- Key Laboratory of Soybean Biology of Ministry of Education, China (Key Laboratory of Biology and Genetics and Breeding for Soybean in Northeast China), Northeast Agricultural University, Harbin, China
Photoperiod is one of the main climatic factors that determine flowering time and yield. Some members of the INDETERMINATE DOMAIN (IDD) transcription factor family have been reported to be involved in regulation of flowering time in Arabidopsis, maize, and rice. In this study, the domain analysis showed that GmIDD had a typical ID domain and was a member of the soybean IDD transcription factor family. Quantitative real-time PCR analysis showed that GmIDD was induced by short day conditions in leaves and regulated by circadian clock. Under long day conditions, transgenic Arabidopsis overexpressing GmIDD flowered earlier than wild-type, and idd mutants flowered later, while the overexpression of GmIDD rescued the late-flowering phenotype of idd mutants. Chromatin immunoprecipitation sequencing assays of GmIDD binding sites in GmIDD-overexpression (GmIDD-ox) Arabidopsis further identified potential direct targets, including a transcription factor, AGAMOUS-like 18 (AGL18). GmIDD might inhibit the transcriptional activity of flower repressor AGL18 by binding to the TTTTGGTCC motif of AGL18 promoter. Furthermore, the results also showed that GmIDD overexpression increased the transcription levels of flowering time-related genes FLOWERING LOCUS T (FT), SUPPRESSOR OF OVEREXPRESSION OF CONSTANS1 (SOC1), LEAFY (LFY) and APETALA1 (AP1) in Arabidopsis. Taken together, GmIDD appeared to inhibit the transcriptional activity of AGL18 and induced the expression of FT gene to promote Arabidopsis flowering.
Introduction
Soybean [Glycine max (L.) Merrill] is a typical short-day (SD) plant, which is particularly sensitive to photoperiod response. SDs can promote flowering, while long-days (LD) can inhibit flower bud growth (Kantolic and Slafer, 2007). Soybean can be regarded as a classical model plant at the beginning of the study of the plant photoperiod pathway due to its photoperiodic response characteristics. The cultivation of soybeans on the earth spans 85 latitudes, with different soybean varieties ranging from Vancouver in the north to New Zealand in the south. However, due to the limitation of latitude, cultivated area of each soybean variety is scarce, resulting in its limited adaptability (Cober and Morrison, 2010). Therefore, flowering time and mature period have become important agronomic traits of soybean, which can influence the yield, quality, and versatility of soybean varieties. By reasonable planting of soybean varieties under different environmental conditions in different regions, the cultivated land resources in this area can be fully utilized, which is of great significance to increase the yield of soybean. Thus, it is a hotspot in the field of reducing the breeding pressure, by exploring new photoperiod genes that control soybean flowering and maturation, and further clarifying the molecular mechanism of these genes involved in soybean photoperiod effect.
INDETERMINATE DOMAIN (IDD) transcription factors are one of the largest and most conserved gene families in the plant kingdom and play an important role in various processes of plant growth and development, such as regulating flower induction (Ali et al., 2019). The IDD transcription factor family has a highly conserved ID domain, which was initially described as consisting of two typical C2H2 and C2HC zinc finger motifs. The functions of some members of the IDD transcription factor family have been identified, particularly in Arabidopsis, maize, and rice. In Arabidopsis, three IDD family members, MAGPIE/IDD3 (MAG), NUTCRACKER/IDD8 (NUT), and JACKDAW/IDD10 (JKD), and GRAS domain proteins SHR and SCR, have been shown to jointly regulate root development (Levesque et al., 2006; Cui et al., 2007; Welch et al., 2007). SHOOT GRAVITROPISM5/IDD15 (SGR5) was mainly expressed in the endodermis of the inflorescence stem, which was involved in regulating the early gravity perception and starch accumulation of Arabidopsis (Long et al., 2015). AtIDD8 controlled the photoperiod-dependent flowering pathway in Arabidopsis by regulating sugar signal transduction (Funck et al., 2012). In maize, ID1 gene was the first characteristic gene of the IDD transcription factor family and played an important role in regulating the blooming period of corn (Colasanti et al., 1998). NAKED ENDOSPERM 1 and 2(NKD1 and NKD2) were two other members of the maize IDD family and involved in seed development (Yi et al., 2015). In rice, EARLY HEADING DATE 2 (Ehd2), a homolog of ZmID1 in rice, which could up-regulate the expression of the EARLY HEADING DATE 1 (Ehd1) gene to promote a FT-like gene HEADING DATE 3a (Hd3a) and promote flowering under SDs (Matsubara et al., 2008; Gontarek et al., 2016). OsId1, a homologous gene of maize ID1, regulates Ehd1 and its downstream genes, including Hd3a and RICE FLOWERING LOCUS T1 (RFT1) to regulate flowering (Park et al., 2008). When RICE INDETERMINATE 1 (RID1) gene was activated to induce phase transition, flowering signals were transduced and regulated through various pathways, and finally integrated with FT-like proteins to induce flowering (Wu et al., 2008). However, the function of IDD transcription factor family members in soybean has not been reported.
MADS-domain proteins form a large and diverse family in plants and play a variety of regulatory roles. Previous reports showed that flowering was determined by the additive effect of multiple MADS-domain flower inhibitors, among which AGAMOUS-like 15 (AGL15) and AGAMOUS-like 18 (AGL18) made important contributions (Adamczyk et al., 2007). The double mutants of AGL18 and AGL15, members of the AGL15-like branch of the MADS domain regulator, showed early flowering phenotype. It was proposed that AGL15 and AGL18 act upstream of the floral integrator FT. The combination of AGL15 and AGL18 mutations partially inhibited the photoperiodic pathway defects (Adamczyk et al., 2007).
In the current study, soybean GmIDD was identified as a member of IDD transcription factor protein family. Overexpression of GmIDD promotes flowering in Arabidopsis. Putative target gene AGL18 and TTTTGGTCC motif for DNA binding of GmIDD were predicted by ChIP-seq and ChIP-qPCR, and it was preliminarily verified that GmIDD promoted flowering by inhibiting AGL18 activity. Furthermore, the transcription levels of flowering time related genes such as FT, SUPPRESSOR OF OVEREXPRESSION OF CONSTANS1 (SOC1), LEAFY (LFY) and APETALA1 (AP1) were increased by GmIDD overexpression in Arabidopsis.
Materials and Methods
Plant Materials and Growth Conditions
For the diurnal rhythm analysis of GmIDD gene, seeds from “DongNong 42,” a photoperiod sensitive soybean variety, were provided by Soybean Research Institute of Northeast Agricultural University (Harbin, China). Seeds of “DongNong 42” were planted in a greenhouse at 25°C with 250 μmol m–2 sec–1 white light under LDs (16 h/8 h light/dark). When the first trifoliate leaves were expanded, part of the seedlings were transferred to SDs (8 h/16 h light/dark) under the same temperature regime. Seedlings were cultured under LDs and SDs for 30 days (transferred at 15 days) and sampled at 3 h intervals for 24 h, and sampled under continuous light (LL) and dark (DD) conditions for 48 h, and then immediately frozen in liquid nitrogen. Samples of different tissues (including roots, stems, leaves, flowers, pods, and seeds) of plants cultured under LDs and SDs were collected for tissue specific expression analysis of GmIDD gene.
In this study, Arabidopsis thaliana (Col-0) was used as control and the background plant of genetic transformation. Seeds of the idd mutant (SALK_129969c) were obtained from the Arabidopsis Biological Resource Center (ABRC, Columbus, United States). The seeds of GmIDD-overexpression (GmIDD-ox), idd mutant, 35S:GmIDD/idd restoration and wild-type (WT) Arabidopsis were surface sterilized with 10% hypochlorite and then planted on MS agar medium. The seeds were placed at 4°C for 72 h and transferred to room temperature (22°C). The 10-day-old seedlings were transplanted into 1:1 vermiculite turbidite soil and cultured under LDs and SDs for flowering phenotype analysis. WT and GmIDD-ox transgenic Arabidopsis plants were cultured under LDs on MS agar medium for 15 days and sampled for quantitative real-time PCR (qRT-PCR) analysis of flowering time-related genes. These experiments were performed in three biological replicates.
Plasmid Construction and Generation of Transgenic Arabidopsis Plants
For the FLAG and HIS tag construct, we first synthesized the tandem repeats of 3 × FLAG and 6 × Histidine (3F6H) tags with NotI at 5′ end and XbaI at 3′ end (5′-GCGGCCGCCCTGGAGCTCGGTACCCGGG(SmaI)GATCCC AGGATCTGATTACAAGGATCATGATGGTGATTACAAGG ATCACGACATCGACTACAAGGATGACGATGACAAGCAC CATCATCACCACCATTGATCTCTAGA-3′, the sequences encoding 3F6H tag were in bold) (Song et al., 2012). The synthesized products above were cloned into NotI-XbaI sites of pENTRY vector (named pENTRY-3F6H) which contained the 3F6H sequence in the C terminus of the cloning site, and the sequences were verified. The full-length coding region of GmIDD was amplified from total RNA of “Dongnong 42” using GmIDD-3F6H-F and GmIDD-3F6H-R primers (Supplementary Table S1). The PCR product was purified and cloned into pENTRY-3F6H vector linearized by SmaI using In-Fusion cloning system (Clontech, United States) to construct recombinant vector 35S:GmIDD-3F6H-pENTRY. LR reaction was conducted by 35S:GmIDD-3F6H-pENTRY and pB7WG2 to generate 35S:GmIDD-3F6H-pB7WG2 fusion expression vector. The recombinant vector 35S:GmIDD-3F6H -pB7WG2 was introduced into the Agrobacterium GV3101 which used to transform Arabidopsis thaliana (Col-0) and idd mutant using the floral dip method with Agrobacterium tumefaciens strain GV3101 (Clough and Bent, 1998). Transformants were selected on MS agar medium with 8 mg/L phosphinothricin. T3 transgenic seeds of two homozygous lines were selected for further study.
Subcellular Localization of GmIDD
A cDNA fragment of GmIDD was amplified by PCR with GmIDD-TOPO-F and GmIDD-TOPO-R primers (Supplementary Table S1) from total RNA of “DongNong 42” and cloned into pENTR/D-TOPO (Life technologies) and then transferred to the expression vector pGWB506 through LR reaction to generate the 35S:GFP-GmIDD fusion vector. The recombinant construct was introduced into Agrobacterium GV3101 and subsequently transformed into N. benthamiana (Sheikh et al., 2014). After infiltration, the tobacco leaves were grown for 2 days and the GFP signal was detected by fluorescence microscopy.
ChIP-Seq and ChIP-qPCR
One representative GmIDD-ox-1 lines were selected for ChIP-seq. GmIDD-ox-1 transgenic Arabidopsis plants were cultured on MS agar medium under LDs for 14 days, and then 1 g seedlings were quick-frozen in liquid nitrogen. With the purpose of minimizing the deviation between different parallel samples under the same treatment, the sample was retrieved from independent three plots of Arabidopsis seedlings. After crushing, the seedlings were fixed with 1% (V/V) formaldehyde for 15 min at 4°C. Final concentration of 0.125 M glycine was subsequently added to quench the cross-linking reaction. After nuclei were isolated and lysed, and the chromatin solution was then sonicated to approximately 200–1,000 bp DNA fragments. Immunoprecipitation reactions were performed using Anti-Flag antibody (Monoclonal ANTI-FLAG® M2 antibody produced in mouse, F1804, Sigma-Aldrich). The complex of chromatin antibody was captured with protein G beads (Invitrogen), and DNA was purified using a QIAquick PCR purification kit (QIAGEN). The purified DNA was sent to a sequencing company for library construction and deep sequencing. The ChIP-seq sequencing project was completed on Illumina sequencing platform. Illumina PE library (∼300 bp) was constructed for sequencing and quality control of the obtained sequencing data. Bioinformatics methods were then used to analyze ChIP-seq data. The raw sequence data were aligned to the Arabidopsis thaliana(TAIR10)1. MACS was used to predict the length of the protein binding sequence through modeling, and the relative abundance of the corresponding peak of the sequence was determined by the length of the sequence and the numbers of pair end reads mapped on the sequence. ChIP-seq was completed by SeqHealth Tech Co., Ltd., Wuhan, China.
ChIP-qPCR was performed using the whole WT and GmIDD-ox-1 seedlings. The relative enrichment of WT was set to 1. IPP2 gene (isopentenyl pyrophosphate: dimethyl allyl pyrophosphate isomerase 2, AT3G02780) was used as a negative control. DNA samples were analyzed by real-time quantitative PCR using the appropriate DNA primers (Supplementary Table S1) and SuperReal PreMix Plus (TIANGEN, Beijing, China). IgG was used as an antibody control. Data are shown as the mean ± SD of three biological replicates.
Transient Assay of AGL18 Promoters Affected by GmIDD in N. Benthamiana
To generate AGL18 promoter driven LUC constructs proAGL18:LUC, promoter DNA was amplified from genomic DNA of Arabidopsis (Col-0) using proAGL18:LUC-F and proAGL18:LUC-R primers (Supplementary Table S1). The PCR product was purified and cloned into binary vector pGreenII-0800-LUC linearized by SmaI using In-Fusion cloning system. The recombinant constructs were introduced into Agrobacterium GV3101 and subsequently transformed into N. benthamiana (Sheikh et al., 2014). The transient activity of recombinant vectors was assayed using dual-luciferase assay kit (Promega, United States) and Multiscan Spectrum (TECAN Infinite 200 PRO, Männedorf, Switzerland). The construct 35S:GmIDD-3F6H-pB7WG2 and proAGL18:LUC were simultaneously transferred into N. benthamiana to measure transient assay of the AGL18 promoters affected by GmIDD protein. A floral repressor GmRAV effector construct (35S:GmRAV-3F6H-pB7WG2) and proAGL18:LUC were simultaneously transferred into N. benthamiana as the negative control of this experiment. The recombinant vector 35S::GmRAV-3F6H-pB7WG2 was obtained and introduced into Agrobacterium GV3101 by referring to the construction method of 35S::GmIDD-3F6H-pB7WG2 above. Expression vector pB7WG2 and proAGL18:LUC was simultaneously transferred into N. Benthamiana as the blank control of this experiment. Three independent experiments were performed and each experiment was repeated three times to obtain reproducible results. The luminescence signal was captured using Amersham Imager 600 (General Electric Company)2 after spraying 1 mM luciferin (Heliosense)3 on N. benthamiana leaves.
Quantitative RT-PCR Analysis
RNA isolation has been described previously (Zhao et al., 2013). qRT-PCR amplifications were performed using the SuperReal PreMix Plus (TIANGEN, Beijing, China) on Applied BiosystemsTM 7500 Fast Dx Real-Time PCR Instrument (ABI). The PCR cycling conditions were as follows: 95°C for 15 min; 40 cycles of 95°C for 10 s, 60°C for 20 s, and 72°C for 30 s. GmActin4 (GenBank accession number AF049106) and IPP2 (AT3G02780) were used as endogenous regulatory genes of soybean and Arabidopsis, respectively. Three biological replicates and three technical replicates were applied for the whole assays. The primers used in qRT-PCR analyses were shown in Supplementary Table S1.
Flowering Time Measurements
Flowering time of Arabidopsis was determined by scoring the numbers of rosette leaves and the numbers of days from germination to bolting time. At least 20 plants were analyzed each time, and the analysis was repeated for three times.
Results
Sequence Analysis of the GmIDD
We have identified that both genes such as GmRAV and GmGBP1 induced by SD detected by suppression subtractive hybridization (SSH) functioned in regulating flowering time (Zhao et al., 2008, 2018). In this study, we further detected a zinc finger transcription factor GmIDD (Glyma.14G095900) gene also induced by SD from SSH library. The GmIDD sequence information was obtained from soybean genome database4. The cDNA sequence of GmIDD is 2,034 bp and contains 1,227 bp open reading frame, which encoded 408 amino acids with predicted molecular mass of 45,129 kDa. The domain analysis showed that GmIDD had a typical ID domain, which consisted of a nuclear localization signal motif (KKKR), four zinc finger domains including two types of C2H2 (72–92 aa and 114–142 aa), and two types of C2HC (149–169 aa and 176–195 aa) (Figure 1A). GmIDD showed high homology with AtIDD8, ZmID1, and Ehd2, which are typical IDD family members in Arabidopsis, maize and rice (Figure 1B). Therefore, GmIDD is a member of the soybean IDD transcription factor family. A phylogenetic tree containing 10 soybean proteins with complete ID domain and 15 IDD proteins from other species was constructed by MEGA 6.0. Phylogenetic tree analysis showed that GmIDD was located on the same branch with Vigna angularis (NC_030643.1), Cajanus cajan (KYP72220.1), Vigna radiata (XP_017428159.1), Phaseolus vulgaris (Phvul.001G036500), Lupinus angustifolius (XP_019458210.1), and Medicago truncatula (Medtr1g016010.1) (Figure 1C). Soybean IDD proteins Glyma.08G192300 and Glyma.15G024500 were located on the same branch with Nelumbo nucifera (xP_010242234.1) and Juglans regia (xP_018848593.1) (Figure 1C). Soybean IDD protein Glyma.07G158200 was located on the same branch with Zea Mays (GRMZM2G320287) and Oryza sativa (LOC_Os02g31890) (Figure 1C). Therefore, the evolution distance between GmIDD and other soybean IDD proteins was relatively far, indicating that soybean IDD protein might have certain differences in function.
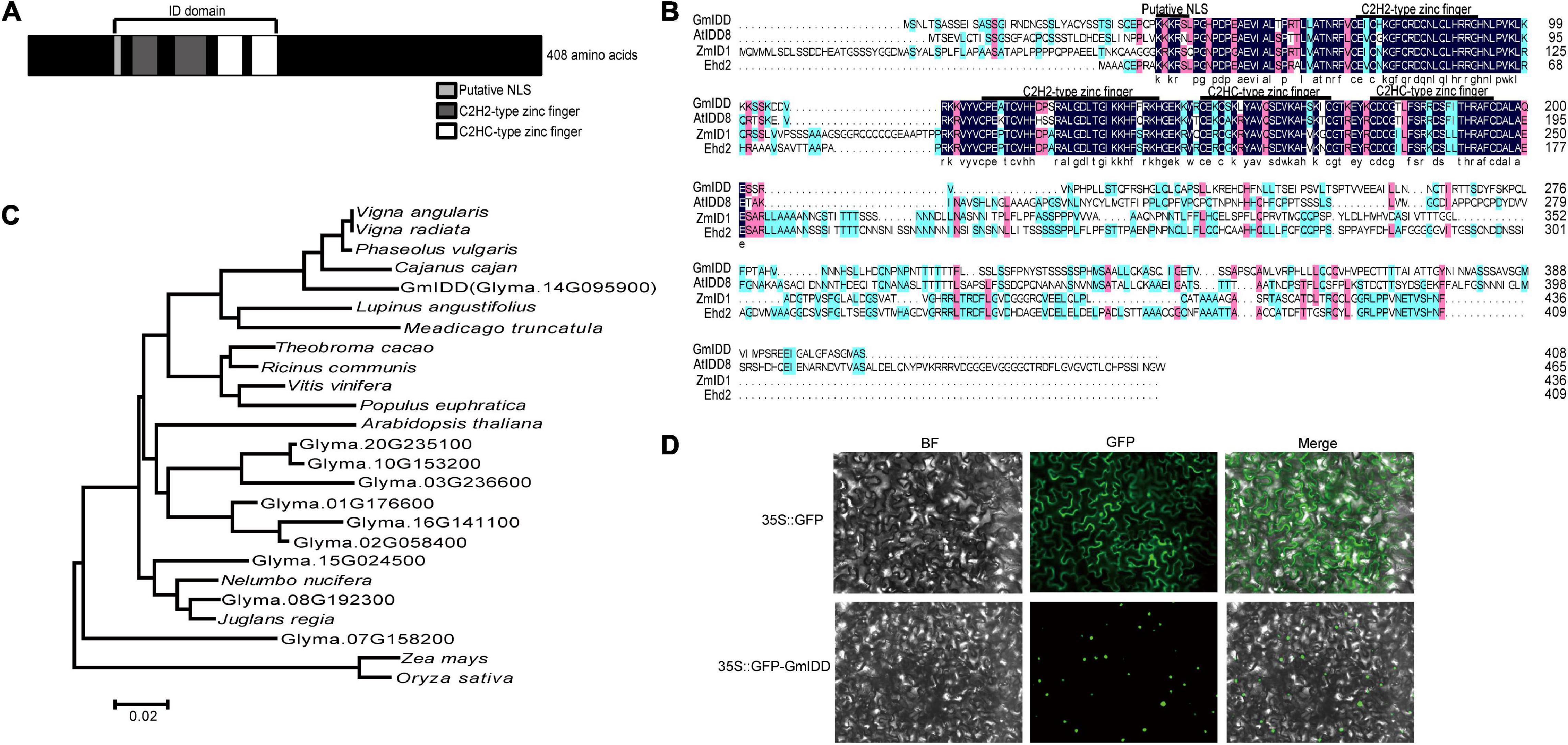
Figure 1. Sequence analysis and subcellular localization of GmIDD. (A) Predicted domains of GmIDD paralogs known from Phytozome which contain a nuclear localization signal motif (KKKR) and four zinc finger domains including two types of C2H2 (72–92, 114–142 aa) and two types of C2HC (149–169, 176–195 aa). (B) Multiple alignments of the amino acid sequences encoded by GmIDD and known IDD family members in Arabidopsis, maize, and rice. The nuclear localization signal motif (KKKR) and four zinc finger domains were indicated by the line on the top. Blue, homology 100%; pink, homology ≥ 75%; light blue, homology ≥ 50%. (C) Phylogenetic tree analysis was performed on GmIDD and proteins from other species with high similarity in NCBI. All the amino acid sequence information comes from the Phytozome database (accession numbers are listed in Supplementary Table S2). A phylogenetic tree containing 10 soybean proteins with complete ID domain and 15 IDD proteins from other species (Theobroma cacao, Ricinus communis, Vitis vinifera, Populus euphratica, Arabidopsis thaliana, Nelumbo nucifera, Juglans regia, Lupinus angustifolius, Cajanus cajan, Phaseolus vulgaris, Vigna angularis, Vigna radiata, Zea mays, Medicago truncatula, and Oryza sativa. Phylogenetic tree was constructed using the neighbor joining method of MEGA 6.0. (D) Subcellular localization of GmIDD protein. The 35S:GFP-GmIDD fusion expression vector was used for agroinfection in N.benthamiana. The tobacco leaves infected with 35S:GFP agrobacterium were used as control. After infiltration, the tobacco leaves were grown for 2 days and the GFP signal was detected by fluorescence microscopy. GFP, Green Fluorescent Protein; BF, bright field; Merge, GFP, and bright-field images.
GmIDD Protein Located in Cell Nucleus
The subcellular localization of the GmIDD protein might be crucial for its function. The expression of 35S:GFP-GmIDD fusion protein under the control of the 35S promoter in tobacco mesophyll cells showed that the GmIDD fusion protein was concentrated in the nucleus (Figure 1D), whereas GFP was dispersed throughout the entire tobacco mesophyll cells in the 35S:GFP control. The results clearly showed that GmIDD was a nuclear-localized protein.
Photoperiod and Circadian Rhythm Regulate the Accumulation of GmIDD Transcript
The trifoliate leaves of soybean grown for 30 days (transferred at 15 days) were collected every 3 h to show the diurnal expression patterns of GmIDD in LDs and SDs by qRT-PCR analysis. GmIDD exhibited photoperiod-specific expression patterns under both LDs and SDs. The expression levels of GmIDD mRNA under SDs were significantly higher than those under LDs (Figure 2A). To further determine GmIDD gene expression patterns, we analyzed GmIDD expression under LL and DD conditions after SDs and LDs transfer. GmIDD mRNA level maintained a strong rhythm under SDs-DD and SDs-LL, reaching the peak at 12 h after dawn (Figure 2B), but no strong cycling was detected under LDs-LL (Figure 2C). Therefore, GmIDD gene was induced by SDs in soybean leaves, and regulated by circadian clock.
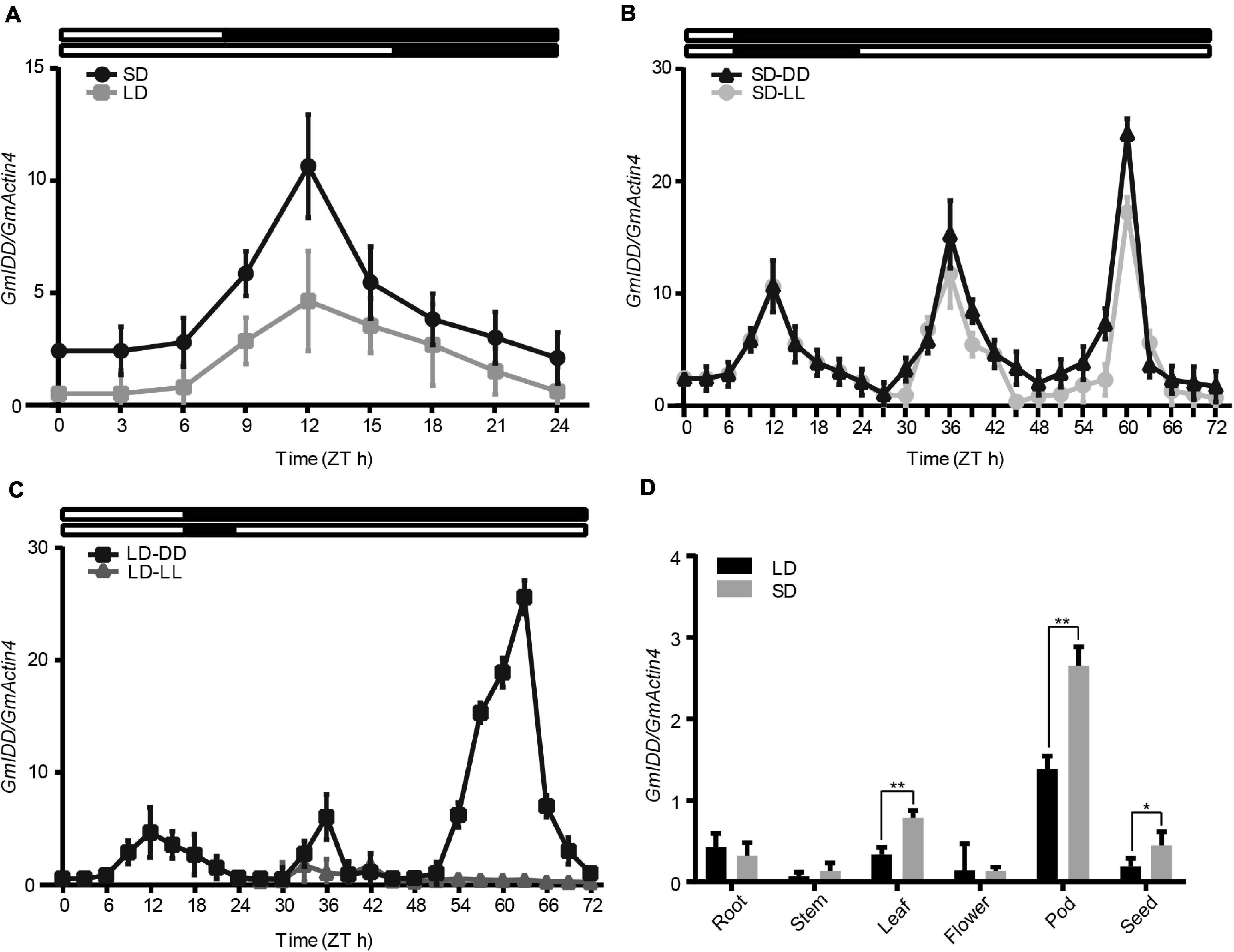
Figure 2. qRT-PCR analyses of soybean GmIDD expression patterns. (A–C) Soybean “Dongnong 42” plants, which were grown in LDs (16 h/8 h light/dark) for 15 days, were transferred to LDs or SDs (8 h/16 h light/dark) for 15 days for sampling. Trifoliate leaves were sampled at 3 h intervals. (A) GmIDD diurnal expression under SDs and LDs. (B) Expression patterns of GmIDD transcripts under constant darkness (DD) and constant light (LL) conditions from SDs. (C) Expression patterns of GmIDD transcripts under constant darkness (DD) and constant light (LL) conditions from LDs. White and black bars at the top represented light and dark phases, respectively. (D) Tissue-specific expression of GmIDD at 12 h after dawn under SDs and LDs. All the data were normalized with soybean GmActin4 gene as internal reference. For each experiment, three technical replicates were conducted. Data shown are mean ± SD of three independent experiments. Significant difference between the expression level of GmIDD under SDs and LDs was indicated by asterisk (*P < 0.05 and **P < 0.01, Student’s t-test).
Temporal and Spatial Expression Patterns of GmIDD in Soybean
Samples of different tissues (including roots, stems, leaves, flowers, pods, and seeds) of plants cultured under LDs and SDs were collected at 12 h after dawn for tissue specific expression analysis of GmIDD gene to determine the expression pattern of GmIDD gene during soybean growth and development. The GmIDD mRNA was present in all organs examined, which included roots, stems, leaves, flowers, pods, and seeds (Figure 2D). The mRNA abundance of GmIDD was the highest in pods among all organs under both SDs and LDs. All the mRNA abundance of GmIDD in leaves, stems, pods, and seeds in SDs was higher than that in LDs. However, the mRNA abundance of GmIDD in roots in SDs plants was lower than that in LDs. These expression patterns were different from those of AtIDD8, which was expressed at a relatively high level in vegetative organs, but at a lower level in pods (Seo et al., 2011). Although GmIDD and AtIDD8 have high amino acid homology and belong to IDD transcription factor family, there may be some differences in biological function.
Overexpression of GmIDD Promotes Flowering in Arabidopsis
In order to verify the function of GmIDD in the control of flowering time, the GmIDD gene was genetically transformed into WT Arabidopsis under the regulation of cauliflower-mosaic virus (CaMV) 35S promoter to obtain GmIDD-ox plants. We observed the flowering phenotype of WT Arabidopsis, GmIDD-ox, idd mutant plants under LDs and SDs. Under LDs, the flowering time of GmIDD-ox plants were significantly promoted for 3 days, and the idd mutants were significantly delayed for 4 days (Figures 3A,B). In addition, the total number of rosette leaves of GmIDD-ox plants and idd mutants was fewer and more than that of WT plants at bolting, respectively (Figure 3B). Under SDs, the flowering time of GmIDD-ox plants were significantly promoted for 5 days, idd mutants showed no significant difference (Figures 3A,C). A complementation experiment was conducted on idd mutants to further determine the roles of GmIDD in promoting flowering time. The phenotype of idd mutants was rescued by the expression of a 35S:GmIDD fusion gene under the control of the 35S promoter. The results indicated that overexpression of GmIDD could promote flowering in Arabidopsis.
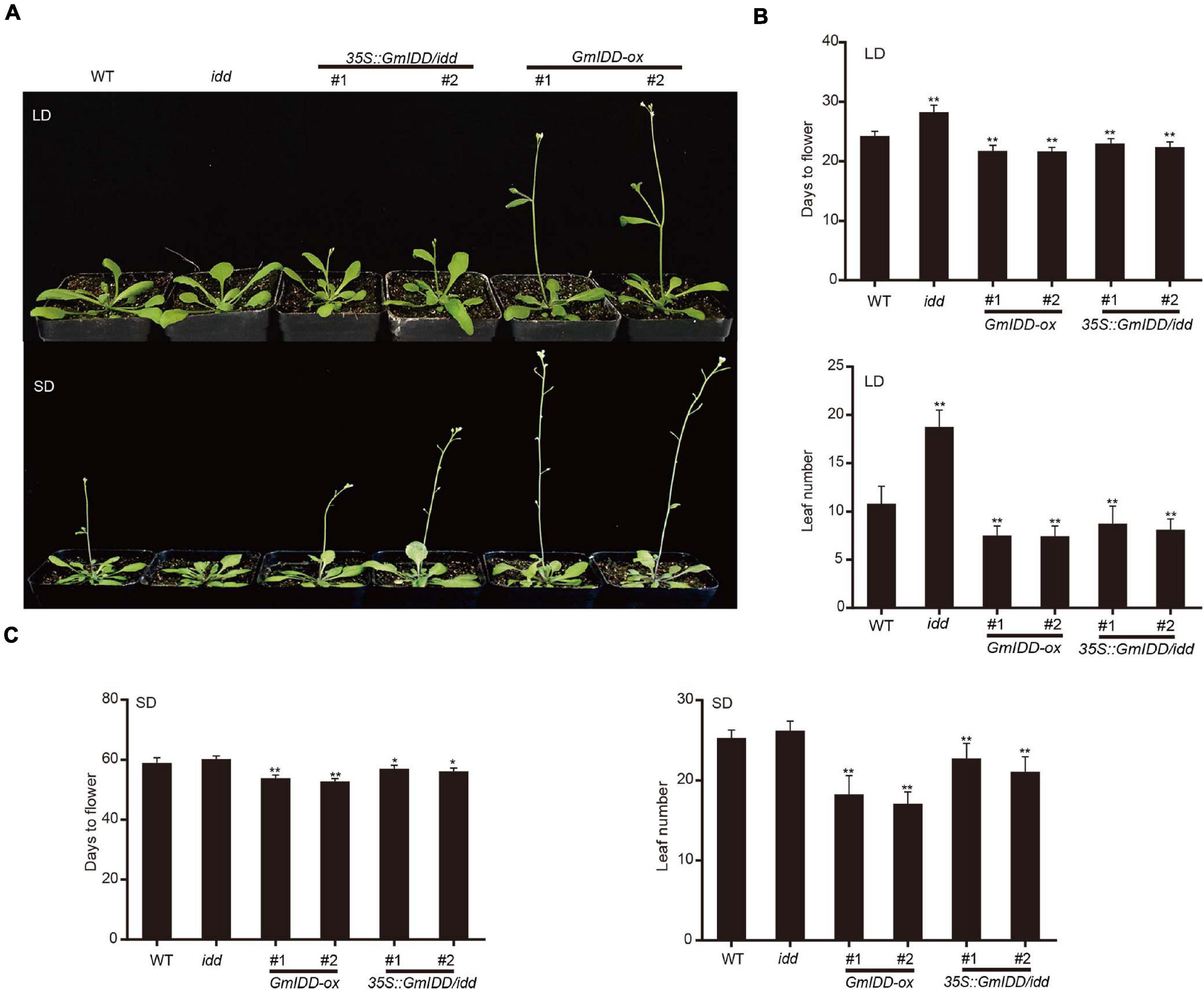
Figure 3. GmIDD conferred early flowering phenotypes in transgenic Arabidopsis. (A) Flowering phenotype of GmIDD plants under LDs and SDs. GmIDD-ox, idd mutant, 35S:GmIDD/idd restoration and WT Arabidopsis plants were cultured under LDs and SDs. Photographed after the plants have grown in the soil under LDs and SDs for 25 days and 56 days, respectively. (B) Days to flowering and average total leaf numbers of GmIDD-ox, idd mutant, 35S:GmIDD/idd restoration and WT Arabidopsis plants under LDs. (C) Days to flowering and average total leaf numbers of GmIDD-ox, idd mutant, 35S:GmIDD/idd restoration and WT Arabidopsis plants under SDs. Data represent means ± SD of at least 20 seedlings. Asterisks indicate significant differences between GmIDD-ox, idd mutant, 35S:GmIDD/idd restoration plants and WT. For each experiment, three technical replicates were conducted. Data shown are mean ± SD of three independent experiments (*P < 0.05 and **P < 0.01, Student’s t-test).
Genome-Wide Identification of GmIDD-Target Genes by ChIP-seq
ChIP-seq was performed to identify the DNA binding sites and target genes of GmIDD in overexpressed transgenic Arabidopsis, and further elucidate the potential mechanism of GmIDD in promoting flowering. Among the 588 GmIDD-binding sites detected by ChIP-seq, 446 (75.85%) were located in genic regions. These binding sites comprised gene bodies and their flanking regulatory sequences, including 2 kb upstream regions that were assumed to harbor promoter regions and 2 kb downstream regions that were assumed to contain terminator regions. Among the 446 sites in genic regions, 32.51, 48.88, and 18.61% were located in defined promoter regions, gene bodies, and terminator regions, respectively (Figure 4A).
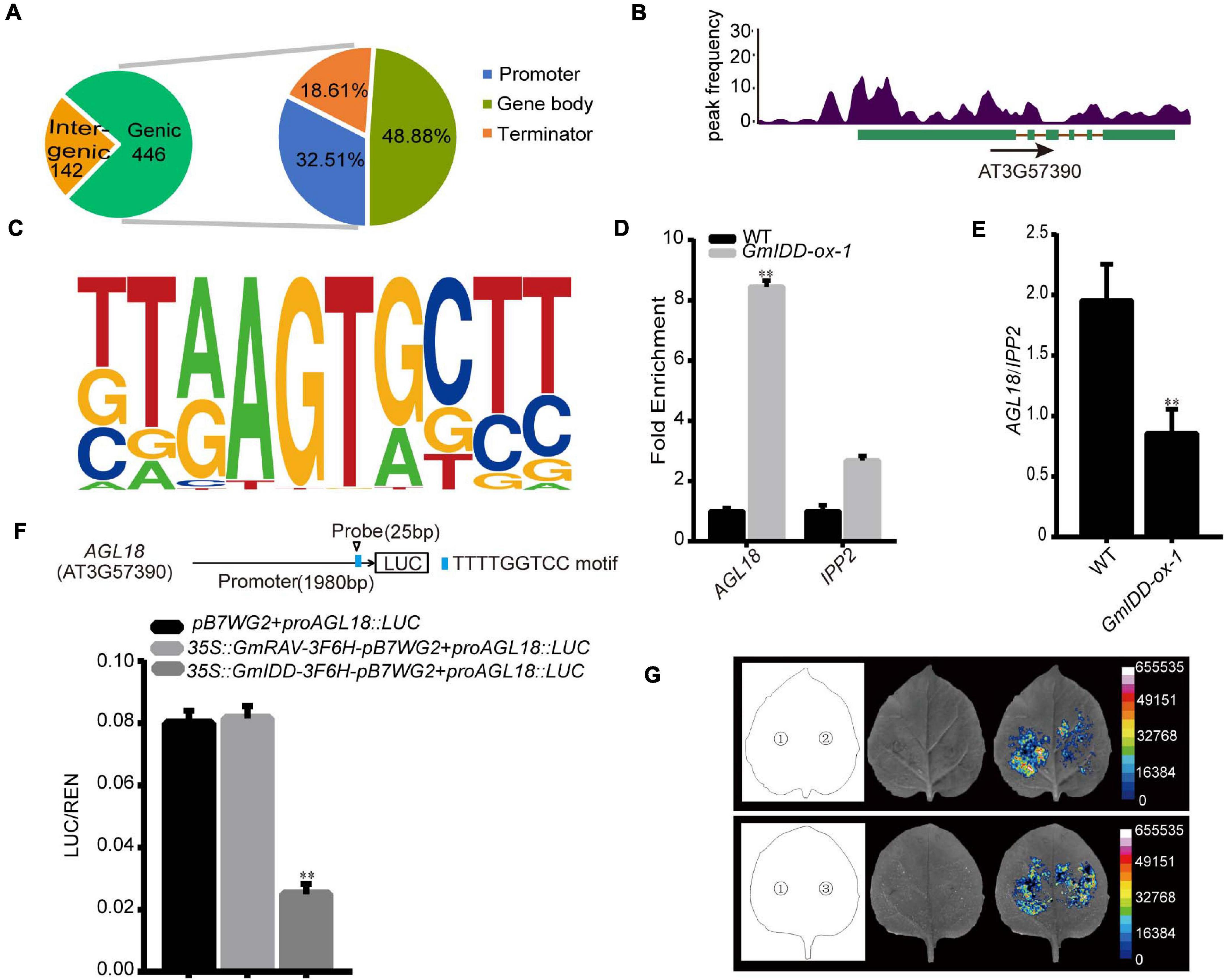
Figure 4. Validation and expression analyses of selected GmIDD target genes. (A) Distribution of the locations of binding sites relative to target genes. Promoter: a sequence within 2 kb upstream of transcription start site; Terminator: the sequence within 2 kb downstream of the transcription termination site; Gene body: 5′ UTR, CDS, intron, and 3′ UTR. (B) Peak graphs showing the ChIP-seq raw reads at the indicated gene loci in Integrative Genomics Viewer. The arrows indicate the directions of transcription, and the green bars indicate the transcripts of gene. (C) Motif analysis of GmIDD-binding sequences using HOMER software. (D) Anti-Flag antibody was used to precipitate chromatin prepared from GmIDD-ox transgenic Arabidopsis plants. The relative enrichment of the WT fragment was set as 1.0 and those of other fragments were adjusted accordingly. IgG was used as an antibody control. IPP2 was used as the internal gene control. (E) qRT-PCR analysis of the transcript levels of AGL18 in the 14-day-old WT, and GmIDD-ox-1 transgenic Arabidopsis plants. (F) The effect of GmIDD protein on the AGL18 promoter activity. Relative luciferase activity of co-transfected effector and reporter genes in tobacco leaves was detected in LDs. The activities of firefly LUC were normalized by the activities of 35S:Renilla LUC. Results represent means ± SD of eight independent samples (∗∗P < 0.01 vs. no effector, Student t-test). Upper panel: physical locations of fragments harboring putative motifs are shown in the schematic diagram. (G) Luciferase activity of AGL18 under LDs. 1: pB7WG2 + proAGL18:LUC as the blank control; 2: 35S:GmIDD-3F6H-pB7WG2 + proAGL18:LUC; 3: 35S:GmRAV-3F6H-pB7WG2 + proAGL18:LUC as the negative control; D-luciferin was used as the substrate of LUC. For each experiment, three technical replicates were conducted. Data shown are mean ± SD of three independent experiments (∗∗P < 0.01, Student’s t-test).
AGL18 (AT3G57390) related to flowering time might be a GmIDD candidate target. The MADS-domain factor AGL18 acted redundantly as a repressor of the floral transition in Arabidopsis (Adamczyk et al., 2007). AGL18 acted upstream of the floral integrator FT, and a combination of agl18 and agl15 mutations partially suppressed defects in the photoperiod pathway. The GmIDD binding site in AGL18 gene regions was located in the promoter region (Figure 4B). According to the consensus sequences at the detected GmIDD-binding sites, putative GmIDD-binding motifs were predicted using the Hypergeometric Optimization of Motif EnRichment (HOMER) software (Heinz et al., 2010). Based on the prediction, we identified that GmIDD combined with target gene AGL18 motif [(T/G/C/A)(T/G/A)(A/G/C/T)(A/T)(G/T)(T/G)(G/A/T)(C/G/T) (T/C/G)(T/C/G/A)] (P-value = 1e-6) (Figure 4C). The previous study reported that AtIDD8 regulated SUS4 by binding with the conserved CTTTTGTCC motif of its promoter (Seo et al., 2011). Sequence analysis of AGL18 promoter showed that the AGL18 promoter contained the TTTTGGTCC motif, which was similar to the CTTTTGTCC motif of AtIDD8 combined with SUS4 promoter.
The identified GmIDD-binding sites were further validated by ChIP–qPCR. The 14-day-old GmIDD-ox-1 transgenic lines were used to perform ChIP-qPCR to verify potential GmIDD-binding sites. Anti-Flag antibody was used to precipitate chromatin prepared from GmIDD-ox-1 transgenic lines. IgG was used as an antibody control. The fragments harboring identified GmIDD-binding site in the promoter region of AGL18 was highly enriched in DNA chromatin-immunoprecipitated (ChIPed) with Anti-Flag in ChIP-qPCR experiments, indicating GmIDD bound to the promoter of AGL18 (Figure 4D).
GmIDD Inhibits the Transcriptional Function of AGL18
It was found that the AGL18 promoter was bound by GmIDD using ChIP-qPCR, and its expression levels was decreased in GmIDD-ox-1 Arabidopsis plants using qRT-PCR (Figure 4E). The reporter proAGL18:LUC was constructed by AGL18 promoter containing GmIDD-binding site TTTTGGTCC motif driving LUC reporter gene. When co-infiltrating Agrobacterium expressing 35S:GmIDD-3F6H-pB7WG2 effectors together with the proAGL18:LUC reporters into tobacco leaves, the activity of LUC was significantly lower than that of blank control of pB7WG2 and proAGL18:LUC co-transformed tobacco leaves (Figures 4F,G), thus demonstrating that GmIDD could inhibit the transcriptional activation activities of AGL18 gene. However, the LUC activity of the floral repressor GmRAV (Zhao et al., 2008) and proAGL18:LUC co-transformed tobacco leaves showed no significant difference compared with the blank control. Together, our results suggested that GmIDD protein significantly repressed the expression of AGL18 by directly binding to its promoter.
GmIDD Affects the Expression of Flowering Time-Related Genes
Overexpression of GmIDD shortened the flowering time of Arabidopsis. The expression levels of flowering time-related genes (including CO, FT, FLC, SOC1, LFY, and AP1) in the 15-day-old GmIDD-transgenic seedlings were further investigated to determine the mechanism of GmIDD regulating flowering time. The results showed that the mRNA levels of FT, floral homeotic gene AP1, floral meristem identity gene LFY and floral integrator gene SOC1 were obviously increased in the GmIDD-ox transgenic plants compared to WT plants, but they were significantly decreased in idd mutants. In 35 S:GmIDD/idd complementary lines, the down-regulated trend of FT, AP1, LFY, and SOC1 genes were rescued (Figures 5B,D–F). The expression of CO, FLC and the other genes functioning in the autonomous pathway showed no significant difference compared to WT (Figures 5A,C). FLC is a floral repressor of the vernalization and autonomous pathway and GmIDD might not affect flowering time through the autonomous pathway. Taken together, GmIDD inhibited the transcriptional activities of AGL18 gene, and induced the expression of FT gene to promote flowering in Arabidopsis.
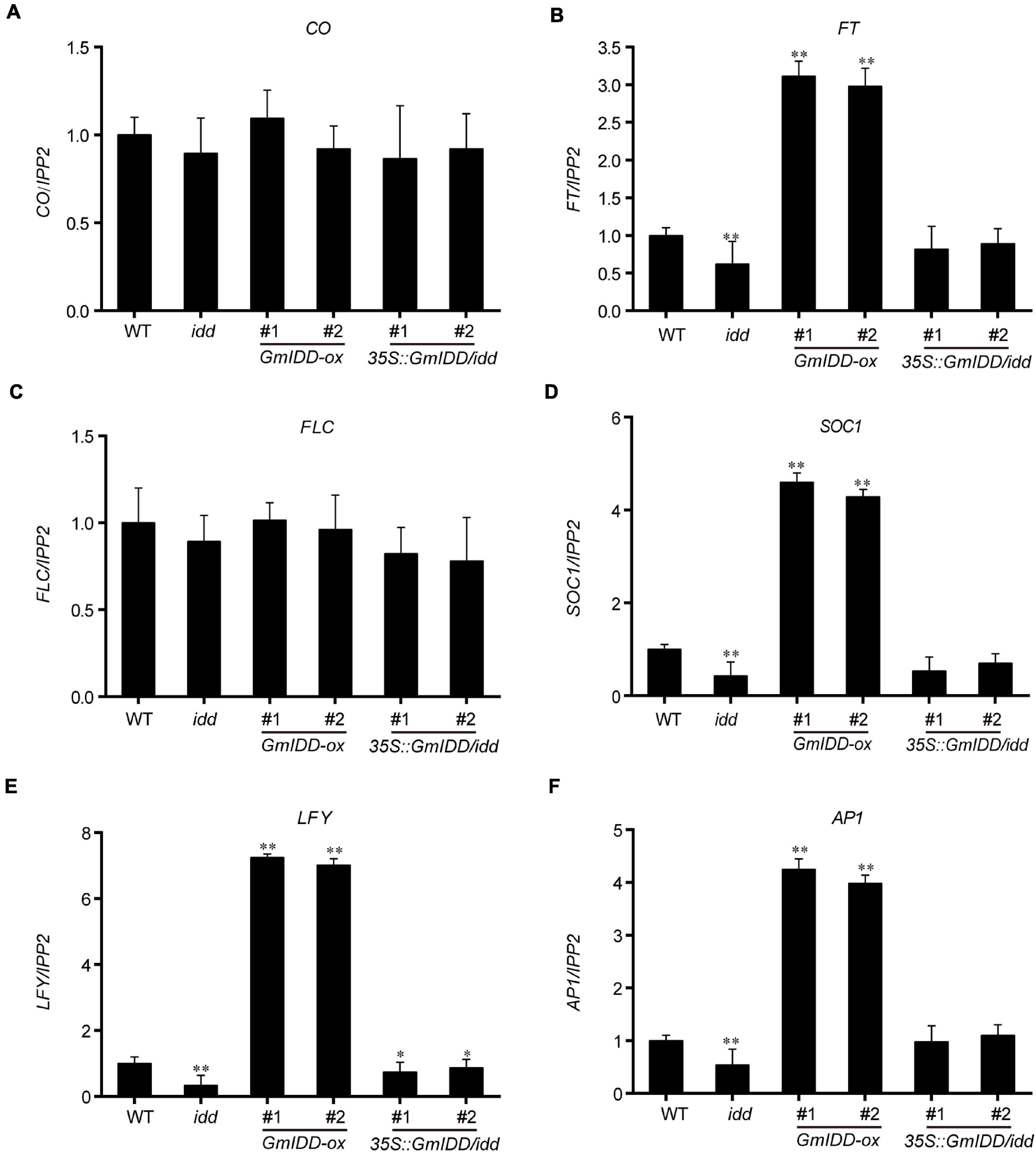
Figure 5. Expression levels of flowering time-related genes in GmIDD-ox, idd mutants, 35S:GmIDD/idd restoration and WT Arabidopsis plants were investigated by qRT-PCR. WT and GmIDD-transgenic plants were cultured in LDs for 15 days, and sampled 12 h after dawn for qRT-PCR analysis the expression levels of flowering time-related genes to determine the mechanism of GmIDD regulating flowering time. (A–F) Relative expression of the CO, FT, FLC, SOC1, LFY, and AP1, respectively. For each experiment, three technical replicates were conducted. Data shown are mean ± SD of three independent experiments, the significant differences between GmIDD-transgenic and WT plants are indicated by asterisks (∗P < 0.05 and ∗∗P < 0.01, Student’s t-test).
Discussion
INDETERMINATE DOMAIN (IDD) is a plant-specific subfamily of C2H2 zinc finger transcription factors (Colasanti et al., 2006; Seo et al., 2011). IDD transcription factor family shares a highly conserved N-terminal domain (ID domain) consisting of two C2H2, two C2HC zinc finger domains and a nuclear localization signal (Englbrecht et al., 2004). The functions of some members of the IDD transcription factor family have been identified, particularly in Arabidopsis, maize, and rice. However, the function of IDD transcription factor family members in soybean has not been reported. This study analyzed the structural domains of GmIDD protein and found that GmIDD consisted of four zinc finger domains: two types of C2H2 (72-92 aa and 114–142 aa) and two types of C2HC (149–169 aa, and 176–195 aa) (Figure 1A). According to the subcellular localization of GmIDD in tobacco leaf cells, the GmIDD-GFP fusion protein was specifically localized on the nucleus of tobacco leaf cells, which indicated that GmIDD was a predicted nuclear transcriptional regulator and performed its biological functions in the plant nucleus (Figure 1D).
Soybean is a typical SD plant that is particularly sensitive to photoperiod response. Photoperiod is one of the main climatic factors that determine soybean floral development (Câmara et al., 1997). Maize ID1 gene expression and protein levels were basically not affected by the change of day length and did not follow the circadian rhythm pattern, indicating that the expression of ID1 was not controlled by photoperiod (Coneva et al., 2007). Similar to ID1, rice Ehd2 was not regulated by daylength, but Ehd2 was involved in photoperiod inducing pathway (Matsubara et al., 2008). The expression of Arabidopsis AtIDD8 gene level was basically not affected by the change of day length, but might be involved in photoperiod inducing pathway to promote Arabidopsis flowering (Seo et al., 2011). Expression pattern analysis of GmIDD showed that expression level of GmIDD was induced by SDs and maintained a stable circadian rhythm under SDs (Figures 2A,B). GmIDD-ox Arabidopsis plants showed early flowering phenotype under LDs and SDs. The idd mutants showed late flowering phenotype under LDs but not SDs. The phenotypes of 35S:GmIDD/idd complementary lines were rescued compared with idd mutants (Figure 3A). Therefore, GmIDD expression was regulated by daylength and might be involved in promoting of Arabidopsis flowering time. The different photoperiodic responses of Ehd2 and maize ID1 could be due to functional differentiation of downstream genes (Matsubara et al., 2008). This may also be the reason why the response of GmIDD to the change of day length was different from that of Ehd2 and ID1, but this speculation has not been confirmed. Both ID1 and Ehd2 were specifically expressed in developing leaves (Kozaki et al., 2004; Wong and Colasanti, 2007). GmIDD mRNA in soybean tissues accumulated most abundantly in pods (Figure 2D), but was present at very low levels in leaves, patterns that are different from ID1 to Ehd2. ID1 regulated a leaf-derived floral inductive signal that might be unique to monocots (Colasanti et al., 2006). Soybean belongs to dicotyledonous. The mechanism of GmIDD regulating flowering in soybean might be different from ID1 and needs to be clarified. Arabidopsis AtIDD8 transcript levels were relatively high in vegetative organs, but at a lower level in pods (Seo et al., 2011). This suggests that GmIDD and AtIDD8 may be functionally distinct in some aspects, although both promote Arabidopsis flowering.
Maize ID1 is a founding member of the plant-specific zinc finger protein family, which consists of highly conserved amino acid sequences in the ID domain (Colasanti et al., 1998). Previous studies had found that ID1, as a regulator of gene expression, bound to specific 11 bp DNA consensus motif 5-TTTGTCG/CT/CT/aT/aT-3 in vitro. It was also proposed that all proteins with ID domains had unique DNA binding characteristics and could recognize the same DNA target sequence (Kozaki et al., 2004). Furthermore, it also showed that AtIDD8 regulated SUS4 by binding with the conserved CTTTTGTCC motif of its promoter (Seo et al., 2011). To identify possible targets of GmIDD protein, the target genes of GmIDD binding were predicted by ChIP-seq analysis using GmIDD-ox and WT Arabidopsis as materials. A flowering-related gene AGL18 was finally identified by screening the genes that were bound by GmIDD to the promoter region. AGL18 is a member of the MADS-box gene family. MADS-box proteins influenced root growth of Arabidopsis (Zhang and Forde, 1998), and also influenced development of ovules, fruits, and seed coat (Nesi et al., 2002; Pinyopich et al., 2003). In addition, AGL18 was a floral repressor in Arabidopsis and located upstream of the FT (Adamczyk et al., 2007). AGL18 promoter region has a continuous TTTTGGTCC motif similar to the reported AtIDD8 and SUS4 binding motif CTTTTGTCC. This difference might be due to differences between species. AGL18 was highly enriched in chromatin of GmIDD-ox transgenic lines precipitated by anti-marker antibodies (Figure 4D). The presence of GmIDD protein reduced the transcriptional activity of AGL18 (Figures 4F,G). qRT-PCR analysis of GmIDD-ox plants showed that the expression level of AGL18 decreased (Figure 4E) while mRNA levels of FT, AP1, LFY, and SOC1 increased significantly (Figures 5B,D–F).
Conclusion
In conclusion, the results of this study indicated that GmIDD molecular regulatory mechanism played a key role in the regulation of flowering in transgenic Arabidopsis. GmIDD binding with TTTTGGTCC motif of target gene AGL18 promoter inhibited the expression of AGL18, thus weakened the inhibition of AGL18 on FT and finally promoted flowering of Arabidopsis. The CO/FT module had been demonstrated in Arabidopsis flowering regulation. In this module, CONSTANS (CO) transcription factor promoted the transcription of FT. FT protein interacted with FD-encoded transcription factor to activate downstream floral organ genes and thus induced flowering (Kardailsky et al., 1999; Valverde et al., 2004; Abe et al., 2005). It has been proposed that the common CO/FT induction pathway was conserved during photoperiodic regulation of flowering in Arabidopsis and rice (Matsubara et al., 2008). In rice, Ehd2 was located in the upstream of HEADING DATE1 (Hd1) (a CO ortholog in rice) and promoted the expression of FT by up-regulating the expression of Hd1, thus promoting flowering. However, the overexpression of GmIDD promoted the expression of FT while the transcription level of CO remained unchanged (Figures 5A,B). This phenomenon showed that the functions of members of IDD transcription factor family had some similarities but also some differences. Therefore, the molecular regulation mechanism of GmIDD in soybean needs to be further investigated.
Data Availability Statement
The datasets presented in this study can be found in online repositories. The names of the repository/repositories and accession number(s) can be found below: NCBI-SRA database under the BioProject no. PRJNA681294 and accession Nos. SRR13165421 and SRR13165420 for the ChIP-seq data.
Author Contributions
XY performed the gene cloning and transformation and ChIP assays. YZ and JSh performed the phenotype observations and measurements. JSu, DL, and XZ performed the dual-luciferase assay and data analysis. XY, LZ, and WL wrote the manuscript. All authors contributed to the article and approved the submitted version.
Funding
This study was financially supported by the National Natural Science Foundation of China (31771820 and 32072086), Heilongjiang Province Natural Science Foundation (ZD2020C002), Chinese Key Projects of Soybean Transformation (2016ZX08004-005), and Key Special Project National Key Research and Development Program “seven crop breeding” (2016YFD0101005). None of the funding bodies participated in the design of the study, collection, analysis, and interpretation of data, or writing the manuscript.
Conflict of Interest
The authors declare that the research was conducted in the absence of any commercial or financial relationships that could be construed as a potential conflict of interest.
Supplementary Material
The Supplementary Material for this article can be found online at: https://www.frontiersin.org/articles/10.3389/fpls.2021.629069/full#supplementary-material
Footnotes
- ^ https://www.arabidopsis.org/
- ^ https://www.ge.com/
- ^ http://www.heliosense.com/
- ^ https://phytozome.jgi.doe.gov/
References
Abe, M., Kobayashi, Y., Yamamoto, S., Daimon, Y., Yamaguchi, A., Ikeda, Y., et al. (2005). FD, a bZIP protein mediating signals from the floral pathway integrator FT at the shoot apex. Science 309, 1052–1056. doi: 10.1126/science.1115983
Adamczyk, B. J., Lehti-Shiu, M. D., and Fernandez, D. E. (2007). The MADS domain factors AGL15 and AGL18 act redundantly as repressors of the floral transition in Arabidopsis. Plant J. 50, 1007–1019. doi: 10.1111/j.1365-313X.2007.03105.x
Ali, F., Qanmber, G., Li, Y., Ma, S., Lu, L., Yang, Z., et al. (2019). Genome-wide identification of Gossypium INDETERMINATE DOMAIN genes and their expression profiles in ovule development and abiotic stress responses. J. Cotton Res. 2:3.
Câmara, G. M. S., Sediyama, T., Dourado-Neto, D., and Bernardes, M. S. (1997). Influence of photoperiod and air temperature on the growth, flowering and maturation of soybean (Glycine max (L.) Merrill). Sci. Agric. 54, 149–154.
Clough, S., and Bent, A. (1998). Floral dip: a simplified method for agrobacterium-mediated transformation of Arabidopsis thaliana. Plant J. 16, 735–743. doi: 10.1046/j.1365-313x.1998.00343.x
Cober, E. R., and Morrison, M. J. (2010). Regulation of seed yield and agronomic characters by photoperiod sensitivity and growth habit genes in soybean. Theor. Appl. Genet. 120, 1005–1012. doi: 10.1007/s00122-009-1228-6
Colasanti, J., Tremblay, R., Wong, A., Coneva, V., Kozaki, A., and Mable, B. (2006). The maize INDETERMINATE1 flowering time regulator defines a highly conserved zinc finger protein family in higher plants. BMC Genom. 7:158. doi: 10.1186/1471-2164-7-158
Colasanti, J., Yuan, Z., and Sundaresan, V. (1998). The indeterminate gene encodes a zinc finger protein and regulates a leaf-generated signal required for the transition to flowering in maize. Cell 93, 593–603. doi: 10.1016/s0092-8674(00)81188-5
Coneva, V., Zhu, T., and Colasanti, J. (2007). Expression differences between normal and indeterminate1 maize suggest downstream targets of ID1, a floral transition regulator in maize. J. Exp. Bot. 58, 3679–3693. doi: 10.1093/jxb/erm217
Cui, H., Levesque, M., Vernoux, T., Jung, J., Paquette, A., Gallagher, K., et al. (2007). An evolutionarily conserved mechanism delimiting SHR movement defines a single layer of endodermis in plants. Science 316, 421–425. doi: 10.1126/science.1139531
Englbrecht, C., Schoof, H., and Böhm, S. (2004). Conservation, diversification and expansion of C2H2 zinc finger proteins in the Arabidopsis thaliana genome. BMC Genom. 5:39. doi: 10.1186/1471-2164-5-39
Funck, D., Clauss, K., Frommer, W. B., and Hellmann, H. A. (2012). The Arabidopsis CstF64-like RSR1/ESP1 protein participates in glucose signaling and flowering time control. Front. Plant Sci. 3:80. doi: 10.3389/fpls.2012.00080
Gontarek, B. C., Neelakandan, A. K., Wu, H., and Becraft, P. W. (2016). NKD transcription factors are central regulators of maize endosperm development. Plant Cell 28, 2916–2936. doi: 10.1105/tpc.16.00609
Heinz, S., Benner, C., Spann, N., Bertolino, E., Lin, Y., Laslo, P., et al. (2010). Simple combinations of lineage-determining transcription factors prime cis-regulatory elements required for macrophage and B cell identities. Mol. cell 38, 576–589. doi: 10.1016/j.molcel.2010.05.004
Kantolic, A. G., and Slafer, G. A. (2007). Development and seed number in indeterminate soybean as affected by timing and duration of exposure to long photoperiods after flowering. Ann. Bot. 99, 925–933. doi: 10.1093/aob/mcm033
Kardailsky, I., Shukla, V., Ahn, J., Dagenais, N., Christensen, S., Nguyen, J., et al. (1999). Activation tagging of the floral inducer FT. Science 286, 1962–1965. doi: 10.1126/science.286.5446.1962
Kozaki, A., Hake, S., and Colasanti, J. (2004). The maize ID1 flowering time regulator is a zinc finger protein with novel DNA binding properties. Nucleic Acids Res. 32, 1710–1720. doi: 10.1093/nar/gkh337
Levesque, M., Vernoux, T., Busch, W., Cui, H., Wang, J., Blilou, I., et al. (2006). Whole-genome analysis of the SHORT-ROOT developmental pathway in Arabidopsis. PLoS Biol. 4:e143. doi: 10.1371/journal.pbio.0040143
Long, Y. C., Smet, W., Cruz-Ramirez, A., Castelijns, B., de Jonge, W., Mahonen, A. P., et al. (2015). Arabidopsis BIRD Zinc finger proteins jointly stabilize tissue boundaries by confining the cell fate regulator SHORT-ROOT and contributing to fate specification. Plant Cell 27, 1185–1199. doi: 10.1105/tpc.114.132407
Matsubara, K., Yamanouchi, U., Wang, Z.-X., Minobe, Y., Izawa, T., and Yano, M. (2008). Ehd2, a rice ortholog of the maize INDETERMINATE1 gene, promotes flowering by up-regulating Ehd1. Plant Physiol. 148, 1425–1435. doi: 10.1104/pp.108.125542
Nesi, N., Debeaujon, I., Jond, C., Stewart, A. J., Jenkins, G. I., Caboche, M., et al. (2002). The TRANSPARENT TESTA16 locus encodes the ARABIDOPSIS BSISTER MADS domain protein and is required for proper development and pigmentation of the seed coat. Plant Cell 14, 2463–2479. doi: 10.1105/tpc.004127
Park, S. J., Kim, S. L., Lee, S., Je, B. I., Piao, H. L., Park, S. H., et al. (2008). Rice indeterminate 1 (OsId1) is necessary for the expression of Ehd1 (Early heading date 1) regardless of photoperiod. Plant J. 56, 1018–1029. doi: 10.1111/j.1365-313X.2008.03667.x
Pinyopich, A., Ditta, G., Savidge, B., Liljegren, S., Baumann, E., Wisman, E., et al. (2003). Assessing the redundancy of MADS-box genes during carpel and ovule development. Nature 424, 85–88. doi: 10.1038/nature01741
Seo, P. J., Ryu, J., Kang, S. K., and Park, C. M. (2011). Modulation of sugar metabolism by an INDETERMINATE DOMAIN transcription factor contributes to photoperiodic flowering in Arabidopsis. Plant J. 65, 418–429. doi: 10.1111/j.1365-313X.2010.04432.x
Sheikh, A., Raghuram, B., Eschen-Lippold, L., Scheel, D., Lee, J., and Sinha, A. (2014). Agroinfiltration by cytokinin-producing Agrobacterium sp. strain GV3101 primes defense responses in Nicotiana tabacum. Mol. Plant Microbe Interact. 27, 1175–1185. doi: 10.1094/mpmi-04-14-0114-r
Song, Y., Smith, R., To, B., Millar, A., and Imaizumi, T. (2012). FKF1 conveys timing information for CONSTANS stabilization in photoperiodic flowering. Science 336, 1045–1049. doi: 10.1126/science.1219644
Valverde, F., Mouradov, A., Soppe, W., Ravenscroft, D., Samach, A., and Coupland, G. (2004). Photoreceptor regulation of CONSTANS protein in photoperiodic flowering. Science 303, 1003–1006. doi: 10.1126/science.1091761
Welch, D., Hassan, H., Blilou, I., Immink, R., Heidstra, R., and Scheres, B. (2007). Arabidopsis JACKDAW and MAGPIE zinc finger proteins delimit asymmetric cell division and stabilize tissue boundaries by restricting SHORT-ROOT action. Genes Dev. 21, 2196–2204. doi: 10.1101/gad.440307
Wong, A., and Colasanti, J. (2007). Maize floral regulator protein INDETERMINATE1 is localized to developing leaves and is not altered by light or the sink/source transition. J. Exp. Bot. 58, 403–414. doi: 10.1093/jxb/erl206
Wu, C., You, C., Li, C., Long, T., Chen, G., Byrne, M. E., et al. (2008). RID1, encoding a Cys2/His2-type zinc finger transcription factor, acts as a master switch from vegetative to floral development in rice. Proc. Natl. Acad. Sci. U.S.A. 105, 12915–12920. doi: 10.1073/pnas.0806019105
Yi, G., Neelakandan, A. K., Gontarek, B. C., Vollbrecht, E., and Becraft, P. W. (2015). The naked endosperm genes encode duplicate INDETERMINATE domain transcription factors required for maize endosperm cell patterning and differentiation. Plant Physiol. 167, 443–456. doi: 10.1104/pp.114.251413
Zhang, H., and Forde, B. (1998). An Arabidopsis MADS box gene that controls nutrient-induced changes in root architecture. Science 279, 407–409. doi: 10.1126/science.279.5349.407
Zhao, L., Li, M. M., Xu, C. J., Yang, X., Li, D. M., Zhao, X., et al. (2018). Natural variation in GmGBP1 promoter affects photoperiod control of flowering time and maturity in soybean. Plant J. 96, 147–162. doi: 10.1111/tpj.14025
Zhao, L., Luo, Q., Yang, C., Han, Y., and Li, W. (2008). A RAV-like transcription factor controls photosynthesis and senescence in soybean. Planta 227, 1389–1399. doi: 10.1007/s00425-008-0711-7
Keywords: soybean, photoperiod, flowering, AGL18, GmIDD
Citation: Yang X, Zhang Y, Shan J, Sun J, Li D, Zhang X, Li W and Zhao L (2021) GmIDD Is Induced by Short Days in Soybean and May Accelerate Flowering When Overexpressed in Arabidopsis via Inhibiting AGAMOUS-LIKE 18. Front. Plant Sci. 12:629069. doi: 10.3389/fpls.2021.629069
Received: 07 December 2020; Accepted: 22 January 2021;
Published: 26 February 2021.
Edited by:
Chris Helliwell, Commonwealth Scientific and Industrial Research Organization (CSIRO), AustraliaReviewed by:
Joanna Putterill, The University of Auckland, New ZealandKazuki Matsubara, Institute of Crop Science (NARO), Japan
Copyright © 2021 Yang, Zhang, Shan, Sun, Li, Zhang, Li and Zhao. This is an open-access article distributed under the terms of the Creative Commons Attribution License (CC BY). The use, distribution or reproduction in other forums is permitted, provided the original author(s) and the copyright owner(s) are credited and that the original publication in this journal is cited, in accordance with accepted academic practice. No use, distribution or reproduction is permitted which does not comply with these terms.
*Correspondence: Lin Zhao, emhhb2xpbm5lYXVAMTI2LmNvbQ==