómez, R., Vicino, P., Carrillo, N., and Lodeyro, A. F. (2019). Manipulation of oxidative stress responses as a strategy to generate stress-tolerant crops. from damage to signaling to tolerance. Critic. Rev. Biotechnol. 39, 693–708. doi: 10.1080/07388551.2019.1597829
Guo, W., Li, G., Wang, N., Yang, C., Zhao, Y., Peng, H., et al. (2020). A Na+/H+ antiporter, K2-NhaD, improves salt and drought tolerance in cotton (Gossypium hirsutum L.). Plant Mol. Biol. 102, 553–567. doi: 10.1007/s11103-020-00969-1
Hasan, H., Gul, A., Amir, R., Ali, M., Kubra, G., Yousaf, S., et al. (2020). “Role of osmoprotectants and drought tolerance in wheat,” in Climate Change and Food Security With Emphasis on Wheat, eds M. Ozturk, and A. Gul (Cambridge: Academic Press), 207–216. doi: 10.1016/B978-0-12-819527-7.00013-3
Huang, H., Ullah, F., Zhou, D. X., Yi, M., and Zhao, Y. (2019). Mechanisms of ROS regulation of plant development and stress responses. Front. Plant Sci. 10:800. doi: 10.3389/fpls.2019.00800
Huang, H. E., Ho, M. H., Chang, H., Chao, H. Y., and Ger, M. J. (2020). Overexpression of plant ferredoxin-like protein promotes salinity tolerance in rice (Oryza sativa). Plant Physiol. Biochem. 155, 136–146. doi: 10.1016/j.plaphy.2020.07.025
Isayenkov, S. V. (2019). Genetic sources for the development of salt tolerance in crops. Plant Growth Regul. 89, 1–17. doi: 10.1007/s10725-019-00519-w
Isayenkov, S. V., and Maathuis, F. J. (2019). Plant salinity stress: many unanswered questions remain. Front. Plant Sci. 10:80. doi: 10.3389/fpls.2019.00080
Islam, M., Kim, J. W., Begum, M., Sohel, M., Taher, A., and Lim, Y. S. (2020). Physiological and biochemical changes in sugar beet seedlings to confer stress adaptability under drought condition. Plants 9:1511. doi: 10.3390/plants9111511
Jiang, Y., Zhu, S., Yuan, J., Chen, G., and Lu, G. (2016). A betaine aldehyde dehydrogenase gene in quinoa (Chenopodium quinoa): structure, phylogeny, and expression pattern. Genes Genom. 38, 1013–1020. doi: 10.1007/s13258-016-0445-z
Jin, R., Kim, B. H., Ji, C. Y., Kim, H. S., Li, H. M., and Kwak, S. S. (2017). Overexpressing IbCBF3 increases low temperature and drought stress tolerance in transgenic sweetpotato. Plant Physiol. Biochem. 118, 45–54. doi: 10.1016/j.plaphy.2017.06.002
Karlusich, J. J. P., Arce, R. C., Shahinnia, F., Sonnewald, S., Sonnewald, U., Zurbriggen, M. D., et al. (2020). Transcriptional and metabolic profiling of potato plants expressing a plastid-targeted electron shuttle reveal modulation of genes associated to drought tolerance by chloroplast redox poise. Int. J. Mol. Sci. 21:7199. doi: 10.3390/ijms21197199
Latif, S., Shah, T., Nawaz, R., Munsif, F., Ali, M., ur Rehman, M., et al. (2020). Programmed cell death and drought stress signaling, in Salt and Drought Stress Tolerance in Plants. Signaling and Communication in Plants, eds M. Hasanuzzaman and M. Tanveer (Cham: Springer), 211–229. doi: 10.1007/978-3-030-40277-8_8
Li, M., Li, Z., Li, S., Guo, S., Meng, Q., Li, G., et al. (2014). Genetic engineering of glycine betaine biosynthesis reduces heat-enhanced photoinhibition by enhancing antioxidative defense and alleviating lipid peroxidation in tomato. Plant Mol. Biol. Report. 32, 42–51. doi: 10.1007/s11105-013-0594-z
Li, P., Cai, J., Luo, X., Chang, T., Li, J., Zhao, Y., et al. (2019). Transformation of wheat Triticum aestivum with the HvBADH1 transgene from hulless barley improves salinity-stress tolerance. Acta Physiol. Plant. 41:155. doi: 10.1007/s11738-019-2940-8
Li, X., Lai, P., Li, P., and Zhao, Y. (2016). Transformation of Cichorium intybus with the HvBADH1 gene enhanced the salinity tolerance of the transformants. South Afr. J. Bot. 102, 110–119. doi: 10.1016/j.sajb.2015.07.009
Li, Z., Yuan, S., Jia, H., Gao, F., Zhou, M., Yuan, N., et al. (2017). Ectopic expression of a cyanobacterial flavodoxin in creeping bentgrass impacts plant development and confers broad abiotic stress tolerance. Plant Biotechnol. J. 15, 433–446. doi: 10.1111/pbi.12638
Lin, Y. H., Huang, L. F., Hase, T., Huang, H. E., and Feng, T. Y. (2015). Expression of plant ferredoxin-like protein (PFLP) enhances tolerance to heat stress in Arabidopsis thaliana. New Biotechnol. 32, 235–242. doi: 10.1016/j.nbt.2014.12.001
Liu, Y., Song, Y., Zeng, S., Patra, B., Yuan, L., and Wang, Y. (2018). Isolation and characterization of a salt stress-responsive betaine aldehyde dehydrogenase in Lycium ruthenicum Murr. Physiol. Plant 163, 73–87. doi: 10.1111/ppl.12669
Lodeyro, A. F., Ceccoli, R. D., Karlusich, J. J. P., and Carrillo, N. (2012). The importance of flavodoxin for environmental stress tolerance in photosynthetic microorganisms and transgenic plants. mechanism, evolution and biotechnological potential. FEBS Lett. 586, 2917–2924. doi: 10.1016/j.febslet.2012.07.026
Ma, Y., Zhou, T., Hong, Y., Fan, Z., and Li, H. (2008). Decreased level of ferredoxin I in Tobacco mosaic virus-infected tobacco is associated with development of the mosaic symptom. Physiol. Mol. Plant Pathol. 72, 39–45. doi: 10.1016/j.pmpp.2008.05.004
Mayta, M. L., Arce, R. C., Zurbriggen, M., Valle, E. M., Hajirezaei, M. R., Zanor, M. I., et al. (2019). Expression of a chloroplast-targeted cyanobacterial flavodoxin in tomato plants increases harvest index by altering plant size and productivity. Front. Plant Sci. 10:1432. doi: 10.3389/fpls.2019.01432
Mayta, M. L., Lodeyro, A. F., Guiamet, J. J., Tognetti, V. B., Melzer, M., Hajirezaei, M. R., et al. (2018). Expression of a plastid-targeted flavodoxin decreases chloroplast reactive oxygen species accumulation and delays senescence in aging tobacco leaves. Front. Plant Sci. 9:1039. doi: 10.3389/fpls.2018.01039
Mickelbart, M. V., and Boine, B. (2020). Glycinebetaine enhances osmotic adjustment of ryegrass under cold temperatures. Agronomy 10:210. doi: 10.3390/agronomy10020210
Nawaz, M., and Wang, Z. (2020). Abscisic acid and glycine betaine mediated tolerance mechanisms under drought stress and recovery in Axonopus compressus: a new insight. Sci. Rep. 10:6942. doi: 10.1038/s41598-020-63447-0
Nazila, N., Amin, B., Masoud, T., and Shahram, P. (2013). Agrobacterium mediated transformation of Fld and Gus genes into canola for Salinity stress. J. Stress Physiol. Biochem. 9, 74–85.
Niazian, M., Sadat-Noori, S. A., Tohidfar, M., Galuszka, P., and Mortazavian, S. M. M. (2019). Agrobacterium-mediated genetic transformation of ajowan (Trachyspermum ammi (L.) Sprague): an important industrial medicinal plant. Ind. Crops Prod. 132, 29–40. doi: 10.1016/j.indcrop.2019.02.005
Niazian, M., and Shariatpanahi, M. E. (2020). In vitro-based doubled haploid production: recent improvements. Euphytica 216, 1–21. doi: 10.1007/s10681-020-02609-7
Niazian, M., Tohidfar, M., and Galuszka, P. (2017). Optimization of Agrobacterium-mediated gene transformation in Ajowan medicinal plant (Trachyspermum ammi L.) using fld gene. J. Cell. Mol. Res. 30, 313–327.
Ozturk, M., Turkyilmaz Unal, B., García-Caparrós, P., Khursheed, A., Gul, A., and Hasanuzzaman, M. (2020). Osmoregulation and its actions during the drought stress in plants. Physiol. Plant. doi: 10.1111/ppl.13297
Pan, T., Liu, M., Kreslavski, V. D., Zharmukhamedov, S. K., Nie, C., Yu, M., et al. (2020). Non-stomatal limitation of photosynthesis by soil salinity. Crit. Rev. Environ. Sci. Technol. 1–35. doi: 10.1080/10643389.2020.1735231
Paschalidis, K., Tsaniklidis, G., Wang, B. Q., Delis, C., Trantas, E., Loulakakis, K., et al. (2019). The interplay among polyamines and nitrogen in plant stress responses. Plants 8:315. doi: 10.3390/plants8090315
Pierella Karlusich, J. J., Lodeyro, A. F., and Carrillo, N. (2014). The long goodbye: the rise and fall of flavodoxin during plant evolution. J. Exp. Bot. 65, 5161–5178. doi: 10.1093/jxb/eru273
Qin, D., Zhao, C. L., Liu, X. Y., and Wang, P. W. (2017). Transgenic soybeans expressing betaine aldehyde dehydrogenase from Atriplex canescens show increased drought tolerance. Plant Breed. 136, 699–709. doi: 10.1111/pbr.12518
Rady, M. O., Semida, W. M., Abd El-Mageed, T. A., Hemida, K. A., and Rady, M. M. (2018). Up-regulation of antioxidative defense systems by glycine betaine foliar application in onion plants confer tolerance to salinity stress. Sci. Hortic. 240, 614–622. doi: 10.1016/j.scienta.2018.06.069
Redondo, F. J., De La Peña, T. C., Morcillo, C. N., Lucas, M. M., and Pueyo, J. J. (2009). Overexpression of flavodoxin in bacteroids induces changes in antioxidant metabolism leading to delayed senescence and starch accumulation in alfalfa root nodules. Plant Physiol. 149, 1166–1178. doi: 10.1104/pp.108.129601
Rezaei Qusheh Bolagh, F., Solouki, A., Tohidfar, M., Zare Mehrjerdi, M., Izadi-Darbandi, A., and Vahdati, K. (2020). Agrobacterium-mediated transformation of Persian walnut using BADH gene for salt and drought tolerance. J. Hortic. Sci. Biotechnol. 96, 162–171. doi: 10.1080/14620316.2020.1812446
Rodriguez, R. E., Lodeyro, A., Poli, H. O., Zurbriggen, M., Peisker, M., Palatnik, J. F., et al. (2007). Transgenic tobacco plants overexpressing chloroplastic ferredoxin-NADP (H) reductase display normal rates of photosynthesis and increased tolerance to oxidative stress. Plant Physiol. 143, 639–649. doi: 10.1104/pp.106.090449
Semida, W. M., Abdelkhalik, A., Rady, M. O., Marey, R. A., and Abd El-Mageed, T. A. (2020). Exogenously applied proline enhances growth and productivity of drought stressed onion by improving photosynthetic efficiency, water use efficiency and up-regulating osmoprotectants. Sci. Hortic. 272:109580. doi: 10.1016/j.scienta.2020.109580
Shahinnia, F., Tula, S., Hensel, G., Reiahisamani, N., Nasr, N., Kumlehn, J., et al. (2020). Integrating cyanobacterial flavodiiron proteins within the chloroplast photosynthetic electron transport chain maintains carbohydrate turnover and enhances drought stress tolerance in barley. bioRxiv. doi: 10.1101/2020.09.29.318394
Shailani, A., Joshi, R., Singla-Pareek, S. L., and Pareek, A. (2020). Stacking for future: pyramiding genes to improve drought and salinity tolerance in rice. Physiol. Plant. 1–25. doi: 10.1111/ppl.13270
Sheikh Beig Goharrizi, M. A., Dejahang, A., Tohidfar, M., Izadi Darbandi, A., Carrillo, N. J., Hajirezaei, M. R., et al. (2016). Agrobacterium mediated transformation of somatic embryos of Persian walnut using fld gene for osmotic stress tolerance. J. Agric. Sci. Technol. 18, 423–435.
Shvaleva, A., de la Peña, T. C., Rincón, A., Morcillo, C. N., de la Torre, V. S. G., Lucas, M. M., et al. (2010). Flavodoxin overexpression reduces cadmium-induced damage in alfalfa root nodules. Plant Soil 326, 109–121. doi: 10.1007/s11104-009-9985-1
Smith-Moore, C. M., and Grunden, A. M. (2018). Bacteria and archaea as the sources of traits for enhanced plant phenotypes. Biotechnol. Adv. 36, 1900–1916. doi: 10.1016/j.biotechadv.2018.07.007
Song, J., Zhang, R., Yue, D., Chen, X., Guo, Z., Cheng, C., et al. (2018). Co-expression of ApGSMT2g and ApDMT2g in cotton enhances salt tolerance and increases seed cotton yield in saline fields. Plant Sci. 274, 369–382. doi: 10.1016/j.plantsci.2018.06.007
Sun, Y., Liu, X., Fu, L., Qin, P., Li, T., Ma, X., et al. (2019). Overexpression of TaBADH increases salt tolerance in Arabidopsis. Canad. J. Plant Sci. 99, 546–555. doi: 10.1139/cjps-2018-0190
Surabhi, G. K., and Rout, A. (2020). “Glycine betaine and crop abiotic stress tolerance: an update,” in Protective Chemical Agents in the Amelioration of Plant Abiotic Stress: Biochemical and Molecular Perspectives, eds A. Roychoudhury, and D. K. Tripathi (John Wiley & Sons Ltd), 24–52. doi: 10.1002/9781119552154.ch2
Takahashi, F., and Shinozaki, K. (2019). Long-distance signaling in plant stress response. Curr. Opin. Plant Biol. 47, 106–111. doi: 10.1016/j.pbi.2018.10.006
Tian, F., Wang, W., Liang, C., Wang, X., Wang, G., and Wang, W. (2017). Overaccumulation of glycine betaine makes the function of the thylakoid membrane better in wheat under salt stress. Crop J. 5, 73–82. doi: 10.1016/j.cj.2016.05.008
Tisarum, R., Theerawitaya, C., Samphumphuang, T., Singh, H. P., and Cha-um, S. (2020). Foliar application of glycinebetaine regulates soluble sugars and modulates physiological adaptations in sweet potato (Ipomoea batatas) under water deficit. Protoplasma 257, 197–211. doi: 10.1007/s00709-019-01429-4
Tognetti, V. B., Palatnik, J. F., Fillat, M. F., Melzer, M., Hajirezaei, M. R., Valle, E. M., et al. (2006). Functional replacement of ferredoxin by a cyanobacterial flavodoxin in tobacco confers broad-range stress tolerance. Plant Cell. 18, 2035–2050. doi: 10.1105/tpc.106.042424
Wai, A. H., Naing, A. H., Lee, D. J., Kim, C. K., and Chung, M. Y. (2020). Molecular genetic approaches for enhancing stress tolerance and fruit quality of tomato. Plant Biotechnol. Rep. 14, 515–537. doi: 10.1007/s11816-020-00638-1
Wu, W., Su, Q., Xia, X. Y., Wang, Y., Luan, Y. S., and An, L. J. (2008). The Suaeda liaotungensis kitag betaine aldehyde dehydrogenase gene improves salt tolerance of transgenic maize mediated with minimum linear length of DNA fragment. Euphytica 159, 17–25. doi: 10.1007/s10681-007-9451-1
Xu, Z., Sun, M., Jiang, X., Sun, H., Dang, X., Cong, H., et al. (2018). Glycinebetaine biosynthesis in response to osmotic stress depends on jasmonate signaling in watermelon suspension cells. Front. Plant Sci. 9:1469. doi: 10.3389/fpls.2018.01469
Yamada, N., Takahashi, H., Kitou, K., Sahashi, K., Tamagake, H., Tanaka, Y., et al. (2015). Suppressed expression of choline monooxygenase in sugar beet on the accumulation of glycine betaine. Plant Physiol. Biochem. 96, 217–221. doi: 10.1016/j.plaphy.2015.06.014
Yang, X., Wen, X., Gong, H., Lu, Q., Yang, Z., Tang, Y., et al. (2007). Genetic engineering of the biosynthesis of glycinebetaine enhances thermotolerance of photosystem II in tobacco plants. Planta 225, 719–733. doi: 10.1007/s00425-006-0380-3
You, L., Song, Q., Wu, Y., Li, S., Jiang, C., Chang, L., et al. (2019). Accumulation of glycine betaine in transplastomic potato plants expressing choline oxidase confers improved drought tolerance. Planta 249, 1963–1975. doi: 10.1007/s00425-019-03132-3
Youssef, S. M., Abd Elhady, S. A., Aref, R. M., and Riad, G. S. (2018). Salicylic acid attenuates the adverse effects of salinity on growth and yield and enhances peroxidase isozymes expression more competently than proline and glycine betaine in cucumber plants. Gesunde Pflanzen 70, 75–90. doi: 10.1007/s10343-017-0413-9
Yu, H. Q., Zhou, X. Y., Wang, Y. G., Zhou, S. F., Fu, F. L., and Li, W. C. (2017). A betaine aldehyde dehydrogenase gene from Ammopiptanthus nanus enhances tolerance of Arabidopsis to high salt and drought stresses. Plant Growth Regul. 83, 265–276. doi: 10.1007/s10725-016-0245-0
Zhang, T., Li, Z., Li, D., Li, C., Wei, D., Li, S., et al. (2020). Comparative effects of glycinebetaine on the thermotolerance in codA-and BADH-transgenic tomato plants under high temperature stress. Plant Cell Rep. 39, 1525–1538. doi: 10.1007/s00299-020-02581-5
Zhang, T., Liang, J., Wang, M., Li, D., Liu, Y., Chen, T. H., et al. (2019). Genetic engineering of the biosynthesis of glycinebetaine enhances the fruit development and size of tomato. Plant Sci. 280, 355–366. doi: 10.1016/j.plantsci.2018.12.023
Zhang, X., Wei, X., Wang, M., Zhu, X., Zhao, Y., Wei, F., et al. (2019). Overexpression of NtabDOG1L promotes plant growth and enhances drought tolerance in Nicotiana tabacum. Plant Sci. 287:110186. doi: 10.1016/j.plantsci.2019.110186
Zhao, H., Zhang, Q., Zhang, M., Jin, Y. K., Jiang, Z. Z., Jiang, N., et al. (2020). Drought tolerance in high-generation transgenic maize inbred lines overexpressing the betaine aldehyde dehydrogenase gene. Cereal Res. Commun. 1–10. doi: 10.1007/s42976-020-00093-2
Zhou, M., Thompson, W. A., and Tang, W. (2020a). The Arabidopsis AtAAE13.1 gene enhances salt stress tolerance in angiosperms and gymnosperm plant cells. In Vitro Cell. Dev. Biol. Plant 56, 750–764. doi: 10.1007/s11627-020-10083-y
Zhou, X., Dong, Y., Zhang, Q., Xiao, D., Yang, M., and Wang, J. (2020b). Expression of multiple exogenous insect resistance and salt tolerance genes in Populus nigra L. Front. Plant Sci. 11:1123. doi: 10.3389/fpls.2020.01123
Zurbriggen, M. D., Hajirezaei, M. R., and Carrillo, N. (2010). Engineering the future. development of transgenic plants with enhanced tolerance to adverse environments. Biotechnol. Genetic Eng. Rev. 27, 33–56. doi: 10.1080/02648725.2010.10648144
Zurbriggen, M. D., Tognetti, V. B., and Carrillo, N. (2007). Stress-inducible flavodoxin from photosynthetic microorganisms. the mystery of flavodoxin loss from the plant genome. IUBMB Life 59, 355–360. doi: 10.1080/15216540701258744
Keywords: compatible solutes, flavoproteins, glycine betaine, osmotic adjustment, photosynthetic electron transport chain, stress
Citation: Niazian M, Sadat-Noori SA, Tohidfar M, Mortazavian SMM and Sabbatini P (2021) Betaine Aldehyde Dehydrogenase (BADH) vs. Flavodoxin (Fld): Two Important Genes for Enhancing Plants Stress Tolerance and Productivity. Front. Plant Sci. 12:650215. doi: 10.3389/fpls.2021.650215
Received: 06 January 2021; Accepted: 03 March 2021;
Published: 01 April 2021.
Edited by:
Sergio J. Ochatt, INRA UMR1347 Agroécologie, FranceReviewed by:
Néstor Carrillo, Consejo Nacional de Investigaciones Científicas y Técnicas (CONICET), ArgentinaJesus Alfredo Rosas Rodríguez, University of Sonora, Mexico
Elisa M. Valenzuela-Soto, Consejo Nacional de Ciencia y Tecnología (CONACYT), Mexico
Copyright © 2021 Niazian, Sadat-Noori, Tohidfar, Mortazavian and Sabbatini. This is an open-access article distributed under the terms of the Creative Commons Attribution License (CC BY). The use, distribution or reproduction in other forums is permitted, provided the original author(s) and the copyright owner(s) are credited and that the original publication in this journal is cited, in accordance with accepted academic practice. No use, distribution or reproduction is permitted which does not comply with these terms.
*Correspondence: Mohsen Niazian, mniazian@ut.ac.ir; m.niazian@areeo.ac.ir; orcid.org/0000-0002-7032-4938