- School of Pharmacy, Zhejiang Chinese Medical University, Hangzhou, China
Plants benefit extensively from endophytic bacteria, which live in host plant tissues exerting no harmful effects. Bacterial endophytes promote the growth of host plants and enhance their resistance toward various pathogens and environmental stresses. They can also regulate the synthesis of secondary metabolites with significant medicinal properties and produce various biological effects. This review summarizes recent studies on the relationships between bacterial endophytes and medicinal plants. Endophytic bacteria have numerous applications in agriculture, medicine, and other industries: improving plant growth, promoting resistance toward both biotic and abiotic stresses, and producing metabolites with medicinal potential. Their distribution and population structure are affected by their host plant’s genetic characteristics and health and by the ecology of the surrounding environment. Understanding bacterial endophytes can help us use them more effectively and apply them to medicinal plants to improve yield and quality.
Introduction
The quality and yield of medicinal plants are significantly influenced by environmental factors, such as temperature, illumination, moisture, soil conditions, and the presence and identity of soil fauna (Namdar et al., 2019). It is increasingly recognized that medicinal plants can also be strongly influenced by their relationships with specific bacterial endophytes (Ek-Ramos et al., 2019). Long-term, symbiotic relationships between host plants and endophytes can promote the growth of plants and be especially useful in agricultural practice (Compant et al., 2010).
Bacterial endophytes are present in the flowers, leaves, roots, seeds, and stems of plants (Qin et al., 2009; Compant et al., 2011; Elmagzob et al., 2019). Bacterial colonization occurs at particular stages of plant development, and even at the seed stage, there may already be a stable endophytic bacterial community (Mocali et al., 2003). These endophytes use the plant’s internal environment (i.e., the endosphere) as a unique niche to protect themselves from drastic altered external environments (Senthilkumar et al., 2011). The evolution of these highly specialized symbioses requires tight coordination of physiology, structure, and life cycles between the partner organisms (Saikkonen et al., 2004; Reinhold-Hurek and Hurek, 2011), and the resulting partnership benefits both species (Aravind et al., 2010). Such mutualisms serve essential functions in terrestrial ecosystems: host plants house and protect the endophytes, which in turn promote the growth of plants via nitrogen fixation, phosphorus enrichment, and the synthesis of phytohormones (Qin et al., 2015; Borah et al., 2019). Moreover, pathogenic microorganisms and endophytic bacteria occupy the same niche within plants, and inoculation with endophytic bacteria is a suitable method to biologically control pathogens (Senthilkumar et al., 2011). Medicinal plants-isolated bacterial endophytes can produce bioactive metabolites and to significantly induce the secondary metabolite production by their host plants. The host-endophyte relationship can be regarded as a flexible, dynamic interaction, in which endophytic bacteria alter their gene expression or produce different metabolites based on small changes in host plant growth and vice versa (Ek-Ramos et al., 2019). Despite their importance to the plant micro-ecosystem, bacterial endophytes’ relationship to their host plants remains poorly understood (Tiwari et al., 2013).
The community of bacterial endophytes is influenced by both biotic and abiotic factors, which shape their species composition, community structure, diversity, and functions (Walitang et al., 2018). Environmental factors not only affect the distribution of a medicinal plant, but also determine the species of bacterial endophyte which can colonize host plant throughout its life cycle (Deng et al., 2011). Endophyte diversity is also influenced by host plant characteristics, including genotype (Walitang et al., 2018), tissue (Dai et al., 2014), growth stage (age) (Vendan et al., 2010), and health status (Bogas et al., 2015). The non-random distribution of endophytic bacterial species thus provides clues as to their biology and ecology. However, there is limited information on the plant and environmental factors that shape endophytic bacterial community structure and how endophytes regulate their hosts by synthesizing primary and secondary metabolites.
Here, we summarize the external factors that affect endophytic bacteria, and we survey the potential uses of them in growth promotion, pathogen resistance, and secondary metabolism of medicinal plants. Understanding and using these symbiotic relationships can enable us to more effectively cultivate valuable plants for human use and improve the quality and yield of medicinal materials.
Factors That Affect Endophytic Bacteria: Environment and Host Plant
Environmental Effects on Community Structure of Endophytic Bacteria
The community structure of endophytic bacteria is affected by multiple external factors, including season, altitude, latitude, longitude, and soil conditions (Chiellini et al., 2014; Yang et al., 2017; Table 1). For example, Actinobacteria and Proteobacteria were the most abundant endophytic bacteria of mulberry (Morus L.) in the spring, whereas only Proteobacteria were found in the fall. The spring endophytic bacteria were also characterized by greater diversity and a larger number of species (Shannon, 14.00; Simpson, 0.30; Chao, 1018) compared with autumn endophytes (Shannon, 6.62; Simpson, 1.26; Chao, 654) (Ou et al., 2019). Another study revealed that the Pyrus ussuriensi community structure substantially correlated to the carbon, nitrogen, pH, and temperature of soil. In particular, the richness and diversity of root endophytic bacteria increased with increased nitrogen content (Yarte et al., 2020). Xu et al. (2014) reported that soil water content and annual precipitation were strongly correlated with the endophytic bacterial RFLP (restriction fragment length polymorphism) type variation in Caragana jubata and Oxytropis ochrocephala, followed by latitude, longitude, soil nitrogen content, and soil potassium content (Xu et al., 2014). Bacterial endophyte community structure changes with changing environmental conditions and the maintenance of a shifting and diverse endophytic community may form part of the physiological strategy by which plants adapt to their environment.
Host Plant Effects on Endophytic Bacteria
The relationship between host plants and specific endophytic bacterial isolates may exhibit characteristics of either parasitism or mutualism, depending on host genotype, tissue, and health status (Cope-Selby et al., 2017), and multiple studies showed that endophytic bacterial communities are considerably affected by their host plants. A study in the Patagonian ecosystem showed that bacterial abundance and diversity were higher on Hieracium pilosella than on Gaultheria mucronata (Zhang et al., 2019), a result consistent with the assumption that the diverse physiological structures, metabolites, and growth habits of different plants affect their ability to recruit various endophytic bacteria (Kawaguchi and Minamisawa, 2010; Campisano et al., 2014). Host plant health status also influences endophyte colonization: the bacterial endophytic community of Paullinia cupana with asymptomatic anthracnose comprised mainly Firmicutes, whereas that of plants with symptomatic anthracnose comprised mainly Acidobacteria (Bogas et al., 2015). Such results may reflect pathogen-mediated surface damage to the host, promoting the establishment of some endophytes and disrupting the original stable microbial ecological environment (Hallmann et al., 1998). In one study, PCR-based molecular techniques were employed to investigate the cultivable bacteria isolated from the leaf, root, and stem compartments of Echinacea angustifolia (DC.) Hell and Echinacea purpurea (L.) Moench, which demonstrated that these two medicinal plants and the respective compartments possessed different types of bacterial communities, suggesting the strong selective pressure and the low-degree strain sharing in the plant tissues (Chiellini et al., 2014). These differences might be attributed to differential nutritional and environmental conditions to which the aerial parts and the roots of the plants were exposed, or due to the phytochemical and anatomical features, which in turn established particular ecological niches for the endophytes. Endophytic bacteria are possibly selected based on their strategies for adaptation and the tolerance under differential conditions in a variety of plant compartments. The differences in the medicinal properties can be explained by the presence of distinct bacterial communities in differential plant species and among the compartments of a single plant species. In general, endophytic bacteria can adjust their structure and diversity in response to different plant genotypes, organs, health statuses, and growth stages in order to obtain a consistent supply of nutrients. Moreover, in an olive system, it was found that belowground communities of endophytic bacteria were mainly determined by the cultivar genotype grown under same agronomic, environmental, and pedological conditions, indicating that the plant genotype serves as a main factor to shape the belowground bacterial communities of olive (Fernandez-Gonzalez et al., 2019).
Beneficial Effects of Endophytic Bacteria on Host Plants
Collected Medicinal Plants and the Associated Endophytic Bacteria
Our survey and analysis have documented the presence of mutually beneficial relationships between bacterial endophytes and 86 medicinal plants from 40 families (Figure 1). Surveyed plants included Amaranthaceae (2 taxa), Amaryllidaceae (1 taxon), Anacardiaceae (1 taxon), Apiaceae (4 taxa), Apocynaceae (1 taxon), Araceae (1 taxon), Araliaceae (2 taxa), Asteraceae (11 taxa), Berberidaceae (1 taxon), Brassicaceae (2 taxa), Cactaceae (1 taxon), Caprifoliaceae (1 taxon), Celastraceae (2 taxa), Chenopodiaceae (3 taxa), Dryopteridaceae (1 taxon), Euphorbiaceae (4 taxa), Fabaceae (11 taxa), Ginkgoaceae (1 taxon), Lamiaceae (7 taxa), Lauraceae (2 taxa), Liliaceae (2 taxa), Meliaceae (1 taxon), Moraceae (2 taxa), Myrtaceae (1 taxon), Orchidaceae (1 taxon), Plantaginaceae (1 taxon), Poaceae (4 taxa), Polygonaceae (1 taxon), Pteridaceae (1 taxon), Rosaceae (1 taxon), Rubiaceae (2 taxa), Sapindaceae (2 taxa), Sarraceniaceae (1 taxon), Saururaceae (1 taxon), Theaceae (2 taxa), Thymelaeaceae (1 taxon), Ulmaceae (1 taxon), Vitaceae (1 taxon), Winteraceae (1 taxon), and Zingiberaceae (2 taxa). As shown in Figure 2, the medicinal plants we surveyed were mainly distributed in Asia and Europe; there were a few studies on medicinal plant endophytes from North America and Australia, but none from extreme regions. Future research should focus on endophytic bacteria and medicinal plants in areas where there has been relatively less research. A total of 11 orders and 88 genera of endophytic bacteria had documented associations with medicinal plants in the literature. The most common orders were Bacillales, Enterobacterales, and Pseudomonadales, which accounted for 72.62%. The most common genera were Bacillus, Pantoea and Pseudomonas, which accounted for 58.92%. Streptomyces are widely reported as promoting the growth and development of plants, and Bacillus, Pseudomonas and Paenibacillus can influence the growth, stress resistance and metabolism of medicinal plants (Gao et al., 2015; Zhao et al., 2015; Qi et al., 2021). Based on the information collected above, we suggest that future studies prioritize exploring these beneficial endophytic bacteria. However, such endophytic bacteria mentioned in the literature have been isolated by culture techniques. Our present understanding of medicinal plant endophytes derives almost entirely from culture-based diversity analyses (Liu et al., 2017), though most of the environmental bacteria are unculturable. This constraint limits our understanding of medicinal plant endophytic bacteria and the influence of plant hosts on the structure of bacterial community: the impact of unculturable bacteria on host plant responses should focus on future research.
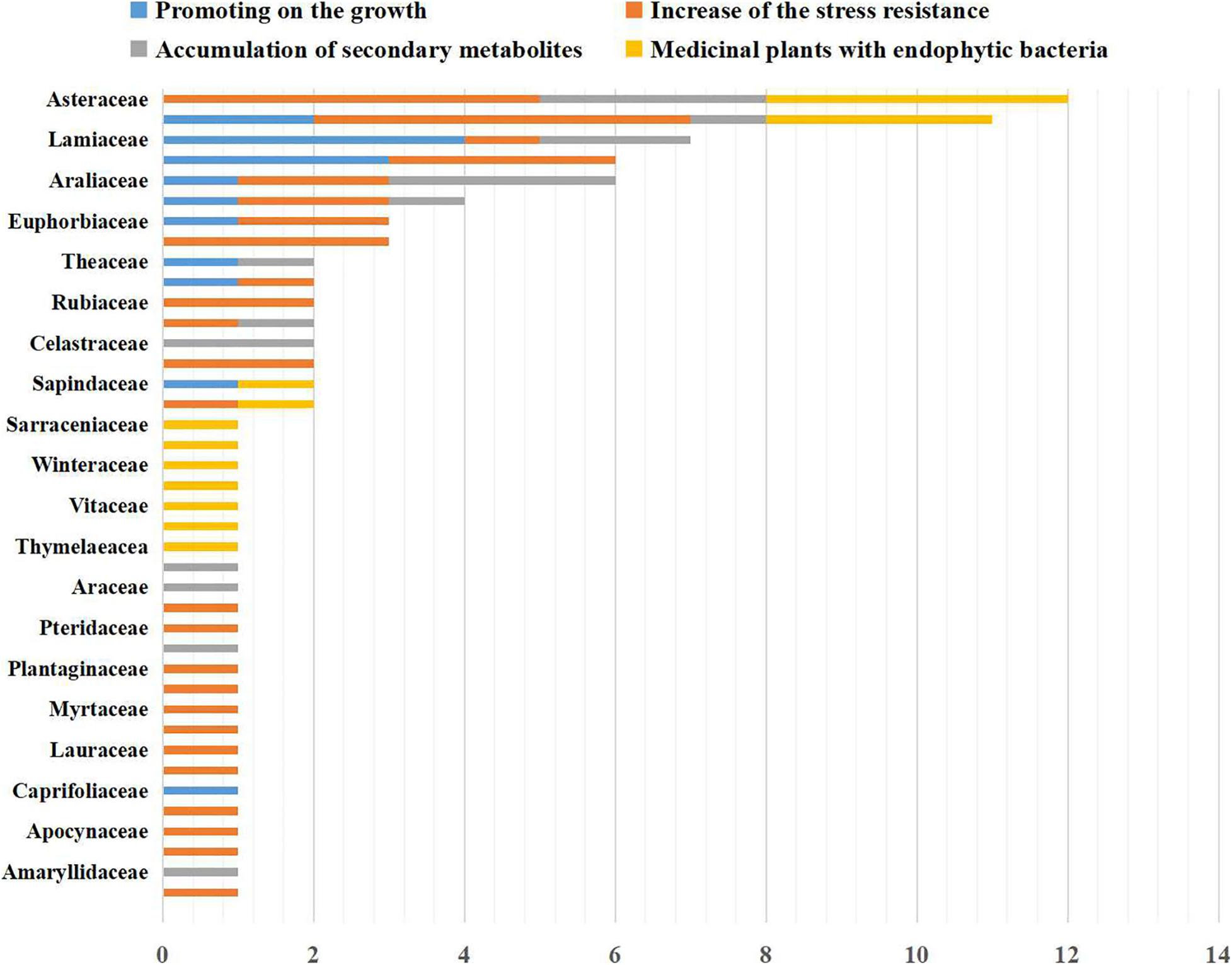
Figure 1. Taxonomy of the 86 species of medicinal plants included in the survey and reference analysis (x-axis: number of species in the family; y-axis: type of family).
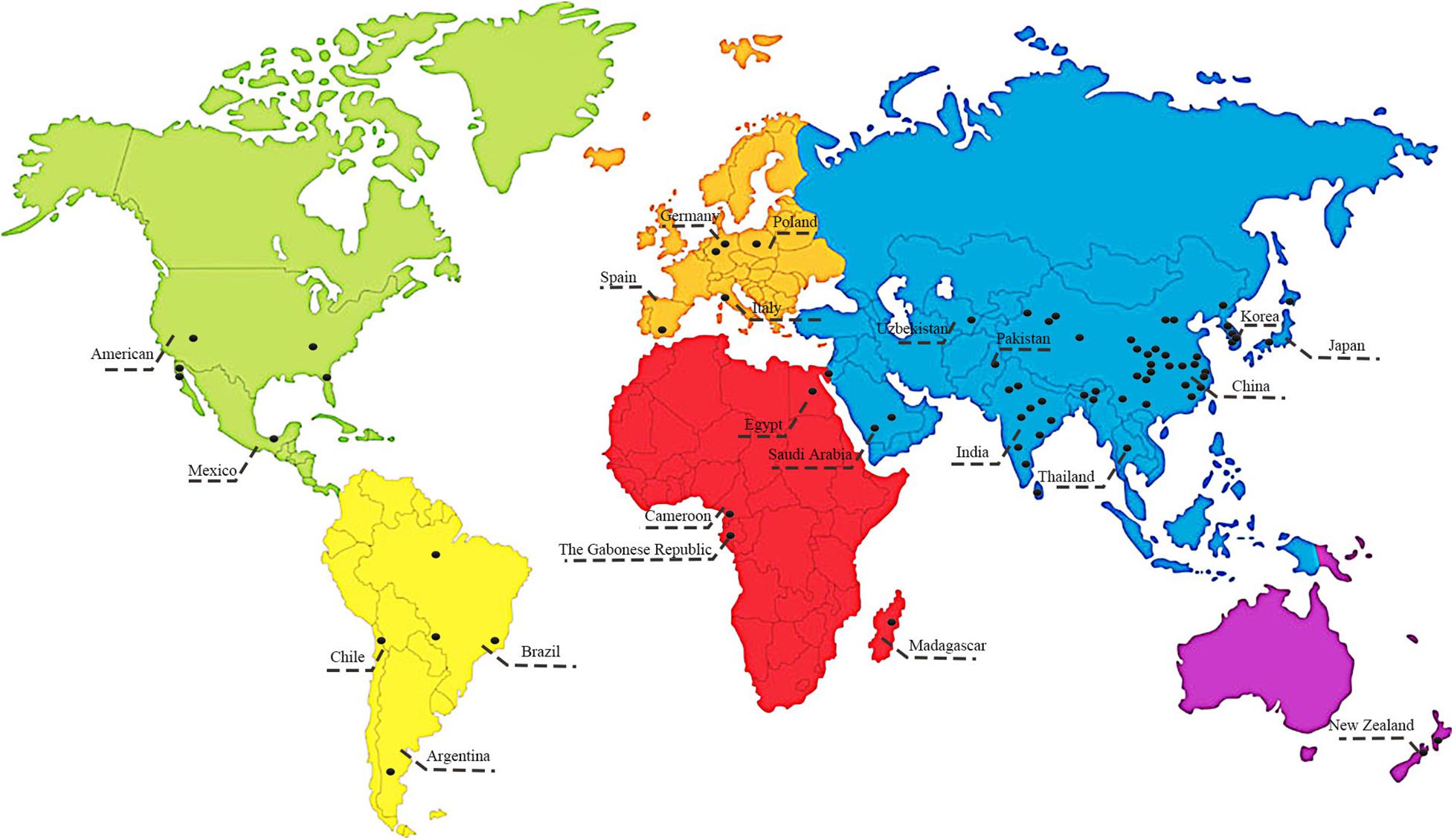
Figure 2. Geographical distribution of medicinal plants and related endophytic bacteria, considered in the survey.
Promotion of Host Plant Growth
Endophytic bacteria are potential candidates to promote the growth of medicinal plants, such as to enhance root and shoot biomass and stimulate seed germination (Vendan et al., 2010). Previously, they were reported to increase the growth of plants through the synthesis of indole acetic acid (IAA) (Table 2; Fouda et al., 2021). In particular, microbial synthesis of IAA by the tryptophan-dependent pathway can affect the growth of plants (Gravel et al., 2007). However, the underlying mechanisms have primarily been studied in vitro, and future studies need to investigate specific metabolites and mechanisms of growth promotion during the actual interaction between the endophytic bacterium and its host. One bacterial endophyte, Serratia marcescens AL2-16, can fix nitrogen in Achyranthes aspera by capturing atmospheric N and converting it into an available nitrogen form through enzymatic reduction (Devi et al., 2016). Wheat seedlings inoculated with a Paenibacillus ehimensis strain from Lonicera japonica showed marked increases in growth associated with greater photosynthetic carbon uptake and light use efficiency (Zhao et al., 2015). Furthermore, multiple other benefits on the growth of plants that are attributed to endophytes include modification of root morphology, osmotic adjustment, phosphate siderophore production improvement, solubilization activity enhancement, and stomatal regulation (Compant et al., 2005; Chen et al., 2016; Zhu, 2019). These plant growth-promotive bacterial endophytes are presently used for forest regeneration and contaminated soil phytoremediation (Ryan et al., 2008; Huang et al., 2020). However, these studies only applied single bacterial strains. The combined effects of endophytic bacterial population should be further studied.
Promotion of Host Plant Abiotic Stress Resistance
Some endophytic bacteria can enhance the resistance of host plants to abiotic stresses, such as heavy metals and salinity (Sheng et al., 2011). Salinity primarily inhibits the growth of plants by lowering soil osmotic potential, forcing the plant to lower its own water potential in an effort to obtain and conserve water. Host plant survival under such conditions may be enhanced by mechanisms such as phytohormone modulation that alleviate osmotic stress impacts (Hasanuzzaman et al., 2014). One experiment demonstrated that the presence of Achromobacter xylosoxidans reduced ethylene levels in Catharanthus roseus and increased the content of antioxidant enzymes such as ascorbate peroxidase, catalase, and superoxide dismutase under saline conditions (Karthikeyan et al., 2012). Moreover, endophytic bacteria stimulated the growth of plants through increasing the nutrient absorption capacity of rhizosphere and enhancing photosynthesis. In a study of Cicer arietinum, inoculation with Bacillus subtilis facilitated the synthesis of photosynthetic pigments and enhanced the plants’ contents of calcium, magnesium, nitrogen, and potassium (Abd_Allah et al., 2018). Silene vulgaris with P. helmanticensis H16, Proteus vulgaris H7, or Pseudomonas sp. H15 treatment had higher fresh shoot biomass under Cd and Zn stress than controls (Płociniczak et al., 2019). These mechanisms of abiotic stress tolerance are through production of antibiotics, enzymatic and non-enzymatic antioxidants, and phytohormones (Khare et al., 2018). Future studies are needed on the interactions among the environment, host plants, and endophytic bacteria, particularly on mechanisms by which endophytic bacteria help their hosts resist environmental stress.
Plants in xenobiotics-contaminated soil naturally recruit endophytes possessing essential genes to degrade the contaminants (Siciliano et al., 2001). In fact, the genes for degradation of nitro-aromatic compounds were found more prevalent in endophytic strains in the fields with nitro-aromatic contaminations than in rhizospheric or soil microbial communities (Ryan et al., 2008). Barac et al. (2004) described an application of bacterial endophytes possessing substantial biotechnological potentials, who demonstrated that engineered Burkholderia cepacia G4 increased plant tolerance toward toluene and decreased the transpiration of toluene to atmosphere (Barac et al., 2004). Newman and Reynolds (2005) summarized the potential advantages of applying endophytic microorganisms for improving xenobiotic remediation, the major of which is the required genetic engineering of a xenobiotic degradation pathway, while gene manipulation is more easily accomplished in bacteria than in plants (Newman and Reynolds, 2005). Moreover, quantitative expression of pollutant catabolic genes in the endophytic populations could serve as a valuable monitoring tool to assess the efficiency of the process of remediation. The unique niche of interior plant environment provides xenobiotic-degradation strains with larger sizes of population attributed to reduced competition. Furthermore, toxic xenobiotics taken up by plants might be degraded in the planta, thereby reducing phytotoxic effects and preventing potential toxicity on herbivorous fauna residing near or on the contaminated sites. Understanding relevant mechanisms that enable the interactions between these endophytic bacteria and the host plants is essential for fully achievement of their biotechnological potentials for various applications. One of the promising areas of future research is to develop endophytic bacteria that enhance the sustainable production of biomass and bioenergy crops as well as soil contaminant phytoremediation.
Increased Biotic Resistance of Host Plants
Crop pests and diseases are among the most significant causes of economic losses in agriculture, and at least one study has demonstrated a correlation between the bioactive compounds produced by endophytic bacteria and host plant disease tolerance (Bibi et al., 2012). Likewise, certain endophytic bacteria from Panax ginseng, termed pathogen antagonists, demonstrate antimicrobial activity against Botrytis cinerea and Cylindrocarpon destructans, as well as hydrogen cyanide production in vitro (Hong et al., 2018). Some Pseudomonas spp. can impede the growth of soil pathogens (Paulin et al., 2009). Endophytic bacteria might protect medicinal plants from pathogens through various mechanisms, including displacing them from their ecological niche within plant tissues and producing antibiotics that directly inhibit their growth (Lacava and Azevedo, 2013). In addition, Bacillus amyloliquefaciens Fy11 appears to suppress Phytophthora blight on pepper indirectly by promoting induced systemic resistance (ISR) (Yang et al., 2014).
There is evidence suggesting that resistant peach cultivars harbor a greater abundance and diversity of bacterial endophytes and more bacterial antagonists of the pathogen Agrobacterium tumefaciens bacterial community may constitute an important component of their A. tumefaciens resistance (Li Q. et al., 2019). Furthermore, numerous non-medicinal plants take use of endophytic bacterial consortia or the combinations of other microorganisms and/or inhibitors to combat harmful phytopathogens as well as enhance their growth (Nagpal et al., 2020; Sundaramoorthy, 2012). Therefore, further in vitro development of endophyte-plant models is essential. As a matter of fact, although several crop microbiota have been investigated in detail for examining their interactions with the respective hosts, medicinal plant model systems are still missing. The complementary information obtained from modern “omics” studies combining with other system biological techniques are crucial for establishing models in predicting and explaining endophyte-mediated processes (Kaul et al., 2016). Additionally, the market-oriented application of biological control agents is essential as well, though a series of issues need repetitive examination, such as the effects of complex external condition alterations, the best application time of endophytes, and the potential pathogenicity of endophytes under condition changes (30). There is a dynamic, intricate intermingling of multiple bacterial species with the host plant, and significant research will be required to fully understand the effects of these complex relationships on host plant disease resistance.
Increased Bioactive Compound Accumulation in Medicinal Plants
Research on endophytic bacterial contribution to their medicinal host plant metabolism is complicated by the fact that some secondary metabolites may be produced by the combined activity of both the bacteria and the host (Brader et al., 2014). Some endophytic bacteria are known to induce the production of secondary metabolites in medicinal plants (Tiwari et al., 2010). For example, Bacillus altitudinis KX230132.1 serves as an effective elicitor that increases ginsenoside concentrations in the valuable medicinal herb, ginseng (Song et al., 2017). Moreover, such elicitors may also participate directly in the biochemical transformation of active ingredients in medicinal plants. Previous work has demonstrated that Burkholderia sp. GE 17-7 can convert the major ginsenoside Rb1 into the minor ginsenoside Rg3, which may possess practical importance to develop the antitumor compound ginsenoside Rg3 (Fu et al., 2018). Such biotransformations using endophytic bacteria have significant potential for promoting the accumulation of rare active ingredients in medicinal plants. However, the specific mechanisms by which endophytic bacteria regulate plant physiology and metabolism remain unknown. Likewise, the processes by which they use intermediate compounds of primary and secondary metabolism as nutrients and precursors for producing new compounds or enhancing existing metabolites are poorly understood.
Previous studies have shown that the synthesis of multiple bioactive secondary metabolites, including alkaloids, sesquiterpenes, polyketones, lactones, organic acids, cyclopeptides, flavonoids, and saponins, with novel applications can be accomplished by endophytes present in host plants (Ek-Ramos et al., 2019). Further activity studies revealed that these endophytic bacteria and their host plants share several similar bio-properties such as antimicrobial, anticancer, anti-inflammatory and anti-HIV activities (Table 3; Rustamova et al., 2020). Endophytes are extremely crucial biological resources, the exploration of which in the future can facilitate environmental sustainability, and they can act as unlimited biomolecule sources for various industrial sectors and benefiting human health. Therefore, it is essential to investigate their genomics as well as the integrated metabolism of endophyte-host plant relationship. Furthermore, it is recommended to deduce the biochemical and physiological characteristics of these endophytes at genomic and metabolomic levels, respectively. To date, no database is exclusively available for endophytes and their metabolites, and the building of which is of great importance in providing solutions for all the aforementioned issues.
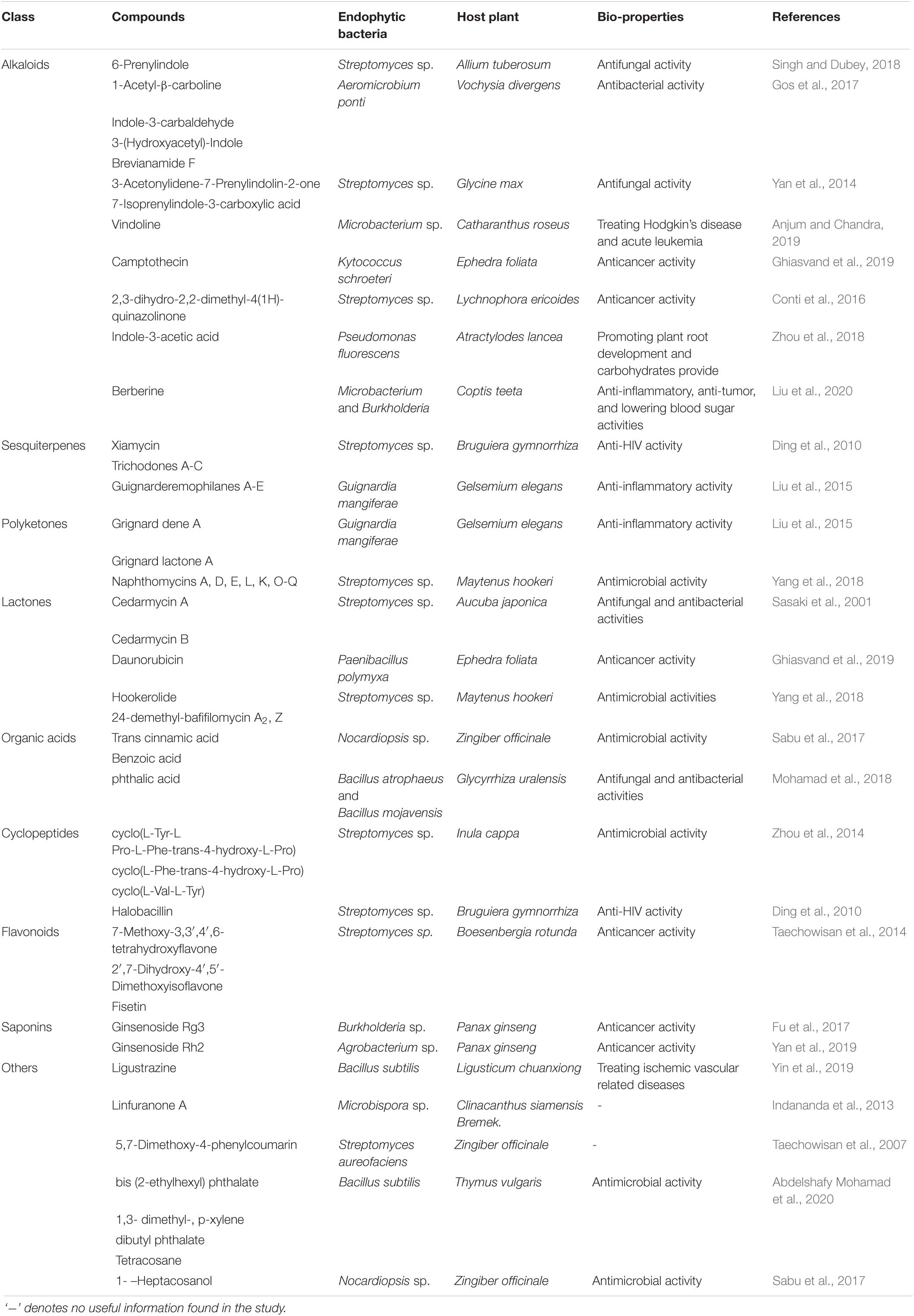
Table 3. Secondary metabolites originated from endophytic bacteria in medicinal plants and their bio-properties.
Conclusion and Perspectives
The genotype, morphology, life history, and health status of medicinal plants can affect the composition, distribution, and structure of their endophytic bacterial community through various physiological mechanisms. Therefore, individual plants have unique endophytic bacteria or bacterial communities, which may inhabit specific plant tissues depending on their roles or preferred niches. On the other hand, the distribution and community structure of bacterial endophytes are also strongly influenced by the external environment (Mitter et al., 2013): temperature, humidity, illumination, and geographic location all determine the distribution of medicinal plants and in turn influence their associated endophytic bacterial species. A better understanding on the influences of the external environment on specific endophytic bacteria will allow us to maximize plant benefits by appropriately modifying external conditions after inoculation.
Bacterial endophytes can promote the growth of plants and protect them from environmental stresses and harmful microorganisms. In return, endophytic bacteria obtain greater access to nutrients and improve their growth (Shi et al., 2010). Endophytic bacteria can stimulate medicinal plant growth through improving seed germination, and indeed this mutualistic association may be necessary for successful germination in some species (Verma et al., 2019). Bacteria applied in biological fertilizer can improve plant nutrition and provide an environmentally sustainable means of improving the growth and yield of plants (Vaishnav et al., 2018). Furthermore, the ability of endophytic bacteria to stimulate both production and accumulation of secondary metabolites lends itself to valuable practical applications (Liu et al., 2015). Inoculation with one or several endophytic bacteria has enormous potentials for enhancing bio-active compound production by medicinal plants (Zhou et al., 2015). Moreover, it may be beneficial to explore the fluctuations in medicinal plant yield and quality caused by environmental factors in order to understand the reasons why certain areas produce Dao-di medicinal plants, which are very high-quality medicinal herbs produced from specific regions and have a long tradition of use and excellent medicinal properties. Given the importance of the endophytic bacteria-medicinal plants interactions, studies of such bacteria may enable the successful development of new areas of natural medicine. Under natural environments, microbial communities with mixed species can exhibit competitive advantages in metabolic complexity, productivity, resistance to invasion, and resource efficiency over monocultures (Karkaria et al., 2021). Being capable of reproducibly and predictably constructing microbial communities for biotechnological or synthetic biological applications would guarantee the application of such advantages. Furthermore, the endophytic associations were studied only in approximately 1–2% of the known plant species (Khare et al., 2018), most of which were the land plants, leaving aquatic plants in lakes, ocean, etc., completely untouched. In situ similis culturing and isolation strategy in different plant niches can be used for find more endophytic bacteria (Castronovo et al., 2021).
Research on beneficial bacterial strains has been primarily limited to laboratory studies, and future research should therefore focus more on field experiments and practical applications to obtain higher quality medicinal plants. Moreover, we know little about the mechanisms of endophytic bacteria-medicinal plants interactions. In the coming decades, we recommend several priority topics for additional research: (1) the development of innovative approaches for the separation and cultivation of endophytic bacteria in order to build a functional library of endophytic bacteria and investigate the effects of unculturable endophytes on medicinal plants; (2) studies on the effects of endophytic bacterial communities on medicinal plants; (3) artificial transformation of functional bacteria to give them additional beneficial functions; (4) strategies to establish symbiotic endophyte-host plant associations, and simulation of the symbiotic vs. parasitic relationships between endophytic bacteria and medicinal plants; (5) transmission mode (endophytes are transmitted vertically as well from plant reproductive tissues to the next generation), and (6) explorations of the mechanisms by which Dao-di medicinal materials are formed, with an emphasis on the role of endophytic bacterial community structure.
Plants have evolved through continuous interaction with microbes, and it is evident that endophytic bacteria play significant roles in improving plant survival and adaptation (Singh et al., 2017). This review outlines the biotic and abiotic factors that influence community structure and endophytic bacterial distribution and summarizes the beneficial effects of endophytes on their host plants. Such information provides a foundation for further studies and can be applied to obtain better bioactive materials from medicinal plants.
Author Contributions
LQ and BZ reviewed and finalized manuscript. WW and WC completed the article writing. JW, SL, and YZ integrated information of tables, analyzed, data, and made pictures. All authors reviewed and approved the manuscript.
Funding
This study was supported by the National Natural Science Foundation of China (82003896 and 81673528) and the Natural Science Foundation of Zhejiang Province (LQ21H280003).
Conflict of Interest
The authors declare that the research was conducted in the absence of any commercial or financial relationships that could be construed as a potential conflict of interest.
References
Abdelshafy Mohamad, O. A., Ma, J. B., Liu, Y. H., Zhang, D., Hua, S., Bhute, S., et al. (2020). Beneficial endophytic bacterial populations associated with medicinal plant Thymus vulgaris alleviate salt stress and confer resistance to Fusarium oxysporum. Front. Plant Sci. 11:47. doi: 10.3389/fpls.2020.00047
Abd_Allah, E. F., Alqarawi, A. A., Hashem, A., Radhakrishnan, R., Al-Huqail, A. A., Al-Otibi, F. O. N., et al. (2018). Endophytic bacterium Bacillus subtilis BERA 71 improves salt tolerance in chickpea plants by regulating the plant defense mechanisms. J. Plant Interact. 13, 37–44. doi: 10.1080/17429145.2017.1414321
Anjum, N., and Chandra, R. (2019). Endophytic bacteria of Catharanthus roseus as an alternative source of vindoline and application of response surface methodology to enhance its production. Arch. Biol. Sci. 71, 27–38. doi: 10.2298/abs180802044a
Aravind, R., Eapen, S. J., Kumar, A., Dinu, A., and Ramana, K. V. (2010). Screening of endophytic bacteria and evaluation of selected isolates for suppression of burrowing nematode (Radopholus similis Thorne) using three varieties of black pepper (Piper nigrum L.). Crop Prot. 29, 318–324. doi: 10.1016/j.cropro.2009.12.005
Aswathy, A. J., Jasim, B., Jyothis, M., and Radhakrishnan, E. K. (2013). Identification of two strains of Paenibacillus sp. as indole 3 acetic acid-producing rhizome-associated endophytic bacteria from Curcuma longa. 3 Biotech. 3, 219–224. doi: 10.1007/s13205-012-0086-0
Barac, T., Taghavi, S., Borremans, B., Provoost, A., Oeyen, L., Colpaert, J. V., et al. (2004). Engineered endophytic bacteria improve phytoremediation of water-soluble, volatile, organic pollutants. Nat. Biotechnol. 22, 583–588. doi: 10.1038/nbt960
Bibi, F., Yasir, M., Song, G., Lee, S., and Chung, Y. (2012). Diversity and characterization of endophytic bacteria associated with tidal flat plants and their antagonistic effects on Oomycetous plant pathogens. Plant Pathology J. 28, 20–31. doi: 10.5423/ppj.Oa.06.2011.0123
Bogas, A. C., Ferreira, A. J., Araújo, W. L., Astolfi-Filho, S., Kitajima, E. W., Lacava, P. T., et al. (2015). Endophytic bacterial diversity in the phyllosphere of Amazon Paullinia cupana associated with asymptomatic and symptomatic anthracnose. Springer Plus 4, 1–13. doi: 10.1186/s40064-015-1037-0
Borah, A., Das, R., Mazumdar, R., and Thakur, D. (2019). Culturable endophytic bacteria of Camellia species endowed with plant growth promoting characteristics. J. Appl. Microbiol. 127, 825–844. doi: 10.1111/jam.14356
Brader, G., Compant, S., Mitter, B., Trognitz, F., and Sessitsch, A. (2014). Metabolic potential of endophytic bacteria. Curr. Opin. Biotech. 27, 30–37. doi: 10.1016/j.copbio.2013.09.012
Campisano, A., Antonielli, L., Pancher, M., Yousaf, S., Pindo, M., and Pertot, I. (2014). Bacterial endophytic communities in the Grapevine depend on pest management. PLoS One 9:e112763. doi: 10.1371/journal.pone.0112763
Castronovo, L. M., Vassallo, A., Mengoni, A., Miceli, E., Bogani, P., Firenzuoli, F., et al. (2021). Medicinal plants and their bacterial microbiota: a review on antimicrobial compounds production for plant and human health. Pathogens 10, 1–17. doi: 10.3390/pathogens10020106
Chen, C., Xin, K., Li, M., Li, X., Cheng, J., Zhang, L., et al. (2016). Paenibacillus sinopodophylli sp. nov., a siderophore-producing endophytic bacterium isolated from roots of Sinopodophyllum hexandrum (Royle) Ying. Int. J. Syst. Evol. Micr. 66, 4993–4999. doi: 10.1099/ijsem.0.001458
Chiellini, C., Maida, I., Emiliani, G., Mengoni, A., Mocali, S., Fabiani, A., et al. (2014). Endophytic and rhizospheric bacterial communities isolated from the medicinal plants Echinacea purpurea and Echinacea angustifolia. Int. Microbiol. 17, 165–174. doi: 10.2436/20.1501.01.219
Compant, S., Clément, C., and Sessitsch, A. (2010). Plant growth-promoting bacteria in the rhizo- and endosphere of plants: their role, colonization, mechanisms involved and prospects for utilization. Soil Biol. Biochem. 42, 669–678. doi: 10.1016/j.soilbio.2009.11.024
Compant, S., Mitter, B., Colli-Mull, J. G., Gangl, H., and Sessitsch, A. (2011). Endophytes of grapevine flowers, berries, and seeds: identification of cultivable bacteria, comparison with other plant parts, and visualization of niches of colonization. Microb. Ecol. 62, 188–197. doi: 10.1007/s00248-011-9883-y
Compant, S., Reiter, B., Sessitsch, A., Nowak, J., Clement, C., and Ait Barka, E. (2005). Endophytic colonization of Vitis vinifera L. by plant growth-promoting bacterium Burkholderia sp. strain PsJN. Appl. Environ. Microbiol. 71, 1685–1693. doi: 10.1128/AEM.71.4.1685-1693.2005
Conti, R., Chagas, F. O., Caraballo-Rodriguez, A. M., Melo, W. G., do Nascimento, A. M., Cavalcanti, B. C., et al. (2016). Endophytic qctinobacteria from the Brazilian medicinal plant Lychnophora ericoides Mart. and the biological potential of their secondary metabolites. Chem. Biodivers. 13, 727–736. doi: 10.1002/cbdv.201500225
Cope-Selby, N., Cookson, A., Squance, M., Donnison, I., Flavell, R., and Farrar, K. (2017). Endophytic bacteria in Miscanthus seed: implications for germination, vertical inheritance of endophytes, plant evolution and breeding. GCB Bioenergy 9, 57–77. doi: 10.1111/gcbb.12364
Dai, J. X., Liu, X. M., and Wang, Y. J. (2014). Diversity of endophytic bacteria in Caragana microphylla grown in the desert grassland of the Ningxia Hui autonomous region of China. Genet. Mol. Res. 13, 2349–2358. doi: 10.4238/2014.April.3.7
Deng, Z. S., Zhao, L. F., Kong, Z. Y., Yang, W. Q., Lindstrom, K., Wang, E. T., et al. (2011). Diversity of endophytic bacteria within nodules of the Sphaerophysa salsula in different regions of Loess Plateau in China. FEMS Microbiol. Ecol. 76, 463–475. doi: 10.1111/j.1574-6941.2011.01063.x
Devi, K. A., Pandey, P., and Sharma, G. D. (2016). Plant growth-promoting endophyte Serratia marcescens AL2-16 enhances the growth of Achyranthes aspera L., a medicinal plant. HAYATI J. Biosci. 23, 173–180. doi: 10.1016/j.hjb.2016.12.006
Ding, L., Munch, J., Goerls, H., Maier, A., Fiebig, H. H., Lin, W. H., et al. (2010). Xiamycin, a pentacyclic indolosesquiterpene with selective anti-HIV activity from a bacterial mangrove endophyte. Bioorg. Med. Chem. Lett. 20, 6685–6687. doi: 10.1016/j.bmcl.2010.09.010
Ek-Ramos, M. J., Gomez-Flores, R., Orozco-Flores, A. A., Rodriguez-Padilla, C., Gonzalez-Ochoa, G., and Tamez-Guerra, P. (2019). Bioactive products from plant-endophytic gram-positive bacteria. Front. Microbiol. 10:463. doi: 10.3389/fmicb.2019.00463
Elmagzob, A. A. H., Ibrahim, M. M., and Zhang, G. F. (2019). Seasonal diversity of endophytic bacteria associated with Cinnamomum camphora (L.) Presl. Diversity 11, 1–12. doi: 10.3390/d11070112
Fernandez-Gonzalez, A. J., Villadas, P. J., Gomez-Lama Cabanas, C., Valverde-Corredor, A., Belaj, A., Mercado-Blanco, J., et al. (2019). Defining the root endosphere and rhizosphere microbiomes from the world olive germplasm collection. Sci. Rep. 9:20423. doi: 10.1038/s41598-019-56977-9
Fouda, A., Eid, A. M., Elsaied, A., El-Belely, E. F., Barghoth, M. G., Azab, E., et al. (2021). Plant growth-promoting endophytic bacterial community inhabiting the leaves of Pulicaria incisa (Lam.) DC inherent to arid regions. Plants 10:76. doi: 10.3390/plants10010076
Fu, Y., Yin, Z., and Yin, C. (2018). Biotransformation of ginsenoside Rb1 to Gyp-XVII and minor ginsenoside Rg3 by endophytic bacterium Flavobacterium sp. GE 32 isolated from Panax ginseng. Lett. Appl. Microbiol. 68, 134–141. doi: 10.1111/lam.13090
Fu, Y., Yin, Z. H., and Yin, C. Y. (2017). Biotransformation of ginsenoside Rb1 to ginsenoside Rg3 by endophytic bacterium Burkholderia sp. GE 17-7 isolated from Panax ginseng. J. Appl. Microbiol. 122, 1579–1585. doi: 10.1111/jam.13435
Gao, Y., Liu, Q., Zang, P., Li, X., Ji, Q., He, Z., et al. (2015). An endophytic bacterium isolated from Panax ginseng C.A. Meyer enhances growth, reduces morbidity, and stimulates ginsenoside biosynthesis. Phytochem. Lett. 11, 132–138. doi: 10.1016/j.phytol.2014.12.007
Ghiasvand, M., Makhdoumi, A., Matin, M. M., and Vaezi, J. (2019). Exploring the bioactive compounds from endophytic bacteria of a medicinal plant: Ephedra foliata (Ephedrales: Ephedraceae). Adv. Tradit. Med. 20, 61–70. doi: 10.1007/s13596-019-00410-z
Gos, F., Savi, D. C., Shaaban, K. A., Thorson, J. S., Aluizio, R., Possiede, Y. M., et al. (2017). Antibacterial activity of endophytic actinomycetes isolated from the medicinal plant Vochysia divergens (Pantanal, Brazil). Front. Microbiol. 8:1642. doi: 10.3389/fmicb.2017.01642
Govarthanan, M., Mythili, R., Selvankumar, T., Kamala-Kannan, S., Rajasekar, A., and Chang, Y. C. (2016). Bioremediation of heavy metals using an endophytic bacterium Paenibacillus sp. RM isolated from the roots of Tridax procumbens. 3 Biotech. 6:242. doi: 10.1007/s13205-016-0560-1
Gravel, V., Antoun, H., and Tweddell, R. J. (2007). Growth stimulation and fruit yield improvement of greenhouse tomato plants by inoculation with Pseudomonas putida or Trichoderma atroviride: possible role of indole acetic acid (IAA). Soil Biol. Biochem. 39, 1968–1977. doi: 10.1016/j.soilbio.2007.02.015
Hallmann, J., Quadt-Hallmann, A., Rodríguez-Kábana, R., and Kloepper, J. W. (1998). Interactions between Meloidogyne incognita and endophytic bacteria in cotton and cucumber. Soil Biol. Biochem. 30, 925–937. doi: 10.1016/S0038-0717(97)00183-1
Hasanuzzaman, M., Nahar, K., Alam, M. M., Bhowmik, P. C., Hossain, M. A., Rahman, M. M., et al. (2014). Potential use of halophytes to remediate saline soils. Biomed. Res. Int. 2014, 1–12. doi: 10.1155/2014/589341
Hassan, S. E. (2017). Plant growth-promoting activities for bacterial and fungal endophytes isolated from medicinal plant of Teucrium polium L. J. Adv. Res. 8, 687–695. doi: 10.1016/j.jare.2017.09.001
He, R., Wang, G., Liu, X., Zhang, C., and Lin, F. (2009). Antagonistic bioactivity of an endophytic bacterium isolated from Epimedium brevicornu Maxim. Afr. J. Biotechnol. 8, 191–195. doi: 10.5897/AJB2009.000-9035
Hong, C. E., Jo, S. H., Jo, I., and Park, J. M. (2018). Diversity and antifungal activity of endophytic bacteria associated with Panax ginseng seedlings. Plant Biotechnol. Rep. 12, 409–418. doi: 10.1007/s11816-018-0504-9
Huang, H., Zhao, Y., Fan, L., Jin, Q., Yang, G., and Xu, Z. (2020). Improvement of manganese phytoremediation by Broussonetia papyrifera with two plant growth promoting (PGP) Bacillus species. Chemosphere 260:127614. doi: 10.1016/j.chemosphere.2020.127614
Ibrahim, E., Fouad, H., Zhang, M., Zhang, Y., Qiu, W., Yan, C., et al. (2019). Biosynthesis of silver nanoparticles using endophytic bacteria and their role in inhibition of rice pathogenic bacteria and plant growth promotion. RSC Adv. 9, 29293–29299. doi: 10.1039/c9ra04246f
Indananda, C., Igarashi, Y., Ikeda, M., Oikawa, T., and Thamchaipenet, A. (2013). Linfuranone A, a new polyketide from plant-derived Microbispora sp. GMKU 363. J. Antibiot. 66, 675–677. doi: 10.1038/ja.2013.67
Jayakumar, A., Krishna, A., Mohan, M., Nair, I. C., and Radhakrishnan, E. K. (2019). Plant growth enhancement, disease resistance, and elemental modulatory effects of plant probiotic endophytic Bacillus sp. Fcl1. Probiotics Antimicrob. Proteins 11, 526–534. doi: 10.1007/s12602-018-9417-8
Jin, H., Yang, X., Yan, Z., Liu, Q., Li, X., Chen, J., et al. (2014). Characterization of rhizosphere and endophytic bacterial communities from leaves, stems and roots of medicinal Stellera chamaejasme L. Syst. Appl. Microbiol. 37, 376–385. doi: 10.1016/j.syapm.2014.05.001
Karkaria, B. D., Fedorec, A. J. H., and Barnes, C. P. (2021). Automated design of synthetic microbial communities. Nat. Commun. 12:672. doi: 10.1038/s41467-020-20756-2
Karthikeyan, B., Joe, M. M., Islam, M. R., and Sa, T. (2012). ACC deaminase containing diazotrophic endophytic bacteria ameliorate salt stress in Catharanthus roseus through reduced ethylene levels and induction of antioxidative defense systems. Symbiosis 56, 77–86. doi: 10.1007/s13199-012-0162-6
Kaul, S., Sharma, T., and Dhar, M. K. (2016). “Omics” Tools for better understanding the plant-endophyte interactions. Front. Plant Sci. 7:955. doi: 10.3389/fpls.2016.00955
Kawaguchi, M., and Minamisawa, K. (2010). Plant-microbe communications for symbiosis. Plant Cell Physiol. 51, 1377–1380. doi: 10.1093/pcp/pcq125
Khaksar, G., Siswanto, D., Treesubsuntorn, C., and Thiravetyan, P. (2016). Euphorbia milii-endophytic bacteria interactions affect hormonal levels of the native host differently under various airborne pollutants. Mol. Plant Microbe Interact. 29, 663–673. doi: 10.1094/MPMI-06-16-0117-R
Khare, E., Mishra, J., and Arora, N. K. (2018). Multifaceted interactions between endophytes and plant: developments and prospects. Front. Microbiol. 9:2732. doi: 10.3389/fmicb.2018.02732
Lacava, P. T., and Azevedo, J. L. (2013). Biological control of insect-pest and diseases by endophytes. Adv. Endophy. Res. 12, 231–256. doi: 10.1007/978-81-322-1575-2_13
Li, F., He, X., Sun, Y., Zhang, X., Tang, X., Li, Y., et al. (2019). Distinct endophytes are used by diverse plants for adaptation to karst regions. Sci. Rep. 9, 5246–5257. doi: 10.1038/s41598-019-41802-0
Li, J., Zhao, G. Z., Varma, A., Qin, S., Xiong, Z., Huang, H. Y., et al. (2012). An endophytic Pseudonocardia species induces the production of artemisinin in Artemisia annua. PLoS One 7:e51410. doi: 10.1371/journal.pone.0051410
Li, Q., Guo, R., Li, Y., Hartman, W. H., Li, S., Zhang, Z., et al. (2019). Insight into the bacterial endophytic communities of peach cultivars related to crown gall disease resistance. Appl. Environ. Microbiol. 85:e02931-18. doi: 10.1128/AEM.02931-18
Liu, T. H., Zhang, X. M., Tian, S. Z., Chen, L. G., and Yuan, J. L. (2020). Bioinformatics analysis of endophytic bacteria related to berberine in the Chinese medicinal plant Coptis teeta Wall. 3 Biotech. 10:96. doi: 10.1007/s13205-020-2084-y
Liu, Y., Jianwei, G., Li, L. D., Asem, M. D., Zhang, Y., Mohamad, O. A., et al. (2017). Endophytic bacteria associated with endangered plant Ferula sinkiangensis K. M. Shen in an arid land: diversity and plant growth-promoting traits. J. Arid Land. 9, 432–445. doi: 10.1007/s40333-017-0015-5
Liu, Y., Liu, W., and Liang, Z. (2015). Endophytic bacteria from Pinellia ternata, a new source of purine alkaloids and bacterial manure. Pharm. Biol. 53, 1545–1548. doi: 10.3109/13880209.2015.1016580
Ma, L., Cao, Y. H., Cheng, M. H., Huang, Y., Mo, M. H., Wang, Y., et al. (2013). Phylogenetic diversity of bacterial endophytes of Panax notoginseng with antagonistic characteristics towards pathogens of root-rot disease complex. Antonie Van Leeuwenhoek 103, 299–312. doi: 10.1007/s10482-012-9810-3
Mitter, B., Brader, G., Afzal, M., Compant, S., Naveed, M., Trognitz, F., et al. (2013). Advances in elucidating beneficial interactions between plants, soil, and bacteria. Adv. Agron. 121, 381–445. doi: 10.1016/B978-0-12-407685-3.00007-4
Mocali, S., Bertelli, E., Cello, F. D., Mengoni, A., Sfalanga, A., Viliani, F., et al. (2003). Fluctuation of bacteria isolated from elm tissues during different seasons and from different plant organs. Res. Microbiol. 154, 105–114. doi: 10.1016/S0923-2508(03)00031-7
Mohamad, O. A. A., Li, L., Ma, J. B., Hatab, S., Xu, L., Guo, J. W., et al. (2018). Evaluation of the antimicrobial activity of endophytic bacterial populations from Chinese traditional medicinal plant Licorice and characterization of the bioactive secondary metabolites produced by Bacillus atrophaeus against Verticillium dahliae. Front. Microbiol. 9:924. doi: 10.3389/fmicb.2018.00924
Nagpal, S., Sharma, P., Sirari, A., and Gupta, R. K. (2020). Coordination of Mesorhizobium sp. and endophytic bacteria as elicitor of biocontrol against Fusarium wilt in chickpea. Eur. J. Plant Pathol. 158, 143–161. doi: 10.1007/s10658-020-02062-1
Namdar, D., Charuvi, D., Ajjampura, V., Mazuz, M., Ion, A., Kamara, I., et al. (2019). LED lighting affects the composition and biological activity of Cannabis sativa secondary metabolites. Ind. Crop. Prod. 132, 177–185. doi: 10.1016/j.indcrop.2019.02.016
Newman, L. A., and Reynolds, C. M. (2005). Bacteria and phytoremediation: new uses for endophytic bacteria in plants. Trends Biotechnol. 23, 6–8. doi: 10.1016/j.tibtech.2004.11.010
Ou, T., Xu, W., Wang, F., Strobel, G., Zhou, Z., Xiang, Z., et al. (2019). A microbiome study reveals seasonal variation in endophytic bacteria among different Mulberry cultivars. Comput. Struct. Biotechnol. J. 17, 1091–1100. doi: 10.1016/j.csbj.2019.07.018
Paulin, M. M., Novinscak, A., St-Arnaud, M., Goyer, C., Decoste, N. J., Prive, J. P., et al. (2009). Transcriptional activity of antifungal metabolite-encoding genes phlD and hcnBC in Pseudomonas spp. using qRT-PCR. FEMS Microbiol. Ecol. 68, 212–222. doi: 10.1111/j.1574-6941.2009.00669.x
Pereira, S. I. A., Monteiro, C., Vega, A. L., and Castro, P. M. L. (2016). Endophytic culturable bacteria colonizing Lavandula dentata L. plants: isolation, characterization and evaluation of their plant growth-promoting activities. Ecol. Eng. 87, 91–97. doi: 10.1016/j.ecoleng.2015.11.033
Płociniczak, T., Chodór, M., Pacwa-Płociniczak, M., and Piotrowska-Seget, Z. (2019). Metal-tolerant endophytic bacteria associated with Silene vulgaris support the Cd and Zn phytoextraction in non-host plants. Chemosphere 219, 250–260. doi: 10.1016/j.chemosphere.2018.12.018
Purushotham, N., Jones, E., Monk, J., and Ridgway, H. (2020). Community structure, diversity and potential of endophytic bacteria in the primitive New Zealand medicinal plant Pseudowintera colorata. Plants 9, 156–170. doi: 10.3390/plants9020156
Qi, Y., Li, X., Wang, J., Wang, C., and Zhao, S. (2021). Efficacy of plant growth-promoting bacteria Streptomyces werraensis F3 for chemical modifications of diseased soil of ginseng. Biocontrol Sci. Technol. 31, 219–233. doi: 10.1080/09583157.2020.1843598
Qin, S., Feng, W. W., Zhang, Y. J., Wang, T. T., Xiong, Y. W., and Xing, K. (2018). Diversity of bacterial microbiota of coastal Halophyte Limonium sinense and amelioration of salinity stress damage by symbiotic plant growth-promoting actinobacterium Glutamicibacter halophytocola KLBMP 5180. Appl. Environ. Microbiol. 84:e01533-18. doi: 10.1128/AEM.01533-18
Qin, S., Li, J., Chen, H. H., Zhao, G. Z., Zhu, W. Y., Jiang, C. L., et al. (2009). Isolation, diversity, and antimicrobial activity of rare actinobacteria from medicinal plants of tropical rain forests in Xishuangbanna, China. Appl. Environ. Microbiol. 75, 6176–6186. doi: 10.1128/AEM.01034-09
Qin, S., Miao, Q., Feng, W., Wang, Y., Zhu, X., Xing, K., et al. (2015). Biodiversity and plant growth promoting traits of culturable endophytic actinobacteria associated with Jatropha curcas L. growing in Panxi dry-hot valley soil. Appl. Soil Ecol. 93, 47–55. doi: 10.1016/j.apsoil.2015.04.004
Rakotoniriana, E. F., Rafamantanana, M., Randriamampionona, D., Rabemanantsoa, C., Urveg-Ratsimamanga, S., El Jaziri, M., et al. (2013). Study in vitro of the impact of endophytic bacteria isolated from Centella asiatica on the disease incidence caused by the hemibiotrophic fungus Colletotrichum higginsianum. Antonie Van Leeuwenhoek 103, 121–133. doi: 10.1007/s10482-012-9791-2
Reinhold-Hurek, B., and Hurek, T. (2011). Living inside plants: bacterial endophytes. Curr. Opin. Plant Biol. 14, 435–443. doi: 10.1016/j.pbi.2011.04.004
Rustamova, N., Wubulikasimu, A., Mukhamedov, N., Gao, Y., Egamberdieva, D., and Yili, A. (2020). Endophytic bacteria associated with medicinal plant Vernonia anthelmintica: diversity and characterization. Curr. Microbiol. 77, 1457–1465. doi: 10.1007/s00284-020-01924-5
Ryan, R. P., Germaine, K., Franks, A., Ryan, D. J., and Dowling, D. N. (2008). Bacterial endophytes: recent developments and applications. FEMS Microbiol. Lett. 278, 1–9. doi: 10.1111/j.1574-6968.2007.00918.x
Sabu, R., Soumya, K. R., and Radhakrishnan, E. K. (2017). Endophytic Nocardiopsis sp. from Zingiber officinale with both antiphytopathogenic mechanisms and antibiofilm activity against clinical isolates. 3 Biotech. 7:115. doi: 10.1007/s13205-017-0735-4
Saikkonen, K., Wali, P., Helander, M., and Faeth, S. H. (2004). Evolution of endophyte-plant symbioses. Trends Plant Sci. 9, 275–280. doi: 10.1016/j.tplants.2004.04.005
Santana, R. S. M., Fernandes, G. W., Ávila, M. P., Reis, M. P., Araújo, F. M. G. D., Salim, A. C. M., et al. (2016). Endophytic microbiota associated with the root tips and leaves of Baccharis dracunculifolia. Braz. Arch. Biol. Technol. 59:e16160287. doi: 10.1590/1678-4324-2016160287
Santos, J. F., Sacramento, B. L., Mota, K. N. A. B., Souza, J. T. D., and Neto, A. D. D. A. (2014). Sunflower growth according to seed inoculation with endophytic bacteria. Pesqui. Agropecu. Trop. 44, 142–150. doi: 10.1590/s1983-40632014000200008
Sasaki, T., Igarashi, Y., Saito, N., and Furumai, T. (2001). Cedarmycins A and B, new antimicrobial antibiotics from Streptomyces sp. TP-A0456. J. Antibiot. 547, 567–572. doi: 10.7164/antibiotics.54.567
Senthilkumar, M., Anandham, R., Madhaiyan, M., Venkateswaran, V., and Sa, T. (2011). Endophytic bacteria: perspectives and applications in agricultural crop production. Bact. Agrobiol. Crop Ecosyst. 3, 61–96. doi: 10.1007/978-3-642-18357-7_3
Sexton, W. K., Fidero, M., Spain, J. C., Jiang, L., Bucalo, K., Cruse-Sanders, J. M., et al. (2019). Characterization of endophytic bacterial communities within greenhouse and field-grown rhizomes of three rare pitcher plant species (Sarracenia oreophila, S. leucophylla, and S. purpurea spp. venosa) with an emphasis on nitrogen-fixing bacteria. Plant Soil 447, 257–279. doi: 10.1007/s11104-019-04372-8
Sheng, H. M., Gao, H. S., Xue, L. G., Ding, S., Song, C. L., Feng, H. Y., et al. (2011). Analysis of the composition and characteristics of culturable endophytic bacteria within subnival plants of the Tianshan Mountains, Northwestern China. Curr. Microbiol. 62, 923–932. doi: 10.1007/s00284-010-9800-5
Shi, Y., Lou, K., and Li, C. (2010). Growth and photosynthetic efficiency promotion of sugar beet (Beta vulgaris L.) by endophytic bacteria. Photosynth. Res. 105, 5–13. doi: 10.1007/s11120-010-9547-7
Siciliano, S. D., Fortin, N., Mihoc, A., Wisse, G., Labelle, S., Beaumier, D., et al. (2001). Selection of specific endophytic bacterial genotypes by plants in response to soil contamination. Appl. Environ. Microbiol. 67, 2469–2475. doi: 10.1128/AEM.67.6.2469-2475.2001
Singh, M., Kumar, A., Singh, R., and Pandey, K. D. (2017). Endophytic bacteria: a new source of bioactive compounds. 3 Biotech. 7, 315–329. doi: 10.1007/s13205-017-0942-z
Singh, R., and Dubey, A. K. (2018). Diversity and applications of endophytic actinobacteria of plants in special and other ecological niches. Front. Microbiol. 9:1767. doi: 10.3389/fmicb.2018.01767
Song, X., Wu, H., Yin, Z., Lian, M., and Yin, C. (2017). Endophytic bacteria isolated from Panax ginseng improves ginsenoside accumulation in adventitious Ginseng root culture. Molecules 22, 837–849. doi: 10.3390/molecules22060837
Sundaramoorthy, S. (2012). Consortial effect of endophytic and plant growth promoting rhizobacteria for the management of early blight of tomato incited by Alternaria solani. J. Plant Pathol. Microbiol. 03:e1000145. doi: 10.4172/2157-7471.1000145
Taechowisan, T., Chanaphat, S., Ruensamran, W., and Phutdhawong, W. S. (2014). Antibacterial activity of new flavonoids from Streptomyces sp. BT01; an endophyte in Boesenbergia rotunda (L.) Mansf. J. Appl. Pharm. Sci. 4, 8–13. doi: 10.7324/japs.2014.40402
Taechowisan, T., Lu, C., Shen, Y., and Lumyong, S. (2007). Antitumor activity of 4-arylcoumarins from endophytic Streptomyces aureofaciens CMUAc130. J. Cancer Res. Ther. 3, 86–91. doi: 10.4103/0973-1482.34685
Tiwari, R., Awasthi, A., Mall, M., Shukla, A. K., Srinivas, K. V. N. S., Syamasundar, K. V., et al. (2013). Bacterial endophyte-mediated enhancement of in planta content of key terpenoid indole alkaloids and growth parameters of Catharanthus roseus. Ind. Crop. Prod. 43, 306–310. doi: 10.1016/j.indcrop.2012.07.045
Tiwari, R., Kalra, A., Darokar, M. P., Chandra, M., Aggarwal, N., Singh, A. K., et al. (2010). Endophytic bacteria from Ocimum sanctum and their yield enhancing capabilities. Curr. Microbiol. 60, 167–171. doi: 10.1007/s00284-009-9520-x
Vaishnav, A., Shukla, A. K., Sharma, A., Kumar, R., and Choudhary, D. K. (2018). Endophytic bacteria in plant salt stress tolerance: current and future prospects. J. Plant Growth Regul. 38, 650–668. doi: 10.1007/s00344-018-9880-1
Vendan, R. T., Yu, Y. J., Lee, S. H., and Rhee, Y. H. (2010). Diversity of endophytic bacteria in ginseng and their potential for plant growth promotion. J. Microbiol. Res. 48, 559–565. doi: 10.1007/s12275-010-0082-1
Verma, S. K., Kharwar, R. N., and White, J. F. (2019). The role of seed-vectored endophytes in seedling development and establishment. Symbiosis 78, 107–113. doi: 10.1007/s13199-019-00619-1
Walitang, D. I., Kim, C. G., Kim, K., Kang, Y., Kim, Y. K., and Sa, T. (2018). The influence of host genotype and salt stress on the seed endophytic community of salt-sensitive and salt-tolerant rice cultivars. BMC Plant Biol. 18:51. doi: 10.1186/s12870-018-1261-1
Xu, J. Y., Han, Y. H., Chen, Y., Zhu, L. J., and Ma, L. Q. (2016). Arsenic transformation and plant growth promotion characteristics of As-resistant endophytic bacteria from As-hyperaccumulator Pteris vittata. Chemosphere 144, 1233–1240. doi: 10.1016/j.chemosphere.2015.09.102
Xu, L., Zhang, Y., Wang, L., Chen, W., and Wei, G. (2014). Diversity of endophytic bacteria associated with nodules of two indigenous legumes at different altitudes of the Qilian Mountains in China. Syst. Appl. Microbiol. 37, 457–465. doi: 10.1016/j.syapm.2014.05.009
Yan, H., Jin, H., Fu, Y., Yin, Z., and Yin, C. (2019). Production of rare Ginsenosides Rg3 and Rh2 by endophytic bacteria from Panax ginseng. J. Agric. Food Chem. 67, 8493–8499. doi: 10.1021/acs.jafc.9b03159
Yan, Y., Zhang, S., Zhang, J., Ma, P., Duan, J., and Liang, Z. (2014). Effect and mechanism of endophytic bacteria on growth and secondary metabolite synthesis in Salvia miltiorrhiza hairy roots. Acta Physiol. Plant. 36, 1095–1105. doi: 10.1007/s11738-014-1484-1
Yang, H. R., Yuan, J., Liu, L. H., Zhang, W., Chen, F., and Dai, C. C. (2019). Endophytic Pseudomonas fluorescens induced sesquiterpenoid accumulation mediated by gibberellic acid and jasmonic acid in Atractylodes macrocephala Koidz plantlets. Plant Cell Tissue Organ Cult. 138, 445–457. doi: 10.1007/s11240-019-01640-4
Yang, R., Fan, X., Cai, X., and Hu, F. (2014). The inhibitory mechanisms by mixtures of two endophytic bacterial strains isolated from Ginkgo biloba against pepper phytophthora blight. Biol. Control. 85, 59–67. doi: 10.1016/j.biocontrol.2014.09.013
Yang, R., Liu, P., and Ye, W. (2017). Illumina-based analysis of endophytic bacterial diversity of tree peony (Paeonia Sect. Moutan) roots and leaves. Braz. J. Microbiol. 48, 695–705. doi: 10.1016/j.bjm.2017.02.009
Yang, Y.-H., Yang, D.-S., Li, G.-H., Liu, R., Huang, X.-W., Zhang, K.-Q., et al. (2018). New secondary metabolites from an engineering mutant of endophytic Streptomyces sp. CS. Fitoterapia 130, 17–25. doi: 10.1016/j.fitote.2018.07.019
Yarte, M. E., Gismondi, M. I., Llorente, B. E., and Larraburu, E. E. (2020). Isolation of endophytic bacteria from the medicinal, forestal and ornamental tree Handroanthus impetiginosus. Environ. Technol. [Epub ahead of print]. doi: 10.1080/09593330.2020.1818833
Yin, D. D., Wang, Y. L., Yang, M., Yin, D. K., Wang, G. K., and Xu, F. (2019). Analysis of Chuanxiong Rhizoma substrate on production of ligustrazine in endophytic Bacillus subtilis by ultra high performance liquid chromatography with quadrupole time-of-flight mass spectrometry. J. Sep. Sci. 42, 3067–3076. doi: 10.1002/jssc.201900030
Zhang, Q., Acuna, J. J., Inostroza, N. G., Mora, M. L., Radic, S., Sadowsky, M. J., et al. (2019). Endophytic bacterial communities associated with roots and leaves of plants growing in Chilean extreme environments. Sci. Rep. 9, 4950–4962. doi: 10.1038/s41598-019-41160-x
Zhao, L., Xu, Y., Lai, X. H., Shan, C., Deng, Z., and Ji, Y. (2015). Screening and characterization of endophytic Bacillus and Paenibacillus strains from medicinal plant Lonicera japonica for use as potential plant growth promoters. Braz. J. Microbiol. 46, 977–989. doi: 10.1590/S1517-838246420140024
Zhou, H., Yang, Y., Yang, X., Li, W., Xiong, Z., Zhao, L., et al. (2014). A new cyclic tetrapeptide from an endophytic Streptomyces sp. YIM67005. Nat. Prod. Res. 28, 318–323. doi: 10.1080/14786419.2013.863198
Zhou, J. Y., Li, X., Zhao, D., Deng-Wang, M. Y., and Dai, C. C. (2016). Reactive oxygen species and hormone signaling cascades in endophytic bacterium induced essential oil accumulation in Atractylodes lancea. Planta 244, 699–712. doi: 10.1007/s00425-016-2536-0
Zhou, J. Y., Sun, K., Chen, F., Yuan, J., Li, X., and Dai, C. C. (2018). Endophytic Pseudomonas induces metabolic flux changes that enhance medicinal sesquiterpenoid accumulation in Atractylodes lancea. Plant Physiol. Bioch. 130, 473–481. doi: 10.1016/j.plaphy.2018.07.016
Zhou, J. Y., Yuan, J., Li, X., Ning, Y. F., and Dai, C. C. (2015). Endophytic bacterium-triggered reactive oxygen species directly increase oxygenous sesquiterpenoid content and diversity in Atractylodes lancea. Appl. Environ. Microbiol. 82, 1577–1585. doi: 10.1128/AEM.03434-15
Zhu, X., Jin, L., Sun, K., Li, S., Ling, W., and Li, X. (2016). Potential of endophytic bacterium Paenibacillus sp. PHE-3 isolated from Plantago asiatica L. for reduction of PAH contamination in plant tissues. Int. J. Environ. Res. Public Health 13, 633–645. doi: 10.3390/ijerph13070633
Keywords: bacterial community, endophytic bacteria, medicinal plants, plant growth-promoting bacteria, plant-microbe relationships, secondary metabolites
Citation: Wu W, Chen W, Liu S, Wu J, Zhu Y, Qin L and Zhu B (2021) Beneficial Relationships Between Endophytic Bacteria and Medicinal Plants. Front. Plant Sci. 12:646146. doi: 10.3389/fpls.2021.646146
Received: 15 January 2021; Accepted: 06 April 2021;
Published: 22 April 2021.
Edited by:
Kalliope K. Papadopoulou, University of Thessaly, GreeceReviewed by:
Evdokia Syranidou, Technical University of Crete, GreeceErica Lumini, Institute for Sustainable Plant Protection, National Research Council (CNR), Italy
Copyright © 2021 Wu, Chen, Liu, Wu, Zhu, Qin and Zhu. This is an open-access article distributed under the terms of the Creative Commons Attribution License (CC BY). The use, distribution or reproduction in other forums is permitted, provided the original author(s) and the copyright owner(s) are credited and that the original publication in this journal is cited, in accordance with accepted academic practice. No use, distribution or reproduction is permitted which does not comply with these terms.
*Correspondence: Luping Qin, lpqin@zcmu.edu.cn; Bo Zhu, zhubo@zcmu.edu.cn