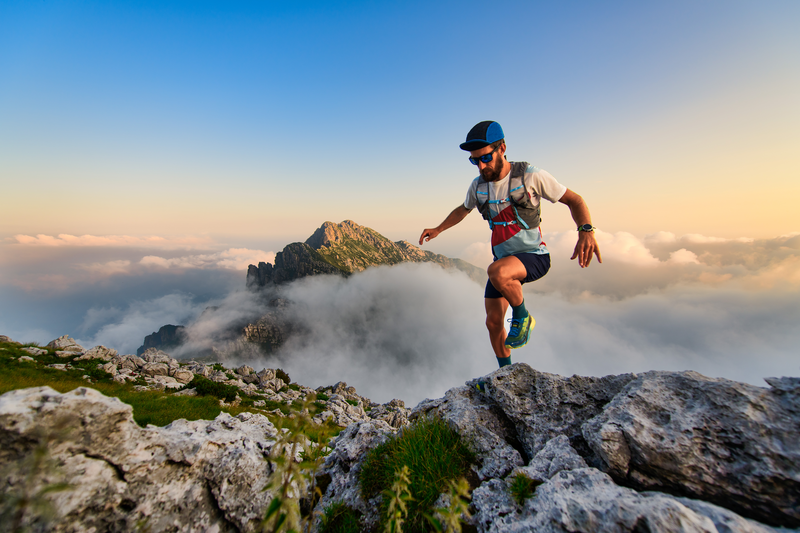
94% of researchers rate our articles as excellent or good
Learn more about the work of our research integrity team to safeguard the quality of each article we publish.
Find out more
ORIGINAL RESEARCH article
Front. Plant Sci. , 16 February 2021
Sec. Plant Abiotic Stress
Volume 12 - 2021 | https://doi.org/10.3389/fpls.2021.620544
This article is part of the Research Topic Biological and Genetic Basis of Agronomical and Seed Quality Traits in Legumes View all 30 articles
Plant C2 domain proteins play essential biological functions in numerous plants. In this study, 180 soybean C2 domain genes were identified by screening. Phylogenetic relationship analysis revealed that C2 domain genes fell into three distinct groups with diverged gene structure and conserved functional domain. Chromosomal location analysis indicated that C2 domain genes mapped to 20 chromosomes. The transcript profiles based on RNA-seq data showed that GmC2-58, GmC2-88, and GmC2-148 had higher levels of expression under salt, drought, and abscisic acid (ABA) treatments. GmC2-148, encoding a cell membrane-localized protein, had the highest level of response to various treatments according to real-time quantitative polymerase chain reaction (RT-qPCR) analysis. Under salt and drought stresses, the soybean plants with GmC2-148 transgenic hairy roots showed delayed leaf rolling, a higher content of proline (Pro), and lower contents of H2O2, O2– and malondialdehyde (MDA) compared to those of the empty vector (EV) plants. The results of transgenic Arabidopsis in salt and drought treatments were consistent with those in soybean treatments. In addition, the soybean plants with GmC2-148 transgenic hairy roots increased transcript levels of several abiotic stress-related marker genes, including COR47, NCDE3, NAC11, WRKY13, DREB2A, MYB84, bZIP44, and KIN1 which resulted in enhanced abiotic stress tolerance in soybean. These results indicate that C2 domain genes are involved in response to salt and drought stresses, and this study provides a genome-wide analysis of the C2 domain family in soybean.
Environmental stresses caused by such factors as salt and drought significantly affect plant growth and metabolic processes, threaten the survival of plants, and often lead to reduction in yield (Bohnert et al., 1995). In response to stress, plants have developed suitable transduction pathways and a variety of tools to resist cell damage caused by stress (Chenu et al., 2008).
Many gene families related to abiotic stress have been identified, including regulatory factors and regulatory proteins, such as NAC, AP2/ERF, MYB, MYC transcription factors (Zhao et al., 2017), and C2 domain proteins, that are involved in plant signaling pathways (Liu et al., 2019). Many intracellular and extracellular proteins are partially or entirely composed of protein modules. The C2 domain is a typical intracellular protein module (Chapman et al., 1996). Being a Ca2+-dependent membrane targeting domain, this domain shows Ca2+-dependent phospholipid binding activity and a higher sensitivity to salt and drought stresses (Banci et al., 2002). The Ca2+ binding sites of most C2 domain proteins are associated with the presence of five aspartic acid residues, which are highly conserved in plants and animals (Lopez-Nicolas et al., 2006).
It is common knowledge that Ca2+ is widespread across in plants as a subordinate messenger and is involved in plant environmental response, growth, development and hormonal signaling (Cho et al., 2016). In recent years, most research have indicated that Ca2+ signaling takes part in plant answers to abioticstresses, including salt and drought stresses (Pak Dek et al., 2020). When Ca2+ binding proteins are bound to Ca2+, these proteins help transmit a signal to a downstream signaling pathway to induce expression of stress response genes. Four main Ca2+-binding proteins have been identified in the reaction of plants to modification in the exterior surroundings (Molz et al., 1996).
In plants, C2 domain genes play important roles, but they vary from species to species (Corbalan-Garcia et al., 2003). It is reported that Cmpp16-1, a C2 domain gene identified in pumpkin, was associated with RNA delivery (Ramirez-Ortega et al., 2014). Another C2 domain gene, OsERG1, was identified from rice suspension-cultured cells treated with fungal elicitors and reportedly respond to abiotic stresses and membrane translocation in a Ca2+-dependent manner (Kang et al., 2013). In recent years, the proteins of C2 domain family have been recognized as innovative monitoring proteins involved in a variety of abiotic stresses in plants. Three C2 domain proteins, containing the barley HvC2d1 (Ouelhadj et al., 2006), mung bean VrPLC3 (Wang, 2002; Vasseur et al., 2014), and Arabidopsis SYT1 proteins (Yamazaki et al., 2008) were all involved in stresses reaction through a Ca2+-dependent signaling-pathway. In pepper, a gene encoding a C2 domain protein was considerably upregulated under abiotic stresses, containing salt stress (Kim et al., 2008). In transgenic Arabidopsis, overexpression of OsSMCP1 augmented tolerance to stresses compared to the control group (Yokotani et al., 2009). Together these are some of the studies in the literature that demonstrate the significant roles of C2 domain genes in numerous biological processes, so it is of great importance to study the functions of the C2 domain genes in plants (Nalefski and Falke, 1996). Although the C2 domain family has been analyzed in several plant species, no systematic investigations have been conducted in soybean (Glycine max).
Soybean is both a major global cereal crop and an essential tool in plant research. Particularly for countries such as China, soybean is a vital economic crop for cooking oil, soy products, soy sauce and protein extracts (Cerezini et al., 2020). However, in recent years shortages of water resources have exacerbated drought stress in plants which negatively affect crop cultivation and production in China (Kim et al., 2020). Thus, researchers have been employing molecular biology and genetic approaches to improve soybean yield and quality by enhancing its stress tolerance. Recently, with the expansion of biotechnology, it is effective to predict the gene function of a large gene family through genome-wide identification and analysis (Agarwal et al., 2019). Thus, we endeavored to systematically study the C2 domain family in soybean, given our current knowledge of its importance in plant stress tolerance and the availability of genomic information.
In this study, we identified 180 soybean C2 domain genes and analyzed their phylogenetic relationship, chromosomal distributions, motif compositions, cis-elements, and expression patterns in various treatments. To establish the role of the soybean C2 domain genes in response to stresses, we evaluated their response to various treatments, which include salt and drought stresses, as well as ABA treatment. Using real-time quantitative polymerase chain reaction (RT-qPCR) analysis, we revealed a gene, GmC2-148, with the strongest responses to these treatments. We simultaneously verified the resistance of GmC2-148 to abiotic stresses in soybean and Arabidopsis demonstrated that GmC2-148 possibly will improve acceptance to salt and drought stresses. The results presented in this report will expand our knowledge of the C2 domain family in soybean.
Protein sequences of the entire C2 domain in soybean and Arabidopsis were attained from Phytozome1. We downloaded the Hidden Markov model (HMM) profiles of the C2 domain (PF00168) to search C2 domain genes in the soybean dataset using the software HMMER 3.0 (HMMER2) with a threshold of e-value < e–5. The CDD3, Pfam4, and SMART5 databases were used to determine the conservative C2 domain genes. Calculation biophysical properties of C2 domain genes using the online server tool ExPASy6.
Multiple sequence alignment of the C2 domain genes from soybean and Arabidopsis was performed by ClustalW27. Using pairwise distances and the neighbor-joining (NJ) algorithm, we constructed a phylogenetic tree by MEGA7.0 with 1,000 bootstrap. All C2 domain genes were separately mapped on the 20 soybean chromosomes based on information from the Phytozome database. Multiple collinear scanning toolkits (MCScanX8) with the default parameters were used to analyze the gene replication events.
The gene structure map is generated from the online website9. Motif information was obtained by MEME10. Then we imported the images generated by the online websites into TBtools11 to generate a cluster composition (Chen et al., 2020). As for the analysis of tissue expression pattern, we uploaded the information of C2 domain genes to the online website soybase12 and drew the heat map of gene expression patterns in different tissues by TBtools. Another online tool, PLACE13, was applied to predict the soybean C2 domain gene promoters. We selected the 1,500 bp upstream from the ATG start codon of GmC2-58, GmC2-88, and GmC2-148 to analyze their cis-acting elements. The genes were submitted to PLACE to identify the cis-acting elements that are related to abiotic stresses.
We cultivated 10 “Williams 82” soybean seeds in a 12 cm × 12 cm pot filled with vermiculite and soil at a 1:3 ratio and established a total of 18 pots for all treatments. Fresh soybean leaves of 10-day-old plants were obtained to extract RNA after individuals were exposed to the various treatments. For the drought treatment, soybean seedlings were dried on filter paper before obtaining 0.1 g of leaf tissue at different time periods 0, 1, 2, 4, 8, 12, and 24 h. We pulled the soybean seedlings out of the soil and put them in a cup for another treatment. The salt, ABA and BR treatments of soybean seedlings contained 200 mM NaCl, 100 μM ABA and 100 μM BR, respectively. Additionally, we established a high temperature treatment by putting soybean seedlings in an oven set at 45°C and a low temperature treatment by putting soybean seedlings in a refrigerator at 4°C. Sampling of 0.1 g of leaf tissue occurred at the same time period as sampling occurred in the drought treatment.
The RNA of soybean roots treated with salt and dry pot culture was extracted and reverse transcribed to analyze the expression level of salt and drought related marker genes. We used the RNA prep plant kit produced by (TianGen, China) and the cDNA synthesized kit (Transgen, China) to obtain cDNA for follow-up experiments. The concentration of cDNA was diluted to about 200 ng/ul.
The expression levels of three C2 domain genes, GmC2-58, GmC2-88, and GmC2-148 exposed to the various treatments were analyzed in soybean. RT-qPCR was accompanied with an ABI7500 real-time PCR system (ABI, United States) using TransStart Top Green qPCR SuperMix (Transgen, China). The data of each gene expression is calculated by using the method of 2−ΔΔCt method. Expression of GmC2-58, GmC2-88, and GmC2-148 were measured relative to the control (0 h). Using actin gene as internal control, the template cDNA was normalized. The total volume containing 2 × Taq PCR Master Mix was 20 μl (TianGen, China). The primer sequences used in this paper are all shown in the Supplementary Figure 2.
According to Conesa and Gotz (2008), we used the on line website Blast2GO14 to annotate and analyze the C2 domain family.
We constructed an expression vector with green fluorescent protein (GFP) marker for subsequent subcellular localization analysis as described earlier (Cui et al., 2019). The coding sequence length (CDS) of GmC2-148 was specifically amplified by PCR, then the correctly sequenced plasmid was amplified with the splice primer with GFP, and transformed in Escherichia coli to extract the fusion plasmid of GmC2-148-GFP. The recombinant plasmid of GmC2-148-GFP was transformed into Arabidopsis protoplasts using PEG4000-mediated method (Momota et al., 2020). After incubating in the dark at 23°C for 18–24 h, the protoplasts were detected by laser confocal microscope (Carl Zeiss LSM 700, Germany).
To generate the soybean plants with GmC2-148 transgenic hairy roots, the GmC2-148-3301 recombinant vector and empty vector (EV) were introduced into Agrobacterium competent cells of strain K599 and then transformed into soybean hairy roots by A. rhizogenes-mediated transformation as described earlier (Kereszt et al., 2007). We covered each infected soybean plant with a transparent plastic cup that was 25cm high and 8cm wide (at the bottom) to maintain the temperature at 25°C and air humidity at 70%, and the gaps in cups were filled with soil. After 2 weeks, hairy roots were observed at the infected site on the outside of the plastic cup, and then the main root 1 cm below the infected site was removed. Four seedlings were transplanted into a pot, totaling 24 pots containing mixed soil (1:1 vermiculite/humus) and then cultured at 25°C and a 16 h light/8 h dark photoperiod in a greenhouse.
We ligated the CDS sequence of GmC2-148 to vector pCAMBIA1302 to construct the transgenic vector of GmC2-148 in Arabidopsis. After being transformed into E. coli and sequenced rightly, the plasmid was extracted and transformed by Agrobacterium competent cell GV3101. The OD600 value of Arabidopsis infection solution was adjusted to 0.8, then the inflorescence of Arabidopsis was soaked in the infection solution for 3 min, repeated twice, and the second infection was carried out 1 week after infection. Two weeks after infection, DNA was extracted from the leaves of Arabidopsis for positive detection, and RNA was extracted to detect the expression level. After harvesting the seeds of Arabidopsis positive lines with high expression level, the seedlings were screened on the medium of hygromycin, then planted and cultured to T2 generation for experiment.
For the salt stress assay of soybean, EV and the soybean plants with GmC2-148 transgenic hairy roots were treated with 250 mM NaCl solution for 7 days. For the drought stress assay, EV and the soybean plants with GmC2-148 transgenic hairy roots were grown while watering was withheld for 7 days. After the drought treatment, we re-watered soybean plants for 3 days and then observed their growth. A control was also established for both treatments where plants were watered with 250 ml of water per pot three times a week.
As for experiment on salt treatment of Arabidopsis, wild type (WT) and GmC2-148 transgenic plants were treated with 250 mM NaCl solution for 7 days. During the drought treatment, Arabidopsis were grown while watering was withheld for 10 days. In the Arabidopsis root length experiment, we used 6 and 9% (w/v) polyethylene glycol (PEG) to simulate drought, and treated Arabidopsis with 90 and 110 mM NaCl, 10 and 12 μM ABA to observe the root length phenotype.
During this study, we measured malondialdehyde (MDA), Pro, H2O2, and O2– contents from soybean leaves after the salt and drought stress treatments. For each physiological index, we sampled leaves from three individuals. Contents of MDA, Pro, H2O2, and O2– were measured 0.1 g from leaf samples follow the instructions of the kit (Solarbio, China). Determination of absorbance value by Varioskan LUX multimode enzyme labeling instrument (Thermo Fisher Scientific, United States).
To determine the content of chlorophyll, took 0.1 g from soybean leaves and cut them up, placed in a centrifuge tube, and filled up to 10 ml with 80% acetone solution before placing the tube in the dark for 24 h. The chlorophyll extract’s reflectance was then measured at wavelengths of 665 and 649 nm to determine the chlorophyll content. An 80% acetone solution was used as the control.
To prepare materials for measurement of electrical conductivity, plant leaves of the same size were cleaned with paper and any water remaining on their surfaces was absorbed with filter paper. Then we cut the leaves into pieces of the same size, mixed them evenly and took 0.1 g of the cut pieces to soak them in 10 ml water for 12 h. Next, we measured the conductivity R1 of the solution of steeped leaf sample with a conductivity meter then putting samples in boiling water for 30 min and then cooling them to 25°C. We then measured R2 to calculate relative conductivity of samples based on the following equation: R1/R2 × 100%.
Individual intact leaf samples from salt and drought stressed soybean and a control soybean group of plants were used in two staining procedures to observe the degree of damage to the leaves. For Nitrogen Blue Tetrazole (NBT) staining, the cut leaves were soaked in NBT solution for 14 h, and then immersed in 75% ethanol for decoloration until the sample became transparent. For trypan blue staining, we first prepared 10 ml of 0.4% trypan blue solution that was filtered and sterilized before adding 90 ml 1 × PBS solution. The leaves were placed in 100 ml of the mixed solution and kept at 37°C in the dark for 24 h. Dyed leaves were then added to a 1:3 ethanol:glacial acetic acid solution to decolorize leaves at 37°C in the dark for 24 h until the samples became transparent.
Relative water content was determined using the following equation: relative water content (%) = (fresh weight − dry weight)/(saturated fresh weight − dry weight) × 100%. Soybean leaves were cut into pieces and then weighed to obtain fresh weight. They were subsequently soaked for a few hours until the leaves were saturated with water. Then their leaf surfaces were dried with absorbent paper and the leaves were weighed immediately to obtain their saturated fresh weight. The same leaves were then oven-dried at about 70–80°C for 2 days until they reached a constant weight. Dried samples were placed them on a table at 25°C for 2 h before measuring the dry weight (Li et al., 2020).
We found 196 potential C2 domain genes in the soybean genome (G. max Wm82.a2.v1). After screening from SMART and Pfam databases, we identified 180 C2 domain family members in soybean. The statistical results exhibited that the amino acid sequence length of the 180 C2 domain genes varied from the shortest of 128 amino acids to the longest of 1,181 amino acids, and the length of CDS varied from 384 to 3,543. The isoelectric point (pI) varied from 4.8 to 5.32, and the molecular weights (MW) ranged from 27.9 to 292.5 kDa (Supplementary Table 1). We constructed an unrooted NJ phylogenetic tree to analyze the evolutionary relationships in the C2 domain family. The tree, constructed using the 180 soybean C2 domain genes and included 102 Arabidopsis C2 domain genes, classified the proteins into three major groups (I–III) and six subgroups (Ia, Ib, IIa, IIb, IIIa, and IIIb) according to their genetic relationships (Figure 1).
Figure 1. Phylogenetic relationship of C2 domain genes between soybean and Arabidopsis. The phylogenetic tree was generated by comparison of the C2 domain amino acid sequences in MEGA 7. The neighbor-joining method was used and the bootstrap value was set to 1,000. All the genes were divided into three groups, I, II, and III.
The chromosome information of C2 domain genes was extracted and the chromosomal positions indicated that the 180 C2 domain genes were unequally distributed in 20 soybean chromosomes (Supplementary Figure 1). Each chromosome contained ≥1 C2 domain gene. Chromosome 7 contained the highest number of C2 domain genes, while chromosomes11, 16, and 20 contained the lowest numbers of C2 domain genes. In order to disclose the extension mechanism of the C2 domain family, all soybean replication data files are filtered by MCScanX (Figure 2). Fragmented duplicated genes were the most frequently found on chromosome 8, followed by chromosome 7, whereas there were no pairs of duplicated fragmented genes on chromosome 16.
Figure 2. Orthologous relationships of 180 C2 domain genes in soybean. Numbers along each chromosome box indicate sequence lengths in mega bases. Lines between two chromosomes represent the syntonic relationships.
To further understand the evolutionary relationship of the 180 C2 domain genes, we identified structural features and motifs of the C2 domain genes from soybean, including the locations of exons and introns and conserved motifs (Figure 3). According to our statistics, the number of introns in C2 domain family genes is varied from 1 to 18 and the number of introns considerably varied among the C2 domain genes. Most C2 domain genes in group Ia and IIa contain 8 introns, GmC2-70 in group Ia has the largest number of introns, containing 18 introns, GmC2-102 in group IIa has the largest number of introns, containing 17 introns. Most C2 domain genes in group Ib contain 15 introns, while most C2 domain genes in group IIb contain 9 introns. Most of the genes in group IIIa and IIIb have no introns. Also, the number of motifs contained in different genes varied greatly. Among the C2 domain genes, 44 had 1 motif, 34 had 2 motifs and 32 had 3 motifs, respectively accounting for 24.5, 18.8, and 17.7% of the total number of C2 domain genes. As for motif, group Ia containing motif 2 or 7, C2 domain genes in group Ib containing motif 7, most C2 domain genes in group IIa containing motif 7 and 9, group IIb containing motif 6 and 7, group IIIa and some C2 domain genes in group IIIb contained one motif 7, and the rest of the group IIIb genes contained other eight different motifs. Ten motifs are shown in the table (Table 1).
Figure 3. Motif and gene structure analyses of 180 C2 domain genes. The gene sequences of 180 C2 domain genes were analyzed with online tools. The maximum number for motif is 10. There are great differences in the number of motifs and intron-exons in different genes.
The date of C2 domain genes differentially expressed under NaCl, drought and ABA treatments are from the RNA-seq results of our laboratory, which have been used in the paper by Shi et al. This RNA-Seq date comes from 10-day-old soybean seedlings that were subjected to NaCl, drought and ABA treatments (Shi et al., 2018). To study the soybean C2 domain genes in response to these treatments, we investigated the transcript profiles of them. Because under abiotic stress, compared with the other two groups, group I had the largest number of gene responses, implying that C2 domain genes in group I are more likely involved in soybean reaction to abiotic stress. Hence, we used a heat map to visually analyze the expression patterns of these potential stress-related genes in group I. The results presented that most of the soybean C2 domain genes in group I showed varying degrees of response to stress. What is more, we found that GmC2-58, GmC2-88, and GmC2-148 all exhibited greater levels of expression in response to the stress treatments, so then we selected these three genes for further investigation (Figure 4).
Figure 4. RNA-seq analyses of the C2 domain genes from group I under various treatments. (A) Salt treatment transcript profiles, (B) Drought treatment transcript profiles, (C) ABA treatment transcript profiles. The heat map was produced by TBtools software, and different colors on the scale bar represent different transcript levels.
To comprehend the tissue-specific expression patterns of soybean C2 domain genes, their expression profiles in different tissues were obtained from the soybean database. We explored profiles of the C2 domain genes from group I in various tissues because they showed the highest responses in stressful abiotic conditions, and the hierarchical grouping of tissue-specific expression patterns could reflect the expression of these genes under normal conditions (Figure 5). Expression data displayed most soybean C2 domain genes showed diverse transcript levels in various tissues. In particular, GmC2-58, GmC2-88, and GmC2-148 all produced higher expression levels in roots, the important vegetative organ that functions to absorb water and inorganic salts from the soil, suggesting that the selected genes may have an important role in plant growth. The relevant data expressed by the organization are shown in the Supplementary Table 6.
Figure 5. Tissue-specific heat map of expression profiles (in log10-based FPKM) of C2 domain genes in group I. The expression levels of different transcripts are represented by the color bar; red indicates high expression levels, while blue indicates low expression levels.
In order to further elucidate the regulatory mechanism of C2 domain genes, we isolated the promoter regions of three selected genes GmC2-58, GmC2-88, and GmC2-148. The salt-responsive element GT1 and ABA-responsive element ABRE4 are two types of cis-elements associated to abiotic stress (Yang et al., 2020). We found these two elements, as well as the GA-responsive element W-BOX (Dhatterwal et al., 2019), drought-responsive elements MYB and DPBF (Carre and Kim, 2002), and wound-responsive element MYC (Ciznadija et al., 2009) were located within our three selected genes. These results further supported that C2 domain genes may confer abiotic stress tolerance to soybean.
To better understand the possible biological function of C2 domain genes, Blast2GO software were used to performed the GO ontology annotation (Supplementary Figure 3). According to the GO annotation results, the C2 domain proteins were annotated in various biological processes, including response to stress, negative regulation of cell death, intracellular signal transduction, phosphatidylcholine metabolic process, and lipid catabolic process. There were 134 C2 domain proteins were predicted to be related to molecular functions, such as calcium ion binding, calcium-dependent phospholipid binding, lipid binding, N-acylphosphatidylethanolamine-specific phospholipase D activity, signal transducer activity and GTPase activator activity. Finally, 105 C2 domain proteins were belonged to cellular components, which were located to integral component of membrane, membrane, intracellular, endoplasmic reticulum and plasma membrane. Through the GO annotation of C2 domain family, it is found that most of C2 domain genes respond to stress and depend on calcium phospholipid binding. This analysis is helpful for us to further understand and analyze C2 domain family.
To further illuminate salt and drought stress responses of C2 domain genes, GmC2-58, GmC2-88, and GmC2-148 were investigated by RT-qPCR from soybean seedlings exposed to salt, drought and ABA treatments (Figure 6). Among the three genes, GmC2-148 obtained the highest expression levels in the various treatments, thus it was selected for subsequent experiments. In order to verify that GmC2-148 could respond to a variety of treatments, we performed RT-qPCR on soybean exposed to additional treatments of high temperature, low temperature and external spraying of BR (Figure 6). In all of these additional treatments, GmC2-148, again showed high levels of expression, further supporting that C2 domain genes respond to various treatments.
Figure 6. Expression patterns obtained by RT-qPCR of the three selected C2 domain genes from soybean exposed to salt, drought, and ABA treatments. (A) Durations of salt treatment exposure were 0, 1, 2, 4, 8, 12, and 24 h. (B) Drought treatment durations were 0, 1, 2, 4, 8, 12, and 24 h. (C) ABA-treated leaves were sampled at the same times as the samples for the salt and drought treatments. (D) Expression patterns of GmC2-148 under heat, cold and BR treatments by RT-qPCR. The vertical coordinates are fold changes, and the horizontal ordinates are treatment times. The actin gene was used as an internal reference. Data are means of three biological repeats ± SD.
Through online website analysis, we found that GmC2-148 is a transmembrane protein with typical C2 domain (Supplementary Figures 4,5). To scrutinize the subcellular localization of GmC2-148, we prepared protoplasts from Arabidopsis and carried out subcellular localization experiments. The 16318hGFP was used as a control, GFP fluorescence was observed throughout cells. Meanwhile the GmC2-148-GFP fusion protein was mainly localized in cell membrane indicating that GmC2-148 was located in the cytomembrane (Figure 7).
Figure 7. Localization of C2 domain protein GmC2-148 in Arabidopsis protoplasts. The images of the construct transferred into Arabidopsis protoplasts were observed under a laser scanning confocal microscope. Bar = 10 μm.
To further explore the biological function of the C2 domain gene GmC2-148 in salt response, we carried out salt treatment on EV and the soybean plants with GmC2-148 transgenic hairy roots to observe the phenotype and mark data statistics. When under normal growth conditions, no significant differences were observed between the EV and the soybean plants with GmC2-148 transgenic hairy roots. In contrast, apparent differences were found in the growth and physiology between the EV and the soybean plants with GmC2-148 transgenic hairy roots after salt treatment for several days. Compared with the control group, the salt treatment group clearly exhibited curled and yellow leaves and a lower number of green leaves. Additional physiological data differed between control and treatment plants. The soybean plants with GmC2-148 transgenic hairy roots leaves showed delayed leaf rolling, higher Pro content, and lower contents of MDA, H2O2, and O2– compared with those of the EV control plants. The results of NBT and trypan blue-staining of leaves and other physiological indexes, such as electrical conductivity, chlorophyll content, survival rate, and relative water content, also showed that the resistance of the soybean plants with GmC2-148 transgenic hairy roots under salt stress was significantly better than that of EV control plants (Figure 8). At the same time, the root length of the soybean plants with GmC2-148 transgenic hairy roots after salt treatment was significantly longer than that of EV, because the root is an important organ for plants to absorb nutrients (Figure 10). The results of leaf staining and other physiological indicators also verify that the soybean plants with GmC2-148 transgenic hairy roots may enhance soybean resistance to salt treatment.
Figure 8. Salt stress analyses of EV and the soybean plants with GmC2-148 transgenic hairy roots. (A) Phenotypes of EV and the soybean plants with GmC2-148 transgenic hairy roots under control and salt treatments. (B) NBT and (C) Trypan blue staining of EV and the soybean plants with GmC2-148 transgenic hairy roots under control and salt treatments; dead cells stain, while living cells do not stain. (D) MDA content, (E) Pro content, (F) H2O2 content, (G) O2− content, (H) electrical conductivity, (I) chlorophyll content, (J) survival rate, and (K) relative water content of EV and the soybean plants with GmC2-148 transgenic hairy roots under normal and salt conditions. Data are means ± SD obtained from three biological replicates. Significant differences determined by ANOVA at *p < 0.05 and **p < 0.01.
To determine the roles of GmC2-148 in drought response, a drought treatment was carried out on EV and the soybean plants with GmC2-148 transgenic hairy roots. Similarly, we found that there was no noteworthy difference between EV and the soybean plants with GmC2-148 transgenic hairy roots under normal growth conditions, but the leaf wilting and shedding of EV was more serious than that of the soybean plants with GmC2-148 transgenic hairy roots under drought conditions, indicating that the soybean plants with GmC2-148 transgenic hairy roots had stronger stress resistance under drought conditions. Similar to the salt stress treatment, the soybean plants with GmC2-148 transgenic hairy roots accumulated more Pro and had lower MDA, H2O2, and O2– contents (Figure 9). The results of NBT and trypan blue-staining of leaves and other physiological indicators measured after drought stress treatment verified that the soybean plants with GmC2-148 transgenic hairy roots heightened soybean resistance to drought stress. Correspondingly, the root length of the soybean plants with GmC2-148 transgenic hairy roots was considerably longer than that of EV after drought treatment (Figure 10).
Figure 9. Root length investigations of EV and the soybean plants with GmC2-148 transgenic hairy roots. (A–C) Root length pictures of EV and the soybean plants with GmC2-148 transgenic hairy roots after salt and drought treatments. (D) Fresh weight of root after salt treatment. (E) Fresh weight of root after drought treatment. (F) Root length after salt treatment. (G) Root length after drought treatment. *p < 0.05 and **p < 0.01.
Figure 10. Drought stress investigations of EV and the soybean plants with GmC2-148 transgenic hairy roots. (A) Phenotypes of EV and the soybean plants with GmC2-148 transgenic hairy roots under control and drought treatments. (B) NBT and (C) Trypan blue staining of EV and the soybean plants with GmC2-148 transgenic hairy roots under control and drought treatments; dead cells can be stained, while living cells cannot. (D) MDA content, (E) Pro content, (F) H2O2 content, (G) O2− content, (H) electrical conductivity, (I) chlorophyll content, (J) survival rate, and (K) relative water content of EV and the soybean plants with GmC2-148 transgenic hairy roots under normal and drought conditions. Data shown are means ± SD obtained from three biological replicates. Significant differences determined by ANOVA. *p < 0.05 and **p < 0.01.
In Arabidopsis, we also verified the GmC2-148 transgenic plants resistance to abiotic stress. After Arabidopsis grown in 25°C and 70% humidity greenhouse for 7 days, and then treated them with salt and drought treatment respectively. In the root length experiment of Arabidopsis (Figure 11), the root length of transgenic plants treated with NaCl, PEG and ABA were longer than those of WT, indicating that GmC2-148 improved the ability of Arabidopsis root to resist abiotic stress. As shown by Figure 12, the growth status of GmC2-148 transgenic plants in Arabidopsis after adverse treatments was considerably better than that of WT, and the physiological indicators, survival rate, MDA and Pro also substantiated our results, showing that GmC2-148 transgenic plants augmented the resistance of Arabidopsis to abiotic stress.
Figure 11. Root length investigations of EV and GmC2-148 transgenic Arabidopsis. (A–C) Root length of transgenic plants and WT after NaCl, PEG and ABA treatments. (D) Statistics of root length data of transgenic plants and WT after salt treatment. (E) Root length of transgenic Arabidopsis and WT after PEG treatment. (F) Root length of transgenic Arabidopsis and WT after ABA treatment. *p < 0.05 and **p < 0.01.
Figure 12. Phenotypic investigations of Arabidopsis after salt and drought treatments. (A) Phenotypes of EV and GmC2-148 transgenic Arabidopsis plants under salt and drought treatments. (B) Statistics on the survival rate of transgenic plants and WT after salt and drought treatment. (C) MDA content of Arabidopsis after salt and drought treatments. (D) Pro content in transgenic Arabidopsis and WT after abiotic treatments.
Several genes, COR47 (Guo et al., 1992), NCDE3 (Pandey and Gautam, 2020), NAC11 (An et al., 2018), WRKY13 (Xiao et al., 2013), DREB2A (Agarwal et al., 2007), MYB84 (Agarwal et al., 2020), bZIP44 (Yang et al., 2019), and KIN1 (Wang et al., 1995) play significant roles in salt and drought stresses. Analysis of the transcripts of these marker genes between EV and the soybean plants with GmC2-148 transgenic hairy roots revealed that the soybean plants with GmC2-148 transgenic hairy roots produced more transcripts of all of these genes under abiotic stress conditions (Figure 13). Thus the relationship between these marker genes and C2 domain family in resisting abiotic stress is worthy of further study.
Figure 13. The soybean plants with GmC2-148 transgenic hairy roots revealed greater levels of transcription of salt and drought-stress marker genes (A–H). Two-week-old soybean seedlings were obtained for RT-qPCR analyses. The actin gene was used as an internal control. Data shown are means ± SD obtained from three biological replicates.
C2 domain proteins are abundant, however, their functions are still elusive (Antal et al., 2015). In order to gain more insights, we analyzed abiotic stress responses of C2 domain genes by obtaining transcriptome data from soybean exposed to salt, drought, and ABA treatments. To clarify the functions of C2 domain genes, we isolated and identified the GmC2-148 gene from 180 soybean C2 domain genes. The observed phenotypes and potential molecular mechanisms of GmC2-148 demonstrate that this gene improved salt and drought tolerance in soybean.
When plants are subjected to various stresses, a large amount of active oxygen is produced. A primary mechanism of harm to plants is the imbalance of the production and removal of active oxygen in the plant body (Adamipour et al., 2020). The imbalance between systems may cause excessive accumulation of reactive oxygen species, which in turn damages proteins, membrane lipids and other cellular components, as well as causes oxidative damage to plants. Our results showed that GmC2-148 showed strong responses to the salt, drought, and ABA treatments, as well as displayed a certain degree of response to BR. As reported, BR can enhance plant resistance to abiotic stresses through its effect on cell membranes (Marin-de la Rosa et al., 2019), and GmC2-148 protein is located in on the cell membrane. We suspect that the response of GmC2-148 to abiotic stresses may be involved in the BR signaling pathway. Brassinolide is a sterol plant hormone that participates in plant growth, various physiological reactions and resistance to oxidative stress development and various physiological reactions. It is also plays an essential role in plant resistance to oxidative stress. Reportedly, BR enhances the tolerance to oxidative stress by promoting the synthesis of another plant hormone, ABA, and inducing the antioxidant defense system. However, it is not clear how BR interacts with ABA to enhance C2 domain proteins in regards to plant tolerance to oxidative stress. Our research found that the gene expression levels of GmC2-148 were simultaneously induced by BR and ABA. Therefore, we assume that C2 domain proteins may be involved in the crosstalk of various stresses and hormones in plants.
The plant root functions to absorb water and minerals provide them to stems and leaves, while storing nutrients (Zhao et al., 2020). In order to maintain and improve plant vigor in salt and drought conditions and thus increase chances of survival, roots help maintain ion balance by controlling ion migration into or out of cells through the cell membrane. A large number of studies have shown that the expression of C2 domain genes are unlike in diverse plants, for example, CaSRC2-1 was mainly expressed in roots (Kim et al., 2008). Yang et al. (2008) found that rice OsPBP1 expression was highest in non-pollinated pistils. We found the expression of GmC2-148 was highest in roots (Figure 5). These results altogether suggest that C2 domain genes have different tissue specificities. We observed root length of the soybean plants with GmC2-148 transgenic hairy roots were significantly longer than that of EV after salt and drought treatments (Figure 10), indicating that C2 domain genes respond to abiotic stresses and may enhance soybean’s ability to resist abiotic stresses. We suspect that GmC2-148 can increase the osmotic balance of water and the balance of anions and cations in cells throughout the root under stressful circumstances, which can improve salt and drought tolerance in soybean. We also did root length experiments in Arabidopsis under NaCl, PEG and ABA treatments to verify the function of GmC2-148 (Figure 11). The results showed that the GmC2-148 transgenic plants in Arabidopsis improved the growth status of Arabidopsis compared with WT under these conditions. Shinozaki et al. have studied in detail the regulation of gene expression in Arabidopsis under abiotic stresses. They believe that there are at least four independent signal transduction pathways between the initial signal of dehydration stress and gene expression 2 are ABA-dependent and 2 are ABA-independent (Nakashima and Yamaguchi-Shinozaki, 2013). We speculate that C2 domain family members are ABA-dependent under abiotic stresses and gene expression. So the longer roots of Arabidopsis after ABA treatment compared to control WT suggested that the GmC2-148 may act in stress tolerance through/reduction of ABA signaling or responses. The relationship between C2 domain genes and ABA will be the focus of our next experimental study.
In plants, the C2 domain proteins are reportedly involved in diverse processes, including abiotic stress, pollen fertility, and membrane targeting and leaf senescence in a Ca2+-dependent manner (Kang et al., 2013). Previous studies have shown that C2 domain proteins can interact with Ca2+ ions because of their five conserved aspartic acid (Asp) residues (Rizo and Sudhof, 1998). As report goes that Ca2+ plays a vital role as a second messenger in response to abiotic stresses (Zheng et al., 2014). Elevation of cytosolic Ca2+ is induced by salt and high osmotic pressure, and the elevated level is connected to the expression of stress-responsive genes (Zhao et al., 2010). Through our research we found that although plant C2 domain genes are not well known, a number of reports suggested their function participation in Ca2+ signaling (Xie et al., 2011). The SYT1, an Arabidopsis C2 domain protein, involved in response to freeze stress with Ca2+-dependent manner (Yamazaki et al., 2008). In this study, the mode of binding between C2 domain protein GmC2-148 and Ca2+ needs further elaboration.
In the present study, we implemented a genome-wide analysis of the C2 domain gene family in soybean. A total of 180 C2 domain genes were identified based on the conserved C2 domain, and the genes were classified into three major groups, I, II, and III. Most members of group I responded to salt, drought and ABA treatments. GmC2-148, encoding a cell membrane-localized protein, is a positive regulator of plant tolerance to salt and drought stresses by activating abiotic stress-related genes. However, further study is needed to determine the specific functions of the C2 domain genes. To sum up, the present study investigates the importance of C2 domain genes in abiotic stress-tolerance in soybean.
The raw data supporting the conclusions of this article will be made available by the authors, without undue reservation.
Z-SX coordinated the project, conceived and designed the experiments, and edited the manuscript. YS performed the experiments and wrote the first draft. J-YZ revised the manuscript. Y-TL and P-GZ contributed to the data analysis and managed reagents. Z-WF contributed with the valuable discussions. JG put forward valuable opinions when revising the manuscript. All authors reviewed and approved the final manuscript.
This research was financially supported by the National Transgenic Key Project of the Ministry of Agriculture of China (2020ZX08009-15B), the National Natural Science Foundation of China (31871624), and Fundamental Research Funds for Central Non-Profit of Institute of Crop Sciences, Chinese Academy of Agricultural Sciences.
The authors declare that the research was conducted in the absence of any commercial or financial relationships that could be construed as a potential conflict of interest.
We are grateful to Drs. Lijuan Qiu and Tianfu Han of the Institute of Crop Science, Chinese Academy of Agricultural Sciences (CAAS) for kindly providing soybean seeds. Also, we are grateful to Drs. Wensheng Hou and Hui Zhang of the Institute of Crop Science, CAAS for kindly providing vectors and procedure of high-efficiency A. rhizogenes-mediated transformation, respectively.
The Supplementary Material for this article can be found online at: https://www.frontiersin.org/articles/10.3389/fpls.2021.620544/full#supplementary-material
Supplementary Figure 1 | Chromosomal location of C2 domain genes. Blue bars represent the chromosomes with their chromosome number above the bar. The names of C2 domain genes are labeled at both sides of the chromosomes. Scale bars on the left show chromosome lengths (Mb).
Supplementary Figure 2 | Putative cis-elements in a 1.5-kb 5′-flanking region upstream from the start codon. Various cis-elements are indicated by colored symbols and placed in positions relative to the promoter.
Supplementary Figure 3 | GO enrichment annotation map.
Supplementary Figure 4 | Annotation diagram of GmC2-148 C2 domain.
Supplementary Figure 5 | Annotated diagram of GmC2-148 transmembrane protein.
Supplementary Table 1 | Physicochemical properties related description of C2 domain genes in soybean.
Supplementary Table 2 | Primers and sequences used in this study.
Supplementary Table 3 | Data about the response of C2 domain genes in salt treated transcriptome.
Supplementary Table 4 | Data on the response of C2 domain genes in drought treated transcriptome.
Supplementary Table 5 | Data on the response of C2 domain genes in ABA treated transcriptome.
Supplementary Table 6 | Data of tissue-specific expression levels of C2 domain genes.
ABA, abscisic acid; RT-qPCR, real-time quantitative polymerase chain reaction; GFP, green fluorescent protein; MDA, malondialdehyde; Pro, proline; PEG, polyethylene glycol; BR, brassinolide; CDS, coding sequence length; HMM, Hidden Markov model; NJ, neighbor-joining; EV, empty vector; NBT, Nitrogen Blue Tetrazole; WT, wild type.
Adamipour, N., Khosh-Khui, M., Salehi, H., Razi, H., Karami, A., and Moghadam, A. (2020). Metabolic and genes expression analyses involved in proline metabolism of two rose species under drought stress. Plant Physiol. Biochem. 155, 105–113. doi: 10.1016/j.plaphy.2020.07.028
Agarwal, P., Agarwal, P. K., Nair, S., Sopory, S. K., and Reddy, M. K. (2007). Stress-inducible DREB2A transcription factor from Pennisetum glaucum is a phosphoprotein and its phosphorylation negatively regulates its DNA-binding activity. Mol. Genet. Genom. 277, 189–198. doi: 10.1007/s00438-006-0183-z
Agarwal, P., Baranwal, V. K., and Khurana, P. (2019). Genome-wide analysis of bZIP transcription factors in wheat and functional characterization of a TabZIP under abiotic stress. Sci. Rep. 9:4608. doi: 10.1038/s41598-019-40659-40657
Agarwal, P., Mitra, M., Banerjee, S., and Roy, S. (2020). MYB4 transcription factor, a member of R2R3-subfamily of MYB domain protein, regulates cadmium tolerance via enhanced protection against oxidative damage and increases expression of PCS1 and MT1C in Arabidopsis. Plant Sci. 297:110501. doi: 10.1016/j.plantsci.2020.110501
An, J. P., Li, R., Qu, F. J., You, C. X., Wang, X. F., and Hao, Y. J. (2018). An apple NAC transcription factor negatively regulates cold tolerance via CBF-dependent pathway. J. Plant Physiol. 221, 74–80. doi: 10.1016/j.jplph.2017.12.009
Antal, C. E., Callender, J. A., Kornev, A. P., Taylor, S. S., and Newton, A. C. (2015). Intramolecular C2 Domain-Mediated autoinhibition of protein kinase C betaII. Cell Rep. 12, 1252–1260. doi: 10.1016/j.celrep.2015.07.039
Banci, L., Cavallaro, G., Kheifets, V., and Mochly-Rosen, D. (2002). Molecular dynamics characterization of the C2 domain of protein kinase Cbeta. J. Biol. Chem. 277, 12988–12997. doi: 10.1074/jbc.M106875200
Bohnert, H. J., Nelson, D. E., and Jensen, R. G. (1995). Adaptations to environmental stresses. Plant Cell 7, 1099–1111. doi: 10.1105/tpc.7.7.1099
Carre, I. A., and Kim, J. Y. (2002). MYB transcription factors in the Arabidopsis circadian clock. J. Exp. Bot. 53, 1551–1557. doi: 10.1093/jxb/erf027
Cerezini, P., Kuwano, B. H., Grunvald, A. K., Hungria, M., and Nogueira, M. A. (2020). Soybean tolerance to drought depends on the associated Bradyrhizobium strain. Braz. J. Microbiol. 51, 1977–1986. doi: 10.1007/s42770-020-00375-371
Chapman, E. R., An, S., Edwardson, J. M., and Jahn, R. (1996). A novel function for the second C2 domain of synaptotagmin. Ca2+-triggered dimerization. J. Biol. Chem. 271, 5844–5849. doi: 10.1074/jbc.271.10.5844
Chen, C., Chen, H., Zhang, Y., Thomas, H. R., Frank, M. H., He, Y., et al. (2020). TBtools: an integrative toolkit developed for interactive analyses of big biological data. Mol. Plant 13, 1194–1202. doi: 10.1016/j.molp.2020.06.009
Chenu, K., Chapman, S. C., Hammer, G. L., McLean, G., Salah, H. B., and Tardieu, F. (2008). Short-term responses of leaf growth rate to water deficit scale up to whole-plant and crop levels: an integrated modelling approach in maize. Plant Cell Environ. 31, 378–391. doi: 10.1111/j.1365-3040.2007.01772.x
Cho, K. M., Nguyen, H. T., Kim, S. Y., Shin, J. S., Cho, D. H., Hong, S. B., et al. (2016). CML10, a variant of calmodulin, modulates ascorbic acid synthesis. New Phytol. 209, 664–678. doi: 10.1111/nph.13612
Ciznadija, D., Tothill, R., Waterman, M. L., Zhao, L., Huynh, D., Yu, R. M., et al. (2009). Intestinal adenoma formation and MYC activation are regulated by cooperation between MYB and Wnt signaling. Cell Death Differ. 16, 1530–1538. doi: 10.1038/cdd.2009.94
Conesa, A., and Gotz, S. (2008). Blast2GO: a comprehensive suite for functional analysis in plant genomics. Int. J. Plant. Genom. 2008:619832. doi: 10.1155/2008/619832
Corbalan-Garcia, S., Sanchez-Carrillo, S., Garcia-Garcia, J., and Gomez-Fernandez, J. C. (2003). Characterization of the membrane binding mode of the C2 domain of PKC epsilon. Biochemistry 42, 11661–11668. doi: 10.1021/bi034850d
Cui, X. Y., Gao, Y., Guo, J., Yu, T. F., Zheng, W. J., Liu, Y. W., et al. (2019). BES/BZR transcription factor TaBZR2 positively regulates drought responses by activation of TaGST1. Plant Physiol. 180, 605–620. doi: 10.1104/pp.19.00100
Dhatterwal, P., Basu, S., Mehrotra, S., and Mehrotra, R. (2019). Genome wide analysis of W-box element in Arabidopsis thaliana reveals TGAC motif with genes down regulated by heat and salinity. Sci. Rep. 9:1681. doi: 10.1038/s41598-019-38757-38757
Guo, W., Ward, R. W., and Thomashow, M. F. (1992). Characterization of a cold-regulated wheat gene related to Arabidopsis cor47. Plant Physiol. 100, 915–922. doi: 10.1104/pp.100.2.915
Kang, C. H., Moon, B. C., Park, H. C., Koo, S. C., Chi, Y. H., Cheong, Y. H., et al. (2013). Rice small C2-domain proteins are phosphorylated by calcium-dependent protein kinase. Mol. Cells 35, 381–387. doi: 10.1007/s10059-013-2185-2180
Kereszt, A., Li, D., Indrasumunar, A., Nguyen, C. D., Nontachaiyapoom, S., Kinkema, M., et al. (2007). Agrobacterium rhizogenes-mediated transformation of soybean to study root biology. Nat. Protoc. 2, 948–952. doi: 10.1038/nprot.2007.141
Kim, S. L., Kim, N., Lee, H., Lee, E., Cheon, K. S., Kim, M., et al. (2020). High-throughput phenotyping platform for analyzing drought tolerance in rice. Planta 252:38. doi: 10.1007/s00425-020-03436-3439
Kim, Y. C., Kim, S. Y., Choi, D., Ryu, C. M., and Park, J. M. (2008). Molecular characterization of a pepper C2 domain-containing SRC2 protein implicated in resistance against host and non-host pathogens and abiotic stresses. Planta 227, 1169–1179. doi: 10.1007/s00425-007-0680-682
Li, Q., Zhao, M., Wang, N., Liu, S., Wang, J., Zhang, W., et al. (2020). Water use strategies and drought intensity define the relative contributions of hydraulic failure and carbohydrate depletion during seedling mortality. Plant. Physiol. Biochem. 153, 106–118. doi: 10.1016/j.plaphy.2020.05.023
Liu, X.-J., Dong, Y.-H., Liu, X., You, C.-X., and Hao, Y.-J. (2019). A C2-domain phospholipid-binding protein MdCAIP1 positively regulates salt and osmotic stress tolerance in apple. Plant Cell Tissue Organ Culture (PCTOC) 138, 29–39. doi: 10.1007/s11240-019-01600-y
Lopez-Nicolas, R., Lopez-Andreo, M. J., Marin-Vicente, C., Gomez-Fernandez, J. C., and Corbalan-Garcia, S. (2006). Molecular mechanisms of PKCalpha localization and activation by arachidonic acid. the C2 domain also plays a role. J. Mol. Biol. 357, 1105–1120. doi: 10.1016/j.jmb.2006.01.018
Marin-de la Rosa, N., Lin, C. W., Kang, Y. J., Dhondt, S., Gonzalez, N., Inze, D., et al. (2019). Drought resistance is mediated by divergent strategies in closely related Brassicaceae. New Phytol. 223, 783–797. doi: 10.1111/nph.15841
Molz, L., Chen, Y. W., Hirano, M., and Williams, L. T. (1996). Cpk is a novel class of Drosophila PtdIns 3-kinase containing a C2 domain. J. Biol. Chem. 271, 13892–13899. doi: 10.1074/jbc.271.23.13892
Momota, M., Nagayama, M., Okude, H., Ishii, K. J., Ori, D., Kawasaki, T., et al. (2020). The Ca(2+)-dependent pathway contributes to changes in the subcellular localization and extracellular release of interleukin-33. Biochem. Biophys. Res. Commun. 530, 699–705. doi: 10.1016/j.bbrc.2020.07.127
Nakashima, K., and Yamaguchi-Shinozaki, K. (2013). ABA signaling in stress-response and seed development. Plant Cell Rep. 32, 959–970. doi: 10.1007/s00299-013-1418-1411
Nalefski, E. A., and Falke, J. J. (1996). The C2 domain calcium-binding motif: structural and functional diversity. Protein Sci. 5, 2375–2390. doi: 10.1002/pro.5560051201
Ouelhadj, A., Kuschk, P., and Humbeck, K. (2006). Heavy metal stress and leaf senescence induce the barley gene HvC2d1 encoding a calcium-dependent novel C2 domain-like protein. New Phytol. 170, 261–273. doi: 10.1111/j.1469-8137.2006.01663.x
Pak Dek, M. S., Padmanabhan, P., Tiwari, K., Todd, J. F., and Paliyath, G. (2020). Structural and functional characterization of Solanum lycopersicum phosphatidylinositol 3-kinase C2 domain. Plant Physiol. Biochem. 148, 180–192. doi: 10.1016/j.plaphy.2020.01.014
Pandey, A. K., and Gautam, A. (2020). Stress responsive gene regulation in relation to hydrogen sulfide in plants under abiotic stress. Physiol. Plant 168, 511–525. doi: 10.1111/ppl.13064
Ramirez-Ortega, F. A., Herrera-Pola, P. S., Toscano-Morales, R., Xoconostle-Cazares, B., and Ruiz-Medrano, R. (2014). Overexpression of the pumpkin (Cucurbita maxima) 16 kDa phloem protein CmPP16 increases tolerance to water deficit. Plant Signal Behav. 9:e973823. doi: 10.4161/15592324.2014.973823
Rizo, J., and Sudhof, T. C. (1998). C2-domains, structure and function of a universal Ca2+-binding domain. J. Biol. Chem. 273, 15879–15882. doi: 10.1074/jbc.273.26.15879
Shi, W. Y., Du, Y. T., Ma, J., Min, D. H., Jin, L. G., Chen, J., et al. (2018). The WRKY transcription factor GmWRKY12 confers drought and salt tolerance in soybean. Int. J. Mol. Sci. 19:4087. doi: 10.3390/ijms19124087
Vasseur, F., Bontpart, T., Dauzat, M., Granier, C., and Vile, D. (2014). Multivariate genetic analysis of plant responses to water deficit and high temperature revealed contrasting adaptive strategies. J. Exp. Bot. 65, 6457–6469. doi: 10.1093/jxb/eru364
Wang, H., Datla, R., Georges, F., Loewen, M., and Cutler, A. J. (1995). Promoters from kin1 and cor6.6, two homologous Arabidopsis thaliana genes: transcriptional regulation and gene expression induced by low temperature, ABA, osmoticum and dehydration. Plant Mol. Biol. 28, 605–617. doi: 10.1007/BF00021187
Wang, X. (2002). Phospholipase D in hormonal and stress signaling. Curr. Opin. Plant Biol. 5, 408–414. doi: 10.1016/s1369-5266(02)00283-282
Xiao, J., Cheng, H., Li, X., Xiao, J., Xu, C., and Wang, S. (2013). Rice WRKY13 regulates cross talk between abiotic and biotic stress signaling pathways by selective binding to different cis-elements. Plant Physiol. 163, 1868–1882. doi: 10.1104/pp.113.226019
Xie, X., Gong, Z., Mansuy-Aubert, V., Zhou, Q. L., Tatulian, S. A., Sehrt, D., et al. (2011). C2 domain-containing phosphoprotein CDP138 regulates GLUT4 insertion into the plasma membrane. Cell Metab. 14, 378–389. doi: 10.1016/j.cmet.2011.06.015
Yamazaki, T., Kawamura, Y., Minami, A., and Uemura, M. (2008). Calcium-dependent freezing tolerance in Arabidopsis involves membrane resealing via synaptotagmin SYT1. Plant Cell 20, 3389–3404. doi: 10.1105/tpc.108.062679
Yang, Q., Yang, B., Li, J., Wang, Y., Tao, R., Yang, F., et al. (2020). ABA-responsive ABRE-BINDING FACTOR3 activates DAM3 expression to promote bud dormancy in Asian pear. Plant Cell Environ. 43, 1360–1375. doi: 10.1111/pce.13744
Yang, S., Xu, K., Chen, S., Li, T., Xia, H., Chen, L., et al. (2019). A stress-responsive bZIP transcription factor OsbZIP62 improves drought and oxidative tolerance in rice. BMC Plant Biol. 19:260. doi: 10.1186/s12870-019-1872-1871
Yang, W. Q., Lai, Y., Li, M. N., Xu, W. Y., and Xue, Y. B. (2008). A novel C2-domain phospholipid-binding protein, OsPBP1, is required for pollen fertility in rice. Mol. Plant 1, 770–785. doi: 10.1093/mp/ssn035
Yokotani, N., Ichikawa, T., Kondou, Y., Maeda, S., Iwabuchi, M., Mori, M., et al. (2009). Overexpression of a rice gene encoding a small C2 domain protein OsSMCP1 increases tolerance to abiotic and biotic stresses in transgenic Arabidopsis. Plant Mol Biol 71, 391–402. doi: 10.1007/s11103-009-9530-x
Zhao, L., Hu, Y. H., Sun, L., and Sun, J. S. (2010). SmC2P1, a C2 domain protein from Scophthalmus maximus that binds bacterial pathogen-infected lymphocytes and reduces bacterial survival. Fish Shellfish Immunol. 29, 786–792. doi: 10.1016/j.fsi.2010.07.013
Zhao, Q., Guo, J., Shu, M., Wang, P., and Hu, S. (2020). Impacts of drought and nitrogen enrichment on leaf nutrient resorption and root nutrient allocation in four Tibetan plant species. Sci. Total Environ. 723:138106. doi: 10.1016/j.scitotenv.2020.138106
Zhao, Y., Tian, X., Wang, F., Zhang, L., Xin, M., Hu, Z., et al. (2017). Characterization of wheat MYB genes responsive to high temperatures. BMC Plant Biol. 17:208. doi: 10.1186/s12870-017-1158-1154
Keywords: C2 domain protein, drought tolerance, salt tolerance, Arabidopsis, soybean
Citation: Sun Y, Zhao J-Y, Li Y-T, Zhang P-G, Wang S-P, Guo J, Chen J, Zhou Y-B, Chen M, Ma Y-Z, Fang Z-W and Xu Z-S (2021) Genome-Wide Analysis of the C2 Domain Family in Soybean and Identification of a Putative Abiotic Stress Response Gene GmC2-148. Front. Plant Sci. 12:620544. doi: 10.3389/fpls.2021.620544
Received: 23 October 2020; Accepted: 05 January 2021;
Published: 16 February 2021.
Edited by:
Jose C. Jimenez-Lopez, Estación Experimental de Zaidín (CSIC), SpainReviewed by:
Xiaoli Sun, Heilongjiang Bayi Agricultural University, ChinaCopyright © 2021 Sun, Zhao, Li, Zhang, Wang, Guo, Chen, Zhou, Chen, Ma, Fang and Xu. This is an open-access article distributed under the terms of the Creative Commons Attribution License (CC BY). The use, distribution or reproduction in other forums is permitted, provided the original author(s) and the copyright owner(s) are credited and that the original publication in this journal is cited, in accordance with accepted academic practice. No use, distribution or reproduction is permitted which does not comply with these terms.
*Correspondence: Zheng-Wu Fang, ZmFuZ3poZW5nd3U4OEAxNjMuY29t; Zhao-Shi Xu, eHV6aGFvc2hpQGNhYXMuY24=
†These authors have contributed equally to this work
Disclaimer: All claims expressed in this article are solely those of the authors and do not necessarily represent those of their affiliated organizations, or those of the publisher, the editors and the reviewers. Any product that may be evaluated in this article or claim that may be made by its manufacturer is not guaranteed or endorsed by the publisher.
Research integrity at Frontiers
Learn more about the work of our research integrity team to safeguard the quality of each article we publish.