- 1Brandon Bioscience, Tralee, Ireland
- 2Plant Biostimulant Group, Shannon Applied Biotechnology Centre, Munster Technological University Kerry, Tralee, Ireland
- 3Instituto de Ciências Agrárias, Universidade Federal de Uberlândia/UFU, Uberlândia, Brazil
- 4Instituto de Biotecnologia, Universidade Federal de Uberlândia/UFU, Uberlândia, Brazil
- 5Fundação Educacional de Ituverava FAFRAM, Faculdade Agronomia, Ituverava, Brazil
Soybean is one of the most valuable commercial crops because of its high protein, carbohydrate, and oil content. The land area cultivated with soybean in subtropical regions, such as Brazil, is continuously expanding, in some instances at the expense of carbon storing natural habitats. Strategies to decrease yield/seed losses and increase production efficiency are urgently required to meet global demand for soybean in a sustainable manner. Here, we evaluated the effectiveness of an Ascophyllum nodosum extract (ANE), SealicitTM, in increasing yields of different soybean varieties, in two geographical regions (Canada and Brazil). In addition, we investigated the potential of SealicitTM to reduce pod shattering at the trials in Brazil. Three different concentrations of SealicitTM were applied to pod shatter-susceptible (SS) UFUS 6901 and shatter-resistant (SR) UFUS 7415 varieties to assess their impact on pod firmness. SS variety demonstrated a significant decrease in pod shattering, which coincided with deregulation of GmPDH1.1 and GmSHAT1–5 expression, genes that determine pod dehiscence, and higher seed weight per pod. SealicitTM application to the SR variety did not significantly alter its inherent pod shatter resistance, but provided higher increases in seed yield at harvest. This yield increase maybe associated with to other yield components stimulated by the biostimulant. This work demonstrates that SealicitTM, which has previously been shown to improve pod firmness in Arabidopsis and selected commercial oilseed rape varieties through IND gene down-regulation, also has the potential to improve pod resistance and seed productivity in soybean, a member of the legume family sharing a similar strategy for seed dispersal.
Introduction
The increased food production requirement, in the context of limited availability of arable land and a climate emergency driven by greenhouse gas emissions, makes the development of more efficient agricultural production a necessity. At the same time, consumer trends for consumption of more plant protein and less meat result in surging demand for plant proteins and oils that are very efficiently produced by legumes, especially soybean. Since 1961, the world production of soybean has increased 13-fold through the rapid expansion of the production area (from 23.8 to 124.9 Mha) and a significant boost of average harvested seed yield (from 1,128 to 2,791 kg/ha). In 2018, United States (35%), Brazil (34%), and Argentina (11%) produced approximately 80% of the world’s harvested soybean, which is equivalent to almost 350 Mt (FAO, 2018) and steadily growing.
Soybean (Glycine max) is a relatively young crop; it is estimated that it was domesticated only 3,000–5,000 years ago in China (Prince et al., 2020), with subsequent cultivation spreading to other Asian regions. It is believed that during that time soybean acquired a number of shatter-resistant genes to impair an evolutionary-conserved seed dispersal mechanism (Dong et al., 2014). Current crops have been optimized for desired traits, starting from the selection by first farmers (Meng et al., 2013), through genome-wide association analysis (GWAS) combined with bioinformatics (Moreira et al., 2019). Independently of the method, the decrease of seed loss by dispersal and maximization of seed production was always the main goal. The major discoveries revealing the mechanism of seed dispersal are mostly attributed to the acquisition of Arabidopsis thaliana as a model plant system for fundamental research. Arabidopsis, a member of the Brassicaceae, develops a characteristic dry dehiscent fruit composed of two fused carpels that shatter the seeds through breaking of the dehiscence zones, along the silique, after maturity (Ferrándiz, 2002; Łangowski et al., 2016). Apart from Arabidopsis, several important genes involved in pod shattering, namely, IND, ALC, SHP1, SHP2, and FUL, and their complex regulatory network involved in pod dehiscence, were identified in a number of other members of the brassicas (Liljegren et al., 2000, 2004; Rajani and Sundaresan, 2001; Lewis et al., 2006; Girin et al., 2011; Pabón-Mora et al., 2014; Balanzà et al., 2016) such as Capsella rubella (Eldridge et al., 2016; Dong et al., 2019) and Brassica juncea (Østergaard et al., 2006), rapa (Mongkolporn et al., 2003), or napus (Raman et al., 2014), as well as in rice and soybean (Konishi et al., 2006; Li et al., 2006; Suzuki et al., 2010; Funatsuki et al., 2014; Yoon et al., 2014; Dong and Wang, 2015). In contrast to the bicarpellate silique of crucifers, legumes (including soybean) develop a fruit consisting of a single carpel fused on both sides. The pod walls, composed of several layers and cell types, are connected along the ventral and dorsal sutures. The opening of the soybean pod is triggered by the tension built inside the senescing pod and starts on the dorsal side (Tiwari and Bhatia, 1995; Zhang et al., 2018). Dong et al. (2014) found that excessive lignification of fiber cap cells (FCC) conferred the shatter-resistant phenotype. This process is controlled by SHATTERING1–5 (SHAT1–5), homologs to AtNST1/2, which is known to promote secondary wall biosynthesis. The same study compared gene expression between the wild allele and domesticated soybean, revealing that SHAT1–5 was 15-fold higher expressed than its wild counterpart. In parallel, Funatsuki et al. (2014) reported another major QTL (quantitative trait locus) involved in soybean pod shattering. Complementation assays showed that POD DEHISCENCE1 (GmPDH1.1) was found to be highly expressed in inner sclerenchyma, which correlated with lignin deposition. GmPDH1.1 encodes a dirigent-like protein involved in lignin biosynthesis (Suzuki et al., 2010) and pod dehiscence by increasing the twisting force in the pod wall (Dong and Wang, 2015). Therefore, the loss-of-function of Gmpdh1 has been widely used as a shattering-resistant gene in soybean breeding (Funatsuki et al., 2014). Additionally, more than half of Chinese landraces and most of South Asian landraces were shown to possess PDH1 and its shatter-resistant pdh1 allele, which suggested that pdh1 was indispensable to effectively grow soybean in a dry climate (Kaga et al., 2012; Funatsuki et al., 2014). The soybean pod is designed to withstand the natural fluctuations of humidity and temperature; however, the longer it stays in the field and the more cycles of wetting/drying occur, the higher the chance the pods will burst open with loss of the seeds to the soil. Unfortunately, because of rapidly changing weather conditions, with unexpectedly long periods of drought or rainfall and therefore delayed harvest, soybean shattering in the field, or during harvest, are becoming more frequent. These factors have been directly correlated to higher losses (Menezes et al., 2018; Zhang et al., 2018; Silveira et al., 2019), averaging 120 kg/ha in Brazil (Schanoski et al., 2011), or up to 319 kg/ha in the Southeastern United States (Philbrook and Oplinger, 1989).
In the last decade, a class of crop inputs known as plant biostimulants has gained significant attention for improving plant productivity. Plant biostimulants, especially those extracted from the brown alga Ascophyllum nodosum, have been reported to deliver a number of benefits to plants/crops (Craigie, 2011; Vera et al., 2011; Sharma et al., 2013; Ali et al., 2016; Goñi et al., 2016, 2018; Shukla et al., 2018, 2019; Carmody et al., 2020). Previously, we reported the efficacy of a specialty A. nodosum extract (ANE) biostimulant, namely, SealicitTM, and explored the mode of action (MOA) in reducing pod dehiscence in A. thaliana and winter oilseed rape (WOSR) (Łangowski et al., 2019). SealicitTM is a novel ANE containing PSI-759 biomolecule complex, produced using a targeted plant signal induction (PSI) approach to formulation development. In this study, the goal was to test the efficacy of SealicitTM in increasing yield and conferring pod shattering resistance in another major podded crop, soybean. The impact of SealicitTM on soybean yield was assessed using a randomized block field trial design at two distinct geographical locations (Canada and Brazil) with four different varieties. Employing multiple experimental approaches, the impact of SealicitTM on plant and pod phenotypic traits and pod firmness were assessed for two Brazilian soybean varieties UFUS 6901 [shattering-susceptible (SS)] and UFUS 7415 [shattering-resistant (SR)] (Bicalho et al., 2019). In order to decipher the MOA at the molecular level, the expression of soybean pod shattering genes, GmPDH1.1 and GmSHAT1–5 were also evaluated. Overall, the current studies aimed to further support the efficacy of a specialty biostimulant from A. nodosum, with a defined composition, to reduce seed loss and increase yields in crops producing dry pods, bringing exciting opportunities for sustainable agriculture.
Materials and Methods
Study Site, Experimental Conditions, and Treatment Application
The field trials in Canada were conducted in 2017 on two commercial glyphosate herbicide-resistant varieties (McLeod R2 referred to as V1 and NSC Austin RR2Y referred to as V2 throughout the manuscript). V1 and V2 varieties were evaluated in Minto midwestern Ontario (located at 49°24′26″ N, 100°01′26″ W and 474-m altitude) and Elm Creek, Manitoba (located at 49°40′34″ N, 97°59′32″ W and 252-m altitude), respectively. V1 was seeded on May 17, 2017, and harvested on September 28, 2017. V2 variety was seeded on May 11, 2017, and harvested on September 29, 2017. The climate for both locations is classified as humid continental climate (Dfa) according the Köppen climate classification. A mean temperature of 15.8°C, ranging from 4.6 to 25.8°C, and a mean accumulated precipitation of 262.8 mm was recorded during the trial with V1 in Minto. The V2 trial was characterized by a mean temperature of 22.5°C, ranging from 5.2 to 27.5°C, and a mean accumulated precipitation of 284.5 mm. The soil type for clay loam and loamy sand for V1 and V2 field trials, respectively, and the previous crop in both sites was flax. V1 variety has an early cycle (124 days until harvest) with average Sclerotinia resistance and high seed yield potential (3.3 Mt/ha). V2 has an early cycle (124 days until harvest), superior white mold resistance, and high seed yield potential (3.2 Mt/ha). Soybeans were sown by mechanical drilling with a row space of 20 cm, at a seed rate of 140 and 100 kg/ha for V1 and V2, respectively. The pest management program consisted of the application of the herbicide Round-up (900 g/ha, Bayer) and the insecticide Matador (83 mL/ha; Syngenta).
SealicitTM is a proprietary PSI-759 ANE produced under high temperatures and alkaline conditions through a targeted PSI approach to formulation development and was provided by Brandon Bioscience (Tralee, Ireland). SealicitTM was applied at 1.5 L/ha by single foliar spray at vegetative stage V2–V3, which corresponds to the stage 14–16 according to the soybean BBCH scale (SoyBase, 2020). This system is used for uniform coding for phenologically similar growth stages of all monocotyledonous and dicotyledonous plant species (Hack et al., 1992). A portable CO2 spraying system at a constant pressure of 2.76 bars was used to apply an equivalent volume of 100 L/ha. Control plants were sprayed with an equal volume of water. The experimental design was a randomized block design with four replicates per condition and each plot was 9 m2.
The field experiments in Brazil were conducted at the FAFRAM experimental station located in the city of Ituverava-SP (20°00′00″ S, 47°47′20″ W and 631-m altitude) between December 2, 2019, and March 25, 2020. The climate is classified as a tropical wet and dry climate (Aw) according the Köppen climate classification. A mean temperature of 25.2°C, ranging from 13.6°C to 34.9°C, and a mean accumulated precipitation of 250.7 mm were recorded during the field trial period in the weather observing station INM759 of Ituverava [20°36′00″ S, 47°77′00″ W and 613-m altitude (Agritempo, 2020)]. The soil type was red clay latosol, and the previous crop grown in the field trial site was soybean. Both tested commercial soybean varieties were provided by the Soybean Improvement Program of the Federal University of Uberlândia (UFU, 2020). SS variety (UFUS 6901) has a very early cycle (108 days until harvest) with resistance to the nematode Pratylenchus brachyurus and high seed potential yield (3.9 Mt/ha). SR variety (UFUS 7415) has an early cycle (110 days until harvest) and is highly resistant to Asian soybean rust (Phakopsora pachyrhizi) with tolerance to drought stress and high seed potential yield (4.3 Mt/ha) (Hamawaki et al., 2018). Soybeans were sown by mechanical drilling with a row space of 50 cm, at a seed rate of 65.3 kg/ha, on December 2, 2019, with the fertilizer application of 340 kg/ha of formulated 04-14-08 (NPK). The pest management program consisted of the application of the broad-spectrum insecticide Kaiso (100 mL/ha; Nufarm), the post emergent herbicide Dribble (400 mL/ha; Sumitomo), and the systemic fungicide Fox (500 mL/ha; Bayer). SealicitTM was applied by single foliar spray at vegetative stage V5–V7, before the emergence of the inflorescence, which corresponds to stages 16–18 according to the soybean BBCH scale. Dosage rates were 0.75, 1.5, and 3.0 L/ha of SealicitTM. A portable CO2 spraying system at a constant pressure of 2.1 bars was used to apply an equivalent volume of 200 L/ha. Control plants were sprayed with an equal volume of water. The distance between the spray lance and the plants was 50 cm, promoting a better cover of the sprayed biostimulant on the leaf tissue. The experimental design was a randomized block design in a 4 × 2 factorial design (number of treatments × number of varieties) with 13 replicates per condition (156 plots per field trial). The plots measured 2 m wide by 5 m long (10 m2) and had four rows. These rows were spaced 0.5 m; 0.5-m buffer zones were established for each plot, and only a central 4 m2 was used for phenotypic determinations or sampling purposes. The final plant stand per plot averaged 43 for both soybean varieties.
Plant and Yield Measurements
In the Canadian trials, harvesting was performed using a calibrated plot combine, and final harvested plot yields were recorded for at least four independent biological replicates per variety and treatment with soybean seed yield extrapolated to kg/ha. In Brazil, plant and yield measurements were taken in at least eight independent biological replicates per variety and treatment. Three independent biological pod samples were collected per variety and treatment and pooled for further analysis. Plant height was measured from the surface of the soil to the end of the main stem (hypocotyl) at the stages of early flowering (R1; BBCH60) and full maturity (R8; BBCH89). Plant lodging was assessed visually according to the following scale (1–5); 1: almost all plants standing, 2: less than 25% plants show stem lodging, 3: 25–50% of plants show stem lodging, 4: 50–80% of plants show stem lodging, 5: 100% plants show stem lodging. The presence of Asian soybean rust disease pressure caused by the fungus P. pachyrhizi was assessed visually according a scale of one to five (1: no disease symptoms; 2: 25% plants with disease symptoms; 3: 50% plants with disease symptoms; 4: 75% plants with disease symptoms; 5: 100% plants with disease symptoms). Soybean plants at stage R8 were harvested manually and processed by a soybean threshing machine. The seed yield was determined from the central 4-m2 area of each plot. After weighing, the seed weight per plot was extrapolated to kg/ha.
Evaluation of Pod Shattering Resistance and Pod Phenotypic Traits
Soybean plants from the trials in Brazil were used for determination of pod firmness and evaluation of phenotypic traits. Before harvesting (114 days after sowing), five plants at full maturity (R8 stage or BBCH89 when the fruit ripening is complete) were picked randomly from each plot. Pods were collected randomly from these plants. In contrast to Arabidopsis and oilseed rape, where a mechanical test is the most common technique to measure pod firmness (Morgan et al., 2001), the oven drying method is the most convenient and widely accepted assessment of pod shattering resistance in soybean. The test implemented during the course of these field trials was based on peer-reviewed published methods with minor modifications (Funatsuki et al., 2014; Krisnawati and Adie, 2017; Krisnawatiorcid et al., 2020). First, harvested pod samples were allowed to equilibrate their moisture levels at room temperature. After this acclimatization period, pod length was measured using ImageJ software (available at1) with a minimum of 60 biological replicates (pods) per variety and treatment. Evaluation of pod shattering resistance and seed weight per pod was performed on five biological replicates with 20 pods per replicate. Pod shattering resistance was analyzed after incubating pod samples at 65°C for the time required to open ≥ 50% of the control pods (Figure 2). This time period was calibrated for both SS and SR varieties. Pod shattering was calculated as the percentage of open pods versus total number of pods. After completing the pod shattering resistance test, the same dried soybean pods were used to determine the average seed weight per pod.
RNA Isolation and qRT-PCR
RNA was obtained from soybean pods at stage R5 (BBCH 75–79), which corresponds to the stage 17b according to fruit developmental scale for the model plant A. thaliana (Smyth et al., 1990; Roeder and Yanofsky, 2006). Four green fruits per plant were collected from at least five plants per plot and immediately frozen in liquid nitrogen, ground, and stored in −80°C to prevent RNA degradation. All pods collected per plot were considered a single biological replicate. All samples were collected within 2 h of midday. For quantitative reverse transcriptase–polymerase chain reaction (qRT-PCR) analysis, at least three biological replicates of each treatment and variety were analyzed. Three technical replicates per biological replicate were analyzed. Expression analyses were performed by real-time PCR using a Roche LightCycler 96 System (Roche, United Kingdom). Quantitative PCR was performed using the LightCycler RNA Master SYBR Green I one-step kit (Roche, United Kingdom) according to the manufacturer’s instructions. The expression level of genes of interest was expressed in n-fold change and calculated according 2–ΔΔCT (Livak and Schmittgen, 2001). Primers used for qRT-PCR reactions are as follows: GmPDH1 FW: 5′-GAGGGAGGCGTTTTACGAC-3′; REV: 5′-GACGTGGCAACCATGACTC-3′ (Funatsuki et al., 2014); GmSHAT1–5 FW: 5′-GGAGAACCACCACAACACCA-3′; REV: 5′-GTCCGTGCCCATCTCTACTG-3′ (AHJ81058.1); GmCYP2 FW: 5′-CGGGACCAGTGTGCTTCTTCA-3′; REV: 5′-CCCCTCCACTACAAAGGCTCG-3′ (Jian et al., 2008). GmCYP2 was used as the reference gene for normalization.
Statistical Analysis
Statistics were evaluated with Sigma Plot 12 and Statgraphics Centurion XVI software. The seed yield differences between control and SealicitTM treatment for V1 and V2 were analyzed using t test at p ≤ 0.05. The effects of SealicitTM on pod shattering, GmPDH1 and GmSHAT1–5 gene expression was analyzed with one-way analysis of variance (ANOVA) by Tukey honestly significant difference (HSD) test at p ≤ 0.05. The rest of plant data were compared by using two-way ANOVA, with Tukey’s HSD test at p ≤ 0.05. Where the interaction between the two factors, variety (SS and SR) and SealicitTM treatment (0, 0.75, 1.5, and 3 L/ha) (V × S), was significant, data were subjected to one-way ANOVA, comparing all SealicitTM treatments with each other within the same soybean variety. Where V × S interaction was not significant, the effects of variety and SealicitTM treatments were evaluated separately, comparing the respective means through t test (variety) or one-way ANOVA Tukey’s HSD test (SealicitTM treatment) at p ≤ 0.05. The application of all parametric tests was performed after checking the normality of the data (Shapiro–Wilk test) and equal variance assumptions. Unless stated otherwise, all data are expressed as average ± standard error (SE). Details of the individual sample size for each analysis are mentioned in the tables and figure legends.
Results
Effects of SealicitTM on Soybean Seed Yield
The Canadian trial seed yields for control plots of both tested varieties (V1: 3,241 kg/ha; V2: 3,388 kg/ha) were similar to average values obtained by Canadian growers in the same growing season (V1: 3,256 kg/ha; V2: 3,188 kg/ha) (Manitoba Pulse Soybean Growers, 2017). The application of SealicitTM in V1 at vegetative stage did produce a small statistically nonsignificant yield increase (+1.08%; p = 0.796). However, the V2 variety had a larger increase in yield of 4.91% (p = 0.207), producing 164 kg/ha more than control plots (Figure 1). The impact of SealicitTM on yield in the Brazilian trials was also evaluated. A two-way ANOVA analysis demonstrated that SealicitTM treatment had a statistically significant effect on seed yield (p = 0.026). This parameter was also highly affected by the interaction variety x SealicitTM (p = 0.047) (Table 1). The SS variety had increased seed yield of 6.6% (p = 0.078) and 6.2% (p = 0.154) at low (0.75 L/ha) and high (3 L/ha) doses, respectively. Only a minor increase of 2.2% (p = 0.555) was recorded at the 1.5 L/ha rate for the SS variety. SealicitTM provided a more pronounced positive yield effect on the SR variety when it was applied at low (13.1%; p = 0.047) and medium (17.9%, p = 0.016) doses, respectively. However, only a small increase was recorded for the high SealicitTM dose (1.9%; p = 0.774).
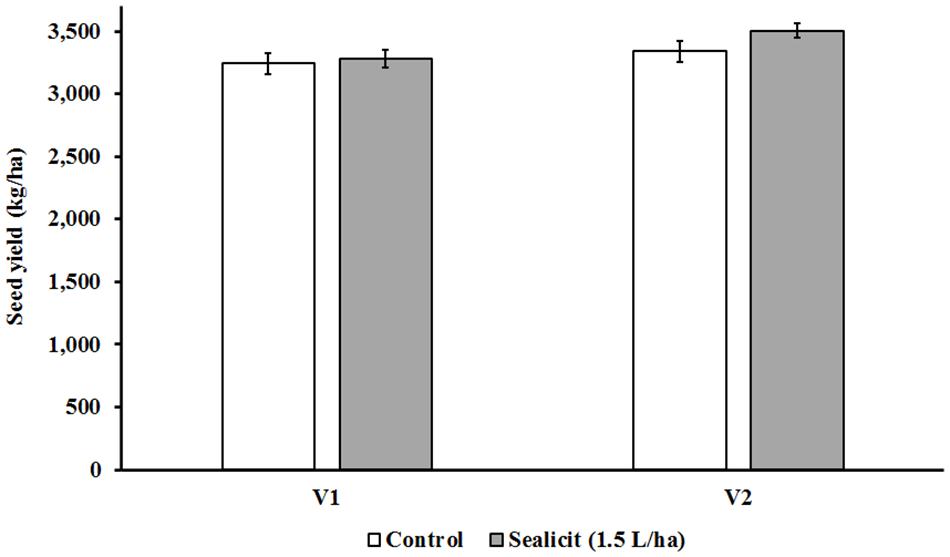
Figure 1. Effects of SealicitTM on seed yield in two commercial soybean varieties with glyphosate herbicide resistance. SealicitTM was applied by foliar spray (1.5 L/ha) at vegetative stage (V2–V3), and its performance was benchmarked against untreated plants. Field trials with V1 and V2 were carried out in Minto (ON, Canada) and Elm Creek (MB, Canada) in 2017 under standard grower practice, respectively. Each bar represents seed yield (kg/ha). Number of biological replicates (n = 4).
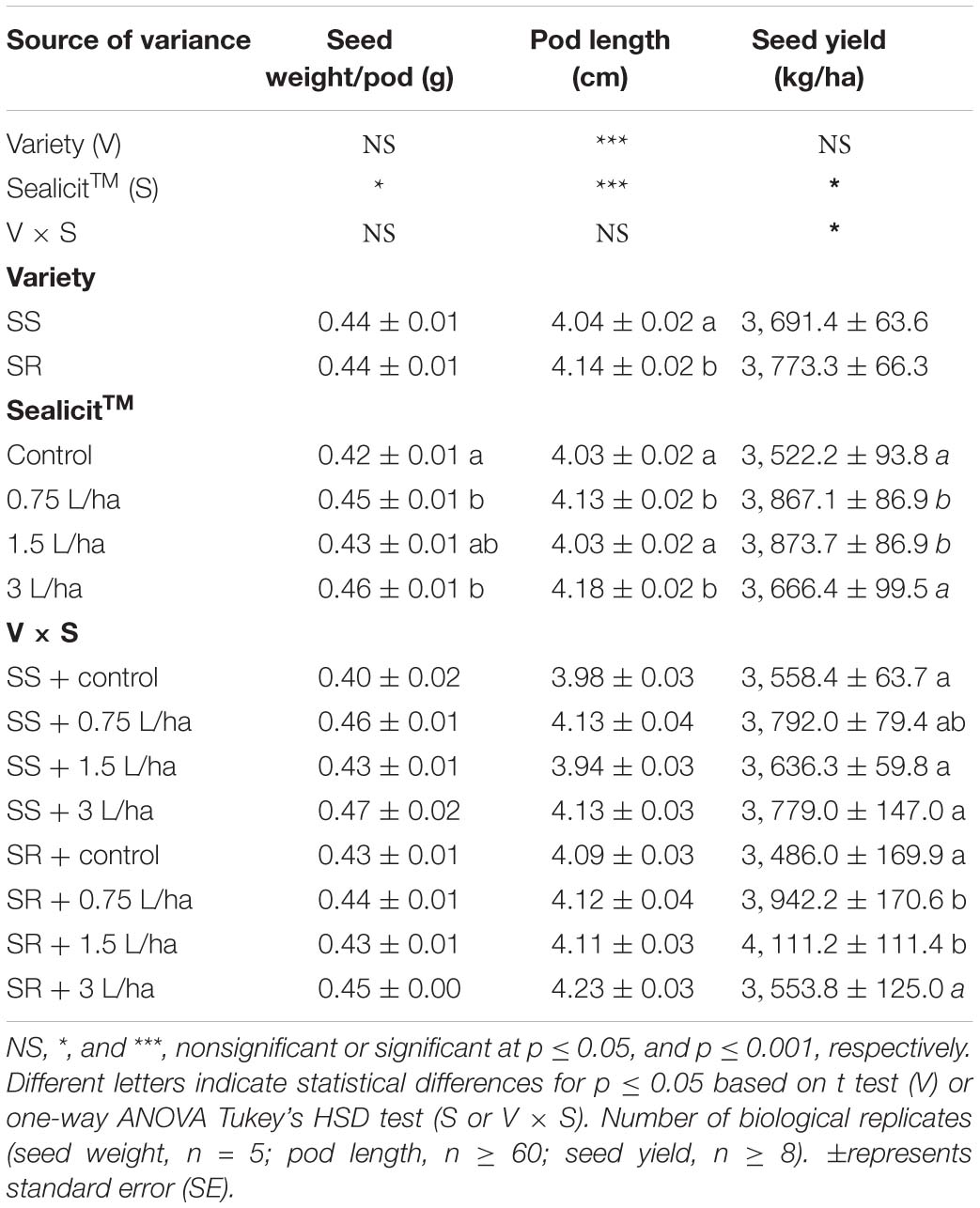
Table 1. Analysis of variance and mean comparisons for fresh seed weight per pod, pod length, and harvested seed yield at full maturity stage (R8) with two commercial soybean varieties treated with water and three concentrations of SealicitTM.
The Effects of SealicitTM Treatment on Soybean Plant Development
The impact of soybean variety and SealicitTM treatment on a number of plant phenotypic parameters including height, degree of lodging, and the prevalence of Asian soybean rust was assessed in both Brazilian varieties. A two-way ANOVA test revealed that in conjunction both parameters (variety × SealicitTM) had no significant effect on height or lodging parameters (Table 2). The SS plants showed an overall statistically significant increase of plant height at flowering (+9.2%; p = 0.006) and full maturity (+9.8%; p ≤ 0.001) stage compared to SR plants. SS plants had increased their lodging degree 1.6 times compared to SR plants (p ≤ 0.001); however, the absolute values of this parameter confirmed that less than 25% of the soybean plants were not erect. The effect of SealicitTM on plant height and the degree of lodging were not statistically significant with respect to the control. Finally, soybean plants did not show any symptoms of Asian rust disease indicating the quality of the field trial (Table 2).
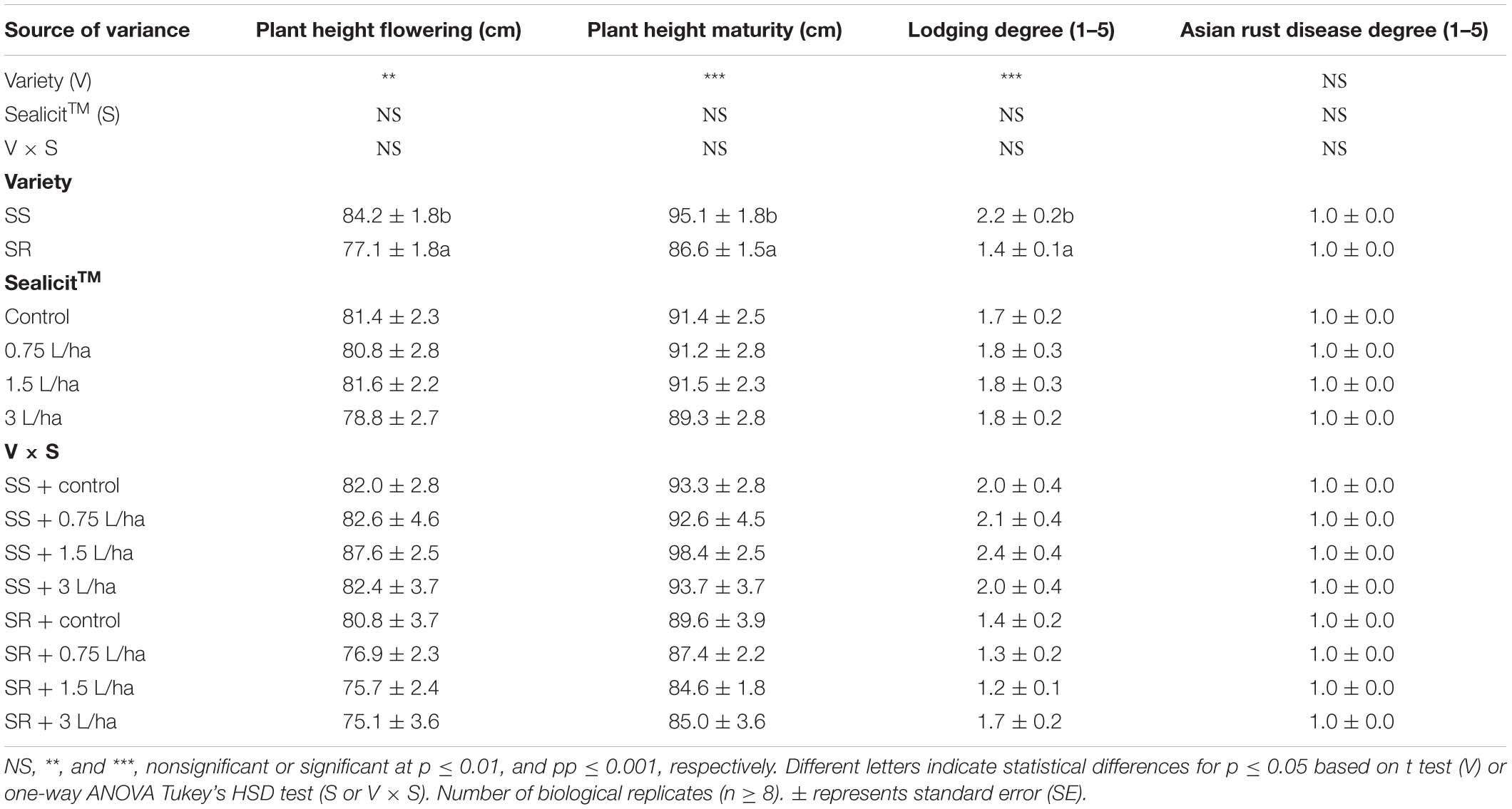
Table 2. Analysis of variance and mean comparisons for plant height at flowering (R1) and full maturity (R8) stage, lodging degree, and degree of Asian rust disease with two commercial soybean varieties, treated with water and three concentrations of SealicitTM.
Effects of SealicitTM on Pod Morphology
In order to assess whether SealicitTM had any impacts on soybean pod growth and development, as reported previously for Arabidopsis and WOSR (Łangowski et al., 2019), the average fresh seed weight per pod and pod length were measured in SS and SR varieties. A two-way ANOVA revealed that SealicitTM had a significant effect on the average fresh seed weight per pod (p = 0.024) (Table 1). In comparison to the control, the average fresh seed weight was the highest at 0.75 L/ha with the 3 L/ha application rate (7.1 and 9.5%), followed by a lower yet significant increase at the 1.5-L/ha application rate (2.4%). The two-way ANOVA test did not show any significant interactions across varieties and SealicitTM treatments on pod length (p = 0.060). However, there was a significant increase in pod length in the SR, when compared to the SS variety (p ≤ 0.001). When these differences were examined in detail, there were also significant differences between the treated and untreated plants (p ≤ 0.001). Similarly, to seed weight per pod, the low and higher application rates, for both tested varieties, positively affected the pod length, which was longer by 2.5 and 3.4% as compared to control, respectively (Table 1).
Effects of SealicitTM on Pod Shattering Resistance
The effect of SealicitTM and control treatments on the shatter resistance of harvested pods from the Brazilian field trial was assessed. As expected, the SS variety required a shorter calibration time (4 h) compared to the SR variety (48 h), demonstrating the sensitivity of the method used. Interestingly, when applying the same incubation times for the treated samples, we observed a strong effect of SealicitTM on SS with very few pods bursting compared to the controls across all treatments (p ≤ 0.001). This suggested that the lowest dose (0.75 L/ha) was sufficient to confer shattering resistance to the susceptible variety by decreasing pod opening by nearly fivefold. The SR samples showed minor decreases in shatter at low and high doses, but a slight increase at 1.5 L/ha dose; however, these fluctuations were not statistically significant (Figure 2).
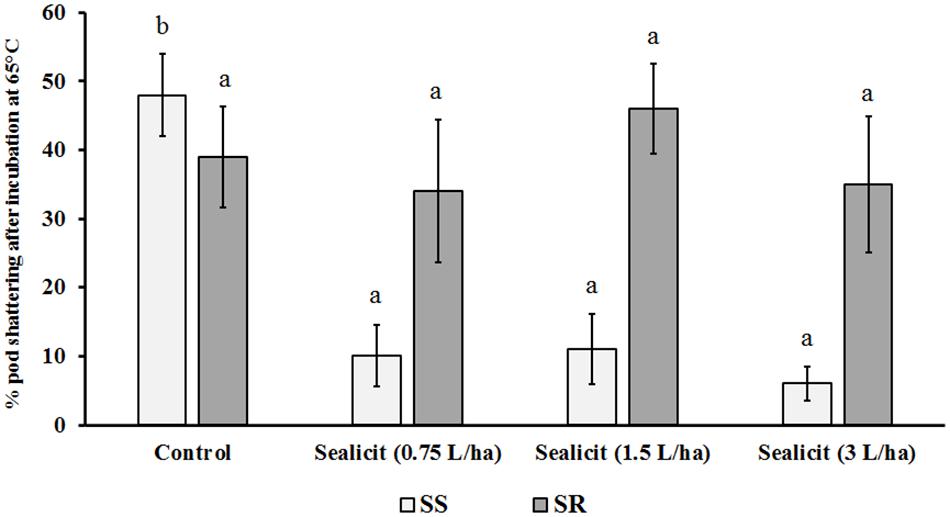
Figure 2. Effects of SealicitTM rate on soybean pod shattering. Each bar represents the percentage of open pods versus total number of pods. Different letters within the same soybean variety indicate statistically significant differences between the treatments based on one-way ANOVA Tukey’s HSD test at p ≤ 0.05. Number of biological replicates (n = 5).
SealicitTM Affects the Development of Dehiscence Zone
To further assess the impact of SealicitTM on pod shatter, the expression level of two major genes regulating pod dehiscence, GmPDH1.1 (active in inner sclerenchyma) and GmSHAT1–5 (active in FCCs), was examined (Funatsuki et al., 2014). The relative gene expression change was measured in reference to the housekeeping gene CYCLOPHILIN2 (CYP2) (Jian et al., 2008). Funatsuki et al. (2014) showed that the transcript level of GmPDH1.1, which is involved in secondary wall biosynthesis and lignin deposition, decreased due to an early stop codon mutation, which is characteristic for pod-shatter resistant varieties. In our current studies, we observed that SealicitTM significantly and gradually decreased GmPDH1.1 expression in a concentration-dependent manner, in the variety SS (Figure 3). However, the expression analysis performed in the SR variety showed the opposite trend, which supports a variety-dependent effect. Next, the expression level of GmSHAT1–5, which similarly to GmPDH1 is involved in secondary wall biosynthesis, was examined. Interestingly, the transcript level in pods collected from treated plants was increased in both varieties (Figure 4). The SR variety had a small increase that was statistically significant at the highest dose. On the other hand, a significant increase (1.6-fold) was observed for the SS variety, which is in agreement with GmPDH1 expression level and the pod firmness test (Figure 3).
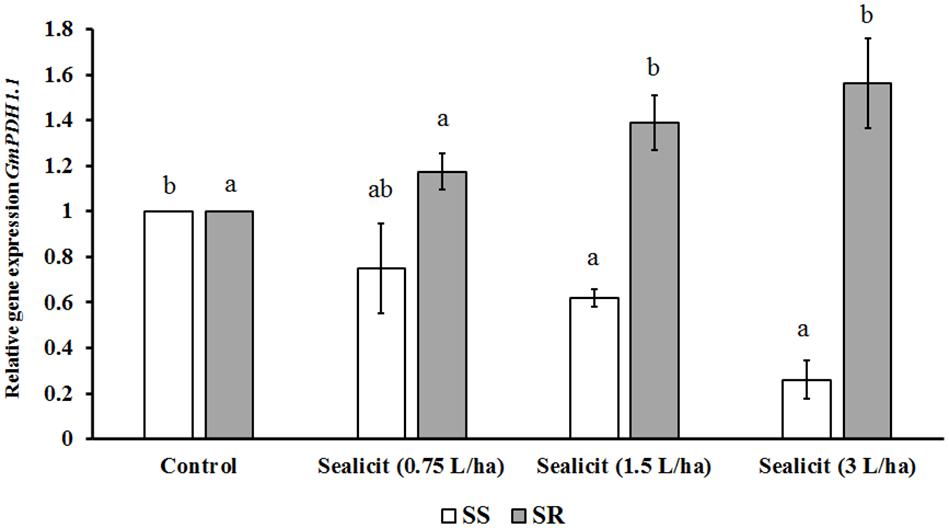
Figure 3. Relative expression of GmPDH1.1 in soybean pods treated with SealicitTM. Different letters within the same soybean variety indicate statistically significant differences between the treatments based on one-way ANOVA Tukey’s HSD test at p ≤ 0.05. Number of biological replicates (n = 3).
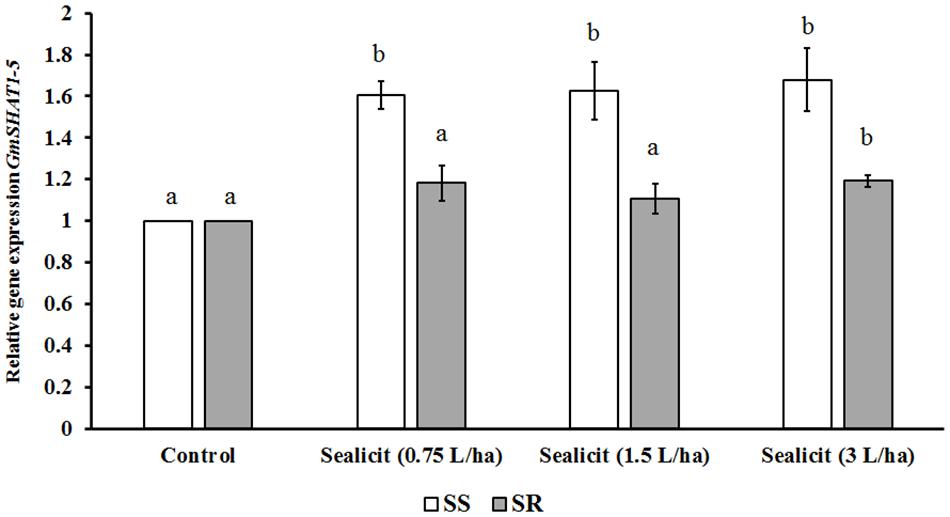
Figure 4. Relative expression of GmSHAT1–5 in soybean pods treated with SealicitTM. Different letters within the same soybean variety indicate statistically significant differences between the treatments based on one-way ANOVA Tukey’s HSD test at p ≤ 0.05. Number of biological replicates (n = 3).
Discussion
Soybean (G. max) is one of the most important global crops because of its high protein and fat content. Its broad use for the production of food, oil, and fodder creates a continuously growing demand, having serious implications for the natural environment (Kocira et al., 2019). In order to reduce the impact of agricultural-related deforestation and pollution, new solutions for more efficient and sustainable soybean production are urgently needed. In our previous work, we have shown that ANE biostimulant SealicitTM is capable of reducing pod shattering, influencing fruit development and yield in the model plant system Arabidopsis, as well as selected varieties of WOSR (Łangowski et al., 2019). Following on from the previous detailed analysis of SealicitTM action, we have tested whether similar effects can be observed in soybean grown in field conditions. In this study, the impact of SealicitTM on soybean productivity and pod shattering, as assessed by (i) testing pod-shatter in low humidity conditions and (ii) analyzing the expression level of major genes determining pod shattering, was determined. Productivity parameters were complemented with morphological analysis of plant height, seed weight per pod, pod length, and finally seed yield assessment. Here, we demonstrated that the same biostimulant can be successfully utilized not only in the Brassicaceae family species but also legumes, providing a new solution for a pressing problem.
Soybean Yield Evaluation
SealicitTM field testing in Canada and Brazil using a randomized block field trial design showed consistent and agronomically sound yield results (Table 1 and Figure 1). V1 and V2 seed yield values in control plots were very similar to average values obtained by Canadian growers in the same growing season (V1: 3,256 kg/ha; V2: 3,188 kg/ha, respectively) (Manitoba Pulse Soybean Growers, 2017). The effect of SealicitTM appears to be variety specific, which is similar to that previously observed for WOSR. The yield increase for V2 was 4.91% with a p = 0.207. Although the p value did not meet the typical 0.05 scientific threshold, the magnitude of the yield increase was agronomically interesting, and it provided a basis for running additional trials with a larger number of replicates to reduce the p value. It should be noted that little information is available on the susceptibility of these short-cycle Canadian varieties to pod shatter, so additional trials were performed with soybean varieties with known shatter susceptibility to investigate the potential of SealicitTM in this crop. The harvested seed yields at full maturity for the Brazilian varieties SS and SR were in the range expected in previous field trials developed in four consecutive sowing seasons in Uberlândia-MG (Bicalho et al., 2019). All treated soybean varieties with SealicitTM produced increased yield with respect to their untreated counterparts. Yield increases were statistically significant with p ≤ 0.05 for treatments, likely due to the higher replicate numbers versus the Canadian trials. It is evident that there was a significant interaction between the dose (0.75 and 1.5 L/ha) and the yield for the SR variety, which was not the case for the SS variety. Therefore, dose optimization on specific varieties may be important to gain maximum benefit from SealicitTM in soybean crops.
Impact of SealicitTM Treatments on Soybean Architecture and Pod Phenotype
While the SS variety was significantly taller than the SR variety, SealicitTM did not demonstrate an ability to influence plant height. From published work on ANEs in soybean and tomato plants, we have learned that this class of plant biostimulants did not have a statistically significant effect on plant height despite yield increase (Guerreiro et al., 2017; Carmody et al., 2020). It indicates that this phenotypic trait may not be sufficient to predict yield benefits provided by ANE biostimulants. We have previously reported that SealicitTM is able to increase the pod weight in Arabidopsis and seed weight of some WOSR varieties (Łangowski et al., 2019). Here we observed a substantial seed weight increase in both soybean varieties tested. This result is contrary to data published by Kocira et al. (2019), which presents a 1,000-soybean-seed weight decrease after foliar spray with a nonspecialty seaweed extract from Ecklonia maxima. This finding highlights the importance of the seaweed specie and the process used for extraction on bioactivity-related parameters of seaweed extract biostimulants (Goñi et al., 2016, 2018; Shukla et al., 2019; Carmody et al., 2020). Moreover, both soybean varieties showed an increased pod length, which is in agreement with SealicitTM ability to dysregulate FUL, RPL, and IND expression and pod length in Arabidopsis and some WOSR varieties (Łangowski et al., 2019), suggesting that this specialized ANE biostimulant may also modulate soybean pod auxin homeostasis and signaling, crucial for fruit growth and development (Liljegren et al., 2004; Sorefan et al., 2009; Dong et al., 2019).
SealicitTM Impact on Pod Shattering Resistance and Pod Dehiscence Genes
Data derived from multiple trials in commercial WOSR varieties demonstrated a positive association between pod firmness and yield (Łangowski et al., 2019). Soybean pod architecture and dehiscence mechanism show significant differences in relation to Brassicaceae fruits, yet the principle of seed dispersal is still relying on lignin deposition that determines physical resistance (Dong et al., 2014; Funatsuki et al., 2014). Pod shattering resistance tests performed on soybean control pods showed variability in treated varieties, ranging from 4 h for SS to 48 h for SR, proving the robustness and sensitivity of the method. The difference in calibration time was to be expected as both varieties are genetically diverse (Bicalho et al., 2019). On the other hand, the SR variety, classified as resistant to shatter, showed no statistically significant improvement after SealicitTM treatments, whereas the SS variety, classified as susceptible to pod shattering, showed a very significant fruit firmness increase across all the doses applied. In addition, SealicitTM decreased GmPDH1.1 and increased GmSHAT1–5 expression in the SS variety, which is consistent with a reduction in shatter. Moreover, GmPDH1.1 transcript levels were decreasing with increasing SealicitTM concentration in SS variety, yet increasing in SR, indicating that the effect is dose and genome/variety dependent. It is important to note that the SR variety, despite showing insignificant increase in GmPDH1.1 transcript level, also showed slight increase in GmSHAT1–5. Taking into account that the transcript levels of both might be extremely different, a small increase in SR GmSHAT1–5 transcript could not only counteract the insignificant increase of GmPDH1, but also account for a small increase of SR in pod firmness test (Figure 2). Therefore, it is tempting to speculate that pod firmness in soybean fruit is a balance between the amount of the lignin deposited in the FCCs and inner sclerenchyma, which may be determined by multiple genes that ultimately regulate the dorsoventral tension. In order to gain an in-depth understanding of the mechanism and critical signaling pathways, transcriptome and proteome analysis of multiple genes involved in fruit development (i.e., FUL, RPL, IND, SHP, and ALC) (Ferrándiz, 2002), their mutants, and complementation lines are necessary (Dong and Wang, 2015). Most importantly, the enhanced productivity of SS was positively associated with GmPDH1.1 and GmSHAT1–5 gene expression, increased pod shattering resistance, and higher seed weight per pod. However, the seed yield increase in treated SR soybean plants was not clearly associated with any of the parameters evaluated in this study, being likely due to additional effects promoted by SealicitTM that have not been the subject of investigation. As observed previously by Guerreiro et al. (2017), foliar applications of ANE biostimulant can stimulate additional yield-related parameters in soybean such as increased number of pods per plant, which could be one of the contributing factors. Briglia et al. (2019) also showed an improvement in soybean biovolume and green area after foliar spray treatment with different combinations of seaweed and plant extracts. Those additional benefits of ANE application could potentially account for increased seed weight and fresh seed yield in the SR variety.
Agronomical and Environmental Implications
The gradually increasing soybean production (by 4–5% annually) in Brazil means it will become the biggest world producer with an estimated 129 Mt in season 2020/2021. The harvested area is forecasted at a record 36.8 Mha, up by 1.7 Mha from last season’s record (USDA, 2020). While soybean production in Brazil is growing, losses due to adverse weather conditions become more frequent (Rowntree et al., 2013; Van Roekel et al., 2015; Zhang et al., 2018). The Brazilian Agricultural Research Corporation (EMBRAPA) has established that one 60-kilo bag per hectare is an acceptable loss threshold value (Silveira et al., 2019). However, in the last 30 years, a wide range of yield losses (54–561 kg/ha) has been recorded in different regions of Brazil due to adverse weather events and delayed and/or inadequate mechanical harvesting (Chioderoli et al., 2012; Barbosa and Schmitz, 2015; Bandeira, 2017), averaging 120 kg/ha loss at a national level (Schanoski et al., 2011). In this study, we have demonstrated that this lost yield can be recovered and safeguarded using the ANE biostimulant SealicitTM at a low dose rate (0.75 L/ha), increasing seed yield by an average of 9.8% in two commercial soybean varieties (+344.9 kg/ha). The use of the biostimulant would allow production output to grow in a sustainable manner contrary to expanding crop area. It is important to note that the yield uplift that can be obtained with SealicitTM application provides a compelling return on investment for the grower (>3:1). According to the current average market price of soybean, growers will be able to obtain added revenues of $135/ha by applying SealicitTM in the Brazilian market, which creates a strong financial incentive for the growers to switch to a more environment-friendly and sustainable agriculture.
Conclusions and Future Perspectives
ANE-based biostimulants are a rapidly growing crop input, because of their efficacy in a number of applications in modern agriculture. The investigations reported were undertaken to determine if SealicitTM has an impact on pod development in soybean. The data generated suggest that SealicitTM has a strong antishattering effect in a non–shatter-resistant soybean variety. It is also increasingly evident that SealicitTM treatments, despite impacting on pod dehiscence, may have in some species and varieties accompanying effects stimulating average seed weight per pod and pod length, which ultimately reflects on yield. In order to gain a better understanding of SealicitTM’s MOA in soybean, lignin staining and transversal sections presenting fruit morphology and lignin deposition could be further analyzed. Pod shattering is determined by multiple contributing genes, which makes their identification challenging even by GWAS. Therefore, a lack of sufficient understanding of physiological and genetic mechanisms hinders improvements of such traits by intensive breeding or genome editing (Verdugo et al., 2010; Korte and Farlow, 2013). SealicitTM appears to provide a solution for a yield reducing concealed developmental trait that is pod shattering, not only in oilseed rape but also in soybean and possibly other species relying on similar, conserved, seed dispersal mechanisms. Biostimulants that mobilize genetic plant potential to achieve high-quality crop with maximum yield represent an attractive tool to currently applied methods for efficient food production.
Data Availability Statement
The original contributions presented in the study are included in the article.
Author Contributions
ŁŁ, OG, JG, OH, and SO’C conceived and designed the experiments. ŁŁ, FM, MT, MP, CS, and AN performed the experiments. ŁŁ, OG, OH, FM, and SO’C analyzed the data. ŁŁ, OG, and SO’C wrote the article. All authors contributed to the article and approved the submitted version.
Funding
This research has been conducted with the assistance of funding from the INNOWIDE call of the EU-Horizon 2020 Program under grant agreement No. 2019-1591 (SUSBEAN project).
Conflict of Interest
The authors declare that the research was conducted in the absence of any commercial or financial relationships that could be construed as a potential conflict of interest.
Acknowledgments
The authors would like to acknowledge the support of Luiz Ricardo Goulart’s research group in the Nanobiotechnology Laboratory of the Federal University of Uberlândia-UFU for providing the equipment necessary for isolating RNA. The authors would also like to thank Brandon Bioscience for the gift of the ANE-based biostimulant SealicitTM used in this study.
Footnotes
References
Agritempo (2020). Agromteorological Monitoring Sytem. Available online at: http://www.agritempo.gov.br/agritempo/index.jsp (accessed May 17, 2017).
Ali, N., Farrell, A., Ramsubhag, A., and Jayaraman, J. (2016). The effect of Ascophyllum nodosum extract on the growth, yield and fruit quality of tomato grown under tropical conditions. J. Appl. Phycol. 28, 1353–1362. doi: 10.1007/s10811-015-0608-3
Balanzà, V., Roig-Villanova, I., Di Marzo, M., Masiero, S., and Colombo, L. (2016). Seed abscission and fruit dehiscence required for seed dispersal rely on similar genetic networks. Development 143, 3372–3381. doi: 10.1242/dev.135202
Bandeira, G. J. (2017). Perdas na Colheita da Soja em Diferentes Velocidades de Deslocamento da Colhedora.
Barbosa, E. J. A., and Schmitz, R. (2015). Avaliação de Perdas na Colheita da Cultura da Soja na Região Noroeste do rio Grande do sul. Available online at: https://docplayer.com.br/31039598-Avaliacao-de-perdas-na-colheita-dacultura-da-soja-na-regiao-noroeste-do-rio-grande-do-sul-erlei-jose-alessiobrabosa-1-ricardo-schmitz-2.html (accessed November 17, 2020).
Bicalho, T. F., Nogueira, A. P. O., Hamawaki, O. T., Costa, S. C., Júnior, I. J. D. M., Silva, N. S., et al. (2019). Adaptability and stability of soybean cultivars in four sowing seasons. Biosci. J. 35, 1450–1462. doi: 10.14393/BJ-v35n5a2019-42351
Briglia, N., Petrozza, A., Hoeberichts, F. A., Verhoef, N., and Povero, G. (2019). Investigating the impact of biostimulants on the row crops corn and soybean using high-efficiency phenotyping and next generation sequencing. Agronomy, 9:761. doi: 10.3390/agronomy9110761
Carmody, N., Goñi, O., Łangowski, Ł, and O’connell, S. (2020). Ascophyllum nodosum extract biostimulant processing and its impact on enhancing heat stress tolerance during tomato fruit set. Front. Plant Sci. 11:807. doi: 10.3389/fpls.2020.00807
Chioderoli, C. A., Silva, R. P. D., Noronha, R. H. D. F., Cassia, M. T., and Santos, E. P. D. (2012). Perdas de grãos e distribuição de palha na colheita mecanizada de soja. Bragantia 71, 112–121. doi: 10.1590/s0006-87052012005000003
Craigie, J. S. (2011). Seaweed extract stimuli in plant science and agriculture. J. Appl. Phycol. 23, 371–393. doi: 10.1007/s10811-010-9560-4
Dong, Y., Jantzen, F., Stacey, N., Łangowski, Ł, Moubayidin, L., Šimura, J., et al. (2019). Regulatory diversification of indehiscent in the capsella genus directs variation in fruit morphology. Curr. Biol. 29, 1038–1046.e4. doi: 10.1016/j.cub.2019.01.057
Dong, Y., and Wang, Y. Z. (2015). Seed shattering: from models to crops. Front. Plant Sci. 6:476. doi: 10.3389/fpls.2015.00476
Dong, Y., Yang, X., Liu, J., Wang, B. H., Liu, B. L., and Wang, Y. Z. (2014). Pod shattering resistance associated with domestication is mediated by a nac gene in soybean. Nat. Commun. 5:3352. doi: 10.1038/ncomms4352
Eldridge, T., Langowski, L., Stacey, N., Jantzen, F., Moubayidin, L., Sicard, A., et al. (2016). Fruit shape diversity in the brassicaceae is generated by varying patterns of anisotropy. Development 143, 3394–3406. doi: 10.1242/dev.135327
FAO (2018). Food and Agriculture Organisation of United Nations. Available online at: http://www.fao.org/faostat/en/#data/QC (accessed November 11, 2020)
Ferrándiz, C. (2002). Regulation of fruit dehiscence in arabidopsis. J. Exp. Bot. 53, 2031–2038. doi: 10.1093/jxb/erf082
Funatsuki, H., Suzuki, M., Hirose, A., Inaba, H., Yamada, T., Hajika, M., et al. (2014). Molecular basis of a shattering resistance boosting global dissemination of soybean. Proc. Natl. Acad. Sci. U.S.A. 111, 17797–17802.
Girin, T., Paicu, T., Stephenson, P., Fuentes, S., Körner, E., O’brien, M., et al. (2011). Indehiscent and spatula interact to specify carpel and valve margin tissue and thus promote seed dispersal in arabidopsis. Plant Cell 23, 3641–3653. doi: 10.1105/tpc.111.090944
Goñi, O., Fort, A., Quille, P., Mckeown, P. C., Spillane, C., and O’connell, S. (2016). Comparative transcriptome analysis of two ascophyllum nodosum extract biostimulants: same seaweed but different. J. Agric. Food Chem. 64, 2980–2989. doi: 10.1021/acs.jafc.6b00621
Goñi, O., Quille, P., and O’connell, S. (2018). Ascophyllum nodosum extract biostimulants and their role in enhancing tolerance to drought stress in tomato plants. Plant Physiol. Biochem. 126, 63–73. doi: 10.1016/j.plaphy.2018.02.024
Guerreiro, J. C., Blainski, E., da Silva, D. L., Caramelo, J. P., Pascutti, T. M., de Oliveira, N. C., et al. (2017). Effect of the seaweed extract applied on seeds and/or foliar sprays on soybean development and productivity. J. Food Agric. Environ. 15, 18–21.
Hack, H., Bleiholder, H., Buhr, L., Meier, U., Schnock-Fricke, U., Stauss, R., et al. (1992). Einheitliche codierung der phänologischen entwicklungsstadien mono- und dikotyler pflanzen. – erweiterte bbch-skala, allgemein Nachrichtenbl. Deut. Pflanzenschutzd. 44, 265–270.
Hamawaki, O. T., Hamawaki, R. L., Nogueira, A. P. O., Glasenapp, J. S., Hamawaki, C. D. L., Juliatti, F. C., et al. (2018). Development of brazilian soybean cultivars well adapted to cerrado and rust-tolerant. Int. J. Adv. Eng. Res. Sci. 5, 130–136.
Jian, B., Liu, B., Bi, Y., Hou, W., Wu, C., and Han, T. (2008). Validation of internal control for gene expression study in soybean by quantitative real-time pcr. BMC Mol. Biol. 9:59. doi: 10.1186/1471-2199-9-59
Kaga, A., Shimizu, T., Watanabe, S., Tsubokura, Y., Katayose, Y., Harada, K., et al. (2012). Evaluation of soybean germplasm conserved in nias genebank and development of mini core collections. Breed. Sci. 61, 566–592. doi: 10.1270/jsbbs.61.566
Kocira, S., Szparaga, A., Kuboñ, M., Czerwiñska, E., and Piskier, T. (2019). Morphological and biochemical responses of glycine max (l.) merr. To the use of seaweed extract. Agronomy 9:93. doi: 10.3390/agronomy9020093
Konishi, S., Izawa, T., Lin, S. Y., Ebana, K., Fukuta, Y., Sasaki, T., et al. (2006). An snp caused loss of seed shattering during rice domestication. Science 312, 1392–1396. doi: 10.1126/science.1126410
Korte, A., and Farlow, A. (2013). The advantages and limitations of trait analysis with gwas: a review. Plant Methods 9:29. doi: 10.1186/1746-4811-9-29
Krisnawati, A., and Adie, M. M. (2017). Identification of soybean genotypes for pod shattering resistance associated with agronomical and morphological characters. Biosaintifika J. Biol. Biol. Educ. 9:8. doi: 10.15294/biosaintifika.v9i2.8722
Krisnawatiorcid, A., Soegianto, A., Waluyo, B., and Kuswanto, K. (2020). The pod shattering resistance of soybean lines based on the shattering incidence and severity. Czech J. Genet. Plant Breed. 56, 111–122. doi: 10.17221/20/2020-CJGPB
Łangowski, Ł, Goñi, O., Quille, P., Stephenson, P., Carmody, N., Feeney, E., et al. (2019). A plant biostimulant from the seaweed ascophyllum nodosum (sealicit) reduces podshatter and yield loss in oilseed rape through modulation of ind expression. Sci. Rep. 9:16644. doi: 10.1038/s41598-019-52958-0
Łangowski, Ł, Stacey, N., and Ostergaard, L. (2016). Diversification of fruit shape in the brassicaceae family. Plant Reprod. 29, 149–163. doi: 10.1007/s00497-016-0278-6
Lewis, M. W., Leslie, M. E., and Liljegren, S. J. (2006). Plant separation: 50 ways to leave your mother. Curr. Opin. Plant Biol. 9, 59–65. doi: 10.1016/j.pbi.2005.11.009
Li, C., Zhou, A., and Sang, T. (2006). Rice domestication by reducing shattering. Science 311, 1936–1939. doi: 10.1126/science.1123604
Liljegren, S. J., Ditta, G. S., Eshed, Y., Savidge, B., Bowman, J. L., and Yanofsky, M. F. (2000). Shatterproof mads-box genes control seed dispersal in arabidopsis. Nature 404, 766–770. doi: 10.1038/35008089
Liljegren, S. J., Roeder, A. H., Kempin, S. A., Gremski, K., Østergaard, L., Guimil, S., et al. (2004). Control of fruit patterning in arabidopsis by indehiscent. Cell 116, 843–853. doi: 10.1016/s0092-8674(04)00217-x
Livak, K. J., and Schmittgen, T. D. (2001). Analysis of relative gene expression data using real-time quantitative pcr and the 2(-delta delta c(t)) method. Methods 25, 402–408. doi: 10.1006/meth.2001.1262
Manitoba Pulse Soybean Growers (2017). Soybean Variety Guide. Available online at: https://www.manitobapulse.ca/wp-content/uploads/2017/11/2017-Soybean-Variety-Guide-FINAL_WR.pdf (accessed May 17, 2017).
Menezes, P. C. D., Silva, R. P. D., Carneiro, F. M., Girio, L. A. D. S., Oliveira, M. F. D., and And Voltarelli, M. A. (2018). Can combine headers and travel speeds affect the quality of soybean harvesting operations? Revist. Bras. Engenharia Agríc. Ambiental. 20, 732–738. doi: 10.1590/1807-1929/agriambi.v22n10p732-738
Meng, Q., Hou, P., Wu, L., Chen, X., Cui, Z., and Zhang, F. (2013). Understanding production potentials and yield gaps in intensive maize production in china. Field Crops Res. 143, 91–97. doi: 10.1016/j.fcr.2012.09.023
Mongkolporn, O., Kadkol, G. P., Pang, E. C. K., and Taylor, P. W. J. (2003). Identification of rapd markers linked to recessive genes conferring siliqua shatter resistance in brassica rapa. Plant Breed. 122, 479–484. doi: 10.1046/j.0179-9541.2003.00910.x
Moreira, F. F., Hearst, A. A., Cherkauer, K. A., and Rainey, K. M. (2019). Improving the efficiency of soybean breeding with high-throughput canopy phenotyping. Plant Methods 15:139. doi: 10.1186/s13007-019-0519-4
Morgan, C. L., Ladbrooke, Z. L., Bruce, D. M., Child, R., and Arthur, A. E. (2001). Breeding oilseed rape for pod shattering resistance. J. Agric. Sci. 135, 347–359. doi: 10.1017/S0021859699008424
Østergaard, L., Kempin, S. A., Bies, D., Klee, H. J., and Yanofsky, M. F. (2006). Pod shatter-resistant brassica fruit produced by ectopic expression of the fruitfull gene. Plant Biotechnol. J. 4, 45–51. doi: 10.1111/j.1467-7652.2005.00156.x
Pabón-Mora, N., Wong, G. K.-S., and Ambrose, B. A. (2014). Evolution of fruit development genes in flowering plants. Front. Plant Sci. 5:300. doi: 10.3389/fpls.2014.00300
Philbrook, B. D., and Oplinger, E. S. (1989). Soybean field losses as influenced by harvest delays. Agron. J. 81, 251–258. doi: 10.2134/agronj1989.00021962008100020023x
Prince, S. J., Vuong, T. D., Wu, X., Bai, Y., Lu, F., Kumpatla, S. P., et al. (2020). Mapping quantitative trait loci for soybean seedling shoot and root architecture traits in an inter-specific genetic population. Front. Plant Sci. 11:1284. doi: 10.3389/fpls.2020.01284
Rajani, S., and Sundaresan, V. (2001). The arabidopsis myc/bhlh gene alcatraz enables cell separation in fruit dehiscence. Curr. Biol. 11, 1914–1922. doi: 10.1016/s0960-9822(01)00593-0
Raman, H., Raman, R., Kilian, A., Detering, F., Carling, J., Coombes, N., et al. (2014). Genome-wide delineation of natural variation for pod shatter resistance in brassica napus. PLoS One 9:e101673. doi: 10.1371/journal.pone.0101673
Roeder, A. H., and Yanofsky, M. F. (2006). Fruit development in arabidopsis. Arabidopsis Book 4:e0075. doi: 10.1199/tab.0075
Rowntree, S. C., Suhre, J. J., Weidenbenner, N. H., Wilson, E. W., Davis, V. M., Naeve, S. L., et al. (2013). Genetic gain × management interactions in soybean: I. Planting date. Crop Sci. 53, 1128–1138. doi: 10.2135/cropsci2012.03.0157
Schanoski, R., Righi, E. Z., and Werner, V. (2011). Perdas na colheita mecanizada de soja (glycine max) no município de maripá-pr. Revist. Bras. Engenharia Agríc. Ambiental. 15, 1206–1211. doi: 10.1590/s1415-43662011001100015
Sharma, S., Fleming, C., Selby, C., Rao, J., and Martin, T. (2013). Plant biostimulants: a review on the processing of macroalgae and use of extracts for crop management to reduce abiotic and biotic stresses. J. Appl. Phycol. 26, 465–490. doi: 10.1007/s10811-013-0101-9
Shukla, P. S., Borza, T., Critchley, A. T., Hiltz, D., Norrie, J., and Prithiviraj, B. (2018). Ascophyllum nodosum extract mitigates salinity stress in Arabidopsis thaliana by modulating the expression of mirna involved in stress tolerance and nutrient acquisition. PLoS One 13:e0206221. doi: 10.1371/journal.pone.0206221
Shukla, P. S., Mantin, E. G., Adil, M., Bajpai, S., Critchley, A. T., and Prithiviraj, B. (2019). Ascophyllum nodosum-based biostimulants: sustainable applications in agriculture for the stimulation of plant growth, stress tolerance, and disease management. Front. Plant Sci. 10:655.
Silveira, J., Conte, O., and Mesquita, C. D. M. (2019). Determinação de Perdas de Grãos na Colheita de soja: Copo Medidor da Embrapa. Available online at: https://www.infoteca.cnptia.embrapa.br/infoteca/handle/doc/979883?mode=full (accessed November 17, 2020).
Smyth, D. R., Bowman, J. L., and Meyerowitz, E. M. (1990). Early flower development in arabidopsis. Plant Cell 2, 755–767. doi: 10.1105/tpc.2.8.755
Sorefan, K., Girin, T., Liljegren, S. J., Ljung, K., Robles, P., Galvan-Ampudia, C. S., et al. (2009). A regulated auxin minimum is required for seed dispersal in arabidopsis. Nature 459, 583–586. doi: 10.1038/nature07875
SoyBase (2020). Soybase Soybean Growth Stages Conversion Page. Available online at: https://www.soybase.org/OntologyConversion.php (accessed May 17, 2017).
Suzuki, M., Fujino, K., Nakamoto, Y., Ishimoto, M., and Funatsuki, H. (2010). Fine mapping and development of DNA markers for the qpdh1 locus associated with pod dehiscence in soybean. Mol. Breed. 25, 407–418. doi: 10.1007/s11032-009-9340-5
Tiwari, S. P., and Bhatia, V. S. (1995). Characters of pod anatomy associated with resistance to pod-shattering in soybean. Ann. Bot. 76, 483–485. doi: 10.1006/anbo.1995.1123
UFU (2020). Uberland Federal University Soybean Improvement Program. Soybean cultivars. Available online at: http://www.pmsoja.iciag.ufu.br/ (accessed November 2020)
USDA (2020). Oilseeds and Products Annual-Brazil. Washington, DC: United States Department of Agriculture.
Van Roekel, R. J., Purcell, L. C., and Salmerón, M. (2015). Physiological and management factors contributing to soybean potential yield. Field Crops Res. 182, 86–97. doi: 10.1016/j.fcr.2015.05.018
Vera, J., Castro, J., Gonzalez, A., and Moenne, A. (2011). Seaweed polysaccharides and derived oligosaccharides stimulate defense responses and protection against pathogens in plants. Mar. Drugs 9, 2514–2525. doi: 10.3390/md9122514
Verdugo, R. A., Farber, C. R., Warden, C. H., and Medrano, J. F. (2010). Serious limitations of the qtl/microarray approach for qtl gene discovery. BMC Biol. 8:96. doi: 10.1186/1741-7007-8-96
Yoon, J., Cho, L.-H., Kim, S. L., Choi, H., Koh, H.-J., and An, G. (2014). The bel1-type homeobox gene sh5 induces seed shattering by enhancing abscission-zone development and inhibiting lignin biosynthesis. Plant J. 79, 717–728.
Keywords: seaweed, Ascophyllum nodosum extract, pod shattering, soybean (Glycine max), seed loss, sustainable agriculture, plant biostimulants
Citation: Łangowski Ł, Goñi O, Marques FS, Hamawaki OT, da Silva CO, Nogueira APO, Teixeira MAJ, Glasenapp JS, Pereira M and O’Connell S (2021) Ascophyllum nodosum Extract (SealicitTM) Boosts Soybean Yield Through Reduction of Pod Shattering-Related Seed Loss and Enhanced Seed Production. Front. Plant Sci. 12:631768. doi: 10.3389/fpls.2021.631768
Received: 20 November 2020; Accepted: 25 January 2021;
Published: 24 February 2021.
Edited by:
Youssef Rouphael, University of Naples Federico II, ItalyReviewed by:
Alan T. Critchley, Cape Breton University, CanadaTommaso Frioni, Catholic University of the Sacred Heart, Piacenza, Italy
Copyright © 2021 Łangowski, Goñi, Marques, Hamawaki, da Silva, Nogueira, Teixeira, Glasenapp, Pereira and O’Connell. This is an open-access article distributed under the terms of the Creative Commons Attribution License (CC BY). The use, distribution or reproduction in other forums is permitted, provided the original author(s) and the copyright owner(s) are credited and that the original publication in this journal is cited, in accordance with accepted academic practice. No use, distribution or reproduction is permitted which does not comply with these terms.
*Correspondence: Shane O’Connell, shane.oconnell@staff.ittralee.ie