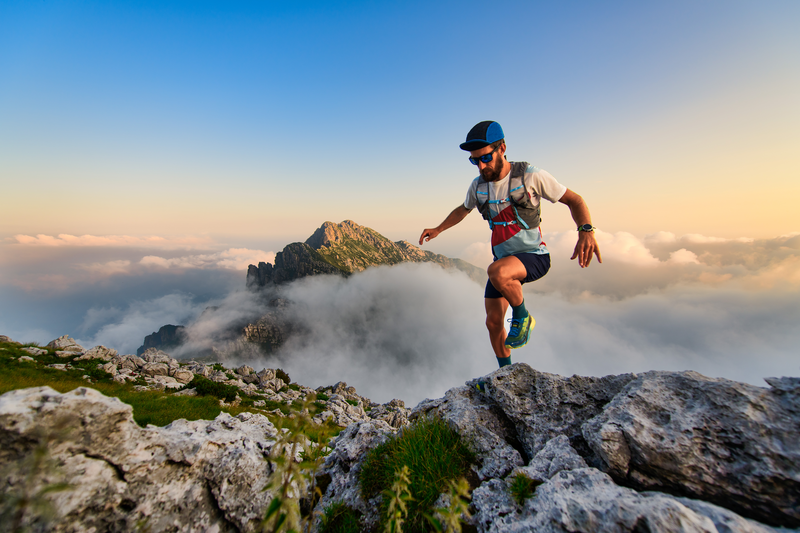
94% of researchers rate our articles as excellent or good
Learn more about the work of our research integrity team to safeguard the quality of each article we publish.
Find out more
ORIGINAL RESEARCH article
Front. Plant Sci. , 20 May 2021
Sec. Aquatic Photosynthetic Organisms
Volume 12 - 2021 | https://doi.org/10.3389/fpls.2021.643425
This article is part of the Research Topic Current Advances in Seagrass Research View all 14 articles
Seagrass meadows are critical ecosystems, and they are among the most threatened habitats on the planet. As an anthropogenic biotic invader, Spartina alterniflora Loisel. competes with native plants, threatens native ecosystems and coastal aquaculture, and may cause local biodiversity to decline. The distribution area of the exotic species S. alterniflora in the Yellow River Delta had been expanding to ca.4,000 ha from 1990 to 2018. In this study, we reported, for the first time, the competitive effects of the exotic plant (S. alterniflora) on seagrass (Zostera japonica Asch. & Graebn.) by field investigation and a transplant experiment in the Yellow River Delta. Within the first 3 months of the field experiment, S. alterniflora had pushed forward 14 m into the Z. japonica distribution region. In the study region, the area of S. alterniflora in 2019 increased by 516 times compared with its initial area in 2015. Inhibition of Z. japonica growth increased with the invasion of S. alterniflora. Z. japonica had been degrading significantly under the pressure of S. alterniflora invasion. S. alterniflora propagates sexually via seeds for long distance invasion and asexually by tillers and rhizomes for short distance invasion. Our results describe the invasion pattern of S. alterniflora and can be used to develop strategies for prevention and control of S. alterniflora invasion.
Biological invasion is a significant component of human-caused global environmental change. Biotic invaders can establish a new range in which they proliferate, spread, and persist to damage the environment (Mack et al., 2000). Animal invaders can cause extinctions of vulnerable native species. For example, the Nile perch (Lates niloticus) has contributed to the extinction of more than 200 endemic fish species through predation and competition for food (Lowe et al., 2000). Plant invaders can completely alter the energy budgets and nutrient cycling in a native ecosystem and can greatly diminish the diversity of native species. For example, the water hyacinth (Eichhornia crassipes) dramatically reduces biological diversity in aquatic ecosystems through shading and crowding of native aquatic plants (Lowe et al., 2000).
Spartina alterniflora is a perennial halophyte that is native to the Atlantic coast of the Americas from Canada to Argentina (Meng et al., 2020). S. alterniflora has high tolerance and adaptability to environmental stressors due to fast growth, well-developed below-ground tissues, high salt tolerance, and high reproductive capacity through both asexual and sexual reproduction, making it a good “ecosystem engineer” (Simenstad and Thom, 1995; Qin et al., 1998; Crooks, 2002; Chung et al., 2004; Zhang et al., 2004; Chung, 2006; Huang and Zhang, 2007). Due to these biological traits, S. alterniflora has been introduced throughout Europe, North America, Australia, and Asia in efforts to prevent shoreline erosion (Maricle and Lee, 2002). In December of 1979, S. alterniflora was introduced into China (Chung, 1993). In 1985, S. alterniflora in China covered ∼260 ha (Meng et al., 2020). In 2003, S. alterniflora was listed as an invasive species by the State Environment Protection Administration of China. Satellite images showed that the area covered by S. alterniflora had reached 34,451 ha in 2007 (Zuo et al., 2012). Today, S. alterniflora has expanded to around 50,000 ha of the coastal regions of China (Meng et al., 2020).
As a biotic invader, S. alterniflora competes with native plants, threatens native ecosystems and coastal aquaculture, and may cause local biodiversity to decline (Callaway and Josselyn, 1992; Daehler and Strong, 1996; Brusati and Grosholz, 2007; Zuo et al., 2012). The introduction of S. alterniflora resulted in a significant decrease of abundance, coverage, and seed and fresh corm output of Scirpus mariqueter which is a native species of sedge in Dongtan of Chongming Island (Chen et al., 2004; Wang et al., 2012). Xia et al. (2015) reported that S. alterniflora actively accumulated and stored sulfur in its tissues, leading to a high sulfide level in the invaded environment, which was harmful to some native species. Populations of native plants such as Suaeda glauca and Phragmites communis have decreased rapidly in Yancheng National Nature Reserve due to expansion of alien S. alterniflora, and the habitat changes are believed to be at least partially responsible for dramatic declines of wintering red-crowned crane populations (Liu C. et al., 2013). Ge et al. (2012) reported that the biodiversity of macrobenthic communities increased in the initial stage of S. alterniflora invasion and then decreased in the middle and final stage of invasion in Wenzhou Bay, China.
Seagrasses provide habitat, food, and nurseries for a variety of marine organisms, attenuate currents and waves, alter nutrient cycling and food web structures, and stabilize sediments (Costanza et al., 1997; Jackson et al., 2001; Barbier et al., 2011; Liu X.J. et al., 2013; Nordlund et al., 2018; Unsworth et al., 2019). Seagrass meadows are critical ecosystems, and they are among the most threatened habitats on the planet. Waycott et al. (2009) reported that 29% of the known areal extent of seagrass meadows has disappeared since seagrass areas were initially recorded in 1879. Human disturbances such as eutrophication and habitat loss due to dredging, anchoring, and coastal construction play a key role in the loss of seagrasses (Orth et al., 2006; Ralph et al., 2006; Salinas et al., 2020).
After S. alterniflora was first introduced to the Yellow River Delta in 1990, it grew and spread rapidly. By 2018, its distribution region had expanded to 4005.89 ha, which was 2557 times as its initial colonization area (Ren et al., 2019). Zhang X.M. et al. (2019) reported that the seagrass Zostera japonica Asch. & Graebn. on both sides of the mouth of the Yellow River covered about 1031.8 ha. Z. japonica is an intertidal seagrass species that is native to the Western Pacific Ocean from Russia to Vietnam (Miki, 1933). This species is widely threatened by climate change and human activity in its native range in Korea, Japan, and China (Lee et al., 2004; Abe et al., 2009; Hodoki et al., 2013; Zhang et al., 2014, 2015, 2020a,b; Lin et al., 2018; Yue et al., 2020). The niche of S. alterniflora overlaps with that of Z. japonica on low tidal beaches. However, competition between S. alterniflora and Z. japonica has rarely been studied experimentally in the field.
In May 2015, we found an initial invasion of S. alterniflora (ca.100 m2) at Z. japonica meadows in our study region, and S. alterniflora had been occurring in small patches surrounded by Z. japonica meadows. In this study, we investigated the competitive effects of S. alterniflora on Z. japonica in the study region to assess the possible consequences of the introduced S. alterniflora on the native Z. japonica communities in the Yellow River Delta. We document the invasion pattern of S. alterniflora by in situ investigation and our work can be used to develop strategies for prevention and control of S. alterniflora invasion.
The study region (37° 51′ 7″ N, 119° 5′ 47″ E) is located in the Yellow River Delta, Shandong Province, China (Figure 1). The climate is temperate monsoonal, with an average annual precipitation of 560 mm and average annual temperature of 12.9°C (Han et al., 2018). The tides are irregular semidiurnal, with an average tidal amplitude of 1.1–1.5 m (Hu and Cao, 2003). The broad mudflat of the Yellow River Delta displays distinct vegetation zones, producing a unique wetland landscape extending from sea to land. Z. japonica meadows occur in the sea, with S. alterniflora, Suaeda salsa, Tamarix chinensis, and reed marsh occurring in the landward direction (Zhang X.M. et al., 2019). Based on the vegetation differences, the study region can be divided into the S. alterniflora distribution region, the ecotone (S. alterniflora and Z. japonica) and the Z. japonica distribution region.
In May 2015, we used mobile phone to record the distribution of S. alterniflora in the study region. In October 2016 and July 2019, the total distribution region of S. alterniflora was photographed by aerial vehicle (DJI Phantom 3 Advanced) during low tide. Then we estimated the distribution area of S. alterniflora by using road as a reference.
In July 2019, during peak biomass, the range of Z. japonica meadows was examined by walking during low tide or by rowing during mid to high tide, using GPS to record accessible boundary points.
To estimate the sediment accretion due to the invasion of S. alterniflora in the study region, we recorded the corresponding tide level from the same place (37° 51′ 6″ N, 119° 5′ 53″ E) exposed to the sea surface in May 2015 and May 2019. The tidal regime for the study region was provided by the National Marine Data Information Center of China.
Four sediment cores (diameter = 10.5 cm, depth = 12 cm) were collected from each region to measure grain size distribution using laser diffraction analysis (Short and Coles, 2001). These sediment cores were also used to determine the concentration of sediment organic matter (OM, in %DW), which was calculated as the fractional weight loss of dry sediment in the samples after combustion at 550°C for 4 h (Heiri et al., 2001). To compare the sulfide content in sediment in the S. alterniflora and the Z. japonica distribution regions, four sediments cores (diameter = 10.5 cm, depth = 12 cm) were collected from the two regions and measured in the laboratory by using the iodometric method (National marine environmental monitoring center [NMEMC], 2007).
Three parallel transect lines running perpendicular to the shoreline were marked for sampling in the study region. All transects began in the S. alterniflora distribution region and ended in the Z. japonica distribution region. Eleven sampling points were marked at an interval of 7 m along each transect line. Sampling was conducted in April, July, and October, 2019. One sediment core (diameter = 10.5 cm, depth = 12 cm) was haphazardly collected within 1 m of each sampling point along each transect.
All samples were sieved (2 mm) with seawater in situ to remove most of the sediment and then cleaned using tap water in the laboratory. For each sample, total number of shoots was counted to provide shoot density (shoots ⋅ m–2). The height (cm) of 15 shoots was measured. All shoots were dried to a constant weight at 60°C to estimate total shoot biomass (g DW ⋅ m–2). We also counted the density (shoots ⋅ m–2), height (cm), and biomass (g WW ⋅ m–2) of seedlings, which were only observed in April, 2019. All of these biological parameters were measured separately for S. alterniflora and Z. japonica.
We designated three transplant sites (S1, S2, and S3) for the experiment in which we transplanted Z. japonica. S1 was located in the S. alterniflora distribution region, which represented the completed stage of invasion. S2 was located at the edge of the S. alterniflora distribution region, which represented the intermediate stage of invasion. S3 was located in the ecotone, which represented the initial stage of invasion.
All transplant experiments began in July, 2019. For each transplant site, four transplant plots (1 m × 1 m) were designed parallelly to the coast with an interval of 2 m. We assumed that Z. japonica might not grow at S1, thus we added another three transplant plots (1 m × 1 m) at S1 to ensure adequate monitoring data. In each plot, all S. alterniflora were removed using a sickle and hoe. Sod consisting of Z. japonica and sediment was collected from the center of the Z. japonica distribution region using tools for pushing the plant material horizontally below the rhizome layer. Then, these sods were transplanted into the pre-dug plots from which S. alterniflora were removed.
After transplantation, seven sediment cores (diameter = 10.5 cm, depth = 12 cm) were collected semimonthly at S1, and four sediment cores (diameter = 10.5 cm, depth = 12 cm) were collected semimonthly at S2 and S3. All cores were sieved (2 mm) with seawater in situ to remove most of the sediment, and the plant materials then were taken back to the laboratory. For each sample, plant material was washed using tap water and divided into above-ground (shoots: sheath and leaves) and below-ground (rhizomes and roots) parts. The shoot height (cm) was measured and the numbers of vegetative and flowering shoots were counted to provide shoot density (shoots ⋅ m–2). Total tissues were dried to a constant weight at 60°C to estimate total above-ground and below-ground biomass (g DW ⋅ m–2).
For the field investigation data, we used a one-way analysis of variance (ANOVA) to test the significance of differences in the total shoot density, biomass of S. alterniflora, and Z. japonica among time and sampling points and to test for significance of differences in seedling density and biomass among the 11 sampling points along the transect line. The significance of differences in grain sizes and OM among three regions was also tested by using a one-way ANOVA. An independent-samples t-test was used to test the significance of differences in the sulfide content in sediment among S. alterniflora and Z. japonica distribution regions. For the transplant experiment data, we used one-way ANOVA or independent-samples t-test to test the significance of differences in the reproductive shoot density, vegetative shoot density, total shoot density, below-ground biomass, above-ground biomass, total biomass, vegetative shoot height, and reproductive shoot height among time points and transplant sites. When the data did not satisfy the homogeneity of variance requirement, a Kruskal-Wallis Test was used to test the significance of differences. Multiple comparisons were performed using the Duncan method, and the level of significance was set at p < 0.05. Statistical analyses were conducted using SPSS 17.0. All values are reported as mean ± SD.
In 2015, the area of S. alterniflora was ca.100 m2. In 2016, it had extended over ca. 1.25 ha. By 2019, the distribution region had expanded to ca. 5.16 ha (Figures 2A,B; Supplementary Figure 1).
Figure 2. Spartina alterniflora invasion of Zostera japonica meadows [(A) 2015; (B) 2019] and the sediment accretion in the study region (C).
The distance between the shore at the study region to the lower limits of Z. japonica meadows was 537 m, while the distance between the upper and lower limits of Z. japonica meadows was only 165 m in July 2019.
The place was exposed to the sea surface at the tidal water level of 50 cm in May 2015, while it was exposed to the sea surface at the tidal water level of 80 cm in May 2019. It shows that approximately 30 cm sediment was accumulated owing to the invasion of S. alterniflora in the study region (Figure 2C).
The sediment particulate size composition in all the regions was mainly sand (Figure 3), while the percentage of sand at S. alterniflora distribution region was greater than at Z. japonica distribution region [F(2,9) = 8.887, p < 0.05]. There was no significant difference in OM among region [Table 1; F(2,9) = 0.646, p > 0.05]. The sulfide content of sediment in S. alterniflora distribution region (237.50 ± 88.88 mg ⋅ kg–1) was greater than in Z. japonica distribution region (156.25 ± 58.55 mg ⋅ kg–1).
Figure 3. Sediment grain sizes at the three regions. Values are mean ± SD. Different letters indicate significant difference between different regions at same size of grain.
There were significant differences among sampling points in total shoot density, biomass of S. alterniflora, and Z. japonica in each sampling time (p < 0.05). The total shoot density of S. alterniflora and Z. japonica decreased and increased with moving seaward, respectively (Figure 4). Biomass showed the same trend as shoot density (Figure 5). In April, the biomass of S. alterniflora at points 5 and 8 was higher than at the adjacent points (Figure 5A). In July and October, the biomass of S. alterniflora at point 8 was still higher than that of the adjacent points (Figures 5B,C).
Figure 4. Total shoot density of Spartina alterniflora and Zostera japonica at the 11 sampling points in April (A), July (B), and October (C) 2019. All S. alterniflora shoots collected at sampling points 9 and 10 in July 2019 were grown from dormant seeds that germinated in May 2019. Values are mean ± SD.
Figure 5. Biomass of Spartina alterniflora and Zostera japonica at the 11 sampling points in April (A), July (B), and October (C) 2019. Values are mean ± SD.
At point 1, the total shoot density of S. alterniflora in April (4,852.90 ± 808.82 shoots ⋅ m–2) was higher than that in October (2,503.48 ± 657.02 shoots ⋅ m–2) and July (1771.69 ± 546.05 shoots ⋅ m–2) [F(2,6) = 16.852, p < 0.05]. At point 3, the biomass of S. alterniflora in April (1,153.14 ± 1,280.93 g DW ⋅ m–2) was lower than that in July (2,612.86 ± 903.89 g DW ⋅ m–2) and October (3,799.51 ± 1,176.13 g DW ⋅ m–2) [F(2,6) = 13.330, p < 0.05]. At points 4 and 5, the total shoot density of Z. japonica decreased with sampling time [F(2,6) = 9.188, p < 0.05; F(2,6) = 9.046, p < 0.05, respectively]. At point 9, the total shoot density of Z. japonica was highest in July (3,851.51 ± 1110.28 shoots ⋅ m–2), followed by April (1848.72 ± 115.55 shoots ⋅ m–2) and October (1,502.09 ± 200.13 shoots ⋅ m–2) [F(2,6) = 11.256, p < 0.05]. At points 7, 9, 10, and 11, the biomass of Z. japonica was highest in July, followed by April and October (p < 0.05).
In April, Z. japonica was observed from point 3 to point 11 and S. alterniflora was observed from point 1 to point 8 (Figure 6A). In July and October, Z. japonica was no longer observed at points 3 and 4, and S. alterniflora was observed at points 9 and 10 (Figures 6B,C). S. alterniflora shoots collected at sampling points 9 and 10 in July 2019 had small shoot height (<20 cm), biomass (<10 g WW ⋅ m–2), and density (<50 shoots ⋅ m–2); these shoots could be easily uprooted and were obviously grown from dormant seeds that germinated in May 2019. This finding shows that S. alterniflora had pushed forward 14 m into the Z. japonica distribution region.
Figure 6. Shoot height of Spartina alterniflora and Zostera japonica at the 11 sampling points in April (A), July (B), and October (C) 2019. Values are mean ± SD.
In April, seedlings of S. alterniflora and Z. japonica were found in the samples. The seedling density and biomass of S. alterniflora differed significantly among sampling points [F(10,22) = 5.809, p < 0.05; F(10,22) = 8.459, p < 0.05, respectively]. The seedling biomass of S. alterniflora and Z. japonica decreased and increased with moving seaward, respectively (Figure 7A). Seedling density showed the same as biomass (Figure 7B). At point 8, the seedling density (115.55 ± 200.13 shoots ⋅ m–2) and biomass (2.70 ± 4.67 g WW ⋅ m–2) of S. alterniflora were higher than at adjacent points (Figure 7). However, seedling density and biomass of Z. japonica did not differ significantly among sampling points [F(10,22) = 0.741, p > 0.05; F(10,22) = 0.883, p > 0.05, respectively].
Figure 7. Seedling biomass (A), density (B), and height (C) of Spartina alterniflora and Zostera japonica at the 11 sampling points in April 2019. Values are mean ± SD.
Fifteen days after transplantation, the reproductive shoot density, vegetative shoot density, and total shoot density of Z. japonica at S1 were lower than those at S2 and S3 [Figure 8; Chi-square(2) = 11.343, p < 0.05; Chi-square(2) = 11.809, p < 0.05; F(2,12) = 36.916, p < 0.05, respectively]. The above-ground biomass, total biomass, vegetative shoot height, and reproductive shoot height at S1 were lower than those at S2 and S3 [Figures 8, 10; F(2,12) = 20.697, p < 0.05; F(2,12) = 6.973, p < 0.05; F(2,12) = 8.073, p < 0.05; Chi-square(2) = 8.480, p < 0.05, respectively]. One month after transplantation, all Z. japonica at S1 had died. The total shoot density and biomass at S2 were lower than those at S3 [Figures 7, 8; Chi-square(2) = 7.659, p < 0.05; Chi-square(2) = 8.199, p < 0.05, respectively].
Figure 8. Reproductive shoot density (A), vegetative shoot density (B), and total shoot density (C) of Zostera japonica in the three transplant sites at 0.5 and 1 month after transplantation. Values are mean ± SD. ∗Indicates significant difference between different time points in the same transplant site. Different letters indicate significant difference between different transplant sites at the same time point.
No significant differences among time points for total shoot density and biomass at S3 were detected [Figures 8, 9; t(6) = 2.289, p > 0.05; t(6) = 0.586, p > 0.05, respectively]. The vegetative shoot height and reproductive shoot height at S3 decreased over time (Figure 10).
Figure 9. Below-ground biomass (A), above-ground biomass (B), and total biomass (C) of Zostera japonica in the three transplant sites at 0.5 and 1 month after transplantation. Values are mean ± SD. ∗Indicates significant difference between time points in the same transplant site. Different letters indicate significant difference between different transplant sites at the same time point.
Figure 10. Reproductive shoot height (A) and vegetative shoot height (B) of Zostera japonica in the three transplant sites at 0.5 and 1 month after transplantation. Values are mean ± SD. ∗Indicates significant difference between time points in the same transplant site. Different letters indicate significant difference between different transplant sites at the same time point.
In this study, we reported, for the first time, the competitive effects of the exotic plant (S. alterniflora) on seagrass (Z. japonica) by field investigation and a transplant experiment in the Yellow River Delta. Our results indicated that the seagrass meadow had been degrading significantly under the pressure of S. alterniflora invasion. Our results document the invasion pattern of S. alterniflora and can be used to develop strategies for prevention and control of S. alterniflora invasion.
By 2019, S. alterniflora covered ca. 5.16 ha, which was 516 times greater than its initial area in 2015. In Jiangsu Province, the total planting area of S. alterniflora was 278.93 ha along the north coast of Sheyang in 1982–1992, but it rapidly spread and increased to 1160.69 ha by July 1, 1994 (Zuo et al., 2012). Ren et al. (2019) used remote sensing technology to monitor distributional changes of S. alterniflora in the Yellow River Delta. They reported that S. alterniflora was first identified in Landsat 5 images in 2008 on the north bank with an initial area of 0.72 ha, whereas it was first identified on the south bank in 2011 with an initial area of 1.61 ha. Since 2011, S. alterniflora has rapidly colonized the modern Yellow River Delta. Chen et al. (2020) reported that Fast Super-Resolution Convolutional Neural Networks (FSRCNN) were very effective at discerning and estimating the size of small S. alterniflora patches (<50 m2). They found that the total patch area of S. alterniflora at one site increased 13 times from 11.54 ha to 157.42 ha since 2012.
The distance between the upper and lower limits of Z. japonica meadows was greater than 550 m in 2015 (Zhang X.M. et al., 2019), while the distance we observed was only 165 m in July 2019. The result means Z. japonica was seriously degraded and had not moving seaward under the pressure of S. alterniflora invasion.
In the Yellow River Delta, the invasion of S. alterniflora is a common phenomenon at the Z. japonica meadows. At another site, a limited initial invasion of S. alterniflora (ca.30 m2) at Z. japonica meadows was observed in August, 2015; however, after 5 years, large areas of this site were also invaded by S. alterniflora (Supplementary Figure 2).
In our field investigation, S. alterniflora pushed forward 14 m into the Z. japonica distribution region between April and July 2019, which illustrates its powerful invasion ability. Liu et al.(2020a,b) reported that the height, density, above-ground biomass, and reproductive ability of S. alterniflora in the Chinese range were greater than those in the native range.
Seedlings of S. alterniflora are smaller than adult shoots and can reach sexual maturity within 3–4 months (Meng et al., 2020). In this study, S. alterniflora seedlings were recorded in spring, while seed maturation occurred in Autumn, indicating that seeds did not germinate immediately after maturity. S. alterniflora propagates sexually via seeds and asexually by tillers and rhizomes. S. alterniflora shoots collected at sampling points 9 and 10 in July 2019 could be easily uprooted and were very small; these shoots were obviously grown from dormant seeds that germinated in May 2019.
S. alterniflora has aerenchyma, making it adaptable to the anoxic environment (Meng et al., 2020). Therefore, seedlings could survival in the Z. japonica distribution region that was characterized by relatively deep seawater. We observed multiple peaks of biomass of S. alterniflora as the sampling point number increased, which represented the irregularly shaped patches of S. alterniflora. In the initial stages of S. alterniflora invasion, sparse patches arising from germinating seeds are distributed on the tidal flats (Ma et al., 2019).
S. alterniflora has a high tolerance and adaptability to environmental stressors (including lower temperature). During winter, the above-ground tissues of S. alterniflora turn yellow but keep standing (Supplementary Figure 3). Therefore, S. alterniflora would not die off at sampling points 9 and 10 throughout the winter. In addition, we investigated the growth of S. alterniflora at sampling points 9 and 10 in June, 2020; the results showed that the shoot height was greater than 25 cm, and the shoot density was greater than 250 shoots ⋅ m–2. These values were greater than those in October 2019 (except the shoot density at point 10). These data indicated that S. alterniflora at sampling points 9 and 10 had spread locally by clonal growth in 2020.
We found that the biomass of Z. japonica increased in July and decreased in October within the Z. japonica distribution region. However, the biomass of Z. japonica decreased over time in the front part of the ecotone. Zhang X.M. et al. (2019) previously described the seasonal changes in Z. japonica in the Yellow River Delta. They reported that growth increased in June and July, peaked in August, and dramatically declined by October. The higher height of S. alterniflora shielded the sunlight originally used by Z. japonica and thus inhibited growth of the seagrass. The biomass of S. alterniflora in October was higher than that in July, which indicated that the growth ability of S. alterniflora was greater than that of Z. japonica.
Over the past century, the coastal erosion of many deltas was triggered by the decrease of sediment loads of many rivers owing to damming and irrigation as well as improved land-use practices, such as the Yellow River Delta (Milliman, 1997; Wang et al., 2007; Syvitski et al., 2009; Bi et al., 2014; Jiang et al., 2017). Waves impacting the shoreline can suspend sediment while currents can transport these materials elsewhere, causing erosion (Fan et al., 2018). Higher growth rates of S. alterniflora promote greater standing biomass, which in turn slows water velocity on the marsh platform, lowers wave height, reduces erosion, and enhances mineral sediment deposition (Kirwan and Megonigal, 2013). It is suggested that the invasion of S. alterniflora increased the accumulation of sediment. Gao et al. (2014) reported that S. alterniflora can enhance the settling flux of suspended sediment and the deposition rate on the tidal flats by reducing the near-bed shear stress associated with tidal currents. Sediment accretion may increase the ecological niche of S. alterniflora, which is one potential explanation for the successful invasion of S. alterniflora into Z. japonica meadows.
S. alterniflora propagates sexually via seeds for long distance invasion and asexually by tillers and rhizomes for short distance invasion (Figure 11). The seeds fall off the plant after maturing and float with the tide into the seagrass bed, where sparse patches of the invader arise from germinating seeds. Subsequently, the deposition rate increases gradually as the density of clonal ramets increases, and over time the growth of Z. japonica is inhibited. Ultimately, patches of S. alterniflora connect and replace the seagrass bed community through vegetative reproduction.
Results of the transplant experiment showed that inhibition of Z. japonica growth increased with the invasion stage of S. alterniflora. The ecological functions of Z. japonica meadows may be reduced by invasion of S. alterniflora. Zhang Y. et al. (2019) reported that the invasion of S. alterniflora into the Chinese coastal wetlands caused profound biotic homogenization of soil communities across latitudes. In the Chongming wetland of Shanghai, the species and number of birds in the S. alterniflora-vegetated community were much lower than in native reed communities (Meng et al., 2020). The Yellow River Delta National Nature Reserve is an important transit point, habitat, and breeding ground for bird migration. A high-density S. alterniflora population can act as an “isolation belt” between birds and their food resources, which may decrease bird diversity.
Significant quantities of carbon can be sequestered by, stored in, and released from seagrass meadows (Prentice et al., 2020). Salinas et al. (2020) reported that the loss of seagrass due to human and natural disturbances may have caused the release of 11–21 Tg CO2–eq in Australia since the 1950s. Yang et al. (2013) reported that soil organic carbon was significantly increased in the upper 0–30 cm soil layer after S. alterniflora invaded the S. salsa and Cyperus malaccensis communities. Wang et al. (2019) found that the soil total C content was 13.3 ± 0.3 t ⋅ ha–1 in the native mangrove regions, while the soil total C content was 10.9 ± 0.3 t ⋅ ha–1 in the invasive S. alterniflora regions. Thus, the carbon buried in Z. japonica meadows may change after S. alterniflora invasion. Measuring soil organic carbon (SOC,%) by organic matter (OM,%) analyzer can significantly reduce cost per sample analysis over the long-term (Howard et al., 2014). Craft et al. (1991) reported the relationships between OM (%) and SOC (%) in tidal salt marsh [SOC = 0.40 × OM + 0.0025 (OM)2, r2 = 0.99]. Also, Fourqurean et al. (2012) reported the relationships between OM (%) and SOC (%) in seagrass meadows (SOC = 0.43 × OM – 0.33, r2 = 0.96). Therefore, we used the first equation to estimate the SOC content in the S. alterniflora distribution region and the ecotone. The SOC content in the Z. japonica distribution region was estimated by the second equation. The results showed that SOC content (%) increased significantly in the upper 0–12 cm soil layer after S. alterniflora invaded the Z. japonica communities (Supplementary Table 1; p < 0.05). Due to the limitation of sampling methods, the difference in SOC density was unknown; and the difference in SOC in deep sediment (12–100 cm) at different regions should be investigated in the future.
In conclusion, we found that S. alterniflora pushed 14 m into the Z. japonica distribution region within 3 months and that the invasion ability of S. alterniflora was greater than the growth ability of Z. japonica. The growth of Z. japonica was inhibited gradually with the invasion of S. alterniflora. Our results describe the invasion pattern of S. alterniflora and can be used to develop strategies for the prevention and control of S. alterniflora invasion.
The raw data supporting the conclusions of this article will be made available by the authors, without undue reservation.
SY and YZ conceived the ideas and designed methodology and led the writing of the manuscript. SY, SCX, XZ, ML, YQ, RG, SX, and YuZ collected the data. SY analyzed the data. All authors contributed critically to the drafts and gave final approval for publication.
This research was supported by the National Key R&D Program of China (2019YFD0900704 and 2019YFD0901300), the National Science & Technology Basic Work Program (2015FY110600), the Key Research Project of Frontier Sciences of CAS (QYZDB-SSW-DQC041-1), and the Taishan Scholars Program (Distinguished Taishan Scholars).
The authors declare that the research was conducted in the absence of any commercial or financial relationships that could be construed as a potential conflict of interest.
We would like to thank Huaxin Li, Chuang Li, and Cong Yu for their help in the field survey.
The Supplementary Material for this article can be found online at: https://www.frontiersin.org/articles/10.3389/fpls.2021.643425/full#supplementary-material
Supplementary Table 1 | The SOC content (%) at the three regions. Values are mean ± SD. Different letters indicate significant difference between different regions.
Supplementary Figure 1 | Spartina alterniflora invasion of Zostera japonica meadows [(A) May 2015, low tide; (B) October 2016, high tide; (C) July 2019, low tide)].
Supplementary Figure 2 | Spartina alterniflora invasion of Zostera japonica meadows in another site [(A,B) August 2015; (C,D) June 2020; the red five-pointed star was represented the location of this site]. ZJ, Zostera japonica; SA, Spartina alterniflora.
Supplementary Figure 3 | Field photo of the study region [(A) April 27, 2019; (B) July 21, 2019].
Abe, M., Yokota, K., Kurashima, A., and Maegawa, M. (2009). Temperature characteristics in seed germination and growth of Zostera japonica Ascherson & Graebner from Ago Bay, Mie Prefecture, central Japan. Fish. Sci. 75, 921–927. doi: 10.1007/s12562-009-0123-z
Barbier, E. B., Hacker, S. D., Kennedy, C., Koch, E. W., Stier, A. C., and Silliman, B. R. (2011). The value of estuarine and coastal ecosystem services. Ecol. Monogr. 81, 169–193. doi: 10.1890/10-1510.1
Bi, N., Wang, H., and Yang, Z. (2014). Recent changes in the erosion–accretion patterns of the active Huanghe (Yellow River) delta lobe caused by human activities. Cont. Shelf Res. 90, 70–78. doi: 10.1016/j.csr.2014.02.014
Brusati, E. D., and Grosholz, E. D. (2007). Effect of native and invasive cordgrass on Macoma petalum density, growth, and isotopic signatures. Estuar. Coast. Shelf Sci. 71, 517–522. doi: 10.1016/j.ecss.2006.08.026
Callaway, J. C., and Josselyn, M. N. (1992). The introduction and spread of smooth cordgrass (Spartina alterniflora) in south San Francisco Bay. Estuaries 15, 218–226. doi: 10.2307/1352695
Chen, M., Ke, Y., Bai, J., Li, P., Lyu, M., Gong, Z., et al. (2020). Monitoring early stage invasion of exotic Spartina alterniflora using deep-learning super-resolution techniques based on multisource high-resolution satellite imagery: a case study in the Yellow River Delta, China. Int. J. Appl. Earth Obs. Geoinf. 92:102180. doi: 10.1016/j.jag.2020.102180
Chen, Z. Y., Li, B., Zhong, Y., and Chen, J. K. (2004). Local competitive effects of introduced Spartina alterniflora on Scirpus mariqueter at Dongtan of Chongming Island, the Yangtze River estuary and their potential ecological consequences. Hydrobiologia 528, 99–106. doi: 10.1007/s10750-004-1888-9
Chung, C.-H. (1993). Thirty years of ecological engineering with Spartina plantations in China. Ecol. Eng. 2, 261–289. doi: 10.1016/0925-8574(93)90019-C
Chung, C.-H. (2006). Forty years of ecological engineering with Spartina plantations in China. Ecol. Eng. 27, 49–57. doi: 10.1016/j.ecoleng.2005.09.012
Chung, C. H., Zhuo, R. Z., and Xu, G. W. (2004). Creation of Spartina plantations for reclaiming Dongtai, China, tidal flats and offshore sands. Ecol. Eng. 23, 135–150. doi: 10.1016/j.ecoleng.2004.07.004
Costanza, R., d’Arge, R., de Groot, R., Farber, S., Grasso, M., Hannon, B., et al. (1997). The value of the world’s ecosystem services and natural capital. Nature 387, 253–260. doi: 10.1038/387253a0
Craft, C., Seneca, E., and Broome, S. (1991). Loss on ignition and Kjeldahl digestion for estimating organic carbon and total nitrogen in estuarine marsh soils: calibration with dry combustion. Estuaries 14, 175–179. doi: 10.2307/1351691
Crooks, J. A. (2002). Characterizing ecosystem-level consequences of biological invasions: the role of ecosystem engineers. Oikos 97, 153–166. doi: 10.1034/j.1600-0706.2002.970201.x
Daehler, C. C., and Strong, D. R. (1996). Status, prediction and prevention of introduced cordgrass Spartina spp invasions in Pacific estuaries, USA. Biol. Conserv. 78, 51–58. doi: 10.1016/0006-3207(96)00017-1
Fan, Y., Chen, S., Zhao, B., Pan, S., Jiang, C., and Ji, H. (2018). Shoreline dynamics of the active yellow river delta since the implementation of water-sediment regulation scheme: a remote-sensing and statistics-based approach. Estuar. Coast. Shelf Sci. 200, 406–419. doi: 10.1016/j.ecss.2017.11.035
Fourqurean, J. W., Duarte, C. M., Kennedy, H., Marbà, N., Holmer, M., Mateo, M. A., et al. (2012). Seagrass ecosystems as a globally significant carbon stock. Nat. Geosci. 5, 505–509. doi: 10.1038/ngeo1477
Gao, S., Du, Y., Xie, W., Gao, W., Wang, D., and Wu, X. (2014). Environment-ecosystem dynamic processes of Spartina alterniflora salt-marshes along the eastern China coastlines. Sci. China Earth Sci. 57, 2567–2586. doi: 10.1007/s11430-014-4954-9
Ge, B.-M., Bao, Y.-X., Cheng, H.-Y., Zhang, D.-Z., and Hu, Z.-Y. (2012). Influence of Spartina alterniflora invasion stages on macrobenthic communities on a tidal flat in Wenzhou Bay, China. Braz. J. Oceanogr. 60, 441–448. doi: 10.1590/s1679-87592012000300014
Han, G., Sun, B., Chu, X., Xing, Q., Song, W., and Xia, J. (2018). Precipitation events reduce soil respiration in a coastal wetland based on four-year continuous field measurements. Agric. For. Meteorol. 256, 292–303. doi: 10.1016/j.agrformet.2018.03.018
Heiri, O., Lotter, A. F., and Lemcke, G. (2001). Loss on ignition as a method for estimating organic and carbonate content in sediments: reproducibility and comparability of results. J. Paleolimn. 25, 101–110. doi: 10.1023/a:1008119611481
Hodoki, Y., Ohbayashi, K., Tanaka, N., and Kunii, H. (2013). Evaluation of genetic diversity in Zostera japonica (Aschers. et Graebn.) for seagrass conservation in brackish lower reaches of the Hii River System, Japan. Estuar. Coast. 36, 127–134. doi: 10.1007/s12237-012-9564-1
Howard, J., Hoyt, S., Isensee, K., Telszewski, M., and Pidgeon, E. (2014). Coastal Blue Carbon: Methods for Assessing Carbon Stocks and Emissions Factors in Mangroves, Tidal Salt Marshes, and Seagrasses. Gland: International Union for Conservation of Nature, 62–63.
Hu, C., and Cao, W. (2003). Variation, regulation and control of flow and sediment in the Yellow River Estuary I: mechanism of flow-sediment transport and evolution. J. Sediment Res. 5, 1–8.
Huang, H., and Zhang, L. (2007). A study of the population dynamics of Spartina alterniflora at Jiuduansha shoals, Shanghai, China. Ecol. Eng. 29, 164–172. doi: 10.1016/j.ecoleng.2006.06.005
Jackson, E. L., Rowden, A. A., Attrill, M. J., Bossey, S. J., and Jones, M. B. (2001). The importance of seagrass beds as a habitat for fishery species. Oceanogr. Mar. Biol. 39, 269–303. doi: 10.1016/S0029-8018(99)00063-3
Jiang, C., Pan, S., and Chen, S. (2017). Recent morphological changes of the Yellow River (Huanghe) submerged delta: causes and environmental implications. Geomorphology 293, 93–107. doi: 10.1016/j.geomorph.2017.04.036
Kirwan, M. L., and Megonigal, J. P. (2013). Tidal wetland stability in the face of human impacts and sea-level rise. Nature 504, 53–60. doi: 10.1038/nature12856
Lee, S., Ma, S., Lim, Y., Choi, H. K., and Shin, H. (2004). Genetic diversity and its implications in the conservation of endangered Zostera japonica in Korea. J. Plant Biol. 47, 275–281. doi: 10.1007/bf03030519
Lin, H. Y., Sun, T., Adams, M. P., Zhou, Y., Zhang, X. M., Xu, S. C., et al. (2018). Seasonal dynamics of trace elements in sediment and seagrass tissues in the largest Zostera japonica habitat, the Yellow River Estuary, northern China. Mar. Pollut. Bull. 134, 5–13. doi: 10.1016/j.marpolbul.2018.02.043
Liu, C., Jiang, H., Zhang, S., Li, C., Hou, Y., and Qian, F. (2013). Multi-scale analysis to uncover habitat use of red-crowned cranes: implications for conservation. Curr. Zool. 59, 604–617. doi: 10.1093/czoolo/59.5.604
Liu, W., Chen, X., Strong, D. R., Pennings, S. C., Kirwan, M. L., Chen, X., et al. (2020a). Climate and geographic adaptation drive latitudinal clines in biomass of a widespread saltmarsh plant in its native and introduced ranges. Limnol. Oceanogr. 65, 1399–1409. doi: 10.1002/lno.11395
Liu, W., Zhang, Y., Chen, X., Maung-Douglass, K., Strong, D. R., and Pennings, S. C. (2020b). Contrasting plant adaptation strategies to latitude in the native and invasive range of Spartina alterniflora. New Phytol. 226, 623–634. doi: 10.1111/nph.16371
Liu, X. J., Zhou, Y., Yang, H. S., and Ru, S. G. (2013). Eelgrass detritus as a food source for the sea cucumber Apostichopus japonicus Selenka (Echinidermata: Holothuroidea) in coastal waters of North China: an experimental study in flow-through systems. PLoS One 8:e58293. doi: 10.1371/journal.pone.0058293
Lowe, S., Browne, M., Boudjelas, S., and De Poorter, M. (2000). 100 of the World’s Worst Invasive Alien Species a Selection From The Global Invasive Species Database. Gland: IUCN.
Ma, X., Yan, J., Wang, F., Qiu, D., Jiang, X., Liu, Z., et al. (2019). Trait and density responses of Spartina alterniflora to inundation in the Yellow River Delta, China. Mar. Pollut. Bull. 146, 857–864. doi: 10.1016/j.marpolbul.2019.07.022
Mack, R. N., Simberloff, D., Lonsdale, W. M., Evans, H., Clout, M., and Bazzaz, F. A. (2000). Biotic invasions: causes, epidemiology, global consequences, and control. Ecol. Appl. 10, 689–710. doi: 10.2307/2641039
Maricle, B. R., and Lee, R. W. (2002). Aerenchyma development and oxygen transport in the estuarine cordgrasses Spartina alterniflora and S.anglica. Aquat. Bot. 74, 109–120. doi: 10.1016/s0304-3770(02)00051-7
Meng, W., Feagin, R. A., Innocenti, R. A., Hu, B., He, M., and Li, H. (2020). Invasion and ecological effects of exotic smooth cordgrass Spartina alterniflora in China. Ecol. Eng. 143:105670. doi: 10.1016/j.ecoleng.2019.105670
Miki, S. (1933). On the Sea-Grasses in Japan: 1. Zostera and Phyllospadix, With Special Reference to Morphological and Ecological Characters. Tokyo: The Botanical Magazine.
National marine environmental monitoring center [NMEMC] (2007). The Specification for Marine Monitoring – Part 5: Sediment Analysis (GB 17378.5 - 2007). Beijing: National marine environmental monitoring center.
Nordlund, L. M., Jackson, E. L., Nakaoka, M., Samper-Villarreal, J., Beca-Carretero, P., and Creed, J. C. (2018). Seagrass ecosystem services – what’s next? Mar. Pollut. Bull. 134, 145–151. doi: 10.1016/j.marpolbul.2017.09.014
Orth, R. J., Carruthers, T. J. B., Dennison, W. C., Duarte, C. M., Fourqurean, J. W., Heck, K. L., et al. (2006). A global crisis for seagrass ecosystems. Bioscience 56, 987–996. doi: 10.1641/0006-3568200656[987:agcfse]2.0.co;2
Prentice, C., Poppe, K. L., Lutz, M., Murray, E., Stephens, T. A., Spooner, A., et al. (2020). A synthesis of blue carbon stocks, sources, and accumulation rates in eelgrass (Zostera marina) meadows in the Northeast Pacific. Glob. Biogeochem. Cycle 34:e2019GB006345. doi: 10.1029/2019gb006345
Qin, P., Xie, M., and Jiang, Y. S. (1998). Spartina green food ecological engineering. Ecol. Eng. 11, 147–156. doi: 10.1016/s0925-8574(98)00030-5
Ralph, P. J., Tomasko, D., Moore, K., Seddon, S., and Macinnis-Ng, C. M. O. (2006). “Human impacts on seagrasses: eutrophication, sedimentation, and contamination,” in Seagrasses: Biology, Ecology and Conservation, eds A. W. D. Larkum, R. J. Orth, and C. M. Duarte (Dordrecht: Springer), 567–593.
Ren, G.-B., Wang, J.-J., Wang, A.-D., Wang, J.-B., Zhu, Y.-L., Wu, P.-Q., et al. (2019). Monitoring the invasion of smooth cordgrass Spartina alterniflora within the modern Yellow River Delta using remote sensing. J. Coast. Res. 90:135. doi: 10.2112/si90-017.1
Salinas, C., Duarte, C. M., Lavery, P. S., Masque, P., Arias-Ortiz, A., Leon, J. X., et al. (2020). Seagrass losses since mid-20th century fuelled CO2 emissions from soil carbon stocks. Glob. Change Biol. 26, 4772–4784. doi: 10.1111/gcb.15204
Short, F. T., and Coles, R. G. (2001). Global Seagrass Research Methods. Amsterdam: Elsevier Science Press.
Simenstad, C., and Thom, R. (1995). Spartina alterniflora (smooth cordgrass) as an invasive halophyte in Pacific northwest estuaries. Hortus Northwest 6, 9–13.
Syvitski, J. P., Kettner, A. J., Overeem, I., Hutton, E. W., Hannon, M. T., Brakenridge, G. R., et al. (2009). Sinking deltas due to human activities. Nat. Geosci. 2, 681–686. doi: 10.1038/ngeo629
Unsworth, R. K. F., Nordlund, L. M., and Cullen-Unsworth, L. C. (2019). Seagrass meadows support global fisheries production. Conserv. Lett. 12:e12566. doi: 10.1111/conl.12566
Wang, H., Yang, Z., Saito, Y., Liu, J. P., Sun, X., and Wang, Y. (2007). Stepwise decreases of the Huanghe (Yellow River) sediment load (1950–2005): impacts of climate change and human activities. Glob. Planet Change 57, 331–354. doi: 10.1016/j.gloplacha.2007.01.003
Wang, W., Sardans, J., Wang, C., Zeng, C., Tong, C., Chen, G., et al. (2019). The response of stocks of C, N, and P to plant invasion in the coastal wetlands of China. Glob. Change Biol. 25, 733–743. doi: 10.1111/gcb.14491
Wang, X. Y., Shen, D. W., Jiao, J., Xu, N. N., Yu, S., Zhou, X. F., et al. (2012). Genotypic diversity enhances invasive ability of Spartina alterniflora. Mol. Ecol. 21, 2542–2551. doi: 10.1111/j.1365-294X.2012.05531.x
Waycott, M., Duarte, C. M., Carruthers, T. J. B., Orth, R. J., Dennison, W. C., Olyarnik, S., et al. (2009). Accelerating loss of seagrasses across the globe threatens coastal ecosystems. Proc. Natl. Acad. Sci. U.S.A. 106, 12377–12381. doi: 10.1073/pnas.0905620106
Xia, L., Yang, W., Zhao, H., Xiao, Y., Qing, H., Zhou, C., et al. (2015). High soil sulfur promotes invasion of exotic Spartina alterniflora into native Phragmites australis marsh. Clean Soil Air Water 43, 1666–1671. doi: 10.1002/clen.201300883
Yang, W., Zhao, H., Chen, X., Yin, S., Cheng, X., and An, S. (2013). Consequences of short-term C4 plant Spartina alterniflora invasions for soil organic carbon dynamics in a coastal wetland of Eastern China. Ecol. Eng. 61, 50–57. doi: 10.1016/j.ecoleng.2013.09.056
Yue, S. D., Zhang, X., Xu, S., Zhang, Y., Zhao, P., Wang, X., et al. (2020). Reproductive strategies of the seagrass Zostera japonica under different geographic conditions in northern China. Front. Mar. Sci. 7:574790. doi: 10.3389/fmars.2020.574790
Zhang, R. S., Shen, Y. M., Lu, L. Y., Yan, S. G., Wang, Y. H., Li, J. L., et al. (2004). Formation of Spartina alterniflora salt marshes on the coast of Jiangsu Province, China. Ecol. Eng. 23, 95–105. doi:10.1016/j.ecoleng.2004. 07.007
Zhang, X. M., Lin, H. Y., Song, X. Y., Xu, S. C., Yue, S. D., Gu, R. T., et al. (2019). A unique meadow of the marine angiosperm Zostera japonica, covering a large area in the turbid intertidal Yellow River Delta. China Sci. Total Environ. 686, 118–130. doi: 10.1016/j.scitotenv.2019.05.320
Zhang, X. M., Zhou, Y., Adams, M. P., Wang, F., Xu, S. C., Wang, P. M., et al. (2020a). Plant morphology and seed germination responses of seagrass (Zostera japonica) to water depth and light availability in Ailian Bay, northern China. Mar. Environ. Res. 162:105082. doi: 10.1016/j.marenvres.2020.105082
Zhang, X. M., Zhou, Y., Liu, P., Wang, F., Liu, B. J., Liu, X. J., et al. (2014). Temporal pattern in the bloom-forming macroalgae Chaetomorpha linum and Ulva pertusa in seagrass beds, Swan Lake lagoon, North China. Mar. Pollut. Bull. 89, 229–238. doi: 10.1016/j.marpolbul.2014.09.054
Zhang, X. M., Zhou, Y., Liu, P., Wang, F., Liu, B. J., Liu, X. J., et al. (2015). Temporal pattern in biometrics and nutrient stoichiometry of the intertidal seagrass Zostera japonica and its adaptation to air exposure in a temperate marine lagoon (China): implications for restoration and management. Mar. Pollut. Bull. 94, 103–113. doi:10.1016/j.marpolbul.2015.03.004
Zhang, X. M., Zhou, Y., Xu, S. C., Wang, P. M., Zhao, P., Yue, S. D., et al. (2020b). Differences in reproductive effort and sexual recruitment of the seagrass Zostera japonica between two geographic populations in northern China. Mar. Ecol. Prog. Ser. 638, 65–81. doi: 10.3354/meps13248
Zhang, Y., Pennings, S. C., Li, B., and Wu, J. (2019). Biotic homogenization of wetland nematode communities by exotic Spartina alterniflora in China. Ecology 100:e02596. doi: 10.1002/ecy.2596
Keywords: biological invasion, anthropogenic introduction, Spartina alterniflora, seagrass, Zostera japonica, intertidal zone
Citation: Yue SD, Zhou Y, Xu SC, Zhang XM, Liu MJ, Qiao YL, Gu RT, Xu S and Zhang Y (2021) Can the Non-native Salt Marsh Halophyte Spartina alterniflora Threaten Native Seagrass (Zostera japonica) Habitats? A Case Study in the Yellow River Delta, China. Front. Plant Sci. 12:643425. doi: 10.3389/fpls.2021.643425
Received: 18 December 2020; Accepted: 06 April 2021;
Published: 20 May 2021.
Edited by:
Eric Marechal, UMR 5168 Laboratoire de Physiologie Cellulaire Vegetale (LPCV), FranceReviewed by:
Mirta Teichberg, Leibniz Centre for Tropical Marine Research (LG), GermanyCopyright © 2021 Yue, Zhou, Xu, Zhang, Liu, Qiao, Gu, Xu and Zhang. This is an open-access article distributed under the terms of the Creative Commons Attribution License (CC BY). The use, distribution or reproduction in other forums is permitted, provided the original author(s) and the copyright owner(s) are credited and that the original publication in this journal is cited, in accordance with accepted academic practice. No use, distribution or reproduction is permitted which does not comply with these terms.
*Correspondence: Yi Zhou, eWl6aG91QHFkaW8uYWMuY24=
Disclaimer: All claims expressed in this article are solely those of the authors and do not necessarily represent those of their affiliated organizations, or those of the publisher, the editors and the reviewers. Any product that may be evaluated in this article or claim that may be made by its manufacturer is not guaranteed or endorsed by the publisher.
Research integrity at Frontiers
Learn more about the work of our research integrity team to safeguard the quality of each article we publish.