- 1Instituto de Conservación y Mejora de la Agrodiversidad Valenciana, Universitat Politècnica de València, Valencia, Spain
- 2Escuela Politécnica Superior de Orihuela, Universidad Miguel Hernández, Orihuela, Spain
- 3Plant Protection Unit/Instituto Agroalimentario de Aragón-IA2, Centro de Investigación y Tecnología Agroalimentaria de Aragón, Universidad de Zaragoza, Zaragoza, Spain
- 4Horticulture Unit/Instituto Agroalimentario de Aragón-IA2, Centro de Investigación y Tecnología Agroalimentaria de Aragón, Universidad de Zaragoza, Zaragoza, Spain
- 5Instituto Agroforestal Mediterráneo, Universitat Politècnica de València, Valencia, Spain
The performance of snake melon [Cucumis melo var. flexuosus (L.)] in organic farming was studied under high biotic and salt stress conditions. Soilborne diseases (mainly caused by Macrophomina phaseolina and Neocosmospora falciformis), combined with virus incidence [Watermelon mosaic virus (WMV), Zucchini yellow mosaic virus (ZYMV), and Tomato leaf curl New Delhi virus (ToLCNDV)] and Podosphaera xanthii attacks, reduced yield by more than 50%. Snake melon susceptibility to M. phaseolina and Monosporascus cannonballus was proved in pathogenicity tests, while it showed some degree of resistance to Neocosmospora keratoplastica and N. falciformis. On the contrary, salt stress had a minor impact, although a synergic effect was detected: yield losses caused by biotic stress increased dramatically when combined with salt stress. Under biotic stress, grafting onto the melon F1Pat81 and wild Cucumis rootstocks consistently reduced plant mortality in different agroecological conditions, with a better performance compared to classic Cucurbita commercial hybrids. Yield was even improved under saline conditions in grafted plants. A negative effect was detected, though, on consumer acceptability, especially with the use of Cucurbita rootstocks. Cucumis F1Pat81 rootstock minimized this side effect, which was probably related to changes in the profile of sugars, acids, and volatiles. Grafting affected sugars and organic acid contents, with this effect being more accentuated with the use of Cucurbita rootstocks than with Cucumis. In fact, the latter had a higher impact on the volatile organic compound profile than on sugar and acid profile, which may have resulted in a lower effect on consumer perception. The use of Cucumis rootstocks seems to be a strategy to enable organic farming production of snake melon targeted to high-quality markets in order to promote the cultivation of this neglected crop.
Introduction
Melon (Cucumis melo L.) is a member of the Cucurbitaceae family, which comprises several economically important vegetables, including cucumber, watermelon, squash, pumpkin, and gourds (Pitrat, 2008). It is a highly variable species with a relevant economic importance worldwide (global production 27 Mt) (FAOSTAT, 2018). The top 10 producing countries are in Asia (China, Turkey, India, Kazakhstan, and Iran), Africa (Egypt), Europe (Spain), and America (United States, Guatemala, and Mexico). Spain is the 8th highest melon producer in the world, and the first producer and exporter of the European Union (EuroStat, 2019). C. melo has been produced in Spain since Roman times. The Roman author Lucius Junius Moderatus Columella already mentioned in his writings the cultivation of a snake-shaped cohombro, a C. melo belonging to the botanical variety flexuosus (Hammer and Gladis, 2014), which produced non-sweet fruits. With the arrival of Islam to the Iberian Peninsula, sweet casaba type melons as well as cucumbers (Cucumis sativus L.) were introduced into Europe from Central Asia (Paris et al., 2012a, b). The preference of sweet casaba melon as fruit and cucumber as vegetable displaced and diminished the consumption of flexuosus-type melons.
Snake melon is also an ancient crop highly appreciated in other Mediterranean, Asia Minor, North Africa, and Near and Middle East countries, where it is known by different local names such as Armenian cucumber, Fakous, Kakri, Cucumaru, Hiti, or Mekte, among others (Merheb et al., 2020). Its long non-sweet, non-aromatic fruits are collected when immature and are consumed as fresh vegetables or pickled (Pitrat, 2016; Solmaz et al., 2016a). They are part of many traditional recipes, used like cucumbers because of their appearance and taste, and are also used in traditional medicine (Ibrahim, 2017). This is one of the less studied melons, although the genetic diversity of Middle East landraces has started to be known (Dastranji et al., 2017; Abu Zaitoun et al., 2018; Merheb et al., 2020).
Despite the fact that it has been a neglected crop, snake melons are still commonly grown in many Mediterranean, Asian, and African countries. In Spain, they remain under cultivation in eastern coastal regions (Valencia, Alicante, and Murcia), where local farmers have conserved landraces locally known as “alficoz or alficoç.” Some of these landraces are cultivated for self-consumption and for local markets as the short shelf life of the fruits, much shorter than that of the cucumbers, hampers their commercialization in distant markets. However, this crop is threatened by severe genetic erosion and Spanish snake melon landraces are maintained ex situ in the Universitat Politècnica de València GeneBank.
There exists a global interest for the recovery of heirloom vegetables. Farmers markets, where consumers have access to locally grown vegetables, have continued to rise in popularity. Consumer demands on traditional products are more focused on those produced under sustainable or organic production systems. Organic farming values the use of plant diversity and may constitute the ideal context to promote the cultivation of snake melon (Reganold and Wachter, 2016). The consumer perception of organic food as providing differentiating benefits related to health, nutritional value, and maximum respect to the environment and animals (Massey et al., 2018) can be the basis to revitalize its demand, encourage its cultivation, and promote in situ conservation.
Melon organic farming faces several limiting factors that need to be overcome to be economically sustainable. One of the major limitations is the negative effect on yield and yield stability (Seufert et al., 2012; Schrama et al., 2018). Pests and diseases are main factors causing this loss of productivity, as the use of agrochemicals is very limited. Also, the fact that the local production of this crop is often restrained to marginable lands, where not only biotic but also abiotic stressful conditions occur, enhances the challenge.
One way of controlling diseases would be the use of resistant varieties. However, the alficoz, as many heirloom landraces, has been often neglected in melon breeding programs as the main focus was on sweet melons. Some breeding has centered on yield and earlier production, but no cultivars with introgressed resistances are available (Abdelmohsin et al., 2015). Grafting is an effective approach to deal with soilborne pathogens, increasing yields in stressful environments (Bie et al., 2017). Grafting is used in Cucurbits, mainly in watermelons (about the 90% of the watermelon production in many countries is obtained from grafted plants). Grafting is not so popular in melons due to the lack of appropriated rootstocks. Melon grafting is performed primarily to face soilborne pathogens such as Fusarium and Monosporascus wilts or nematodes (Kyriacou et al., 2018). Compatibility in rootstock–scion combinations and the lack of negative effect on fruit quality are necessary for the successful performance of grafted plants (Picó et al., 2017; Leonardi et al., 2017).
There are many previous studies that report the use of different rootstocks for sweet melons and describe their impact on their aspect and flavor, which is particularly affected by the sugar/acid content and aroma (Colla et al., 2006; Crinò et al., 2007; Condurso et al., 2012; Verzera et al., 2014). These studies clearly show that grafting can have an impact on the quality profile of sweet melons. Also, the impact of grafting in flavor perception through the accumulation of certain compounds has been reported in watermelons (Guler et al., 2014; Fredes et al., 2017; Tripodi et al., 2020). It seems that these effects would be limited in species harvested before biological maturity such as cucumber (Davis et al., 2008), as it would be the case for snake melon.
Only few studies have analyzed flavor preferences of snake melons and their relationship with metabolite accumulation. A recent study with accessions from the Middle East suggested that crispy and non-hollow fruits are not necessarily tastier, but softer and hollow fruits are seldom associated with very good taste (Omari et al., 2018). They also highlighted the existence of a high diversity of taste perception within types. Grafting snake melon has been studied (Maroto et al., 2003), but not the impact of grafting on the quality of this crop. The objective of this study is to fill the gap in the knowledge of the effect of grafting on snake melon agronomic performance under organic farming. The effect of biotic and abiotic stressful conditions is evaluated, as well as the impact of the different types of rootstocks, melon, wild Cucumis, and Cucurbita hybrids, on fruit characteristics, sensory perception, and sugar, acid, and volatile accumulation.
Materials and Methods
Fields Characteristics
The study was performed in three different fields of the Valencian Community (Eastern Spain) (Supplementary Figure 1). The first was located in Moncada, a small town in the province of Valencia (39°33′26.8″ N, 0°25′06.5″ W), in a field with no previous history of melon cultivation, as it had been a Citrus orchard for the previous 20 years (Supplementary Figure 1A). The second field assay was located in La Punta, a suburban area of the city of Valencia (39°26′41.3″ N, 0°21′’14.9″ W), with a long history of melon cultivation (Supplementary Figure 1B). The third field assay was in the province of Alicante, in the Natural Park of Carrizales (38°08′32.8″ N, 0°42′44.7″ W). The cultivation plots of Carrizales used in 2018 and 2019 were cultivated with alfalfa and oat, respectively, for the three previous years, and then, 6 months before the assay, were fallowed (Supplementary Figure 1C). Climate data were obtained from public databases (La Punta: Agencia Estatal de Meteorología1; Moncada and Carrizales: Sistema de Información Agroclimática para el Regadío2).
Plant Material
A local snake melon cultivar traditionally known as “Alficoz valenciano” obtained from the GeneBank of the Universitat Politècnica de València (accession number BGV004853) was used for the study. This accession was selected in a previous study considering its good field performance regarding yield and quality and its good consumer acceptance.
In order to analyze the effect of grafting on agronomic performance, fruit quality, and sugar, acid, and volatile profile, five rootstocks were selected and used to graft the snake melon cultivar (Supplementary Figure 2). All graftings were compatible, resulting in fully developed adult plants. Rootstocks included F1Pat81, an experimental inter-subspecific cross between C. melo subsp. agrestis Pat 81, resistant to Monosporascus cannonballus (Roig et al., 2012), and C. melo subsp. melo Piel de sapo, two hybrid rootstocks between wild Cucumis species, Fian (hybrid Cucumis ficifolius × Cucumis anguria), and Fimy (hybrid C. ficifolius × Cucumis myriocarpus), resistant to different soilborne diseases (Cáceres et al., 2017), and two commercial hybrid Cucurbita maxima × Cucurbita moschata rootstocks (Shintoza and Cobalt). Non-grafted (NG) snake melon plants were used as controls in all the assays. The grafting technique employed for the experiments was the “Tongue approach grafting” method.
Crop Management
The experiments were conducted under organic production in the three different fields. The three fields represent three different agro-ecological situations, frequent in melon cultivation. All fields have a clay soil (more clay-loam in Moncada). In the latter field, Moncada, the lack of melon cultivation in the 20 previous years provided unstressed conditions. In La Punta, fungal stress had been reported, due to the accumulation of soilborne pathogens after repetitive melon cultivation. In the natural park of Carrizales, the traditional irrigation system uses water coming from a drainage water channel. This water is characterized by its high electrical conductivity, thus resulting in salt stress conditions.
In 2018, the three fields were cultivated. NG plants were cultivated in the three fields. Additionally, in La Punta and Carrizales, snake melon was also cultivated and grafted onto two rootstocks, the experimental melon hybrid F1Pat81 and the commercial Cucurbita hybrid Cobalt. A randomized complete block design with four plants per treatment and block was used (four blocks).
In 2019, plants were grown in La Punta and Carrizales. This year, the snake melon was cultivated NG and grafted onto five selected rootstocks, the two used in 2018 (F1Pat81 and Cobalt), the two experimental wild Cucumis rootstocks Fian and Fimy, and one additional commercial Cucurbita hybrid, Shintoza, using the same experimental design.
Snake melon plants were transplanted at the 2–3 true leaf stage, between the end of March and the first week of April in the three cultivation sites, in 2018, and the first week of May in 2019. During both years, in the fields, sweet melon Spanish varieties were also cultivated, both grafted and NG.
In Moncada, plants were transplanted onto ridges with black mulch with a separation of 1.1 m between ridges and 1 m between plants. The field was prepared by subsoiling and sheep manure (1 kg m–2) was applied, and afterward, the soil was milled to break down clods of soil and mix the manure. Two applications of azadirachtin with Equisetum arvense and diatomaceous earth were performed to control aphids and whiteflies. In La Punta, a black plastic mulch was also used, with the separation of 2 m between ridges and 0.6 m between plants. The only further treatment performed was the weeding of the soil between ridges. In Carrizales, plants were spaced 2 m between ridges and 0.9 m between plants, with an additional 0.5 m between each treatment and using black plastic mulch. The field was prepared by subsoiling and applying sheep manure (3 kg m–2), and afterward, the soil was milled to break down clods of soil and mix the manure. After transplanting, the plants were covered with a thermal blanket until they reached the appropriate size. The blanket enabled the control of both temperature and humidity, also acting as a barrier against pests. During the crop cycle in Carrizales, two applications were performed with humic and fulvic acids diluted in the water supply. During fruit ripening, one application through the water supply was done of potassium sulfate. Every 15–20 days, sulfur (15–25 kg ha) and Vibafusan G (15 kg ha–1) were applied. Finally, two foliar treatments of biostimulator F-Aspir (5 L ha–1) were applied. Finally, in terms of irrigation, drip irrigation was used in Carrizales and Moncada, whereas in La Punta, water was supplied using flood irrigation once every 2 weeks.
Soil and Water Conductivity
For each assayed field, 10 soil samples from different sites were collected, and their conductivity was measured. The soil samples were homogenized and dried at room temperature. The dry soil samples were then sieved (2 mm) and the soil conductivity (dS m–1) was determined using the method described by Primo and Carrasco (1980). The conductivity of the water supply was measured in collected water samples with an electrical conductivity meter (CM35, CRISON, Barcelona, Spain).
Pathogen Detection
All the plants showing virus symptoms were sampled, and the viruses were identified following Pérez-de-Castro et al. (2019). Root samples were obtained from plants that showed symptoms of soilborne pathogens and they were analyzed to identify the causal agents. Small pieces (0.5–1 cm) from the cortical necrosis of both lower stem and upper root were surface disinfected for 1 min in 1.5% NaClO, washed four times with sterilized bi-distilled water, and plated onto potato dextrose agar (PDA) amended with streptomycin sulfate (0.5 g/L) to avoid bacterial contamination. Plates were incubated at 25°C in the dark for 3–5 days. Then, emerging colonies were transferred to 6 cm Ø PDA plates, and each isolate was subcultured to get pure cultures for subsequent characterization.
Fungal isolates obtained were identified and characterized morphologically on the basis of comparison of their different somatic and/or sexual and asexual reproductive structures. A molecular characterization was made by PCR amplification of the ribosomal ITS fragment for most of the isolates, and TEF-1α and RPB2 gene fragments in the case of certain Fusarium species, using ITS1/ITS4 (White et al., 1990), EF1/EF2 (O’Donnell et al., 1998), and fRPB2-7cF/fRPB2-11aR (Reeb et al., 2004) primers, respectively. Sequences obtained allowed the identification of isolates by their comparison with homologous sequences deposited in public databases like GenBank (using BLASTn tool) or Fusarium ID Database3, as well as by performing phylogenetic reconstructions employing Bayesian inference methods from multilocus alignments of combined genomic regions for some of the mentioned Fusarium taxa.
Pathogenicity Tests Against Fungal Pathogens
The degree of susceptibility/tolerance of the snake melon cultivar against four of the most frequently isolated soilborne pathogens, the fungi Neocosmospora keratoplastica and Neocosmospora falciformis, recently detected causing Fusarium wilt in melon in Spain for the first time (González et al., 2020b, c), and M. cannonballus and Macrophomina phaseolina, previously reported to cause severe vine decline and charcoal root rot in melons (Castro et al., 2020; de Sousa Linhares et al., 2020), was evaluated. Isolates from Carrizales were used for Neocosmospora spp. and from La Punta for M. cannonballus and M. phaseolina, respectively.
For N. keratoplastica and N. falciformis, plants of the snake melon cultivar and “Don Quixote” Piel de Sapo (commercial control not previously tested against these pathogens) were grown with a sterilized substrate in plastic trays. When plants reached the 15-day-old stage, they were uprooted and artificially inoculated by root dip for 2 min into a conidial suspension of 5 × 106 conidia ml–1 of each isolate (seven plants with each pathogen and three non-inoculated controls). Then, inoculated plants were planted into pots containing sterilized substrate and maintained in a growth chamber for 30 days at 26°C (González et al., 2020c). Disease severity was evaluated at 30 days after inoculation (DAI), using the following scale: 1 = no symptoms; 2 = beginning of wilting or yellowing on leaves; 3 = all leaves completely wilted, stem standing; and 4 = dead plant (Cothiere et al., 2016). Plants with a disease severity score (at 30 DAI) lower than 2 were considered to be resistant (R); between 2 and 3, moderately resistant (MR); and higher than 3, susceptible (S). The area under the disease progress curve (AUDPC) was calculated for each inoculation (Perchepied and Pitrat, 2004) with the formula:
where i = 1 to 4 scorings, xi = mean disease score of each plant at date i, xi + 1 = mean disease score of each plant at date i + 1, and ti + 1−ti = number of days between scoring date i and scoring date i + 1. The AUDPC value is effective for determining the progress of the disease; it gathers different observations during the epidemic and summarizes all the values in a single one that reflects the severity of disease. Finally, dead or severely wilted plants were removed and processed to check the cause of death and fulfill Koch’s postulates.
For both M. cannonballus and M. phaseolina, six plants of the snake melon accession were inoculated and three were used as non-inoculated controls. Also, six plants of the “Piñonet” Piel de Sapo cultivar were used as commercial susceptible control (Ambrósio et al., 2015; Castro et al., 2020). The inoculum of M. cannonballus was prepared as described in Castro et al. (2020). Briefly, inoculated PDA plates were incubated at 25°C for 1 week and used to inoculate autoclaved bottles with hydrated wheat seeds. These bottles were incubated at 25°C for 1 month, being shaken weekly. After this period, the final inoculum was prepared by mixing 200 g of the inoculated wheat seeds kg–1 of peat. Plants were grown in a greenhouse, and 30 DAI roots were washed and extended on an acetate film. Root damage of the primary and lateral roots was scored from 0, healthy with no lesions, to 4, highly damaged roots with root rots, rootlets pruning, etc. The inoculum of M. phaseolina was prepared as reported in Ambrósio et al. (2015). Briefly, the isolate was inoculated in PDA + antibiotic (tetracycline 0.05 g/L) plates. This culture was used to inoculate plates containing toothpicks in PDA medium that was incubated at 28°C for 1 week. Inoculated toothpicks were inserted at the base of the stem of plants 20 days after transplanting. Plants were grown in a greenhouse, and the severity of the infection was scored at 7 and 15 DAI, with a severity index, from 0, asymptomatic, to 4, severe lesions on the stem.
Fruit Characterization
All fruits of marketable size were weighted at the time of harvest to estimate total marketable yield per plant (Figures 1A,B). Two fruits per plant were selected for characterization (Figures 1C,D) in order to obtain data for fruit weight (FW, g, measured with a digital scale), fruit length and diameter (FL and FD, in cm, measured with a graduated ruler), rind and flesh firmness (RF and FF, measured with a penetrometer in kg cm–2), fruit pH (universal pH indicator paper), and soluble solids content [SSC, measured as °Brix from drops of juice using a hand-held Pocket refractometer (PAL-α), Atago CO., LTD, Tokyo, Japan]. Both fruit flesh and rind colors were measured with a CR-400 colorimeter (Konica Minolta, Inc., Tokyo, Japan), obtaining Hunter L, a, and b coordinates.
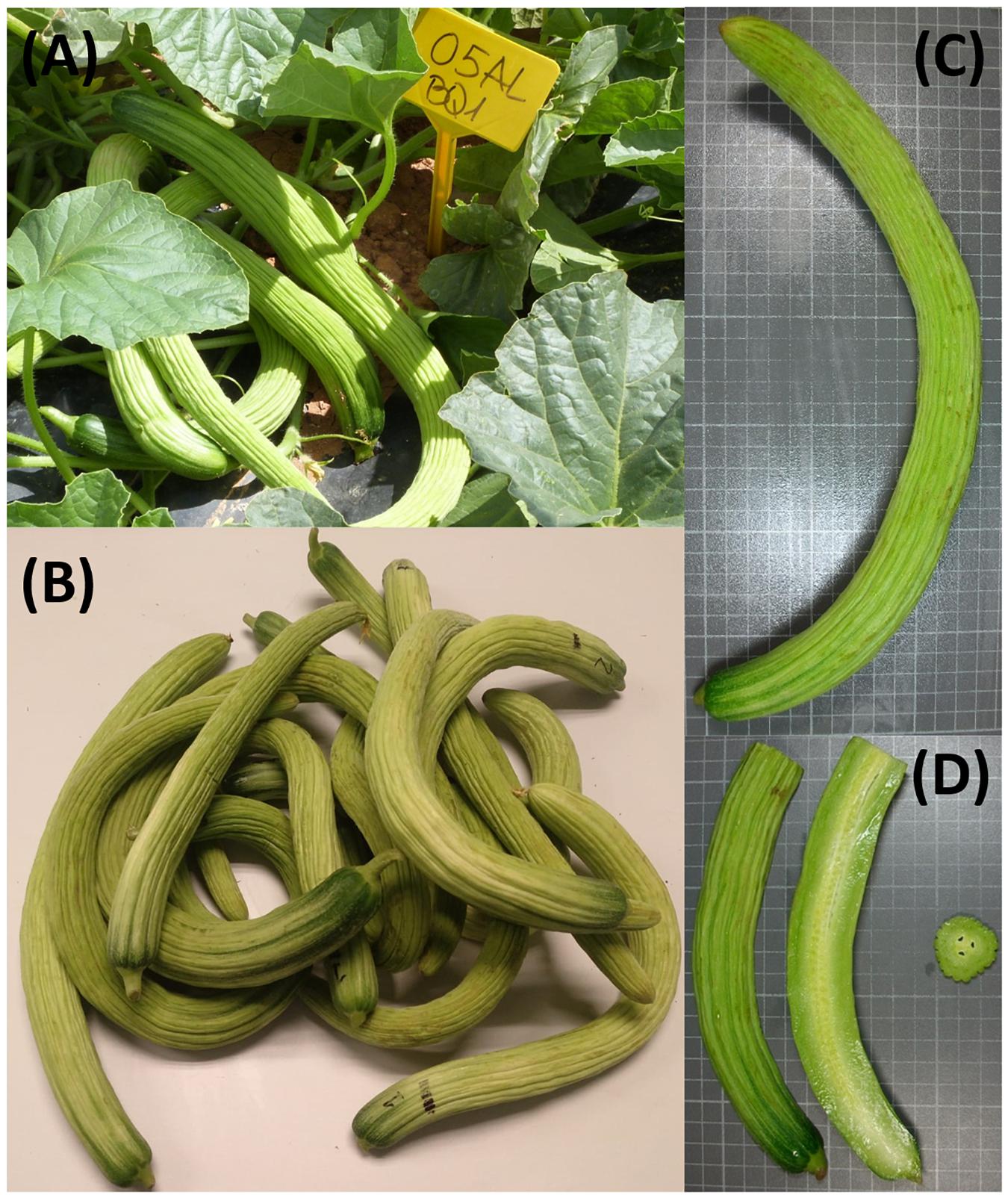
Figure 1. Snake melon fruit in the field ready to be harvested (A) and harvested fruit (B). Characterization of snake melons (C,D).
Fruit Sensorial and Metabolomics Analysis
Sensory evaluations were performed employing a modified methodology of Saftner et al. (2006).
In 2018, four sensory evaluations using a consumer panel (20 tasters) were performed with snake melon fruits from La Punta (evaluations 1 and 2) and Carrizales (evaluations 3 and 4). In each evaluation, the fruits harvested from different plants of each treatment were arranged in three biological replicates for each of the three treatments, NG and grafted onto F1Pat81 and Cobalt. The panelists were asked to score the nine samples randomly arranged (three biological replicates of each of the three treatments) in a 1–5 scale (5 representing the highest acceptability), considering flavor, texture, and aroma together. During the evaluations, panelists could add extra comments regarding the scores provided. Panelists were given water and low salt cracker to cleanse the palate. All snake melon samples were collected the day of the evaluation, as snake melons have a short shelf life.
In 2019, five sensory evaluations (20 tasters) were performed, the first three using fruits obtained from La Punta and the last two from Carrizales. The same design as that in 2018 was used, and fruits harvested from different plants of each treatment were arranged in three biological replicates. Evaluations 1 and 5 compared fruits from NG plants and plants grafted onto the two Cucumis rootstocks, F1Pat81 and Fian. Evaluations 2, 3, and 4 compared fruits from NG plants and grafted onto the Cucumis and Cucurbita rootstocks, F1Pat81 and Shintoza. Panelists were asked to provide a score between 1 and 5 to flavor, texture, and aroma to each of the nine samples randomly arranged (three biological replicates of each treatment). Aliquots of fruit tissue from the biological replicates used for the sensory evaluations were used for sugars, organic acids, and volatile organic compounds (VOCs) analysis.
Sugar and Acid Analysis
The aliquots of fruit tissue from the biological replicates used in sensory evaluations of year 2019 (La Punta and Carrizales, NG plants and plants grafted onto F1Pat81, Fian, and Shintoza) were homogenized (Silent Crusher M; Heidolph, Schwabach, Germany) and frozen at −80°C until analysis. A half of each sample was used for sugars (sucrose, glucose, and fructose) and organic acids (citric, malic, and glutamic) analysis. Only sporadic contents of glutamic acid were found, and these data were not included in the results. These compounds were quantified following the methodology described by Cebolla-Cornejo et al. (2012) based in capillary electrophoresis. For that purpose, an Agilent 7100 system (Agilent Technologies, Waldbronn, Germany) was used.
For the analysis, samples were thawed in a refrigerator in complete darkness. Then, they were centrifuged at 510 × g for 5 min. The upper phase was diluted (1:20) with deionized water and filtered using centrifuge tube filters with 0.22-μm membranes (Costar® Spin-X®, Corning, Amsterdam). For the separation, fused-silica capillaries (Polymicro Technologies, Phoenix, AZ, United States) with 50 μm internal diameter, 363 μm external diameter, 67 cm total length, and 60 cm effective length were used. They were previously conditioned with flushes at 95,000 Pa of NaOH 1 mol L–1 at 50°C for 5 min, NaOH 0.1 mol L–1 for 5 min at 20°C, and deionized water (Elix 3, Millipore, Billerica, MA, United States) for 10 min. At the beginning of each sequence, the capillary was flushed at 20°C with the running background electrolyte (BGE) for 30 min. BGE consisted of 20 mmol L–1 2,6-piridin dicarboxylic acid at pH 12.1 and 0.1% w:v hexadimethrine bromide. Between runs, the capillary was flushed with 58 mmol L–1 SDS (2 min) and BGE (5 min). Samples were injected hydrodynamically at 3400 Pa for 10 s, and separations were performed at −25 kV and 20°C. Absorbance was measured at 214 nm. Results were expressed in g kg–1 fresh weight (FW). Sucrose equivalents were calculated by multiplying sucrose, glucose, and fructose contents by their relative sweetening power, 1, 0.74, and 1.73, respectively, and adding them up (Koehler and Kays, 1991).
Analysis of Volatile Compounds
The second half of each homogenized sample used in sugar and acid analysis was employed for the analysis of VOCs listed in Supplementary Table 1. The method described by Fredes et al. (2017) was followed. Solid phase extraction (SPE) cartridges were conditioned with 5 ml of diethyl ether, 5 ml of n-hexane, and dried for 10 min. Frozen samples were defrosted in the fridge. Once thawed, 30 g of the sample was weighed into a 150-ml Erlenmeyer flask with a stopper. The extraction was carried on using a purge and trap headspace system, using nitrogen gas for the inlet tube and the conditioned SPE cartridge for the outlet tube. The samples were extracted for 49 min at 40°C using magnetic agitation and nitrogen flow of 1.6 ml min–1. Subsequently, the cartridges were eluted using 5 ml of a diethyl ether-hexane 1:1 (v:v) solution and 5 ml of diethyl ether. Finally, the collected elution solvents were evaporated to 0.5 ml at 35°C under a nitrogen flow. The resulting extracts were divided into two aliquots in sealed Gas Chromatography (GC) vials, and frosted at −40°C until analysis.
The chromatographic analysis of VOCs was carried on using a Shimadzu GC-2010 Plus system (Shimadzu, Kioto, Japan) coupled with a single quadrupole mass spectrometry system (GCMS QP2010 Ultra, Shimadzu, Kioto, Japan). A Supelcowax 10 column of 30 m × 0.25 mm (Sigma-Aldrich, St. Louis, MO, United States) was used. Helium was used as carrier gas at a flow of 1 ml min–1. The injection was performed in split mode (split ratio 1/50) with a volume of injection of 1 μl at 250°C. The temperature program started at 30°C during 4 min after the injection followed by a rise to 160°C (10°C min–1), and finally, a rise to 250°C (30°C min–1), which was maintained for 3 min. The mass spectra were acquired in Selected ion monitoring (SIM) mode using the m/z for each compound. Electron ionization in positive mode was used at a temperature of 250 and 230°C for the interphase and the ion source, respectively.
From those treatments included in the five sensory evaluations of 2019, NG and F1Pat81, 10 biological replicates were analyzed for sugar and VOC contents (two of the three biological replicates used per each sensory evaluation), and 7 and 4 biological replicates were independently analyzed for the Shintoza and Fian treatments that were included in three and two of the sensory evaluations performed, respectively.
Statistical Analysis
Fruit characterization, agronomic, sugars, acids, and volatiles data were analyzed using a Dunnett’s test. For each location, the effect of each rootstock was compared to the NG control. StatGraphics Centurion version 17.2.04 for Windows and IBM SPSS Statistics 25 for Windows were used for this purpose. Principal component analysis (PCA) of VOCs data were performed using S-Plus v. 8.01 for Windows (Insightful Corp., Seattle, WA, United States). A biplot representation was then obtained, including the scores of data points and the loadings of each VOC for each principal component. Pairwise correlations for metabolite and sensory analysis data were graphically represented as heatmaps using the software heatmapper4.
Results
Growth-Limiting Factors
Climate, Water, and Soil Properties
Mean temperatures were similar in the three fields (Supplementary Figure 3) except a significantly higher temperature (around 1°C) in April in Carrizales and La Punta compared to Moncada in 2018, and higher temperature in June and July 2019 in Carrizales compared to La Punta. Rainfall was higher in La Punta in the middle of the growing cycle (June) in 2018 and at the beginning (April) of the 2019 assay, and in Carrizales at the end of the growing cycle in 2019.
The irrigation water of Carrizales showed high values of conductivity, reaching 4.5 dS m–1 in 2018 and 5.95 dS m–1 in 2019. Thus, the high salinity in this area of cultivation was confirmed. In fact, soil conductivity reached values of 3.17 ± 0.05 dS m–1 in 2018 and 1.66 ± 0.10 dS m–1 in 2019. The other two fields showed rather standard values for the area, with water conductivity below 2.2 dS m–1 and soil conductivity under 0.7 dS m–1 in both years.
Pests and Diseases
Aphids were the main pest in all the fields in the 2 years, with a higher incidence in La Punta and Moncada than in Carrizales (Supplementary Figure 4).
During 2018, in La Punta, some snake melon plants showed mosaic symptoms (Supplementary Figure 4) and the aphid borne potyvirus WMV was detected, with 9% of snake melons affected by this virus. This year in Moncada, the presence of Cucumber mosaic virus (CMV), also transmitted by aphids, was not detected in snake melons, although it was present in sweet melons cultivated in the same field. No viruses were detected in the plants grown in Carrizales, likely associated to a reduced presence of the insect vectors. Podosphaera xanthii, the fungus responsible for the cucurbit powdery mildew, was detected in La Punta and Moncada, but only caused mild infections to the snake melon plants, whereas the sweet melons were severely affected.
In 2019, WMV was also the most prevalent virus in La Punta, with 26% of the snake melon plants affected by the virus. Other aphid-transmitted potyviruses such as ZYMV and the whitefly transmitted Begomovirus ToLCNDV, both affecting approximately 18% of the snake melon plants, were also detected in this field. P. xanthii (Supplementary Figure 4) affected the snake melon plants in La Punta. Again, the field of Carrizales was free of virus and powdery mildew.
In accordance with the history of fungal stress, we observed some mortality of snake melon plants with vine decay symptoms likely due to fungal soilborne pathogens in 2018 in La Punta. Twelve percent of the NG plants of snake melon died at early developmental stages, and M. phaseolina was isolated from their roots. Twelve percent of the snake melon plants grafted onto the Cucurbita rootstock Cobalt died at later stages, with roots affected by Fusarium oxysporum, whereas plants grafted on F1Pat81 were less affected by fungi. Other soilborne pathogens, detected in roots of sweet melon plants cultivated in the same field, but not affecting snake melons, were Fusarium equiseti, N. falciformis, Fusarium solani, F. solani f. sp. cucurbitae, M. cannonballus, Torula herbarum, Fusarium incarnatum, Rhizopus sp., Alternaria alternata, Alternaria sp., Rhizoctonia solani, Plectosphaerella cucumerina, Aspergillus flavus, Aspergillus sp., Fusarium chlamydosporum, Acremonium sp., Geotrichum candidum, Gibberella fujikuroi, Gibberella sp., Chaetomium acropullum, Chalaropsis radicicola, Collariella bostrychodes, and Penicillium sp.
In 2018, no snake melon plants were affected by soilborne diseases in Carrizales, although different soilborne pathogens were detected in the roots of sweet melon plants cultivated in the same field (M. phaseolina, N. falciformis, N. keratoplastica, F. solani, M. cannonballus, F. oxysporum, Alternaria sp., Cladosporium herbarum, Fusarium sp., C. bostrychodes, Mortierella alpina, and Acremonium sp.). No fungal stress was observed in Moncada.
The fungal attack in 2019 was less severe in La Punta, where the lower mean temperature in May, June, and August and higher rainfall by the end of the cycle could have contributed to the reduced fungal damage (Supplementary Figure 3). No snake melon plant died by soilborne pathogens. Although some soilborne pathogens were detected in the sweet melon plants cultivated on the field (M. phaseolina, F. oxysporum, F. equiseti, F. solani, Fusarium sp., N. falciformis, Alternaria tenuissima, A. alternata, Gibberella sp., Pyxidiophora arvernensis, Botryosphaeriaceae, C. herbarum, Pythium aphanidermatum, Gibberella avenacea, and Mucor sp.), they did not affect the snake melon plants.
This year, a more severe fungal attack was observed in Carrizales (Supplementary Figure 5) that might be associated to the different characteristics of the cultivation plot and to the higher average temperatures in July along with a reduced rainfall until the end of the growing cycle compared to the previous year (Supplementary Figure 3). The snake melons showing symptoms of soilborne pathogens were mainly those grafted onto Cucurbita rootstocks, Shintoza and Cobalt, and the NG plants (44, 31, and 25% mortality, respectively). The main pathogens detected were M. phaseolina, N. falciformis, F. equiseti, Gibberella spp., and Fusarium longipes. No symptoms of fungal attack were observed in plants grafted onto Cucumis rootstocks, F1Pat81, Fimy, and Fian. Sweet melons planted on Carrizales were also affected by the same pathogens.
Response of Snake Melon to M. phaseolina, M. cannonballus, and Neocosmospora spp.
The field survey results indicated that the main pathogen associated with dead snake melon plants or plants showing decay symptoms in our conditions is M. phaseolina. To test the level of susceptibility to this pathogen under controlled conditions, we conducted an artificial inoculation assay, using a M. phaseolina isolate collected in La Punta. Results showed that snake melon is highly susceptible to this pathogen. Symptoms started at 7 DAI and were severe at 15 DAI (Figure 2A). Average damage score was higher in snake melons than in the control sweet melon Piel de Sapo that has also proven to be susceptible to this disease (de Sousa Linhares et al., 2020). All the snake melon plants were dead after 30 DAI, while the mortality of the susceptible control Piel de Sapo was 20%.
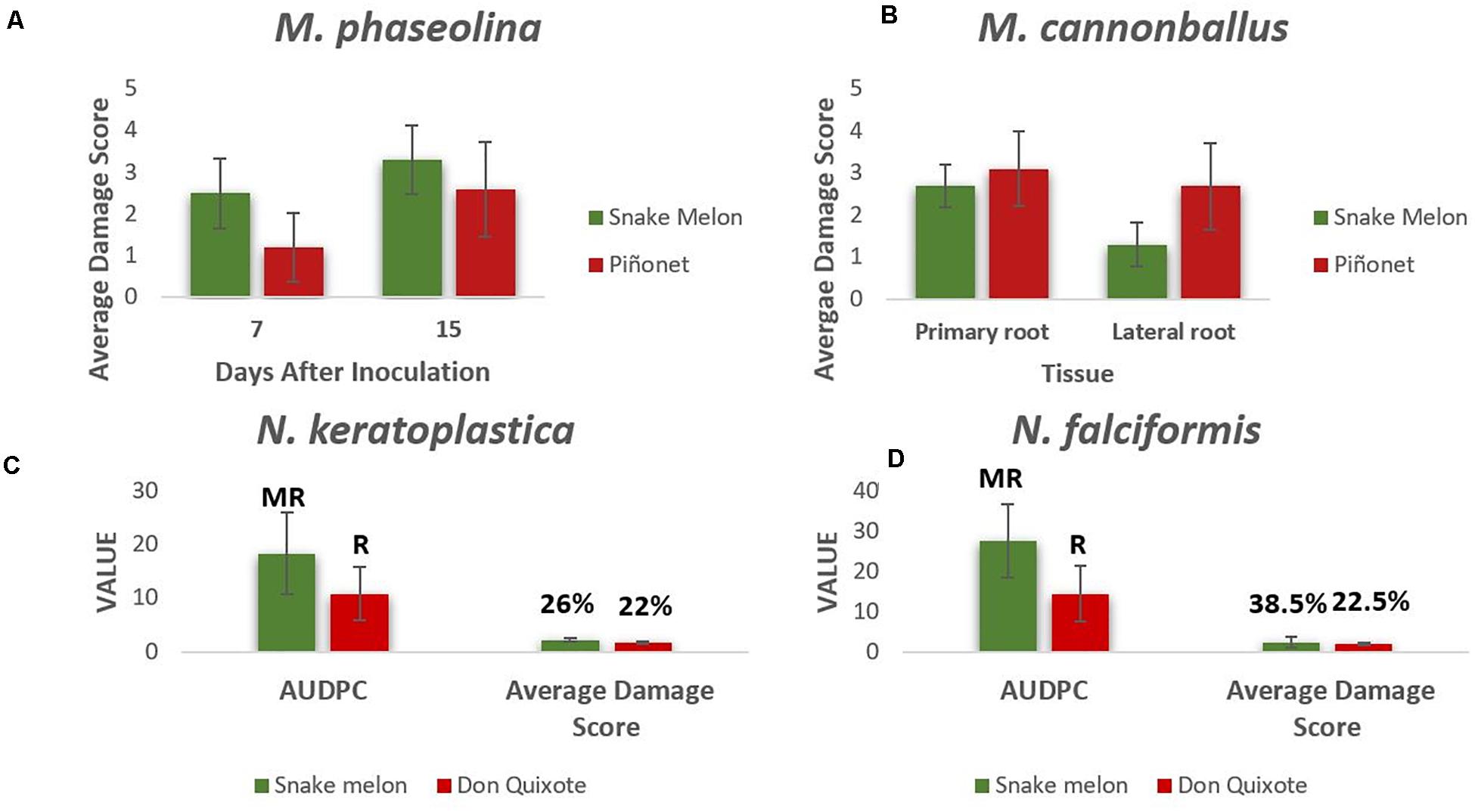
Figure 2. (A) Average damage scores (from 0 to 4) of the stem lesions caused by M. phaseolina in snake melon and sweet melon control (Piel de Sapo Piñonet) at 7 and 15 days after inoculation (DAI). (B) Average damage scores (from 0 to 4) caused by M. cannonballus in primary and lateral roots of snake melon and sweet melon control at 30 DAI. Average area under the disease progress curve (AUDPC), average damage score, type of reaction (R, resistant; MR, moderately resistant), and disease incidence (%) for snake melon and the sweet melon control plants (Piel de Sapo Don Quixote) inoculated with N. falciformis (C) and N. keratoplastica (D). Six (A,B) and seven (C,D) biological replicates (plants) were used for each genotype.
We also tested the response to M. cannonballus, despite the fact that it was less frequent than Macrophomina in our fields, because in many hot and dry regions of melon cultivation, these are two of the main soilborne pathogens affecting melons (Cohen et al., 2016). Snake melon plants were also susceptible to M. cannonballus (Figure 2B), with similar average damage scores in primary roots, although less severe lesions in the lateral roots compared to Piel de Sapo.
Field results also showed the importance of Fusarium species. Snake melons are known to be highly susceptible to several pathogenic forms of the so-called F. oxysporum species complex (Solmaz et al., 2016b; Al-Taae and Al-Taae, 2019), but their response to other species belonging to the F. solani species complex (FSSC) (e.g., some of them currently included in the genus Neocosmospora) had not been tested yet. For both pathogens, N. falciformis and N. keratoplastica, snake melon plants showed considerably higher AUDPC than the commercial control sweet melon Piel de Sapo Don Quixote, as well as a higher disease incidence for N. falciformis (Figures 2C,D). Supplementary Figures 6, 7 show symptoms on snake melon caused by the different pathogens.
Yield and Fruit Characteristics
The agronomic performance of NG melons in the three fields was compared to analyze the effect of the agro-ecological conditions (Figure 3 and Table 1). Moncada, representing unstressed conditions, could be considered as control, as the lack of previous melon cultivation led to the absence of soilborne diseases, while La Punta and Carrizales represented stressful conditions due to the incidence of diseases and the use of saline water and soil, respectively. The yield of NG snake melon plants in La Punta was reduced compared to Moncada (≈ 4 kg/plant vs ≈ 10 kg/plant, respectively) (Figure 3). This reduction was likely a consequence of the incidence of viruses and soilborne diseases, as stated above. Conversely, the high salinity conditions at Carrizales did not affect yield per plant, which was similar to that of Moncada. No differences were found for most fruit traits among the three fields (Table 1), although the stressful conditions of both La Punta and Carrizales resulted in lower fruit FF. Also, regarding basic quality characteristics, the SSC was higher at Carrizales, which is expected under saline conditions. At this field, fruits showed higher Hunter a value, representing a less greenish color of the flesh.
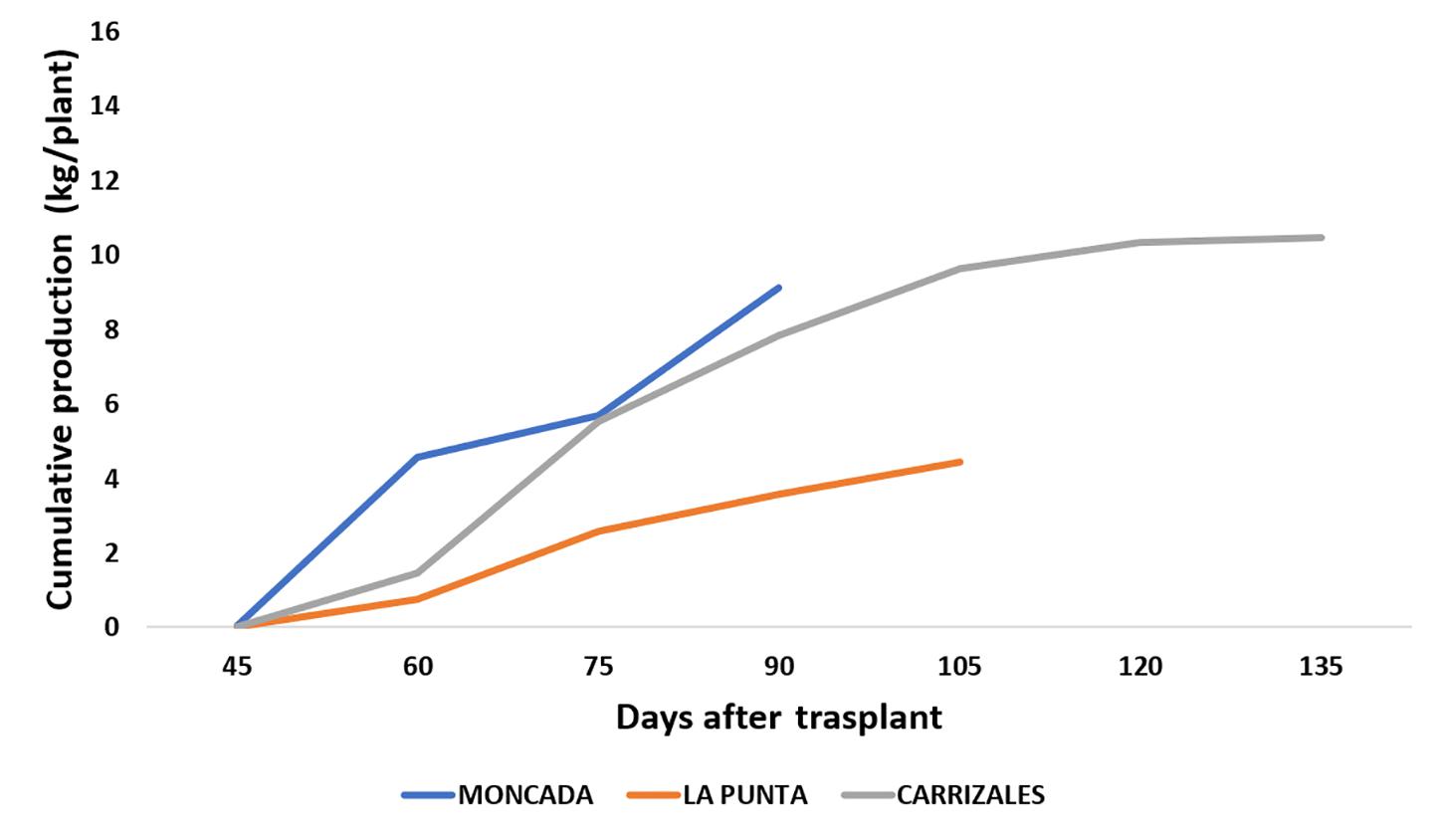
Figure 3. Cumulative production (kg plant–1) of non-grafted (NG) snake melons in 2018, cultivated in the three fields. Sixteen NG plants were cultivated per field. All fruits of marketable size were weighted at the time of harvest to estimate total yield per plant.
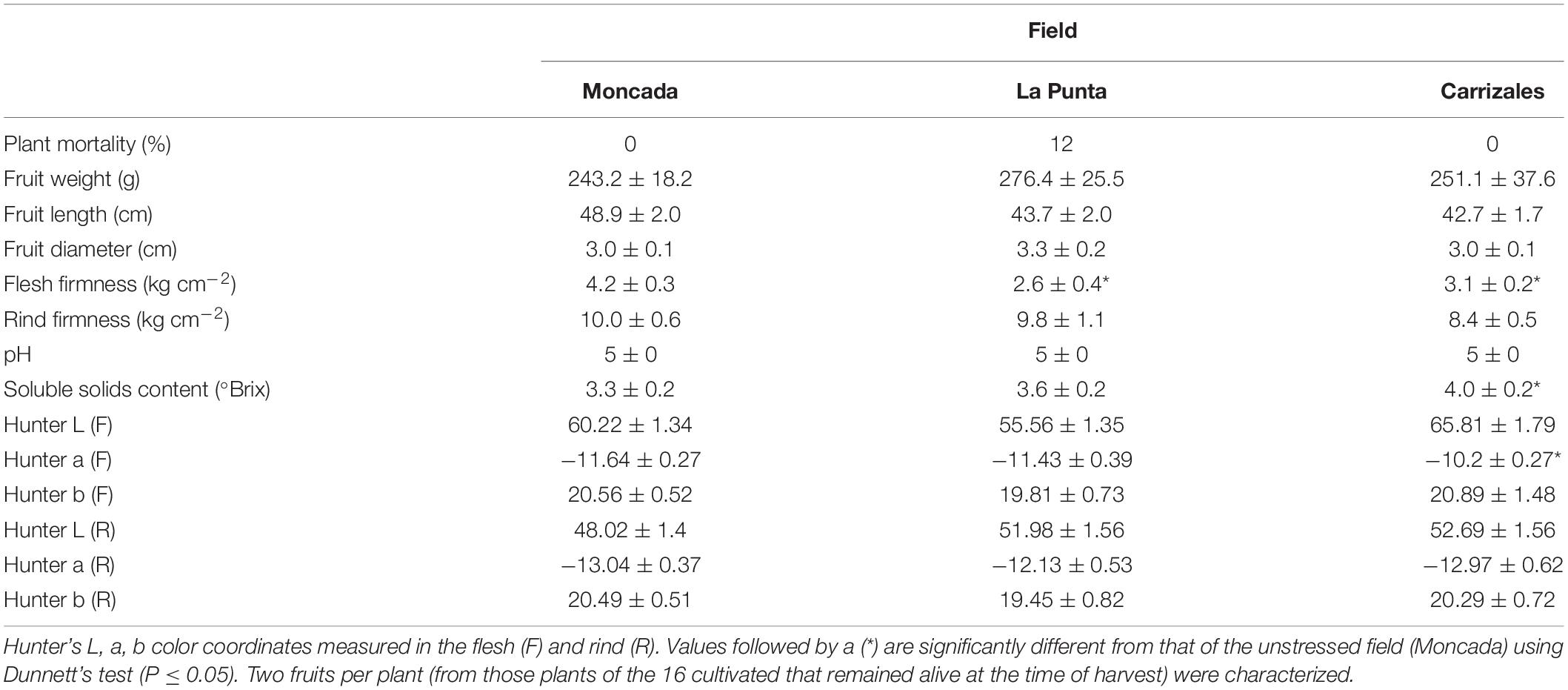
Table 1. Agronomic performance and fruit characterization of non-grafted (NG) plants in the three fields (Moncada, La Punta, and Carrizales) in 2018.
The effect of grafting during 2018 was evaluated in the fields of La Punta and Carrizales using the commercial Cucurbita hybrid Cobalt and the experimental C. melo hybrid F1Pat81. Some mortality was observed in grafted snake melon plants in La Punta, with the F1Pat81 rootstock being the one that displayed the best performance under these epidemiological conditions. The plants grafted onto this rootstock displayed less mortality and higher yield ≈ 6 kg/plant compared to the ≈ 4 kg/plant (Figure 4A) produced by the plants grafted onto Cobalt and NG. The higher susceptibility of snake melon roots and the Cobalt rootstock to the fungal pathogens may account for these differences. Production in Carrizales (Figure 4B) was higher than in La Punta. In this field, fungal stress was lower, and the salt stress had a much less severe impact on snake melon production. Grafting had a favorable effect on snake melon production under saline conditions. The plants grafted onto the melon rootstocks F1Pat81 were as productive as those grafted onto Cobalt (≈ 14 kg/plant), both much more productive than NG plants (≈ 10 kg/plant).
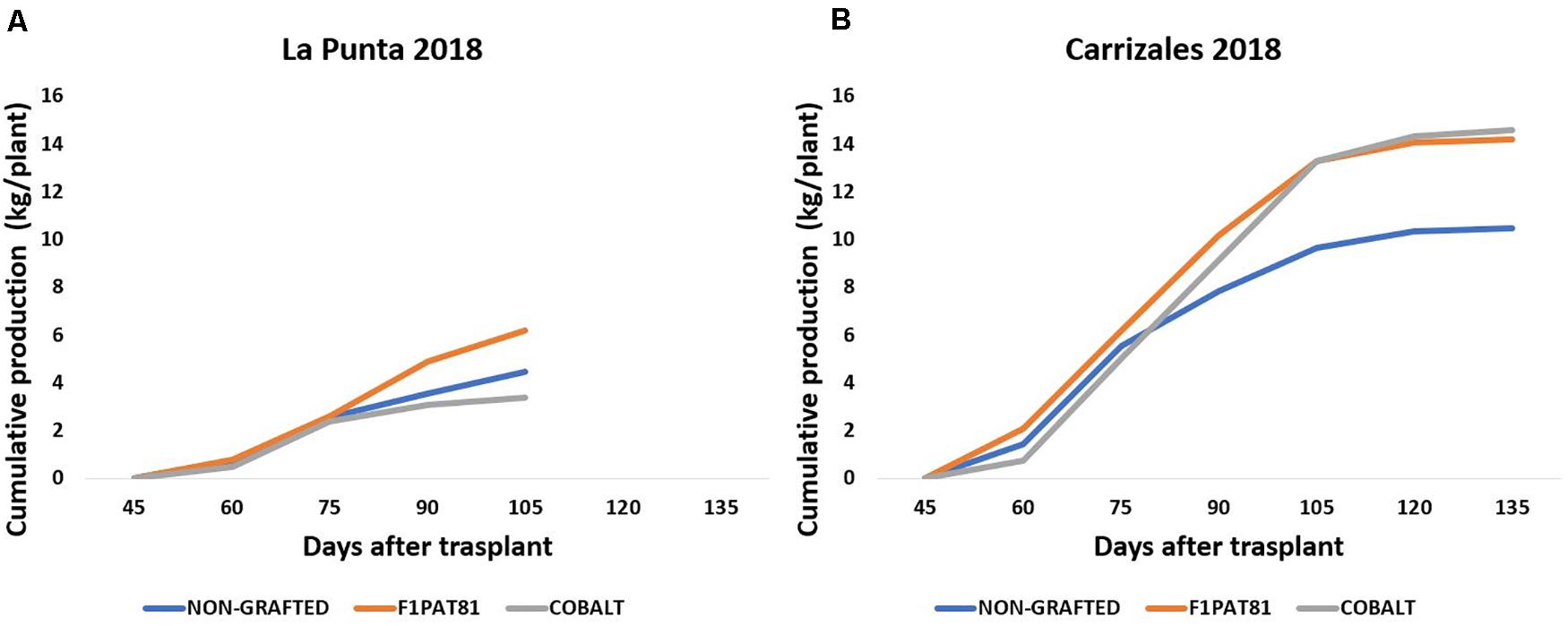
Figure 4. Cumulative production (kg plant–1) of snake melons in 2018 grown in La Punta (A) and Carrizales (B) obtained from non-grafted plants and plants grafted onto the commercial Cucurbita hybrid Cobalt and the experimental C. melo hybrid F1Pat81. Sixteen plants were cultivated per field and treatment. All fruits of marketable size were weighted at the time of harvest to estimate total yield per plant.
Regarding snake melon fruit traits, no differences were found between fruits from the NG and those of grafted for fruit shape, firmness, pH, and °Brix (Table 2). Only Hunter L and a values were altered in fruits from plants grafted on F1Pat81 in La Punta. They were less green with a lighter color. Lower Hunter a values were obtained in fruits from plants grafted onto Cobalt in Carrizales (more greenish color). As stated before, the saline conditions of Carrizales produced snake melon fruits with higher °Brix in all treatments (Table 2).
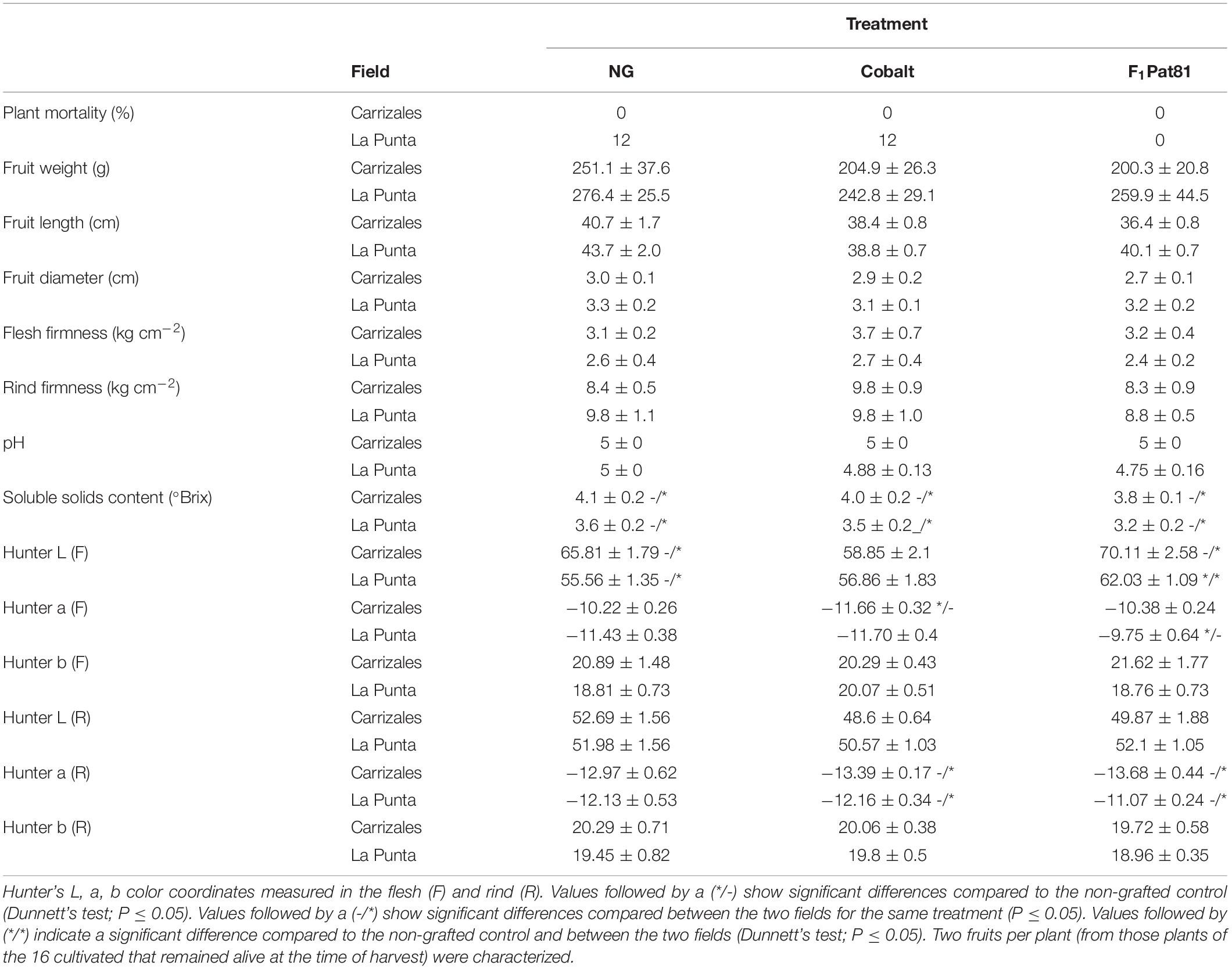
Table 2. Agronomic performance and fruit characterization of the snake melons produced in plants grafted onto commercial Cucurbita hybrid Cobalt and experimental C. melo hybrid F1Pat81 compared to non-grafted in two fields (La Punta and Carrizales) in 2018.
In 2019, the fungal stress in La Punta did not cause plant mortality in snake melons. However, despite lower mortality, the impact on yield was important (Figure 5A). Yield per plant ranged from ≈ 4 to 5 kg plant–1, except for the snake melon plants grafted onto the Fimy rootstock that was the less productive. In Carrizales, the yield in 2019 dropped significantly compared to 2018 (Figure 5B). The fungal attack was more severe. Also, this year, conductivity of irrigation water was higher. Additionally, the fact that an extensive crop like oat, and not a nitrogen fixing crop like alfalfa, was cultivated for the three previous years might have resulted in a poorer and less productive soil. The fungal attack resulted in a higher plant mortality in both NG and grafted onto Cucurbita rootstocks plants, whereas Cucumis rootstocks were again the most tolerant to fungi. Among them, the wild Fian and the cultivated hybrid F1Pat81 maintained a moderate yield per plant compared to NG plants. Fimy was again the less productive rootstock.
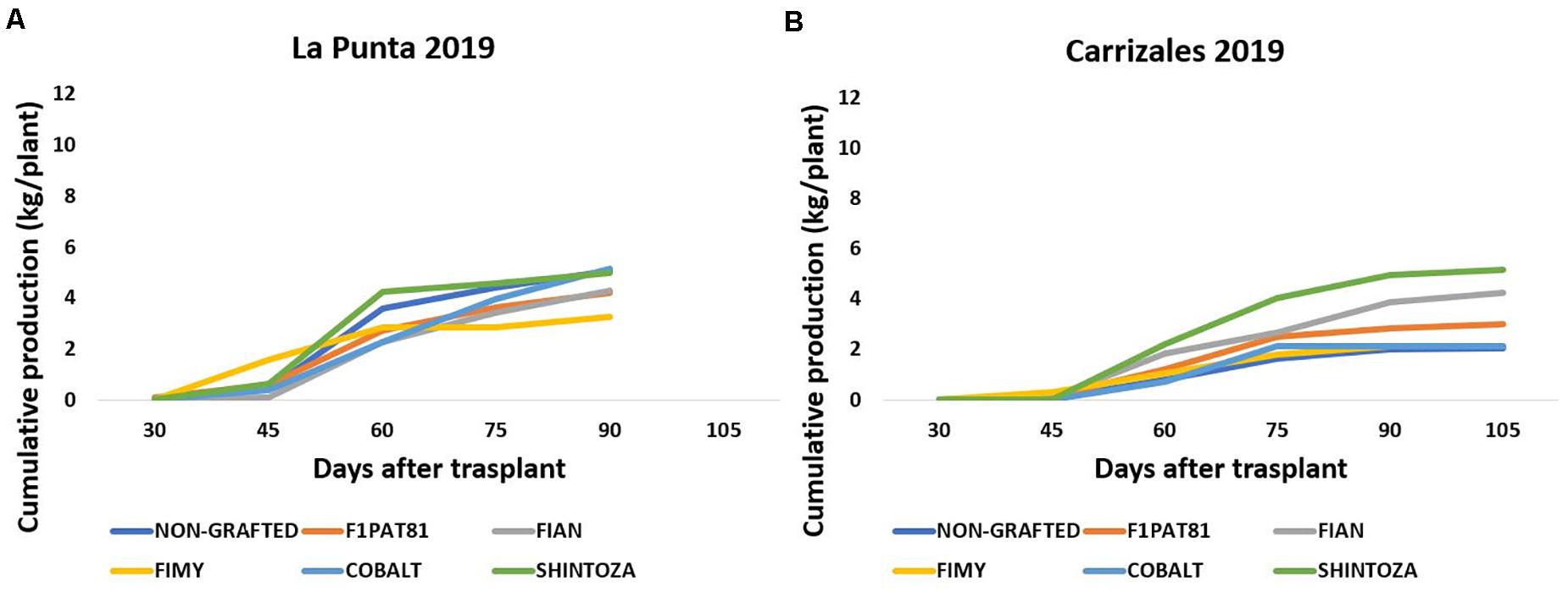
Figure 5. Cumulative production (kg plant–1) of snake melons in 2019 from non-grafted plants and plants grafted on commercial Cucurbita hybrids (Cobalt and Shintoza), the C. melo hybrid F1Pat81, and hybrids between C. ficifolius and C. anguria (Fian) or C. myriocarpus (Fimy) in the two sites of cultivation La Punta (A) and Carrizales (B). Sixteen plants were cultivated per field and treatment. All fruits of marketable size were weighted at the time of harvest to estimate total yield per plant.
No significant effects were found regarding fruit traits in grafted plants compared to the NG ones, except for a reduction in °Brix of the fruits harvested from plants grafted onto Shintoza in Carrizales (Table 3). Again, differences between fields were mainly associated with °Brix, higher in Carrizales, and fruit color, with a more yellowish fruit with less firm flesh in La Punta (Table 3).
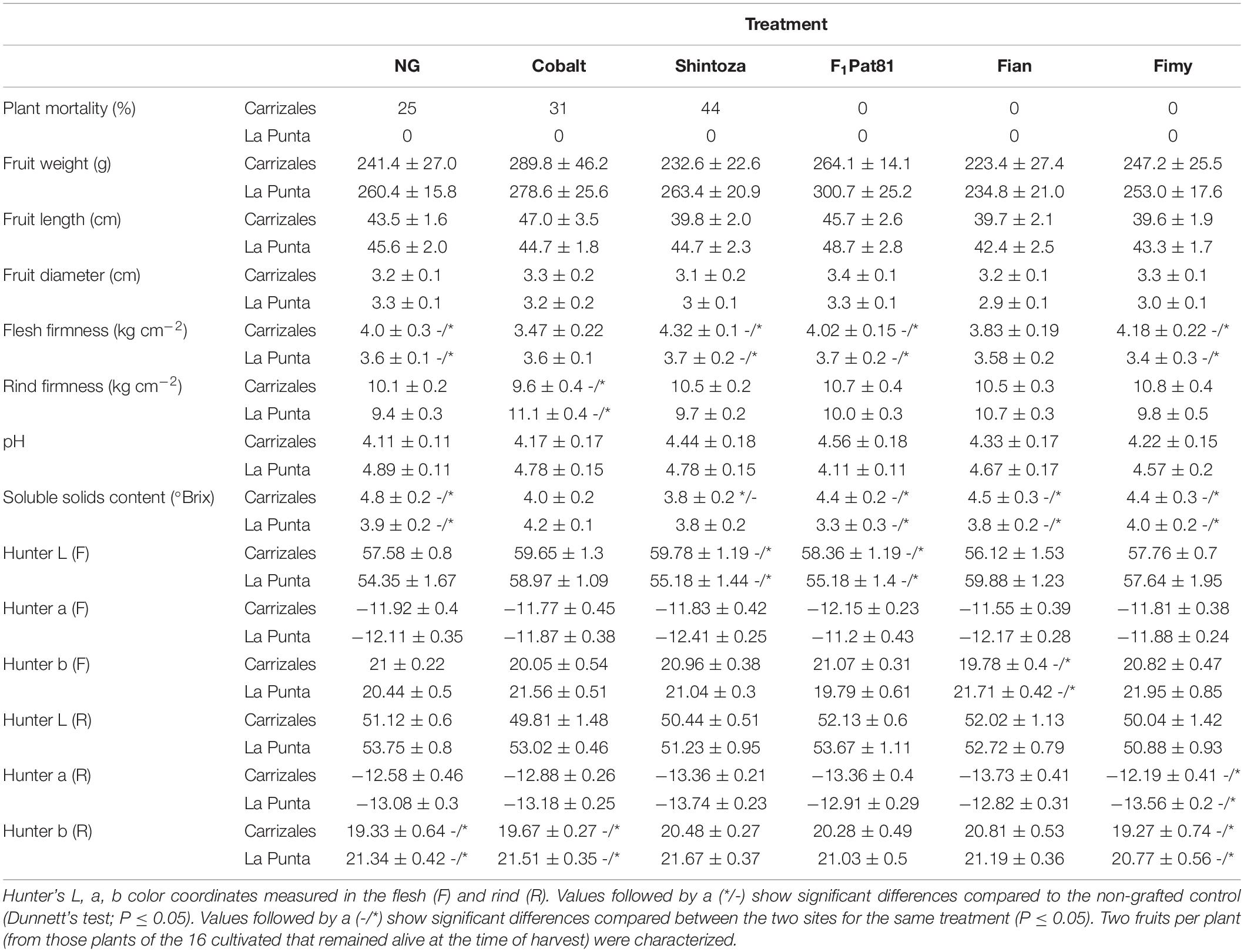
Table 3. Agronomic performance and fruit characterization of the non-grafted control compared to plants grafted onto two commercial Cucurbita hybrids (Cobalt and Shintoza), the C. melo hybrid F1Pat81, and hybrids between C. ficifolius and C. anguria (Fian) or C. myriocarpus (Fimy) grown in the two sites of cultivation (La Punta and Carrizales) in 2019.
Sensory Evaluation
Four sensory evaluations were performed in 2018, two with fruits from La Punta and two with fruits from Carrizales (Figure 6). Except for the second evaluation using fruits from La Punta, which did not result in significant differences among treatments, panelists gave higher scores of flavor acceptability to the fruits from NG plants compared to the fruits from plants grafted onto F1Pat81 and Cobalt, which had similar scores. Therefore, an effect of grafting on consumer acceptability was found in fruits from both fields.
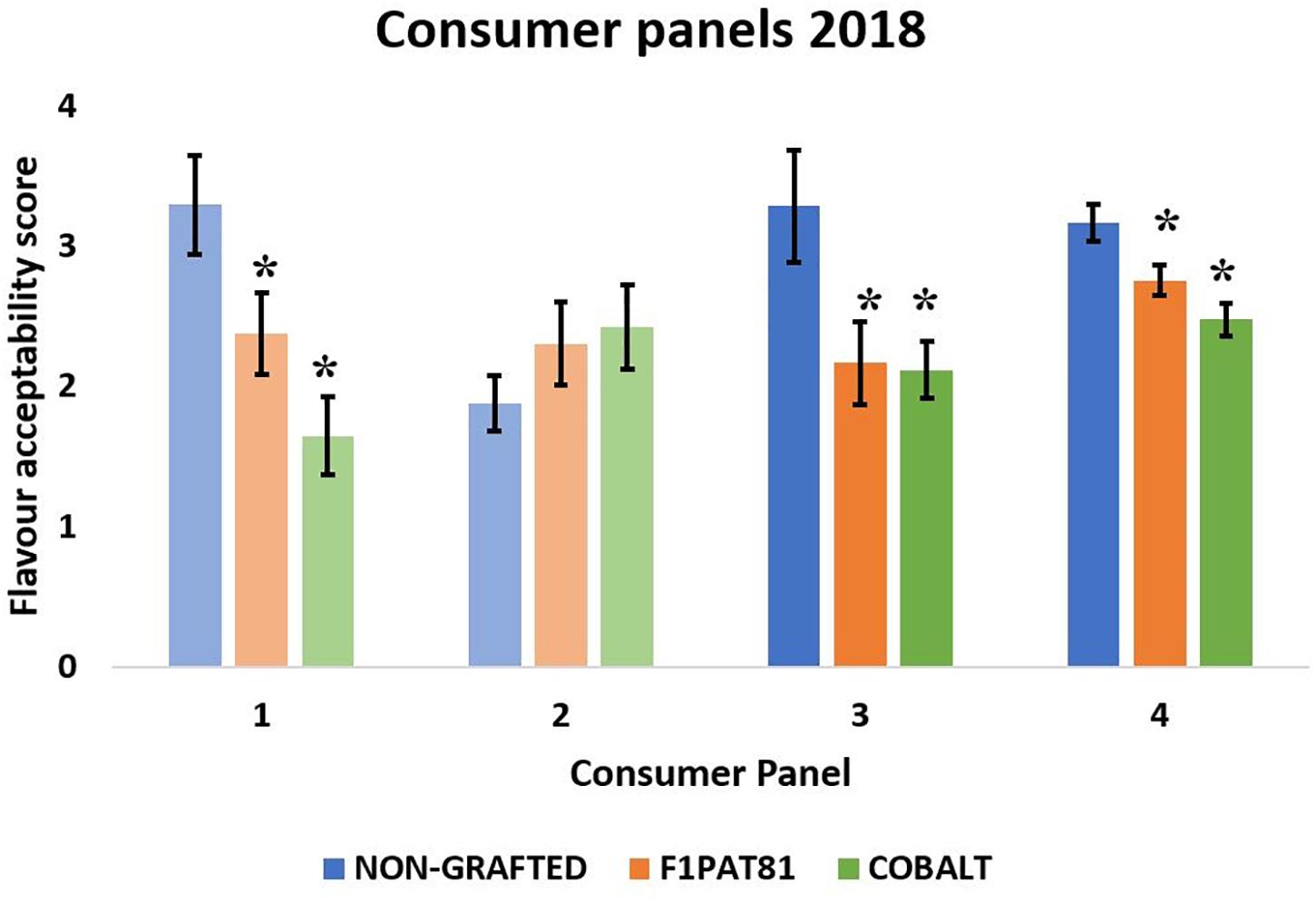
Figure 6. Mean flavor acceptability (1 being the lowest and 5 being the highest) obtained in the sensory evaluations of 2018. Columns of lighter color indicate that the fruit samples were from La Punta and those of darker color indicate that they are from Carrizales. Values followed by (*) show significant differences compared to the NG control (Dunnett’s test, P ≤ 0.05). Averages are calculated with the mean scores of 20 panelists on three biological replicates (fruit samples) of each treatment.
Five sensory evaluations were performed in 2019 (represented as 1–5 in Figure 7), two comparing fruit from the NG control with those from plants grafted onto two Cucumis rootstocks, the melon F1Pat81 and Fian (sensory evaluations 1 and 5 for La Punta and Carrizales, respectively), and three comparing the NG with the melon F1Pat81 and the Cucurbita rootstock Shintoza (sensory evaluations 2 and 3 for La Punta and 4 for Carrizales). This time, flavor, texture, and aroma were scored independently. Significant differences were found for flavor scores, but not for aroma and texture; the Shintoza rootstocks had consistently lower scores compared to NG and F1Pat81, and the F1Pat81 and Fian fruits from Carrizales were less valued than NG snake melons.
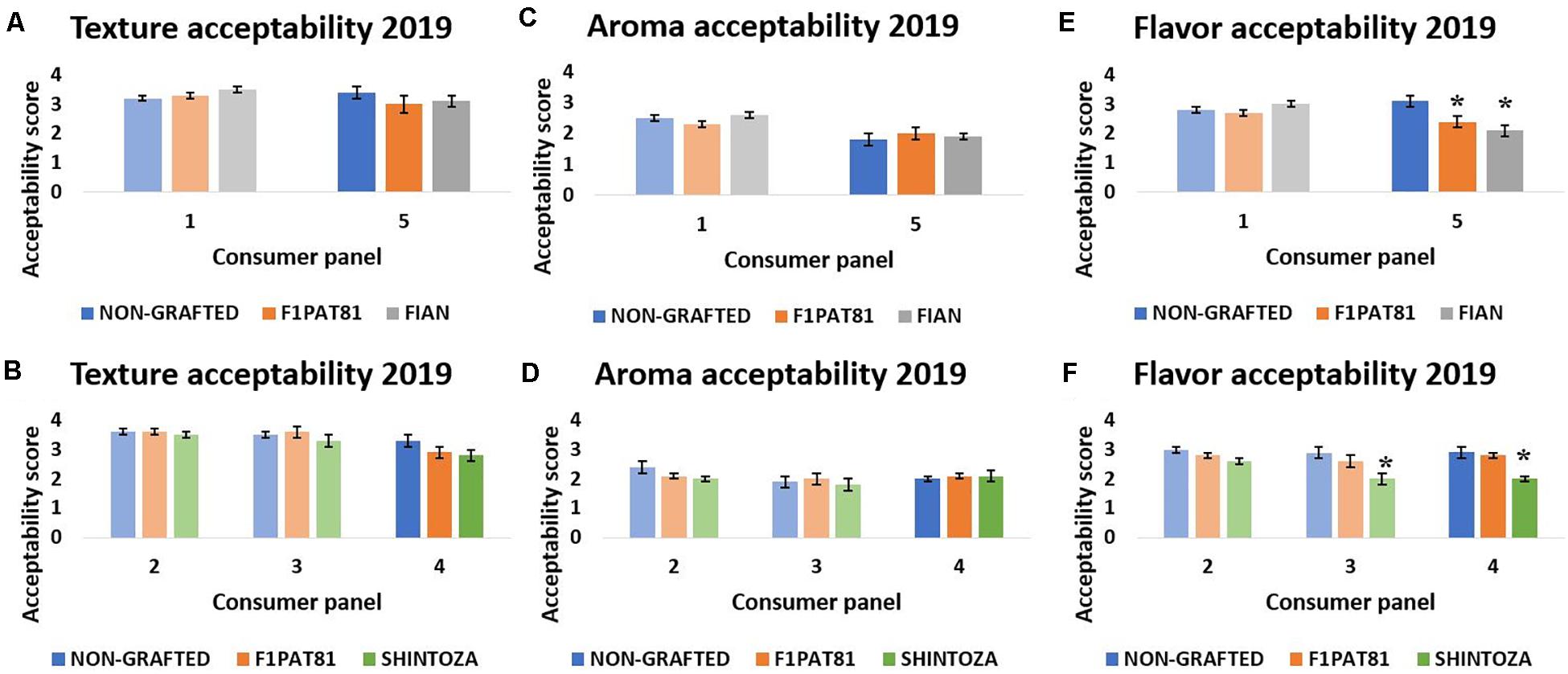
Figure 7. Mean texture, aroma, and flavor acceptability scores (1 being the lowest and 5 being the highest) obtained in the sensory evaluations of 2019. Columns of lighter color indicate that the fruit samples were obtained from La Punta and those of darker color indicate that they were obtained from Carrizales. Columns with (*) show significant differences compared to the NG control (Dunnett’s test, P ≤ 0.05). Averages are calculated with the mean scores of 20 panelists on three biological replicates (fruit samples) of each treatment. (A,C,E) Correspond to sensory evaluations 1 and 5. (B,D,F) Correspond to sensory evaluations 2, 3, and 4.
Accumulation of Sugars, Acids, and Volatiles
Since the sensory evaluations suggested differences in acceptability, we used samples of the evaluation of 2019 to determine sugar and acid accumulation and volatile compounds. The sugar and acid profiles are shown in Figure 8. In general, snake melon fruits have similar levels of fructose and glucose, whereas sucrose levels remained under the limit of detection, expected for a non-sweet fruit. Malic acid was predominant in the samples, with contents up to 20 times higher than those of citric acid.
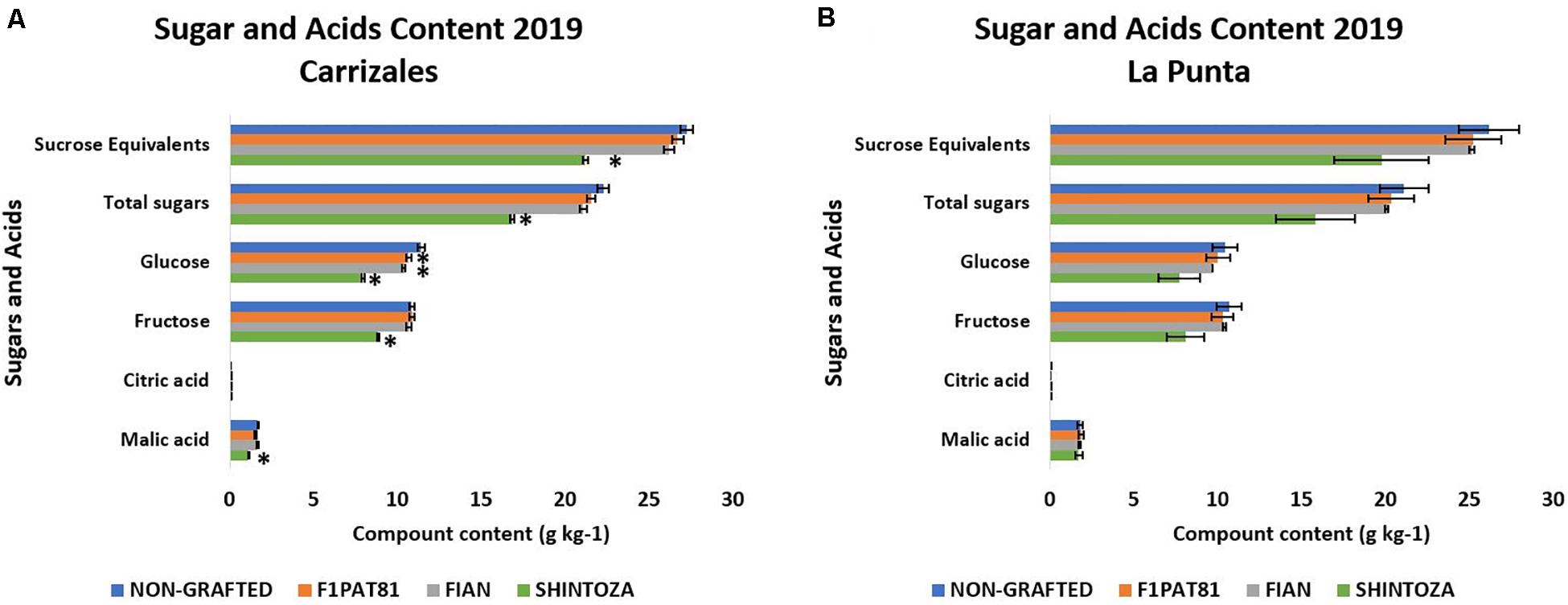
Figure 8. Accumulation of sugars and acids in fruits from non-grafted and grafted (Shintoza, F1Pat81, and Fian) snake melon plants from Carrizales (A) and La Punta (B). Columns with (∗) show significant differences with respect to the NG control (Dunnett’s test, P ≤ 0.05). Averages were calculated from the biological replicates used in the sensory evaluations. From those treatments included in the five sensory evaluations of 2019, NG and F1Pat81, averages were calculated from six and four biological replicates, respectively, from the three sensory evaluations of la Punta and the two sensory evaluations of Carrizales. Four and three biological replicates were averaged, respectively, for the Shintoza treatment, included in two sensory evaluations from La Punta and one sensory evaluation from Carrizales. Two biological replicates were averaged for the Fian treatment from each of the two sensory evaluations in which this treatment was included (one from La Punta and one from Carrizales).
Grafting had a higher impact in the sugar content than in the organic acid profile (Figure 8). This effect was significant in Carrizales. Although the same trend seemed evident in La Punta, a higher variability hindered the identification of significance. The rootstock that had a higher impact on sugar accumulation was the Cucurbita rootstock Shintoza, with a significantly lower amount of both glucose and fructose; malic acid was also reduced with this rootstock. Sugar reduction also occurred, although to a lesser extent, in Cucumis rootstocks, both F1Pat81 and Fian, but only glucose content was significantly affected. In fact, this reduction did not affect the sucrose equivalents, a variable that accounts for the sweetening power of each sugar and which is more related to sweetness perception.
Regarding VOCs, aldehydes represented, by far, the main compounds of the snake melon aroma profile, followed by alcohols, and a very low amount of esters, as expected for a low-aroma melon, and apocarotenoids (Figure 9). The alcohol profile was rich in 2-phenylethanol, associated to floral odor (Table 4). The ester compounds, which are the major contributors to the aroma of sweet melons, were nearly absent in this immature fruit, with ethyl butanoate, the major ester contributor to melon aroma, being the most abundant, although in very low amounts. The apocarotenoid beta-ionone was the only one consistently detected, but in very low amounts.
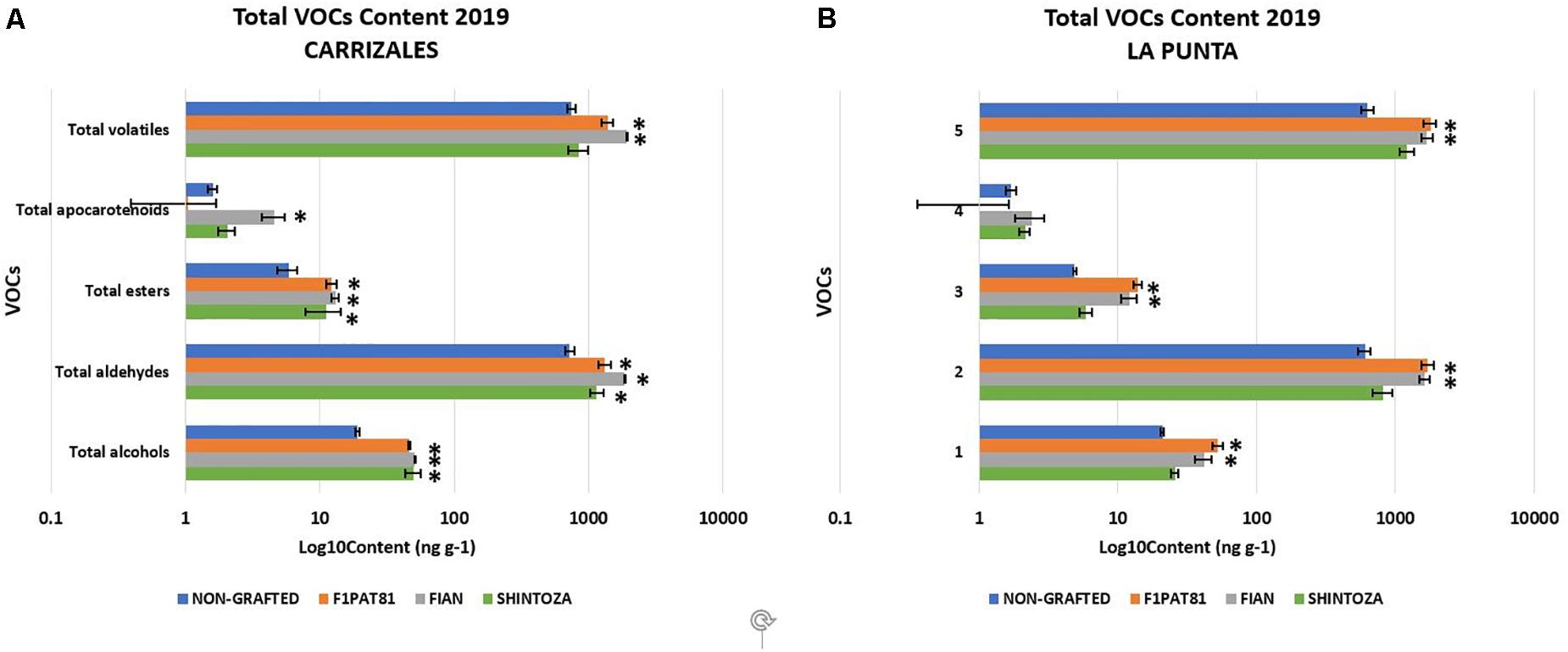
Figure 9. Accumulation of volatile organic compounds in fruits from non-grafted and grafted (Shintoza, F1Pat81, and Fian) snake melon plants from Carrizales (A) and La Punta (B). Samples obtained from the sensory analyses performed in 2019. Columns with (∗) show significant differences with respect to the NG control (Dunnett’s test, P ≤ 0.05). Averages were calculated from the biological replicates used in the sensory evaluations as described in legend of Figure 8.
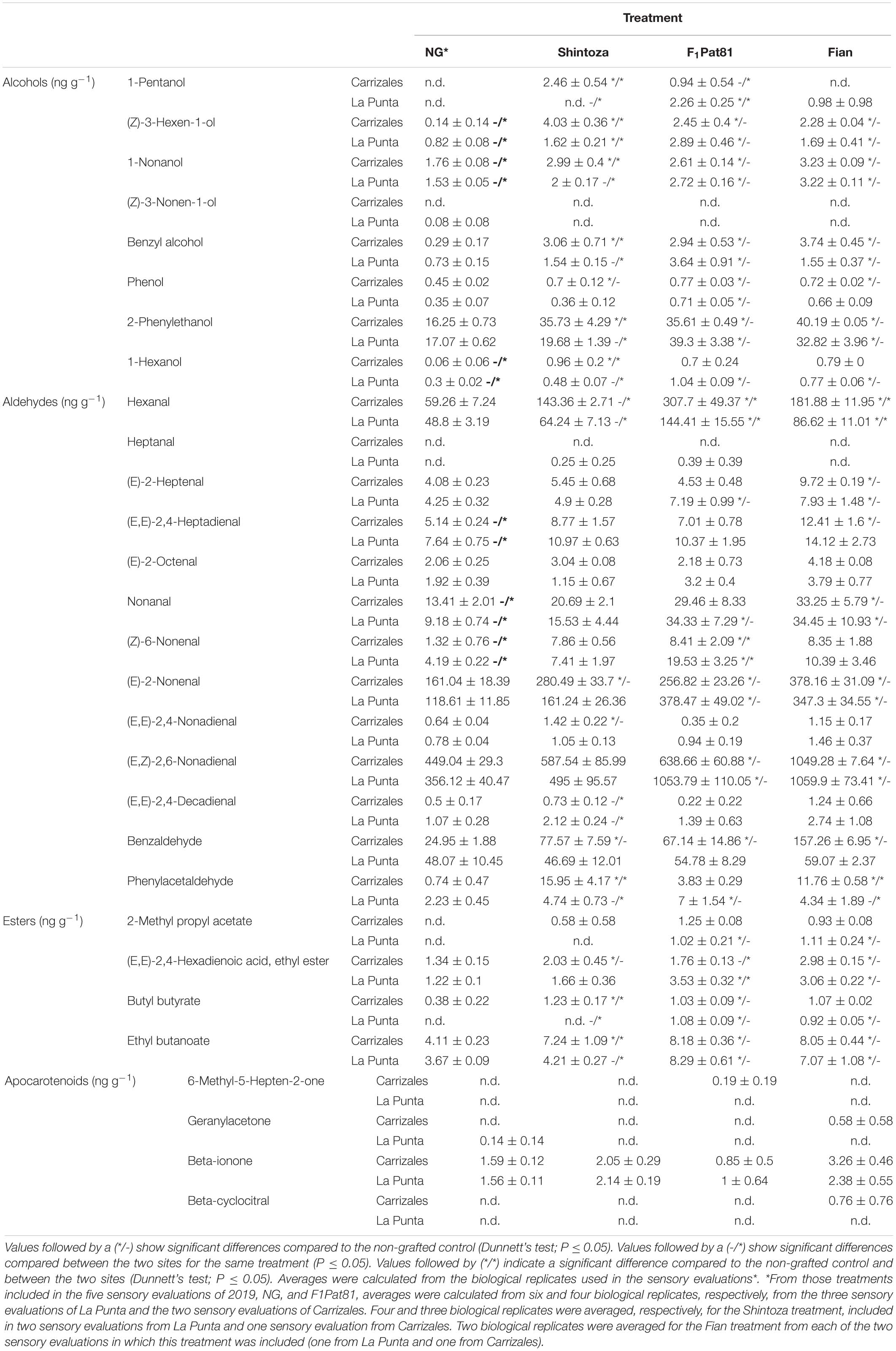
Table 4. Detailed accumulation of volatile organic compounds (VOCs) in alficoz fruits from plants non-grafted (NG) and grafted onto F1PTA81, Shintoza, and Fian harvested in the 2019 campaign.
A significant impact of grafting on the total VOCs was observed in both fields (Figure 9), with the Cucumis rootstocks being those that produced fruits with higher VOC content. The fruits produced in plants grafted onto these two rootstocks had VOC profiles with more aldehydes, more alcohols, and more esters. The Cucurbita rootstock also increased volatile content, especially alcohols and aldehydes, although this increase was not so important as that of Cucumis rootstocks and was only significant in Carrizales.
Principal component analyses showed that the different fruit samples were grouped according to their VOC profiles in both fields (Figure 10). Consistently, the samples from NG plants were grouped apart from those obtained from grafted plants, especially those grafted onto Cucumis. They were separated according to the first component that explained more than the 50% of the observed variation. This effect was observed in both fields, and fruits grafted onto Cucumis rootstocks, both the melon F1Pat81 and the wild Fian, resulted in fruits richer in most volatile compounds. This was also true for fruits produced by plants grafted onto the Cucurbita rootstock, but the effect of this rootstock was dependent on the field assay, having a similar effect to that of the F1Pat81 in Carrizales, but with less impact on plants grown in La Punta. The second component, which explained 15% of the observed variation, separated fruits produced in F1Pat81 grafted plants from those produced on Fian grafted plants, with the latter having higher contents in some aldehydes and apocarotenoids (Figure 10).
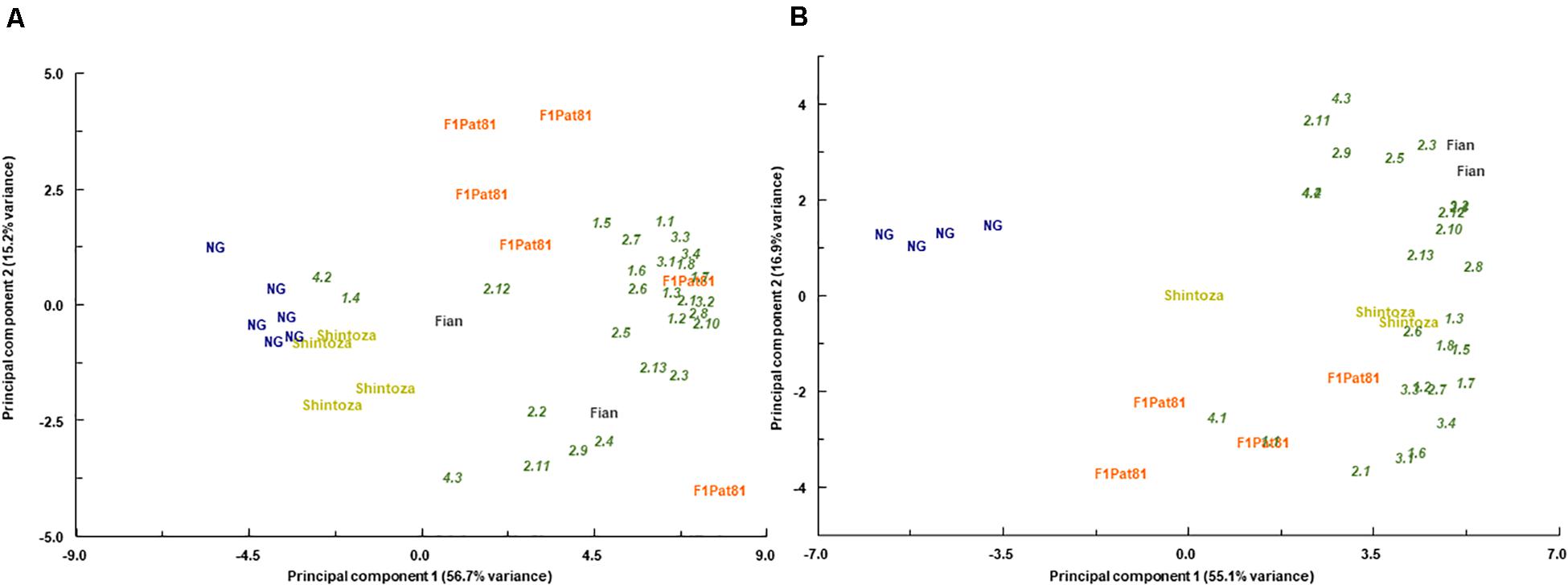
Figure 10. Biplots of scores (bold) and loadings (italics) obtained in the principal component analyses performed with the contents of volatile organic compounds in fruits from non-grafted (NG) and grafted (Shintoza, F1Pat81, and Fian) grown at La Punta (A) and Carrizales (B). Samples obtained from the sensory analyses performed in 2019 as described in legend of Figure 8. 1_1, 1-pentanol; 1_2, (Z)-3-hexen-1-ol; 1_3, 1-nonanol; 1_4, (Z)-3-nonen-1-ol; 1_5, benzyl alcohol; 1_6, phenol; 1_7, phenylethanol; 1_8, 1-hexanol; 2_1, hexanal; 2_2, heptanal; 2_3, (E)-2-heptenal; 2_4, (E,E)-2,4-heptadienal; 2_5, (E)-2-octenal; 2_6, nonanal; 2_7, (Z)-6-nonenal; 2_8, (E)-2-nonenal; 2_9, (E,E)-2,4-nonadienal; 2_10, (E,Z)-2,6-nonadienal; 2_11, (E,E)-2,4-decadienal; 2_12, benzaldehyde; 2_13, phenylacetaldehyde; 3_1, 2-methyl propyl acetate; 3_2, (E,E)-2,4-headienoic acid, ethyl ester; 3_3, butyl butyrate; 3_4, ethyl butanoate; 4_1, 6-methyl-5-hepten-2-one; 4_2, geranylacetone; 4_3, beta-ionone; 4_4, beta-cyclocitral.
When analyzed in detail (Table 4), we observed in both fields that the F1Pat81 rootstock increased the content of some of the most abundant aldehydes, (E,Z)-2-6-nonadienal, E-2-nonenal, hexanal, and benzaldehyde (the latter more in Carrizales than in La Punta), of most alcohols, with the higher increase occurring in 2-phenylethanol, but also in (Z)-3-hexen-1-ol, 1-nonanol, and benzyl alcohol, and also affected ester content, doubling the amount of ethyl butanoate, compared to the NG melons. Most of these effects were also observed in fruits produced by plants grafted onto the wild melon rootstock (Fian), with some differences, the latter with a higher increase in most aldehydes, except from hexanal.
The analysis of specific products also showed the effect of the Cucurbita rootstock, Shintoza. Conversely to the consistent effect found in F1Pat81 and Fian, the Cucurbita effect was affected by the field, with the impact on VOCs being higher in Carrizales. The impact on aldehydes was less important, with a significant increase in some compounds regarding NG, but lower than that observed in the Cucumis rootstocks, for example, for main aldehydes such as (E,Z)-2-6-nonadienal and hexanal, which did not significantly increase in any of the fields. Benzaldehyde and phenylacetaldehyde, however, significantly increased in the saline conditions of Carrizales, even more than in the F1Pat81. A similar situation was found for the increase in alcohols and ethyl butanoate, which were only significant in Carrizales.
Correlation analysis performed with sensory scores and metabolite contents is shown in Figure 11. We found moderately significant correlations between flavor and aroma (R = 0.42) and between flavor and texture (R = 0.53). Flavor acceptability was positively correlated to the sugars (R = 0.56 to R = 0.63) and malic acid contents (R = 0.47), with a higher correlation being detected between flavor and glucose (R = 0.63). Significant negative correlations were found between flavor acceptability and contents of (Z)-3-hexen-1-ol (R = −0.59), benzyl alcohol (R = −0.43), 1-hexanol (R = −0.40), benzaldehyde (R = −0.50), and phenylacetaldehyde (R = −0.64).
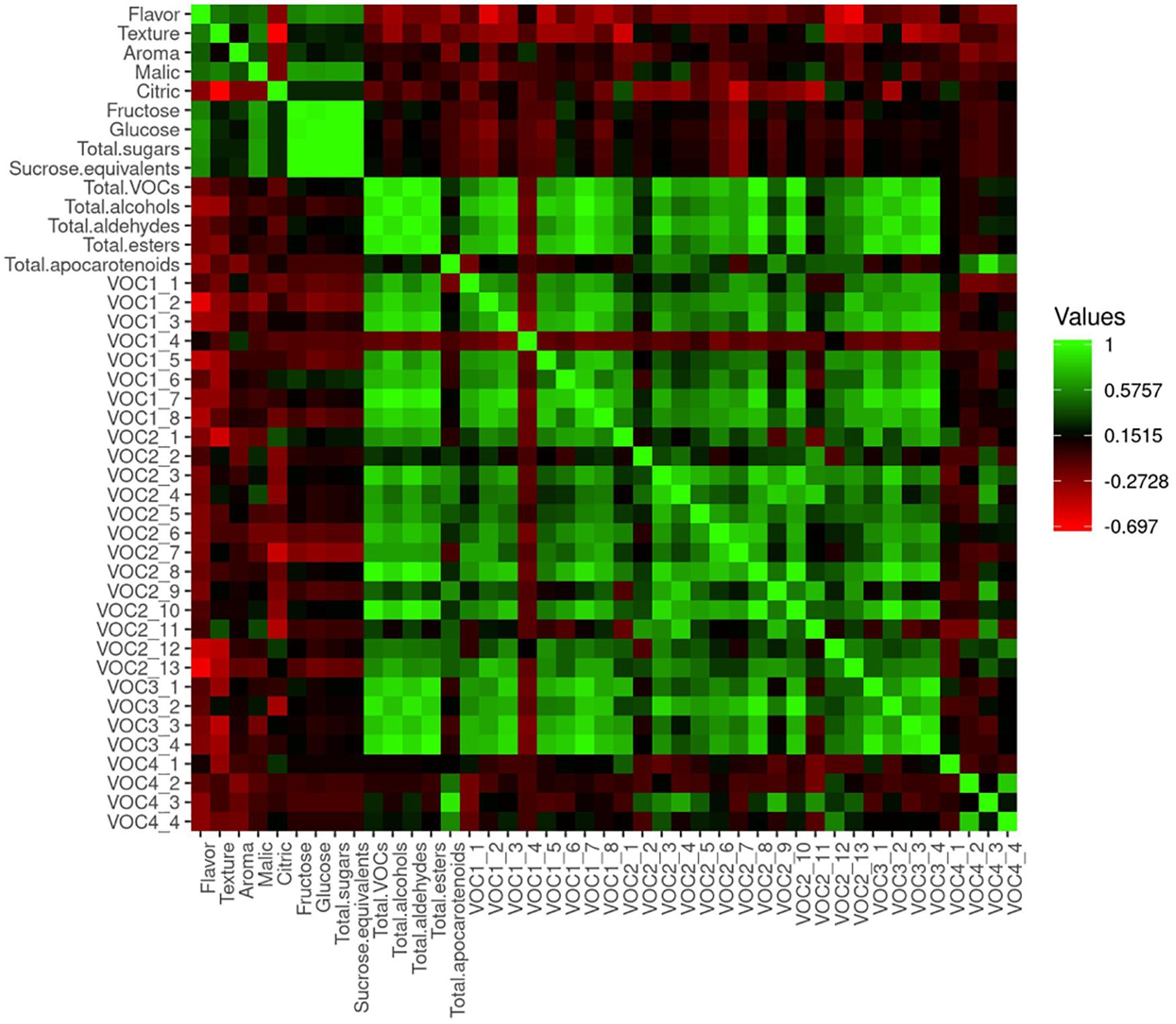
Figure 11. Heatmap of correlation analyses performed with data from the sensory evaluation and metabolite contents of the samples of 2019. 1_1, 1-pentanol; 1_2, (Z)-3-hexen-1-ol; 1_3, 1-nonanol; 1_4, (Z)-3-nonen-1-ol; 1_5, benzyl alcohol; 1_6, phenol; 1_7, 2-phenylethanol; 1_8, 1-hexanol; 2_1, hexanal; 2_2, heptanal; 2_3, (E)-2-heptenal; 2_4, (E,E)-2,4-heptadienal; 2_5, (E)-2-octenal; 2_6, nonanal; 2_7, (Z)-6-nonenal; 2_8, (E)-2-nonenal; 2_9, (E,E)-2,4-nonadienal; 2_10, (E,Z)-2,6-nonadienal; 2_11, (E,E)-2,4-decadienal; 2_12, benzaldehyde; 2_13, phenylacetaldehyde; 3_1, 2-methyl propyl acetate; 3_2, (E,E)-2,4-hexadienoic acid, ethyl ester; 3_3, butyl butyrate; 3_4, ethyl butanoate; 4_1, 6-methyl-5-hepten-2-one; 4_2, geranylacetone; 4_3, beta-ionone; 4_4, beta-cyclocitral.
Discussion
Melon production is limited by viral and fungal diseases (García-Jiménez et al., 2008; Lecoq and Katis, 2014; González et al., 2020a). Resistances to the main viruses affecting melons have been reported and introgressed into commercial cultivars. However, traditional varieties, such as snake melons, have been neglected in breeding programs, and most of them are susceptible to most viruses (Solmaz et al., 2016b; Yousif et al., 2007). In the current study, we detected several viruses in different years and fields affecting snake melon plants. The aphid-transmitted Potyvirus WMV was the most prevalent, but another potyvirus, ZYMV, and the Begomovirus ToLCNDV were also found with a lower incidence. Resistances to all these viruses are available (Sáez et al., 2017; Pérez-de-Castro et al., 2019; Martín-Hernández and Picó, 2021), but have not been introgressed into the snake melon background. Therefore, introgression programs are needed to develop snake melon cultivars with resistance to these viruses.
Regarding the incidence of fungi, global warming is favoring the increasing incidence of highly damaging fungi to melon (Cohen et al., 2012; de Sousa Linhares et al., 2020; Timmusk et al., 2020). M. phaseolina and N. falciformis were identified as major pathogens in snake melon NG plants. Our inoculation studies confirm the worse response of snake melon, compared to Piel de Sapo sweet melon, to both pathogens, being highly susceptible to M. phaseolina and MR to N. falciformis. The former has been described as a main pathogen of melon worldwide, with recently available resistant sources (Ambrósio et al., 2015; Cohen et al., 2016; de Sousa Linhares et al., 2020) and the determination of the genetic control under study. The latter has been very recently reported for the first time as a melon pathogen in Spain (González et al., 2020b, c). The high susceptibility of snake melon to Brazilian isolates of M. phaseolina compared to sweet melons was already reported by Ambrósio et al. (2015). The response of this melon to N. falciformis is reported here for the first time.
Despite the fact that plant mortality caused by these pathogens was moderate, the impact on the production was very important. The dramatic impact of diseases on snake melon production under organic farming management, almost halving yield, was confirmed in the experiment of 2018, when the production of snake melon in infested soils with previous melon cultivation (La Punta) was compared to that obtained in a soil with no previous cultivation of melon (Moncada). Despite production losses, there was not an important effect on fruit traits, other than reduction in FF. Compared to the impact of fungal attack, salts stress of Carrizales in 2018 was less damaging for snake melon plants, and productions similar to those of the unstressed control field were obtained. Therefore, snake melons seem to be much more susceptible to fungal stress than to salinity. This is consistent with the general idea that melons, like other Cucurbits, are only moderately sensitive to salinity, compared to other vegetables (Villalobos et al., 2016; Wang et al., 2016). As occurred with fungal stress, FF was slightly reduced under salt stress, which also increases SSC. These effects have been previously reported in sweet melons with higher SSC when grown at high salinity levels (Colla et al., 2006), but our results also show a similar effect even in this non-sweet melon. Also, an effect of salinity on fruit firmness has been reported (Trajkova et al., 2006; Yarsi et al., 2012).
Grafting can be used to reduce the impact of stressful conditions on snake melon production and quality. In fact, the use of Cucumis rootstocks, both melon, F1Pat81, and wild Cucumis, Fian and Fimy, reduced plant mortality. M. phaseolina, M. cannonballus, and different species of Fusarium were detected in plants cultivated in both fields. Previous studies showed that F1Pat81, Fian, and Fimy are quite tolerant to M. cannonballus and M. phaseolina (Ambrósio et al., 2015; Cáceres et al., 2017; Gisbert et al., 2020; Castro et al., 2020), although the tolerance derived from Pat 81 seems to be temperature dependent (Castro et al., 2020; de Sousa Linhares et al., 2020). The wild Cucumis are also resistant to F. oxysporum (Matsumoto et al., 2011; Gisbert et al., 2020). These tolerances may account for the reduced mortality found in snake melon plants grafted onto these rootstocks. Cucurbita rootstocks are known to have resistance to F. oxysporum and tolerance to M. cannonballus and M. phaseolina. However, in our study, plant mortality similar to that found on NG snake melon occurred in Cucurbita grafted plants. Regarding the lower performance of Cucurbita rootstocks, it should be considered that these are usually employed to control F. oxysporum. This fungus, along with M. phaseolina and M. cannonballus, were detected in Cucurbita roots. However, in this case, the high levels of mortality could be due to the presence of other fungi as N. falciformis and N. keratoplastica of the FSSC (O’Donnell et al., 2008; González et al., 2020a), as F. solani has been found to very seriously affect the Cucurbita hybrid rootstocks in watermelon (Armengol et al., 2000). It is then necessary to include these new pathogens in the selection process of new rootstocks for melon to overcome future pathogenic problems in these Cucurbita species, due to the possible spread of these soilborne fungi in our producing areas.
As stated before, grafting onto the melon F1Pat81 and wild Cucumis rootstocks reduced consistently plant mortality in different agroecological conditions, but the impact on the production per plant was variable between years and fields, and similar to that caused by the Cucurbita rootstocks. Despite the variability, in most cases, grafted plants displayed lower production losses than NG, except those grafted onto the Fimy rootstock, which were less productive. Under salt stress, grafting significantly increased production per plant, as occurred in 2018 in Carrizales, with similar effect of melon and Cucurbita rootstocks. The combined effect of salinity and fungal stress, and the environmental conditions that favored the stressful scenario, caused the highest impact on production in Carrizales in 2019. Increased susceptibility to soil borne diseases has been reported in tomato under high salinity (Bai et al., 2018). Also, in melons, enhanced fungal damage has been reported under saline conditions (Roustaee et al., 2012; Mirtalebi and Banihashemi, 2019). Grafting contributed to alleviate the impact of these extreme conditions. The lower performance of F1Pat81 in these conditions could be related to the higher temperatures, as the level of resistance against M. phaseolina and M. cannonballus of materials derived from F1Pat81 drop at higher temperatures (Castro et al., 2020; de Sousa Linhares et al., 2020). Therefore, grafting is a good strategy to reduce plant mortality due to fungal stress and can alleviate yield losses, depending on the resistance of the rootstocks and the fungal profile of the soil, and increase production under saline conditions.
The use of grafting in melon is prevented by the impact that the different rootstocks can have on fruit quality (Fita et al., 2007; Fallik and Ilic, 2014; Fredes et al., 2017; Leonardi et al., 2017). In the case of snake melons, the effect of the different rootstocks on fruit traits, such as shape, firmness, pH, or Brix degree, was minimum for both Cucumis and Cucurbita rootstocks, and only limited effects in certain combinations and environments were detected. Most of these mild effects were associated to the flesh and rind color. Variable effects on fruit color have also been reported for different types of rootstocks in sweet melons (Cáceres et al., 2017). The increase in Brix degree observed in NG snake melons cultivated under salt pressure in Carrizales was also consistently observed both years in grafted plants, which is more intense in plants grafted onto Cucumis rootstocks.
Even when no effect of rootstocks on basic fruit traits is detected, grafting can alter metabolite profiles, which may affect consumers’ preferences. Trained sensory panels have been successfully used for the evaluation of sweet melons (Bianchi et al., 2016; Park et al., 2018; Ayres et al., 2019), Spanish sweet melons (traditional landraces) (Escribano and Lázaro, 2012), and even snake melons (Omari et al., 2018). Training such panels is time-consuming and much more difficult to implement in neglected crops such as snake melon. Despite these difficulties, in this study, they consistently showed preferences for snake melon fruits produced in NG plants. In this sense, Cucurbita hybrid rootstocks clearly scored below the NG control in most sessions, while the Cucumis rootstock F1Pat81 showed no significant differences with the NG control. It is also important to note that some tasters reported fibrous or course textures and strange tastes for the Cucurbita grafted plant fruits.
We performed analysis of sugars, acids, and volatiles to evaluate if these consumer preferences might be associated to metabolic profile differences due to grafting. Snake melons do not accumulate sucrose, or present it at very low levels. Burger et al. (2003) related this trait with a high acid invertase activity that prevents the accumulation of sucrose and described the recessive gene suc as responsible for high sugar accumulation in sweet melons. The fruits analyzed in this work have shown this profile, with sucrose remaining under the limits of quantification and limited accumulation of hexoses. The main acids detected in our snake melon fruits were citric and malic, with malic being predominant. Burger et al. (2003) described the same profile in the variety “Faqqous” of snake melon, with high acid values being conferred by the dominant gene So. Snake melons (along with Indian Momordica and Acidulus melons) are considered sour melons and, in contrast to sweet melons, have malic acid as the main organic acid. The non-sweet and acidic profile found in our snake melon is typical of the So/So, Suc/Suc combination, described in other C. melo var. flexuosus varieties (Burger et al., 2003). Cohen et al. (2014) identified the gene CmPH with a major effect on fruit acidity. They found a high acid profile in the cultivars that lacked a four-amino acid duplication in this gene, among them the flexuosus melons.
Grafting seems to affect the sugars and organic acid profile, with this effect being more accentuated with Cucurbita rootstocks that significantly decrease both hexoses, affecting sucrose equivalents and malic acid. Melon rootstocks did not alter malic acid content, and the effect on sugars was only significant for glucose, not affecting sucrose equivalents. The sugar and organic acid contents can affect consumer acceptability. In fact, flavor scores of sensory evaluations were positively correlated with sugars and malic acid contents.
Snake melons are climacteric melons, aromatic when fully mature (Esteras et al., 2018), but we characterized the aroma profile at commercial maturity, when fruits are physiologically immature. At this ripening stage, VOC profile was more like that of non-climacteric melons, very rich in aldehydes, followed at a considerable distance by alcohols, and low levels of esters and apocarotenoids. The aldehyde profile of the snake melon was characterized by high contents of (E,Z)-2-6-nonadienal, followed by E-2-nonenal, hexanal, and benzaldehyde, which differ from the aldehyde profile usually found on aromatic and non-aromatic sweet melons. These compounds are known to be some of those with the main impact in melon aroma (Gonda et al., 2016). (E,Z)-2-6-nonadienal is reported to contribute with cucumber-like odor, whereas E-2-nonenal and hexanal contribute with green and fresh odor notes. This profile differs from that of sweet climacteric melons, poorer in these aldehydes, but also from other non-climacteric melons, such as the inodorus group, rich in aldehydes, but with a different aldehyde profile, richer in hexanal (Esteras et al., 2018). The alcohol profile was also different to that of aromatic sweet melons, richer in hexanol, stale odor, and fermented notes, and Z-3-hexen–1-ol, grassy-green odor, instead of 2-phenylethanol, floral odor, the main alcohol present in snake melons. The ester compounds, which are the major contributors to the aroma of sweet melons, are nearly absent in this immature fruit, with ethyl butanoate being a major ester contributor to melon aroma and the most abundant, although in very low amounts compared to sweet melons. Also, the apocarotenoid beta-ionone was the only consistently detected, but in very low amounts. This profile was similar to that reported for the “Cai Gua” snake melon by Tang et al. (2015) and Chen et al. (2016), who also reported high levels of phenylethanol among alcohols, and (E-Z)-2-6-nonedienal, (E)-2-nonenal, hexanal, and benzaldehyde among aldehydes.
There was a clear impact of grafting on VOC profile, with a higher effect in Cucumis vs Cucurbita rootstocks. Grafting onto Cucumis rootstocks resulted in fruits with a VOC profile richer in most aldehydes, alcohols, and esters. This effect was lower and more dependent on the field conditions in Cucurbita rootstocks. Flavor acceptability showed significant negative correlations with some of these compounds, (Z)-3-hexen-1-ol, benzyl alcohol, and 1-hexanol, among alcohols, and phenylacetaldehyde and benzaldehyde, among aldehydes. All grafted plants had significant increases of most of these compounds compared to NG plants. The increase was higher for Cucurbita vs Cucumis rootstocks in Carrizales for (Z)-3-hexen-1-ol, the compound with the highest negative correlation with flavor acceptability. The increase in the two aldehydes more negatively correlated with flavor acceptability, phenylacetaldehyde and benzaldehyde, was more important in Cucurbita in the Carrizales assay, associated with the lower scoring of fruits produced by plants grafted onto these rootstocks in the sensory evaluations.
Our results showed that Cucurbita rootstock has a higher impact in sugar and organic acid profile than in VOC profile, resulting in a less favorable consumer perception, and that Cucumis rootstocks affect VOC profile more than sugar and acid profile, which may result in a lower effect on consumer perception, although the increase in specific alcohols and aldehydes could also be related to the less positive perception of consumers of fruits coming from grafted plants.
Conclusion
Snake melon seems to be moderately susceptible to biotic stress and especially to soilborne diseases. Nonetheless, under high incidence conditions, yield losses can be higher than 50%. This yield loss would be even higher when combined with high salinity, due to a synergic effect. In organic farming, strategies against diseases are limited, and in this context, the use of rootstocks seems to be an efficient alternative. The use of Cucumis rootstocks seems to be more favorable. F1Pat81 not only reduced yield losses under biotic stress but also increased yield under salt stress. Nonetheless, grafting may have a side effect on consumer acceptability. This effect seems to be related to changes in the profiles of the analyzed metabolites caused by grafting, but it depends on the specific scion–rootstock combination. Cucumis rootstocks had a major effect on the VOC profile, but the incidence on sugar accumulation was limited compared to Cucurbita rootstocks, probably reducing the negative side impact on flavor.
Data Availability Statement
The original contributions presented in the study are included in the article/Supplementary Material, further inquiries can be directed to the corresponding author/s.
Author Contributions
BP, JC-C, SG-M, JR, and AF-L conceived and designed the research. AF-L, SG-M, and JR managed field assays, fruit characterization, and sampling with contributions from AS, MF, CL, CG, and BP. BP, CG, and AF-L performed the sensory evaluations. MD contributed to the selection of the snake melon landraces. CG contributed to the selection of the rootstocks. VG, AG-C, and CJ performed fungal isolations and identification. CL, AP-D-C, and AS contributed to the analysis of virus. JC-C and RM performed the metabolite analysis. AF-L, JC-C, and BP wrote the manuscript with important contributions from CG, AP-D-C, VG, and AG-C. All authors critically read and approved the final manuscript.
Funding
This work was supported by the Spanish Ministerio de Ciencia, Innovación y Universidades, cofunded with FEDER funds [project nos. AGL2017-85563-C2-1-R and RTA2017-00061-C03-03 (INIA)], and by PROMETEO project 2017/078 (to promote excellence groups) by the Conselleria d’Educació, Investigació, Cultura i Esports (Generalitat Valenciana). AF-L is a recipient of a predoctoral fellowship from Generalitat Valenciana.
Conflict of Interest
The authors declare that the research was conducted in the absence of any commercial or financial relationships that could be construed as a potential conflict of interest.
Acknowledgments
The authors would like to thank Cristina Saez, Gorka Perpiñá, Mercedes Valcarcel, and Eva Martínez for their technical support.
Supplementary Material
The Supplementary Material for this article can be found online at: https://www.frontiersin.org/articles/10.3389/fpls.2021.613845/full#supplementary-material
Supplementary Figure 1 | Three fields used for melon cultivation: Moncada (Valencia) (A), La Punta (Valencia) (B), and Carrizales (Alicante) (C).
Supplementary Figure 2 | Rootstock-scion union of snake melon plants grafted onto different rootstocks, F1Pat81 (A), Fian (B), Fimy (C), Shintoza (D), Cobalt (E), and non-grafted snake melon (F). All graftings were compatible and resulted in fully developed adult plants. Differences in hypocotyl diameters between scion and rootstocks can be observed, thicker in Cucurbita (D,E) and thinner in wild Cucumis (B,C) compared to the melon scion.
Supplementary Figure 3 | Mean temperature (lines) and total rainfall (columns) for each field in 2018 (A) and 2019 (B).
Supplementary Figure 4 | Pests and diseases detected in La Punta. Aphids on a snake melon plant (A). Snake melon plants with WMV (B) and ZYMV symptoms (C). Plants affected by P. xanthii (D).
Supplementary Figure 5 | Snake melon plants affected by different soil borne pathogens in Carrizales (2019), with their corresponding roots. (A,B) Non-grafted, (C,D) grafted onto Shintoza, (E,F) grafted onto Cobalt.
Supplementary Figure 6 | Response of snake melon to Monosporascus cannonballus (left) and Macrophomina phaseolina (right). Healthy root of non-inoculated control (A). Roots of plants inoculated with M. cannonballus at 30 DAI, root lesions and pruning are apparent (B). Response of snake melon to M. phaseolina. Plant inoculated with the toothpick method (C). Lesions of M. phaseolina on the stem at 15 DAI (D) and lesion on the stem of a dead plant at 30 DAI (E).
Supplementary Figure 7 | Response of snake melon to N. falciformis and N. keratoplastica. Pictures show the symptoms at 7 and 14 DAI, and the non-inoculated control.
Supplementary Table 1 | Volatiles analyzed in the fruit samples. (CAS, Chemical Abstract Service, MW: molecular weight; Chromatographic parameters obtained from GC-MS chromatograms: Rt: retention time, RI: retention index; Quan ion: quantitation ion). Retention index calculated with n-alkanes on Supelcowax 10 (bonded polyethylene glycol) capillary column. Linearity range corresponding to the real concentration of standards used for calibration.
Footnotes
- ^ www.aemet.es
- ^ http://riegos.ivia.es/red-siar
- ^ http://www.westerdijkinstitute.nl/fusarium/
- ^ http://www.heatmapper.ca
References
Abdelmohsin, M. E., El Jack, A. E., Yousif, M. T., El Naim, A. M., Ahmed, E. A., and Pitrat, M. (2015). Impact of breeding hermaphroditic melon on early production and yield: case of snake melon (Cucumis melo var. flexuosus) and Tibish (C. melo var. tibish). Int. J. Life Sci. Eng. 1, 171–176.
Abu Zaitoun, S. Y., Jamois, R. M., Shtaya, M. J., Mallah, O. B., Eid, I. S., and Ali-Shtayeh, M. S. (2018). Characterizing Palestinian snake melon (Cucumis melo var. flexuosus) germplasm diversity and structure using SNP and DArTseq markers. BMC Plant Biol. 18:246. PMID:NOiuPMID
Al-Taae, H.H.W. and Al-Taae, A.K. (2019). First molecular identification of Fusarium oxysporum causing fusarium wilt of Armenian cucumber in Iraq. in Proceedings of the IOP Conference Series: Earth and Environmental Science. Bristol: IOP
Ambrósio, M. M. Q., Dantas, A. C. A., Martínez-Perez, E., Medeiros, A. C., Nunes, G. H. S., and Picó, M. B. (2015). Screening a variable germplasm collection of Cucumis melo L. for seedling resistance to Macrophomina phaseolina. Euphytica 206, 287–300. doi: 10.1007/s10681-015-1452-x
Armengol, J., José, C. M., Moya, M. J., Sales, R., Vicent, A., and Garcia-Jiménez, J. (2000). Fusarium solani f. sp. Cucurbitae race 1, a potential pathogen of grafted watermelon production in Spain. Bull. OEPP 30, 179–183. doi: 10.1111/j.1365-2338.2000.tb00875.x
Ayres, E. M. M., Lee, S. M., Boyden, L., and Guinard, J. X. (2019). Sensory properties and consumer acceptance of cantaloupe melon cultivars. J. Food Sci. 84, 2278–2288. doi: 10.1111/1750-3841.14724
Bai, Y., Kissoudis, C., Yan, Z., Visser, R. G., and van der Linden, G. (2018). Plant behaviour under combined stress: tomato responses to combined salinity and pathogen stress. Plant J. 93, 781–793. doi: 10.1111/tpj.13800
Bianchi, T., Guerrero, L., Gratacós-Cubarsí, M., Claret, A., Argyris, J., García-Mas, J., et al. (2016). Textural properties of different melon (Cucumis melo L.) fruit types: sensory and physical-chemical evaluation. Sci. Horticult. 201, 46–56. doi: 10.1016/j.scienta.2016.01.028
Bie, Z., Azher Nawa, M., Huang, Y., Lee, J. M., and Colla, G. (2017). Introduction to Vegetable Grafting in Vegetable Grafting: Principles and Practices 216-244, eds G. Colla, F. P. érez-Alfocea, and D. Schwarz (UK: CAB International Oxfordshire).
Burger, Y., Sa’ar, U., Distelfeld, A., Katzir, N., Yeselson, Y., Shen, S., et al. (2003). Development of sweet melon (Cucumis melo) genotypes combining high sucrose and organic acid content. J. Am. Soc. Horticult. Sci. 128, 537–540. doi: 10.21273/jashs.128.4.0537
Cáceres, A., Perpiña, G., Ferriol, M., Picó, B., and Gisbert, C. (2017). New cucumis rootstocks for melon: ‘UPV-FA’ and ‘UPV-FMy’. HortScience 52, 792–797. doi: 10.21273/hortsci11791-17
Castro, G., Perpiñá, G., Esteras, C., Armengol, J., Picó, B., and Pérez-de-Castro, A. (2020). Resistance in melon to Monosporascus cannonballus and M. eutypoides: fungal pathogens associated with Monosporascus root rot and vine decline. Ann. Appl. Biol. 177, 101–111. doi: 10.1111/aab.12590
Cebolla-Cornejo, J., Valcárcel, M., Herrero-Martínez, J. M., Roselló, S., and Nuez, F. (2012). High efficiency joint CZE determination of sugars and acids in vegetables and fruits. Electrophoresis 33, 2416–2423. doi: 10.1002/elps.201100640
Chen, H., Cao, S., Jin, Y., Tang, Y., and Qi, H. (2016). The relationship between CmADHs and the diversity of volatile organic compounds of three aroma types of melon (Cucumis melo). Front. Physiol. 7:254.
Cohen, R., Elkabetz, M., and Edelstein, M. (2016). Variation in the responses of melon and watermelon to Macrophomina phaseolina. Crop Protection 85, 46–51. doi: 10.1016/j.cropro.2016.03.015
Cohen, R., Pivonia, S., Crosby, K. M., and Martyn, R. D. (2012). “Advances in the biology and management of monosporascus vine decline and wilt of melons and other cucurbits,” in Horticultural Reviews, ed. J. Janick (Hoboken, NJ: Wiley-Blackwell), 77–120. doi: 10.1002/9781118100592.ch2
Cohen, S., Itkin, M., Yeselson, Y., Tzuri, G., Portnoy, V., Harel-Baja, R., et al. (2014). The PH gene determines fruit acidity and contributes to the evolution of sweet melons. Nat. Commun. 5:4026.
Colla, G., Rouphael, Y., Cardarelli, M., Massa, D., Salerno, A., and Rea, E. (2006). Yield, fruit quality and mineral composition of grafted melon plants grown under saline conditions. J. Horticult. Sci. Biotechnol. 81, 146–152. doi: 10.1080/14620316.2006.11512041
Condurso, C., Verzera, A., Dima, G., Tripodi, G., Crinò, P., Paratore, A., et al. (2012). Effects of different rootstocks on aroma volatile compounds and carotenoid content of melon fruits. Sci. Horticult. 148, 9–16. doi: 10.1016/j.scienta.2012.09.015
Cothiere, O., González, V., Sales, E., Montaner-Otín, C., and Garcés-Claver, A. (2016). Optimización de la inoculación artificial de Fusarium oxysporum f. sp. niveum en sandía. Actas de Horticultura 74, 99–100.
Crinò, P., Lo Bianco, C., Rouphael, Y., Colla, G., Saccardo, F., and Paratore, A. (2007). Evaluation of rootstock resistance to fusarium wilt and gummy stem blight and effect on yield and quality of a grafted ‘inodorus’ melon. HortScience 42, 521–525. doi: 10.21273/hortsci.42.3.521
Dastranji, N., Shojaeiyan, A., and Falahati-Anbaran, M. (2017). Assessment of genetic diversity on snake melon (Cucumis melo var. flexuosus) using ISSR markers in Iran. Acta Horticult. 1151, 45–50. doi: 10.17660/actahortic.2017.1151.8
Davis, A. R., Perkins-Veazie, P., Hassell, R., Levi, A., King, S. R., and Zhang, X. (2008). Grafting effects on vegetable quality. HortScience 43, 1670–1672. doi: 10.21273/hortsci.43.6.1670
de Sousa Linhares, C. M., Ambrósio, M. M. Q., Castro, G., Torres, S. B., Esteras, C., de Sousa Nunes, G. H., et al. (2020). Effect of temperature on disease severity of charcoal rot of melons caused by Macrophomina phaseolina: implications for selection of resistance sources. Eur. J. Plant Pathol. 158, 431–441. doi: 10.1007/s10658-020-02083-w
Escribano, S., and Lázaro, A. (2012). Sensorial characteristics of spanish traditional melon genotypes: has the flavor of melon changed in the last century? Eur. Food Res. Technol. 234, 581–592. doi: 10.1007/s00217-012-1661-7
Esteras, C., Rambla, J. L., Sánchez, G., López-Gresa, M. P., González-Mas, M. C., Fernández-Trujillo, J. P., et al. (2018). Fruit flesh volatile and carotenoid profile analysis within the Cucumis melo L. species reveals unexploited variability for future genetic breeding. J. Sci. Food Agricult. 98, 3915–3925. doi: 10.1002/jsfa.8909
EuroStat (2019). Available online at: https://ec.europa.eu/eurostat/data/database (accessed September 2020).
Fallik, E., and Ilic, Z. (2014). Grafted vegetables – the influence of rootstock and scion on postharvest quality. Folia Horticult. 26, 79–90. doi: 10.2478/fhort-2014-0008
FAOSTAT (2018). Available online at: http://www.fao.org/faostat/es/#data (accessed September 2020).
Fita, A., Picó, B., Roig, C., and Nuez, F. (2007). Performance of Cucumis melo ssp. agrestis as a rootstock for melon. J. Horticult. Sci. Biotechnol. 82, 184–190.
Fredes, A., Roselló, S., Beltrán, J., Cebolla-Cornejo, J., Pérez-de-Castro, A., Gisbert, C., et al. (2017). Fruit quality assessment of watermelons grafted onto citron melon rootstock. J. Sci. Food Agricult. 97, 1646–1655. doi: 10.1002/jsfa.7915
García-Jiménez, J., Armengol, J., Sales, R., Jordá, C., and Bruton, B. D. (2008). Fungal pathogens associated with melon collapse in Spain. OEPP Bull. 30, 169–173. doi: 10.1111/j.1365-2338.2000.tb00873.x
Gisbert, C., Cáceres, A., Perpiñà, G., Garcés-Claver, A., González, V., Gómez-Guillamon, M. L., et al. (2020). Interspecific hybrids of wild cucumis species (‘fian’ and ‘fimy’): new rootstocks for melon highly resistant to biotic soil stress. Acta Hortic. 1294, 169–172. doi: 10.17660/actahortic.2020.1294.22
Gonda, I., Burger, Y., Schaffer, A. A., Ibdah, M., Tadmor, Y. A., Katzir, N., et al. (2016). Biosynthesis and Perception of Melon Aroma in Biotechnology in Flavor Production, eds D. Havkin-Frenkel and N. Dudai (Hoboken, NJ: John Wiley Sons, Ltd), 281–305.
González, V., Armijos, E., and Garcés-Claver, A. (2020a). Fungal endophytes as biocontrol agents against the main soil-borne diseases of melon and watermelon in Spain. Agronomy 10:820. doi: 10.3390/agronomy10060820
González, V., García-Martínez, S., Flores-León, A., Ruiz, J. J., Picó, B., and Garcés-Claver, A. (2020b). Neocosmospora keratoplastica, a relevant human fusarial pathogen is found to be associated with wilt and root rot of muskmelon and watermelon crops in Spain:epidemiological and molecular evidences. Eur. J. Plant Pathol. 156, 1189–1196. doi: 10.1007/s10658-020-01931-z
González, V., García-Martínez, S., Ruiz, J. J., Flores-León, A., Pico, B., and Garcés-Claver, A. (2020c). First report of Neocosmospora falciformis causing wilt and root rot of muskmelon in Spain. Plant Dis. 104:1256. doi: 10.1094/PDIS-09-19-2013-PDN
Guler, Z., Candir, E., Yetisir, H., Karaca, F., and Solmaz, I. (2014). Volatile organic compounds in watermelon (Citrullus lanatus) grafted onto 21 local and two commercial bottle gourd (Lagenaria siceraria) rootstocks. J. Horticult. Sci. Biotechnol. 89, 448–452. doi: 10.1080/14620316.2014.11513105
Hammer, K., and Gladis, T. (2014). Notes on infraspecific nomenclature and classifications of cultivated plants in compositae, cruciferae, cucurbitaceae, gramineae (with a remark on Triticum dicoccon Schrank) and Leguminosae. Genet. Resour. Crop Evol. 61, 1455–1467. doi: 10.1007/s10722-014-0148-8
Ibrahim, D. S. (2017). Neuroprotective effect of Cucumis melo var. flexuosus leaf extract on the brains of rats with streptozotocin-induced diabetes. Metabolic Brain Dis. 32, 69–75. doi: 10.1007/s11011-016-9886-y
Koehler, H., and Kays, S. A. (1991). Sweet potato flavor: quantitative and qualitative assessment ofoptimum sweetness. J. Food Qual. 14:241–249.
Kyriacou, M. C., Leskovar, D. I., Colla, G., and Rouphael, Y. (2018). Watermelon and melon fruit quality: the genotypic and agro-environmental factors implicated. Sci. Horticult. 234, 393–408.
Lecoq, H., and Katis, N. (2014). Control of cucurbit viruses. Adv. Virus Res. 90, 255–296. doi: 10.1016/B978-0-12-801246-8.00005-6
Leonardi, C., Kyriacou, M. C., Gisbert, C., Oztekin, G. B., Mourão, I., and Rouphael, Y. (2017). Quality of Grafted Vegetables in Vegetable Grafting: Principles and Practices 216-244, eds G. Colla, F. Pérez-Alfocea, and D. Schwarz (UK: CAB International Oxfordshire).
Maroto, J. V., Pascual, B., Miguel, A., San Bautista, A., López-Galarza, S., Baixauli, C., et al. (2003). Influence of different rootstocks and cycles on serpent melon (Cucumis melo var flexuosus) production in soilless cultivation. Acta Horticult. 614, 319–322. doi: 10.17660/ActaHortic.2003.614.48
Martín-Hernández, A. M., and Picó, B. (2021). Natural resistances to viruses in cucurbits. Agronomy 11:23.
Massey, M., O’Cass, A., and Otahal, P. (2018). A meta-analytic study of the factors driving the purchase of organic food. Appetite 125, 418–427. doi: 10.1016/j.appet.2018.02.029
Matsumoto, Y., Ogawara, T., Miyagi, M., Watanabe, N., and Kuboyama, T. (2011). Response of Wild Cucumis Species to Inoculation with Fusarium oxysporum f. sp. melonis Race 1,2y. J. Jpn. Soc. Horticult. Sci. 80, 414–419. doi: 10.2503/jjshs1.80.414
Merheb, J., Pawełkowicz, M., Branca, F., Bolibok-Bra̧goszewska, H., Skarzyñska, A., Pla̧der, W., et al. (2020). Characterization of lebanese germplasm of snake melon (Cucumis melo subsp. melo var. flexuosus) using morphological traits and SSR markers. Agronomy 10:1293. doi: 10.3390/agronomy10091293
Mirtalebi, M., and Banihashemi, Z. (2019). Effect of salinity on root rot of Cucumis melo L. caused by phytophthora melonis. J. Agricult. Sci. Technol. 21, 209–220.
O’Donnell, K., Kistler, H. C., Cigelnik, E., and Ploetz, R. C. (1998). Multiple evolutionary origins of the fungus causing panama disease of banana: concordant evidence from nuclear and mitochondrial gene genealogies. Proc. Natl. Acad. Sci. USA 95, 2044–2049.
O’Donnell, K., Sutton, D. A., Fothergill, A., McCarthy, D., Rinaldi, M. G., and Brandt, M. E. (2008). Molecular phylogenetic diversity, multilocus haplotype nomenclature, and in vitro antifungal resistance within the Fusarium solani species complex. Am. Soc. Microbiol. J. 46, 2477–2490. doi: 10.1128/jcm.02371-07
Omari, S., Kamenir, Y., Benichou, J. I. C., Pariente, S., Sela, H., and Perl-Treves, R. (2018). Landraces of snake melon, an ancient middle eastern crop, reveal extensive morphological and DNA diversity for potential genetic improvement. BMC Genet. 19:34.
Paris, H. S., Amar, Z., and Lev, E. (2012a). Medieval emergence of sweet melons, Cucumis melo (Cucurbitaceae). Ann. Bot. 110, 23–33. doi: 10.1093/aob/mcs098
Paris, H. S., Daunay, M. C., and Janick, J. (2012b). Occidental diffusion of cucumber (Cucumis sativus) 500–1300 CE: two routes to Europe. Ann. Bot. 109, 117–126. doi: 10.1093/aob/mcr281
Park, E., Luo, Y., Marine, S. C., Everts, K. L., Micallef, S. A., Bolten, S., et al. (2018). Consumer preference and physicochemical evaluation of organically grown melons. Postharvest Biol. Technol. 141, 77–85. doi: 10.1016/j.postharvbio.2018.03.001
Perchepied, L., and Pitrat, M. (2004). Polygenic inheritance of partial resistance to Fusarium oxysporum f. sp. melonis race 1.2 in melon. Phytopathology 94, 1331–1336. doi: 10.1094/phyto.2004.94.12.1331
Pérez-de-Castro, A., Martínez, de Alba, A. E., Sáez, C., Flores, A., Sifres, A., et al. (2019). Incidence and genetic diversity of cucurbit viruses in Spain. Acta Horticult. 1294, 203–210. doi: 10.17660/actahortic.2020.1294.25
Picó, M. B., Thompson, A. J., Gisbert, C., Yetisir, H., and Bebeli, P. (2017). “Genetic resources for rootstock breeding,” in Vegetable Grafting: Principles and Practices 22-69, eds G. Colla, F. Pérez-Alfocea, and D. Schwarz (UK: CAB International Oxfordshire).
Pitrat, M. (2008). “Melon,” in Vegetables I. Handbook of Plant Breeding, vol 1, eds J. Prohens and F. Nuez (New York, NY: Springer).
Pitrat, M. (2016). “Melon genetic resources: phenotypic diversity and horticultural taxonomy,” in Genetics and Genomics of Cucurbitaceae. Plant Genetics and Genomics: Crops and Models, vol 20, eds R. Grumet, N. Katzir, J. Garcia-Mas, New Zealand: PGG.
Primo, E., and Carrasco, J. M. (1980). Química Agrícola I. Suelos y Fertilizantes. Madrid: Alhambra S.A.
Reeb, V., Lutztoni, F., and Roux, C. (2004). Contribution of RPB2 to multilocus phylogenetic studies of the euascomycetes (Pezizomycotina, Fungi) with special emphasis on the lichen-forming Acarosporaceae and evolution of polyspory. Mol. Phylogenet. Evol. 32, 1036–1060. doi: 10.1016/j.ympev.2004.04.012
Reganold, J. P., and Wachter, J. M. (2016). Organic agriculture in the twenty-first century. Nat. Plants 2:15221.
Roig, C., Fita, A., Ríos, G., Hammond, J. P., Nuez, F., and Picó, B. (2012). Root transcriptional responses of two melon genotypes with contrasting resistance to Monosporascus cannonballus (Pollack et Uecker) infection. BMC Genomics 13:601. doi: 10.1186/1471-2164-13-601
Roustaee, A., Karimpour Reyhan, M., and Jafari, M. (2012). Study of interaction between salinity and charcoal rot diseases of melon (Macrophomina phaseolina) in semnan and garmsar. Desert 16, 175–180.
Sáez, C., Esteras, C., Martínez, C., Ferriol, M., Dhillon, N. P. S., López, C., et al. (2017). Resistance to tomato leaf curl New Delhi virus in melon is controlled by a major QTL located in chromosome 11. Plant Cell Rep. 36, 1571–1584. doi: 10.1007/s00299-017-2175-3
Saftner, R., Abbot, J. A., Lester, G., and Vinyard, B. (2006). Sensory and analytical comparison of orange-fleshed honeydew to cantaloupe and green-fleshed honeydew for fresh-cut chunks. PostHarvest Biol. Technol. 42, 150–160. doi: 10.1016/j.postharvbio.2006.02.011
Schrama, M., de Haan, J. J., Krronen, M., Verstegen, H., and Van der Putten, W. H. (2018). Crop yield gap and stability in organic and conventional farming systems. Agricult. Ecosyst. Environ. 256, 123–130. doi: 10.1016/j.agee.2017.12.023
Seufert, V., Ramankutty, N., and Foley, J. (2012). Comparing the yields of organic and conventional agriculture. Nature 485, 229–232. doi: 10.1038/nature11069
Solmaz, I., Kacar, Y. A., Simsek, O., and Sari, N. (2016a). Genetic characterization of Turkish snake melon (Cucumis melo L. subsp. melo flexuosus Group) accessions revealed by SSR Markers. Biochem. Genet. 54, 534–543. doi: 10.1007/s10528-016-9739-8
Solmaz, I., Sari, N., Dogimont, C., and Pitrat, M. (2016b). Evaluation of Turkish melon accessions for resistance to Fusarium wilt, downy mildew, powdery mildew, Cucumber mosaic virus and Zucchini yellow mosaic virus. Acta Horticult. 1127, 133–140. doi: 10.17660/actahortic.2016.1127.22
Tang, Y., Zhang, C., Cao, S., Wang, X., and Qi, H. (2015). The effect of CmLOXs on the production of volatile organic compounds in four aroma types of melon (Cucumis melo). PLoS One 10:e143567.
Timmusk, S., Nevo, E., Ayele, F., Noe, S., and Niinemets, Ü (2020). Fighting fusarium pathogens in the era of climate change: a conceptual approach. Pathogens 9:419. doi: 10.3390/pathogens9060419
Trajkova, F., Papadantonakis, N., and Savvas, D. (2006). Comparative effects of NaCl and CaCl2 salinity on cucumber grown in a closed hydroponic system. HortScience 41, 437–441. doi: 10.21273/hortsci.41.2.437
Tripodi, G., Condurso, C., Cincotta, F., Merlino, M., and Verzera, A. (2020). Aroma compounds in mini-watermelon fruits from different grafting combinations. J. Sci. Food Agricult. 100, 1328–1335. doi: 10.1002/jsfa.10149
Verzera, A., Dima, G., Tripodi, G., Condurso, C., Crinò, P., Romano, D., et al. (2014). Aroma and sensory quality of honeydew melon fruits (Cucumis melo L. subsp. melo var. inodorus H. Jacq.) in relation to different rootstocks. Sci. Horticult. 169, 118–124. doi: 10.1016/j.scienta.2014.02.008
Villalobos, F. J., Mateos, L., Quemada, M., Delgado, A., and Fereres, E. (2016). “Control of Salinity,” in Principles of Agronomy for Sustainable Agriculture, eds F. J. Villalobos and E. Fereres (Berlin: Springer International Publishing).
Wang, L. M., Zhang, L. D., Chen, J. B., Huang, D. F., and Zhang, Y. D. (2016). Physiological analysis and transcriptome comparison of two muskmelon (Cucumis melo L.) cultivars in response to salt stress. Genet. Mol. Res. 15, 1–18.
White, T. J., Bruns, T., Lee, S., and Taylor, J. (1990). Amplification and Direct Sequencing of Fungal Ribosomal RNA Genes for Phylogenetics in PCR Protocols: a Guide to Methods and Applications, eds M. A. Innis, D. H. Gelfand, and T. J. White (San Diego, CA: Academic Press).
Yarsi, G., Sari, N., and Yetisir, H. (2012). Effect of different rootstocks on the yield and quality of grafted melon plants. Acta Horticult. 936, 211–216.
Keywords: flexuosus, grafting, fruit quality, organic agriculture, soilborne pathogens
Citation: Flores-León A, García-Martínez S, González V, Garcés-Claver A, Martí R, Julián C, Sifres A, Pérez-de-Castro A, Díez MJ, López C, Ferriol M, Gisbert C, Ruiz JJ, Cebolla-Cornejo J and Picó B (2021) Grafting Snake Melon [Cucumis melo L. subsp. melo Var. flexuosus (L.) Naudin] in Organic Farming: Effects on Agronomic Performance; Resistance to Pathogens; Sugar, Acid, and VOC Profiles; and Consumer Acceptance. Front. Plant Sci. 12:613845. doi: 10.3389/fpls.2021.613845
Received: 04 October 2020; Accepted: 18 January 2021;
Published: 19 February 2021.
Edited by:
Francisco Perez-Alfocea, Spanish National Research Council, SpainReviewed by:
Halit Yetisir, Erciyes University, TurkeyLuigi Lucini, Catholic University of the Sacred Heart, Italy
Copyright © 2021 Flores-León, García-Martínez, González, Garcés-Claver, Martí, Julián, Sifres, Pérez-de-Castro, Díez, López, Ferriol, Gisbert, Ruiz, Cebolla-Cornejo and Picó. This is an open-access article distributed under the terms of the Creative Commons Attribution License (CC BY). The use, distribution or reproduction in other forums is permitted, provided the original author(s) and the copyright owner(s) are credited and that the original publication in this journal is cited, in accordance with accepted academic practice. No use, distribution or reproduction is permitted which does not comply with these terms.
*Correspondence: Belén Picó, bXBpY29zaUBidGMudXB2LmVz