- Beijing Key Laboratory for Agricultural Application and New Technique, College of Plant Science and Technology, Beijing University of Agriculture, Beijing, China
Mineral nutrition, taken up from the soil or foliar sprayed, plays fundamental roles in plant growth and development. Among of at least 14 mineral elements, the macronutrients nitrogen (N), potassium (K), phosphorus (P), and calcium (Ca) and the micronutrient iron (Fe) are essential to Rosaceae fruit yield and quality. Deficiencies in minerals strongly affect metabolism with subsequent impacts on the growth and development of fruit trees. This ultimately affects the yield, nutritional value, and quality of fruit. Especially, the main reason of the postharvest storage loss caused by physiological disorders is the improper proportion of mineral nutrient elements. In recent years, many important mineral transport proteins and their regulatory components are increasingly revealed, which make drastic progress in understanding the molecular mechanisms for mineral nutrition (N, P, K, Ca, and Fe) in various aspects including plant growth, fruit development, quality, nutrition, and postharvest storage. Importantly, many studies have found that mineral nutrition, such as N, P, and Fe, not only affects fruit quality directly but also influences the absorption and the content of other nutrient elements. In this review, we provide insights of the mineral nutrients into their function, transport, signal transduction associated with Rosaceae fruit quality, and postharvest storage at physiological and molecular levels. These studies will contribute to provide theoretical basis to improve fertilizer efficient utilization and fruit industry sustainable development.
Introduction
Many species of Rosaceae are rich in economic value, such as apple, pear, peach, apricot, plum, cherry, loquat, mango, almond, and strawberry. Fruits are rich in vitamins, sugars, and organic acids, as well as many kinds of health-benefit compounds (Mahmood et al., 2012). These compounds, including anthocyanins and vitamins, may have anti-inflammatory and antioxidant effects, and thus fruits are usually used to maintain a balanced diet (Kumari et al., 2017). In addition, dry fruits, such as almond, are especially abundant in nutrition and also are widely cultivated in the world (Maguire et al., 2004). Fruits not only can be eaten directly but also can be used for food industry in the production of wine, juice, jam, and so on. Thus, fruits are important components in our life, and improvement of fruit quality is going on all the time in orchard management.
It is well known that mineral nutrients, especially macronutrients nitrogen (N), phosphorus (P), potassium (K), and calcium (Ca) as well as micronutrient iron (Fe), are essential for plant growth, fruit yield, and quality (Falchi et al., 2020; Singh et al., 2020). In recent years, many studies have strengthen our insights into the influence and the molecular mechanisms for mineral nutrition (N, P, K, Ca, and Fe) in Rosaceae plant growth, fruit development, quality, and preservation, especially highlighting N in fruit yield and P and K in fruit quality. Mineral nutrition, such as N, P, and Fe, not only affects fruit quality directly but also affects the absorption of other nutrient elements.
In this review, we integrated many previously defined signaling components linked to mineral nutrition transport and signal transduction in the regulation of fruit quality at physiological and molecular levels. This review will not only contribute to provide the theoretical basis to improve fertilizer efficient utilization and fruit industry sustainable development but also highlight the important aims of future work.
Two Faces of Nitrogen Application in Fruit Yield and Quality
Nitrogen (N) is a ubiquitous element that constituted nucleic acids, amino acids, proteins, chlorophyll, as well as many other metabolites in plants. Therefore, it is an indispensable important nutrient element for plant growth and development. Plants acquire inorganic N from the soil, mainly in the form of ammonium and nitrate, and low or excessive N level has a serious influence on fruit yield and quality.
When strawberries were treated with three levels of NO3− (9, 12, and 15 mol/m3), the highest NO3− concentration of nutrient solution produced the maximum yield, but the nutraceutical quality, such as antioxidant capacity and phenolic compounds, significantly declined (Preciado-Rangel et al., 2020). Under sufficient soil moisture, the application of N fertilizer to potted apple trees caused an increase in stomatal conductance, which decreased water use efficiency (WUE; Qu et al., 2000). Under soil drought, the WUE of apple trees under nitrogen fertilizer was significantly higher than that of the control, owing to the promotion of mesophyll capacity and photosynthesis (Qu et al., 2000).
As the increasing of N supply in apple (Malus domestica Borkh.) trees, the concentrations of sucrose (Suc), glucose (Glc), fructose (Fru), and total nonstructural carbohydrates (TNC) decreased (Cheng et al., 2004), resulting in the production of organic acids. It is confirmed that in strawberries, applying high nitrogen amounts could lead to an increase in citric acid content and conceal fruit sweet flavor, whereas low nitrogen levels could decrease acidity and result in an observably lower content of ascorbic acid and higher fruit firmness (Cardeñosa et al., 2015).
Anthocyanin and chlorophyll are remarked signs for fruit quality, and many research studies suggest that increasing N supply decreases anthocyanin synthesis but induces chlorophyll content in fruits. Under high N nutrition in apple trees, shoot growth was enhanced, and the activity of phenylalanine ammonia lyase, a key enzyme involved in flavonoid biosynthesis, seemed to be downregulated, resulting in a generally decreased flavonoid accumulation (Strissel et al., 2005). N fertilization could be used to suppress anthocyanin formation in green apple cultivars, such as “Granny Smith,” in that red blush was undesirable (Ritenour and Khemira, 2007). High N could lead to higher chlorophyll concentration of fruit skin, thus increasing fruit greenness; on the contrary, nitrate deficiency suppressed nitrate absorption and assimilation and inhibited the transformation of 5-aminolevulinic acid (ALA) into porphobilinogen (PBG), thus suppressing chlorophyll synthesis in apple (Wen et al., 2019). Peach fruit from the higher N rates was greener and had higher total soluble solids than fruit from the trees under low N (Rubio Ames et al., 2020).
The application of the nitrification inhibitor, 3,4-dimethylpyrazole phosphate (DMPP) and abscisic acid (ABA), could effectively improve the problem of poor fruit coloring caused by excessive N fertilizer application used in apple trees (Wang et al., 2020a,b). DMPP reduced the capacity of N absorption and N accumulation in fruits and whole apple plant, whereas it increased fruit anthocyanin and soluble solid content (SSC), and it had no significant effect on fruit yield (Wang et al., 2020a). An appropriate ABA concentration could promote the expression of anthocyanin synthesis genes and transcription factors, as well as the anthocyanin content of the “Red Fuji” apple peel. More than that, exogenous ABA reduced the accumulation of N and increased the contents of sugar and carbon in fruits (Wang et al., 2020b).
To improve the anthocyanin content and accordingly the quality and market value of important agricultural commodities, it can be a powerful tool to supply nutrient strategically. In particular, the precise application of N fertilizer should not only improve fruit yield but also positively influence fruit quality including anthocyanin content (Jezek et al., 2018).
Molecular Mechanisms of Nitrogen Assimilate, Transport, and Action
Thirteen ammonium transporters of AMT1 subfamily in the apple rootstock Malus robusta Rehd were grouped into four clusters: clade I (MrAMT1;1 and MrAMT1;3), clade II (MrAMT1;4), clade III (MrAMT1;2), and clade IV (MrAMT1;5). All the AMT1s, except MrAMT1;4, were highly expressed in vegetative organs and significantly responded to different nitrogen supplies. The ammonium absorption activities of these AMT1s were confirmed in the AMT-defective mutant yeast system, though their NH4+ uptake kinetics varied (Li et al., 2017).
There were 73 NPF (Nitrate Transporter 1/Peptide Transporter Family) genes in apple that were organized into eight major groups (Wang et al., 2018b). MdNPF6.5 was strongly induced by both low-nitrate and high-nitrate levels, and the overexpression of MdNPF6.5 in apple calli enhanced the tolerance to low-N stress, suggesting that MdNPF6.5 has greater nitrogen uptake activity (Wang et al., 2018b). Under low N treatment, the expression of nitrate transporter MdNRT2.4 is higher in high nitrogen-use efficiency (NUE) apple cultivars than in low NUE apple (Wang et al., 2019a), which may explain the NUE differences among apple cultivars.
Anion channel PbrSLAH3 of pear (Pyrus bretschneideri) could rescue the ammonium toxicity phenomenon of slah3 mutant grown in high-ammonium/low-nitrate conditions, which was activated by phosphorylation by calcium-dependent protein kinase PbrCPK32 (Chen et al., 2019b). The overexpression of autophagy proteins MdATG18a and MdATG9 enhanced the tolerance to N-deficiency stress and the Suc concentration in apple callus (Sun et al., 2018b; Huo et al., 2020). MdATG18a played a role in nitrate uptake and assimilation by the upregulation of nitrate reductase (NR) MdNIA2 and nitrate transporters MdNRT2.1/2.4/2.5 (Sun et al., 2018b). MdATG9 regulated the expression of MdNRT1.1, MdNRT2.5, MdNIA1, and MdNIA2 in response to N starvation in apple (Huo et al., 2020). Overexpressing MdHY5 (transcription factor LONG HYPOCOTYL 5) in apple callus induced the expression of N acquisition-related genes and increased the activity of NR, finally improving nitrate contents (An et al., 2017).
Phosphorus Fertilizer Improves Fruit Yield, Quality, and Postharvest Storage Quality
Phosphorus (P) supplementation has become a primary agricultural management aspect of fruit production, because P not only affects fruit yield but also modulates the production of the soluble solid and secondary metabolites, such as ascorbic acid and flavonoids, which are closely related to fruit quality.
When appropriate phosphorus was added, the weight of strawberry fruits increased notably (Afroz et al., 2016). The SSC was lower in fruits from P shortage strawberries, indicating that P deficiency could negatively affect sugar biosynthesis (Valentinuzzi et al., 2015a). SSC in strawberry fruits was positively related to the phosphorus content, and P fertilizer could effectively increase SSC in strawberry fruit (Cao et al., 2015). Meanwhile, P content, WUE, and photosynthetic rate (Pn) increased in phosphoric acid-treated strawberry fruits (Cao et al., 2015).
P starvation resulted in a significant change of bioactive compounds in strawberry fruits. The contents of vitamin C, malic acid, galactaric acid, proline, lysine, sorbitol-6-phosphate, malate, and citrate were also inhibited when P was deficient (Valentinuzzi et al., 2015b; Afroz et al., 2016; Zhang et al., 2020), whereas anthocyanin contents were higher (Valentinuzzi et al., 2015a). However, field experiments had shown that the application of phosphite (Phi), a salt of phosphorous acid absorbed and transferred in a way similar to inorganic P, increased the anthocyanin content of strawberry (Estrada-Ortiz et al., 2013). Therefore, the effect of fruit quality formation varies with the type and timing of P fertilizer application (Jezek et al., 2018).
P deficiency also changes other ion contents in plants. The fruit firmness of strawberry fruits under P deficiency increased 60% compared with that of the control, which may be because of the higher concentration of Ca (Valentinuzzi et al., 2015a). Moreover, low P alleviated the Fe deficiency phenotype in apples and improved the ferric-chelated reductase activity of the rhizosphere, because P shortage promotes proton exocytosis and organic acids exudation and enhances Fe absorption, thus increasing the Fe content in both the shoots and roots under Fe deficiency in apples (Zhang et al., 2020). For another, the limited elongation of the main roots under low inorganic phosphate (Pi) conditions may be results of the Fe accumulation in the apical meristem (Zhang et al., 2020).
Many plants can utilize phosphate from places outside the nutrient-depleted zone through symbiotic arbuscular mycorrhizal fungi (AMF; Ezawa and Saito, 2018). The colonization by AMF and appropriate P supplementation of traditional (TF) or organic (OF) fertilizer was regarded as the main approach to raise the yield and quality of strawberry fruits in acidic soils with low P availability (Parada et al., 2019). There was a highly positive correlation between the concentration of P in apple rootstocks and the bacterial genera Bacillus (Chai et al., 2020). The P-efficient hybrid apple lines had better growth characteristics and stronger root structure, as well as more abundant Bacillus in the rhizosphere, than the P-inefficient hybrid line plants (Chai et al., 2020). However, according to some reports, there is no correlation between the AMF association and low P tolerance when plants are grown under optimal nutrient conditions (Cockerton et al., 2020).
Trisodium phosphate (TSP) treatment could maintain the postharvest quality of apple fruit by inhibiting respiration intensity, delaying weight loss, and inhibiting the decline of flesh firmness, ascorbic acid, titratable acid, and SSC (Ge et al., 2019). The TSP treatment also delayed the decrease of the content of ADP, ATP, and energy charge in apple fruit (Ge et al., 2019).
Molecular Pathways of Phosphate Signal in Rosaceae Species
Roots uptake Pi from the soil mainly by PHT1 family transporters that are located in the plasma membrane (Shin et al., 2004; Wang et al., 2020c). The PHT2-type carriers are plastid envelope-located, and the PHT3-type carriers are inner mitochondrial membrane-located (Rausch and Bucher, 2002). Trans-Golgi-located PHT4;6 proteins function as transporters that can release Pi from the Golgi apparatus (Guo et al., 2008; Hassler et al., 2012). The free phosphate is mainly stored in the plant vacuole, and the Arabidopsis VPT1 (PHT5;1) proteins have been identified as vacuolar Pi influx transporters (Liu et al., 2015). OsVPE1 and OsVPE2 are responsible for Oryza sativa vacuolar Pi efflux (Xu et al., 2019).
Phosphate starvation response 1 (PHR1) is a key transcription factor involved in Pi-starvation signaling binding to a cis-element named PHR1-binding sequence (P1BS) and significantly regulates Pi starvation-induced (PSI) genes, including Pi transporters (PHT1) and miR399 (Chiou and Lin, 2011). miR399 could suppress the expression of ubiquitin-conjugating E2 enzyme PHO2 and therefore release PHT1 and PHO1, involved in phosphate transduction from root to shoot, thus promoting Pi acquisition and transport in Arabidopsis (Chiou and Lin, 2011). However, only a few phosphate transporters and signal transduction components were reported in Rosaceae species.
A total of 37 putative phosphate transporters in apple were identified into five clusters (MdPHT1, MdPHT2, MdPHT3, MdPHT1, and MdPHT5; M. domestica), and their expression was tissue specific and significantly regulated by low P treatment (Sun et al., 2017). The overexpression of MdMYB2 could regulate the expression levels of PSI genes and further promote the assimilation and utilization of phosphate in apple (Yang et al., 2020). The levels of SUMOylation and MdSIZ1, a (SUMO) E3 ligase encoding gene, were induced by Pi-deficient conditions, suggesting that MdPHR1 and MdMYB2 might be targets for the SUMO protein (Zhang et al., 2019a).
Some important components involved in phosphate transport and signal regulation have been reported in strawberry. The expression level of FaPHO1;H9, homologous with PHO1 from Arabidopsis thaliana, was related to P content in fruits during different developmental stages and under different P fertilizer supplements (Cao et al., 2017). The overexpression of FvPHR1 and FvmiR399a in woodland strawberries significantly increased fruit quality (Wang and Wu, 2017; Wang et al., 2019b). FvPHR1 was found to directly bind to the promoter of FvmiR399a and positively regulate its expression (Wang et al., 2019b). PHR1-miR399 participates in the phosphate signaling pathway and maintains the phosphorus homeostatic state of woodland strawberry (Wang and Wu, 2017; Wang et al., 2019b). A tonoplast phosphate transporter FaVPT1 induced by Suc was identified to regulate fruit ripening and quality in strawberry (Huang et al., 2019). The overexpression of FaVPT1 in strawberry fruits promoted ripening and increased the contents of P, sugar, and anthocyanin, decreased fruit firmness, and affected the expression of ripening-related genes (Huang et al., 2019).
Appropriate Potassium Fertilizer Improves Fruit Yield, Quality, and Plant Stress Resistance
Potassium (K) affects the growth of plant and fruit yield, quality, and nutrient content of apple, strawberry, pear, sweet cherry, and almond (Kumar and Ahmed, 2014; Lu et al., 2015; Wang et al., 2018b; Zhang et al., 2018b; Shen et al., 2019; Hüseyin and Ömer, 2020; Preciado-Rangel et al., 2020). Furthermore, the application of K fertilizer at different periods may affect utilization efficiency. For example, 50% K fertilizer at post-anthesis + 50% at the expansion stage decreased the content of Ca in “Fuji” apple fruits significantly, which was detrimental to fruits postharvest storage (Lu et al., 2015). In the orchard, the best period for K fertilizer application was the basal and expansion period equally (Lu et al., 2015).
Fruits from K fertilizer application treatment had higher SSC, and the activities of sugar metabolic enzymes significantly increased in apple (Zhang et al., 2018b). The biomass and K+ content of root decreased significantly of pear seedlings was affected by K deficiency treatment (Wang et al., 2018c). Appropriate K supply promoted the transport of nutrients and sugar, by which increased the content of sugar in “Huangguan” pear fruits (Shen et al., 2019).
K fertilizer application treatment not only promotes greater fruit quality but also affects the utilization of nitrogen and the transport of other cations. The deficit or excess of K inhibited the uptake and utilization of nitrogen (N), whereas appropriate K supply could promote photosynthesis, enhance the activity of NR, and then increase the nitrogen absorption of Malus hupehensis (Tian et al., 2017; Xu et al., 2020). Ca2+ concentration increased with increasing K fertilization levels during apple fruit development (Zhang et al., 2018b). The Mg transporters increased under low K, whereas these decreased under medium and high K in pear tree, indicating that Mg had an obvious compensation effect on K, and K had an obvious antagonistic effect on Mg (Shen et al., 2019).
Elevated external K fertilization supply alleviated both biological and abiotic stresses in fruit trees (Araujo et al., 2015; Song et al., 2015a; Peng et al., 2016). K fertilization supply enhanced antioxidant defense systems, by which reducing Zn toxicity of peach seedlings (Song et al., 2015a). Increasing K content in apple trees enhanced resistance to Valsa canker, one of the most destructive diseases of apple, pathogen colonization, and establishment, so improved management of K fertilization could effectively control the disease incidence and development of Valsa canker (Peng et al., 2016). Spraying the plants with K Phi would induce the phenylpropanoid pathway of mango plants to reduce internal necrosis and the disease development of Ceratocystis fimbriata (Araujo et al., 2015).
Molecular Pathways of K Transporters and Channels in Rosaceae Species
K+ usability, uptake efficiency, and transfer efficiency remarkably affect the performance of plants. In order to maintain optimal K+ homeostasis, more than 71 K+ transporters and channels, including three channel families and three transporter families, have already been identified in A. thaliana (Wang and Wu, 2017; Wang et al., 2020c). They are involved in K+ absorption by roots, ion transport between organs and tissues, and K+ storage in vacuoles (Gierth and Mäser, 2007; Chanroj et al., 2012; Gomez-Porras et al., 2012).
Selective K+ channels contain three families: Shaker, TPK, and Kir-like. The Shaker K+ channels display six transmembrane segments (TMS). TPK channels (Tandem-Pore K+ Channels) display a hydrophobic core composed of four TMS. Plant Kir-like channels are associated with animal Kir (K+ inward rectifier) channels, displaying two TMS. There are three different families of plasma membrane K+ transport systems: the HAK/KUP/KT K+ transporters (Gierth and Mäser, 2007), the HKT high-affinity K+ transporters (Rodríguez-Navarro and Rubio, 2006), and the CPA2 subfamily, including K+ efflux (KEA) H+/K+ antiporters and the cation/H+ exchanger (Mäser et al., 2001).
Some K+ channels and transporters have been reported, but there is far less information in Rosaceae species. Thirty-six Shaker K+ channel genes from Rosaceae species were divided into five subgroups, including a K+ channel protein PbrKAT1 that may play a role in regulating salt tolerance of pear, because its activity was inhibited by external sodium ions (Chen et al., 2019a). Similarly, a strawberry K+ channel, FaKAT1, serves as a positive regulator in the regulation of fruit ripening in an ABA-dependent manner (Song et al., 2017). K+ channel AKT1 is activated and phosphorylated by calcineurin B-like protein (CBL)-interacting protein kinases (CIPKs; Xu et al., 2006). FaAKT1 encoded a highly K+-selective channel and showed upregulated expression in strawberry “Camarosa” upon salt stress (Garriga et al., 2017). The overexpression of FaTPK1, a strawberry tonoplast K channel, promoted the expression of fruit ripening-related genes and the contents of anthocyanin, soluble sugars, and ABA (Wang et al., 2018c). FaTPK1 could not only regulate fruit ripening and quality formation but also enhance fruit resistance to Botrytis cinerea (Wang et al., 2018c).
Sixteen KT/HAK/KUP family K transporters in peach (Prunus persica) were identified and highly expressed in fruit and flower, indicating that these transporters may play important roles in K+ uptake and transport as well as fruit development in peach (Song et al., 2015b). PbrHAK1 and PbrHAK12/16 were obviously increased under K+ deficiency, suggesting their significant roles in K+ uptake of pear, especially in response to K+ starvation (Wang et al., 2018d). HKT genes and CPA genes were identified in five Rosaceae species, involving Fragaria vesca, Pyrus communis, M. domestica, P. persica, and Prunus mume, and HKT genes of woodland strawberry were responding to the salt stress (Zhang et al., 2019b). FaHKT1 could transport Na+ selectively, and the increased expression of FaHKT1 in roots correlates with the higher tolerance to salinity of the strawberry genotype (Garriga et al., 2017). CPA genes of pear were mainly expressed in pollen tubes and fruits, which suggested that CPAs may play significant roles in the growth of pollen tubes (Zhou et al., 2016).
Signal network of K regulating fruit enlargement and ripening was studied in “Huangguan” pear (Shen et al., 2017). K contents in the pear leaves and fruits were significantly reduced under low-K treatment. According to the transcriptome sequencing data, AKT1 and HAK/KUP/KT genes may play a vital role on K+ transport in leaves and fruits under K deficiency. High K level could promote photosynthesis and modify the distribution of the carbohydrate and nutrient from leaves to fruits, whereas carbohydrate metabolism was inhibited by low K during maturation. Two Suc synthase (SUS) genes were remarkably downregulated in fruits, and this change may be the reason for the decrease Suc concentration of leaves and fruit under low K. Genes associated with ethylene, cytokinin (CK), jasmonic acid (JA), auxin, and ABA were induced by low K, but the genes encoding transporters of auxin, CK, and brassinosteroid were significantly downregulated under low-K treatment in leaves and fruit (Shen et al., 2017).
Studying the signal network of different K applications on plant growth and fruit development and the molecular mechanism of K application on improving fruit quality can provide scientific basis for improving fruit quality by reasonable fertilizer.
Calcium Fertilizer Regulates Fruit Ripening and Postharvest Quality
Calcium (Ca) is considered to be one of the most important mineral elements to determine fruit quality, and many research studies focus on the function of Ca. Studies have confirmed that Ca has a central role in cell wall interactions, plant signaling, and water relations (Hocking et al., 2016). Since Ca is an important part of cell wall structure, it can affect the integrity of the cell membrane as well as affect a key role in membrane function (Fallahi et al., 1997).
Localized Ca deficiencies observed in particular species or varieties can lead to leakage of membranes, irregular softening of the cell walls, and abnormal development of fruit. It is reported that high concentrations of Ca inhibited ethylene production in apple slices, by delaying the conversion of 1-aminocyclopropane-l-carboxylic acid (ACC) to ethylene (Lieberman and Wang, 1982; Han et al., 2020). The de-esterification of pectin and Ca crosslinking resulted in the changes of the physical properties of the cell wall, which eventually lead to fruit softening (Hocking et al., 2016).
Ca deficiencies in fruits may also cause pathological and physiological disorders (Hocking et al., 2016). Ca2+ deficiency contributed to the occurrence of skin browning spot, which is an important physiology disorder in Huangguan (P. bretschneideri × Pyrus pyrifolia) pear fruit (Dong et al., 2015). The Ca2+ imbalance of pear fruit would lead to the development of hard end disorder (Wang et al., 2018e). Calcium application during sweet cherry development and ripening caused a reduction in respiration rate and water-induced fruit cracking, which was accompanied by numerous changes in the polar/non-polar primary and secondary metabolites according to the metabolome profiling (Michailidis et al., 2020).
Abnormal Ca content, partitioning, and distribution are the major factors related to bitter-pit (BP; De Freitas et al., 2015). Almost all factors that influence BP incidence in preharvest fruits could be directly or indirectly associated with Ca content in apple fruit (Ferguson et al., 1999). It is studied that the main reason for the decrease of BP of apple (M. domestica) is the change of K/Ca ratio (Guerra and Casquero, 2010). Ca-containing fertilizers, such as CaCl2, Ca(NO3)2, or Ca(HCOO)2, were applied in preharvest apple, in order to improve fruit quality, because as the Ca content increased, the BP was reduced (Yu et al., 2018).
Ca-containing substances are widely used to maintain the quality of fruit after harvest. Treatment with CaCl2 or ultrasound and calcium (U + Ca) boosted fruit total phenolics content in strawberry by stimulating the genes expression involving anthocyanin structure (Xu et al., 2014), preventing the decrease in firmness of strawberries to maintain better fruit quality (Zhang et al., 2018a). Sprays of CaCl2 and Ca(NO3)2 effectively reduced respiration rate, spoilage, and physiological weight loss, but maintained fruit firmness, palatability, acidity, and pectin methylesterase (PME) activity during storage of peach, apple, apricot, sweet berry, and plum fruits, which helped fruits to be stored longer with acceptable edible quality (Gupta, 2011; Mauro et al., 2016; Liu et al., 2017; Michailidis et al., 2019; Sinha et al., 2019; Han et al., 2020). The combination of calcium propionate (CP) and sodium chlorite (SC) could increase the firmness of apple slices and inhibited apple browning and the growth of Escherichia coli and yeast on fresh-cut apple slices (Guan and Fan, 2010). However, it is recently reported that postharvest calcium dipping, including CaCl2 and Ca(NO3)2 treatment, in apples caused an increase in lenticel breakdown, the damage during storage appearing as discrete tissue deterioration surrounding the lenticels (Singh et al., 2016, 2021).
Ca nutrition also increases plant resistance to both biological and abiotic stresses. The supplement of Ca2+ could restrict the growth of fungal pathogen B. cinerea in strawberry (Langer et al., 2019). In addition, Ca2+ was reported to enhance resistance to Botryosphaeria dothidea, which is one of the most serious diseases for pear, by increasing autophagic activity and salicylic acid (SA) accumulation (Sun et al., 2020b). Transcriptome and proteome results showed that the application of Ca alleviated the temperature response of apple tree and salinity stress response of pear plants (Xu et al., 2015; Li et al., 2017).
Molecular Pathways of Ca in Rosaceae Species
Ca2+ is a ubiquitous second messenger related to plant cell signaling processes and enables the development as well as biotic and abiotic stress responses in plants. The change of the intracellular free Ca2+ content is one of the earliest events during perceiving changes of plants in the environment. Ca2+ sensors calmodulin (CaM), Ca2+-dependent protein kinases (CDPKs), calmodulin-like proteins (CMLs), and CBLs play important roles in the process that convert Ca2+ signals into appropriate responses (Aldon et al., 2018). In addition to the components of the classical Ca signaling pathway, FaAnn5s and FaAnn8, plant Ca2+-binding proteins, were downstream of Ca signaling during strawberry fruit ripening (Chen et al., 2016). Recently, Ca2+/H+ exchangers MdCAX11 and MdCAX5, tonoplast located Ca2+ transport proteins, were suggested to mediate the influx of Ca from the cytosol into vacuoles, which may be related to the occurrence of BP in apple fruit, because their expression increased as the severity of BP increased (Liu et al., 2021).
A total of 4 MdCaM and 58 MdCML genes were identified in apple (Malus × domestica), and MdCaMs/MdCMLs were expressed in shoots, roots, mature leaves, flowers, and fruits (Li et al., 2019). The overexpression of MdCML3 observably improved the salt tolerance of apple callus (Li et al., 2019). FvCaM and FvCML genes in strawberry were identified (Zhang et al., 2016). The overexpression of four FvCaMs and FvCMLs could enhance the resistance of Nicotiana benthamiana leaves to Agrobacterium tumefaciens (Zhang et al., 2016). PbCBL1 gene, from the birch-leaf pear, is induced by gibberellic acid (GA), ABA, SA, methyl jasmonate (MeJA), and several abiotic stresses (Xu et al., 2015).
In five Rosaceae species, involving strawberry, apple, peach, pear, and plum, a total of 123 CPK genes were identified (Wei et al., 2016). Eleven out of 30 MdCPKs were regulated by pathogen infection (Kanchiswamy et al., 2013). The overexpression of MdCIPK6L could improve the salt tolerance in transgenic Arabidopsis and tomatoes (Wang et al., 2012). The expression of FaCDPK affected strawberry fruit development and ripening and also responded to salt and drought stresses, as well as ABA treatment (Crizel et al., 2020).
Transcriptome and proteome were used to determine the changes after calcium treatment during fruit development and postharvest senescence in sweet cherry (Michailidis et al., 2019, 2020). The expression of several genes involved in tricarboxylic acid (TCA) cycle, pectin degradation, as well as amino acids and sugar metabolism was affected by Ca treatment in sweet cherry (Michailidis et al., 2019). Ca specifically increased the amounts of proteins that were classified as oxidoreductases, transferases, hydrolases, lyases, and ligases during postharvest storage (Michailidis et al., 2020).
Iron Fertilizer Improves Photosynthesis, Fruit Yield, and Quality
Iron (Fe) deficiency not only reduced biomass, chlorophyll, and photosynthesis but also increased oxidative stress response and leaf chlorosis in both peach and strawberry (Eichert et al., 2010; Gama et al., 2016; Kaya and Ashraf, 2019). However, other effects of Fe deficiency on the growth, fruit quality, and yield of different Rosaceae species were varied.
In peach, Fe deficiency led to decreased fruit production and fruit quality, because of the lower total sugar/total organic acid ratios and the less anthocyanin accumulation, and to a slight improvement of phenolic compounds and vitamin C (Alvarez-Fernández et al., 2003). It not only induced leaf chlorosis in peach but also reduced WUE, because normal stomatal functioning was disturbed in chlorotic leaves (Eichert et al., 2010).
In strawberry, deficiency of Fe resulted in a lower N assimilation, photosynthesis, and antioxidant activity, while higher amounts of bioactive compounds, including phenols and anthocyanin (Valentinuzzi et al., 2015a,b). It was shown that increased nitric oxide (NO) in the root system, root ferrous uptake activity, and indole-3-acetic acid (IAA) content in the shoot apex may enhance tolerance to Fe deficiency in Malus xiaojinensis and strawberry (Wu et al., 2012; Zha et al., 2014; Zhai et al., 2016; Kaya et al., 2019).
To improve the tolerance to Fe deficiency in Rosaceae plants, many measures have been taken. Studies have shown that exogenous Suc could enhance tolerance to Fe deficiency through regulating chlorophyll biosynthesis in Malus halliana (Guo et al., 2020). Additionally, foliar spraying with amino acid-Fe compound fertilizer remarkably increased fresh weights, Fe accumulation, total chlorophyll content, photosynthesis, and stomatal conductance of leaves in peach (P. persica L. Batsch; Sheng et al., 2020).
Pre-treatments with 24-epibrassinolide (EB) improved Fe deficiency tolerance via improving Fe2+ and antioxidant enzyme activities in leaves, thus causing an elevation in NR in strawberry (Kaya et al., 2020). The NO donor, sodium nitroprusside (SNP), restored Fe deficiency response in M. xiaojinensis (Zhai et al., 2016), and exogenously applied NO effectively reduced electrolyte leakage (EL), malondialdehyde (MDA), and H2O2 of seedlings in strawberry (Fragaria × ananassa cv. Camarosa) suffering Fe deficiency (Kaya et al., 2019).
The symptoms of Fe deficiency, such as lower chlorophyll contents in young leaves, were decreased by application of sodium hydrosulfide (NaHS), as it could improve endogenous H2S and antioxidant enzyme activities, as well as reduce the H2O2 and MDA generation under Fe deficiency, eventually enhancing the uptake and activation of Fe (Kaya and Ashraf, 2019).
Molecular Pathways of Fe in Rosaceae Species
Fe is a crucial element in plants, and Fe deficiency would lead to common nutritional disorders. Fe deficiency limits the yield of fruit trees, reducing fruit quality, particularly for the pear trees growing in calcareous soil (Donnini et al., 2011). When subjected to Fe deficiency, a series of adaptive responses in plants is induced. For example, H+ secretion of the rhizosphere increased and led to soil acidification, promoting the conversion from Fe3+ to Fe2+, which allowed the apple tree to absorb Fe better (Zhang et al., 2020). Currently, the signal mechanism of Rosaceae responding to different Fe supplies is mainly studied in Malus plants.
The uptake and transport of Fe mainly depend on transporters. Fe-regulated transporter 1 (Mx IRT1), an Fe transporter that was highly effectively inducible in M. xiaojinensis, could rescue the phenotype of Arabidopsis irt1 mutant and restore the growth defect of Fe-limit yeast mutant DEY1453 (fet3fet4; Zhang et al., 2014). In genus Malus, a mutant allele of IRT1 showed increased expression of IRT1, which allows apple to adapt to Fe deficiency (Zhang et al., 2017). In apple, 18 Fe-regulated transporter-like protein (ZIP) family genes were identified (Ma et al., 2019). MdZIP10 could not only rescue the growth of Fe2+ uptake defective yeast mutants but also increase the Fe content and alleviate Fe deficiency symptoms by inducing the expression of Fe uptake and transport-related genes in MdZIP10 overexpression A. thaliana transgenic plants (Ma et al., 2019).
Transcription factors play important roles in Rosaceae species’ responses to Fe deficiency. In the case of Fe deficiency, MxIRO2, a novel basic helix-loop-helix Fe-related transcription factor, was upregulated in M. xiaojinensis leaves and roots (Yin et al., 2013). MdbHLH104 was identified to play a key role in toleration to Fe deficiency in both transgenic apple plants and callus by directly regulating the expression of MdAHA8 to increase the plasma membrane (PM) H (+)-ATPase activity and Fe uptake (Zhao et al., 2016a). Additionally, MdbHLH104 was degraded by MdBT2 and MdSIZ1 (a SIZ/PIAS-type SUMO E3 ligase) dependent SUMOylation and ubiquitination through the 26S proteasome pathway in apple (M. domestica; Zhao et al., 2016b; Zhou et al., 2019).
Fe deficiency response transcription factor MxFIT was induced in roots during Fe deficiency, and the overexpression of MxFIT in Arabidopsis enhanced toleration to Fe deficiency, indicating that it may play roles in Fe uptake and Fe deficiency response (Yin et al., 2014). In addition, ethylene response factor MxERF4 could inhibit MxIRT1 expression via binding to its promoter in M. xiaojinensis, which suggested that ethylene regulated the Fe deficiency response via MxERF4-related Fe acquisition (Liu et al., 2018). MbERF4 and MbERF72 were highly expressed in Fe deficiency-sensitive Malus baccata, whereas they were lowly expressed in Fe deficiency-tolerant M. xiaojinensis, and they repressed the expression of MbHA2 directly, thus increasing the rhizosphere pH in response to Fe deficiency in M. baccata (Zhang et al., 2020).
With overexpressing MdMYB58, Fe deficiency-inducible MYB transcription factor, both apple calli and transgenic Arabidopsis could accumulate Fe under low Fe stress (Wang et al., 2018a). MdMYB58 directly repressed the expression of MdMATE43 and MdFRD3 in Arabidopsis, but it was competitively attenuated by MdSAT1, a member of bHLH transcription factors, through protein-protein interaction (Wang et al., 2018a). Under both deficient and normal Fe conditions, the content of Fe in transgenic Arabidopsis expressing MxMYB1 was lower than that of wild type, that may be because of the lower expression of Fe transporter AtIRT1 and an Fe storage protein ferritin AtFER1 in plants (Shen et al., 2008).
Besides the transport proteins and transcription factors, MxNRAMP1 (natural resistance-associated macrophage protein), MxNas1 (nicotianamine synthase), and lncRNA MSTRG.85814.11 from M. xiaojinensis and M. domestica play important roles in Fe deficiency (Pan et al., 2015; Sun et al., 2018a, 2020a). MxNRAMP1 overexpression could accelerate Fe absorption and accumulation and increase the resistance of plants against Fe deficiency stress (Pan et al., 2015). MxNAS1 overexpression in transgenic tobacco cells could increase the content of active Fe and Na under Fe sufficiency, while related to the redistribution of Fe in M. xiaojinensis under Fe deficiency (Sun et al., 2018a). lncRNA MSTRG.85814.11 positively modulated the small auxin upregulated gene SAUR32 and increased the expression of AHA10, a plasma membrane proton ATPase, which activated proton extrusion involved in response to Fe deficiency (Sun et al., 2020a).
M. halliana, which is an apple rootstock with Fe deficiency resistance, is used to evaluate short-term molecular response under Fe deficiency treatment by RNA sequencing (RNA-Seq) analyses (Hu et al., 2018; Wang et al., 2018f). Fe deficiency induced genes involved in photosynthesis, pigment regulation, and glycolysis pathways, which suggested that Fe deficiency can also affect the synthesis and metabolism of sugar in the M. halliana leaves and roots (Hu et al., 2018; Wang et al., 2018f).
Conclusion and Future Perspectives
In this review, we focused on the effect of mineral nutrition on Rosaceae species’ fruit yield, quality, and postharvest storage and, on the one hand, on the mechanisms by which Rosaceae absorbed, utilized, and transmitted nutritional signals. In conclusion, nitrogen supply mainly affects fruit yield, anthocyanin synthesis, and chlorophyll degradation in the fruit. Phosphorus (P) supplementation affects the production of SSC and secondary metabolites, such as flavonoids and ascorbic acid. Potassium (K) not only affects fruit quality but also induces both biological and abiotic stress tolerance in fruit trees. Calcium (Ca) deficiency is a major factor associated with fruit softening and BP. Iron (Fe) deficiency reduced biomass and photosynthesis, whereas it increased oxidative stress response and the activities of antioxidant enzymes. Many important transport proteins, transcription factors, and other regulators were reported and shown in Figure 1. However, there are still many significant questions to be investigated in the future.
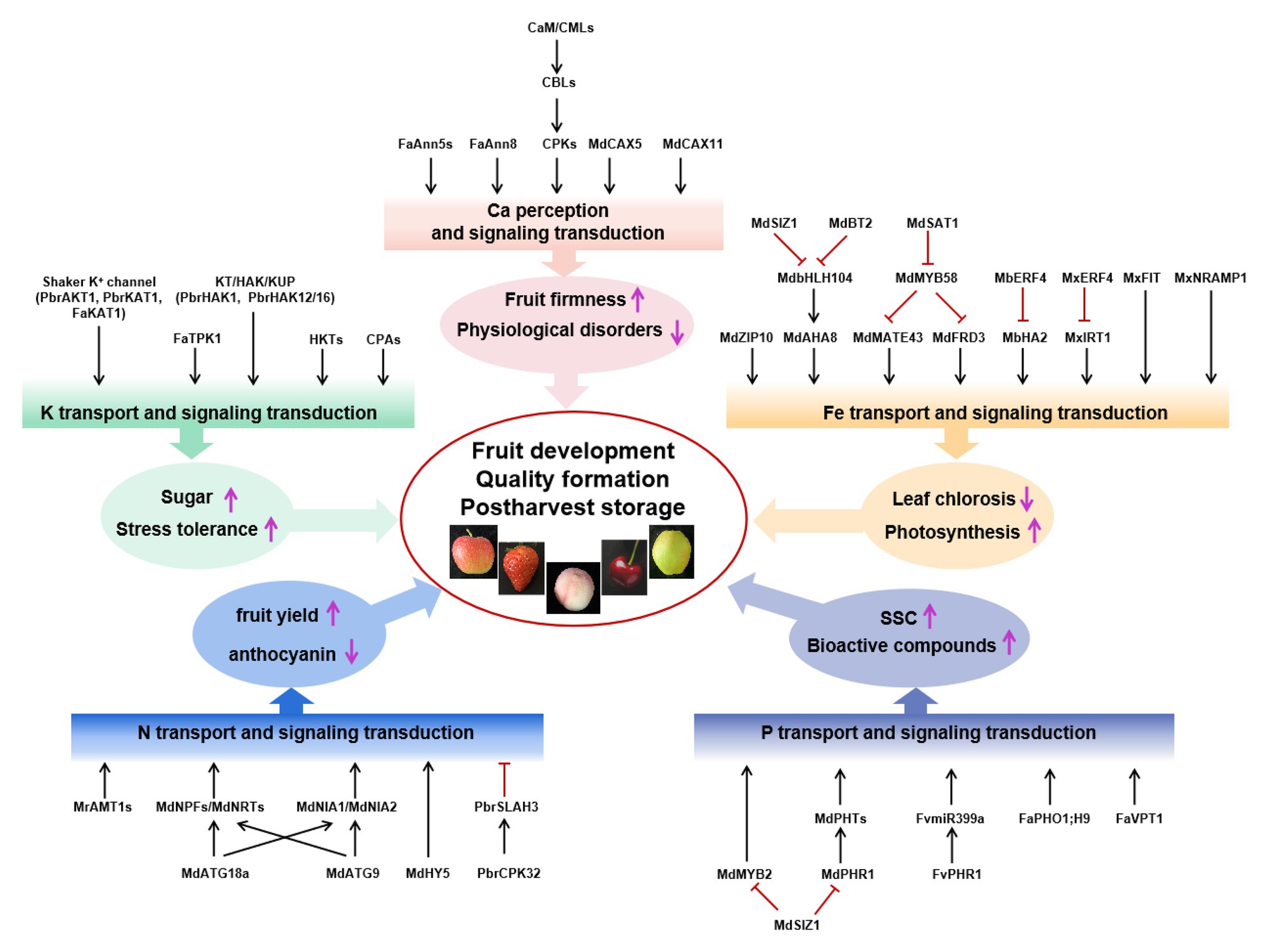
Figure 1. Signal components involved in the regulation of fruit yield, quality, and postharvest storage by N, P, K, Ca, and Fe. Mineral nutrients nitrogen (N; blue box), phosphorus (P; purple box), potassium (K; green box), calcium (Ca; pink box), and iron (Fe; orange box) regulate fruit development, quality formation, and postharvest storage. Black arrows represent positive interactions or regulation, whereas red bars represent repression. Ammonium transporter AMT1 in the apple rootstock Malus robusta Rehd, anion channel PbrSLAH3 of pear, nitrate transporter 1/peptide transporter family, NR MdNIA1/MdNIA2, and autophagy protein MdATG18a/MdATG9 from Malus domestica are identified in N transport or signal transduction, which mainly affect fruit yield and anthocyanin synthesis. Phosphate transporters SUMO E3 ligase MdSIZ1, MdPHR1, and MdMYB2 in apple and FaPHO1;H9, FaVPT1, FvPHR1, and FvmiR399a in strawberry are identified to change the content of soluble solid (SSC) and bioactive compounds. Shaker K+ channel, KT/HAK/KUP family K transporters, HKT and CPA transport proteins in five Rosaceae species, and FaKAT1 and FaTPK1 in strawberry were identified, improving sugar content and stress tolerance. MdCaM, MdCML, MdCAX5, and MdCAX11 in apple; FvCaMs, FvCMLs, FaAnn5s, and FaAnn8 in strawberry; PbCBL1 from the birch-leaf pear; and CPK family from five Rosaceae species are identified to be associated with regulating fruit firmness, keeping plants from physiological disorders, and improving sugar content and stress tolerance. Iron-regulated transporters (MxIRT1 and MdZIP), transcription factors (MxIRO2, MdbHLH104, MbERF72, MxFIT, MxERF4, MdSAT1, MdMYB58, and MxMYB1), E3 ligases (MdBT2 and MdSIZ1), and MxNRAMP1 are identified in Fe transport or signal transduction, which promoted photosynthesis and prevented leaf chlorosis.
First of all, more comprehensive understanding of the uptake and translocation system in Rosaceae species for mineral elements is required. Compared with the model plant Arabidopsis and crops, the transport and regulation system of mineral elements in Rosaceae species are far from fully understood. Although some transporters have been identified through bioinformatics, their function should be verified through more physiological and molecular experiments in the future.
Second, as the change of fertilization of some mineral nutrient elements will affect the absorption and utilization of other elements and the plants would communicate with rhizosphere microorganisms to improve the absorption and utilization of nutrients, we should pay more attention to the molecular mechanisms of the interactions between different nutrients or plants and microbes and take advantage of them.
Third, as it is difficult for the public to accept transgenic plants, especially transgenic fruits, we need to find more natural variations and refine the gene editing techniques to apply in Rosaceae, in order to improve fruit yield and quality.
Author Contributions
YH, YS, and QB wrote the review. QB collected the references. YH designed the model and revised the review. All authors contributed to the article and approved the submitted version.
Funding
This study was supported by the National Natural Science Foundation of China (projects 32030100; 31672125), the Scientific Research Project of Beijing Educational Committee (KM202110020006), the Opening Project of Beijing Key Laboratory of New Technology in Agricultural Application (kf2020021), and the Sichuan Lomon Biotechnology Co., Ltd. (2018001).
Conflict of Interest
The authors declare that the research was conducted in the absence of any commercial or financial relationships that could be construed as a potential conflict of interest.
Acknowledgments
We are very grateful to Prof. Chen Yi-Fang (China Agricultural University) for her advice on the role of P in fruit ripening.
References
Afroz, C. A., Shimul, M. A. H., Ikrum, M., Siddiky, M. A., and Razzaque, M. A. (2016). Effects of nitrogen phosphorus potassium and sulphur on growth yield and nutrient content of strawberry (Fragaria ananassa). J. Environ. Sci. Nat. Resour. 9, 99–108. doi: 10.3329/jesnr.v9i1.30300
Aldon, D., Mbengue, M., Mazars, C., and Galaud, J. P. (2018). Calcium signalling in plant biotic interactions. Int. J. Mol. Sci. 19:665. doi: 10.3390/ijms19030665
Alvarez-Fernández, A., Paniagua, P., Abadía, J., and Abadía, A. (2003). Effects of Fe deficiency chlorosis on yield and fruit quality in peach (Prunus persica L. Batsch). J. Agric. Food Chem. 51, 5738–5744. doi: 10.1021/jf034402c
An, J. P., Qu, F. J., Yao, J. F., Wang, X. N., You, C. X., Wang, X. F., et al. (2017). The bZIP transcription factor MdHY5 regulates anthocyanin accumulation and nitrate assimilation in apple. Hortic. Res. 4, 1–9. doi: 10.1038/hortres.2017.23
Araujo, L., Bispo, W. S., Rios, V. S., Fernandes, S. A., and Rodrigues, F. A. (2015). Induction of the phenylpropanoid pathway by acibenzolar-S-methyl and potassium phosphite increases mango resistance to Ceratocystis fimbriata infection. Plant Dis. 99, 447–459. doi: 10.1094/PDIS-08-14-0788-RE
Cao, F., Guan, C., Dai, H., Li, X., and Zhang, Z. (2015). Soluble solids content is positively correlated with phosphorus content in ripening strawberry fruits. Sci. Hortic. 195, 183–187. doi: 10.1016/j.scienta.2015.09.018
Cao, F., Li, H., Wang, S., Li, X., Dai, H., and Zhang, Z. (2017). Expression and functional analysis of FaPHO1;H9 gene of strawberry (Fragaria × ananassa). J. Integr. Agric. 16, 580–590. doi: 10.1016/S2095-3119(16)61433-8
Cardeñosa, V., Medrano, E., Lorenzo, P., Sánchez-Guerrero, M. C., Cuevas, F., Pradas, I., et al. (2015). Effects of salinity and nitrogen supply on the quality and health-related compounds of strawberry fruits (Fragaria × ananassa cv. Primoris). J. Sci. Food Agric. 95, 2924–2930. doi: 10.1002/jsfa.7034
Chai, X., Xie, L., Wang, X., Wang, H., Zhang, J., Han, Z., et al. (2020). Apple rootstocks with different phosphorus efficiency exhibit alterations in rhizosphere bacterial structure. J. Appl. Microbiol. 128, 1460–1471. doi: 10.1111/jam.14547
Chanroj, S., Wang, G., Venema, K., Zhang, M. W., Delwiche, C. F., and Sze, H. (2012). Conserved and diversified gene families of monovalent cation/H+ antiporters from algae to flowering plants. Front. Plant Sci. 3:25. doi: 10.3389/fpls.2012.00025
Chen, G., Chen, Q., Qi, K., Xie, Z., Yin, H., Wang, P., et al. (2019a). Identification of shaker K+ channel family members in Rosaceae and a functional exploration of PbrKAT1. Planta 250, 1911–1925. doi: 10.1007/s00425-019-03275-3
Chen, J., Mao, L., Mi, H., Lu, W., Ying, T., and Luo, Z. (2016). Involvement of three annexin genes in the ripening of strawberry fruit regulated by phytohormone and calcium signal transduction. Plant Cell Rep. 35, 733–743. doi: 10.1007/s00299-015-1915-5
Chen, G., Wang, L., Chen, Q., Qi, K., Yin, H., Cao, P., et al. (2019b). PbrSLAH3 is a nitrate-selective anion channel which is modulated by calcium-dependent protein kinase 32 in pear. BMC Plant Biol. 19:190. doi: 10.1186/s12870-019-1813-z
Cheng, L., Ma, F., and Ranwala, D. (2004). Nitrogen storage and its interaction with carbohydrates of young apple trees in response to nitrogen supply. Tree Physiol. 24, 91–98. doi: 10.1093/treephys/24.1.91
Chiou, T. J., and Lin, S. I. (2011). Signaling network in sensing phosphate availability in plants. Annu. Rev. Plant Biol. 62, 185–206. doi: 10.1146/annurev-arplant-042110-103849
Cockerton, H. M., Li, B., Stavridou, E., Johnson, A., Karlström, A., Armitage, A. D., et al. (2020). Genetic and phenotypic associations between root architecture, arbuscular mycorrhizal fungi colonisation and low phosphate tolerance in strawberry (Fragaria × ananassa). BMC Plant Biol. 20:154. doi: 10.1186/s12870-020-02347-x
Crizel, R. L., Perin, E. C., Vighi, I. L., Woloski, R., Seixas, A., da Silva Pinto, L., et al. (2020). Genome-wide identification, and characterization of the CDPK gene family reveal their involvement in abiotic stress response in Fragaria x ananassa. Sci. Rep. 10:11040. doi: 10.1038/s41598-020-67957-9
De Freitas, S. T., do Amarante, C. V. T., and Mitcham, E. J. (2015). Mechanisms regulating apple cultivar susceptibility to bitter pit. Sci. Hortic. 186, 54–60. doi: 10.1016/j.scienta.2015.01.039
Dong, Y., Guan, J. F., Ma, S. J., Liu, L. L., Feng, Y. X., and Cheng, Y. D. (2015). Calcium content and its correlated distribution with skin browning spot in bagged Huangguan pear. Protoplasma 252, 165–171. doi: 10.1007/s00709-014-0665-5
Donnini, S., Dell'Orto, M., and Zocchi, G. (2011). Oxidative stress responses and root lignification induced by Fe deficiency conditions in pear and quince genotypes. Tree Physiol. 31, 102–113. doi: 10.1093/treephys/tpq105
Eichert, T., Peguero-Pina, J. J., Gil-Pelegrín, E., Heredia, A., and Fernández, V. (2010). Effects of iron chlorosis and iron resupply on leaf xylem architecture, water relations, gas exchange and stomatal performance of field-grown peach (Prunus persica). Physiol. Plant. 138, 48–59. doi: 10.1111/j.1399-3054.2009.01295.x
Estrada-Ortiz, E., Trejo-Tellez, L. I., Gomez-Merino, F. C., Nunez-Escobar, R., and Sandoval-Villa, M. (2013). The effects of phosphite on strawberry yield and fruit quality. J. Soil Sci. Plant Nutr. 13, 612–620. doi: 10.4067/S0718-95162013005000049
Ezawa, T., and Saito, K. (2018). How do arbuscular mycorrhizal fungi handle phosphate? New insight into fine-tuning of phosphate metabolism. New Phytol. 220, 1116–1121. doi: 10.1111/nph.15187
Falchi, R., Bonghi, C., Drincovich, M. F., Famiani, F., Lara, M. V., Walker, R. P., et al. (2020). Sugar metabolism in stone fruit: source-sink relationships and environmental and agronomical effects. Front. Plant Sci. 11:573982. doi: 10.3389/fpls.2020.573982
Fallahi, E., Conway, W. S., Hickey, K. D., and Sams, C. E. (1997). The role of calcium and nitrogen in postharvest quality and disease resistance of apples. Hortic. Sci. 32, 831–835. doi: 10.1023/A:1008607100742
Ferguson, I., Volz, R., and Woolf, A. (1999). Preharvest factors affecting physiological disorders of fruit. Postharvest Biol. Technol. 15, 255–262. doi: 10.1016/S0925-5214(98)00089-1
Gama, F., Saavedra, T., Da Silva, J. P., Miguel, M. G., de Varennes, A., Correia, P. J., et al. (2016). The memory of iron stress in strawberry plants. Plant Physiol. Biochem. 104, 36–44. doi: 10.1016/j.plaphy.2016.03.019
Garriga, M., Raddatz, N., Véry, A. A., Sentenac, H., Rubio-Meléndez, M. E., González, W., et al. (2017). Cloning and functional characterization of HKT1 and AKT1 genes of Fragaria spp.-relationship to plant response to salt stress. J. Plant Physiol. 210, 9–17. doi: 10.1016/j.jplph.2016.12.007
Ge, Y., Chen, Y., Li, C., Wei, M., Li, X., Li, S., et al. (2019). Effect of trisodium phosphate dipping treatment on the quality and energy metabolism of apples. Food Chem. 274, 324–329. doi: 10.1016/j.foodchem.2018.08.142
Gierth, M., and Mäser, P. (2007). Potassium transporters in plants--involvement in K+ acquisition, redistribution and homeostasis. FEBS Lett. 581, 2348–2356. doi: 10.1016/j.febslet.2007.03.035
Gomez-Porras, J. L., Riaño Pachón, D. M., Benito, B., Haro, R., Sklodowski, K., Rodríguez-Navarro, A., et al. (2012). Phylogenetic analysis of k(+) transporters in bryophytes, lycophytes, and flowering plants indicates a specialization of vascular plants. Front. Plant Sci. 3:167. doi: 10.3389/fpls.2012.00167
Guan, W., and Fan, X. (2010). Combination of sodium chlorite and calcium propionate reduces enzymatic browning and microbial population of fresh-cut "Granny Smith" apples. J. Food Sci. 75, M72–M77. doi: 10.1111/j.1750-3841.2009.01470.x
Guerra, M., and Casquero, P. A. (2010). Summer pruning: an ecological alternative to postharvest calcium treatment to improve storability of high quality apple cv. 'Reinette du Canada'. Food Sci. Technol. Int. 16, 343–350. doi: 10.1177/1082013210366977
Guo, A., Hu, Y., Shi, M., Wang, H., Wu, Y., and Wang, Y. (2020). Effects of iron deficiency and exogenous sucrose on the intermediates of chlorophyll biosynthesis in Malus halliana. PLoS One 15:e0232694. doi: 10.1371/journal.pone.0232694
Guo, B., Jin, Y., Wussler, C., Blancaflor, E. B., Motes, C. M., and Versaw, W. K. (2008). Functional analysis of the Arabidopsis PHT4 family of intracellular phosphate transporters. New Phytol. 177, 889–898. doi: 10.1111/j.1469-8137.2007.02331.x
Gupta, S. (2011). An in vitro study of Diffusibility and degradation of three calcium hydroxide pastes. Int. J. Clin. Pediatr. Dent. 4, 15–23. doi: 10.5005/jp-journals-10005-1075
Han, S., Liu, H., Han, Y., He, Y., Nan, Y., Qu, W., et al. (2020). Effects of calcium treatment on malate metabolism and γ-aminobutyric acid (GABA) pathway in postharvest apple fruit. Food Chem. 334:127479. doi: 10.1016/j.foodchem.2020.127479
Hassler, S., Lemke, L., Jung, B., Möhlmann, T., Krüger, F., Schumacher, K., et al. (2012). Lack of the Golgi phosphate transporter PHT4;6 causes strong developmental defects, constitutively activated disease resistance mechanisms and altered intracellular phosphate compartmentation in Arabidopsis. Plant J. 72, 732–744. doi: 10.1111/j.1365-313X.2012.05106.x
Hocking, B., Tyerman, S. D., Burton, R. A., and Gilliham, M. (2016). Fruit calcium: transport and physiology. Front. Plant Sci. 29:569. doi: 10.3389/fpls.2016.00569
Hu, Y., Zhu, Y. F., Guo, A. X., Jia, X. M., Cheng, L., Zhao, T., et al. (2018). Transcriptome analysis in Malus halliana roots in response to iron deficiency reveals insight into sugar regulation. Mol. Gen. Genomics. 293, 1523–1534. doi: 10.1007/s00438-018-1479-5
Huang, Y., Xu, P. H., Hou, B. Z., and Shen, Y. Y. (2019). Strawberry tonoplast transporter, FaVPT1, controls phosphate accumulation and fruit quality. Plant Cell Environ. 42, 2715–2729. doi: 10.1111/pce.13598
Huo, L., Guo, Z., Zhang, Z., Jia, X., Sun, Y., Sun, X., et al. (2020). The apple autophagy-related gene MdATG9 confers tolerance to low nitrogen in transgenic apple callus. Front. Plant Sci. 11:423. doi: 10.3389/fpls.2020.00423
Hüseyin, Y., and Ömer, A. (2020). Effects of potassium fertilization on leaf nutrient content and quality attributes of sweet cherry fruits (Prunus avium L.). J. Plant Nutr. 1, 1–12. doi: 10.1080/01904167.2020.1862203
Jezek, M., Zörb, C., Merkt, N., and Geilfus, C. M. (2018). Anthocyanin Management in Fruits by fertilization. J. Agric. Food Chem. 66, 753–764. doi: 10.1021/acs.jafc.7b03813
Kanchiswamy, C. N., Mohanta, T. K., Capuzzo, A., Occhipinti, A., Verrillo, F., Maffei, M. E., et al. (2013). Differential expression of CPKs and cytosolic Ca2+ variation in resistant and susceptible apple cultivars (Malus × domestica) in response to the pathogen Erwinia amylovora and mechanical wounding. BMC Genomics 14:760. doi: 10.1186/1471-2164-14-760
Kaya, C., Akram, N. A., and Ashraf, M. (2019). Influence of exogenously applied nitric oxide on strawberry (Fragaria × ananassa) plants grown under iron deficiency and/or saline stress. Physiol. Plant. 165, 247–263. doi: 10.1111/ppl.12818
Kaya, C., and Ashraf, M. (2019). The mechanism of hydrogen sulfide mitigation of iron deficiency-induced chlorosis in strawberry (Fragaria × ananassa) plants. Protoplasma 256, 371–382. doi: 10.1007/s00709-018-1298-x
Kaya, C., Ashraf, M., Alyemeni, M. N., and Ahmad, P. (2020). Nitrate reductase rather than nitric oxide synthase activity is involved in 24-epibrassinolide-induced nitric oxide synthesis to improve tolerance to iron deficiency in strawberry (Fragaria × annassa) by up-regulating the ascorbate-glutathione cycle. Plant Physiol. Biochem. 151, 486–499. doi: 10.1016/j.plaphy.2020.04.002
Kumar, D., and Ahmed, N. (2014). Response of nitrogen and potassium fertigation to "Waris" almond (Prunus dulcis) under northwestern Himalayan region of India. Sci. World J. 2014:141328. doi: 10.1155/2014/141328
Kumari, A., Parida, A. K., Rangani, J., and Panda, A. (2017). Antioxidant activities, metabolic seconda, proximate analysis, mineral nutrient composition of Salvadora persica fruit unravel a potential functional food and a natural source of pharmaceuticals. Front. Pharmacol. 8:61. doi: 10.3389/fphar.2017.00061
Langer, S. E., Marina, M., Burgos, J. L., Martínez, G. A., Civello, P. M., and Villarreal, N. M. (2019). Calcium chloride treatment modifies cell wall metabolism and activates defense responses in strawberry fruit (Fragaria × ananassa, Duch). J. Sci. Food Agric. 99, 4003–4010. doi: 10.1002/jsfa.9626
Li, C., Meng, D., Zhang, J., and Cheng, L. (2019). Genome-wide identification and expression analysis of calmodulin and calmodulin-like genes in apple (Malus × domestica). Plant Physiol. Biochem. 139, 600–612. doi: 10.1016/j.plaphy.2019.04.014
Li, H., Yang, Q. S., Liu, W., Lin, J., and Chang, Y. H. (2017). The AMT1 family genes from Malus robusta display differential transcription features and ammonium transport abilities. Mol. Biol. Rep. 44, 379–390. doi: 10.1007/s11033-017-4119-y
Lieberman, M., and Wang, S. Y. (1982). Influence of calcium and magnesium on ethylene production by apple tissue slices. Plant Physiol. 69, 1150–1155. doi: 10.1104/pp.69.5.1150
Liu, H., Chen, F., Lai, S., Tao, J., Yang, H., and Jiao, Z. (2017). Effects of calcium treatment and low temperature storage on cell wall polysaccharide nanostructures and quality of postharvest apricot (Prunus armeniaca). Food Chem. 225, 87–97. doi: 10.1016/j.foodchem.2017.01.008
Liu, J., Jiang, Z., Qi, Y., Liu, Y., Ding, Y., Tian, X., et al. (2021). MdCAX affects the development of the ‘Honeycrisp’ bitter pit by influencing abnormal Ca distribution. Postharvest Biol. Technol. 171:111341. doi: 10.1016/j.postharvbio.2020.111341
Liu, W., Wu, T., Li, Q., Zhang, X., Xu, X., Li, T., et al. (2018). An ethylene response factor (MxERF4) functions as a repressor of Fe acquisition in Malus xiaojinensis. Sci. Rep. 8, 1–13. doi: 10.1038/s41598-018-19518-4
Liu, J., Yang, L., Luan, M., Wang, Y., Zhang, C., Zhang, B., et al. (2015). A vacuolar phosphate transporter essential for phosphate homeostasis in Arabidopsis. Proc. Natl. Acad. Sci. U. S. A. 112, E6571–E6578. doi: 10.1073/pnas.1514598112
Lu, Y. L., Yang, X. L., Li, R., Li, S. L., and Tong, Y. A. (2015). Effects of different potassium fertilizer application periods on the yield and quality of Fuji apple. Ying Yong Sheng Tai Xue Bao 26, 1179–1185. doi: 10.13287/j.1001-9332.2015.0030
Ma, X., Liu, H., Cao, H., Qi, R., Yang, K., Zhao, R., et al. (2019). Genome-wide analysis of zinc‐ and iron-regulated transporter-like protein family members in apple and functional validation of ZIP10. Biometals 32, 657–669. doi: 10.1007/s10534-019-00203-6
Maguire, L. S., O'Sullivan, S. M., Galvin, K., O'Connor, T. P., and O'Brien, N. M. (2004). Fatty acid profile, tocopherol, squalene and phytosterol content of walnuts, almonds, peanuts, hazelnuts and the macadamia nut. Int. J. Food Sci. Nutr. 55, 171–178. doi: 10.1080/09637480410001725175
Mahmood, T., Anwar, F., Abbas, M., Boyce, M. C., and Saari, N. (2012). Compositional variation in sugars and organic acids at different maturity stages in selected small fruits from Pakistan. Int. J. Mol. Sci. 13, 1380–1392. doi: 10.3390/ijms13021380
Mäser, P., Thomine, S., Schroeder, J. I., Ward, J. M., Hirschi, K., Sze, H., et al. (2001). Phylogenetic relationships within cation transporter families of Arabidopsis. Plant Physiol. 126, 1646–1667. doi: 10.1104/pp.126.4.1646
Mauro, M. A., Dellarosa, N., Tylewicz, U., Tappi, S., Laghi, L., Rocculi, P., et al. (2016). Calcium and ascorbic acid affect cellular structure and water mobility in apple tissue during osmotic dehydration in sucrose solutions. Food Chem. 195, 19–28. doi: 10.1016/j.foodchem.2015.04.096
Michailidis, M., Karagiannis, E., Tanou, G., Sarrou, E., Stavridou, E., Ganopoulos, I., et al. (2019). An integrated metabolomic and gene expression analysis identifies heat and calcium metabolic networks underlying postharvest sweet cherry fruit senescence. Planta. 250, 2009–2022. doi: 10.1007/s00425-019-03272-6
Michailidis, M., Karagiannis, E., Tanou, G. M., Samiotaki, G. T., Eirini, S., George, S., et al. (2020). Novel insights into the calcium action in cherry fruit development revealed by high-throughput mapping. Plant Mol. Biol. 104, 597–614. doi: 10.1007/s11103-020-01063-2
Pan, H., Wang, Y., Zha, Q., Yuan, M., Yin, L., Wu, T., et al. (2015). Iron deficiency stress can induce MxNRAMP1 protein endocytosis in M. xiaojinensis. Gene 567, 225–234. doi: 10.1016/j.gene.2015.05.002
Parada, J., Valenzuela, T., Gómez, F., Tereucán, G., García, S., Cornejo, P., et al. (2019). Effect of fertilization and arbuscular mycorrhizal fungal inoculation on antioxidant profiles and activities in Fragaria ananassa fruit. J. Sci. Food Agric. 99, 1397–1404. doi: 10.1002/jsfa.9316
Peng, H. X., Wei, X. Y., Xiao, Y. X., Sun, Y., Biggs, A. R., Gleason, M. L., et al. (2016). Management of valsa canker on apple with adjustments to potassium nutrition. Plant Dis. 100, 884–889. doi: 10.1094/PDIS-09-15-0970-RE
Preciado-Rangel, P., Troyo-Diéguez, E., Valdez-Aguilar, L. A., García-Hernández, J. L., and Luna-Ortega, J. G. (2020). Interactive effects of the potassium and nitrogen relationship on yield and quality of strawberry grown under soilless conditions. Plants 9:441. doi: 10.3390/plants9040441
Qu, G., Wang, H., and Shu, H. (2000). Effect of nitrogen on water use efficiency of apple tree. Ying Yong Sheng Tai Xue Bao 11, 199–201.
Rausch, C., and Bucher, M. (2002). Molecular mechanisms of phosphate transport in plants. Planta 216, 23–37. doi: 10.1007/s00425-002-0921-3
Ritenour, M., and Khemira, H. (2007). Red color development of apple: A literature review. Pullman, WA: Washington State University – Tree Fruit Research and Extension Center.
Rodríguez-Navarro, A., and Rubio, F. (2006). High-affinity potassium and sodium transport systems in plants. J. Exp. Bot. 57, 1149–1160. doi: 10.1093/jxb/erj068
Rubio Ames, Z., Brecht, J. K., and Olmstead, M. A. (2020). Nitrogen fertilization rates in a subtropical peach orchard: effects on tree vigor and fruit quality. J. Sci. Food Agric. 100, 527–539. doi: 10.1002/jsfa.10031
Shen, C., Shi, X., Xie, C., Li, Y., Yang, H., Mei, X., et al. (2019). The change in microstructure of petioles and peduncles and transporter gene expression by potassium influences the distribution of nutrients and sugars in pear leaves and fruit. J. Plant Physiol. 232, 320–333. doi: 10.1016/j.jplph.2018.11.025
Shen, C., Wang, J., Shi, X., Kang, Y., Xie, C., Peng, L., et al. (2017). Transcriptome analysis of differentially expressed genes induced by low and high potassium levels provides insight into fruit sugar metabolism of pear. Front. Plant Sci. 8:938. doi: 10.3389/fpls.2017.00938
Shen, J., Xu, X., Li, T., Cao, D., and Han, Z. (2008). An MYB transcription factor from Malus xiaojinensis has a potential role in iron nutrition. J. Integr. Plant Biol. 50, 1300–1306. doi: 10.1111/j.1744-7909.2008.00761.x
Sheng, Y., Cheng, H., Wang, L., Shen, J., Tang, M., Liang, M., et al. (2020). Foliar spraying with compound amino acid-Iron fertilizer increases leaf fresh weight, photosynthesis, and Fe-S cluster gene expression in peach (Prunus persica (L.) Batsch). Biomed. Res. Int. 2020:2854795. doi: 10.1155/2020/2854795
Shin, H., Shin, H. S., Dewbre, G. R., and Harrison, M. J. (2004). Phosphate transport in Arabidopsis: Pht1;1 and Pht1;4 play a major role in phosphate acquisition from both low‐ and high-phosphate environments. Plant J. 39, 629–642. doi: 10.1111/j.1365-313X.2004.02161.x
Singh, V., Gamrasni, D., Ben Arie, R., Naschitz, S., and Friedman, H. (2016). Identification of open lenticels in apples after harvest in relation to lenticel breakdown development during storage. Postharvest Biol. Technol. 121, 160–170. doi: 10.1016/j.postharvbio.2016.06.004
Singh, V., Gamrasni, D., Parimi, P., Kochanek, B., Naschitz, S., Zemach, H., et al. (2021). Postharvest calcium treatment of apple fruit increased lenticel breakdown and altered cuticle structure. Postharvest Biol. Technol. 171:111331. doi: 10.1016/j.postharvbio.2020.111331
Singh, G., Kachwaya, D. S., Kuchi, V. S., Singh, M., Singh, J., and Singh, B. (2020). Influence of foliar application of mineral nutrients on vegetative and fruit quality of strawberry (Fragaria × ananassa Duch.) cv. Chandler. J. Plant Sci. Res. 36, 323–328. doi: 10.32381/JPSR.2020.36.1-2.41
Sinha, A., Jawandha, S. K., Gill, P. P. S., and Singh, H. (2019). Influence of pre-harvest sprays of calcium nitrate on storability and quality attributes of plum fruits. J. Food Sci. Technol. 56, 1427–1437. doi: 10.1007/s13197-019-03621-z
Song, Z. Z., Duan, C. L., Guo, S. L., Yang, Y., Feng, Y. F., Ma, R. J., et al. (2015a). Potassium contributes to zinc stress tolerance in peach (Prunus persica) seedlings by enhancing photosynthesis and the antioxidant defense system. Genet. Mol. Res. 14, 8338–8351. doi: 10.4238/2015.July.27.22
Song, Z. Z., Ma, R. J., and Yu, M. L. (2015b). Genome-wide analysis and identification of KT/HAK/KUP potassium transporter gene family in peach (Prunus persica). Genet. Mol. Res. 14, 774–787. doi: 10.4238/2015.January.30.21
Song, M., Wang, S., Chai, L., Zhang, S., and Shen, Y. (2017). Characterization of an ABA-induced and K+ channel gene FaKAT1 that regulates strawberry fruit ripening. J. Plant Growth Regul. 36, 312–322. doi: 10.1007/s00344-016-9641-y
Strissel, T., Halbwirth, H., Hoyer, U., Zistler, C., Stich, K., and Treutter, D. (2005). Growth-promoting nitrogen nutrition affects flavonoid biosynthesis in young apple (Malus domestica Borkh.) leaves. Plant Biol. 7, 677–685. doi: 10.1055/s-2005-872989
Sun, Y., Hao, P., Lv, X., Tian, J., Wang, Y., Zhang, X., et al. (2020a). A long non-coding apple RNA, MSTRG.85814.11, acts as a transcriptional enhancer of SAUR32 and contributes to the Fe-deficiency response. Plant J. 103, 53–67. doi: 10.1111/tpj.14706
Sun, X., Jia, X., Huo, L., Che, R., Gong, X., Wang, P., et al. (2018b). MdATG18a overexpression improves tolerance to nitrogen deficiency and regulates anthocyanin accumulation through increased autophagy in transgenic apple. Plant Cell Environ. 41, 469–480. doi: 10.1111/pce.13110
Sun, T., Li, M., Shao, Y., Yu, L., and Ma, F. (2017). Comprehensive genomic identification and expression analysis of the phosphate transporter (PHT) gene family in apple. Front. Plant Sci. 8:426. doi: 10.3389/fpls.2017.00426
Sun, X., Pan, B., Wang, Y., Xu, W., and Zhang, S. (2020b). Exogenous calcium improved resistance to botryosphaeria dothidea by increasing autophagy activity and salicylic acid level in pear. Mol. Plant-Microbe Interact. 33, 1150–1160. doi: 10.1094/MPMI-04-20-0101-R
Sun, C., Yuan, M., Zhai, L., Li, D., Zhang, X., Wu, T., et al. (2018a). Iron deficiency stress can induce MxNAS1 protein expression to facilitate iron redistribution in Malus xiaojinensis. Plant Biol. 20, 29–38. doi: 10.1111/plb.12630
Tian, G., Wang, F., Peng, L., He, L., Jiang, Y. M., and Ge, S. F. (2017). Effects of different potassium levels on growth and NO3− uptake and utilization of Malus hupehensis seedlings. Ying Yong Sheng Tai Xue Bao 28, 2254–2260. doi: 10.13287/j.1001-9332.201707.009
Valentinuzzi, F., Mason, M., Scampicchio, M., Andreotti, C., Cesco, S., and Mimmo, T. (2015a). Enhancement of the bioactive compound content in strawberry fruits grown under iron and phosphorus deficiency. J. Sci. Food Agric. 95, 2088–2094. doi: 10.1002/jsfa.6924
Valentinuzzi, F., Pii, Y., Vigani, G., Lehmann, M., Cesco, S., and Mimmo, T. (2015b). Phosphorus and iron deficiencies induce a metabolic reprogramming and affect the exudation traits of the woody plant Fragaria x ananassa. J. Exp. Bot. 66, 6483–6495. doi: 10.1093/jxb/erv364
Wang, Y., Chen, Y. F., and Wu, W. H. (2020c). Potassium and phosphorus transport and signaling in plants. J. Integr. Plant Biol. 63, 34–52. doi: 10.1111/jipb.13053
Wang, Y. X., Hu, Y., Zhu, Y. F., Baloch, A. W., Jia, X. M., and Guo, A. X. (2018f). Transcriptional and physiological analyses of short-term Iron deficiency response in apple seedlings provide insight into the regulation involved in photosynthesis. BMC Genomics 19:461. doi: 10.1186/s12864-018-4846-z
Wang, R. K., Li, L. L., Cao, Z. H., Zhao, Q., Li, M., Zhang, L. Y., et al. (2012). Molecular cloning and functional characterization of a novel apple MdCIPK6L gene reveals its involvement in multiple abiotic stress tolerance in transgenic plants. Plant Mol. Biol. 79, 123–135. doi: 10.1007/s11103-012-9899-9
Wang, Q., Liu, C., Dong, Q., Huang, D., Li, C., Li, P., et al. (2018b). Genome-wide identification and analysis of apple NITRATE TRANSPORTER 1/PEPTIDE TRANSPORTER family (NPF) genes reveals MdNPF6.5 confers high capacity for nitrogen uptake under low-nitrogen conditions. Int. J. Mol. Sci. 19:2761. doi: 10.3390/ijms19092761
Wang, Q., Liu, C., Huang, D., Dong, Q., Li, P., and Ma, F. (2019a). High-efficient utilization and uptake of N contribute to higher NUE of 'Qinguan' apple under drought and N-deficient conditions compared with ‘Honeycrisp’. Tree Physiol. 39, 1880–1895. doi: 10.1093/treephys/tpz093
Wang, Y., Lü, J., Chen, D., Zhang, J., Qi, K., Cheng, R., et al. (2018d). Genome-wide identification, evolution, and expression analysis of the KT/HAK/KUP family in pear. Genome 61, 755–765. doi: 10.1139/gen-2017-0254
Wang, F., Sha, J., Chen, Q., Xu, X., Zhu, Z., Ge, S., et al. (2020b). Exogenous abscisic acid regulates distribution of 13C and 15N and anthocyanin synthesis in 'Red Fuji' apple fruit under high nitrogen supply. Front. Plant Sci. 10:1738. doi: 10.3389/fpls.2019.01738
Wang, S., Song, M., Guo, J., Huang, Y., Zhang, F., Xu, C., et al. (2018c). The potassium channel FaTPK1 plays a critical role in fruit quality formation in strawberry (Fragaria × ananassa). Plant Biotechnol. J. 16, 737–748. doi: 10.1111/pbi.12824
Wang, F. P., Wang, X. F., Zhang, J., Ma, F., and Hao, Y. J. (2018a). MdMYB58 modulates Fe homeostasis by directly binding to the MdMATE43 promoter in plants. Plant Cell Physiol. 59, 2476–2489. doi: 10.1093/pcp/pcy168
Wang, Y., and Wu, W. H. (2017). Regulation of potassium transport and signaling in plants. Curr. Opin. Plant Biol. 39, 123–128. doi: 10.1016/j.pbi.2017.06.006
Wang, F., Xu, X., Jia, Z., Hou, X., Chen, Q., Sha, J., et al. (2020a). Nitrification inhibitor 3,4-Dimethylpyrazole phosphate application during the later stage of apple fruit expansion regulates soil mineral nitrogen and tree carbon-nitrogen nutrition, and improves fruit quality. Front. Plant Sci. 11:764. doi: 10.3389/fpls.2020.00764
Wang, Y., Zhang, F., Cui, W., Chen, K., Zhao, R., and Zhang, Z. (2019b). The FvPHR1 transcription factor control phosphate homeostasis by transcriptionally regulating miR399a in woodland strawberry. Plant Sci. 280, 258–268. doi: 10.1016/j.plantsci.2018.12.025
Wang, Y., Zhang, X., Wang, Y., Yang, S., and Qu, H. (2018e). The changes of intracellular calcium concentration and distribution in the hard end pear (Pyrus pyrifolia cv. 'Whangkeumbae') fruit. Cell Calcium 71, 15–23. doi: 10.1016/j.ceca.2017.11.002
Wei, M., Wang, S., Dong, H., Cai, B., and Tao, J. (2016). Characterization and comparison of the CPK gene family in the apple (Malus × domestica) and other Rosaceae species and its response to Alternaria alternata infection. PLoS One 11:e0155590. doi: 10.1371/journal.pone.0155590
Wen, B., Li, C., Fu, X., Li, D., Li, L., Chen, X., et al. (2019). Effects of nitrate deficiency on nitrate assimilation and chlorophyll synthesis of detached apple leaves. Plant Physiol. Biochem. 142, 363–371. doi: 10.1016/j.plaphy.2019.07.007
Wu, T., Zhang, H. T., Wang, Y., Jia, W. S., Xu, X. F., Zhang, X. Z., et al. (2012). Induction of root Fe(lll) reductase activity and proton extrusion by iron deficiency is mediated by auxin-based systemic signalling in Malus xiaojinensis. J. Exp. Bot. 63, 859–870. doi: 10.1093/jxb/err314
Xu, X., Du, X., Wang, F., Sha, J., Chen, Q., Tian, G., et al. (2020). Effects of potassium levels on plant growth, accumulation and distribution of carbon, and nitrate metabolism in apple dwarf rootstock seedlings. Front. Plant Sci. 11:904. doi: 10.3389/fpls.2020.00904
Xu, J., Li, H. D., Chen, L. Q., Wang, Y., Liu, L. L., He, L., et al. (2006). A protein kinase, interacting with two calcineurin B-like proteins, regulates K+ transporter AKT1 in Arabidopsis. Cell 125, 1347–1360. doi: 10.1016/j.cell.2006.06.011
Xu, Y., Li, X., Lin, J., Wang, Z., Yang, Q., and Chang, Y. (2015). Transcriptome sequencing and analysis of major genes involved in calcium signaling pathways in pear plants (Pyrus calleryana Decne.). BMC Genomics 16:738. doi: 10.1186/s12864-015-1887-4
Xu, W., Peng, H., Yang, T., Whitaker, B., Huang, L., Sun, J., et al. (2014). Effect of calcium on strawberry fruit flavonoid pathway gene expression and anthocyanin accumulation. Plant Physiol. Biochem. 82, 289–298. doi: 10.1016/j.plaphy.2014.06.015
Xu, L., Zhao, H., Wan, R., Liu, Y., Xu, Z., Tian, W., et al. (2019). Identification of vacuolar phosphate efflux transporters in land plants. Nat. Plants 5, 84–94. doi: 10.1038/s41477-018-0334-3
Yang, Y. Y., Ren, Y. R., Zheng, P. F., Qu, F. J., Song, L. Q., You, C. X., et al. (2020). Functional identification of apple MdMYB2 gene in phosphate-starvation response. J. Plant Physiol. 244:153089. doi: 10.1016/j.jplph.2019.153089
Yin, L., Wang, Y., Yan, M., Zhang, X., Pan, H., Xu, X., et al. (2013). Molecular cloning, polyclonal antibody preparation, and characterization of a functional iron-related transcription factor IRO2 from Malus xiaojinensis. Plant Physiol. Biochem. 67, 63–70. doi: 10.1016/j.plaphy.2013.02.021
Yin, L., Wang, Y., Yuan, M., Zhang, X., Xu, X., and Han, Z. (2014). Characterization of MxFIT, an iron deficiency induced transcriptional factor in Malus xiaojinensis. Plant Physiol. Biochem. 75, 89–95. doi: 10.1016/j.plaphy.2013.12.007
Yu, X. M., Wang, J. Z., Nie, P. X., Xue, X. M., Wang, G. P., and An, M. (2018). Control efficacy of Ca-containing foliar fertilizers on bitter pit in bagged 'Fuji' apple and effects on the Ca and N contents of apple fruits and leaves. J. Sci. Food Agric. 98, 5435–5443. doi: 10.1002/jsfa.9087
Zha, Q., Wang, Y., Zhang, X. Z., and Han, Z. H. (2014). Both immanently high active iron contents and increased root ferrous uptake in response to low iron stress contribute to the iron deficiency tolerance in Malus xiaojinensis. Plant Sci. 214, 47–56. doi: 10.1016/j.plantsci.2013.10.002
Zhai, L., Xiao, D., Sun, C., Wu, T., Han, Z., Zhang, X., et al. (2016). Nitric oxide signaling is involved in the response to iron deficiency in the woody plant Malus xiaojinensis. Plant Physiol. Biochem. 109, 515–524. doi: 10.1016/j.plaphy.2016.11.005
Zhang, G., Liu, W., Feng, Y., Li, D., Li, K., Sun, Q., et al. (2020). Ethylene response factors MbERF4 and MbERF72 suppress iron uptake in woody apple plants by modulating rhizosphere pH. Plant Cell Physiol. 61, 699–711. doi: 10.1093/pcp/pcz234
Zhang, M., Lv, Y., Wang, Y., Rose, J. K., Shen, F., Han, Z., et al. (2017). TATA box insertion provides a selection mechanism underpinning adaptations to Fe deficiency. Plant Physiol. 173, 715–727. doi: 10.1104/pp.16.01504
Zhang, P., Tan, S., Berry, J. O., Li, P., Ren, N., Li, S., et al. (2014). An uncleaved signal peptide directs the Malus xiaojinensis iron transporter protein mx IRT1 into the ER for the PM secretory pathway. Int. J. Mol. Sci. 15, 20413–20433. doi: 10.3390/ijms151120413
Zhang, S., Tong, Y., Li, Y., Cheng, Z. M., and Zhong, Y. (2019b). Genome-wide identification of the HKT genes in five Rosaceae species and expression analysis of HKT genes in response to salt-stress in Fragaria vesca. Genes Genomics 41, 325–336. doi: 10.1007/s13258-018-0767-0
Zhang, K., Yue, D., Wei, W., Hu, Y., Feng, J., and Zou, Z. (2016). Characterization and functional analysis of calmodulin and calmodulin-like genes in Fragaria vesca. Front. Plant Sci. 7:1820. doi: 10.3389/fpls.2016.01820
Zhang, W., Zhang, X., Wang, Y., Zhang, N., Guo, Y., Ren, X., et al. (2018b). Potassium fertilization arrests malate accumulation and alters soluble sugar metabolism in apple fruit. Biol. Open 7:bio024745. doi: 10.1242/bio.024745
Zhang, L., Zhao, S., Lai, S., Chen, F., and Yang, H. (2018a). Combined effects of ultrasound and calcium on the chelate-soluble pectin and quality of strawberries during storage. Carbohydr. Polym. 200, 427–435. doi: 10.1016/j.carbpol.2018.08.013
Zhang, R. F., Zhou, L. J., Li, Y. Y., You, C. X., Sha, G. L., and Hao, Y. J. (2019a). Apple SUMO E3 ligase MdSIZ1 is involved in the response to phosphate deficiency. J. Plant Physiol. 232, 216–225. doi: 10.1016/j.jplph.2018.11.012
Zhao, Q., Ren, Y. R., Wang, Q. J., Wang, X. F., You, C. X., and Hao, Y. J. (2016b). Ubiquitination-related MdBT scaffold proteins target a bHLH transcription factor for iron homeostasis. Plant Physiol. 172, 1973–1988. doi: 10.1104/pp.16.01323
Zhao, Q., Ren, Y. R., Wang, Q. J., Yao, Y. X., You, C. X., and Hao, Y. J. (2016a). Overexpression of MdbHLH104 gene enhances the tolerance to iron deficiency in apple. Plant Biotechnol. J. 14, 1633–1645. doi: 10.1111/pbi.12526
Zhou, H., Qi, K., Liu, X., Yin, H., Wang, P., Chen, J., et al. (2016). Genome-wide identification and comparative analysis of the cation proton antiporters family in pear and four other Rosaceae species. Mol. Gen. Genomics. 291, 1727–1742. doi: 10.1007/s00438-016-1215-y
Keywords: mineral nutrition, Rosaceae fruit, fruit development, quality formation, postharvest storage
Citation: Bai Q, Shen Y and Huang Y (2021) Advances in Mineral Nutrition Transport and Signal Transduction in Rosaceae Fruit Quality and Postharvest Storage. Front. Plant Sci. 12:620018. doi: 10.3389/fpls.2021.620018
Edited by:
Nikos Tzortzakis, Cyprus University of Technology, CyprusReviewed by:
Athanassios Molassiotis, Aristotle University of Thessaloniki, GreeceMatthew John Milner, National Institute of Agricultural Botany (NIAB), United Kingdom
Copyright © 2021 Bai, Shen and Huang. This is an open-access article distributed under the terms of the Creative Commons Attribution License (CC BY). The use, distribution or reproduction in other forums is permitted, provided the original author(s) and the copyright owner(s) are credited and that the original publication in this journal is cited, in accordance with accepted academic practice. No use, distribution or reproduction is permitted which does not comply with these terms.
*Correspondence: Yun Huang, eXVuaHVhbmdAYnVhLmVkdS5jbg==