- 1College of Horticulture and Landscape Architecture, Southwest University, Chongqing, China
- 2Department of Botany, Bharathidasan University, Tiruchirappalli, India
- 3Aditya Degree and P.G. College, Kakinada, India
- 4Department of Biotechnology, Bharathidasan University, Tiruchirappalli, India
- 5China-USA Citrus Huanglongbing Joint Laboratory, National Navel Orange Engineering Research Center, Gannan Normal University, Ganzhou, China
- 6ICMR-National Institute of Nutrition, Hyderabad, India
- 7Department of Environmental Biotechnology, Bharathidasan University, Tiruchirappalli, India
Artemisia vulgaris L. produces a wide range of valuable secondary metabolites. The aim of the present study is to determine the effects of various concentrations of farnesyl diphosphate (FDP) on β-caryophyllene content in both callus and hairy root (HR) cultures regeneration from leaf explants of A. vulgaris L. Murashige and Skoog (MS) medium supplemented with various concentrations of 2,4-dichlorophenoxyacetic acid (2,4D; 4–13 μM), α-naphthaleneacetic acid (NAA; 5–16 μM), and FDP (1 and 3 μM) was used for callus induction and HR regeneration from leaf explants of A. vulgaris L. In this study, precursor-treated (2,4D 13.5 μM + FDP 3 μM) callus displayed the highest biomass fresh weight (FW)/dry weight (DW): 46/25 g, followed by NAA 10.7 μM + FDP 3 μM with FW/DW: 50/28 g. Two different Agrobacterium rhizogenes strains (A4 and R1000) were evaluated for HR induction. The biomass of HRs induced using half-strength MS + B5 vitamins with 3 μM FDP was FW/DW: 40/20 g and FW/DW: 41/19 g, respectively. To determine β-caryophyllene accumulation, we have isolated the essential oil from FDP-treated calli and HRs and quantified β-caryophyllene using gas chromatography–mass spectrometry (GC–MS). The highest production of β-caryophyllene was noticed in HR cultures induced using A4 and R1000 strains on half-strength MS medium containing 3 μM FDP, which produced 2.92 and 2.80 mg/ml β-caryophyllene, respectively. The optimized protocol can be used commercially by scaling up the production of a β-caryophyllene compound in a short span of time.
Introduction
Artemisia vulgaris L. is an important medicinal herb that belongs to the family Asteraceae. These plants are found in several temperate countries in Europe, Asia, Northern Africa, Alaska, and North America. A. vulgaris L. has been widely used as a traditional medicine for treating a number of diseases, such as neonatal jaundice (Fok, 2002), gastric ulcers (Repetto and Llesuy, 2002), and hepatitis, as well as an analgesic agent in acupuncture therapy (Yoshikawa et al., 1996). Traditionally, it has been effective as antiviral (Tan et al., 1999), insect-repellant, fumigant (Wang et al., 2006), insecticide (Chantraine et al., 1998), antibacterial, anti-inflammatory (Tigno et al., 2000), sedative, and flavoring and perfumery agents (Da Silva, 2004). A phytochemical study has identified more than 20 flavonoids in A. vulgaris L. extracts (Lee, 1998). Williams et al. (2012) have identified 22 significant chemical components in the essential oil of A. vulgaris L.—the major components being β-caryophyllene, α-zingiberene, borneol, and α-curcumin. It also has been found that some species of the plant kingdom produce sesquiterpene β-caryophyllene (De Moraes et al., 2001).
Caryophyllene is present in Zingiber nimmonii rhizome essential oil that helps curing fungal and bacterial infection (Sabulal et al., 2006). Gertsch et al. (2008) have reported about β-caryophyllene compound that was shown to interact with the binding site cannabinoid receptor type 2 (CB2), but not to type 1 (CB1). This chemical compound is energetically anti-inflammatory and has anesthetic properties. Amiel et al. (2012) reported that the essential oil (β-caryophyllene) of Commiphora gileadensis (Balm of Gilead, mentioned in the Bible) has an anti-proliferative property against tumor cell lines. They also found that β-caryophyllene caused a strong induction of apoptosis accompanied by DNA ladder formation and caspase-3 catalytic activity in tumor cell lines. However, the plant sesquiterpenes biosynthesis pathway is considered as an isopentenyl diphosphate (IPP), which is the universal biological precursor of all isoprenoids (basic C5 isoprene unit). It can be obtained through either mevalonate (MVA) or 2-methylerythritol 4-phosphate (MEP) pathways (Figure 1). Moreover, it has been well established that the acetyl-coenzyme A (acetyl-CoA) pathway in plants is located in the cytosol, whereas the MEP pathway is targeted to plastids inside (Croteau et al., 2000). There are studies that indicate cross-talk and possible IPP exchange between the other two paths through the cytosol and plastids (Adam and Zapp, 1998; Laule et al., 2003). There were also two independent pathways leading to IPP: the mevalonate pathway (MVA) originating from acetyl-CoA and the pyruvate-derived mevalonate-independent pathway (MEP) (Croteau et al., 2000). IPP instead contributes to the other terpenoids with its isomer dimethylallyl pyrophosphate (DMAPP). The MVA isoprenoid pathway is used by eukaryotes apart from plants, although prokaryotes with many exemptions use the MEP pathway to create IPP and DMAPP separately (Lichtenthaler, 1999; Boucher and Doolittle, 2000). Isopentenyl phosphate kinases (IPKs) generated by plant genomes are derived isopentenyl phosphate (IP) via ATP-dependent phosphorylation, forming the primary metabolite IPP commonly used for isoprenoid/terpenoid biosynthesis (Henry et al., 2018). The objective of the present study is to enhance β-caryophyllene content in in vitro callus and hairy root (HR) cultures and to isolate the essential oil from both calli and HRs in order to understand the metabolic profile of β-caryophyllene using gas chromatography–mass spectrometry (GC–MS).
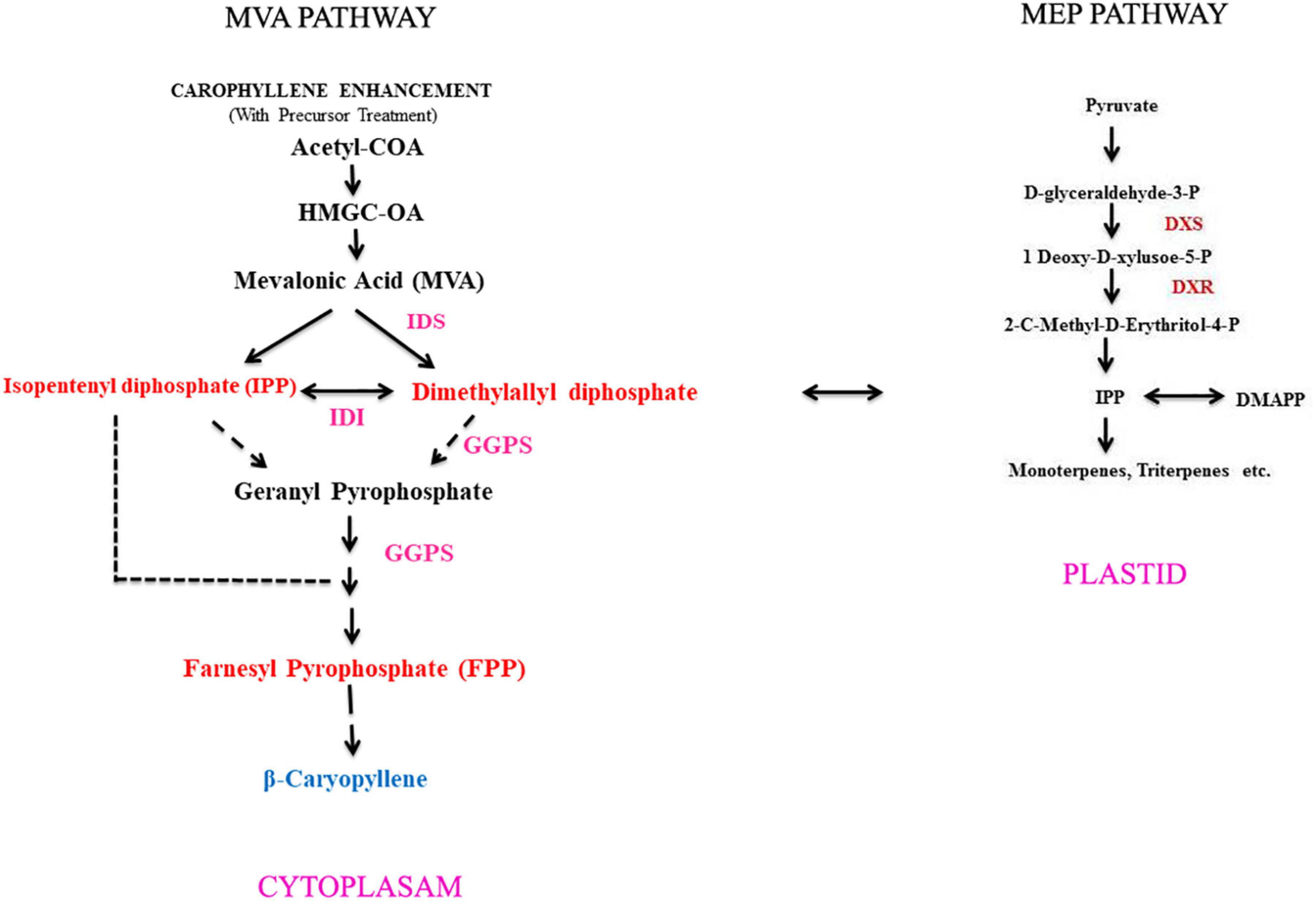
Figure 1. An outline for β-caryophyllene compound biosynthesis pathway operating through in vitro method of A. vulgaris plant. IDS, isopentenyl diphosphate synthase; IDI, isopentenyl diphosphate isomerase; GGPP, geranylgeranyl diphosphate; DXS, 1-deoxy-D-xylulose-5-phosphate synthase; DXR, 1-deoxy-D-xylulose-5-phosphate reductoisomerase.
Materials and Methods
Callus Induction
Seeds of A. vulgaris L. were collected from Johnny’s Selected Seeds, Winslow, ME, United States. The botanical identity of A. vulgaris was confirmed by comparing them with respect to the reference standards at Johnny’s Selected Seeds. After that, 35-day leaf explants excised from old seedlings were inoculated into Murashige and Skoog (MS) medium (Murashige and Skoog, 1962) supplemented with 2,4-dichlorophenoxyacetic acid (2,4D) and α-naphthaleneacetic acid (NAA) (Sigma-Aldrich, Inc., St. Louis, MO, United States) for callus induction. Callus was initiated by inoculating leaf explants from a 35-day-old plant into MS medium supplemented with various concentrations and combinations of 2,4D (4.5–13 μM) and NAA (5–16 μM), with farnesyl diphosphate (FDP; 1 and 3 μM) (Sigma-Aldrich, Inc., St. Louis, MO, United States) as a precursor in the experiment, followed by a previous report by Sundararajan and Kumari (2015). Fresh weight (FW) and dry weight (DW) were recorded in precursor-treated callus after 60 days (Sivanandhan et al., 2014).
HR Culture
Bacterial Culture Preparation
From the overnight glycerol stock, 500 μl was taken and transferred to the Luria–Bertani (LB) medium, and the same was subcultured thrice to obtain active culture. The Gram-negative soil bacteria Agrobacterium rhizogenes (A4 and R1000) transferred to the medium were cultured overnight at 25°C in an orbital shaker at 180 rpm. The bacterial cells were harvested by centrifugation at 5,000 rpm for 15 min at 25°C. The pellet-containing bacterial cells were re-suspended in liquid MS medium, and the final optical density (OD) was adjusted to 0.6.
Induction and Establishment of HR Culture
In aseptic condition, leaf explants derived from 45-day-old plants were wounded with a sterile scalpel, transferred into the A. rhizogenes infection medium, and incubated for 10 min at 25°C. After infection, the explants were dried on a sterile filter paper and placed on semisolid 1/2 MS medium supplemented with 3% sucrose and supplemented separately with 50, 100, and 150 μM of acetosyringone (AS; Sigma, United States). AS was filter-sterilized by using a 0.2-μm syringe filter 109 (Pall Corp., United States) and added to the cooled autoclaved medium. After cocultivation, explants were washed with liquid 1/2 MS containing 500 mg/L cefotaxime, followed by sterile distilled water. The explants were then inoculated on 1/2 MS medium supplemented with 500 mg/L cefotaxime and kept under dark condition for HR induction. HRs of more than 1–2 cm length were excised from the explants and transferred to 30 ml 1/2 MS, 1/2 MS + B5 vitamins, 1/2 MS + FDP 1 μM, 1/2 MS + FDP 3 μM, 1/2 MS + B5 vitamins + FDP 1 μM, and 1/2 MS + B5 vitamins + FDP 3 μM supplemented with 3% sucrose with 500 mg/L cefotaxime until the residual bacteria are killed completely. The experiment was run thrice with 25 explants for each experiment.
Determination of the Growth Index of the HR Cultures
Experiments were conducted to evaluate the growth rate of the hair roots grown in 1/2 MS, 1/2 MS + B5 vitamins, 1/2 MS + FDP 1 μM, 1/2 MS + FDP 3 μM, 1/2 MS + B5 vitamins + FDP 1 μM, and 1/2 MS + B5 vitamins + FDP 3 μM. The growth index (GI) of all the four lines was measured initially and inoculated with 20 mg of FW bacteria-free HRs (3 weeks old). It was transferred into a 30 ml liquid medium in a 250 ml conical flask and maintained on a rotary shaker at 120 rpm in a dark room for 12 weeks. The in vitro grown HRs were subcultured in a fresh medium for 6 weeks to stimulate further growth of the hairs. Each treatment consisted of three replicates, and each replicate contained 25 explants per treatment. The GI was determined by using the following formula (Ashraf et al., 2015):
FW and DW of HRs were recorded after 12 weeks, according to the method of Sivanandhan et al. (2014).
Polymerase Chain Reaction Analysis
Integration of the T-DNA, which is responsible for HR formation, was confirmed by polymerase chain reaction (PCR) analysis using rolB gene and rolC gene-specific primers (Cho et al., 1998). Genomic DNA was isolated from both the control non-transformed roots and the transformed HR cultures by using the cetyltrimethylammonium bromide (CTAB) method (Khanuja et al., 1999). The following primers were used for PCR analysis: for rolB gene, forward primers—5-ATG GAT CCC AAA TTG CTA TTC CCC CAC GA-3 and reverse primers—5-TTA GGC TTC TTT CAT TCG GTT TAC TGC AGC-3 and for rolC gene, forward primers—5-ATG GCT GAA GAC GAC CTG TGT T-3 and reverse primers—5-TTA GCC GAT TGC AAA CTT GCA C-3. The PCR was carried out by amplifying with an initial denaturation at 94°C for 5 min, followed by 35 cycles of denaturation at 94°C for 1 min, annealing at 55°C for 1 min rolB and rolC, and extension at 72°C for 1 min with a final extension of 72°C for 10 min using an Applied Biosystems Veriti Thermal Cycler (Thermo Fisher Scientific, United States). The amplified products were analyzed by electrophoresing 1.4% (w/v) of agarose gel along with 100 bp DNA marker (New England Biolabs, United Kingdom) staining with ethidium bromide.
Essential Oil Isolation and Quantification of β-Caryophyllene Content in Precursor-Treated Callus and HR by GC–MS Analysis
Isolation of Essential Oil
Calli and HRs (10 g each) were subjected to hydrodistillation for 4 h at 60°C using a Clevenger-type apparatus. The obtained essential oil was dried over anhydrous sodium sulfate (Na2SO4). The oil was then filtered and stored at 4°C for further experiments.
GC–MS Analysis
The extracted sample was cleaned by passing it through a silica gel column (glass column) containing glass wool, silica gel 60 (230–400 mesh; Merck, United States), and anhydrous Na2SO4, which helps to remove high-molecular-weight polar substances and impurities that interfere with GC–MS analysis. For quantitative analysis, β-caryophyllene standard and essential oil were dissolved with ethyl acetate and analyzed using a GC-MS-QP2010 (Shimadzu, Japan) equipped with an AOC-20i autosampler. One microliter of the essential oil was injected, and the chromatographic separation of β-caryophyllene was achieved with HP5-MS column (60 m × 0.25 mm I.D. × 0.25 mm thickness; Restek, United States). Helium with a purity of 99.999% was utilized as the carrier gas at a flow rate of 10 ml/min.
The injector port, interface, and ion source temperatures were set at 260, 300, and 230°C, respectively. The programmed oven temperature was as follows: the initial temperature was set at 120°C for 1 min, then raised to 270°C at 6°C/min and finally to 320°C at 7°C/min, and held for 10 min. A solvent delay of 6 min was set to protect the filament from oxidation. The mass spectrometer was operated in electron impact (EI) mode at an ionization potential of 70 eV and at an emission current up to 60 mA. The quantification was performed in selected ion monitoring (SIM) mode by monitoring mass ions for each β-caryophyllene. For quantification, four different calibrators (0.125, 0.250, 0.500, and 1.00 mg/ml) were used for linearity check. It showed good correlation coefficient (r2 = 0.9999). Scanning interval and SIM sampling rate were 0.5 and 0.2 s, respectively.
Statistical Analysis
The evaluation of β-caryophyllene from various callus and HR samples of A. vulgaris L. leaf explants was performed with three replicates each. The data obtained were analyzed statistically, and observations were recorded on the frequency of both FW and DW (callus and HR cultures, respectively), as well as the number of explants responding for HR initiation growth rate. The statistical analyses were performed by ANOVA using SPSS. The differences between means were tested for significance by Duncan’s multiple range test at p = 0.05.
Results and Discussion
Callus Induction
Leaf explants were inoculated into MS medium containing 2,4D (4–13 μM) and NAA (5–16 μM) with FDP as a precursor (1 and 3 μM) individually, as per a previous study by Sundararajan and Kumari (2015). Among various concentrations tested, the highest response of callus (99 and 95.2%) was achieved in MS medium supplemented with a combination of 2,4D (9.0 μM) + FDP (3 μm), followed by MS medium supplemented with NAA (16.1 μM) + FDP (3 μM). There are various similar studies on callus induction from leaf and stem explants of A. vulgaris L. (Sujatha and Kumari, 2007; Borzabad et al., 2010a, b). The precursor- and elicitor-treated calli vary from species to species and often differ even within the same species, for example, Jatropha integerrima (Schmitt and Petersen, 2002). MS medium supplemented with 5-aminolevulinic acid (5-ALA) as a precursor 2, 5, and 10 mg/L concentration has shown an enhancement in the callus biomass and induction of rooting and shooting with a profound effect (Boitel-Conti et al., 2000). Sujatha and Dhingra (1993) reported that feeding the yeast extract elicitor in the suspension culture of J. integerrima promoted the metabolic enzyme activity and alkaloid production several folds. Other studies also reported enhanced biosynthesis of the flavonolignan silymarin compound through jasmonic acid (JA) elicitor treatment of Silybum marianum callus cell culture medium (Sanchez-Sampedro et al., 2005). In recent reports, three key genes, such as phenylalanine ammonia-lyase, chalcone synthase, and dihydroflavonol-4-reductase, were reported to be involved in the phenylpropanoid pathway. A polyphenolic compound has been used in Plantago ovata callus culture media with different growth regulators (Talukder et al., 2016). Arya and Patni (2013) reported significant enhancement of quercetin in callus culture of Pluchea lanceolata by incorporation of cinnamic acid and precursor feeding with L-phenylalanine. In the present study, FW/DW of callus 2,4D treated without precursor was observed at FW/DW: 36/22 g, whereas in precursor-treated callus the highest FW/DW: 48/28 g were noticed at the concentrations (2,4D 13.5 μM + FDP 3 μM) followed by (2,4D 9 μM + FDP μM) fresh and dry weight was exhibited (Figure 2A). Then fresh and dry weight of NAA treated callus without precursor was found to be 36/20 g, respectively, whereas in precursor-treated callus the highest FW/DW: 48/26 g was noticed at the concentrations (NAA 16.1 μM + FDP 3 μM) followed by (NAA 10.7 μM + FDP 3 μM) fresh and dry weight was exhibited (Figure 2B). Similar reports were observed in connection with FW 500 mg withanolides production in callus culture of Withania somnifera after 4 weeks of culture (Doma et al., 2012; Sivanandhan et al., 2012). This study is in agreement with the result of a previous report on the large-scale production of artemisinin with different elicitors and precursors, such as sodium acetate, mevalonic acid, lactone, and casein acid hydrolysate, to increase artemisinin content in cell suspension culture of Artemisia annua (Baldi and Dixit, 2008). Quercetin compound was enhanced by using various precursors, such as phenylalanine and cinnamic acid, in P. lanceolata in vitro callus culture (Arya and Patni, 2013). Similar results were also observed in Psoralea corylifolia with precursor treatment in cotyledonary callus, which was obtained from FW 2,601.8 mg/g (Mohammadparast et al., 2015). A similar phenomenon was also observed with different synthetic precursors, such as phenobarbital, geraniol, trans-cinnamic acid, succinic acid, and tryptamine, in compact callus cluster (CCC) cultures of Catharanthus roseus with the highest biomass production of FW 10 g and DW 6 g (Zhao et al., 2001). Phenylethanoid glycosides were enhanced by feeding precursor into the Cistanche deserticola cell culture medium, with FW 0.6 g and DW 0.4 g (Ouyang et al., 2005). In earlier studies, the intracellular amount of oleanolic acid (OA) reached its final value (DW 0.84 mg/g) after 72 h of treatment with 100 M JA, which was 9.4-fold greater than that observed in untreated control cultures. After 48 h of therapy, the inclusion of chitosan at 50 mg/L induced a 5-fold increase in OA accumulation (DW 0.37 mg/g) (Wiktorowska et al., 2010). The precursor feeding or elicitor treatment is an effective technique to improve the growth of secondary metabolites through plant cell culture (Dedaldechamp and Uhel, 1999 and Rao and Ravishankar, 2002).
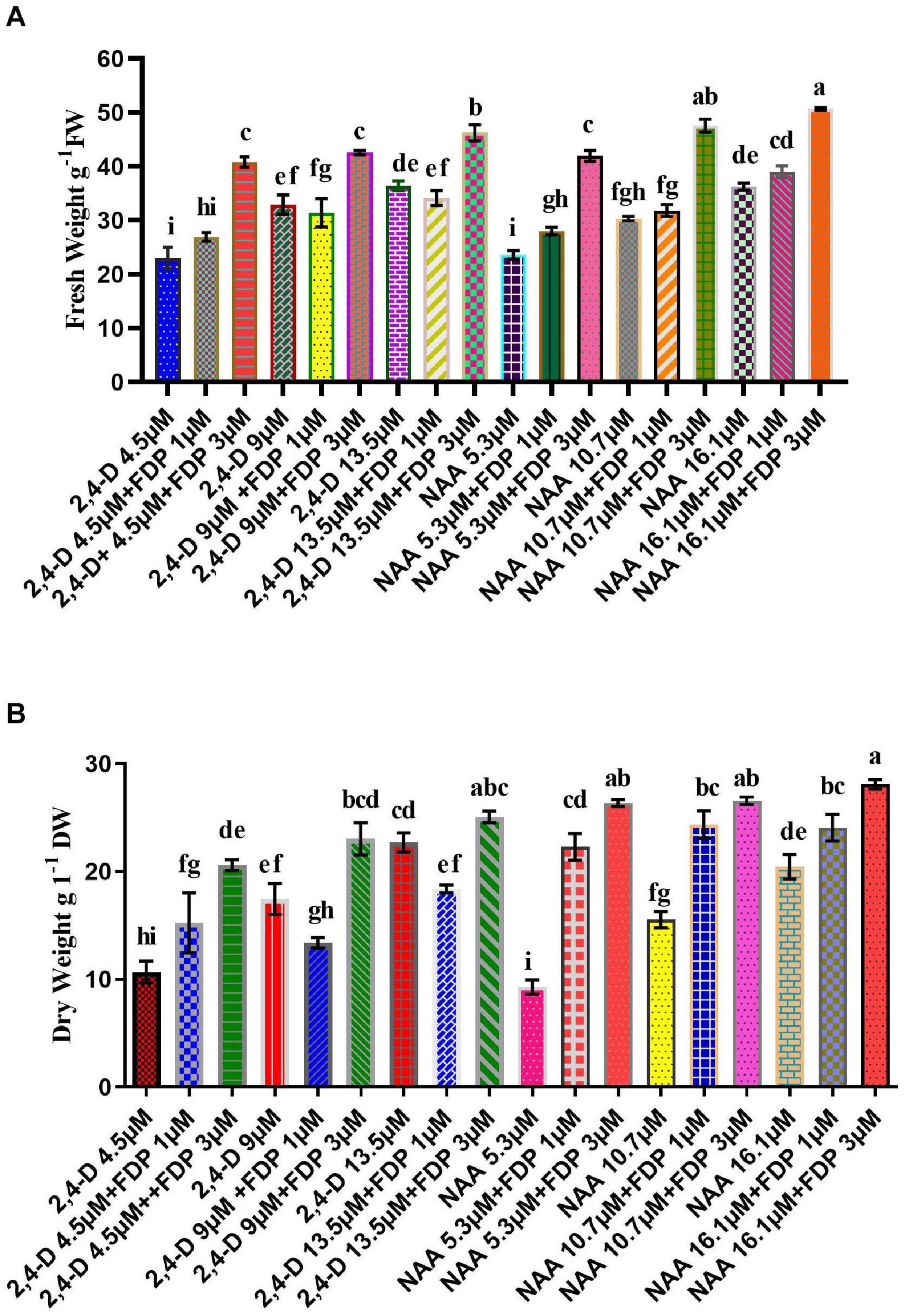
Figure 2. Productivity of precursor treatment of callus culture yield and biomass cultivation. (A) Various concentrations of 2,4D + FDP and NAA + FDP treatments of fresh weight of callus. (B) Various concentrations of 2,4D + FDP and NAA + FDP treatments of dry weight of callus.
HR Culture
Induction and Establishment of HR Culture
The transformation efficiency of A. rhizogenes required a different time and duration for the root induction from leaf explants of A. vulgaris L. (Table 1). The highest transformation frequency with a direct root induction from the wounding site was observed in leaf explants infected with AS 150 μM (A4 and R1000) strains after 10–15 days of culture (Figure 3). The results of the present study were similar to Persicaria minor HR induction treatments with AS at 100 and 200 μM, which suggested that phenolic compounds play a vital role in the induction medium, particularly at wounded sites (Danphitsanuparn et al., 2012; Ashraf et al., 2015). It was reported that AS stimulated A. rhizogenes T-DNA to bring out HR from the wounded cell tissue (Farshad Ashraf et al., 2013). HR induction by different bacterial strains has been documented earlier in different plant species (Zehra et al., 1999). In C. roseus, six different A. rhizogenes strains were used for HR induction (Toivonen et al., 1989). Strain induced HR with the help of Rauwolfia serpentina leaf explants with a transformation frequency of 70% for strain when compared with strain LBA 9402, with a frequency of 45% (Goel et al., 2010). Tao and Li (2006) reported HR induction in Torenia fournieri leaf explant with higher transformation efficiency at 65% than that in A4 strain. Wu et al. (2003) earlier reported that 100 μM of AS is the best concentration for enhancing the genetic transformation in Nicotiana tabacum var. Previous studies indicated that the A. rhizogenes strain, which influenced HR induction in various explant parts, was showing HR induction (Wu et al., 2003; Pietrosiuk et al., 2006; Thimmaraju et al., 2008). In the present study, exogenous application of AS along with naturally produced phenolics from wounded leaf explants increased the transformation frequency by vir-genes induction.
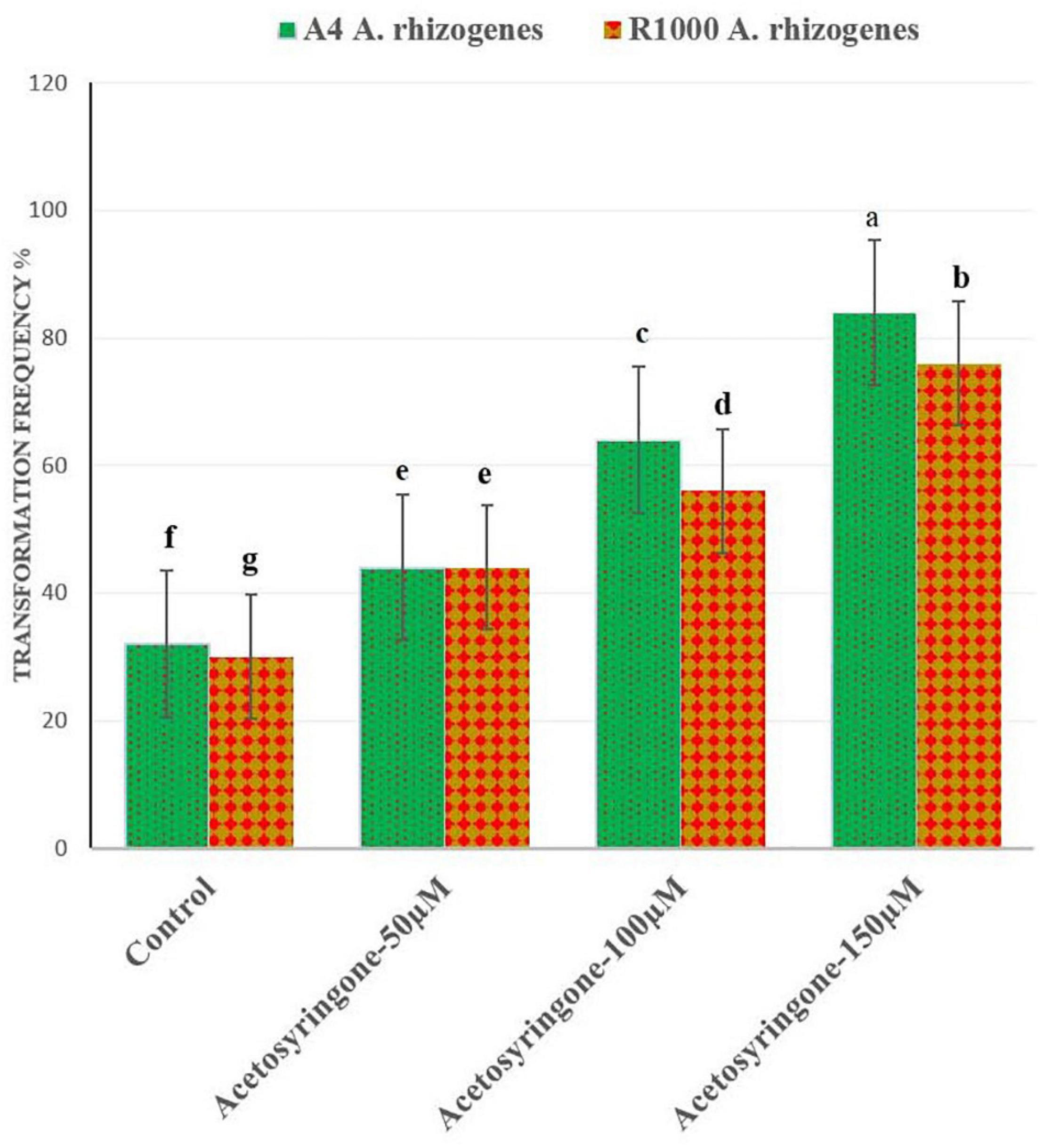
Figure 3. Effect of different concentrations of acetosyringone (AS) on A. rhizogenes (A4 and R1000 strains) transformed frequency (%) of hairy roots inductions in A. vulgaris.
Analysis of the GI of HRs
There are various compositions and types of culture medium known to influence the growth and production of HRs (Giri and Narasu, 2000). Among all the treatments tested (1/2 MS, 1/2 MS + B5 vitamins, 1/2 MS + FDP 1 μM, 1/2 MS +FDP 3 μM, 1/2 MS + B5 vitamins + FDP 1 μM, and 1/2 MS + B5 vitamins + FDP 3 μM), the HR culture attained a maximum and minimum of a 30-fold and a 65-fold increase of the initial weight of 200 mg (strain), and the HR culture attained a maximum and minimum of a 25-fold and a 55-fold increase in the initial weight of 163 mg (R1000) within 12 weeks on 1/2 MS + FDP 1 μM and 1/2 MS + FDP 3 μM, respectively. After 6 weeks, there was no significant difference in the GI% in another precursor-treated HRs. However, after 12 weeks of culture, GI% was greater in the GI of A. vulgaris L. HRs with a strain at 6 and 12 weeks in the rotary shaking system. At 6 weeks GI of A4 HR culture, GI% was found without precursor treatment in 1/2 MS (42.10%) and 1/2 MS + B5 vitamins (32.55%) and with precursor treatment in 1/2 MS + FDP 3 μM (70.85%) and 1/2 MS + B5 vitamins + FDP 3 μM (60.23%). After 12 weeks, the GI of A4 HR culture without precursor treatment was observed as 1/2 MS (27.33%) and 1/2 MS + B5 vitamins (30.33%), and with precursor treatment, it was observed with the highest response in 1/2 MS + FDP 3 μM (55.00%) and 1/2 MS + B5 vitamins + FDP 3 μM (77.33%), respectively (Figures 4, 5A, 6A,B). Figure 4 shows the GI of A. vulgaris L. by HRs at 6 and 12 weeks cultivation in the rotary shaking system. At 6 weeks, the GI of R1000 HR culture without precursor treatment was observed in 1/2 MS (22.11%) and 1/2 MS + B5 vitamins (27.44%), and with precursor treatment, it was observed in 1/2 MS + FDP 3 μM (45.11%) and 1/2 MS + B5 vitamins + FDP 3 μM (61.00%). After 12 weeks, the GI of R1000 HR culture without precursor treatment was observed in 1/2 MS (27.33%) and 1/2 MS + B5 vitamins (30.31%), and with precursor treatment, it was observed in 1/2 MS + FDP 3 μM (55.00%) and 1/2 MS + B5 vitamins + FDP 3 μM (77.33%) (Figures 5B, 6C,D). In an earlier report, A. vulgaris L. HR culture grown in different liquid culture media, such as B5, SH (Schenk and Hilderbrandt), MS, and 1/2 MS, after 30 days showed 3-fold increases in growth rate (Sujatha et al., 2013). Similarly, HRs grown in full-strength and half-strength MS media, respectively, showed 46- and 34-fold increased growth rates in C. roseus L. plant (Hanafy et al., 2016). In another study, A. rhizogenes MTCC 532 used in HR induction with the combination of 1/2 MS liquid medium without hormones showed GI with a 45-fold increase within 57 days of culture (Khatodia et al., 2013). In Gentiana scabra HR culture, a maximum 42-fold increase was achieved through B5 liquid media composition after 8 weeks of cultivation (Huang et al., 2014). The establishment of HR liquid cultures was observed in Rauvolfia micrantha (Sudha et al., 2003) and Gentiana macrophylla (Tiwari et al., 2007). Inconsistent with the above work, the present study noticed a higher growth rate index of HRs in A. vulgaris L. on the same B5 and 1/2 MS liquid medium compositions. The HRs grown in 1/2 MS + B5 vitamins + FDP 3 μM medium showed a higher biomass increase and were expressed in either FW or DW when compared with those grown in 1/2 MS, 1/2 MS + B5 vitamins, 1/2 MS +FDP 1 μM, 1/2 MS + B5 vitamins + FDP 1 μM, and 1/2 MS + FDP 3 μM. A4 strain HR production increased with FW and DW on different media, such as FW 1/2 MS (23 g), 1/2 MS + B5 vitamins (26 g), 1/2 MS + FDP 3 μM (39 g), and 1/2 MS + B5 vitamins + FDP 3 μM (40 g) and DW 1/2 MS (12 g), 1/2 MS + B5 vitamins (14 g), 1/2 MS + FDP 3 μM (17 g), and 1/2 MS + B5 vitamins + FDP 3 μM (20 g). The R1000 HRs showed high production with increased FW and DW on different media: FW 1/2 MS (21 g), 1/2 MS + B5 vitamins (22 g), 1/2 MS + FDP 3 μM (39 g), and 1/2 MS + B5 vitamins + FDP 3 μM (41 g) and DW 1/2 MS (10 g), 1/2 MS + B5 vitamins (11 g), 1/2 MS + FDP 3 μM (19 g), and 1/2 MS + B5 vitamins + FDP 3 μM (19 g) (Figure 7). The present findings are in agreement with the report of Huang et al. (2014) who stated that the use of HR cultures is an excellent alternative to harvesting natural in vitro grown plants to produce pharmaceuticals and important metabolites in less time with the highest quality. In Salvia officinalis L. HR culture with strain, the maximum accumulated DW was achieved with 45 mg for 50 days (Grzegorczyk et al., 2006). The largest biomass was enhanced with 1/2 MS medium of low sucrose concentration to increase the lateral branches. In agreement with the findings, sucrose has been reported to increase HR biomass in Artemisia absinthium (Nin et al., 1997) and Swertia chirata (Keil et al., 2000). Inoguchi et al. (2003) also reported that high-level growth rates of root were induced by using 1/2 MS salt liquid medium in Solidago altissima. In Callerya speciosa, the highest growth of HRs was in 1/2 MS medium 10.85 g of FW and 1.13 g of DW and B5 medium, followed by 7.50 g of FW and 0.63 g of DW (Yao et al., 2016). Perilla frutescens cells of 7-day-old cultures were exposed to a yeast elicitor at 0.5–5% (v/v) in 7 days. Anthocyanin content peaked around 10.2% DW with yeast elicitor at 1% (v/v). Simultaneously, the maximum production of OA and ursolic acid was 19 and 27 mg/L, respectively, and a 46 and 24% increase in cultures administered with a 2% (v/v) yeast elicitor (Wang et al., 2004).
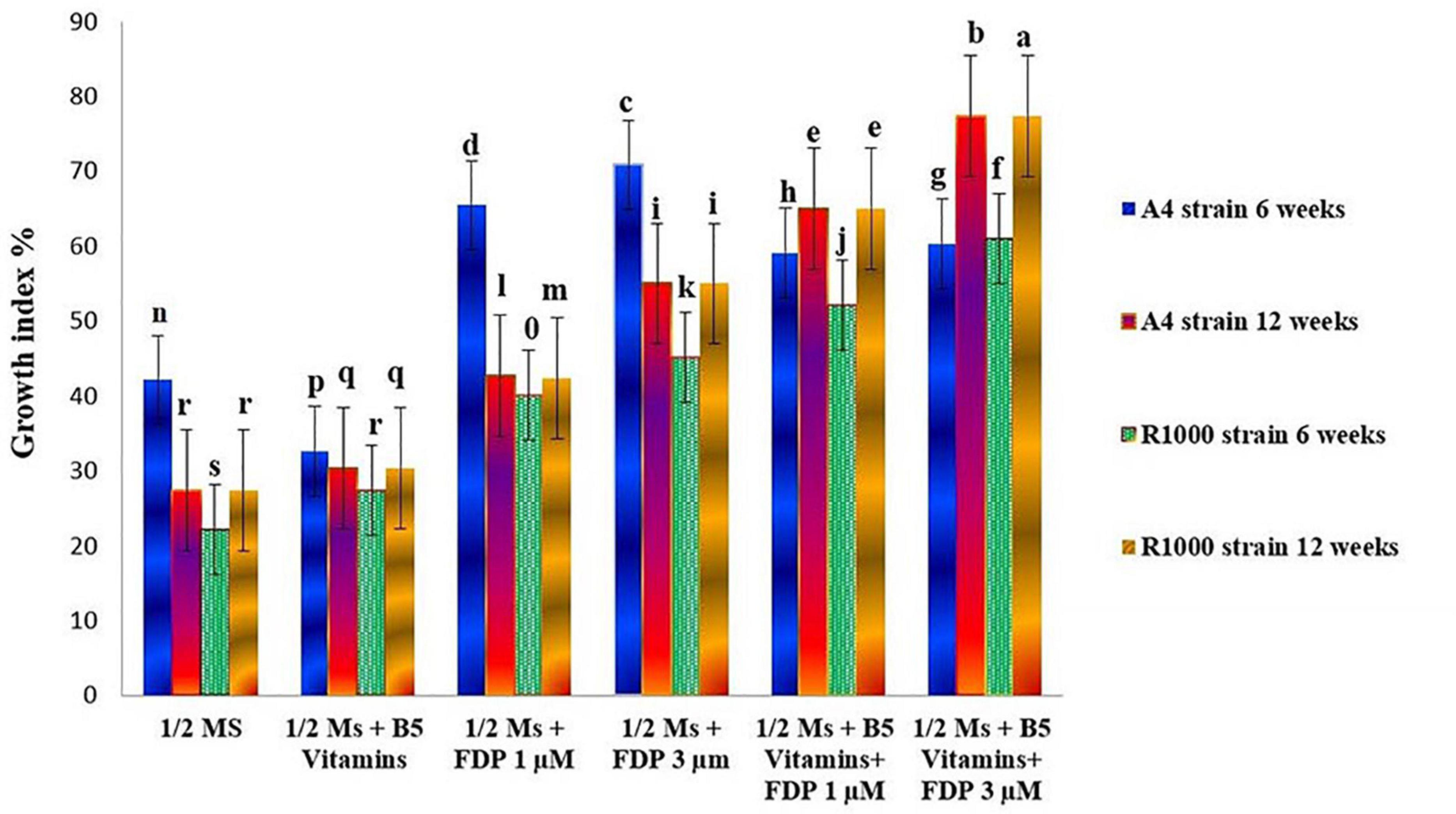
Figure 4. Effect on the mean growth index of A. vulgaris hairy root culture after 6 and 12 weeks (A4 and R1000 strains).
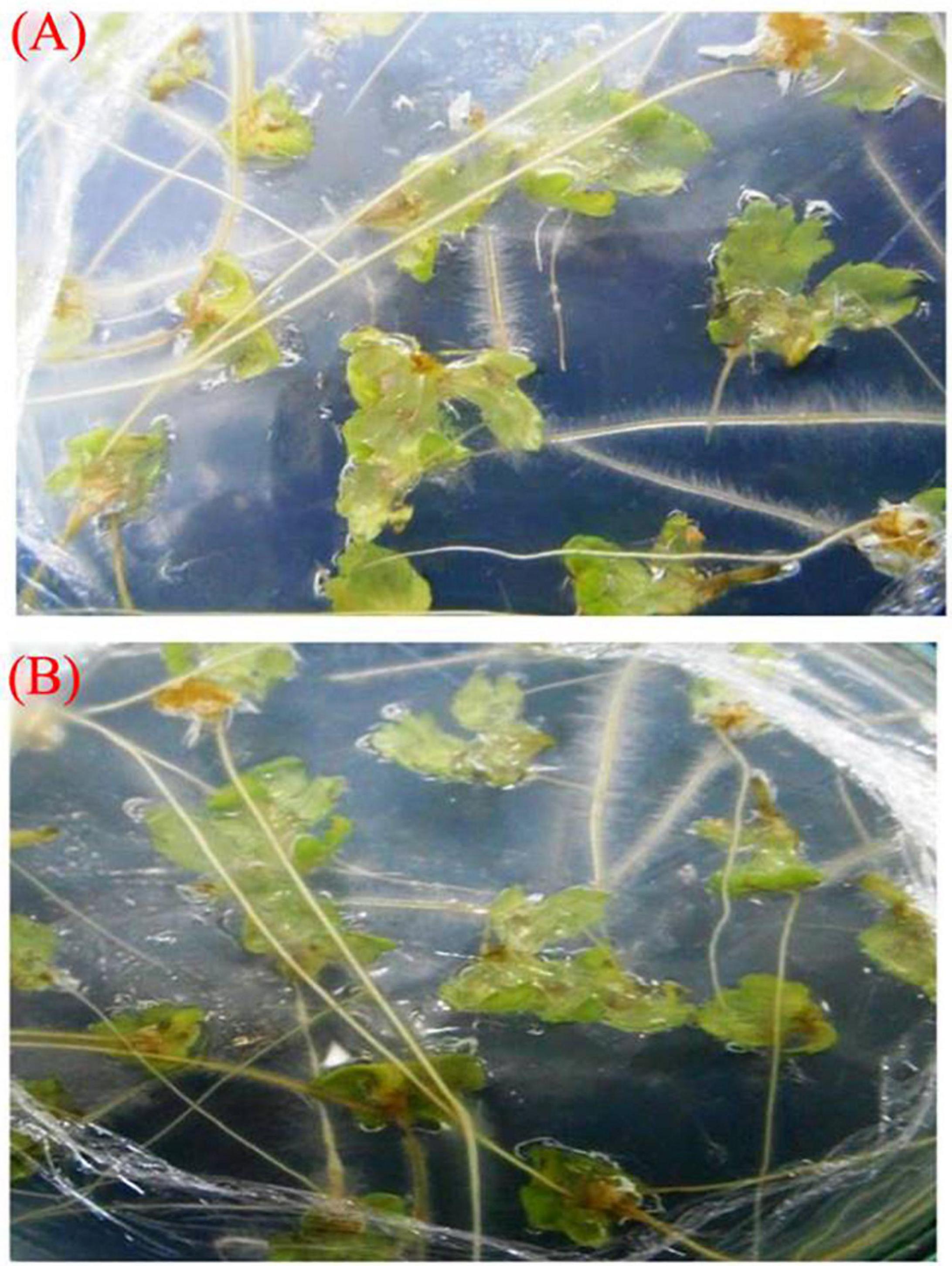
Figure 5. (A) After 2 weeks of coculture of A4 strain on hairy root initiation from leaf explants cultured on MS + 500 mg/L cefotaxime medium. (B) After 2 weeks of coculture of R1000 strain on hairy root initiation from leaf explants cultured on MS + 500 mg/L cefotaxime medium.
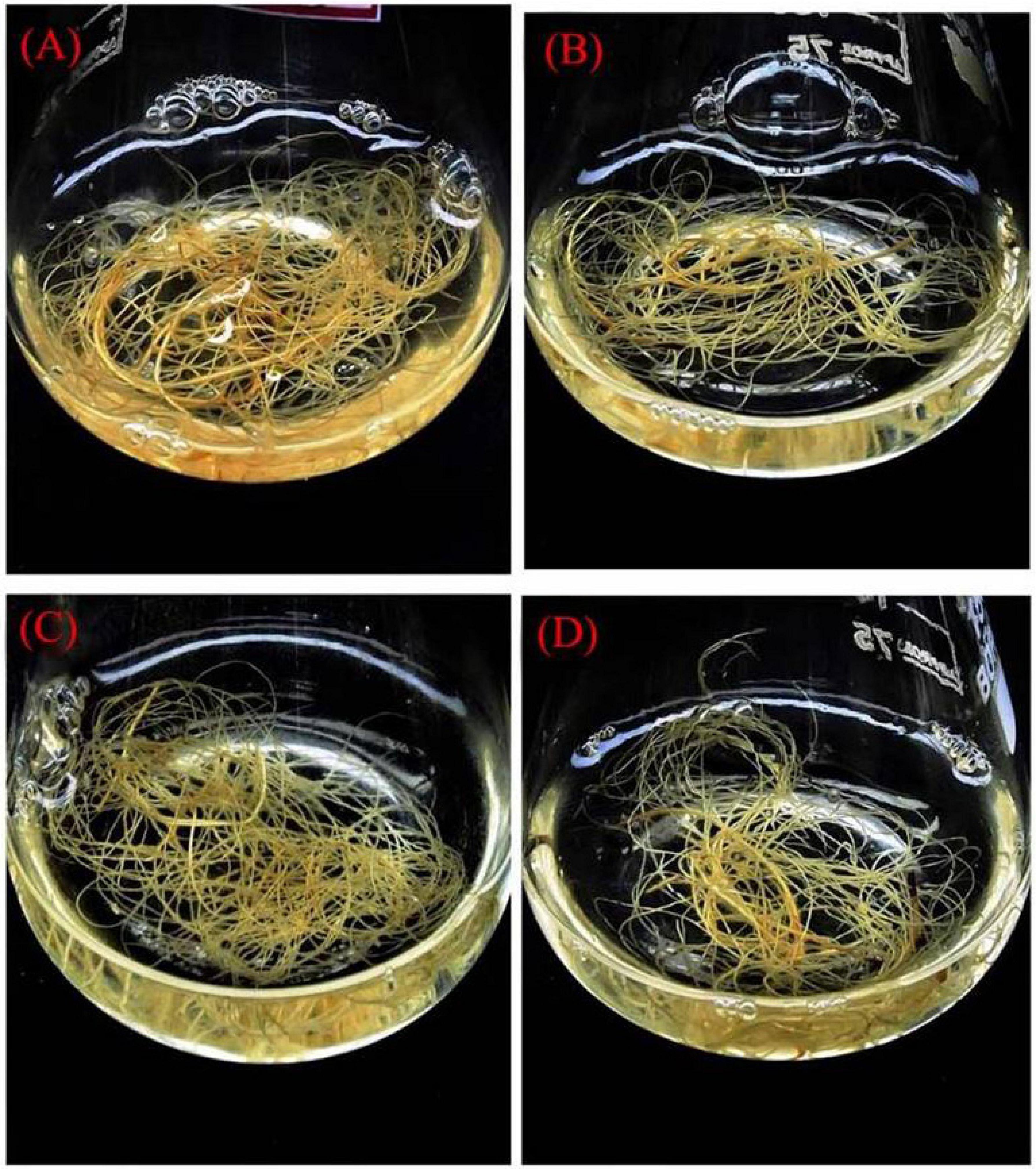
Figure 6. Enhancement of precursor treated with biomass accumulation from hairy root culture after 12 weeks. (A) A4 strain hairy root with 1/2 MS + FDP 3 μM. (B) A4 strain hairy root with 1/2 MS + FDP 1 μM. (C) R1000 strain hairy root with 1/2 MS + FDP 3 μM. (D) R1000 strain hairy root with 1/2 MS + FDP 1 μM.
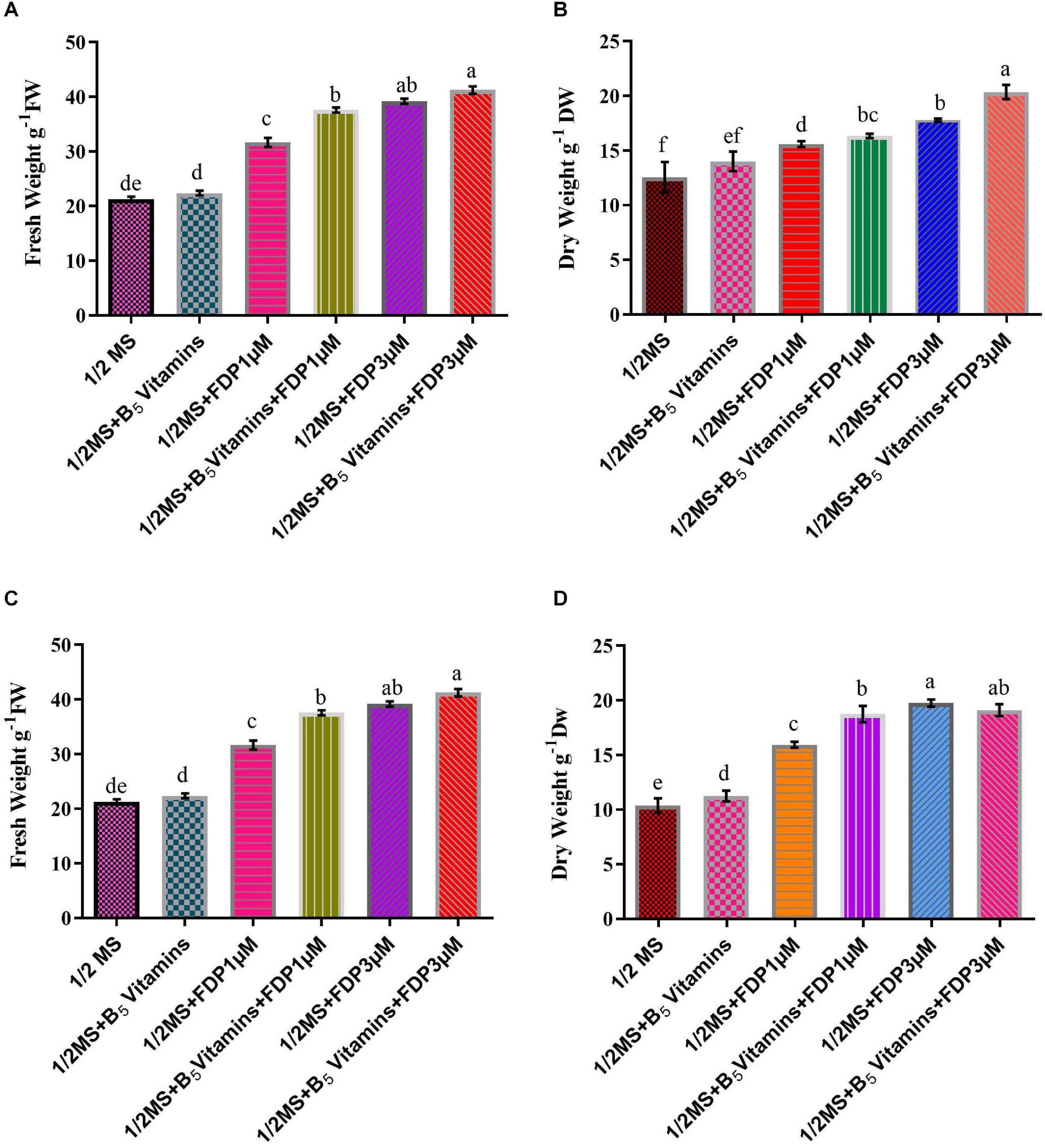
Figure 7. Productivity of precursor treatment of hairy root culture yield and biomass cultivation. (A) Various concentrations of A4 strain treatment of fresh weight of hairy root culture. (B) Various concentrations of A4 strain treatment of dry weight of hairy root culture. (C) Various concentrations of R1000 strain treatment of fresh weight of hairy root culture. (D) Various concentrations of R1000 strain treatment of dry weight of hairy root culture.
PCR Analysis
Molecular confirmation of the transformed HRs was achieved by PCR analysis. The presence of rolB gene fragment (780 bp) and rolC gene fragment (540 bp) was confirmed by using gene-specific primers, and successful transformation was confirmed by the presence of rolB and rolC gene fragments. The results of PCR study indicated that the rolB and rolC gene fragments were incorporated into the A. vulgaris L. HR cultures. It indicates that the presence of the incorporation of the T-DNA into the genome of A. vulgaris L. HRs has been successful (Figure 8 and Supplementary Figure 5). Many studies concluded that the HR culture metabolite production depends on rol gene expression (Tiwari et al., 2007; Bulgakov, 2008). The rol genes play a major role in the pathway that leads to the increased production of secondary metabolites. In many cases, the rol gene of strain is involved in the parthenogenesis of the HR disease and establishment of transgenic plants with simple developmental and morphological alteration (Jouanin et al., 1987). The establishment of the root inducing (Ri) disease in HRs is interrelated with the expression of the rolA, B, C, and D loci in the majority assay of plant species (Taylor et al., 1985; Cardarelli et al., 1987). Along with them, rolB plays the most important role, whereas rolA, rolC, and rolD encourage the root development synergistically (Aoki and Syôno, 1999). The growth model of rolB-induced HR is characterized by high-level growth, branching, and plagiotropism (Capone et al., 1989). Genetically mediated transformation by Ri T-DNA of A. rhizogenes has been established to be an effective indirect way of accumulating and producing high levels of secondary metabolites in plant cells (Guillon et al., 2006). In a confirmation study in agreement with A. rhizogenes, there is a type of Gram-negative well-known soil-borne bacterium that causes HRs in many plants. Root loci (rol) genes harbored by the Ri plasmid of this bacterium are integrated into the host plant genome, causing HRs. rol genes are a reflection to affect the growth and development of transformed roots and induce secondary metabolite synthesis by turning on the mediated transcription defense genes (Ono and Tian, 2011).
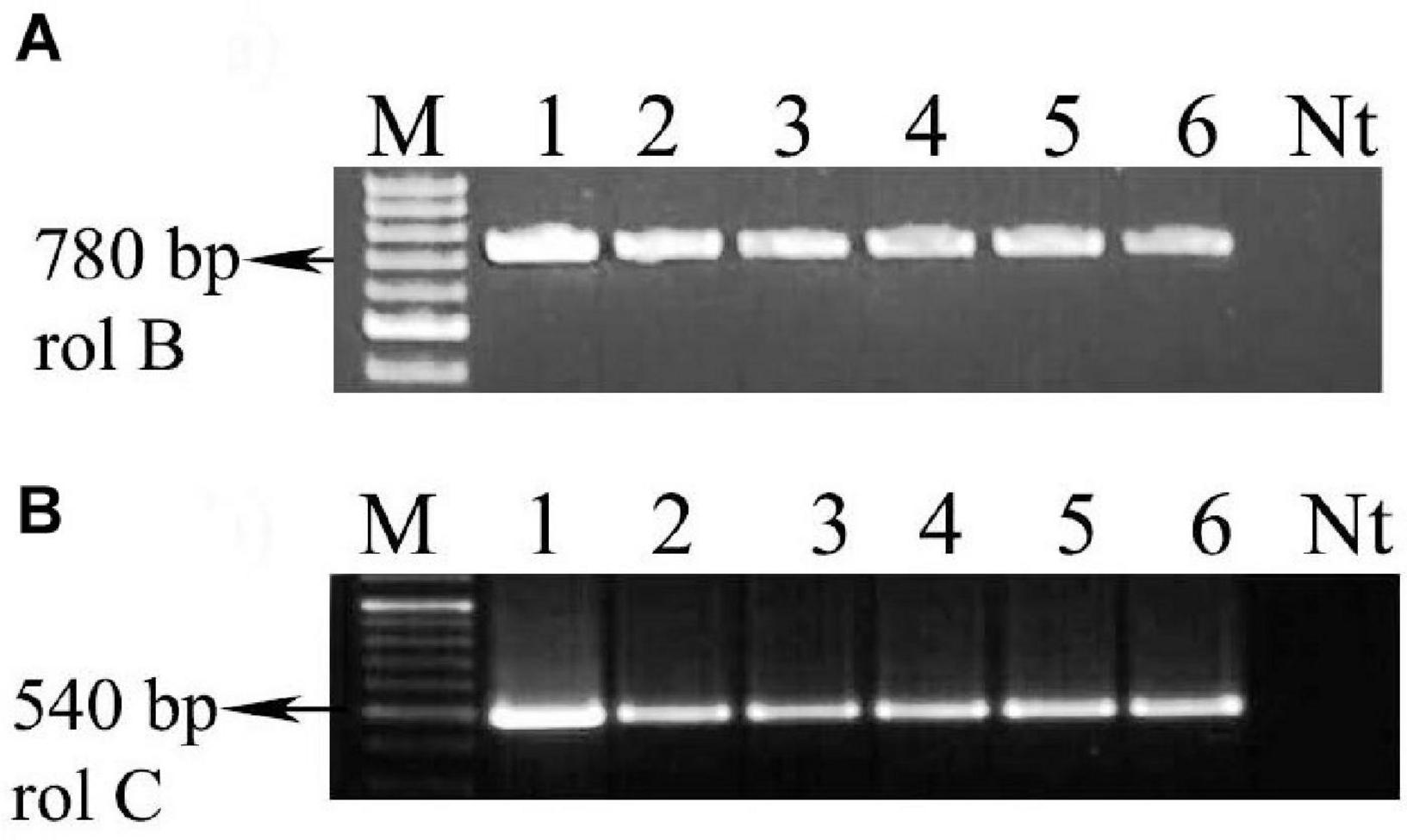
Figure 8. PCR conformations of transgenic hairy roots (A4 and R1000) from A. vulgaris L. leaf explants. (A) rolB gene amplification (780 bp). (B) rolC gene amplification (540 bp). Lane M: marker, lane 1: A4 plasmid DNA, lane 2: R1000 plasmid DNA, lanes 3 and 4: genomic DNA of hairy root culture (A4 strain), lanes 5 and 6: genomic DNA of hairy root culture (R1000 strain), Nt: non-transgenic root.
Isolation of Essential Oil and Quantification of β-Caryophyllene Content
Quantification of β-Caryophyllene in Callus Culture
The essential oil isolated from A. vulgaris L. callus was quantified with various concentrations of the β-caryophyllene standard compound. The calibration curve (SIM method) was determined to be utilized. GC–MS of β-caryophyllene compound retention time peak was observed at 7.34 s. In the present study, extracts of callus samples were analyzed through GC–MS by the scan mode method, and the highest β-caryophyllene compound was observed in without precursor-treated callus 2,4D 13.5 μM (1.61 mg/ml) and precursor-treated callus 2,4D 13.5 μM + FDP 3 μM (2.38 mg/ml), followed by NAA 16.1 μM (2.28 mg/ml) (Supplementary Figures 1, 2 and Table 2). These results were supported by a previous report (Arya and Patni, 2013), where enhanced quercetin compound (0.23 mg/ml) in precursor-treated callus was noticed. Treatment of A. absinthium callus with benzyl adenine (BAP) and NAA (2 mg/L), along with amino acids, such as valine and cysteine (12.5 mg/L), resulted in higher content of artemisinin compound (Zia et al., 2007). Enhanced trigonelline content was noticed through thin-layer chromatography, gas–liquid chromatography, and GC–MS analysis in nicotinic acid used as precursor in in vitro callus culture (Mathur and Kamal, 2012). Similarly, casein hydrolysate precursor feeding in cell suspension culture at 50, 75, and 100 mg/L concentrations resulted in the highest scopoletin compound, as revealed by high-performance thin-layer chromatography analysis (Abyari et al., 2016). Various explants of Simmondsia chinensis (leaves, nodes, internodes, shoot apices, and cotyledons) were used for callus induction from MS media along with various growth regulators [NAA, BAP, indole-3-acetic acid (IAA), and isopentenyl adenine (2ip)], and the final quantitative estimation of essential oils was observed at the highest amount of oil content at a 9-week-old callus culture (Aftab et al., 2008). A similar phenomenon has been observed in several studies (Zhao et al., 2001; Ouyang et al., 2005; Sivanandhan et al., 2012). In this study, calli are compared with previous reports that were treated with different precursors/elicitors as shown in Table 3.
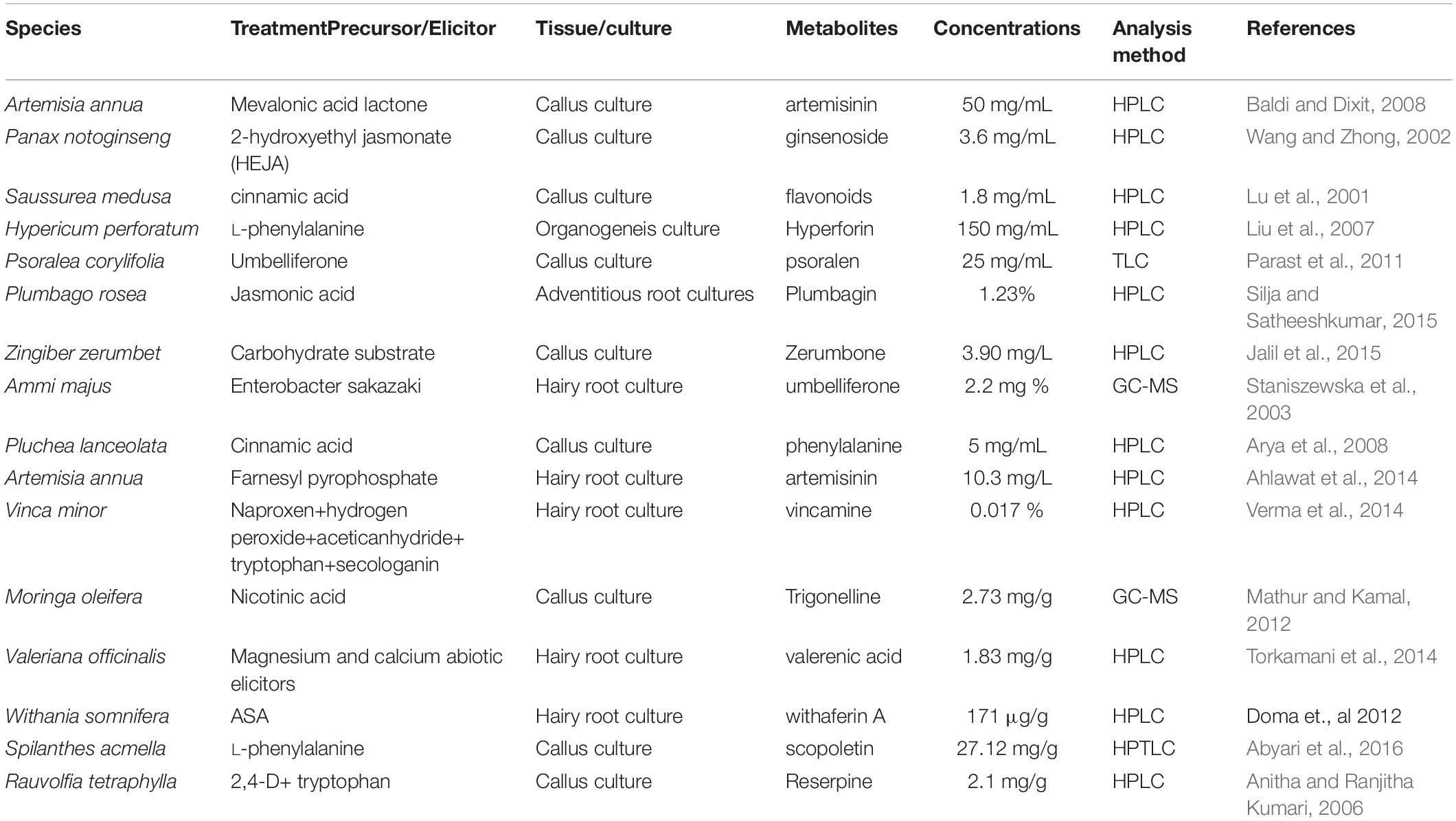
Table 2. Comparative study on various precursor/Elicitor treatment of different types of plant species.
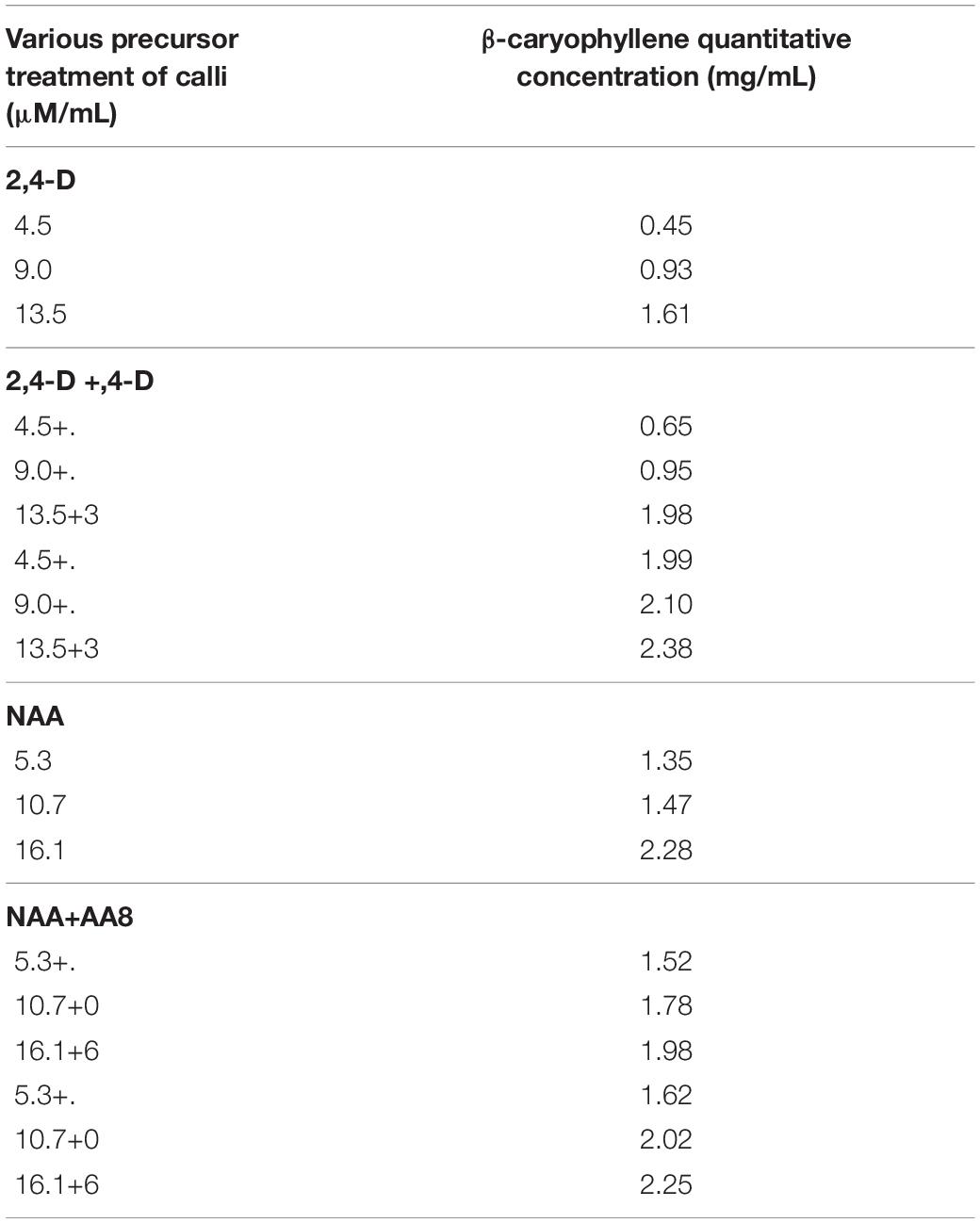
Table 3. Different concentration of with and without precursor treated callus samples quantified by GC-MS.
Quantification of β-Caryophyllene in HR Culture
The essential oil isolated from the HR was quantified with various concentrations of the β-caryophyllene standard compound by the SIM method. The retention time of β-caryophyllene compound was observed at 7.34 s. Among various treatments of the A4 induced HR, the highest β-caryophyllene compound in HR cultures without precursor treatment was 2.44 mg/ml, whereas precursor-treated HR showed 2.92 mg/ml (Supplementary Figure 3 and Table 4). The highest β-caryophyllene compound in R1000 induced HR cultures without precursor treatment was 1/2 MS (2.16 mg/ml) and precursor treatment with 1/2 MS + FDP 3 μM (2.80 mg/ml) (Supplementary Figure 4 and Table 4). The observation was in accordance with the results of P. minor HR induction from different A. rhizogenes strains (A4, ATCC43056, ATCC15834, and ATCC13333) (Ashraf et al., 2015). FDP precursor could be suitable for in vitro secondary metabolites engineering strategies for increasing β-caryophyllene content (17%) that was found in P. minor roots (Ismail et al., 2011), Micromeria fruticosa (Lu et al., 2001), Copaifera species (Liu et al., 2007), Litsea cubeba (Parast et al., 2011), Ailanthus altissima (Ayeb-Zakhama et al., 2014), Rosmarinus officinalis L. (Flamini et al., 2015), and Polygonum minus (Baharum et al., 2010). Recently, β-caryophyllene synthase gene (QHS1) from A. annua was confirmed in the genome of the Cyanobacterium synechocystis species by means of gas chromatography with flame-ionization detection (GC-FID) and GC–MS analyses (Cai et al., 1994; Reinsvold et al., 2011).
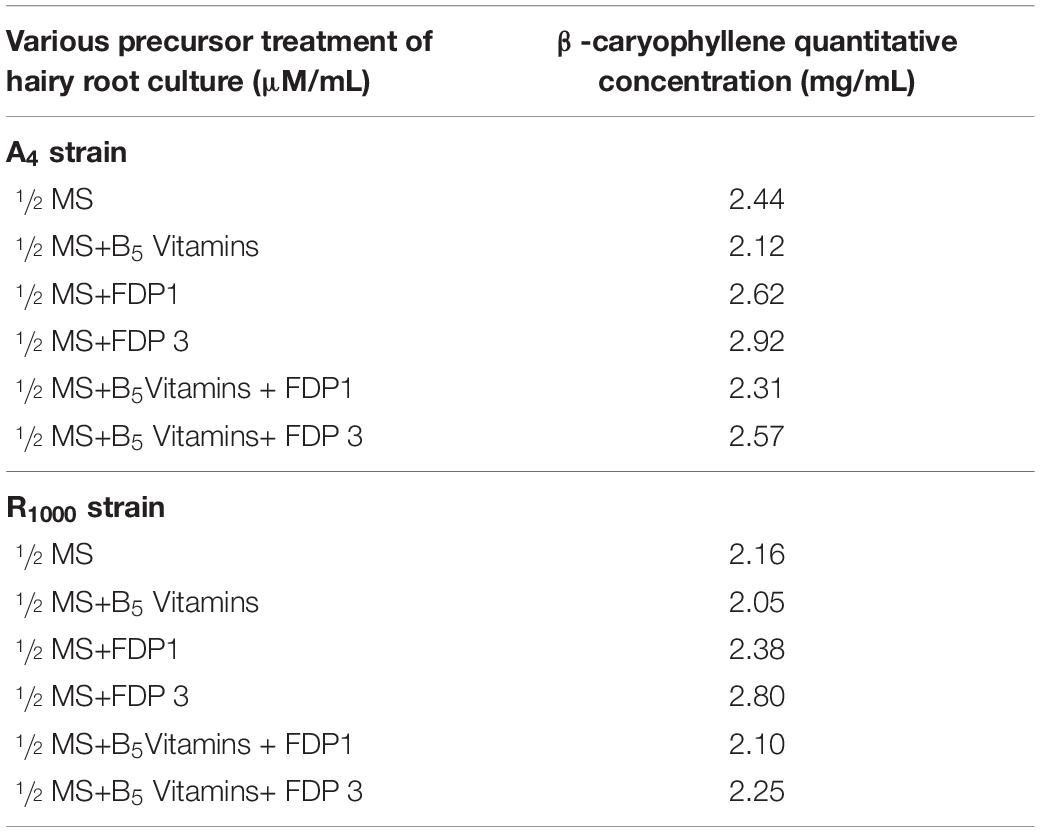
Table 4. Different concentrations of with and without precursor treated hairy root samples quantified by GC-MS.
Similarly, a farnesyl pyrophosphate synthase (VoFPS) gene co-transformation system was developed in Valeriana officinalis HRs for the enhancement of β-caryophyllene compound by recombinant and elicited methods (Ricigliano et al., 2016). The attained values, similar to those reported from different A. rhizogenes mediated transformations of several medicinal plant species, have been studied for the production of essential oil by both transformed and non-transformed root cultures (Santos et al., 2002, 2005; Szoke et al., 2004; Sujatha et al., 2013). The results from the present study agreed with the previous reports on various precursor and elicitor treatments for root induction. Some plants of vincamine biosynthesis were established through Vinca minor HR clone that was determined with various elicitor (acetyltransferase) and precursor treatments (cyclooxygenase inhibitor); the highest vincamine compound was observed at 17% (Verma et al., 2014). Enhanced tanshinones production in Salvia miltiorrhiza HR culture was used with different elicitors combined with yeast extract + Ag+ and Ag+ + methyl jasmonate (MeJA) for yeast extract + Ag+ + MeJA, and elicitation metabolite profiling was analyzed by ultra-performance liquid chromatography (UPLC) (Cheng et al., 2013). The use of MeJA and other signaling compounds for elicitation of transforming root cultures is opening a new way for a possibly profitable in vitro secondary metabolite production (Ahlawat et al., 2014). A soluble recombinant enzyme can catalyze the covalently bonded metal ion-dependent conversion of FDP to β-caryophyllene, sesquiterpene olefin contained in A. annua essential oil, was given by heterologous gene expression in Escherichia coli. Moreover, all through the initial stages, the β-caryophyllene synthase overexpressed in certain plant tissues and triggered in old ones in response to fungal elicitor suggests a role besides β-caryophyllene in host defense (Cai et al., 2002). Recently, Souret et al. (2002) have cloned three genes from A. annua that affect terpenes’ biosynthesis: 1-deoxy-D-xylulose-5-phosphate synthase (DXS), 1-deoxy-D-xylulose-5-phosphate reductoisomerase (DXR), and synthase from squalene (SQS) to use a library of cDNA made from hairy A. annua roots. Previously, overexpression of farnesyl pyrophosphate synthase in A. annua leads to high artemisinin aggregation levels by converting IPP and DMADP into diphenylphosphinic acid pentafluorophenyl ester (FDPP) (Banyai et al., 2010). Cloning and characterization of A. annua 1-hydroxy-2-methyl-2-(E)-butenyl 4-diphosphate reductase (AaHDR) involved in enhanced artemisinin production via improved artemisinin biosynthetic pathway precursor development through the MEP pathway were being reported in A. annua. Tryptophan alkaloid compound enhanced through precursor feeding in HR culture has been identified (Hughes et al., 2004). The effect of precursor-treated HR suspension culture has produced a high level of glucosinolate compound of Nasturtium montanum and Cleome chelidonii (Songsak and Lockwood, 2004). Robins et al. (1990, 1991) also reported hyoscyamine compound biosynthesis during metabolic pathways as amino acid precursors. A. rhizogenes enables the high production of these secondary metabolites for extensive industrial applications (Srivastava and Srivastava, 2007; Suza et al., 2008; Swarna and Ravindhran, 2012) that are useful as pharmaceuticals, cosmetics, and food additives (Signs and Flores, 1990; Guillon et al., 2006). In this study, HR culture has been compared with some previous reports that were treated with different precursors/elicitors (Table 3).
Conclusion and Perspectives
This study, to the best of our knowledge, is the first attempt in reporting in vitro precursor treatment for enhancement of β-caryophyllene content by using callus and HR culture of A. vulgaris L. Although the biosynthesis pathway of secondary metabolites has not been well explored, the terpenoid biosynthesis pathway is well known for the production of β-caryophyllene via FDP. In this study, we aimed to enhance the output by using FDP as a precursor, and our findings proved that by using precursor-treated cultures, we can improve β-caryophyllene production. In conclusion, β-caryophyllene enhancement was successfully done with precursor-treated HR culture of A. vulgaris L. after 12 weeks in combined treatment culture of 1/2 MS + FDP 3 μM (2.92 mg/ml, respectively). These findings could encourage and provide support to synthesize new active natural bioactive compounds as an alternative to synthetic repellent and insecticides.
Data Availability Statement
The original contributions presented in the study are included in the article/Supplementary Material, further inquiries can be directed to the corresponding author/s.
Author Contributions
SB and BR conceived the idea presented. SB developed the study, performed the experimental work, and wrote the main manuscript. RB developed the GC–MS methodology and performed the analysis. AM and DS analyzed the data interpretation. SS and GP revised the manuscript contents. Finally, all authors have read and approved the manuscript.
Funding
The authors acknowledge the University Grants Commission, New Delhi, India for providing financial assistance (UGC letter no. F.No.42-922/2013).
Conflict of Interest
The authors declare that the research was conducted in the absence of any commercial or financial relationships that could be construed as a potential conflict of interest.
Acknowledgments
SB thanks postdoctoral research mentor Prof. Zhiqin Zhou, College of Horticulture and Landscape Architecture, Southwest University, Chongqing, China, for his support and encouragement during the revision time.
Supplementary Material
The Supplementary Material for this article can be found online at: https://www.frontiersin.org/articles/10.3389/fpls.2021.634178/full#supplementary-material
References
Abyari, M., Nasr, N., Soorni, J., and Sadhu, D. (2016). Enhanced accumulation of scopoletin in cell suspension culture of Spilanthes acmella Murr. using precursor feeding. Braz. Arch. Biol. 59:e16150533.
Adam, K., and Zapp, J. (1998). Biosynthesis of the isoprene units of chamomile sesquiterpenes. Phytochemistry 48, 953–959. doi: 10.1016/s0031-9422(97)00992-8
Aftab, F., Akram, S., and Iqbal, J. (2008). Estimation of fixed oils from various explants and in vitro callus cultures of jojoba (Simmondsia chinensis). Pak. J. Bot. 40, 1467–1471.
Ahlawat, S., Saxena, P., Alam, P., Wajid, S., and Abdin, M. Z. (2014). Modulation of artemisinin biosynthesis by elicitors, inhibitor, and precursor in hairy root cultures of Artemisia annua L. J. Plant Interact. 9, 811–824. doi: 10.1080/17429145.2014.949885
Amiel, E., Ofir, R., Dudai, N., Soloway, E., Rabinsky, T., and Rachmilevitch, S. (2012). β-Caryophyllene, a compound isolated from the biblical balm of Gilead (Commiphora gileadensis), is a selective apoptosis inducer for tumor cell lines. Evid. Based Complement. Altern. Med. 2012:872394. doi: 10.1155/2012/872394
Anitha, S., and Ranjitha Kumari, B. D. (2006). Stimulation of reserpine biosynthesis in the callus of Rauvolfia tetraphylla L. by precursor feeding. Afr. J. Biotechnol. 5, 659–661.
Aoki, S., and Syôno, K. (1999). Synergistic function of rolB, rolC, ORF13 and ORF14 of TL-DNA of rhizogenes in hairy root induction in Nicotiana tabacum. Plant Cell Physiol. 40, 252–256. doi: 10.1093/oxfordjournals.pcp.a029535
Arya, D., and Patni, V. (2013). Pharmacognostic profile and phytochemical investigation of Pluchea lanceolata Oliver & Hiern. vivo and in vitro. Int. J. Pharm. Sci. Rev. Res. 22, 157–161.
Arya, D., Patni, V., and Kant, U. (2008). In vitro propagation and quercetin quantification in callus cultures of Rasna (Pluchea lanceolata Oliver & Hiern.). Indian. J. Biotechnol. 7, 383–387.
Ashraf, M. F., Zain, C. R. C. M., Zainal, Z., Noor, N. M., Anuar, N., Markom, M., et al. (2015). Establishment of Persicaria minor hairy roots and analysis of secreted β-Caryophyllene in medium broth. Plant Cell Tissue Organ Cult. 121, 11–20. doi: 10.1007/s11240-014-0674-2
Ayeb-Zakhama, E., Ben Salem, S., Sakka Rouis, L., Flamini, G., Ben Jannet, H., and Harzallah Skhiri, F. (2014). Chemical composition and phytotoxic effects of essential oils obtained from Ailanthus altissima (Mill.) swingle cultivated in Tunisia. Chem. Biodivers. 11, 1216–1227. doi: 10.1002/cbdv.201300409
Baharum, S. N., Bunawan, H., Ghani, M. A. A., Mustapha, W. A. W., and Noor, N. M. (2010). Analysis of the chemical composition of the essential oil of Polygonum minus Huds. using two-dimensional gas chromatography-time-of-flight mass spectrometry (GC-TOF MS). Molecules 15, 7006–7015. doi: 10.3390/molecules15107006
Baldi, A., and Dixit, V. K. (2008). Yield enhancement strategies for artemisinin production by suspension cultures of Artemisia annua. Bioresour. Technol. 99, 4609–4614. doi: 10.1016/j.biortech.2007.06.061
Banyai, W., Kirdmanee, C., Mii, M., and Supaibulwatana, K. (2010). Overexpression of farnesyl pyrophosphate synthase (FPS) gene affected artemisinin content and growth of Artemisia annua L. Plant Cell Tissue Organ Cult. 103, 255–265. doi: 10.1007/s11240-010-9775-8
Boitel-Conti, M., Laberche, J. C., Lanoue, A., Ducrocq, C., and Sangwan-Norreel, B. S. (2000). Influence of feeding precursors on tropane alkaloid production during an abiotic stress in Datura innoxia transformed roots. Plant Cell Tissue Organ Cult. 60, 131–137.
Borzabad, R. K., Sudarshana, M. S., and Niranjan, M. H. (2010a). In vitro plant regeneration from stem derived callus of Artemisia vulgaris L. Rep. Opin. 2, 121–125.
Borzabad, R. K., Sudarshana, M. S., and Niranjan, M. H. (2010b). In vitro plant regeneration from leaf explants of Artemisia vulgaris L.: a medicinal herb. Mod. Appl. Sci. 4:130.
Boucher, Y., and Doolittle, W. F. (2000). The role of lateral gene transfer in the evolution of isoprenoid biosynthesis pathways. Mol. Microbiol. 37, 703–716. doi: 10.1046/j.1365-2958.2000.02004.x
Bulgakov, V. P. (2008). Functions of rol genes in plant secondary metabolism. Biotech. Adv. 26, 318–324. doi: 10.1016/j.biotechadv.2008.03.001
Cai, G. Q., Li, G. Z., and Ye, H. C. (1994). Hairy root culture of Artemisia annua L. by Ri plasmid transformation and biosynthesis of artemisinin. Chin. J. Biotechnol. 11, 227–235.
Cai, Y., Jia, J. W., Crock, J., Lin, Z. X., Chen, X. Y., and Croteau, R. (2002). A cDNA clone for β-caryophyllene synthase from Artemisia annua. Phytochemistry 61, 523–529. doi: 10.1016/s0031-9422(02)00265-0
Capone, I., Spano, L., Cardarelli, M., Bellincampi, D., Petit, A., and Costantino, P. (1989). Induction and growth properties of carrot roots with different complements of Agrobacterium rhizogenes T-DNA. Plant Mol. Biol. 13, 43–52. doi: 10.1007/bf00027334
Cardarelli, M., Mariotti, D., Pomponi, M., Spano, L., Capone, I., and Costantino, P. (1987). Agrobacterium rhizogenes T-DNA genes capable of inducing hairy root phenotype. Mol. Gen. Genet. 209, 475–480. doi: 10.1007/bf00331152
Chantraine, J. M., Laurent, D., Ballivian, C., Saavedra, G., Ibanez, R., and Vilaseca, L. A. (1998). Insecticidal activity of essential oils on Aedes aegypti larvae. Phytother. Res. 12, 350–354. doi: 10.1002/(sici)1099-1573(199808)12:5<350::aid-ptr311>3.0.co;2-7
Cheng, Q., He, Y., Li, G., Liu, Y., Gao, W., and Huang, L. (2013). Effects of combined elicitors on tanshinone metabolic profiling and SmCPS expression in Salvia miltiorrhiza hairy root cultures. Molecules 18, 7473–7485. doi: 10.3390/molecules18077473
Cho, H. J., Widholm, J. M., Tanaka, N., Nakanishi, Y., and Murooka, Y. (1998). Agrobacterium rhizogenes-mediated transformation and regeneration of the legume Astragalus sinicus (Chinese milk vetch). Plant Sci. 138, 53–65. doi: 10.1016/s0168-9452(98)00149-6
Croteau, R., Kutchan, T., and Lewis, N. (2000). “Natural products (secondary metabolites),” in Biochemistry and Molecular Biology of Plants, eds B. Buchanan, W. Gruissem, and R. Jones (Rockville, MD: ASPB), 1250–1318.
Da Silva, J. A. T. (2004). Mining the essential oils of the Anthemideae. Asian J. Biotechnol. 3, 706–720.
Danphitsanuparn, P., Boonsnongcheep, P., Boriboonkaset, T., Chintapakorn, Y., and Prathanturarug, S. (2012). Effects of rhizogenes strains and other parameters on production of isoflavonoids in hairy roots of Pueraria candollei Grah. ex Benth. var. candollei. Plant Cell Tissue Organ Cult. 111, 315–322. doi: 10.1007/s11240-012-0196-8
De Moraes, C. M., Mescher, M. C., and Tumlinson, J. H. (2001). Caterpillar-induced nocturnal plant volatiles repel conspecific females. Nature 410, 577–580. doi: 10.1038/35069058
Dedaldechamp, F., and Uhel, C. (1999). Induction of anthocyanin synthesis in nonpigmented grape cell suspensions by acting on DFR substrate availability or precursors level. Enzyme Microb. Technol. 25, 316–321. doi: 10.1016/s0141-0229(99)00047-2
Doma, M., Abhayankar, G., Reddy, V. D., and Kishor, P. B. (2012). Carbohydrate and elicitor enhanced withanolide (withaferin A and withanolide A) accumulation in hairy root cultures of Withania somnifera (L.). Indian J. Exp. Biol. 50, 484–490.
Farshad Ashraf, M., Abd Aziz, M., Abdul Kadir, M., Stanslas, J., and Farokhian, E. (2013). In vitro tuberization of Chlorophytum borivilianum Sant & Fern (Safed musli) as influenced by sucrose, CCC and culture systems. Plant Cell Physiol. 54, 1356–1364. doi: 10.1093/pcp/pct083
Flamini, G., Melai, B., Pistelli, L., and Chiappe, C. (2015). How to make a green product greener: use of ionic liquids as additives during essential oil hydrodistillation. RSC Adv. 5, 69894–69898. doi: 10.1039/c5ra12649e
Fok, T. F. (2002). Neonatal jaundice: traditional Chinese medicine approach. J. Perinatol. 21, 98–100.
Gertsch, J., Leonti, M., Raduner, S., Racz, I., Chen, J.-Z., Xie, X.-Q., et al. (2008). Beta-caryophyllene is a dietary cannabinoid. Proc. Natl. Acad. Sci. U.S.A. 105, 9099–9104. doi: 10.1073/pnas.0803601105
Giri, A., and Narasu, M. L. (2000). Transgenic hairy roots: recent trends and applications. Biotechnol. Adv. 18, 1–22. doi: 10.1016/s0734-9750(99)00016-6
Goel, M. K., Goel, S., Banerjee, S., Shanker, K., and Kukreja, A. K. (2010). Agrobacterium rhizogenes-mediated transformed roots of Rauwolfia serpentina for reserpine biosynthesis. Med. Aromat. Plant Sci. Biotech. 4, 8–14.
Grzegorczyk, I., Krolicka, A., and Wysokinska, H. (2006). Establishment of Salvia officinalis L. hairy root cultures for the production of rosmarinic acid. Z. Naturforsch. C J. Biosci. 61, 351–356. doi: 10.1515/znc-2006-5-609
Guillon, S., Tremouillaux-Guiller, J., Pati, P. K., Rideau, M., and Gantet, P. (2006). Harnessing the potential of hairy roots: dawn of a new era. Trends Biotechnol. 24, 403–409. doi: 10.1016/j.tibtech.2006.07.002
Hanafy, M. S., Matter, M. A., Asker, M. S., and Rady, M. R. (2016). Production of indole alkaloids in hairy root cultures of Catharanthus roseus L. and their antimicrobial activity. S. Afr. J. Bot. 105, 9–18. doi: 10.1016/j.sajb.2016.01.004
Henry, L. K., Thomas, S. T., Widhalm, J. R., Lynch, J. H., Davis, T. C., Kessler, S. A., et al. (2018). Contribution of isopentenyl phosphate to plant terpenoid metabolism. Nat. Plants 4, 721–729. doi: 10.1038/s41477-018-0220-z
Huang, S. H., Vishwakarma, R. K., Lee, T. T., Chan, H. S., and Tsay, H. S. (2014). Establishment of hairy root lines and analysis of iridoids and secoiridoids in the medicinal plant Gentiana scabra. Bot. Stud. 55:17. doi: 10.1186/1999-3110-55-17
Hughes, E. H., Hong, S. B., Gibson, S. I., Shanks, J. V., and San, K. Y. (2004). Expression of a feedback resistant anthranilate synthase in Catharanthus roseus hairy roots provides evidence for tight regulation of terpenoid indole alkaloid levels. Biotechnol. Bioeng. 86, 718–727. doi: 10.1002/bit.20081
Inoguchi, M., Ogawa, S., Furukawa, S., and Kondo, H. (2003). Production of an allelopathic polyacetylene in hairy root cultures of goldenrod (Solidago altissima L.). Biosci. Biotechnol. Biochem. 67, 863–868. doi: 10.1271/bbb.67.863
Ismail, I., Gor, M. C., Mohamed-Hussein, Z. A., Zainal, Z., and Noor, N. M. (2011). “Alteration of abiotic stress responsive genes in Polygonum minus roots by jasmonic acid elicitation,” in Plants and Environment, eds K. N. V. Hemanth and D. Kambiranda (Rijeka: InTech).
Jalil, M., Annuar, M. S. M., Tan, B. C., and Khalid, N. (2015). Effects of selected physicochemical parameters on zerumbone production of Zingiber zerumbet Smith cell suspension culture. Evid. Based Complement. Altern. Med. 2015:757514.
Jouanin, L., Guerche, P., Pamboukdjian, N., Tourneur, C., Delbart, F. C., and Tourneur, J. (1987). Structure of T-DNA in plants regenerated from roots transformed by rhizogenes strain A4. Mol. Gen. Genet. 206, 387–392. doi: 10.1007/bf00428876
Keil, M., Härtle, B., Guillaume, A., and Psiorz, M. (2000). Production of amarogentin in root cultures of Swertia chirata. Planta Med. 66, 452–457. doi: 10.1055/s-2000-8579
Khanuja, S. P., Shasany, A. K., Darokar, M. P., and Kumar, S. (1999). Rapid isolation of DNA from dry and fresh samples of plants producing large amounts of secondary metabolites and essential oils. Plant Mol. Biol. Rep. 17, 74–74.
Khatodia, S., Biswas, K., and Bhatotia, K. (2013). Induction and establishment of hairy root culture of solanum xanthocarpum using agrobacterium rhizogenes. Int. J. Pharm. Bio. Sci. 1, 59–63.
Laule, O., Furholz, A., Chang, H. S., Zhu, T., Wang, X., Heifetz, P. B., et al. (2003). Crosstalk between cytosolic and plastidial pathways of isoprenoid biosynthesis in Arabidopsis thaliana. Proc. Natl. Acad. Sci. U.S.A. 100, 6866–6871. doi: 10.1073/pnas.1031755100
Lee, S. J. (1998). Estrogenic flavonoids from Artemisia vulgaris L. J. Agric. Food Chem. 46, 3325–3329.
Lichtenthaler, H. (1999). The 1-deoxy-D-xylulose-5-phosphate pathway of isoprenoid biosynthesis in plants. Ann. Rev. Plant Physiol. Plant Mol. Biol. 50, 47–65. doi: 10.1146/annurev.arplant.50.1.47
Liu, X. N., Zhang, X. Q., Zhang, S. X., and Sun, J. S. (2007). Regulation of metabolite production by precursors and elicitors in liquid cultures of Hypericum perforatum. Plant Cell Tissue Organ Cult. 91, 1–7. doi: 10.1007/s11240-007-9271-y
Lu, D., Zhao, D., Huang, Y., and Zhao, Q. (2001). The effect of precursor feeding on flavonoids biosynthesis in cell suspension cultures of Saussurea medusa. Acta Bot. Yunn. 23, 497–503.
Mathur, M., and Kamal, R. (2012). Studies on trigonelline from Moringa oleifera and its in vitro regulation by feeding precursor in cell cultures. Rev. Bras. Farmacogn. 22, 994–1001. doi: 10.1590/s0102-695x2012005000041
Mohammadparast, B., Rustaiee, A. R., Rasouli, M., Zardari, S., and Agrawal, V. (2015). In vitro enhancement of psoralen as an important anticancer compound in Psoralea corylifolia through precursor feeding. Pharm. Biol. 53, 735–738. doi: 10.3109/13880209.2014.942786
Murashige, T., and Skoog, F. (1962). A revised medium for rapid growth and bio agsays with tohaoco tissue cultures. Physiol. Plant 15, 473–497.
Nin, S., Bennici, A., Roselli, G., Mariotti, D., Schiff, S., and Magherini, R. (1997). Agrobacterium-mediated transformation of Artemisia absinthium L. (wormwood) and production of secondary metabolites. Plant Cell Rep. 16, 725–730. doi: 10.1007/s002990050310
Ono, N. N., and Tian, L. (2011). The multiplicity of hairy root cultures: prolific possibilities. Plant Sci. 180, 439–446. doi: 10.1016/j.plantsci.2010.11.012
Ouyang, J., Wang, X. D., Zhao, B., and Wang, Y. C. (2005). Enhanced production of phenylethanoid glycosides by precursor feeding to cell culture of Cistanche deserticola. Process Biochem. 40, 3480–3484. doi: 10.1016/j.procbio.2005.02.025
Parast, B. M., Chetri, S. K., Sharma, K., and Agrawal, V. (2011). In vitro isolation, elicitation of psoralen in callus cultures of Psoralea corylifolia and cloning of psoralen synthase gene. Plant Physiol. Biochem. 49, 1138–1146. doi: 10.1016/j.plaphy.2011.03.017
Pietrosiuk, A., Sykłowska-Baranek, K., Wiedenfeld, H., Wolinowska, R., Furmanowa, M., and Jaroszyk, E. (2006). The shikonin derivatives and pyrrolizidine alkaloids in hairy root cultures of Lithospermum canescens (Michx.) Lehm. Plant Cell Rep. 25, 1052–1058. doi: 10.1007/s00299-006-0161-2
Rao, S. R., and Ravishankar, G. A. (2002). Plant cell cultures: chemical factories of secondary metabolites. Biotechnol. Adv. 20, 101–153. doi: 10.1016/s0734-9750(02)00007-1
Reinsvold, R. E., Jinkerson, R. E., Radakovits, R., Posewitz, M. C., and Basu, C. (2011). The production of the sesquiterpene β-caryophyllene in a transgenic strain of the cyanobacterium Synechocystis. J. Plant Physiol. 168, 848–852. doi: 10.1016/j.jplph.2010.11.006
Repetto, M. G., and Llesuy, S. F. (2002). Antioxidant properties of natural compounds used in popular medicine for gastric ulcers. Braz. J. Med. Biol. Res. 35, 523–534. doi: 10.1590/s0100-879x2002000500003
Ricigliano, V., Kumar, S., Kinison, S., Brooks, C., Nybo, S. E., Chappell, J., et al. (2016). Regulation of sesquiterpenoid metabolism in recombinant and elicited Valeriana officinalis hairy roots. Phytochemistry 125, 43–53. doi: 10.1016/j.phytochem.2016.02.011
Robins, R. J., Parr, A. J., Payne, J., Walton, N. J., and Rhodes, M. J. (1990). Factors regulating tropane-alkaloid production in a transformed root culture of a Datura candida × D. aurea hybrid. Planta 181, 414–422.
Robins, R. J., Parr, A. J., and Walton, N. J. (1991). Studies on the biosynthesis of tropane alkaloids in Datura stramonium L. transformed root cultures. Planta 183, 196–201. doi: 10.1007/bf00197788
Sabulal, B., Dan, M., Kurup, R., Pradeep, N. S., Valsamma, R. K., and George, V. (2006). Caryophyllene-rich rhizome oil of Zingiber nimmonii from South India: chemical characterization and antimicrobial activity. Phytochemistry 67, 2469–2473. doi: 10.1016/j.phytochem.2006.08.003
Sanchez-Sampedro, M. A., Fernandez-Tarrago, J., and Corchete, P. (2005). Yeast extract and methyl jasmonate-induced silymarin production in cell cultures of Silybum marianum (L.) Gaertn. J. Biotechnol. 119, 60–69. doi: 10.1016/j.jbiotec.2005.06.012
Santos, P. A., Figueiredo, A. C., Lourenço, P. M., Barroso, J. G., Pedro, L. G., Oliveira, M. M., et al. (2002). Hairy root cultures of Anethum graveolens (dill): establishment, growth, time-course study of their essential oil and its comparison with parent plant oils. Biotechnol. Lett. 24, 1031–1036.
Santos, P. A., Figueiredo, A. C., Oliveira, M. M., Barroso, J. G., Pedro, L. G., Deans, S. G., et al. (2005). Growth and essential oil composition of hairy root cultures of Levisticum officinale WDJ Koch (lovage). Plant Sci. 168, 1089–1096. doi: 10.1016/j.plantsci.2004.12.009
Schmitt, J., and Petersen, M. (2002). Influence of methyl jasmonate and coniferyl alcohol on pinoresinol and matairesinol accumulation in a Forsythia× intermedia suspension culture. Plant Cell Rep. 20, 885–890. doi: 10.1007/s00299-001-0414-z
Signs, M. W., and Flores, H. E. (1990). The biosynthetic potential of plant roots. Biol. Essays 12, 7–13. doi: 10.1002/bies.950120103
Silja, P. K., and Satheeshkumar, K. (2015). Establishment of adventitious root cultures from leaf explants of Plumbago rosea and enhanced plumbagin production through elicitation. Ind. Crops Prod. 76, 479–486. doi: 10.1016/j.indcrop.2015.07.021
Sivanandhan, G., Arun, M., Mayavan, S., Rajesh, M., Mariashibu, T. S., Manickavasagam, M., et al. (2012). Chitosan enhances withanolides production in adventitious root cultures of Withania somnifera (L.) Dunal. Ind. Crops Prod. 37, 124–129. doi: 10.1016/j.indcrop.2011.11.022
Sivanandhan, G., Selvaraj, N., Ganapathi, A., and Manickavasagam, M. (2014). Enhanced biosynthesis of withanolides by elicitation and precursor feeding in cell suspension culture of Withania somnifera (L.) Dunal in shake-flask culture and bioreactor. PLoS One 9:e104005. doi: 10.1371/journal.pone.0104005
Songsak, T., and Lockwood, G. B. (2004). Production of two volatile glucosinolate hydrolysis compounds in Nasturtium montanum and Cleome chelidonii plant cell cultures. Fitoterapia 75, 296–301. doi: 10.1016/j.fitote.2004.01.007
Souret, F. F., Weathers, P. J., and Wobbe, K. K. (2002). The mevalonate-independent pathway is expressed in transformed roots of Artemisia annua and regulated by light and culture age. In Vitro Cell Dev. Biol. Plant 38, 581–588. doi: 10.1079/ivp2002343
Srivastava, S., and Srivastava, A. K. (2007). Hairy root culture for mass-production of high-value secondary metabolites. Crit. Rev. Biotechnol. 27, 29–43. doi: 10.1080/07388550601173918
Staniszewska, I., Królicka, A., Maliński, E., Łojkowska, E., and Szafranek, J. (2003). Elicitation of secondary metabolites in in vitro cultures of Ammi majus L. enzyme. Microb. Technol. 33, 565–568. doi: 10.1016/s0141-0229(03)00180-7
Sudha, C. G., Reddy, B. O., Ravishankar, G. A., and Seeni, S. (2003). Production of ajmalicine and ajmaline in hairy root cultures of Rauvolfia micrantha Hook f., a rare and endemic medicinal plant. Biotech. Lett. 25, 631–636.
Sujatha, G., and Kumari, B. D. R. (2007). Effect of phytohormones on micropropagation of Artemisia vulgaris L. Acta Physiol. Plant. 29, 189–195. doi: 10.1007/s11738-006-0023-0
Sujatha, G., Zdravkovic-Korac, S., Calic, D., Flamini, G., and Kumari, B. R. (2013). High-efficiency rhizogenes-mediated genetic transformation in Artemisia vulgaris: hairy root production and essential oil analysis. Ind. Crop Prod. 44, 643–652. doi: 10.1016/j.indcrop.2012.09.007
Sujatha, M., and Dhingra, M. (1993). Rapid plant regeneration from various explants of Jatropha integerrima. Plant Cell Tissue Organ Cult. 35, 293–296. doi: 10.1007/bf00037284
Sundararajan, B., and Kumari, B. D. R. (2015). Effect of farnesyl diphosphate on beta caryophyllene content in the calli of Artemisia vulgaris L. Int. J. New Technol. Sci. Eng. 2, 307–316.
Suza, W., Harris, R. S., and Lorence, A. (2008). Hairy roots: from high-value metabolite production to phytoremediation. Electron J. Integr. Biosci. 3, 57–65.
Swarna, J., and Ravindhran, R. (2012). Agrobacterium rhizogenes–mediated hairy root induction of Momordica charantia Linn. and the detection of charantin, a potent hypoglycaemic agent in hairy roots. Res. J. Biotechnol. 7, 227–231.
Szoke, E., Maday, E., Tyihak, E., Kuzovkina, I. N., and Lemberkovics, E. (2004). New terpenoids in cultivated and wild chamomile (in vivo and in vitro). J. Chromatogr. B Biomed. Appl. 800, 231–238. doi: 10.1016/j.jchromb.2003.09.038
Talukder, P., Talapatra, S., Ghoshal, N., and Sen Raychaudhuri, S. (2016). Antioxidant activity and high−performance liquid chromatographic analysis of phenolic compounds during in vitro callus culture of Plantago ovata Forsk. and effect of exogenous additives on accumulation of phenolic compounds. J. Sci. Food Agric. 96, 232–244. doi: 10.1002/jsfa.7086
Tan, R. X., Lu, H., Wolfender, J. L., Yu, T. T., Zheng, W. F., Yang, L., et al. (1999). Mono- and sesquiterpenes and antifungal constituents from Artemisia species. Planta Med. 56, 64–67.
Tao, J., and Li, L. (2006). Genetic transformation of Torenia fournieri L. mediated by rhizogenes. S. Afr. J. Bot. 72, 211–216. doi: 10.1016/j.sajb.2005.07.010
Taylor, B. H., Amasino, R. M., White, F. F., Nester, E. W., and Gordon, M. P. (1985). T-DNA analysis of plants regenerated from hairy root tumors. Mol. Gen. Genet. 201, 554–557. doi: 10.1007/bf00331355
Thimmaraju, R., Venkatachalam, L., and Bhagyalakshmi, N. (2008). Morphometric and biochemical characterization of red beet (Beta vulgaris L.) hairy roots obtained after single and double transformations. Plant Cell Rep. 27, 1039–1052. doi: 10.1007/s00299-008-0527-8
Tigno, X. T., de Guzman, F., and Flora, A. M. (2000). Phytochemical analysis and hemodynamic actions of Artemisia vulgaris L. Clin. Hemorheol. Microcirc. 23, 167–175.
Tiwari, R. K., Trivedi, M., Guang, Z. C., Guo, G. Q., and Zheng, G. C. (2007). Genetic transformation of Gentiana macrophylla with rhizogenes: growth and production of secoiridoid glucoside gentiopicroside in transformed hairy root cultures. Plant Cell Rep. 26, 199–210. doi: 10.1007/s00299-006-0236-0
Toivonen, L., Balsevich, J. W. G. W., and Kurz, W. G. W. (1989). Indole alkaloid production by hairy root cultures of Catharanthus roseus. Plant Cell Tissue Organ Cult. 18, 79–93. doi: 10.1007/bf00033467
Torkamani, M. R. D., Abbaspour, N., Jafari, M., and Samadi, A. (2014). Elicitation of valerenic acid in the hairy root cultures of Valeriana officinalis L (Valerianaceae). Trop. J. Pharm. Res. 13, 943–949. doi: 10.4314/tjpr.v13i6.17
Verma, P., Khan, S. A., Mathur, A. K., Shanker, K., and Lal, R. K. (2014). Regulation of vincamine biosynthesis and associated growth promoting effects through abiotic elicitation, cyclooxygenase inhibition, and precursor feeding of bioreactor grown Vinca minor hairy roots. Appl. Biochem. Biotechnol. 173, 663–672. doi: 10.1007/s12010-014-0883-5
Wang, J., Zhu, F., Zhou, X. M., Iu, C. Y., and Lei, C. L. (2006). Repellent and fumigant activity of essential oil from Artemisia vulgaris to Tribolium castaneum (Herbst) (Coleoptera: Tenebrionidae). J. Stored Prod. Res. 42, 339–347. doi: 10.1016/j.jspr.2005.06.001
Wang, J. W., Xia, Z. H., Chu, J. H., and Tan, R. X. (2004). Simultaneous production of anthocyanin and triterpenoids in suspension cultures of Perilla frutescens. Enzyme Microb. Technol. 34, 651–656. doi: 10.1016/j.enzmictec.2004.02.004
Wang, W., and Zhong, J. J. (2002). Manipulation of ginsenoside heterogeneity in cell cultures of Panax notoginseng by addition of jasmonates. J. Biosci. Bioeng. 93, 48–53. doi: 10.1016/s1389-1723(02)80053-6
Wiktorowska, E., Długosz, M., and Janiszowska, W. (2010). Significant enhancement of oleanolic acid accumulation by biotic elicitors in cell suspension cultures of Calendula officinalis L. Enzyme Microb. Technol. 46, 14–20. doi: 10.1016/j.enzmictec.2009.09.002
Williams, J. D., Xie, T., and Acharya, D. N. (2012). “Headspace solid-phase microextraction (HS-SPME) gas chromatography-mass spectrometry (GC-MS) analysis of the essential oils from the aerial parts of Artemisa vulgaris L. Reveal the possible existence of new chemotypes,” in Proceedings of the 244th American Chemical Society National Meeting, Philadelphia, 19-22 August 2012, Philadelphia, PA, 204.
Wu, H., Sparks, C., Amoah, B., and Jones, H. D. (2003). Factors influencing successful Agrobacterium-mediated genetic transformation of wheat. Plant Cell Rep. 21, 659–668. doi: 10.1007/s00299-002-0564-7
Yao, S. C., Bai, L. H., Lan, Z. Z., Tang, M. Q., Zhai, Y. J., Huang, H., et al. (2016). Hairy root induction and polysaccharide production of medicinal plant Callerya speciosa. Plant Cell Tissue Organ Cult. (PCTOC) 126, 177–186. doi: 10.1007/s11240-016-0988-3
Yoshikawa, M., Shimada, H., Shimoda, H., Murakami, N., Yamahara, J., Matsuda, H., et al. (1996). Bioactive constituents of Chinese natural medicines. II. Rhodiolae Yoshikawa Radix.(1). Chemical structures and antiallergic activity of Rhodio cyanosides A and B from the underground part of Rhodiola guadrifida (PALL.) FISCH. et MEY. (Crassulaceae). Chem. Pharm. Bull. 44, 1656–1662.
Zehra, M., Banerjee, S., Sharma, S., and Kumar, S. (1999). Influence of rhizogenes strains on biomass and alkaloid productivity in hairy root lines of Hyoscyamus muticus and H. albus. Plant Med. 65, 060–063. doi: 10.1055/s-1999-13964
Zhao, J., Hu, Q., Guo, Y. Q., and Zhu, W. H. (2001). Effects of stress factors, bioregulators, and synthetic precursors on indole alkaloid production in compact callus clusters cultures of Catharanthus roseus. Appl. Microbiol. Biotechnol. 55, 693–698. doi: 10.1007/s002530000568
Keywords: Artemisia vulgaris L., farnesyl diphosphate, gas chromatography–mass spectrometry, precursor treatment, essential oil
Citation: Balasubramani S, Ranjitha Kumari BD, Moola AK, Sathish D, Prem Kumar G, Srimurali S and Babu Rajendran R (2021) Enhanced Production of β-Caryophyllene by Farnesyl Diphosphate Precursor-Treated Callus and Hairy Root Cultures of Artemisia vulgaris L. Front. Plant Sci. 12:634178. doi: 10.3389/fpls.2021.634178
Received: 27 November 2020; Accepted: 15 February 2021;
Published: 30 March 2021.
Edited by:
Jens Rohloff, Norwegian University of Science and Technology, NorwayReviewed by:
Akbar Karami, Shiraz University, IranMuthu Thiruvengadam, Konkuk University, South Korea
Copyright © 2021 Balasubramani, Ranjitha Kumari, Moola, Sathish, Prem Kumar, Srimurali and Babu Rajendran. This is an open-access article distributed under the terms of the Creative Commons Attribution License (CC BY). The use, distribution or reproduction in other forums is permitted, provided the original author(s) and the copyright owner(s) are credited and that the original publication in this journal is cited, in accordance with accepted academic practice. No use, distribution or reproduction is permitted which does not comply with these terms.
*Correspondence: Sundararajan Balasubramani, c3VuZGFycGJ0ODdAZ21haWwuY29t; B. D. Ranjitha Kumari, cmFuaml0aGFrdW1hcmkyMDA0QHlhaG9vLmNvLmlu