- 1National Agrobiodiversity Center, National Institute of Agricultural Sciences, Rural Development Administration, Jeonju, South Korea
- 2Department of Industrial Plant Science and Technology, Chungbuk National University, Cheongju, South Korea
Recently, within the Fabaceae family, the Vicia genus has been recognized for its vital role in sustainable agriculture. Vicia species are economically important grain and forage crops. However, the presence of complex morphological characteristics makes identification and recognition of native species difficult. In this study, the possibility of using DNA barcoding regions (ITS2, matK, and rbcL) to distinguish among 19 Vicia taxa (59 accessions) found in South Korea was evaluated. The sequence alignment analysis revealed considerable nucleotide diversity (π) between the loci, in which ITS2 showed the highest mean interspecific distance, whereas there was no intraspecific variability among the barcode regions in 12 of the 19 taxa. Phylogenetic analysis of combined barcoding regions revealed well-resolved phylogeny with the highest species level discrimination. Combinations of barcode loci were also used in classification at the subgenera and section levels. The results revealed that the combined barcoding regions can be used effectively to differentiate the following species: Vicia angustifolia var. segetilis, Vicia bungei, Vicia villosa, Vicia cracca, Vicia dasycarpa, Vicia hirsuta, Vicia tetrasperma, Vicia amurensis, Vicia hirticalycina, and Vicia chosenensis. However, it is difficult to differentiate the species of Vicia unijuga, Vicia unijuga var. kaussanensis, Vicia linearifolia, Vicia unijuga f. angustifolia, Vicia nipponica, Vicia amoena, Vicia venosa var. cuspidata, Vicia pseudo-orobus, and Vicia japonica with the tested barcode regions. These species come under sect. Vicilla and are found to be closely related or species that have recently undergone speciation; thus, it has limitation to distinguish with recommended barcodes. Hence, to differentiate the unclassified species, 39 morphological characteristics were investigated, in which 16 useful characteristics were selected for efficient classification. Finally, the 16 selected morphological useful traits efficiently differentiated all the Vicia species. In conclusion, a combination of barcoding loci together with morphological characteristics of this study efficiently discriminated all the Korean Vicia species.
Introduction
The genus Vicia L. belongs to the Fabaceae (Leguminosae) family and is the third largest family of flowering plants worldwide. Approximately 150–210 species are distributed across Europe, Asia, and North America, and the majority of species is found in the Mediterranean (Cacan et al., 2016; Al-Joboury, 2017). To date, approximately 17–21 Vicia species have been reported in Korea (Lee, 1996; Choi, 2007; Nam et al., 2012). Vicia L. belongs to the Fabeae (syn. Vicieae) tribe along with Lathyrus L., Lens Mill., Pisum L., and Vavilovia Fed. (Kupicha, 1976). Furthermore, the Vicia genus is divided into two subgenera, Vicia L. (22 sections) and Cracca Peterm. [=Vicilla (SCHUR) ROUY, five sections], based on the presence of stipule nectaries, relative peduncle length, subtending leaves, and number of flowers per inflorescence (Kupicha, 1976; Leht, 2009).
Many Fabaceae species are economically important as crops used in food, herbal medicine, petroleum materials, and animal feeds (Koçak et al., 2011). The genus Vicia has the advantage of primarily containing dual-purpose crops (Francis et al., 2000). Representative Vicia species in agriculture are Vicia villosa Roth and Vicia cracca L., which have been used as green manure, cover, and forage crops. The ecological role of Vicia species such as V. villosa and Vicia angustifolia L. as hosts to nitrogen-fixing bacteria (Rhizobium leguminosarum) has the ability to fix free nitrogen from the air (Handi, 1982). It helps to fertilize the soil by the fixed nitrogen released and making it available to other plants in agricultural and natural systems. In South Korea, Vicia species are distributed in various habitats. For example, Vicia tetrasperma (L.) Schreb. and Vicia hirsuta (L.) Gray is widely distributed in range of grasslands, fields, cultivated areas, hill slopes, and roadsides, and Vicia unijuga A. Braun, Vicia linearifolia Y.N.Lee, Vicia pseudo-orobus Fisch. & C.A.Mey., and Vicia nipponica Matsum are mainly distributed in forests, mountain, and hill areas. V. unijuga var. kaussanensis H. Lév., Vicia hirticalycina Nakai, and Vicia chosenensis Ohwi are known as endemic plants of Korea, which have a restricted distribution with more threat to extinction than any other widely distributed species (Chung et al., 2017). Hence, there is an urgency of protecting the species to conserve the biological diversity. Recently, the agricultural utility and ecological roles of Vicia species have been recognized, and many studies on the genus are actively underway (Raveendar et al., 2015, 2017; Sveinsson and Cronk, 2016; Li et al., 2018; Wu et al., 2020).
Several candidate DNA regions in plastids, including matK, rbcL, and trnH-psbA spacers have been recommended for use in plant species identification (Kress and Erickson, 2007; Group et al., 2009). The rbcL and matK genes are well conserved in the plastid coding region of most plant species. They are most promising coding regions in chloroplasts for plant species identification, with low transition and transversion rates. Similarly, due to the high level of sequence divergence, trnH-psbA spacers region has been considered as the best candidate plant barcode (Hollingsworth et al., 2011). However, species discrimination has previously been impossible when using single DNA barcodes for species identification. Hence, the Consortium for the Barcode of Life Plant Working Group suggested seven candidate plastid DNA regions (atpF-atpH spacer, matK gene, rbcL gene, rpoB gene, rpoC1 gene, psbK-psbI spacer, and trnH-psbA spacer) and recommended the two-locus combination of rbcL + matK as a standard plant barcode (Group et al., 2009; Hollingsworth et al., 2011). In addition, combining nuclear [internal transcribed spacer (ITS)] and plastid (matK, rbcL, and psbA-trnH) regions has been recognized as a potential method of classifying various plant species (Chen et al., 2010; Gao et al., 2010; Purty and Chatterjee, 2016). In eukaryotes, the ITS region is present in the nuclear 45S ribosomal RNA (rDNA) genes, which can be further divided into the ITS1 and ITS2 regions. It has been proven to be the most suitable area for DNA barcoding, as it has a high efficiency of PCR amplification (93.8%) in most plant species (Chen et al., 2010). The use of multiple DNA barcodes may provide sufficient identification power for closely related species (Cold Spring Harbor DNA Learning Center, 2014).
Similarly, morphological markers have been studied in combination with molecular markers as an efficient method of species classification (Carneiro de Melo Moura et al., 2019; Xie et al., 2019; Martínez-Arce et al., 2020). Morphological data always play a key role in plant species classification based on phylogeny (Scotland et al., 2003). However, morphological studies require in-depth knowledge of characteristics or the number of species. Molecular tools using DNA barcodes for taxonomic identification, species delimitation, and access to phylogeny have the potential to overcome these difficulties faced by taxonomists (Martínez-Arce et al., 2020). However, it is very difficult to resolve recently diverged species or new species generated through hybridization if there is no universal sequence of common region as DNA barcode, and sufficient sequence divergence preserved in the domains of all living organisms is necessary for species classification (Ali et al., 2014). DNA barcoding can be used together with traditional morphology, and it is necessary to use data derived from morphology in tandem with DNA sequence data (Wen and Pandey, 2005; Ali and Choudhary, 2011; Ali et al., 2014). Thus, integrating DNA sequencing, morphology, and ecology would lead to successful species classification (Dasmahapatra and Mallet, 2006).
Morphological and DNA barcoding studies have been carried out to discriminate among Vicia species. In terms of morphology, style shape, stipule margin, and size, tendril, leaflets, flower length, peduncles, presence/absence of stipule nectaries, calyx teeth, hairs, legume shape, and ovary characteristics are known to be important for species identification (Kupicha, 1976; Jalilian et al., 2014). Karyological studies using chromosomes, pollen morphology, characters, and testa texture of seeds have also been carried out in Vicia, and these traits have been recognized as important taxonomic keys (Maxted et al., 1991; Chernoff et al., 1992; Endo and Ohashi, 1995; Nam et al., 2012). However, most of these taxonomic traits require specialized taxonomic knowledge and experience, and vast quantities of morphological information are required at each plant growth stage.
The ITS region of the nuclear genome was initially recommended for use in identifying Vicia species (Choi et al., 2006; Endo et al., 2008; Shiran et al., 2014). Furthermore, the efficiency of species identification has been evaluated using different plastid barcode regions (Raveendar et al., 2017), and 161 Vicia species have been identified using a combination of barcoding loci such as matK, rbcL, trnH-psbA, trnL-trnF, ITS1, and ITS2 (Wu et al., 2020). Relationships analysis within the Vicia genus has revealed 10 sections, including one composed of East Asian species found in Korea, using molecular and stylar features to create the phylogeny (Choi et al., 2006). However, only the ITS region has been used in barcoding, and there has been no research into the morphological features of the 19 Vicia taxa distributed in South Korea. Therefore, the aim of the present study was to confirm the suitability of various barcoding (ITS2, matK, and rbcL) regions together with morphological traits for the identification of Korean Vicia species.
Materials and Methods
Plant Materials and DNA Extraction
Samples of 19 Vicia taxa were collected from 59 different locations in South Korea (Figure 1 and Table 1). Voucher specimens were deposited to the Herbarium Conservation Center of the National Institute of Agricultural Sciences (Table 1). Barcode sequence of ITS2, matK, and rbcL for 33 Vicia species (about 88 accessions) conserved at the National Agrobiodiversity Center, South Korea were downloaded from NCBI GenBank. These data were combined with the present study to increase the efficient conservation and utilization of Vicia accessions in South Korea (Supplementary Table S1). Genomic DNA (gDNA) was extracted using DNeasy® Plant Mini kits (Qiagen, Hilden, Germany) according to the manufacturer’s instructions. Freeze-dried leaves were used for DNA extraction and were ground into a powder. DNA was resuspended in 100 ml water, and dilutions were made up to 10 ng μl–1, after which samples were stored at −20°C. DNA quality and quantity were measured using 1% (w/v) agarose gel and a spectrophotometer (Epoch, BioTek, Winooski, VT, United States).
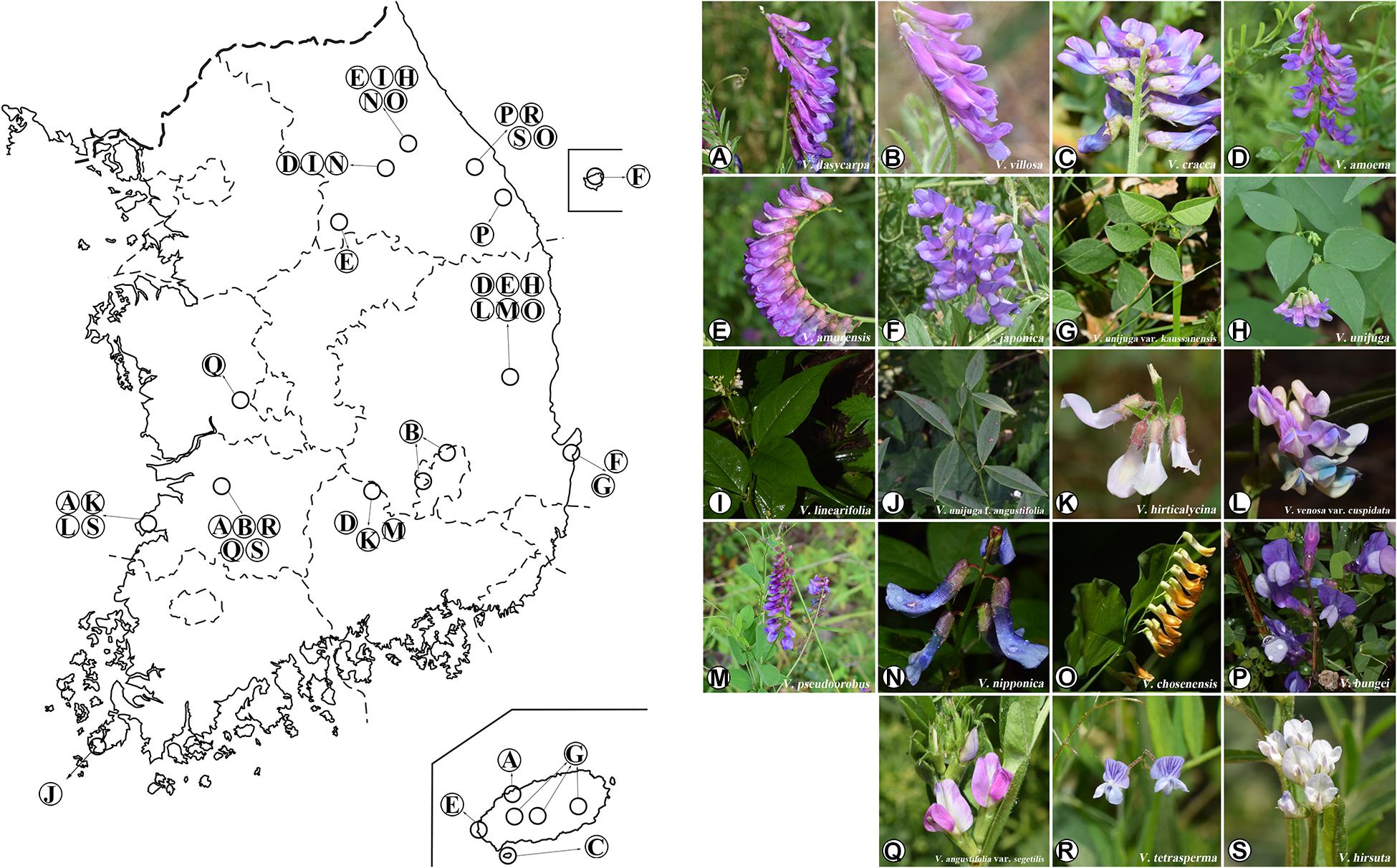
Figure 1. Collection sites of Vicia species in Korea. (A) V. dasycarpa; (B) V. villosa; (C) V. cracca; (D) V. amoena; (E) V. amurensis; (F) V. japonica; (G) V. unijuga var. kaussanensis; (H) V. unijuga; (I) V. linearifolia; (J) V. unijuga f. angustifolia; (K) V. hirticalycina; (L) V. venosa var. cuspidata; (M) V. pseudo-orobus; (N) V. nipponica; (O) V. chosenensis; (P) V. bungei; (Q) V. angustifolia var. segetilis; (R) V. tetrasperma; (S) V. hirsuta.
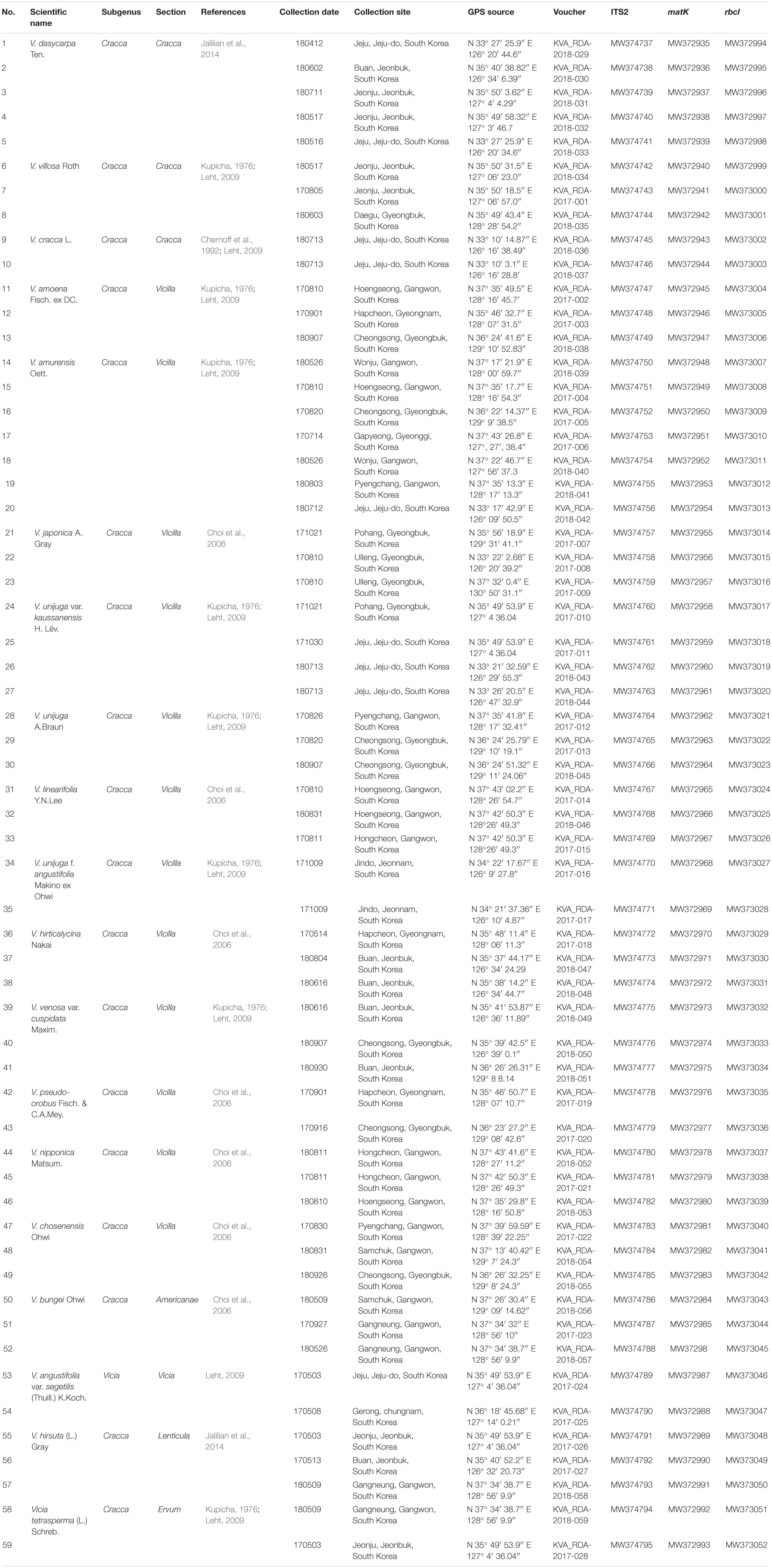
Table 1. List of plant species used in this study along with the collection site, GPS source, and voucher number.
Polymerase Chain Reaction Amplification and Sequencing
The sequences of the universal primers for ITS2, matK, rbcl, and general PCR reaction conditions were obtained from previous studies (Chen et al., 2010; Raveendar et al., 2015). Amplification reactions were carried out in a total volume of 20 μl containing 10× PCR buffer, 0.1 mM primers, 0.2 mM each deoxyribonucleotide triphosphate (dNTP), 1 U Taq DNA polymerase (Inclone, South Korea), and 200 ng template DNA. High-quality PCR products were sequenced from both directions by Macrogen, South Korea to reduce sequencing error. All sequences were submitted to the National Center for Biotechnology Information (NCBI; Table 1).
Data Analysis and Phylogenetic Analysis Based on Barcoding Regions
Consensus sequences of barcoding regions (ITS2, matK, and rbcL) were manually edited using the BioEdit program (ver.7.0.9, Tom Hall Ibis Biosciences, United States). Sequences were aligned using the “ClustalW” package in MEGA version X (Kumar et al., 2018) and were evaluated for nucleotide variation, number of segregating sites, and informative sites. Nucleotide diversity (π) analysis and Tajima’s test (D) were conducted in DnaSP version 5.10.01 (Librado and Rozas, 2009). To assess individual-level discrimination rates for each single marker and all possible combinations, “Best Match” and “Best Close Match” analysis was carried out in the TAXONDNA software package (Meier et al., 2006). For phylogenetic analysis, maximum likelihood trees were obtained using IQ-TREE version 1.6.2 software (Nguyen et al., 2015). In IQ-TREE, the Best Fit Substitution Model option was selected based on the Bayesian information criterion. Model tests were conducted for each region, and Best Fit Substitution Models were obtained for ITS2 (K2P + I), matK (GTR + F), rbcL (JC + I), ITS2 + matK (TIM + G4), matK + rbcL (TVM + G4), ITS2 + rbcL (TNE + I), and ITS2 + matK + rbcL (TIM + G). Maximum likelihood trees were tested with bootstrap analysis adjusted to 1000 nonparametric bootstrap replicates in which Trifolium repens L. was used as outgroup. Species discrimination was considered successful only when all conspecific individuals generated a monophyletic clade, and species identification was evaluated using bootstrap values (Liu et al., 2011).
Phylogenetic Analysis Based on Morphological Data
A list of 39 Vicia morphological characteristics was compiled from previous studies (Kupicha, 1976; Choi et al., 2006; Jalilian et al., 2014). The morphological characteristics of plant specimens were studied using a stereo-microscope (Olympus SZ61, Olympus, Tokyo, Japan). A digital Vernier caliper (CD-15APX, Mitutoyo, Kawasaki, Japan) was used to measure quantitative characteristics. As mentioned, all 39 morphological characters were evaluated and scored for morphological phylogenetic analysis. The characters and character states are listed in Table 2, and the data matrix is given in Supplementary Table S2. Maximum parsimony analysis was carried out in TNT 1.5 (Goloboff and Catalano, 2016). Scores for 19 taxa were constructed, and inapplicable states were denoted as “–.” Characteristic optimization was performed in MRBAyES SLAVER 5.30 (Nixon, 2008), and cladograms of the useful characteristics were visualized using Mesquite 3.61 (Maddison and Maddison, 2019). The morphological characteristics of V. hirsuta was used as outgroup in the phylogenetic analysis.
Results
Characteristics and Genetic Distances of Barcoding Regions
As a result of amplification with universal primers (ITS2, matK, and rbcL) using template gDNA extracted from each sample, the sequence length of ITS2 varied from 339 to 355 bp, whereas matK and rbcL measured 705 and 542 bp, respectively (Table 3). Most Vicia species had amplified ITS2 sequences of 352 bp in length, whereas Vicia dasycarpa Ten. had sequences of 342 bp, V. villosa and V. cracca of 339 bp, and V. hirsuta and V. tetrasperma of 355 bp. The ratio of G to C was high in the ITS2 region (48–51%) and low in the matK region (30–32%). In the aligned dataset, the lengths of ITS2, matK, and rbcL were 358, 705, and 542 bp, respectively. Deletion sites were found at 64–76 bp in V. dasycarpa, V. villosa, and V. cracca, whereas insertion sites were found at 81–83 bp in V. dasycarpa and at 214–216 bp in V. hirsuta and V. tetrasperma. Nucleotide diversity (π) was analyzed for each locus and was calculated as 0.02109 for matK, 0.01262 for ITS2, and 0.00819 for rbcL. When comparing the nucleotide diversity (π) of the barcode combinations, the ITS2 + matK combination produced the highest value, at 0.01837, followed by matK + rbcL, ITS2 + matK + rbcL, and ITS2 + rbcL at 0.01551, 0.01489, and 0.00990, respectively. matK possessed the largest mean intraspecific distance, at 0.00126, followed by ITS2 + matK, matK + rbcL, ITS2, ITS2 + matK + rbcL, ITS2 + rbcL, and rbcL at 0.00101, 0.00083, 0.00075, 0.00075, 0.00048, and 0.00031, respectively. Similarly, ITS2 possessed the largest mean interspecific distance, at 0.028, followed by ITS2 + matK, matK, ITS2 + matK + rbcL, matK + rbcL, ITS2 + rbcL, and rbcL at 0.0248, 0.0231, 0.0195, 0.0171, 0.0167, and 0.0093, respectively (Table 4).
Intraspecific and Interspecific Variability in Vicia Species
The intraspecific and interspecific variability of each barcode region was analyzed in 19 Vicia species. The results showed that there was no intraspecific variability among the barcode regions in 12 of the 19 taxa. The intraspecific variability of ITS2 was 0.000–0.008, and Vicia japonica A. Gray and Vicia venosa var. cuspidata Maxim. measured 0.004 and 0.008, respectively. The intraspecific variability of matK was 0.000–0.005, with V. japonica and V. venosa var. cuspidata each measuring 0.005, V. pseudo-orobus and V. tetrasperma each measuring 0.003, V. hirsuta measuring 0.002, and Vicia bungei Ohwi and Vicia amurensis Oett. each measuring 0.001. The intraspecific variability of rbcL was 0.000–0.001, with V. amurensis and V. venosa var. cuspidata each measuring 0.001. Interspecific variability was discovered in all species, with ITS2 measuring in the range of 0.009–0.028, matK in the range of 0.015–0.040, and rbcL in the range of 0.005–0.028. V. angustifolia var. segetilis K. Koch. produced the highest interspecific variability values for ITS2 and matK of 0.028 and 0.040, respectively, and V. hirsuta possessed the highest interspecific variability in rbcL of 0.028.
Sequence Similarity for Efficient Species Discrimination
TAXONDNA analysis using the “Best Match” and “Best Close Match” methods was used to evaluate the species discrimination capabilities of each barcode region. The results showed similar discrimination successes among regions (Table 5). Of the three single-locus barcodes, ITS2 and matK showed the highest success rates (54.23%) for correct identification of species, and rbcL showed a success rate of 38.98%. Success rate (59.32%) was higher among four combination barcodes, and the success rates of the ITS2 + matK + rbcL and ITS2 + matK combinations were similar.
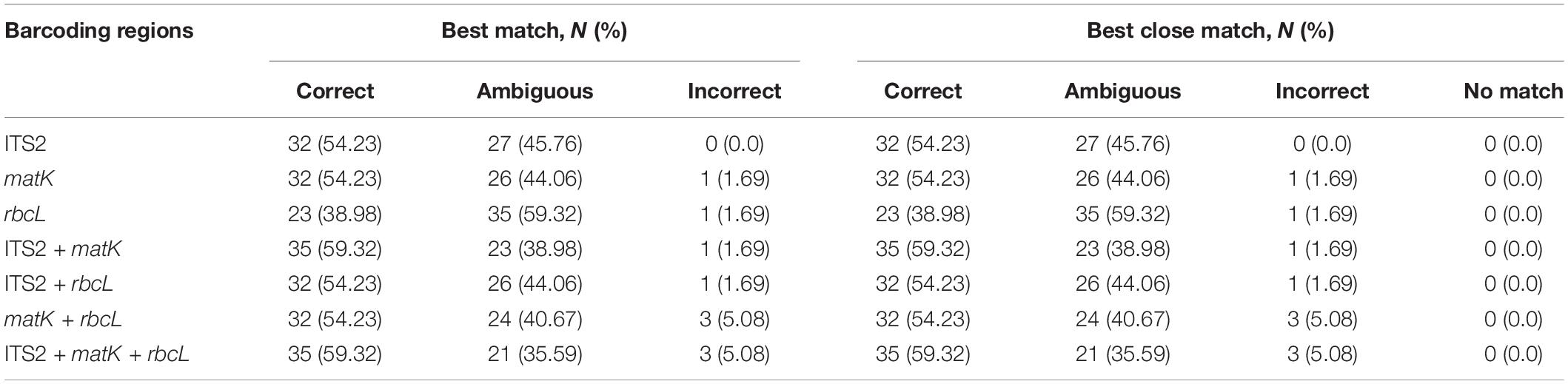
Table 5. Number (rates) of sample identification based on the analysis of the “best match” and “best close match” functions using TAXONDNA software for each DNA barcoding marker and combinations from 59 individuals.
Phylogenetic Analysis and Species Identification
Phylogenetic analysis of 19 Korean Vicia taxa (59 accessions) was performed using the sequences of single regions (ITS2, matK, and rbcL) and of combinations of regions (ITS2 + matK, ITS2 + rbcL, matK + rbcL, and ITS2 + matK + rbcL). The Trifolieae tribe is known to be a sister of the monophyletic Fabeae tribe and was used as an outgroup. Maximum likelihood trees showed that the Vicia species were clearly monophyletic, as each section showed clear discrimination (Figures 2, 3 and Supplementary Figures S1–6). It was confirmed that the ITS2 region separated the species into two clades (clades 1 and 2), and species of sect. Vicilla were grouped into clade 1. Clade 2 was divided into two subclades, with V. angustifolia var. segetilis and V. bungei in subclade 1 and species of sect. Cracca, V. hirsuta, and V. tetrasperma in subclade 2. Based on the phylogenetic tree, the species resolution and discrimination rate of ITS2 were 94.9 and 47.4%, respectively (Supplementary Figure S1 and Table 4). The matK region also separated the species into two clades (clades 1 and 2), and V. hirsuta was grouped into clade 1. Clade 2 was divided into three subclades, with V. angustifolia var. segetilis, V. bungei, and species of sect. Vicilla grouped into subclade 1, species of sect. Cracca clustered into subclade 2, and V. tetrasperma in subclade 3. The species resolution and discrimination rate of matK were 98.1 and 52.6%, respectively (Supplementary Figure S2 and Table 4). Similarly, the rbcL region also separated the species into two clades (clades 1 and 2), with V. hirsuta found in clade 1. Clade 2 was divided into three subclades, with V. tetrasperma grouped into subclade 1, V. angustifolia var. segetilis and species of sect. Vicilla found in subclade 2, and species of sect. Cracca being grouped into subclade 3. Based on the phylogenetic tree, the species resolution and discrimination rate of rbcL were 91.4 and 36.8%, respectively (Supplementary Figure S3 and Table 4). Combining loci improved species discrimination, as the ITS2 + matK + rbcL and ITS2 + matK combinations each produced a value of 57.9%, and ITS2 + rbcL and matK + rbcL produced values of 47.4 and 52.6%, respectively (Supplementary Figures S4–6 and Table 4). The ITS2 + matK + rbcL combination provided the highest species resolution (99.1%), and the rbcL locus alone produced the lowest resolution, at 91.4%.
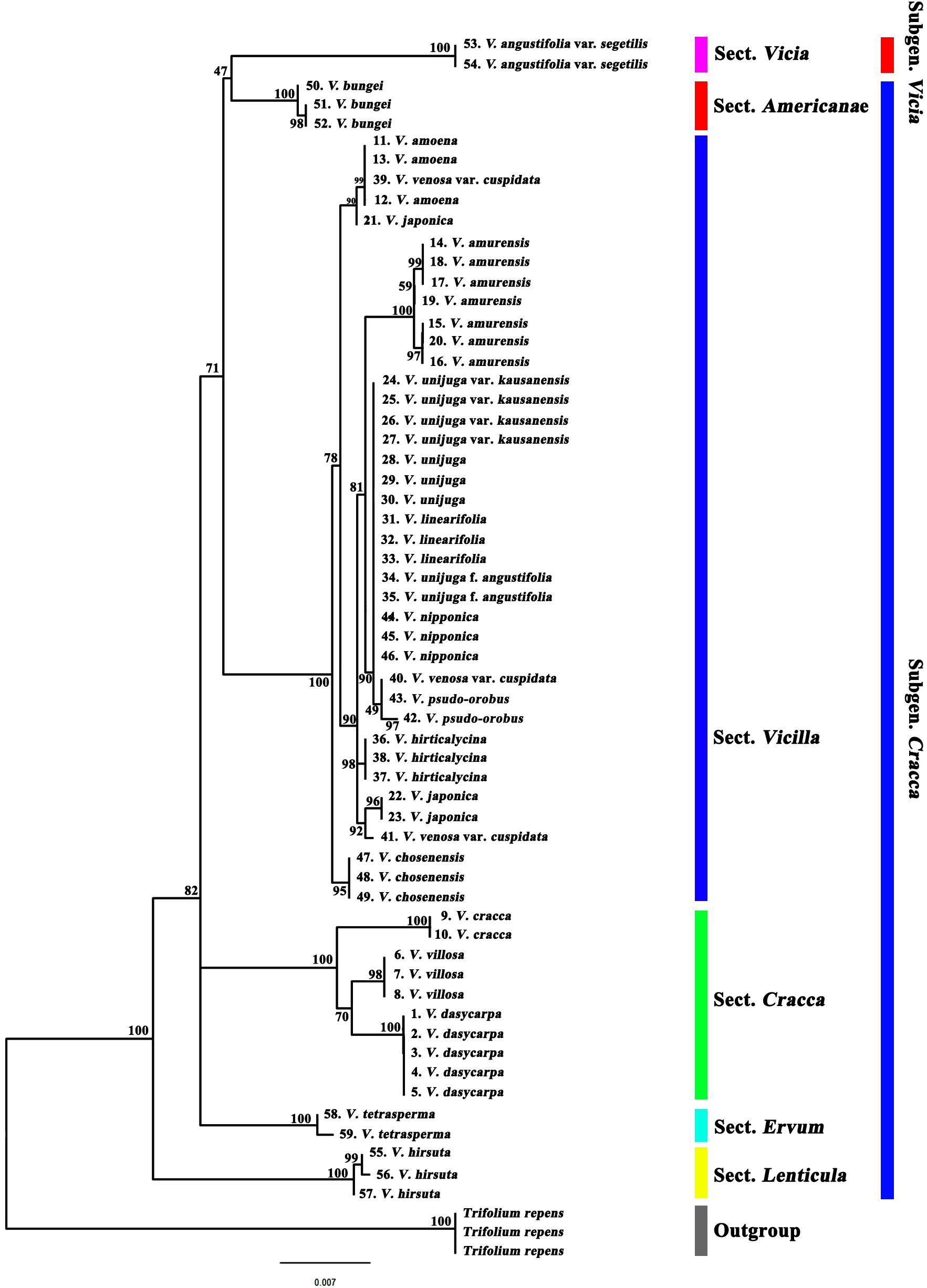
Figure 2. Phylogenetic analysis of 19 Vicia species based on the nucleotide sequences of the combined ITS2 + matK + rbcL regions.
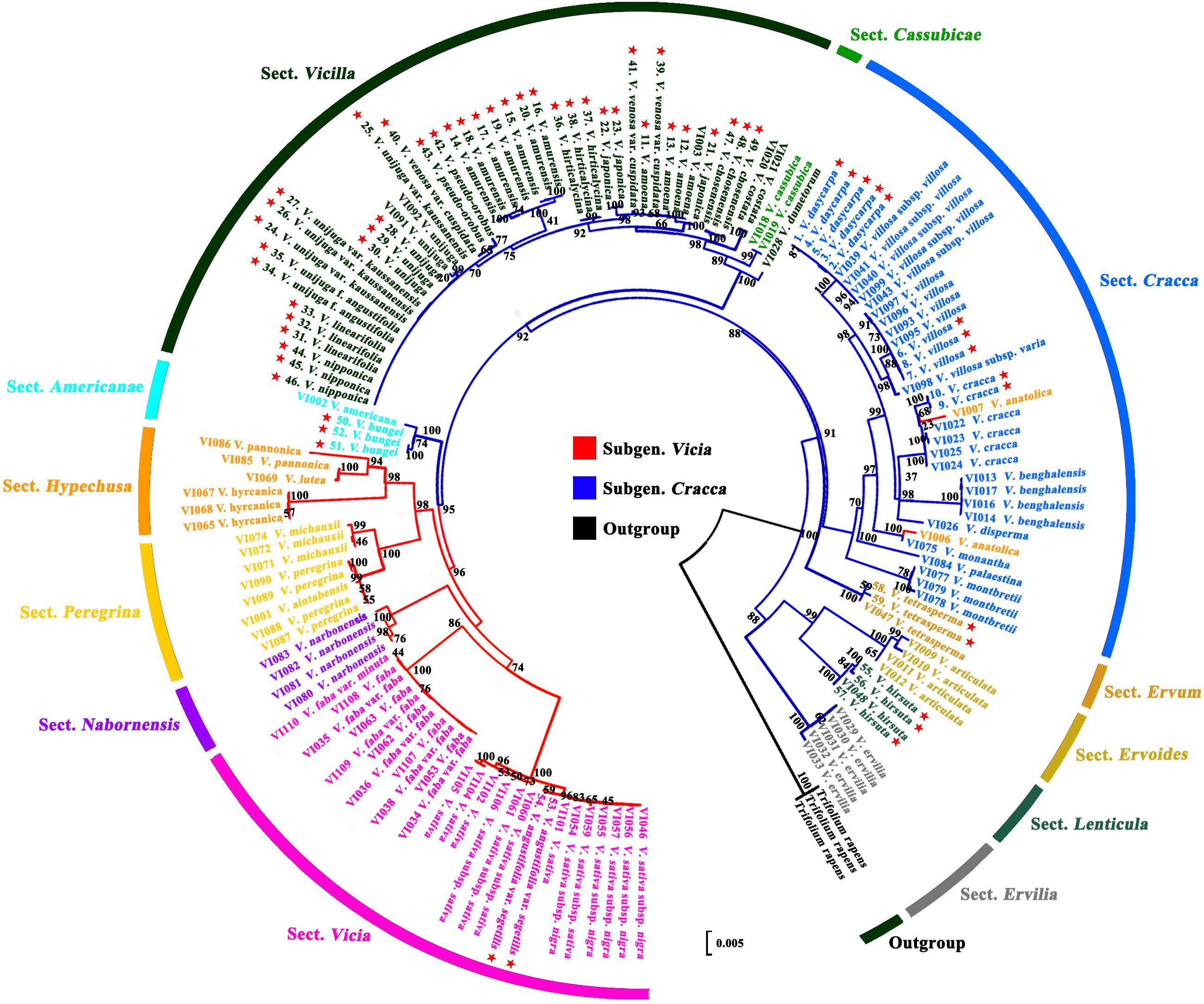
Figure 3. Phylogenetic analysis of Vicia species based on the nucleotide sequences the combined ITS2 + matK + rbcL regions. ★ Collected accessions of native Vicia species in this study.
Barcode sequence of ITS2, matK, and rbcL were downloaded from the NCBI and combined with the present study. The results showed that each section of Vicia appeared to be monophyletic from the outgroup and formed six groups (Figure 3). Sect. Ervilia, sect. Lenticula, and sect. Ervoides are clustered in group 1; sect. Ervum is clustered in group 2; sect. Cracca is clustered in group 3; Cassubicae and sect. Vicilla clustered together in group 4; sect. Americanae found in group 5; and sect. Hypechusa, sect. Peregrina, sect. Narbonensis, and sect. Vicia is clustered into group 6. Almost all Korean species of Vicia, excluding V. angustifolia var. segetilis (subgenus Vicia), included the subgenus Cracca. Most Korean Vicia species, such as Vicia amoena Fisch. ex DC., V. amurensis, V. japonica, V. unijuga var. kaussanensis, V. unijuga, V. linearifolia, V. unijuga f. angustifolia Makino ex Ohwi, V. hirticalycina, V. venosa var. cuspidata, V. pseudo-orobus, V. nipponica, and V. chosenensis are clustered in sect. Vicilla (group 4), and three Vicia species (V. dasycarpa, V. villosa, and V. cracca) are grouped into sect. Cracca (group 3). V. bungei, V. angustifolia var. segetilis, V. hirsuta, and V. tetrasperma are clustered into sect. Americanae (group 5), sect. Vicia (group 6), sect. Lenticula (group 1), and sect. Ervum (group 2), respectively.
Morphological Analysis of Species Identification
Maximum parsimony analysis was performed to identify the optimal morphological traits for efficient identification of Korean Vicia species (Figures 4, 5). The results of character support for phylogenetic positions and character state mapping and 16 useful morphological characteristics, such as tuber presence, stem pubescence, number of leaflet, leaflet shape, leaflet apex, leaflet base, stipule nectariferous spot, stipule present, tendrils, number of flower per inflorescence, relative length of limb and claw in standard, standard shape, seed shape, seed color, style shape, and ovary hairiness were selected for Vicia species identification (Figure 5). V. hirsuta was used as the outgroup as it is a first Vicia species found to diverge from species of the Trifolieae tribe, a known sister to the monophyletic Fabeae, of Vicia ervilia and Vicia sylvatica. V. hirsuta has white flowers and greenish yellow seed color, and small-sized seeds separate the species from others in the Vicia genus. Cladistic analysis revealed that V. angustifolia var. segetilis of sect. Vicia was separated from V. hirsuta by the following characteristics: leaflet width of 10–20 mm, obtuse leaflet base, present stipule nectariferous spot, and abaxially tufted style pubescence and that the other species of sections were supported by unequal calyx teeth length. Characteristic of present nectaferious spot can be a useful trait to distinguish V. angustifolia var. segetilis (Figure 5D). V. tetrasperma of sect. Ervum was separated by the following characteristics: glabrous stem pubescence, elliptic leaflet shape, acute leaflet apex, unbranched tendrils, and 1–(2) flower per inflorescence and that the other species of sections were supported by characteristics of life form, relative length of limb and claw in standard, and seed size. Characteristic of 1–(2) flower per inflorescence can be a useful trait to distinguish V. tetrasperma (Figure 5E). V. bungei is separated by its being erect, the presence of tubers, obovate leaflet shape, obtuse leaflet apex, smooth stipule surface, hilum color that is same as the seed color, and abaxially tufted style pubescence. Characteristic of the presence of tubers can be a useful trait to distinguish V. bungei (Figure 5A).
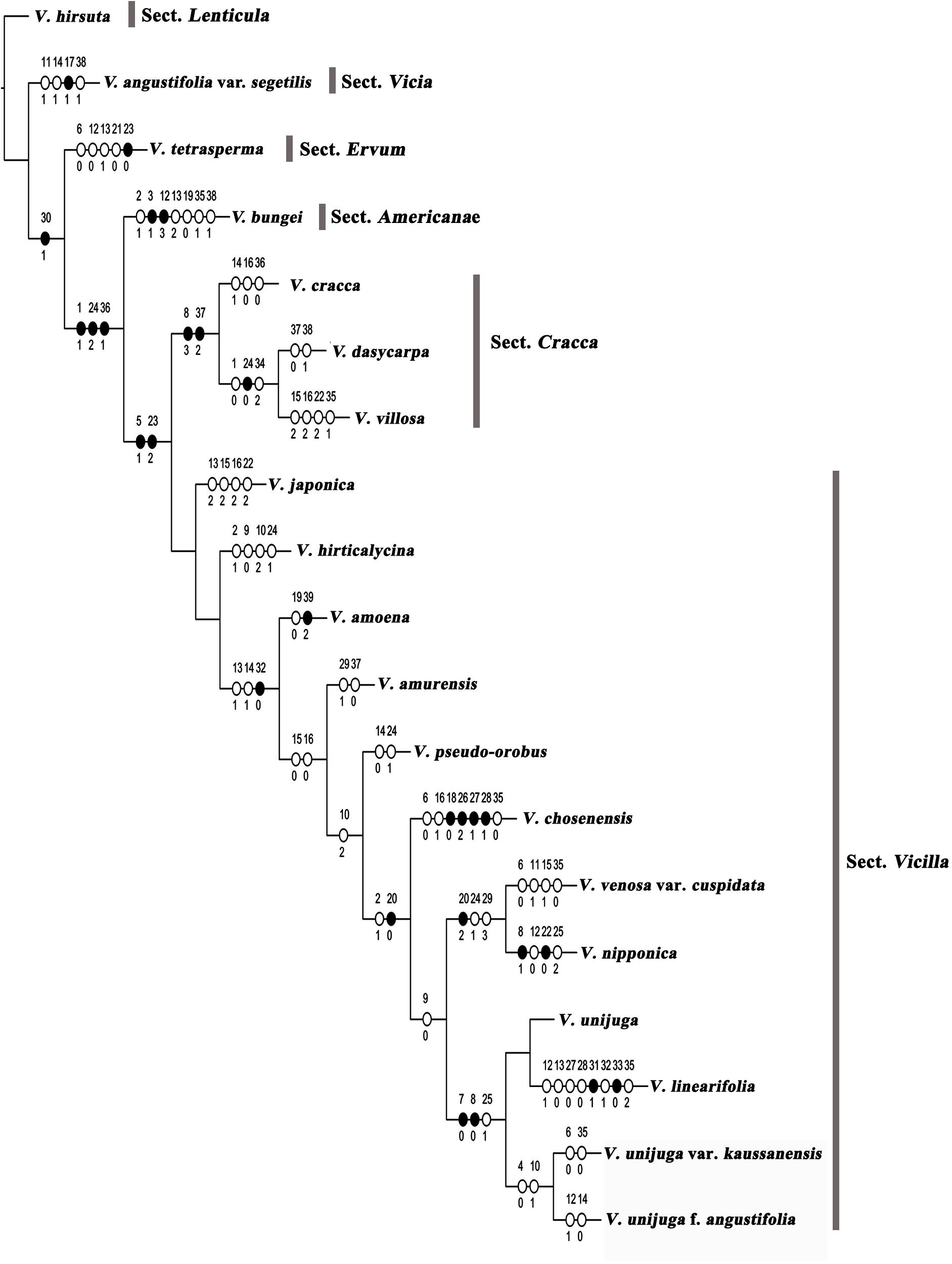
Figure 4. Character support for phylogenetic positions of Vicia species. Strict consensus tree (length = 135 steps; CI = 0.48; RI = 0.56) showing the character state optimizations at each node of the cladogram, represented by circles. Non-homoplastic changes in character states are represented by black circles, and homoplastic changes are shown with white circles. The numbers above and below each circle represent the character and character state number, respectively. Matrix information on characters and character state numbers: (1) life form (0, annual or biannual; 1, perennial); (2) growth habit (0, climbing; 1, erect; 2, ascending); (3) tuber presence (0, absent; 1, present); (4) stem height [0, small (up to 40 cm); 1, high (over 40 cm)]; (5) stem form (0, slender; 1, rigid); (6) stem pubescence [0, glabrous; 1, sparse (epidermis visible); 2, dense (epidermis not visible)]; (7) leaflet pairs per leaf (0, one pair; 1, more than two pairs); (8) number of leaflets (0, 2; 1, 4–8; 2, 6–14; 3, 16–24); (9) leaflet size relative to leaf (0, same size; 1, larger at leaf base); 10, leaflet length (0, <2.0 mm; 1, 20–40 mm; 2, >40 mm); (11) leaflet width (0, <10 mm; 1, 10–20 mm; 2, >20 mm); (12) leaflet shape (0, elliptic; 1, lanceolate; 2, oblong; 3, obovate; 4, ovate); (13) leaflet apex (0, acuminate; 1, acute; 2, obtuse; 3, truncate); (14) leaflet base (0, cuneate; 1, obtuse); (15) leaflet adaxial hair density [0, glabrous; 1, sparse (epidermis visible); 2, dense (epidermis not visible)]; (16) leaflet abaxial hair density [0, glabrous; 1, sparse (epidermis visible); 2, dense (epidermis not visible)]; (17) stipule nectariferous spot (0, absent; 1, present); (18) stipules present (0, absent; 1, present); (19) stipule surface (0, smooth; 1, hairy); (20) tendrils (0, absent; 1, present; 2, on some leaves); (21) tendril branching (0, unbranched; 1, branched); (22) tendril hair density [0, glabrous; 1, sparse (epidermis visible); 2, dense (epidermis not visible)]; (23) number of flowers per inflorescence [0, 1–(2); 1, 2–4; 2, 5 or more]; (24) relative length of limb and claw in standard (0, shorter than claw; 1, as long as; 2, longer than claw); (25) standard shape (0, oblong; 1, stenonychinoid; 2, platonychinoid); (26) standard color pattern (0, absent; 1, differently colored spot; 2, differently colored veins; 3, differently colored back; 4, darker); (27) standard color (0, white; 1, yellow; 2, purple or bluish); (28) wing color [0, white; 1, yellow; 2, purple (bluish)]; (29) wing length (0, 1/4 shorter than the standard; 1, slightly shorter than standard; 2, longer than standard; 3, similar to standard); (30) calyx teeth length (0, equal; 1, unequal); (31) seed shape (0, spherical; 1, oblong); (32) seed color (0, brown; 1, greenish brown; 2, reddish brown; 3, greenish yellow); (33) seed color mottling (0, absent; 1, present); (34) hilum shape (0, circumlinear; 1, linear; 2, oblong; 3, wedge; 4, oval); (35) hilum color (0, pale; 1, seed color; 2, dark); (36) seed size (0, <3 mm; 1, 3–5(6) mm; 2, >6 mm); (37) style shape (0, terete; 1, dorsally compressed; 2, laterally compressed); (38) style pubescence (0, evenly pubescent; 1, abaxially tufted; 2, v-shaped; 3, adaxially tufted); (39) ovary hairiness (0, glabrous; 1, glabrous hairy; 2, short glandular hairy).
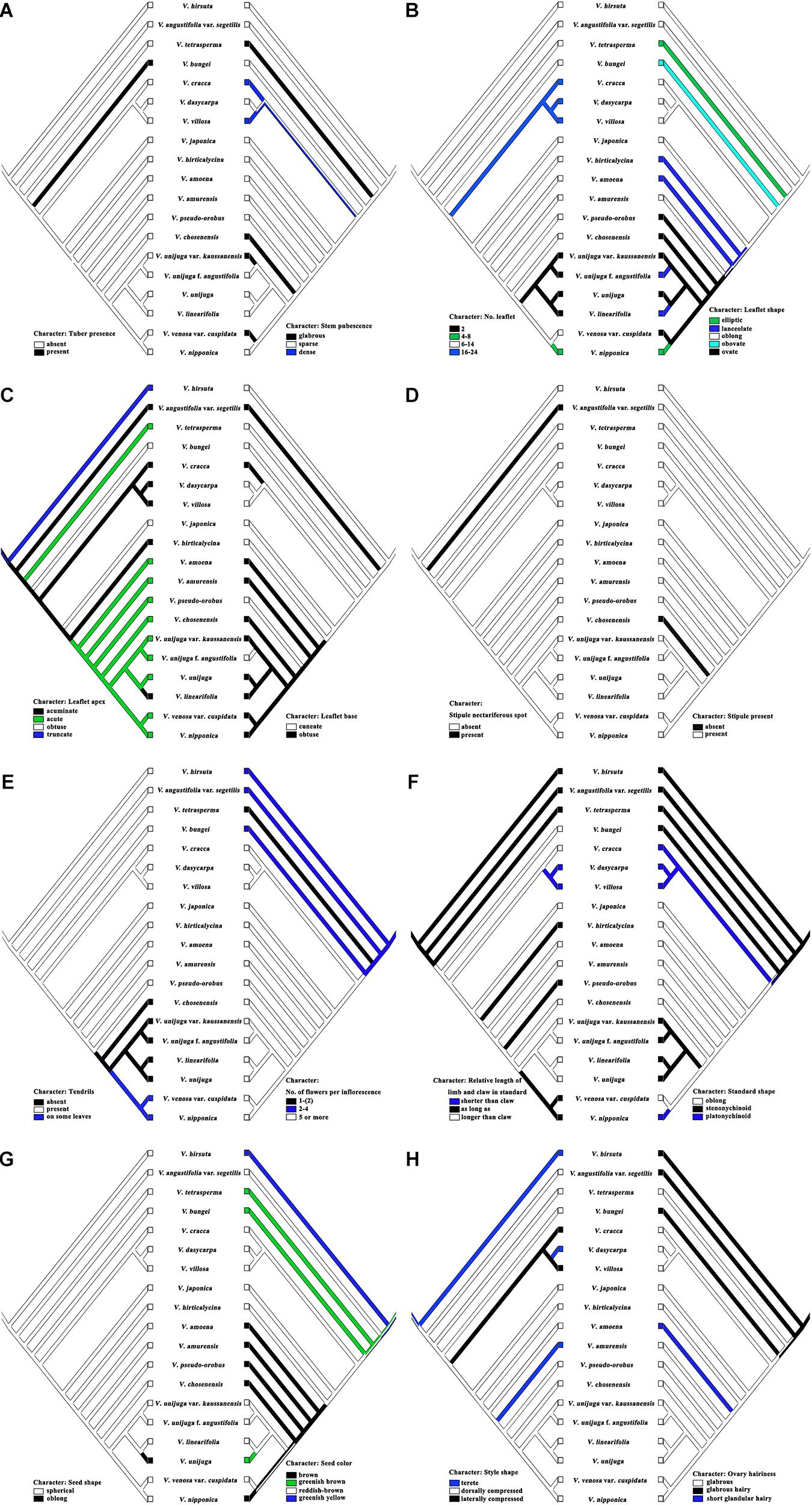
Figure 5. Character state mapping of 16 useful morphological characteristics. (A) Character of Tuber presence and Stem pubescence; (B) Character of No. of leaflets and Leaflet shape; (C) Character of Leaflet apex and Leaflet base; (D) Character of Stipule nectariferous spot and Stipule present; (E) Character of Tendrils and No. of flowers per inflorescence; (F) Character of relative length of limb and claw in standard and Standard shape; (G) Character of Seed shape and Seed color; (H) Character of Style shape and Ovary hairiness.
Species from sect. Cracca and sect. Vicilla formed a sister clade because of their rigid stems and the fact that they form five or more flowers per inflorescence. Species of sect. Cracca were supported by their characteristic of 16–24 leaflets. V. cracca was separated by its obtuse leaflet base, glabrous leaflet abaxial hair density, and the fact that its seeds are <3 mm in size. Characteristics of 16–24 leaflets and obtuse leaflet base can be useful traits to distinguish V. cracca (Figures 5B,C). V. dasycarpa and V. villosa were supported by their annual or biannual life form, limb of standard that is shorter than the claw, and their oblong hilum shape. V. dasycarpa was distinguished by its terete style shape and abaxially tufted style pubescence, and V. villosa was distinguished owing to its dense leaflet adaxial hair density, dense leaflet abaxial hair density, dense tendril hair density, and hilum color that is same as the seed color. Characteristics of a limb that is shorter than the claw and terete style shape can be useful traits to distinguish V. dasycarpa (Figures 5F,H), and characteristics of limb a that is shorter than the claw and laterally compressed style shape can be useful traits to distinguish V. villosa (Figures 5F,H). V. japonica was separated by its obtuse leaflet apex, dense leaflet adaxial hair density, dense leaflet abaxial hair density, and dense tendril hair density. Characteristics of oblong standard shape and obtuse leaflet apex can be useful traits to distinguish V. japonica (Figures 5C,F). V. hirticalycina was separated by its erect, same size leaflet, leaflet length that is more than 40 mm, and limb that is as long as the claw. Characteristics of five or more flower per inflorescence and acuminate leaflet apex can be useful traits to distinguish V. hirticalycina (Figures 5C,E). V. amoena Fisch. ex DC. was separated by its smooth surface and short glandular hairy ovaries. Characteristic of short glandular hair of the ovary can be a useful trait to distinguish V. amoena (Figure 5H). V. amurensis was separated by its wing that is slightly shorter than the standard and terete style shape. Characteristics of brown seed color and terete style shape can be useful traits to distinguish V. amurensis (Figures 5G,H). V. pseudo-orobus was separated by its cuneate leaflet base and limb that is as long as the claw. Characteristics of acute leaflet apex, present tendril, and limb that is as long as the claw can be useful traits to distinguish V. pseudo-orobus (Figures 5C,E,F). V. chosenensis was separated by its absent tendrils, glabrous stem pubescence, sparse leaflet abaxial hair density, absent stipule, standard differently colored veins, yellow standard color, yellow wing color, and pale hilum color. Characteristic of absent stipule can be a useful trait to distinguish V. chosenensis (Figure 5D).
The positions of V. venosa var. cuspidata and V. nipponica sister to V. unijuga A. Braun, V. linearifolia Y.N.Lee, V. unijuga var. kaussanensis, and V. unijuga f. angustifolia Makino were supported by the fact that their leaflets were all the same sizes. V. venosa var. cuspidata was differentiated by glabrous stem pubescence, 10–20 mm leaflet width, sparse leaflet adaxial hair density, and pale hilum color, and V. nipponica was distinguished because of having four to eight leaflets, elliptic leaflet shape, glabrous tendril hair density, and platonychinoid standard shape. Characteristics of the tendril of one some leaves and glabrous stem pubescence can be useful traits to distinguish V. venosa var. cuspidata (Figures 5A,E), and characteristics of the tendril of one leaf and four to eight leaflets can be useful a trait to distinguish V. nipponica (Figures 5B,E). V. unijuga was separated by the fact that it had one pair of leaflets, two leaflets in one pair of leaflets, and stenonychinoid standard shape. V. linearifolia was distinguished by its lanceolate leaflet shape, acuminate leaflet apex, white flower, oblong seed shape, greenish brown seed color, absent seed color mottling, and dark hilum color. Characteristics of two leaflets, sparse stem pubescence, and ovate leaflet shape can be useful traits to distinguish V. unijuga (Figures 5A,B). Characteristics of oblong seed shape and greenish brown seed color can be useful traits to distinguish V. linearifolia (Figure 5G). V. unijuga var. kaussanensis and V. unijuga f. angustifolia were supported by their characteristic of small stem height and 20–40 mm leaflet length. V. unijuga var. kaussanensis was separated by its glabrous stem pubescence and pale hilum color. V. unijuga f. angustifolia was separated by its lanceolate leaflet shape and cuneate leaflet base. Characteristics of two leaflets and glabrous stem pubescence can be useful traits to distinguish V. unijuga var. kaussanensis (Figures 5A,B), and characteristics of two leaflets and cuneate leaflet base can be useful traits to distinguish V. unijuga f. angustifolia (Figures 5B,C).
Discussion
Characteristics of Barcoding Regions in Vicia
The present study investigated the possibility of classifying and examining the molecular relationships among Korean Vicia species using three barcode regions (ITS2, matK, and rbcL). PCR amplification and sequencing of all three barcoding regions showed 100% success rate with Korean Vicia species. The universality of the PCR amplification success is an important criterion for DNA barcoding (Kress and Erickson, 2007). Barcode sequence alignment analysis revealed considerable nucleotide diversity (π) between the loci, in which ITS2 showed the largest mean interspecific distance (Table 4), whereas there was no intraspecific variability among the barcode regions in 12 of the 19 taxa. In general, plastid and rDNA regions were reported as potential loci for barcoding due to the low sequence divergence (Saski et al., 2007; Erickson et al., 2008). However, an ideal DNA barcode should be universal, reliable, cost effective, and provides considerable discriminatory power for plant species identification.
The present study showed, as single barcodes, that the species discrimination abilities of all three barcodes were poor (Supplementary Figures S1, 2), whereas the barcode combinations (ITS2 + matK + rbcL) improved species discrimination as well as species resolution (Table 4) as reported in the previous study (Raveendar et al., 2017). Similarly, in an earlier study, Wu et al. (2020) evaluated a combination of barcodes such as matK, rbcL, trnH-psbA, trnL-trnF, ITS1, and ITS2 to identify 161 Vicia species. However, the present study did not include all known Vicia species, as reported in previous studies (Choi et al., 2006; Raveendar et al., 2017; Wu et al., 2020). There are known endemic Vicia species in Korea, which have a restricted distribution that needed efficient conservation strategy. Moreover, collection and conservation of many Vicia species were available at the Korean gene bank. Hence, the present study was focused mainly on Vicia species distributed in South Korea together with available germplasm resources to preserve the biological diversity.
Phylogenetic Analysis of Barcode Regions
The phylogenetic analysis of ITS2, matK, and rbcL were displayed sufficient markers for V. angustifolia var. segetilis, V. bungei, V. villosa, V. cracca, V. dasycarpa, V. hirsuta, V. tetrasperma, V. amurensis, V. hirticalycina Nakai, and V. chosenensis species identification, whereas V. unijuga, V. unijuga var. kaussanensis, V. linearifolia, V. unijuga f. angustifolia, and V. nipponica species did not form distinct clusters in the phylogenetic tree. In the phylogenetic analysis, V. amoena, V. japonica, V. venosa var. cuspidata, and V. pseudo-orobus were not differentiated, and these species were found to be very closely related to each other, which comes under sect. Vicilla. Phylogenetic analysis can help us to understand the evolutionary history of genes and species. Most importantly, the mode and time of species evolution can be estimated based on characteristics (Som, 2015). Phylogenetic analyses have previously been performed using morphological, anatomical, molecular, and karyological characteristics of the Vicia genus (Leht and Jaaska, 2002; Van de Wouw et al., 2003; Leht, 2005, 2009; Choi et al., 2006; Castiglione et al., 2011). The genus Vicia L. is included in the Fabeae (syn. Vicieae) tribe, along with four other genera: Lathyrus L., Lens Mill., Pisum L., and Vavilovia Fed. In previous studies, it was also reported that Lathyrus, Lens, Pisum, and Vavilovia are nested within Vicia species of all genera analyzed (Smýkal et al., 2011; Schaefer et al., 2012; Omar et al., 2019).
It is well known that most taxa in sect. Vicilla are Asian species distributed across Siberia, China, Mongolia, Japan, and South South Korea, with the exception of European taxa such as Vicia dumetorum and V. sylvatica (Li and Liu, 1996). Most of the native Vicia taxa in South Korea belong to sect. Vicilla (Figure 3), with Korean endemic species such as V. chosenensis, V. hirticalycina, and V. unijuga var. kaussanensis (Chung et al., 2017). Endo et al. (1996) defined the sect. Amurense Y.Endo & H.Ohashi to contain approximately four species: V. amurensis, Vicia dichroantha Diels, Vicia nummularia Hand.-Mazz., and Vicia tibetica Fisch. with similar morphological characteristics such as terete style, globose stigma, and swollen-type pollen mesocolpium. However, terete style was found to be polyphyletic; hence, sect. Amurense has not been reported as monophyletic, even in molecular phylogenetic studies (Endo et al., 2010). Schaefer et al. (2012) suggested that sect. Vicilla should be recircumscribed to include sect. Amurense and sect. Cassubicae. In the present study, V. amurensis was grouped in sect. Vicilla (Schur) Aschers & Graebner (Figure 3), thus supporting the infrageneric position of V. amurensis in sect. Vicilla (Kupicha, 1976; Rong and Shiyuan, 1994).
Species V. bungei distributed in China and Korea and Vicia americana Willd. distributed in North America are phenotypically and ecologically similar to each other, which is found in sect. Americanae (Kupicha, 1976; Endo et al., 2000). However, the two species can be discriminated by their differing root types and chromosome numbers. V. bungei has a well-developed root tuber and a chromosome number of 2n = 42 (hexaploid), whereas V. americana has no root tuber and has a chromosome number of 2n = 14 (diploid). Schaefer et al. (2012) suggested that the V. americana lineage may have spread from North America (V. americana) to Asia (V. bungei). Considering that the Bering land bridge connected northeastern Asia and northwestern North America in the Miocene, migration of flora over the land bridge could have occurred (Wen, 1999). Thus, V. bungei (hexaploid) may have evolved from the V. americana (diploid) lineage and could have developed tuber roots to adapt to cold stress or for energy storage purposes. In the current study, species of sect. Americanae formed sister clade with species of sections in the Vicia subgenus and were more closely related to sections in subgenus Vicia than sections in subgenus Cracca. In phylogenetic relationships analysis based on chloroplast protein-coding genes with 21 Papilionoideae subfamily, V. bungei was found to be closely related with the species of Vicia faba, Vicia sativa, and Vicia sepium in the Vicia subgenus (Yi et al., 2020). Therefore, the species of sect. Americanae may have played a key role in the evolution of subgenera Vicia and Cracca. More evolutionary study including geographic distribution is needed to confirm the evolutionary relationship of these species.
Phylogenetic Analysis of Morphological Characteristics
The phylogenetic analysis based on 39 morphological characters well-supported the classification of species and sections of the genus Vicia in South Korea. The analysis revealed that tuber presence, stem pubescence, number of leaflets, leaflet shape, leaflet apex, leaflet base, stipule nectariferous spot, stipule present, tendrils, number of flowers per inflorescence, relative length of limb and claw in standard, standard shape, seed shape, seed color, style shape, and ovary hairiness were useful traits for section and species level discrimination (Figures 4, 5). Kupicha (1976) divided the genus Vicia into subgenus Vicia and subgenus Cracca by the presence or absence of stipule nectaries, which is known as a useful trait at the subgenus level. In the present study, of the Vicia species distributed in South Korea, only V. angustifolia var. segetilis had stipule nectaries (Figure 5). V. bungei of sect. Americanae was the only species with tubers, and this characteristic clearly distinguished V. bungei from other Vicia species (Endo et al., 2000). The number, length, and width of leaflets are useful characteristics for the primary classification of specific taxa in the genus Vicia (Fu et al., 1996; Abozeid et al., 2017). V. cracca, V. villosa, and V. dasycarpa of sect. Cracca possess 16–24 leaflets, and V. nipponica possesses 4–8 leaflets. V. unijuga, V. unijuga var. kaussanensis, V. linearifolia, and V. unijuga f. angustifolia are known to be related taxa, and all possess two leaflets (Lee, 1972; Seok and Choi, 1997). V. unijuga var. kaussanensis, found on Jeju Island in South Korea, has overall smaller leaflets than V. unijuga, and V. unijuga f. angustifolia has lanceolate-shaped leaves, which is distinguished from V. unijuga that has ovate leaflets. V. linearifolia has been distinguished from other Vicia taxa, as it has lanceolate shape, acuminate apex leaflets, and white flowers (Lee, 1972; Seok and Choi, 1997). In this study, seed morphological characteristics such as seed color (greenish brown), hilum color (dark), and seed mottling (absent), and an oblong seed shape can be additional characteristics to distinguish V. linearifolia.
Style shape and pubescence characteristics are known to be major classification characteristics in the Vicia, Lathyrus, Lens, Pisum, and Vavilovia genera. Most Vicia species are laterally compressed, terete, and dorsiventrally compressed in terms of style shape and have abaxial, V-shaped, and even style pubescence (Kupicha, 1976; Endo and Ohashi, 1995; Choi et al., 2006). In the present study, most Vicia species distributed in South Korea had dorsally compressed styles, with V. cracca and V. villosa possessing laterally compressed styles and a terete shape being observed in V. hirsuta, V. dasycarpa, and V. amurensis. V. dasycarpa of sect. Cracca has similar characteristics to V. villosa, and two are known to be closely related. The two taxa can be divided based on overall hair density; however, owing to the fact that they share very similar characteristics, V. dasycarpa is recognized as a subspecies or by synonyms of V. villosa (Jalilian et al., 2014; Jung et al., 2016). However, the newly investigated terete shape and abaxially tufted style of V. dasycarpa can be used to distinguish the species from V. villosa, which is laterally compressed and evenly pubescent in style (Table 2 and Supplementary Table S2). These characteristics could be utilized as a taxonomic key between the two taxa, allowing V. dasycarpa to be recognized as a species in its own right.
Molecular and Morphological Analysis of South Korean Vicia Species
In the present study, results revealed that the combined barcoding regions (ITS2 + matK + rbcL) efficiently differentiate the following species: V. angustifolia var. segetilis, V. bungei, V. villosa, V. cracca, V. dasycarpa, V. hirsuta, V. tetrasperma, V. amurensis, V. hirticalycina, and V. chosenensis. However, they failed to differentiate the species of V. unijuga, V. unijuga var. kaussanensis, V. linearifolia, V. unijuga f. angustifolia, V. nipponica, V. amoena, V. venosa var. cuspidata, V. pseudo-orobus, and V. japonica with the tested barcode regions. To differentiate the unclassified species, 39 morphological characteristics were investigated, in which 16 useful characteristics such as tuber presence, stem pubescence, number of leaflets, leaflet shape, leaflet apex, leaflet base, stipule nectariferous spot, stipule presence, tendrils, number of flowers per inflorescence, relative length of limb and claw in standard, standard shape, seed shape, seed color, style shape, and ovary hairiness were selected for efficient classification. The 16 selected useful traits were efficiently differentiating the closely related species of V. unijuga, V. unijuga var. kaussanensis, V. linearifolia, V. unijuga f. angustifolia, V. nipponica, V. amoena, V. venosa var. cuspidata, V. pseudo-orobus, and V. japonica when analyzed with tested barcodes.
DNA barcoding and morphological characters have long been studied as efficient methods of species classification (Li et al., 2011; Ghorbani et al., 2017; Veldman et al., 2020). The present study also revealed that combination of DNA barcodes and morphological characterization were shown to be valuable methods in distinguishing among related species to classify the Vicia species found in South Korea. Most of the Vicia taxa collected in South Korea belonged to sect. Vicilla (12 taxa), sect. Cracca (three taxa), sect. Vicia (one taxon), sect. Americanae (one taxon), sect. Ervum (one taxon), and sect. Lenticula (one taxon). V. nipponica, V. unijuga f. angustifolia, V. linearifolia, V. unijuga, and V. unijuga var. kaussanensis H. Lév. of sect. Vicilla have almost the same nucleotide base composition and were more closely related among the examined taxa (Supplementary Figures S1–3). The shape and size of leaflets are important classification keys for V. unijuga f. angustifolia, V. linearifolia, V. unijuga, and V. unijuga var. kaussanensis; however, leaflet sizes are easily differentiated and easily influenced by the external environment (Seok and Choi, 1997). Thus, more stable characteristics such as number of leaflets, leaflet shape, stem pubescence, leaflet shape, seed shape, and seed color are needed to distinguish these species. V. nipponica possesses the very distinct characteristic of having four or more leaflets compared to V. unijuga f. angustifolia, V. linearifolia, V. unijuga var. kaussanensis, and V. unijuga, which only have two leaflets. However, the molecular phylogeny analysis showed that the species is genetically more related with other species.
In the case of closely related species or species that have recently undergone speciation, morphological characteristics are considered important because identification with barcode regions is difficult. The accumulation of genetic and ecological differences can occur because of geographical isolation and ecology (Ba̧czkiewicz et al., 2017). For example, in V. japonica, the accessions collected on Ulleung Island share the same nucleotide sequences in the barcode regions, whereas one specimen collected in Pohang showed variation. This is probably the result of geographic isolation, and further studies are needed to determine whether these regions have different genetic diversity. Moreover, a species with the highest level of intraspecific difference was observed with V. venosa var. cuspidata. The chromosome number of V. venosa var. cuspidata was found to be 2n = 4X = 24, also known as autotetrasperma (Nam et al., 2012). It was reported that in the ITS region, autotetrasperma plants sometimes have two types of nucleotide sequence, which may be the reason for the observed high intraspecific variation (Noh et al., 2018).
Conclusion
In conclusion, based on the molecular phylogenetic analysis, the present study suggests that, within the Vicia species found in South Korea, combined barcode regions (ITS2, matK, and rbcL) could be applied to distinguish the 10 Vicia taxa such as V. angustifolia var. segetilis, V. bungei, V. villosa, V. cracca, V. dasycarpa, V. hirsuta, V. tetrasperma, V. amurensis, V. hirticalycina, and V. chosenensis, whereas nine Vicia taxa such as V. unijuga, V. unijuga var. kaussanensis, V. linearifolia, V. unijuga f. angustifolia, V. nipponica, V. amoena, V. pseudo-orobus, and V. japonica can only be effectively differentiated along with 16 morphologically useful character. Recommended candidate barcode regions for species discrimination could not distinguish all species native to South Korea; thus, further molecular studies on a new barcode locus or effective molecular markers from nuclear and chloroplast genomes are needed for the discrimination of closely related species or species that have recently undergone speciation in the genus Vicia. The results of molecular and morphological characteristics of this study could be a valuable information for the discrimination of Vicia species in agricultural, ecological, and conservation purpose.
Data Availability Statement
The datasets generated in this study can be found in online repositories. All the sequences were deposited to NCBI GenBank with following accession numbers: MW372935-MW373052 and MW374737-MW374795.
Author Contributions
DH and J-WC conceived and designed the experiments. SH, RS, and KL performed the experiments and analyzed the data. G-TC and DH contributed to project coordination and analysis. SH and XW contributed to materials. SH and RS drafted the manuscript and figures. All authors contributed to the article and approved the final version of the submitted manuscript.
Conflict of Interest
The authors declare that the research was conducted in the absence of any commercial or financial relationships that could be construed as a potential conflict of interest.
Acknowledgments
This study was carried out with the support of the “Research Program for Agricultural Science & Technology Development (Project No. PJ01258002)” and was supported by the 2019 collaborative research program between the university and Rural Development Administration, South Korea.
Supplementary Material
The Supplementary Material for this article can be found online at: https://www.frontiersin.org/articles/10.3389/fpls.2021.608559/full#supplementary-material
References
Abozeid, A., Liu, Y., Liu, J., and Tang, Z. (2017). Comparative foliar structure of vicia l. species from China. J. Biosci. Med. 5, 170–175. doi: 10.4236/jbm.2017.53018
Ali, M. A., and Choudhary, R. K. (2011). India needs more plant taxonomists. Nature 471:37. doi: 10.1038/471037d
Ali, M. A., Gyulai, G., Hidvegi, N., Kerti, B., Al Hemaid, F. M., Pandey, A. K., et al. (2014). The changing epitome of species identification-DNA barcoding. Saudi J. Biol. Sci. 21, 204–231. doi: 10.1016/j.sjbs.2014.03.003
Al-Joboury, K. R. (2017). Taxonomical study for some species of Vicia L.(fabaceae family). J. Pharm. Biol. Sci. 12, 61–64. doi: 10.9790/3008-1205076164
Ba̧czkiewicz, A., Szczecińska, M., Sawicki, J., Stebel, A., and Buczkowska, K. (2017). DNA barcoding, ecology and geography of the cryptic species of Aneura pinguis and their relationships with Aneura maxima and Aneura mirabilis (Metzgeriales, Marchantiophyta). PLoS One 12:0188837. doi: 10.1371/journal.pone.0188837
Cacan, E., Kokten, K., Inci, H., Das, A., and Sengul, A. Y. (2016). Fatty acid composition of the seeds of some Vicia Species. Chem. Natural Compounds 52, 1084–1086. doi: 10.1007/s10600-016-1867-1
Carneiro de Melo Moura, C., Brambach, F., Jair Hernandez, Bado, K., Krutovsky, K. V., Kreft, H., et al. (2019). Integrating DNA barcoding and traditional taxonomy for the identification of dipterocarps in remnant lowland forests of Sumatra. Plants 8:461. doi: 10.3390/plants8110461
Castiglione, M. R., Frediani, M., Gelati, M. T., Ravalli, C., Venora, G., Caputo, P., et al. (2011). Cytology of Vicia species. X. Karyotype evolution and phylogenetic implication in Vicia species of the sections Atossa, Microcarinae, Wiggersia and Vicia. Protoplasma 248, 707–716. doi: 10.1007/s00709-010-0232-7
Chen, S., Yao, H., Han, J., Liu, C., Song, J., Shi, L., et al. (2010). Validation of the ITS2 region as a novel DNA barcode for identifying medicinal plant species. PLoS One 5:e8613. doi: 10.1371/journal.pone.0008613
Chernoff, M., Plitmann, U., and Kislev, M. E. (1992). Seed characters and testa texture in species of the Vicieae: their taxonomic significance. Isr. J. Plant Sci. 41, 167–186. doi: 10.1080/0021213X.1992.10677225
Choi, B. H. (2007). “Fabaceae Lindl,” in The Genera of Vascular Plants of Korea, ed. C. W. Park (Seoul: Academy Publishing Co.), 111.
Choi, B. H., Seok, D. I., Endo, Y., and Ohashi, H. (2006). Phylogenetic significance of stylar features in genus Vicia (Leguminosae): an analysis with molecular phylogeny. J. Plant Res. 119, 513–523. doi: 10.1007/s10265-006-0015-6
Chung, G. Y., Chang, K. S., Chung, J. M., Choi, H. J., Paik, W. K., and Hyun, J. O. (2017). A checklist of endemic plants on the Korean Peninsula. Korean J. Plant Taxon. 47, 264–288. doi: 10.11110/kjpt.2017.47.3.264
Cold Spring Harbor DNA Learning Center (2014). Using DNA Barcodes to Identify and Classify Living Things. Available online at: http://www.dnabarcoding101.org/ (accessed March 20, 2020).
Dasmahapatra, K., and Mallet, J. (2006). Taxonomy: DNA barcodes: recent successes and future prospects. Heredity 97, 254–255. doi: 10.1038/sj.hdy.6800858
Endo, Y., Choi, B., Kakinuma, D., Kenicer, G., Zhu, X. Y., and Ohashi, H. (2010). Molecular phylogeny of Vicia sect. Amurense (Leguminosae). J. Japanese Bot. 85, 337–349.
Endo, Y., Choi, B. H., and Ohashi, H. (1996). The Infrageneric Positions of East Asian Species of Vicia (Leguminosae). J. Japanese Bot. 71, 254–261.
Endo, Y., Choi, B. H., and Ohashi, H. (2000). Distinction between Vicia americana and V. bungei (Leguminosae). J. Japanese Bot. 75, 92–97.
Endo, Y., Choi, B. H., Ohashi, H., and Delgado-Salinas, A. (2008). Phylogenetic relationships of new world Vicia (Leguminosae) inferred from nrDNA internal transcribed spacer sequences and floral characters. Syst. Bot. 33, 356–363. doi: 10.1600/036364408784571536
Endo, Y., and Ohashi, H. (1995). The morphology of styles and stigmas in Vicia (Leguminosae), and its systematic implications. J. Plant Res. 108, 17–24.
Erickson, D. L., Spouge, J., Resch, A., Weigt, L. A., and Kress, J. W. (2008). DNA barcoding in land plants: developing standards to quantify and maximize success. Taxon 57, 1304–1316. doi: 10.1002/tax.574020
Francis, C. M., Enneking, D., and El Moneim, A. M. (2000). “When and where will vetches have an impact as grain legumes?” in Linking Research and Marketing Opportunities for Pulses in the 21st Century, ed. R. Knight (Dordrecht: Springer), 375–384.
Fu, S. M., Hampton, J. G., and Forde, M. B. (1996). Identification and seed multiplication of a collection of Vicia and Lathyrus from southwest Europe. N. Z. J. Agric. Res. 39, 185–193. doi: 10.1080/00288233.1996.9513177
Gao, T., Yao, H., Song, J., Liu, C., Zhu, Y., Ma, X., et al. (2010). Identification of medicinal plants in the family Fabaceae using a potential DNA barcode ITS2. J. Ethnopharmacol. 130, 116–121. doi: 10.1016/j.jep.2010.04.026
Ghorbani, A., Saeedi, Y., and De Boer, H. J. (2017). Unidentifiable by morphology: DNA barcoding of plant material in local markets in Iran. PLoS One 12:e0175722. doi: 10.1371/journal.pone.0175722
Goloboff, P. A., and Catalano, S. A. (2016). TNT version 1.5, including a full implementation of phylogenetic morphometrics. Cladistics 32, 221–238. doi: 10.1111/cla.12160
Group, C. P. W., Hollingsworth, P. M., Forrest, L. L., Spouge, J. L., Hajibabaei, M., Ratnasingham, S., et al. (2009). A DNA barcode for land plants. Proc. Natl. Acad. Sci. U.S.A. 106, 12794–12797. doi: 10.1073/pnas.0905845106
Handi, Y. A. (1982). Application of Nitrogen Fixing Systems in Soil Management. Rome: FAO Boletín de suelos.
Hollingsworth, P. M., Graham, S. W., and Little, D. P. (2011). Choosing and using a plant DNA barcode. PLoS One 6:e19254. doi: 10.1371/journal.pone.0019254
Jalilian, N., Rahiminejad, M. R., Maassoumi, A. A., and Maroofi, H. (2014). Taxonomic revision of the genus Vicia L.(Fabaceae) in Iran. Iran. J. Bot. 20, 155–164.
Jung, S. Y., Lee, J. W., and Kwon, Y. H. (2016). Invasive Alien Plants in South Korea. Pocheon: Korea National Arboretum.
Koçak, A., Kokten, K., Bagci, E., Akçura, M., Hayta, S., Bakoglu, A., et al. (2011). Chemical analyses of the seeds of some forage legumes from Turkey. A chemotaxonomic approach. Grasas Y Aceites 62, 383–388. doi: 10.3989/gya.125210
Kress, W. J., and Erickson, D. L. (2007). A two-locus global DNA barcode for land plants: the coding rbcL gene complements the non-coding trnH-psbA spacer region. PLoS One 2:e508. doi: 10.1371/journal.pone.0000508
Kumar, S., Stecher, G., Li, M., Knyaz, C., and Tamura, K. (2018). MEGA X: molecular evolutionary genetics analysis across computing platforms. Mol. Biol. Evol. 35, 1547–1549. doi: 10.1093/molbev/msy096
Kupicha, F. K. (1976). The infrageneric structure of Vicia. Notes Roy. Bot. Gard Edinb. 34, 287–326.
Lee, Y. N. (1972). A cytotaxonomic study of Vicia unijuga complex in Korea. Korean J. Plant Taxon 4, 1–5. doi: 10.11110/kjpt.1972.4.1.001
Leht, M. (2005). Cladistic and phenetic analyses of relationships in Vicia subgenus Cracca (Fabaceae) based on morphological data. Taxon 54, 1023–1032. doi: 10.2307/25065486
Leht, M. (2009). Phylogenetics of Vicia (Fabaceae) based on morphological data. Feddes Repert 120, 379–393. doi: 10.1002/fedr.s200911117
Leht, M., and Jaaska, V. (2002). Cladistic and phenetic analysis of relationships in Vicia subgenus Vicia (Fabaceae) by morphology and isozymes. Plant Syst. Evol. 232, 237–260. doi: 10.1007/s006060200045
Li, C., Zhao, Y., Huang, H., Ding, Y., Hu, Y., and Xu, Z. (2018). The complete chloroplast genome of an inverted-repeat-lacking species, Vicia sepium, and its phylogeny. Mitochondrial DNA B 3, 137–138. doi: 10.1080/23802359.2018.1431071
Li, D. Z., Gao, L. M., Li, H. T., Wang, H., Ge, X. J., Liu, J. Q., et al. (2011). Comparative analysis of a large dataset indicates that internal transcribed spacer (ITS) should be incorporated into the core barcode for seed plants. Proc. Natl. Acad. Sci. U.S.A. 108, 19641–19646. doi: 10.1073/pnas.1104551108
Li, R., and Liu, C. (1996). DNA content of some taxa of Vicia sect. Vicilla in Northeast China. Cytologia 61, 225–228. doi: 10.1508/cytologia.61.225
Librado, P., and Rozas, J. (2009). DnaSP v5: a software for comprehensive analysis of DNA polymorphism data. Bioinformatic 25, 1451–1452. doi: 10.1093/bioinformatics/btp187
Liu, J., Moller, M., Gao, L. M., Zhang, D. Q., and Li, D. Z. (2011). DNA barcoding for the discrimination of Eurasian yews (Taxus L Taxaceae) and the discovery of cryptic species. Mol. Ecol. Resour. 11, 89–100. doi: 10.1111/J.1755-0998.2010.02907.X
Maddison, W. P., and Maddison, D. R. (2019). Mesquite: A Modular System for Evolutionary Analysis Version 3.61. Available online at: http://www.mesquiteproject.org (accessed June 15, 2020).
Martínez-Arce, A., De Jesús-Navarrete, A., and Leasi, F. (2020). DNA barcoding for delimitation of putative Mexican marine nematodes species. Diversity 12:107. doi: 10.3390/d12030107
Maxted, N., Khattab, A. M. A., and Bisby, F. A. (1991). Domesticated legumes and their wild relatives: the newly discovered relatives of Vicia faba L. do little to resolve the enigma of its origin. Botanika Chronika 10, 435–465.
Meier, R., Shiyang, K., Vaidya, G., and Ng, P. K. L. (2006). DNA barcoding and taxonomy in Diptera: a tale of high intraspecific variability and low identification success. Syst. Biol. 55, 715–728. doi: 10.1080/10635150600969864
Nam, B. M., Park, M. S., Oh, B. U., and Chung, G. Y. (2012). A cytotaxonomic study of Vicia L.(Fabaceae) in Korea. Korean J. Plant Taxon 42, 307–315. doi: 10.11110/kjpt.2012.42.4.307
Nguyen, L. T., Schmidt, H. A., Von Haeseler, A., and Minh, B. Q. (2015). IQ-TREE: a fast and effective stochastic algorithm for estimating maximum-likelihood phylogenies. Mol. Biol. Evol. 32, 268–274. doi: 10.1093/molbev/msu300
Nixon, K. C. (2008). ASADO, Version 1.85 TNT-MrBayes Slaver Version 2; Mxram 200 (vl 5.30). Available online at: http://www.diversityoflife.org/winclada (accessed May 24, 2020).
Noh, P., Yeo, S., Kim, W., Yang, S., Park, I., and Moon, B. (2018). Authentication of Brassicae semen and brassicae campestris semen by DNA barcode analysis. Hortic. Sci. Technol. 36, 885–898. doi: 10.12972/kjhst.20180086
Omar, G. I., Saqer, M. M., and Adwan, G. M. (2019). Phylogenetic relationship among some species of the Genera Lens, Vicia, Lathyrus and Pisum (Leguminosae) in Palestine. Jordan J. Biol. Sci. 12, 289–296.
Purty, R. S., and Chatterjee, S. (2016). DNA barcoding: an effective technique in molecular taxonomy. Austin J. Biotechnol. Bioeng. 3:1059.
Raveendar, S., Lee, J. R., Park, J. W., Lee, G. A., Jeon, Y. A., Lee, Y. J., et al. (2015). Potential use of ITS2 and matK as a Two-Locus DNA barcode for identification of Vicia Species. Plant Breed. Biotechnol. 3, 58–66. doi: 10.9787/PBB.2015.3.1.058
Raveendar, S., Lee, J. R., Shim, D., Lee, G. A., Jeon, Y. A., Cho, G. T., et al. (2017). Comparative efficacy of four candidate DNA barcode regions for identification of Vicia species. Plant Genet. Resour. 15, 286–295. doi: 10.1017/S1479262115000623
Rong, H., and Shiyuan, H. (1994). A study of genus Vicia from North China. Acta Bot. Boreal.-Occident. Sin. 14, 303–306.
Saski, C., Lee, S. B., Fjellheim, S., Guda, C., Jansen, R. K., Luo, H., et al. (2007). Complete chloroplast genome sequences of Hordeum vulgare, Sorghum bicolor and Agrostis stolonifera, and comparative analyses with other grass genomes. Theor. Appl. Genet. 115:591. doi: 10.1007/s00122-007-0567-4
Schaefer, H., Hechenleitner, P., Santos-Guerra, A., de Sequeira, M. M., Pennington, R. T., Kenicer, G., et al. (2012). Systematics, biogeography, and character evolution of the legume tribe Fabeae with special focus on the middle-Atlantic island lineages. BMC Evol. Biol. 12:250. doi: 10.1186/1471-2148-12-250
Scotland, R. W., Olmstead, R. G., and Bennett, J. R. (2003). Phylogeny reconstruction: the role of morphology. Syst. Biol. 52, 539–548. doi: 10.1080/10635150309309
Seok, D. I., and Choi, B. H. (1997). Taxonomic study on Vicia unijuga using principal components analysis. Korean J. Plant Taxon. 27, 359–368. doi: 10.11110/kjpt.1997.27.3.359
Shiran, B., Kiani, S., Sehgal, D., Hafizi, A., Chaudhary, M., and Raina, S. N. (2014). Internal transcribed spacer sequences of nuclear ribosomal DNA resolving complex taxonomic history in the genus Vicia L. Genet. Resour. Crop Evol. 61, 909–925. doi: 10.1007/s10722-014-0086-5
Smýkal, P., Kenicer, G., Flavell, A. J., Corander, J., Kosterin, O., Redden, R. J., et al. (2011). Phylogeny, phylogeography and genetic diversity of the Pisum genus. Plant Genet. Resour. 9, 4–18. doi: 10.1017/S147926211000033X
Som, A. (2015). Causes, consequences and solutions of phylogenetic incongruence. Brief. Bioinform. 16, 536–548. doi: 10.1093/bib/bbu015
Sveinsson, S., and Cronk, Q. (2016). Conserved gene clusters in the scrambled plastomes of IRLC legumes (Fabaceae: Trifolieae and Fabeae). bioRxiv [Preprint]. doi: 10.1101/040188
Van de Wouw, M., Maxted, N., and Ford-Lloyd, B. V. (2003). A multivariate and cladistic study of Vicia L. ser. Vicia (Fabaceae) based on analysis of morphological characters. Plant Syst. Evol. 237, 19–39. doi: 10.1007/s00606-002-0247-4
Veldman, S., Ju, Y., Otieno, J. N., Abihudi, S., Posthouwer, C., Gravendeel, B., et al. (2020). DNA barcoding augments conventional methods for identification of medicinal plant species traded at Tanzanian markets. J. Ethnopharmacol. 250:112495. doi: 10.1016/j.jep.2019.112495
Wen, J. (1999). Evolution of eastern Asian and eastern North American disjunct distributions in flowering plants. Ann. Rev. Ecol. Syst. 30, 421–455. doi: 10.1146/annurev.ecolsys.30.1.421
Wen, J., and Pandey, A. K. (2005). “Initiating DNA molecular systematic studies in a developing country,” in Plant Taxonomy: Advances and Relevance, eds A. K. Pandey, J. Wen, and J. V. V. Dogra (New Delhi: CBS Publishers & Distributors), 31–43.
Wu, F. F., Gao, Q., Liu, F., Wang, Z., Wang, J. L., and Wang, X. G. (2020). DNA barcoding evaluation of Vicia (Fabaceae): comparative efficacy of six universal barcode loci on abundant species. J. Syst. Evol. 58, 77–88. doi: 10.1111/jse.12474
Xie, Q., Zhang, H., Yan, F., Yan, C., Wei, S., Lai, J., et al. (2019). Morphology and molecular identification of twelve commercial varieties of kiwifruit. Molecules 24:888. doi: 10.3390/molecules24050888
Keywords: DNA barcoding, morphology, phylogeny, Vicia spp., species discrimination
Citation: Han S, Sebastin R, Wang X, Lee KJ, Cho G-T, Hyun DY and Chung J-W (2021) Identification of Vicia Species Native to South Korea Using Molecular and Morphological Characteristics. Front. Plant Sci. 12:608559. doi: 10.3389/fpls.2021.608559
Received: 21 September 2020; Accepted: 05 January 2021;
Published: 09 February 2021.
Edited by:
Isabel Larridon, Royal Botanic Gardens, Kew, United KingdomReviewed by:
Ann Abozeid, Northeast Forestry University, ChinaKelly Steele, Arizona State University, United States
Copyright © 2021 Han, Sebastin, Wang, Lee, Cho, Hyun and Chung. This is an open-access article distributed under the terms of the Creative Commons Attribution License (CC BY). The use, distribution or reproduction in other forums is permitted, provided the original author(s) and the copyright owner(s) are credited and that the original publication in this journal is cited, in accordance with accepted academic practice. No use, distribution or reproduction is permitted which does not comply with these terms.
*Correspondence: Do Yoon Hyun, dyhyun@korea.kr; Jong-Wook Chung, jwchung73@chungbuk.ac.kr
†These authors have contributed equally to this work