- 1Tsinghua-Peking Center for Life Science, and MOE Key Laboratory of Bioinformatics, School of Life Sciences, Tsinghua University, Beijing, China
- 2Shenzhen Branch, Guangdong Laboratory for Lingnan Modern Agriculture, Genome Analysis Laboratory of the Ministry of Agriculture and Rural Affairs, Agricultural Genomics Institute at Shenzhen, Chinese Academy of Agricultural Sciences, Shenzhen, China
- 3Shenzhen Key Laboratory of Agricultural Synthetic Biology, Agricultural Genomics Institute at Shenzhen, Chinese Academy of Agricultural Sciences, Shenzhen, China
- 4Hunan Provincial Key Laboratory of Phytohormones and Growth Development, College of Bioscience and Biotechnology, Hunan Agricultural University, Changsha, China
Amino acids are the building blocks of biomacromolecules in organisms, among which isoleucine (Ile) is the precursor of JA-Ile, an active molecule of phytohormone jasmonate (JA). JA is essential for diverse plant defense responses against biotic and abiotic stresses. Botrytis cinerea is a necrotrophic nutritional fungal pathogen that causes the second most severe plant fungal disease worldwide and infects more than 200 kinds of monocot and dicot plant species. In this study, we demonstrated that Ile application enhances plant resistance against B. cinerea in Arabidopsis, which is dependent on the JA receptor COI1 and the jasmonic acid-amido synthetase JAR1. The mutant lib with higher Ile content in leaves exhibits enhanced resistance to B. cinerea infection. Furthermore, we found that the exogenous Ile application moderately enhanced plant resistance to B. cinerea in various horticultural plant species, including lettuce, rose, and strawberry, suggesting a practical and effective strategy to control B. cinerea disease in agriculture. These results together showed that the increase of Ile could positively regulate the resistance of various plants to B. cinerea by enhancing JA signaling, which would offer potential applications for crop protection.
Introduction
Botrytis cinerea is a necrotrophic nutritional fungal pathogen that infects more than 200 kinds of monocot and dicot plants and subsequently causes gray mold disease, which is the second most common plant fungal disease worldwide (Williamson et al., 2007; Dean et al., 2012; AbuQamar et al., 2017). B. cinerea infection may occur from the seedling stage to fruit ripening stage and even during the storage and transport in the retail chain (Dean et al., 2012). Global costs are greater than €1 billion annually for B. cinerea control, which includes agronomic and horticultural practices, fungicides, biological control, and postharvest treatments (Dean et al., 2012; Petrasch et al., 2019). However, increasing fungicide resistance is a severe challenge to fungicide applications and the agricultural management practice for B. cinerea (Kretschmer et al., 2009; Rupp et al., 2016). In addition, Bacillus subtilis-based biological control is also limited by insufficient applicability in the field and high costs (Petrasch et al., 2019). Therefore, safer, pollution-free, and affordable strategies for controlling B. cinerea are worthy of discovery.
Amino acids are the building blocks of biomacromolecules in organisms, and they are also used as water-soluble fertilizers to promote plant growth and improve crop quality (Wang et al., 2014; Aghaei et al., 2019; Rahmani Samani et al., 2019). For instance, the application of amino acids promotes root growth and increases leaf area, chlorophyll content, and dry weight in rapeseed (Wang et al., 2014). Some amino acids are precursor components for the synthesis of various secondary defensive metabolites. For instance, aliphatic amino acids (i.e., alanine, leucine, isoleucine [Ile], methionine, and valine) and aromatic amino acids (i.e., phenylalanine, tryptophan, and tyrosine) involve in the formation of aliphatic, aromatic, and indolic glucosinolates (Halkier and Gershenzon, 2006; Albinsky et al., 2010). The hydrolysis products of glucosinolates serve as defense compounds against herbivores and pathogens and act as cancer-preventing agents in humans (Halkier and Gershenzon, 2006). In addition, arginine and citrulline activate the nitric oxide (NO) cycle to restore the susceptibility of brown planthoppers to the insecticide imidacloprid (Elzaki et al., 2020). Glutamate activates the salicylic acid (SA) pathway to trigger the blast resistance in rice (Kadotani et al., 2016). Despite the apparent relevance between amino acid metabolism and disease resistance, the molecular basis for amino acid and plant resistance to B. cinerea has not been elucidated to date.
Isoleucine is synthesized from threonine under the catalysis by threonine deaminase (TD), encoded by the L-O-METHYLTHREONINE-RESISTANT 1 (OMR1) gene in Arabidopsis (Kang et al., 2006; Binder, 2010; Yu et al., 2013). A previous study reported that the low Ile biosynthesis mutant (lib) with a T-DNA insertion in the OMR1 gene exhibits reduced Ile levels in roots and defects in cell proliferation and expansion during root development (Yu et al., 2013). Furthermore, Ile also conjugates with jasmonic acid under the catalysis by jasmonic acid-amido synthetase JAR1 to form JA-Ile, a bioactive molecule of jasmonates (JAs) (Staswick et al., 2002; Staswick and Tiryaki, 2004; Fonseca et al., 2009; Yan et al., 2016). Subsequently, JA-Ile binds to its receptor COI1 (Xie et al., 1998; Xu et al., 2002; Thines et al., 2007; Yan et al., 2009, 2013) to mediate plant resistance against B. cinerea infection (Thaler et al., 2004; Abuqamar et al., 2008; Song et al., 2013). In the JA biosynthetic pathway, α-linolenic acid is catalyzed and converted into jasmonic acid through a series of biosynthetic enzymes, including allene oxide synthase (AOS) and 12-oxophytodienoate reductase 3 (OPR3) (Wasternack and Hause, 2013).
In this study, we screened 20 proteinogenic amino acids and found that exogenous application of Ile enhanced plant resistance to B. cinerea in Arabidopsis. Our results showed that the Ile-enhanced resistance is modulated via JA signaling through COI1 and JAR1. Notably, the application of Ile on horticultural plant species, such as lettuce, white rose, red rose, and strawberry, moderately enhanced resistance to B. cinerea, suggesting that increasing Ile levels to improve disease resistance has broad applicability and great potential in agriculture.
Results
Exogenous Application of Isoleucine Enhances Plant Resistance to B. cinerea
We sprayed 20 amino acids on Arabidopsis wild-type (WT) plants Col-0 for 2 days and subsequently inoculated B. cinerea spores on the leaves to examine the effects of amino acids on plant defense against B. cinerea infection. The lesion area on leaves caused by B. cinerea infection was measured on the third day after inoculation. Ile treatment (10 mM) significantly reduced the leaf lesion size compared with the control (Mock) treatment (Figure 1A). Applications of Ala, Leu, Val, and Met also slightly reduced lesion area from 7 to 13% (Figure 1A). In summary, only exogenous application of Ile obviously enhanced plant resistance to B. cinerea, while other amino acids exhibited no significant difference compared with the Mock treatment (Figure 1A).
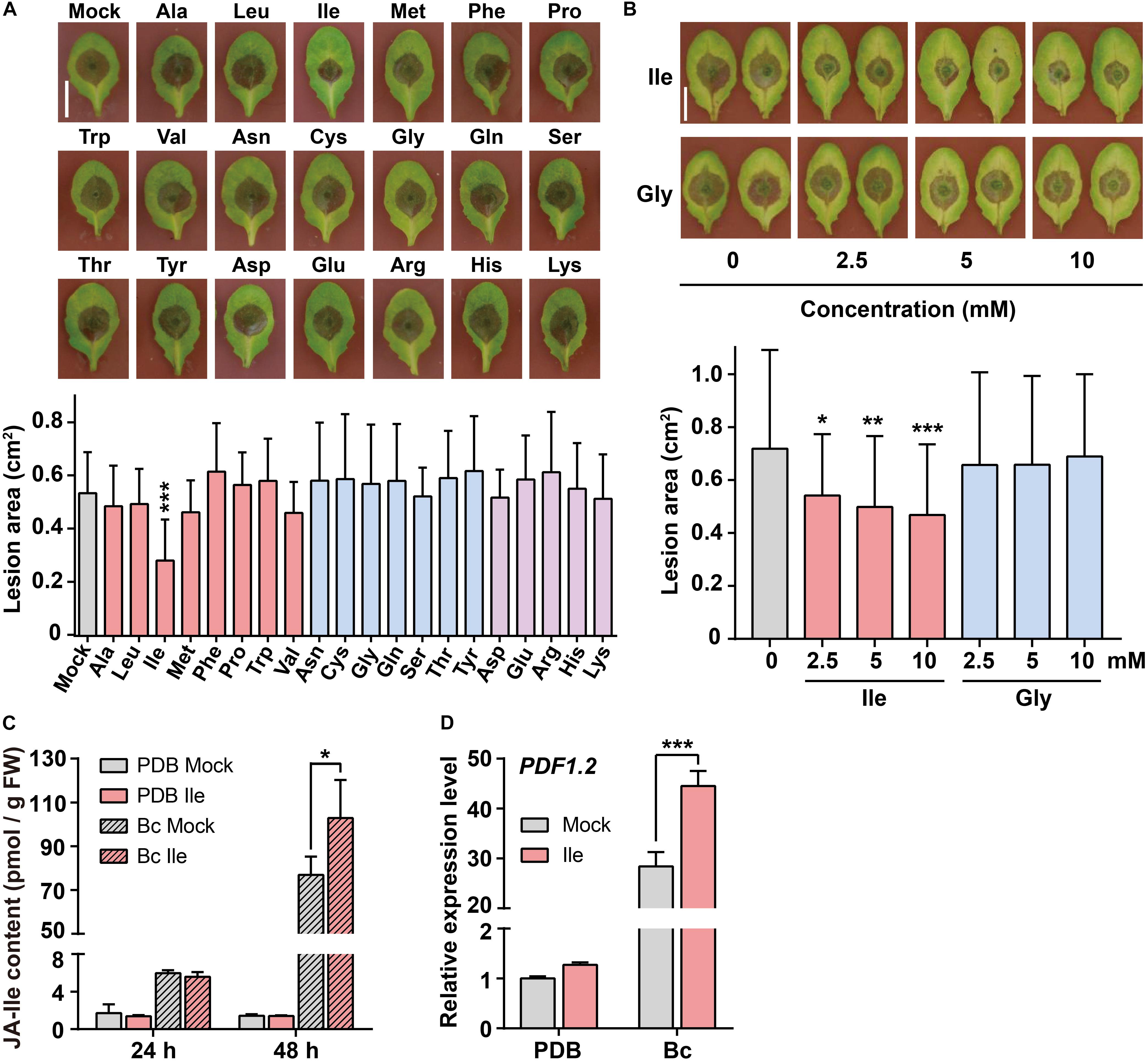
Figure 1. Application of isoleucine (Ile) enhances plant resistance to Botrytis cinerea via elevating JA-Ile (jasmonoyl-isoleucine) level. (A) Representative phenotype and lesion area of wild-type (WT) leaves pretreated with different amino acids after B. cinerea infection for 3 days. Five-week-old WT plants were pretreated with 10 mM amino acids for 2 days before B. cinerea inoculation, including non-polar amino acids (i.e., Ala, Leu, Ile, Met, Phe, Pro, Trp, and Val), polar amino acids (i.e., Asn, Cys, Gly, Gln, Ser, Thr, and Tyr), acidic amino acids (i.e., Asp and Glu), and alkaline amino acids (i.e., Arg, His, and Lys). 0.05% Tween 20 solution (Mock) was used as a control. Scale: 1 cm. Data are means ± SD (n = 28–47 leaves). (B) Representative phenotype and lesion area of WT leaves after B. cinerea infection for 3 days pretreated with different concentrations of Ile or Gly. Five-week-old plants were pretreated with 0, 2.5, 5, and 10 mM Ile or Gly for 2 days. 0.05% Tween 20 solution (0 mM) was used as control. Scale: 1 cm. Data are means ± SD (n = 24–43 leaves). (C,D) JA-Ile concentration (C) and transcript level of the defensive gene PDF1.2 (D) in WT leaves after indicated treatments. Four-week-old WT plants were pretreated with 0.05% Tween 20 solution (Mock) or 10 mM Ile for 2 days, and then leaves were sprayed with Potato Dextrose Broth (PDB) or B. cinerea spores suspension (Bc) for 24 or 48 h. Then, the 7th–9th rosette leaves were collected for quantification of JA-Ile contents, and PDF.1.2 transcript level (48 h). Data are means ± SD. In panel (C), n = 4 samples. In panel (D), n = 3 samples, each sample contains three leaves; ACTIN8 is used as the internal control. Statistical significances were calculated via Student’s t-test Mock and amino acid treatment (*p < 0.05; **p < 0.01; ***p < 0.001).
We further examined the effects of different concentrations of Ile on plant resistance to B. cinerea infection. As shown in Figure 1B, the lesion size on WT leaves was reduced ∼24% when plants were pretreated with 2.5 mM Ile, ∼30% when pretreated with 5 mM Ile, and ∼38% when pretreated with 10 mM Ile. The lesion area on WT leaves pretreated with glycine (Gly) at various concentrations has no significant difference compared with solvent treatment (Figure 1B). These results suggested that the exogenous application of Ile enhanced plant defense against B. cinerea in a concentration-dependent manner.
As Ile is the precursor of JA-Ile, an important defensive signal in response to B. cinerea infection (Thaler et al., 2004; Abuqamar et al., 2008; Song et al., 2013), we further measured JA-Ile accumulation using liquid chromatography–tandem mass spectrometry (LC-MS/MS). LC-MS/MS analysis showed that JA-Ile concentration in Ile-pretreated WT leaves was higher than control WT leaves after B. cinerea infection for 48 h (Figure 1C). Consistently, the induction of defensive gene PDF1.2 (PLANT-DEFENSIN1.2) was also stronger in Ile-pretreated WT leaves compared with control WT leaves at 48 h upon B. cinerea infection (Figure 1D). Collectively, Ile-enhanced resistance may be caused by the higher accumulation of JA-Ile.
The Increase of Endogenous Ile Level Enhances Plant Resistance to B. cinerea
The conversion of threonine to 2-oxobutanoate, catalyzed by OMR1 enzyme, is the first and committed step toward Ile biosynthesis in Arabidopsis (Kang et al., 2006; Binder, 2010; Yu et al., 2013). Our results showed that OMR1 gene transcription level was highly induced in B. cinerea-infected leaves (Figure 2A). A previous study reported that lib mutant, a T-DNA insertion in the last exon of OMR1 gene causing a 13-amino-acid truncation at C-terminal region of OMR1 protein, showed reduced Ile levels in roots (Yu et al., 2013). Hence, we further analyzed the disease resistance response of lib mutant to examine the effect of endogenous Ile levels on plant resistance to B. cinerea. Unexpectedly, the lesion area of lib leaves was ∼23% reduced compared with that of WT (Figure 2B). To clarify whether the enhanced resistance to B. cinerea of lib mutant is linked to free amino acid contents, we further detected the amount of free amino acids in the leaves. As shown in Supplementary Table 1 and Figure 2C, the Ile level was significantly increased in lib leaves by approximately 1-fold higher than WT. In addition, we also found that Gly content in lib leaves was higher than that in WT leaves (Supplementary Table 1). However, external Gly treatment on WT leaves failed to enhance plant resistance to B. cinerea (Figure 1B), which further suggested that the enhanced resistance of lib mutant is linked to the higher level of Ile but not Gly. Based on exogenous and endogenous results, we speculated that Ile plays a positive role in plant resistance to B. cinerea.
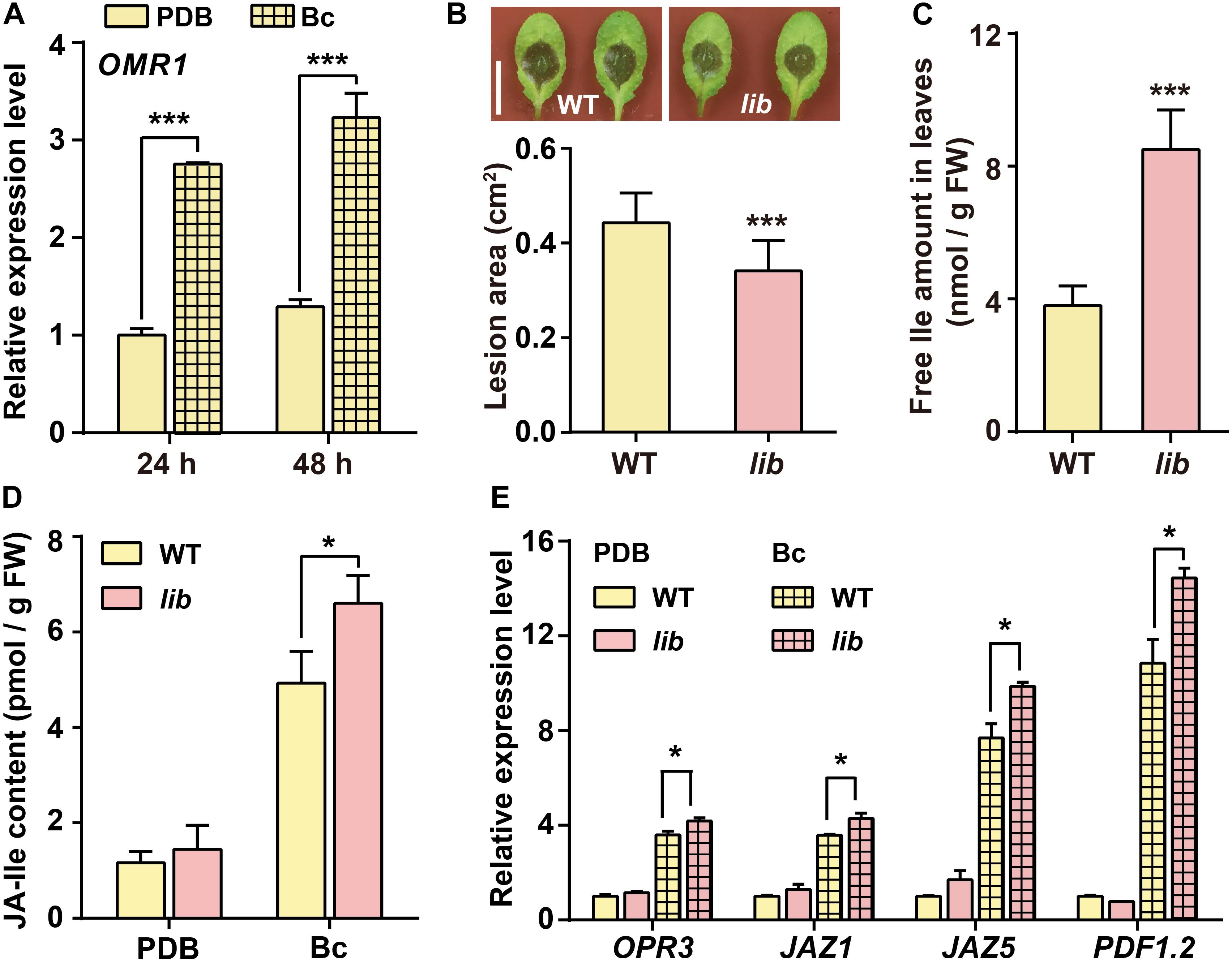
Figure 2. lib mutant shows enhanced plant resistance to B. cinerea. (A) Transcript level of Ile biosynthetic gene OMR1 in WT leaves. Four-week-old WT plants were sprayed with Potato Dextrose Broth (PDB) or B. cinerea spores suspension (Bc) for 24 and 48 h. Data are means ± SD (n = 3 samples, each sample contains three leaves). ACTIN8 was used as the internal control. (B) Representative phenotype and lesion area of WT and lib leaves after B. cinerea infection for 2 days. Data are means ± SD (n = 61–69 leaves). (C) Free Ile amount in the leaves of WT and lib seedlings. Five-day-old seedlings were transferred to vertical MS medium (1.2% agar) plates for 10 days, and then leaves were collected for Ile measurement. Data are means ± SD (n = 7–10). (D,E) JA-Ile content (D) and transcript level of JA biosynthetic gene OPR3, JA-responsive genes JAZ1 and JAZ5, and defensive gene PDF1.2 (E) in the leaves after indicated treatments. Three-week-old WT and lib plants were sprayed with PDB or B. cinerea spores suspension (Bc) for 24 h. Then, rosette leaves were collected for quantification of JA-Ile contents and transcript level. Data are means ± SD. In panel (D) n = 3–7 samples. In panel (E) n = 3 samples, each sample contains three leaves; ACTIN8 is used as the internal control. Asterisks indicate significant differences (Student’s t-test, *p < 0.05; ***p < 0.001).
In line with the enhanced resistance of lib mutant, the transcript level of defensive gene PDF1.2 in lib leaves was higher than that in WT leaves followed by B. cinerea infection for 48 h, whereas OPR3 level in lib leaves was comparable with that in WT (Supplementary Figure 1). Transcriptomic analysis showed that JA-responsive genes are overrepresented around 16 h after B. cinerea infection, suggesting prior JA biosynthesis (Windram et al., 2012). Therefore, we further investigated whether the enhanced disease resistance to B. cinerea of lib mutant was related to JA-Ile biosynthesis and signaling at an earlier stage of B. cinerea infection.
First, after B. cinerea infection, WT and lib leaves were collected for JA-Ile measurement using LC-MS/MS. The LC-MS/MS analysis showed that the JA-Ile content was significantly increased in lib leaves on B. cinerea infection for 24 h. JA-Ile increased to ∼6.6 pmol/g fresh weight (FW) in lib leaves, whereas it increased only to ∼4.9 pmol/g FW in WT leaves (Figure 2D). Consistent with higher JA-Ile contents and stronger disease resistance in lib plants following B. cinerea infection, the transcript levels of the JA biosynthetic gene OPR3, JA-responsive genes JAZ1 and JAZ5, and defensive gene PDF1.2 were significantly induced in lib mutant, which was higher than that in WT plants (Figure 2E). These data demonstrated that an increase of endogenous Ile level could enhance plant disease resistance via triggering JA-Ile biosynthesis and signaling on B. cinerea infection.
Ile-Enhanced Resistance to B. cinerea Depends on Elevated JA-Ile Biosynthesis and Perception
The biosynthesis and perception of JA-Ile are required for plant defense against B. cinerea (Thaler et al., 2004; Abuqamar et al., 2008; Song et al., 2013). Hence, we investigated whether the Ile-enhanced resistance to B. cinerea after Ile application depends on elevated JA-Ile biosynthesis and signaling using jar1-1 (Staswick et al., 2002) and coi1-1 (Xie et al., 1998) mutants. The results showed that Ile application significantly reduced the size of lesion in WT leaves, but it failed to reduce the size of lesion in jar1-1 and coi1-1 mutants (Figures 3A,B). However, treatments with Gly had no significant effect on the lesion size of WT, coi1-1, and jar1-1 mutants (Figures 3A,B). In line with these results, the transcript level of defensive gene PDF1.2 in Ile-pretreated WT leaves on B. cinerea infection was higher than that in Mock-pretreated leaves; however, the acceleration of Ile on PDF1.2 expression on B. cinerea infection was not observed in jar1-1 and coi1-1 mutants (Figure 3C). These results demonstrated that the enhanced resistance to B. cinerea triggered by Ile depends on the elevated JA-Ile biosynthesis and perception through JAR1 and COI1.
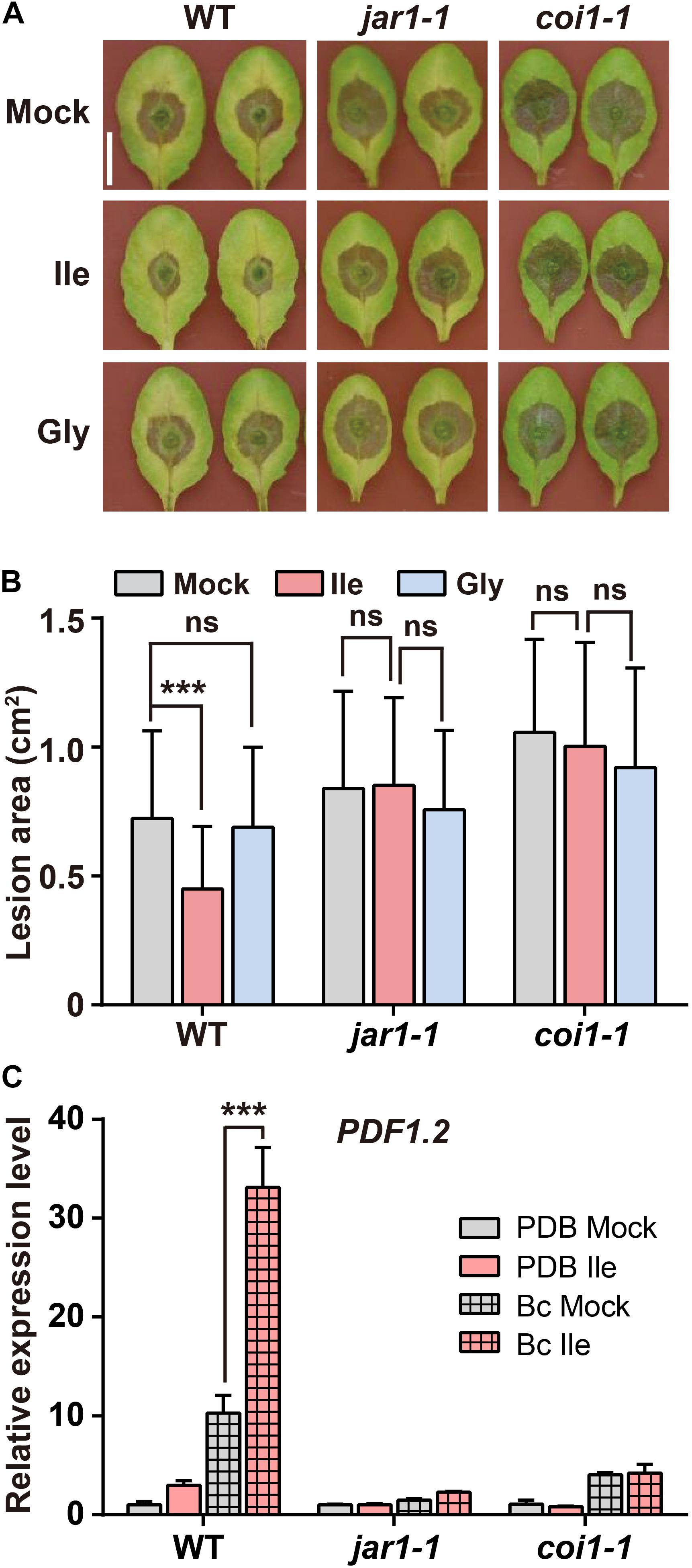
Figure 3. Ile enhances plant resistance to B. cinerea depending on JAR1 and COI1. (A,B) Representative phenotype (A) and lesion area (B) of WT, jar1-1, and coi1-1 leaves after B. cinerea infection for 3 days. Five-week-old plants were pretreated with 0.05% Tween 20 solution (Mock), 10 mM Ile, or 10 mM Gly for 2 days before B. cinerea inoculation. Scale: 1 cm. Data are means ± SD (n = 32–51 leaves). (C) Transcript level of defensive gene PDF1.2 in the leaves of WT, jar1-1, and coi1-1 plants. Four-week-old plants were pretreated with 0.05% Tween 20 solution (Mock) or 10 mM Ile for 2 days. The 7th–9th rosette leaves were detached and inoculated with PDB or B. cinerea spores suspension (Bc) for 48 h, and then collected for quantification of PDF1.2 transcript level. Data are means ± SD (n = 3 samples, each sample contains three leaves). ACTIN8 is used as the internal control. Statistical significances were calculated via Student’s t-test (***p < 0.001, “ns” p > 0.05).
High Endogenous Ile Level Enhances JA Responses to Wounding and MeJA Treatment
In addition to B. cinerea infection, JAs play essential roles in regulating herbivore defenses, wounding responses, fertility, anthocyanin accumulation, UV irradiation, and drought stress (Creelman and Mullet, 1995; Xie et al., 1998; Farmer et al., 2003; Qi et al., 2011; Seo et al., 2011; Wathugala et al., 2012; Yan et al., 2018). Therefore, we performed wounding treatment and MeJA treatment to further investigate whether the increase of Ile level affects other JA responses in addition to B. cinerea infection.
To do so, we first compared the wounding responses in lib, jar1-1, and WT plants. Rosette leaves of these plants were subjected to wounding treatment for 1 h and subsequently collected for the measurement of endogenous JA-Ile concentration using LC-MS/MS (liquid chromatography-tandem mass spectrometry). Consistent with previous studies (Suza and Staswick, 2008; Yan et al., 2016), the induction of JA-Ile level by wounding was impaired in jar1-1 mutants compared with that in WT leaves (Figure 4A). JA-Ile accumulated more dramatically in the wounded leaves of lib mutant. JA-Ile levels increased up to ∼352 pmol/g FW in lib, ∼237 pmol/g FW in WT, and ∼40 pmol/g FW in the jar1-1 mutant (Figure 4A). Consistent with the increased JA-Ile contents in lib mutants, the transcript level of JA-inducible genes in lib leaves, such as OPR3, JAZ1, JAZ5, and JAZ10, was significantly higher than that in WT leaves on wounding treatment (Figure 4B).
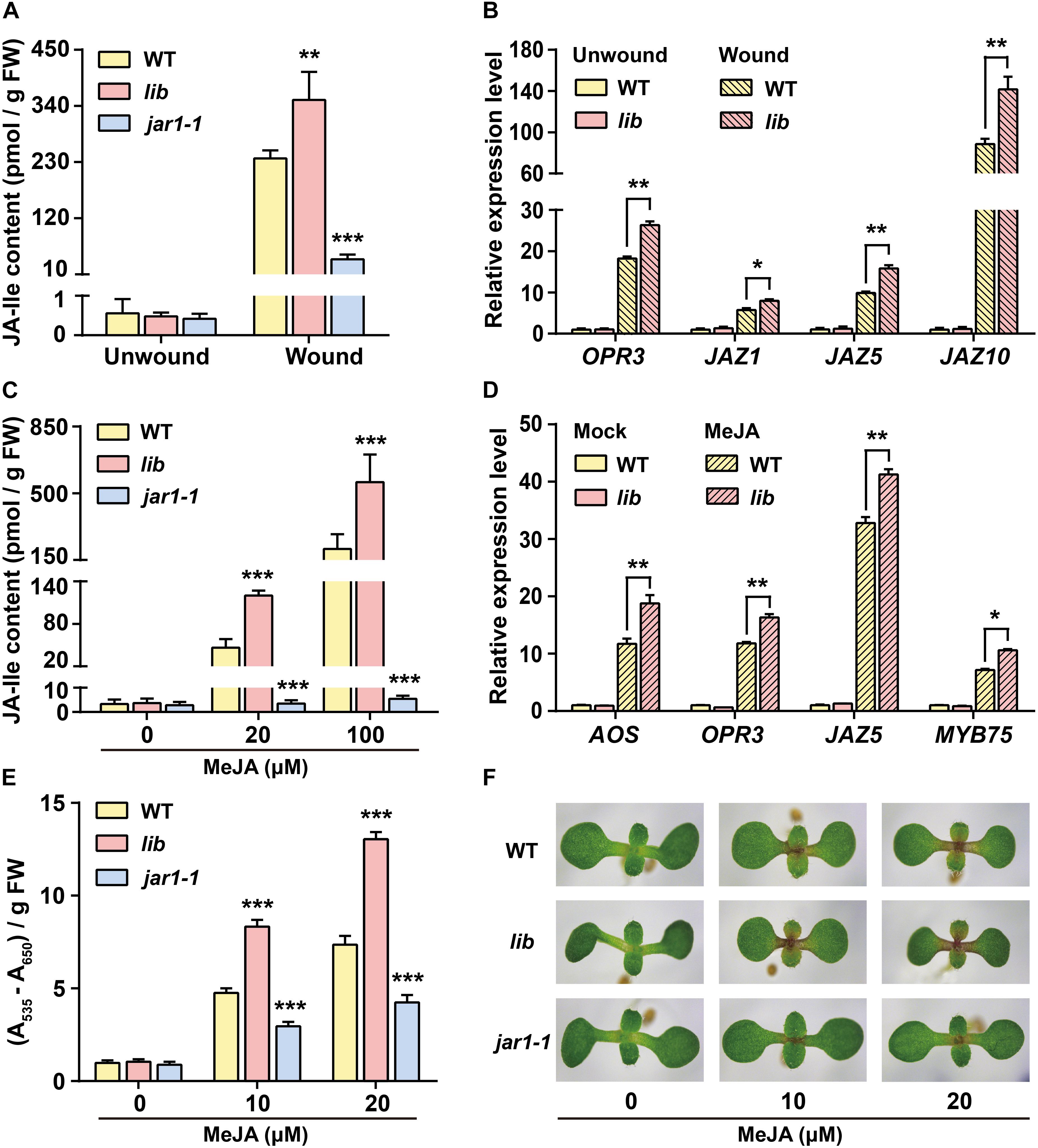
Figure 4. Jasmonate responses are enhanced in lib mutants after wounding and MeJA treatment. (A) JA-Ile content in unwounded and wounded leaves of 4-week-old WT, lib, and jar1-1 seedlings. Data are means ± SD (n = 5–6 samples). (B) Transcript level of JA biosynthetic gene OPR3 and JA-responsive genes JAZ1, JAZ5, and JAZ10 in unwounded and wounded leaves of 4-week-old WT and lib plants. ACTIN8 was used as the internal control. Data are means ± SD (n = 3 samples). (C) JA-Ile content in 14-day-old WT, lib, and jar1-1 seedlings treated with 0, 20, and 100 μM MeJA for 1 h. Data are means ± SD (n = 5–6 samples). (D) Transcript level of the JA biosynthetic genes AOS and OPR3 and the JA-responsive genes JAZ5 and MYB75 in 7-day-old WT and lib seedlings treated with solvent (Mock) or 50 μM MeJA for 1 h. ACTIN8 was used as the internal control. Data are means ± SD (n = 2–3 samples, each sample contains 20 seedlings). (E,F) Anthocyanin contents (E) and representative phenotype (F) of WT, lib, and jar1-1 seedlings grown on MS medium supplemented with 0, 10, and 20 μM MeJA for 8 days. Data are means ± SD (n = 3 samples). Asterisks indicate significant differences compared with WT (Student’s t-test, *p < 0.05; **p < 0.01; ***p < 0.001).
It has been demonstrated by isotope-feeding experiments that exogenous MeJA could be converted into JA and further conjugated with Ile into JA-Ile to activate JA signaling pathway (Tamogami et al., 2008). Therefore, we further examined whether increased endogenous Ile levels in lib leaves enhance its capacity for MeJA-induced JA-Ile biosynthesis. Here, 14-day-old WT, lib, and jar1-1 seedlings were subjected to MeJA treatment for 1 h and then collected for endogenous JA-Ile measurement. On 20 μM MeJA treatment, JA-Ile levels were induced to ∼120 pmol/g FW in lib, to ∼46 pmol/g FW in WT, and ∼3.5 pmol/g FW in jar1-1 plants (Figure 4C). Similarly, on 100 μM MeJA treatment, JA-Ile increased to ∼558 pmol/g FW in lib leaves, ∼208 pmol/g FW in WT, and ∼5.5 pmol/g FW in jar1-1 leaves (Figure 4C). As a result, the induction of JA-Ile biosynthesis in lib leaves was significantly higher than that in WT leaves, suggesting that the high endogenous Ile contributes to more conjugation of Ile with JA (MeJA-derived) into JA-Ile. In addition, JA-Ile biosynthesis was impaired in jar1-1 mutants on MeJA treatment. As in jar1-1 mutants, the mutated JAR1 enzyme lost the ability to catalyze JA and Ile into JA-Ile, suggesting that the exogenous MeJA-induced JA-Ile accumulation is dependent on JAR1. Consistently, JA-responsive genes, such as AOS, OPR3, MYB75, JAZ5, PDF1.2, and VSP1, were obviously induced in lib mutants on MeJA treatment (Figure 4D and Supplementary Figures 2B–E).
We also compared MeJA-induced anthocyanin accumulation among WT, lib, and jar1-1 seedlings. WT, lib, and jar1-1 seedlings were grown on MS medium supplemented with 0, 10, and 20 μM MeJA for 8 days and collected for the measurement of anthocyanin contents as well as expression levels of the anthocyanin biosynthetic gene GL3. These data showed that the anthocyanin content and expression level of GL3 in lib mutants were dramatically increased compared with those in WT leaves. In contrast, the accumulation of anthocyanin and the induction of GL3 were impaired in the jar1-1 mutant (Figures 4E,F and Supplementary Figure 2A). Taken together, our findings suggested that a higher Ile level in lib mutant improved its capacity to convert MeJA into JA-Ile and triggered JA signaling.
In summary, wound- and MeJA-triggered JA responses in lib mutant are significantly higher than WT, which demonstrated that the increase of Ile levels in lib leaves enhanced JA responses. These results provided a possibility that the increase of Ile level may improve plant resistance to other environmental stresses in addition to B. cinerea infection.
Increased Endogenous Ile Level Has No Effect on Aboveground Growth of Arabidopsis
The lib mutant exhibited similar JA-Ile contents with WT plants under resting conditions (Figure 4). Next, the effect of the increase of Ile contents in lib mutants on plant growth and development was investigated. First, we observed the development of the aboveground parts of lib plants at various stages. As shown in Supplementary Figures 3A,B, FW of the aboveground parts of lib mutants had no obvious difference compared with WT at the 18–, 23–, 28–, and 33-day-old stages. In addition, the morphology of flower, the dehiscence of anther, the main inflorescence, and seeds development did not exhibit an obvious difference between WT and lib plants (Supplementary Figure 3A). The number of mature siliques and the seed production also did not exhibit a significant difference between lib and WT plants (Supplementary Figures 3C,D). These results suggested that a proper increase of endogenous Ile level had no obvious effect on plant growth, development, and seed production.
Ile Application Broadly Improves Resistance to B. cinerea in Various Plants
Botrytis cinerea also infects various plants of economic interest, including lettuce, rose, and strawberries. Lettuce (Lactuca sativa, Compositae) is an annual and vitamin-rich leaf vegetable that can be damaged by B. cinerea and causes water-soaked, brownish-gray to brownish-orange symptoms (Shim et al., 2014). Rose (Rosa hybrida L., Rosaceae) is one of the most ornamental plants and is used as a food as well as in traditional medicines in China. Fresh cut roses are generally transported from farmers to customers. During long-distance transportation, gray mold disease caused by B. cinerea leads to the most serious postharvest loss of rose production (Cao et al., 2019). Strawberry (Fragaria × ananassa, Rosaceae) is a popular fruit worldwide and is also threatened by B. cinerea, which infects all aerial parts of the plant, especially flowers and fruits, and results in a greater than 50% yield loss (Petrasch et al., 2019). B. cinerea infection of these plants causes billions of dollars in loss (Qi et al., 2018; Cao et al., 2019). Therefore, we next explored whether the enhanced resistance caused by the Ile application is of universal significance in these plant species.
We applied 10 mM Ile to the leaves of lettuce, the flowers of white rose and red rose, and the fruits of strawberry for 2 days followed by inoculation with B. cinerea. Compared with control treatment (Mock), exogenous Ile application reduced the lesion size on lettuce leaves by 18%, on white rose by 11%, on red rose by 18%, and on strawberry by 14% (Figures 5A,B). These results demonstrated that the application of Ile moderately enhanced plant resistance to B. cinerea in horticultural plant species, suggesting a valuable approach to control B. cinerea in agriculture.
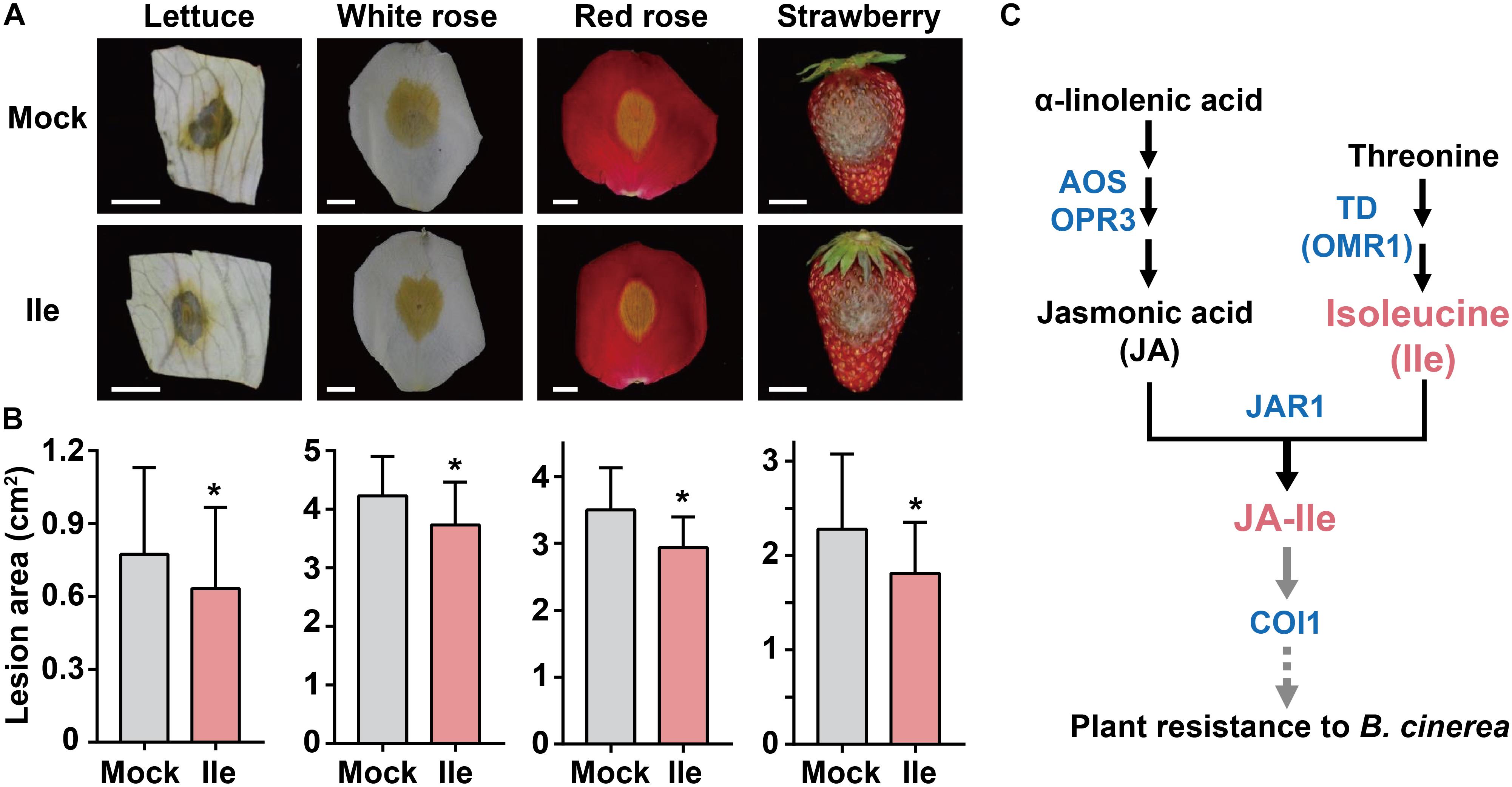
Figure 5. Application of Ile improves plant resistance to B. cinerea in horticultural plants. (A,B) Representative phenotype (A) and lesion area (B) of lettuce, roses, and strawberry after B. cinerea infection. 10 mM Ile and 0.05% Tween 20 solution (Mock) were respectively sprayed on lettuce leaves, flowers (white rose and red rose), and strawberries for 2 days before inoculation. Then, the detached indicated parts of plants were inoculated with B. cinerea spores suspension (1.0 × 106 spores/ml), moisturized in 1% agar dishes, and incubated in the dark for indicated days. Lesion sizes were measured and analyzed after 2 days for white rose (n = 57–58), 3 days for lettuce (n = 54–57) and red rose (n = 24–26), and 4 days for strawberry (n = 32). Scale: 1 cm. Data are means ± SD. Asterisks indicate significant differences compared with Mock treatment (Student’s t-test, *p < 0.05). (C) Regulation model of B. cinerea resistance mediated by Ile through JA signaling. JA is synthesized from α-linolenic acid through serial key enzymes, such as Allene oxide synthase (AOS) and OPDA reductase 3 (OPR3). Threonine deaminase (OMR1) catalyzes the conversion of threonine into 2-oxobutanoate, which is the first and the committed step in Ile biosynthesis. JA-Ile is then synthesized by conjugating jasmonic acid (JA) with Ile via JA-amido synthetase JAR1. On B. cinerea infection, a high Ile level will contribute to stronger induction of JA-Ile biosynthesis and then lead to enhanced JA responses depending on JAR1 and COI1. Black arrows represent the schematic diagram of JA-Ile biosynthesis. Gray arrows represent that JA-Ile is recognized by receptor COI1, subsequentially activating signal transduction to defense against B. cinerea infection.
Discussion
Botrytis cinerea causes gray mold disease, which leads to severe economic losses (Glazebrook, 2005). The risks of fungicide resistance and pesticide residues on fresh fruits and crops are the main problems for crop protection (AbuQamar et al., 2017). It is necessary to identify safer methods to protect crops from B. cinerea infection. This study identified Ile as an enhancer for plant resistance to the necrotrophic pathogen B. cinerea in Arabidopsis and other horticultural plants, such as lettuces, roses, and strawberries (Figures 1–3, 5). Many kinds of signals are involved in the defense against B. cinerea, including JAs, ethylene (ET), SA, abscisic acid (ABA), reactive oxygen species (ROS), and NO (Thomma et al., 1998; Audenaert et al., 2002; Adie et al., 2007; Abuqamar et al., 2008; L’Haridon et al., 2011; Beneloujaephajri et al., 2013; Song et al., 2014; AbuQamar et al., 2017). Among them, JA signaling is well known as one of the most important signals in the regulation of the defense against B. cinerea (Abuqamar et al., 2008; Song et al., 2013, 2014; AbuQamar et al., 2017). Moreover, Ile is involved in the biosynthesis of the bioactive JA molecule: JA-Ile (Staswick and Tiryaki, 2004; Kang et al., 2006; Yan et al., 2016). Using jar1-1 (defect in JA-Ile biosynthesis) and coi1-1 (defect in JA-Ile perception) mutants, we found that application of Ile enhanced plant resistance against B. cinerea in WT but not in jar1-1 and coi1-1 mutants (Figure 3). These findings demonstrate that Ile acts through JA signaling depending on COI1 and JAR1 to trigger plant defense against B. cinerea infection.
Previous study showed that 13C-labeled JA-Ile was greatly synthesized in the [13C6]Ile-treated leaves accompanied with wounding treatment, which was dramatically higher than that in [13C4]Thr-treated leaves (Kang et al., 2006), suggesting that Ile could directly conjugate with JA to form JA-Ile and the conjugation capacity is limited by substrate availability. Consistently, this study showed that the application of exogenous Ile greatly improved JA-Ile concentration and transcript level of defensive gene PDF1.2 triggered by B. cinerea infection (Figure 1). Furthermore, the increase of endogenous Ile level in lib mutant also elevated JA-Ile concentration and the transcript level of defensive gene PDF1.2 and JA biosynthetic gene OPR3 (Figure 2). Collectively, these findings indicate that increased Ile level could enhance plant resistance against B. cinerea infection via acting as a substrate to promote JA-Ile biosynthesis and activate JA signaling. In addition, Ile-triggered disease resistance and the acceleration of PDF1.2 gene expression level were not observed in jar1-1 and coi1-1 mutants on B. cinerea infection (Figure 3), further suggesting that Ile modulated plant resistance to B. cinerea depending on JA-Ile biosynthesis and perception through JAR1 and COI1 (Figure 5C). In addition JA is synthesized from α-linolenic acid under the catalysis of a series of enzymes in plastid and peroxisome, which is then exported to the cytoplasm, where it is conjugated with Ile to form bioactive JA-Ile under the catalysis of JAR1 (Huang et al., 2017). Hence, the application of exogenous Ile may enlarge the intracellular Ile pool and provide more available Ile to act as a substrate for JA-Ile biosynthesis under the catalysis of JAR1 in the cytoplasm. In summary, our findings provide a potential strategy for crop protection via increasing the supply of Ile, the precursor of JA-Ile, to improve plant resistance to B. cinerea.
It has been reported that wounded leaves showed enhanced resistance to B. cinerea infection via producing ROS, which depends on the ABA accumulation (L’Haridon et al., 2011; Beneloujaephajri et al., 2013). JAs could also promote ROS production (Suhita et al., 2004) and interact with ABA signaling pathway (Adie et al., 2007; Brossa et al., 2011; de Ollas et al., 2015; Aleman et al., 2016). Our findings showed that mechanical wounding treatment obviously induced JA-Ile accumulation and the expression of JA biosynthetic genes (such as OPR3) due to 1-fold increase in the Ile levels (Figures 4A,B). Thus, it would be interesting to further investigate whether the enhanced Ile level in lib leaves and Ile treatment affects ROS and ABA accumulation to regulate defense responses.
Nicotiana attenuata mutants with less Ile contents caused by the mildly silenced TD gene, a homolog of the OMR1 gene, are highly susceptible to the attack by Manduca sexta. Moreover, the resistance is restored via the application of JA-Ile or Ile (Kang et al., 2006). Together with our results that mechanical wounding enhanced JA-Ile biosynthesis and JA-responsive gene expression levels in lib leaves (Figures 4A,B), these findings indicate that increasing Ile level may have positive effects on plant defense against herbivore attack, but further studies need to be performed.
The application of MeJA on postharvest fruits could induce a plant defensive response and reduce the detrimental impact of pathogen infection (Yu et al., 2009; Jiang et al., 2015; Liu et al., 2016; Reyes-Diaz et al., 2016). In addition, preharvest and postharvest treatments of fruits with MeJA induced the accumulation of secondary metabolites, including anthocyanins and other antioxidant molecules, to prolong the storage period of fruits (Reyes-Diaz et al., 2016). This study reported that the increase of Ile level enhanced defensive responses to mechanical wounding or pathogen infection and MeJA-induced anthocyanin accumulation (Figures 1–4), suggesting the potential to increase secondary metabolites production and enhance plant resistance during fruit storage and transportation.
Chemical fungicide treatment is the most effective method to control B. cinerea, but repeated applications of fungicides increased the risk of fungicide resistance (Kretschmer et al., 2009; Rupp et al., 2016). Foliar fertilization is advantageous because it is applied at a relatively low concentration, with high efficiency in a uniform distribution, and plants respond to the application quickly (Kumar et al., 2016; Vishekaii et al., 2019). We revealed that Ile application enhanced plant resistance to B. cinerea in many species, including lettuce, rose, and strawberry (Figures 5A,B), which supports the potential to utilize water-soluble fertilizer containing Ile as a new efficient and safe strategy for B. cinerea control.
Materials and Methods
Plant Materials and Growth Conditions
Arabidopsis thaliana mutants lib (Yu et al., 2013), coi1-1 (Xie et al., 1998), and jar1-1 (Staswick et al., 2002) were described previously. In all experiments, the Columbia-0 (Col-0) was used as WT plants. Meanwhile, coi1-1 homozygous seedlings were screened out via supplying JA in MS medium (Xie et al., 1998). Arabidopsis seeds were sterilized with 20% bleach, plated on Murashige and Skoog medium (MS; Sigma-Aldrich, United States), cooled at 4°C for 2 days, and then grown in a growth room under a 16-h light/8-h dark (21–23°C) photoperiod.
Application of Exogenous Amino Acids
L-Ala (A7469, Sigma-Aldrich, United States), L-Leu (L8912, Sigma-Aldrich, United States), L-Ile (I2752, Sigma-Aldrich, United States), L-Met (M5308, Sigma-Aldrich, United States), L-Phe (V900489, VETEC, United States), L-Pro (V900338, VETEC, United States), L-Trp (V900470, VETEC, United States), L-Val (V900465, VETEC, United States), L-Asn (900458, VETEC, United States), L-Cys (V900400, VETEC, United States), Gly (V900144, VETEC, United States), L-Gln (V900419, VETEC, United States), L-Ser (V900406, VETEC, United States), L-Thr (V900466, VETEC, United States), L-Tyr (V900426, VETEC, United States), L-Asp (V900407, VETEC, United States), L-Glu (V900408, VETEC, United States), L-Arg (V900343, VETEC, United States), L-His (V900459, VETEC, United States), and L-Lys (V9 00409, VETEC, United States) were dissolved in 0.05% (v/v) Tween 20 solution to prepare amino acid solutions at a certain concentration. Plants grown in a room under a 10-h light/14-h dark photoperiod (21–23°C) were sprayed with amino acid solution or 0.05% Tween 20 solution (Mock), and then continued to grow for another 2 days. Subsequently, the pretreated plants were inoculated with B. cinerea spore suspension.
Botrytis cinerea Infection Assay
Botrytis cinerea infection assay was based on an established method (Song et al., 2014). For leaf lesion size measurement assay, the 7th–9th rosette leaves were detached from 5-week-old plants grown under a 10-h light/14-h dark photoperiod (21–23°C), placed on 1% agar plates, inoculated with 5 μl B. cinerea spores (1.0 × 106 spores/ml) suspended in Potato Dextrose Broth (PDB) medium, and then cultured in the dark with high humidity for 2–3 days. Subsequently, infected leaves were photographed with a digital camera, and then the lesion area was measured by using Digimizer software (v3.1.2.0, Belgium, Germany).
For JA-Ile quantification, plants were grown under a 10-h light/14-h dark photoperiod (21–23°C) for 3–4 weeks, inoculated with B. cinerea spores suspension (1.0 × 106 spores/ml) or PDB (negative control) for 24 or 48 h under high humidity conditions. Subsequently, the 7th–9th rosette leaves were harvested for JA-Ile content quantification using LC-MS/MS as previously described (Yan et al., 2016). The experiment was performed by three biological replicates.
Quantification of Amino Acids
For quantification of amino acids, 15-day-old plants were used. Seeds were germinated on MS medium for 5 days, and then transferred to vertical MS medium (1.2% agar) plates and grown for 10 days. Leaves were harvested for the measurement of amino acids. Here, 100 mg tissue from each sample was used. Samples were ground with liquid nitrogen. Each sample was extracted overnight at –20°C with 800 μl precooled extraction buffer [MeOH:H2O:HCOOH is 15:4:1 (v:v:v)]. Samples were centrifuged at the highest speed for 20 min at 4°C. Supernatants were diluted 50 times with water containing 1 ng/μl lab AA mix algae extract containing 13C, 15N-labeled amino acids (Sigma-Aldrich, catalog number: 487910). Twenty amino acids including abundant and low amino acids were detected and analyzed by UHPLC–MS/MS based on an established method (Schafer et al., 2016). For the chromatographic separation, UHPLC system equipped with a Zorbax Eclipse XDB-C18 column was used with (A) 0.1% (v/v) ACN, 0.05% (v/v) HCOOH in water and (B) MeOH as the mobile phase in gradient mode with described settings (Schafer et al., 2016). Analysis was performed on an EvoQ Triple quad-MS.
Wound Treatment
Rosette leaves of 4-week-old plants were crushed with an hemostat. Three leaves per plant were treated. Each leaf was wounded thrice across the main leaf vein, which created a wounded area of approximately 40–50%. Each sample consisted of approximately 30 leaves from 10 plants. One hour after wounding treatment, the wounded leaves and unwounded leaves (from untreated plants) were collected for the measurement of JA-Ile content and real-time PCR. JA-Ile content was measured by using LC-MS/MS as previously described (Yan et al., 2016). The experiment was performed by three biological replicates.
MeJA Treatment
For JA-Ile content measurement, Arabidopsis seedlings grown in MS medium for 14 days were treated with MeJA (0, 20, and 100 μM) for 1 h and harvested for the extraction and quantification of JA-Ile. JA-Ile content was measured by using LC-MS/MS as previously described (Yan et al., 2016). For the gene expression analysis as shown in Figure 4D, the 7-day-old seedlings grown in MS medium were soaked into solvent (Mock) and 50 μM MeJA for 1 h. The experiment was performed by three biological replicates.
Anthocyanin Measurement
For the anthocyanin accumulation assay, Arabidopsis seedlings were grown in MS medium supplied with 0, 10, and 20 μM MeJA for 8 days, and then harvested for anthocyanin measurement and gene expression analysis. The phenotype and anthocyanin content were measured based on a previously described method (Song et al., 2013). Briefly, 8-day-old seedlings were photographed on a stereo microscope (Nikon), weighted, and collected in 1.5 ml tubes. We added 1 ml anthocyanin extraction buffer [N-propanol:H2O:HCl is 18:81:1 (v:v:v)] to the tube, which was boiled at 100°C for 5 min to extract anthocyanin in the dark at room temperature overnight. Anthocyanin contents are presented as (A535-A650)/g FW. The experiment was performed by three biological replicates.
Real-Time PCR
Total RNAs were extracted from the indicated materials via using TransZol reagent (ET101-01, TransGen Biotech, China) and reverse transcribed by using TransScript One-Step gDNA Removal and cDNA Synthesis SuperMix (AH311-03, TransGen Biotech, China). Real-time PCR analysis was performed using Power SYBR Master Mix (A25778, ABI, United States) and the ABI7500 Real-Time PCR system. ACTIN8 was used as the internal control. The experiment was performed by three replicates. The primers used in this study are shown in Supplementary Table 2.
Statistical Analysis
Statistically significant differences were determined by using a two-tailed Student’s t-test.
Accession Numbers
AOS (AT5G42650), OPR3 (AT2G06050), JAZ1 (AT1G19180), JAZ5 (AT1G17380), JAZ10 (AT5G13220), GL3 (AT5G41315), MYB75 (AT1G56650), OMR1 (AT3G10050), PDF1.2 (AT5G44420), VSP1 (AT5G24780), and ACTIN8 (AT1G49240).
Data Availability Statement
The original contributions presented in the study are included in the article/Supplementary Material, further inquiries can be directed to the corresponding authors.
Author Contributions
JY, DX, and SL were designed the study. YL and SL were performed all the experiments and analyzed the data with assistance from RD, JW, and HL. YL, SL, JY, and DX wrote the manuscript. All authors contributed to the article and approved the submitted version.
Funding
This work was financially supported by grants from the National Natural Science Foundation of China (31630085 and 31421001). This work was supported by Fundamental Research Funds for Central Non-profit Scientific Institution (Y2020XK23), The Elite Young Scientists Program of CAAS, and The Agricultural Science and Technology Innovation Program.
Conflict of Interest
The authors declare that the research was conducted in the absence of any commercial or financial relationships that could be construed as a potential conflict of interest.
Publisher’s Note
All claims expressed in this article are solely those of the authors and do not necessarily represent those of their affiliated organizations, or those of the publisher, the editors and the reviewers. Any product that may be evaluated in this article, or claim that may be made by its manufacturer, is not guaranteed or endorsed by the publisher.
Acknowledgments
The authors would like to thank Dong Liu (School of Life Sciences) at the Tsinghua University for providing seeds of lib mutant.
Supplementary Material
The Supplementary Material for this article can be found online at: https://www.frontiersin.org/articles/10.3389/fpls.2021.628328/full#supplementary-material
Supplementary Figure 1 | Transcript level of jasmonate (JA)-responsive genes on B. cinerea infection.
Supplementary Figure 2 | Transcript level of JA-responsive genes on MeJA treatment.
Supplementary Figure 3 | lib mutant exhibits normal aboveground growth and development.
Supplementary Table 1 | Amount of free amino acids in the leaves of wild type, lib, and jar1-1 seedlings.
Supplementary Table 2 | Primers used for quantitative real-time PCR (qPCR).
References
Abuqamar, S., Chai, M. F., Luo, H., Song, F., and Mengiste, T. (2008). Tomato protein kinase 1b mediates signaling of plant responses to necrotrophic fungi and insect herbivory. Plant Cell 20, 1964–1983. doi: 10.1105/tpc.108.059477
AbuQamar, S., Moustafa, K., and Tran, L. S. P. (2017). Mechanisms and strategies of plant defense against Botrytis cinerea. Crit. Rev. Biotechnol. 37, 262–274. doi: 10.1080/07388551.2016.1271767
Adie, B. A., Perez-Perez, J., Perez-Perez, M. M., Godoy, M., Sanchez-Serrano, J. J., Schmelz, E. A., et al. (2007). ABA is an essential signal for plant resistance to pathogens affecting JA biosynthesis and the activation of defenses in Arabidopsis. Plant Cell 19, 1665–1681. doi: 10.1105/tpc.106.048041
Aghaei, K., Pirbalouti, A. G., Mousavi, A., Badi, H. N., and Mehnatkesh, A. (2019). Effects of foliar spraying of L-phenylalanine and application of bio-fertilizers on growth, yield, and essential oil of hyssop [Hyssopus officinalis l. subsp. Angustifolius (Bieb.)]. Biocatal. Agric. Biotechnol. 21:101318. doi: 10.1016/j.bcab.2019.101318
Albinsky, D., Sawada, Y., Kuwahara, A., Nagano, M., Hirai, A., Saito, K., et al. (2010). Widely targeted metabolomics and coexpression analysis as tools to identify genes involved in the side-chain elongation steps of aliphatic glucosinolate biosynthesis. Amino Acids 39, 1067–1075. doi: 10.1007/s00726-010-0681-5
Aleman, F., Yazaki, J., Lee, M., Takahashi, Y., Kim, A. Y., Li, Z., et al. (2016). An ABA-increased interaction of the PYL6 ABA receptor with MYC2 transcription factor: a putative link of ABA and JA signaling. Sci. Rep. 6:28941. doi: 10.1038/srep28941
Audenaert, K., De Meyer, G. B., and Hofte, M. M. (2002). Abscisic acid determines basal susceptibility of tomato to Botrytis cinerea and suppresses salicylic acid-dependent signaling mechanisms. Plant Physiol. 128, 491–501. doi: 10.1104/pp.010605
Beneloujaephajri, E., Costa, A., L’Haridon, F., Metraux, J. P., and Binda, M. (2013). Production of reactive oxygen species and wound-induced resistance in Arabidopsis thaliana against Botrytis cinerea are preceded and depend on a burst of calcium. BMC Plant Biol. 13:160. doi: 10.1186/1471-2229-13-160
Binder, S. (2010). Branched-chain amino acid metabolism in Arabidopsis thaliana. Arabidopsis Book 8:e0137. doi: 10.1199/tab.0137
Brossa, R., López-Carbonell, M., Jubany-Marí, T., and Alegre, L. (2011). Interplay between abscisic acid and jasmonic acid and its role in water-oxidative stress in wild-type, ABA-deficient, JA-deficient, and ascorbate-deficient Arabidopsis Plants. J. Plant Growth Regul. 30, 322–333. doi: 10.1007/s00344-011-9194-z
Cao, X., Yan, H., Liu, X., Li, D., Sui, M., Wu, J., et al. (2019). A detached petal disc assay and virus-induced gene silencing facilitate the study of Botrytis cinerea resistance in rose flowers. Hortic. Res. 6:136. doi: 10.1038/s41438-019-0219-2
Creelman, R. A., and Mullet, J. E. (1995). Jasmonic acid distribution and action in plants: regulation during development and response to biotic and abiotic stress. Proc. Natl. Acad. Sci. U.S.A. 92, 4114–4119. doi: 10.1146/annurev.arplant.48.1.355
de Ollas, C., Arbona, V., and Gomez-Cadenas, A. (2015). Jasmonoyl isoleucine accumulation is needed for abscisic acid build-up in roots of Arabidopsis under water stress conditions. Plant Cell Environ. 38, 2157–2170. doi: 10.1111/pce.12536
Dean, R., Van Kan, J. A., Pretorius, Z. A., Hammond-Kosack, K. E., Di Pietro, A., Spanu, P. D., et al. (2012). The top 10 fungal pathogens in molecular plant pathology. Mol. Plant Pathol. 13, 414–430. doi: 10.1111/j.1364-3703.2011.00783.x
Elzaki, M. E. A., Li, Z. F., Wang, J., Xu, L., Liu, N., Zeng, R. S., et al. (2020). Activiation of the nitric oxide cycle by citrulline and arginine restores susceptibility of resistant brown planthoppers to the insecticide imidacloprid. J. Hazard Mater. 396:122755. doi: 10.1016/j.jhazmat.2020.122755
Farmer, E. E., Almeras, E., and Krishnamurthy, V. (2003). Jasmonates and related oxylipins in plant responses to pathogenesis and herbivory. Curr. Opin. Plant Biol. 6, 372–378. doi: 10.1016/S1369-5266(03)00045-1
Fonseca, S., Chini, A., Hamberg, M., Adie, B., Porzel, A., Kramell, R., et al. (2009). (+)-7-iso-Jasmonoyl-L-isoleucine is the endogenous bioactive jasmonate. Nat. Chem. Biol. 5, 344–350. doi: 10.1038/nchembio.161
Glazebrook, J. (2005). Contrasting mechanisms of defense against biotrophic and necrotrophic pathogens. Annu. Rev. Phytopathol. 43, 205–227. doi: 10.1146/annurev.phyto.43.040204.135923
Halkier, B. A., and Gershenzon, J. (2006). Biology and biochemistry of glucosinolates. Annu. Rev. Plant Biol. 57, 303–333. doi: 10.1146/annurev.arplant.57.032905.105228
Huang, H., Liu, B., Liu, L., and Song, S. (2017). Jasmonate action in plant growth and development. J. Exp. Bot. 68, 1349–1359. doi: 10.1093/jxb/erw495
Jiang, L., Jin, P., Wang, L., Yu, X., Wang, H., and Zheng, Y. (2015). Methyl jasmonate primes defense responses against Botrytis cinerea and reduces disease development in harvested table grapes. Sci. Hortic. 192, 218–223. doi: 10.1016/j.scienta.2015.06.015
Kadotani, N., Akagi, A., Takatsuji, H., Miwa, T., and Igarashi, D. (2016). Exogenous proteinogenic amino acids induce systemic resistance in rice. BMC Plant Biol. 16:60. doi: 10.1186/s12870-016-0748-x
Kang, J. H., Wang, L., Giri, A., and Baldwin, I. T. (2006). Silencing threonine deaminase and JAR4 in Nicotiana attenuata impairs jasmonic acid-isoleucine-mediated defenses against Manduca sexta. Plant Cell 18, 3303–3320. doi: 10.1105/tpc.106.041103
Kretschmer, M., Leroch, M., Mosbach, A., Walker, A. S., Fillinger, S., Mernke, D., et al. (2009). Fungicide-driven evolution and molecular basis of multidrug resistance in field populations of the grey mould fungus Botrytis cinerea. PLoS Pathog. 5:e1000696. doi: 10.1371/journal.ppat.1000696
Kumar, J., Kumar, R., Rai, R., Mishra, D. S., Singh, S. K., and Nimbolkar, P. K. (2016). Influence of foliar application of mineral nutrients at different growth stages of guava. J. Plant Nutr. 40, 656–661. doi: 10.1080/01904167.2016.1246568
L’Haridon, F., Besson-Bard, A., Binda, M., Serrano, M., Abou-Mansour, E., Balet, F., et al. (2011). A permeable cuticle is associated with the release of reactive oxygen species and induction of innate immunity. PLoS Pathog. 7:e1002148. doi: 10.1371/journal.ppat.1002148
Liu, Y., Yang, X., Zhu, S., and Wang, Y. (2016). Postharvest application of MeJA and NO reduced chilling injury in cucumber (Cucumis sativus) through inhibition of H2O2 accumulation. Postharvest Biol. Technol. 119, 77–83. doi: 10.1016/j.postharvbio.2016.04.003
Petrasch, S., Knapp, S. J., Van Kan, J. A. L., and Blanco-Ulate, B. (2019). Grey mould of strawberry, a devastating disease caused by the ubiquitous necrotrophic fungal pathogen Botrytis cinerea. Mol. Plant Pathol. 20, 877–892. doi: 10.1111/mpp.12794
Qi, T., Song, S., Ren, Q., Wu, D., Huang, H., Chen, Y., et al. (2011). The Jasmonate-ZIM-domain proteins interact with the WD-Repeat/bHLH/MYB complexes to regulate Jasmonate-mediated anthocyanin accumulation and trichome initiation in Arabidopsis thaliana. Plant Cell 23, 1795–1814. doi: 10.1105/tpc.111.083261
Qi, W., Chen, X., Fang, P., Shi, S., Li, J., Liu, X., et al. (2018). Genomic and transcriptomic sequencing of Rosa hybrida provides microsatellite markers for breeding, flower trait improvement and taxonomy studies. BMC Plant Biol. 18:119. doi: 10.1186/s12870-018-1322-5
Rahmani Samani, M., Ghasemi Pirbalouti, A., Moattar, F., and Golparvar, A. R. (2019). L-Phenylalanine and bio-fertilizers interaction effects on growth, yield and chemical compositions and content of essential oil from the sage (Salvia officinalis L.) leaves. Ind. Crops Prod. 137, 1–8. doi: 10.1016/j.indcrop.2019.05.019
Reyes-Diaz, M., Lobos, T., Cardemil, L., Nunes-Nesi, A., Retamales, J., Jaakola, L., et al. (2016). Methyl jasmonate: an alternative for improving the quality and health properties of fresh fruits. Molecules 21:567. doi: 10.3390/molecules21060567
Rupp, S., Weber, R. W., Rieger, D., Detzel, P., and Hahn, M. (2016). Spread of Botrytis cinerea strains with multiple fungicide resistance in German horticulture. Front. Microbiol. 7:2075. doi: 10.3389/fmicb.2016.02075
Schafer, M., Brutting, C., Baldwin, I. T., and Kallenbach, M. (2016). High-throughput quantification of more than 100 primary- and secondary-metabolites, and phytohormones by a single solid-phase extraction based sample preparation with analysis by UHPLC-HESI-MS/MS. Plant Methods 12:30. doi: 10.1186/s13007-016-0130-x
Seo, J. S., Joo, J., Kim, M. J., Kim, Y. K., Nahm, B. H., Song, S. I., et al. (2011). OsbHLH148, a basic helix-loop-helix protein, interacts with OsJAZ proteins in a jasmonate signaling pathway leading to drought tolerance in rice. Plant J. 65, 907–921. doi: 10.1111/j.1365-313X.2010.04477.x
Shim, C. K., Kim, M. J., Kim, Y. K., and Jee, H. J. (2014). Evaluation of lettuce germplasm resistance to gray mold disease for organic cultivations. Plant Pathol. J. 30, 90–95. doi: 10.5423/PPJ.NT.07.2013.0064
Song, S., Huang, H., Gao, H., Wang, J., Wu, D., Liu, X., et al. (2014). Interaction between MYC2 and ETHYLENE INSENSITIVE3 modulates antagonism between jasmonate and ethylene signaling in Arabidopsis. Plant Cell 26, 263–279. doi: 10.1105/tpc.113.120394
Song, S., Qi, T., Fan, M., Zhang, X., Gao, H., Huang, H., et al. (2013). The bHLH subgroup IIId factors negatively regulate jasmonate-mediated plant defense and development. PLoS Genet. 9:e1003653. doi: 10.1371/journal.pgen.1003653
Staswick, P. E., and Tiryaki, I. (2004). The oxylipin signal jasmonic acid is activated by an enzyme that conjugates it to isoleucine in Arabidopsis. Plant Cell 16, 2117–2127. doi: 10.1105/tpc.104.023549
Staswick, P. E., Tiryaki, I., and Rowe, M. L. (2002). Jasmonate response locus JAR1 and several related Arabidopsis genes encode enzymes of the firefly luciferase superfamily that show activity on jasmonic, salicylic, and indole-3-acetic acids in an assay for adenylation. Plant Cell 14, 1405–1415. doi: 10.1105/tpc.000885
Suhita, D., Raghavendra, A. S., Kwak, J. M., and Vavasseur, A. (2004). Cytoplasmic alkalization precedes reactive oxygen species production during methyl jasmonate- and abscisic acid-induced stomatal closure. Plant Physiol. 134, 1536–1545. doi: 10.1104/pp.103.032250
Suza, W. P., and Staswick, P. E. (2008). The role of JAR1 in Jasmonoyl-L-isoleucine production during Arabidopsis wound response. Planta 227, 1221–1232. doi: 10.1007/s00425-008-0694-4
Tamogami, S., Rakwal, R., and Agrawal, G. K. (2008). Interplant communication: airborne methyl jasmonate is essentially converted into JA and JA-Ile activating jasmonate signaling pathway and VOCs emission. Biochem. Biophys. Res. Commun. 376, 723–727. doi: 10.1016/j.bbrc.2008.09.069
Thaler, J. S., Owen, B., and Higgins, V. J. (2004). The role of the jasmonate response in plant susceptibility to diverse pathogens with a range of lifestyles. Plant Physiol. 135, 530–538. doi: 10.1104/pp.104.041566
Thines, B., Katsir, L., Melotto, M., Niu, Y., Mandaokar, A., Liu, G., et al. (2007). JAZ repressor proteins are targets of the SCFCOI1 complex during jasmonate signalling. Nature 448, 661–665. doi: 10.1038/nature05960
Thomma, B. P., Eggermont, K., Penninckx, I. A., Mauch-Mani, B., Vogelsang, R., Cammue, B. P., et al. (1998). Separate jasmonate-dependent and salicylate-dependent defense-response pathways in Arabidopsis are essential for resistance to distinct microbial pathogens. Proc. Natl. Acad. Sci. U.S.A. 95, 15107–15111. doi: 10.1073/pnas.95.25.15107
Vishekaii, Z. R., Soleimani, A., Fallahi, E., Ghasemnezhad, M., and Hasani, A. (2019). The impact of foliar application of boron nano-chelated fertilizer and boric acid on fruit yield, oil content, and quality attributes in olive (Olea europaea L.). Sci. Hortic. 257:108689. doi: 10.1016/j.scienta.2019.108689
Wang, J., Liu, Z., Wang, Y., Cheng, W., and Mou, H. (2014). Production of a water-soluble fertilizer containing amino acids by solid-state fermentation of soybean meal and evaluation of its efficacy on the rapeseed growth. J. Biotechnol. 187, 34–42. doi: 10.1016/j.jbiotec.2014.07.015
Wasternack, C., and Hause, B. (2013). Jasmonates: biosynthesis, perception, signal transduction and action in plant stress response, growth and development. An update to the 2007 review in Annals of Botany. Ann. Bot. 111, 1021–1058. doi: 10.1093/aob/mct067
Wathugala, D. L., Hemsley, P. A., Moffat, C. S., Cremelie, P., Knight, M. R., and Knight, H. (2012). The Mediator subunit SFR6/MED16 controls defence gene expression mediated by salicylic acid and jasmonate responsive pathways. New Phytol. 195, 217–230. doi: 10.1111/j.1469-8137.2012.04138.x
Williamson, B., Tudzynski, B., Tudzynski, P., and Van Kan, J. A. (2007). Botrytis cinerea: the cause of grey mould disease. Mol. Plant Pathol. 8, 561–580. doi: 10.1111/j.1364-3703.2007.00417.x
Windram, O., Madhou, P., McHattie, S., Hill, C., Hickman, R., Cooke, E., et al. (2012). Arabidopsis defense against Botrytis cinerea: chronology and regulation deciphered by high-resolution temporal transcriptomic analysis. Plant Cell 24, 3530–3557. doi: 10.1105/tpc.112.102046
Xie, D. X., Feys, B. F., James, S., Nieto-Rostro, M., and Turner, J. G. (1998). COI1: an Arabidopsis gene required for jasmonate-regulated defense and fertility. Science 280, 1091–1094. doi: 10.1126/science.280.5366.1091
Xu, L., Liu, F., Lechner, E., Genschik, P., Crosby, W. L., Ma, H., et al. (2002). The SCFCOI1 ubiquitin-ligase complexes are required for jasmonate response in Arabidopsis. Plant Cell 14, 1919–1935. doi: 10.1105/tpc.003368
Yan, C., Fan, M., Yang, M., Zhao, J., Zhang, W., Su, Y., et al. (2018). Injury activates Ca2+/Calmodulin-dependent phosphorylation of JAV1-JAZ8-WRKY51 complex for jasmonate biosynthesis. Mol. Cell 70, 136–149. doi: 10.1016/j.molcel.2018.03.013
Yan, J., Li, H., Li, S., Yao, R., Deng, H., Xie, Q., et al. (2013). The Arabidopsis F-box protein CORONATINE INSENSITIVE1 is stabilized by SCFCOI1 and degraded via the 26S proteasome pathway. Plant Cell 25, 486–498. doi: 10.1105/tpc.112.105486
Yan, J., Li, S., Gu, M., Yao, R., Li, Y., Chen, J., et al. (2016). Endogenous bioactive jasmonate is composed of a set of (+)-7-iso-JA-amino acid conjugates. Plant Physiol. 172, 2154–2164. doi: 10.1104/pp.16.00906
Yan, J., Zhang, C., Gu, M., Bai, Z., Zhang, W., Qi, T., et al. (2009). The Arabidopsis CORONATINE INSENSITIVE1 protein is a jasmonate receptor. Plant Cell 21, 2220–2236. doi: 10.1105/tpc.109.065730
Yu, H., Zhang, F., Wang, G., Liu, Y., and Liu, D. (2013). Partial deficiency of isoleucine impairs root development and alters transcript levels of the genes involved in branched-chain amino acid and glucosinolate metabolism in Arabidopsis. J. Exp. Bot. 64, 599–612. doi: 10.1093/jxb/ers352
Keywords: isoleucine, Botrytis cinerea, JA-Ile, COI1, JAR1
Citation: Li Y, Li S, Du R, Wang J, Li H, Xie D and Yan J (2021) Isoleucine Enhances Plant Resistance Against Botrytis cinerea via Jasmonate Signaling Pathway. Front. Plant Sci. 12:628328. doi: 10.3389/fpls.2021.628328
Received: 11 November 2020; Accepted: 23 July 2021;
Published: 19 August 2021.
Edited by:
Antonieta De Cal, Instituto Nacional de Investigación y Tecnología Agroalimentaria (INIA), SpainReviewed by:
Mario Serrano, National Autonomous University of Mexico, MexicoThierry Heitz, UPR 2357 Institut de Biologie Moléculaire des Plantes (IBMP), France
Copyright © 2021 Li, Li, Du, Wang, Li, Xie and Yan. This is an open-access article distributed under the terms of the Creative Commons Attribution License (CC BY). The use, distribution or reproduction in other forums is permitted, provided the original author(s) and the copyright owner(s) are credited and that the original publication in this journal is cited, in accordance with accepted academic practice. No use, distribution or reproduction is permitted which does not comply with these terms.
*Correspondence: Daoxin Xie, ZGFveGlubGFiQHRzaW5naHVhLmVkdS5jbg==; Jianbin Yan, amlhbmJpbmxhYkBjYWFzLmNu; Suhua Li, bGlzdWh1YTAxQGNhYXMuY24=
†These authors have contributed equally to this work