- 1Division of Vegetable Science, Indian Council of Agricultural Research-Indian Agricultural Research Institute, New Delhi, India
- 2Centre for Environment Science and Climate Resilient Agriculture, Indian Council of Agricultural Research-Indian Agricultural Research Institute, New Delhi, India
- 3Division of Genetics, Indian Council of Agricultural Research-Indian Agricultural Research Institute, New Delhi, India
Cauliflower is an important extensively grown cool season vegetable in India. Black rot and downy mildew are major devastating diseases reducing yield and quality of the crop. To tackle these through host plant resistance, a marker-assisted backcross breeding method was followed to pyramid a black rot-resistant gene (Xca1bo) and a downy mildew-resistant gene (Ppa3) from donors BR-161 and BR-2, respectively, into the background of Pusa Meghna cauliflower cultivar. Marker-assisted backcross breeding was followed up to BC2 generation using SCAR marker ScOPO-04833 and SSR marker BoGMS0624 for black rot and downy mildew resistance genes in foreground selection, respectively. In background selection, at each stage of backcrossing, 47 parental polymorphic SSR markers were used. The graphical genotyping of the five two-gene (Xca1boXca1boPpa3Ppa3) homozygous BC2F2 plants showed an average recovery of 85.44% of the Pusa Meghna genome with highest genome recovery of 91.7%. The genome contribution of donor parents (BR-161 and BR-2) was 8.26 with 6.34% of residual heterozygousity. The backcross derived pyramided lines BC2F2:3-7-16 and BC2F2:3-7-33 showed high resistance to both the diseases and exhibited higher yield and vitamin C content as compared with recipient parent Pusa Meghna. It is, therefore, evident from this study that resistant genes can be introgressed successfully into a Pusa Meghna cultivar without any yield penalty, benefitting farmers with reduced input cost and consumers with chemical residue free produce. Besides, the pyramided lines carrying dominant resistant genes can be exploited in a hybridization programme to develop hybrid(s) in cauliflower.
Introduction
Cauliflower (Brassica oleracea var. botrytis L.) is one of the most popular vegetables in the Brassicaceae family because of its high nutritive value as well as value added foods in the processing sector (Varalakshmi et al., 2011; Vanlalneihi et al., 2020). This herbaceous annual plant develops “curd” composed of highly suppressed pre-floral apical meristem, which is the main edible part (Sidki, 1962). Cauliflower originated in the island of Cyprus from where it was taken to Middle East and North Western Europe (Boswell, 1949). Dr. Jemson, a famous botanist at Kew garden, London, United Kingdom, the then In-charge of the Company Bagh (United Provinces, Saharanpur in the Northern plains of India), introduced cauliflower in India in the year 1822. Since then, cauliflower has been grown by farmers in North Indian plains from May to July (hot and humid weather). Farmers raised their own seeds from selected survived plants for raising next season crop and continued this process year after year, which led to adaptation of these new types to hot and humid climate later known as having wavy leaves, loosely arranged broad with short or long stalk Indian cauliflower (Swarup and Chatterjee, 1972). This plant matures from mid-September to mid-October and is sold at high market prices.
China is the leading country for production of cauliflower followed by India, Spain, Italy, France, United States, and United Kingdom. India has very low productivity (19.76 MT/ha) in contrast to other countries (Egypt, 29.93 MT/ha; Italy, 24.73 MT/ha; Poland 22.29 MT/ha) (FAO, 2014). The low productivity is mainly due to susceptibility of commercial varieties and hybrids to various biotic and abiotic stresses. Black rot and downy mildew are the two most devastating diseases that cause tremendous quality and yield loss in cauliflower, and these are predominant in most cauliflower-growing states of India (Singh et al., 2012, 2015; Saha et al., 2014, 2016, 2020; Verma and Singh, 2018). Black rot disease causing bacteria Xanthomonas campestris pv. campestris enter inside the edge of the leaves through hydathodes or wounds. The disease proliferates through vascular tissue by clogging the vessels and produces V shaped chlorotic lesions. The color of the infected veins turns black with chlorotic or necrotic interveinal tissue. Affected plants develop small-sized curds resulting in huge crop loss to farmers (Vicente et al., 2001; Doullah et al., 2011; Vicente and Holub, 2013; Saha et al., 2014; Kalia et al., 2017; Bernabé et al., 2019; Sheng et al., 2020). Downy mildew caused by obligate fungal parasite Hyaloperonospora parasitica (Pers. Fr.) Fr. (Giovannelli et al., 2002) is another important disease of cauliflower, which damages seedlings as well as mature crops (Laemmlen and Mayberry, 1984; Verma and Thakur, 1989; Singh et al., 2012; Verma and Singh, 2018; Saha et al., 2020). This also damages the crop in the postharvest stage (Lund and Wyatt, 1978; McKay et al., 1992). The disease reduces yield as well as curd quality because of adult plant defoliation; and if the damage is severe, the produce becomes unmarketable. Butler (1918) first reported this disease in India and presently, it is predominant in most cauliflower growing-states of India. Both the diseases, i.e., black rot and downy mildew, can be managed through cultural practices and using bactericide or fungicide, respectively (Thakur and Mathur, 2002). Nevertheless, these practices are time-consuming besides chemicals being environment polluter and health hazardous. Therefore, most sustainable approach is exploration of host plant resistance to develop resistant varieties that can offer a reliable and practical solution for effective disease management. The development of high-yielding cauliflower with multiple disease resistance through conventional backcross methods requires 8–9 years or more. Marker-assisted selection (MAS) is a simple method for transferring gene(s)/QTL(s) effectively and precisely besides hastening the process of varietal development. Resistant gene-linked molecular markers can provide another powerful approach to pyramid various resistant gene(s) into a single variety (Das and Rao, 2015; Arunakumari et al., 2016; Nguyen et al., 2018; Hsu et al., 2020).
Black rot resistance is governed by a single dominant gene at the seedling stage in the SN 445 genotype and at the adult plant stage in the BR-161 and BR-207 genotypes of cauliflower (Jamwal and Sharma, 1986; Saha et al., 2014, 2016). There are many R genes/QTLs as well as co-segregating DNA markers for resistance to black rot disease reported in cauliflower and other related crops (Camargo et al., 1995; Soengas et al., 2007; Kaur et al., 2009; Doullah et al., 2011; Kifuji et al., 2013; Tonu et al., 2013; Saha et al., 2014; Lee J. et al., 2015; Lee Y. G. et al., 2015; Sharma et al., 2016; Bernabé et al., 2019). SCAR markers linked to the black rot-resistant gene Xca1bo in cauliflower were developed by Kalia et al. (2017). Downy mildew resistance is governed by a single dominant gene in cauliflower (Mahajan et al., 1995; Jensen et al., 1999; Farnham et al., 2001; Verma and Singh, 2018; Saha et al., 2020) and broccoli (Coelho and Monteiro, 2003); two independent dominant genes (Carvalho and Monteiro, 1996), and three to four complementary dominant genes (Hoser Krauze et al., 1995). Previous workers also tagged downy mildew-resistant genes (Agnola et al., 2000; Farinhó et al., 2000, 2007). A resistance locus at the cotyledon stage was reported by Giovannelli et al. (2002) that led to the identification of some putative-resistant gene homologs (Gao et al., 2003). A monogenic dominant locus Pp523 was identified in adult plants of broccoli by Coelho and Monteiro (2003). Four closely linked molecular markers along with a marker linked in repulsion phase were also developed (Farinhó et al., 2004). Singh et al. (2013) identified three markers linked to a downy mildew resistant-gene (Ppa3). Very recently, SSR markers linked to the downy mildew resistant-gene were identified (Saha et al., 2020).
Pusa Meghna, an early maturity group Indian cauliflower is susceptible to both black rot and downy mildew diseases causing huge losses to the farmers. Therefore, this study was under taken with the aim to pyramid genes resistant to black rot (Xca1bo) and downy mildew (Ppa3) diseases in Pusa Meghna through marker-assisted backcross breeding. A large number of mapped cauliflower or other Brassica specific microsatellite markers (>1,500) were available at the time when this program was initiated (Lowe et al., 2004; Li et al., 2011). These are sequence-specific co-dominant markers ideal for background selection. These allow accurate determination of the allelic arrangement of the offspring by detecting alleles coming from both the parents. Through marker-assisted breeding, it is expected that both black rot- and downy mildew-resistant loci will be introgressed into Pusa Meghna Indian cauliflower, and that the inherent morphological traits will be maintained.
Materials and Methods
Plant Materials and Development of Black Rot and Downy Mildew Resistant Lines
Pusa Meghna, an early maturity group cauliflower variety with excellent curd quality, was used as the recurrent parent. It forms white compact medium sized curd (350–400 g) with an average yield of 175 q/ha. The genotypes BR-161 (carrying black rot-resistant gene Xca1bo) and BR-2 (carrying downy mildew-resistant gene Ppa3) were used as donor parent for black rot- and downy mildew disease-resistant genes, respectively (Singh et al., 2013; Saha et al., 2014). The genotypes BR-161 and BR-2 are the homozygous inbred lines maintained at the Division of Vegetable Science, ICAR-Indian Agricultural Research Institute, New Delhi, India. For targeted introgression of Xca1bo and Ppa3 genes into Pusa Meghna, marker-assisted a backcross breeding (MABB) strategy was followed (Figure 1). Initially, both the resistant donor parents were crossed among themselves and the resultant F1 (BR-161 × BR-2) was crossed with Pusa Meghna. The resultant double cross F1 {Pusa Meghna × (BR-161 × BR-2)} was backcrossed with recurrent parent “Pusa Meghna” and a BC1F1 population was generated. Foreground selection was performed for the desired resistant genes (Xca1bo and Ppa3), and the background analysis was conducted to know the recurrent parent genome recovery. The selected plants having maximum recurrent parent genome recovery along with the desired resistant genes were again backcrossed with Pusa Meghna and BC2F1 generation was generated. The best plant was selected and selfed to develop BC2F2 generation. To identify homozygous black rot-resistant plant in the BC2F2 generation, BC2F3 plants were generated by selfing each BC2F2 plant. In every backcross generation, the plants that showed the heterozygous allele for BR-161 and BR-2 were selected. In the BC2F3 population, five homozygous plants carrying both resistant genes along with a maximum genome of Pusa Meghna were selected finally.
DNA Isolation and Marker Assisted Foreground Selection for Black Rot- and Downy Mildew-Resistant Genes
CTAB method with slight modifications was used for isolating genomic DNA of parents and that of each backcross generation plants (Doyle and Doyle, 1990). The closely linked SCAR marker ScOPO-04833 linked to the Xca1bo gene and SSR marker BoGMS0624 linked to the Ppa3 gene were used for selecting the target genes under foreground selection. The SCAR marker ScOPO-04833 was previously developed by Kalia et al. (2017) and is linked to the black rot-resistant locus Xca1bo at 1.6 cM distance. The SSR marker BoGMS0624 was previously identified from the 92 RIL population of Pusa Himjyoti × BR-2 (unpublished data). This marker is linked to the downy mildew-resistant locus Ppa3 at 3.26 cM distance. Backcross plants (BC1F1) were screened with the SCAR marker ScOPO-04833 to identify plants carrying the Xca1bo gene for black rot disease resistance. The sequences of primers are mentioned in Table 1. PCR and gel electrophoresis protocols followed by Williams et al. (1990) and Kalia et al. (2017) were used for the marker-assisted selection of Xca1bo and Ppa3, respectively. The plants that had the marker for black rot resistance in the BC1F1 population were then screened with a BoGMS0624 marker for the presence of the Ppa3 gene under heterozygous conditions.

Table 1. SCAR and SSR markers used for foreground selection of the black rot (Xca1bo) and downy mildew resistance (Ppa3) genes.
Parental Polymorphism Survey for Background Analysis of Pyramided Lines
The polymorphism survey was carried out using previously reported 225 SSR markers (Li et al., 2011). The chromosome-wise markers used for polymorphism survey are given in Table 2. The PCR protocol was followed as per Saha et al. (2020). The identified BC1F1 plants were genotyped with 47 parental polymorphic SSR markers. The same steps as described above were followed in BC2F1 generation. In the BC2F2, generation, the plants were screened with the linked markers to identify resistant plants showing homozygous condition. These plants were then screened using parental polymorphic SSR markers for the identification of the best BC2F2 plants. As the SCAR marker for the black rot-resistant gene Xca1bo is dominant in nature, progeny testing/phenotyping in BC2F3 was performed to identify plants carrying Xca1bo under homozygous conditions in BC2F2.
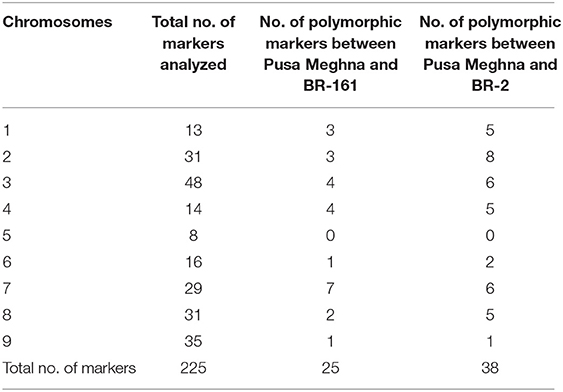
Table 2. Chromosome-wise microsatellite markers used for polymorphism survey between the parents Pusa Meghna, BR-161 and BR-2, and the number of polymorphic markers.
Raising of Parents and Pyramided Lines
In the research farm of Division of Vegetable Science, ICAR-Indian Agricultural Research Institute, New Delhi, India, all parental lines, F1, BC1F1, BC2F1, BC2F2, and BC2F3 were grown. The research farm is situated at an altitude of about 228.61 m above mean sea level, with 28°08′N latitude and 77°12′E longitude. The climate is sub-temperate and semiarid, and the soil type is alluvial. Summer is very hot and relatively dry, with an average temperature of above 40°C, and winter is cool with a temperature of below 5°C.
During the 1 week of July, the seeds were sown in nursery and 1-month-old seedlings were transplanted in the main field with 60 cm distance between the rows and 45 cm within rows. Recommended package of practices were followed to raise the crop as described by Thamburaj and Singh (2003). Before transplanting, 22 tons of farmyard manure; 55 kg N: 70 kg P: 60 kg K per hectare were applied to the field. A month after transplanting, a dose of 35 kg N was applied again at the time of first earthing up. During curd initiation, a similar dose of N was applied at second earthing up. Before transplanting, Stomp (Pendimethalin @ 3.5 l/hectare) was applied for controlling the weed. For maintaining sufficient humidity, broad bed furrow method of irrigation was applied at a fortnight interval.
Screening for Black Rot Resistance in BC2F3
The strain of Xanthomonas campestris pv. campestris race 1 (isolate number Xcc-C 1) was used for screening the parents and BC2F3 population. The isolate was provided by Dr. Dinesh Singh, Division of Plant Pathology, ICAR-Indian Agricultural Research Institute, New Delhi, India. The bacterial culture having 0.1 optical density at 600 nm was diluted to 19 × 108 CFU ml−1 before inoculation. The first inoculation was done at 15 days after transplanting and the second at 45 days after transplanting. Three inner whorl young leaves were selected in each plant and inoculated by following leaf cut and dip technique as described by Kapoor et al. (1985). The scoring of inoculated plants was done in a scale of 0–4 for three times in each inoculation at 20 days interval as per Tewari et al. (1987). The results were observed after 60 days of inoculation, and infected leaves were scored accordingly. The disease score was 0 for no visible symptom, 1 for minute symptom, (one or two tiny lesions at the leaf edge), and 2 was for a handful medium-sized slow-progressing “V” shaped lesions spreading up to 20% of leaf area, which were rated as resistant. On the other hand, 3 was scored for numerous large V-shaped lesions spreading almost half of the leaves, which resulted in leaf wilting and distortion, and 4 for major infection spreading more than 75% of the entire leaf area, which leads to the wilting of leaves, scored as susceptible.
Screening for Downy Mildew Resistance in BC2F3
The conidial suspension of H. parasitica Delhi isolate at 5 × 103 spores ml−1 was used for artificial inoculation of parents and BC2F3 plants in the field (Mahajan et al., 1995). The inoculum was sprayed on both sides of all leaves using a pneumatic knapsack sprayer at 30 days after transplanting. All diseased leaves of the downy mildew-susceptible parent were retained to increase the disease pressure in the infector rows of the experimental block. A five-point scale, as suggested by Mahajan et al. (1995) and modified by Pandey et al. (2001), was used to score the plants 15 days after inoculation. The percentage disease incidence (DI) was calculated by observing the infected areas of each leaf along with infected leaves per plant. The plants were categorized as resistant (R; 1 < DI ≤ 10); moderately resistant (MR; 10 < DI ≤ 25); moderately susceptible (MS; 25 < DI ≤ 50); susceptible (MS; 50 < DI ≤ 75), or highly susceptible (HS; 75 < DI ≤ 100) on the basis of their DI values.
Microstructure Analysis of Pyramided Lines
For microstructure analysis, the STRUCTURE 2.3.4 software, which uses a systematic Bayesian clustering approach based on Markov chain Monte Carlo (MCMC) estimation, was utilized to study the fundamental genetic structure within pyramided lines (Pritchard et al., 2000). K, the number of presumed populations, was set from 1 to 10. For each run, the burn-in period was set to 10,000, and MCMC (Markov Chain Monte Carlo) was set to 1; 50,000 each with iterations going up 7. The value of K in DK (log probability for the rate of change of the data) by the Evanno Test (Evanno et al., 2005) was identified to determine the optimal number of subgroups in the lines. To visualize the outputs, “Structure Harvester” was used from which plots, such as Evanno plots, were constructed.
Evaluation for Agro-Morphological and Quality Traits of the Pyramided Lines
The selected BC2F3 plants along with the parental lines were evaluated for 2 years in replicated trials. Twenty plants in each of pyramided lines were planted in a randomized block design (RBD) at a spacing of 60 × 45 cm at the research farm of Division of Vegetable Science, ICAR-Indian Agricultural Research Institute, New Delhi, India. The details of experimental location and package of practices are already given in the section of raising of parents and pyramided lines. Observations were recorded from three randomly selected plants in each line for five agro-morphological and yield related traits viz., plant height (cm), days to 50% curd maturity, curd angle (°), marketable curd weight (kg), curd yield (q/ha) as per distinctness, uniformity, and stability (DUS) guidelines of cauliflower (PPV and FRA, 2009). Vitamin C was estimated from the curd at the edible stage of maturity as per the protocol with some modifications as given by Jagota and Dani (1982) and Vanlalneihi et al. (2020).
Statistical Analysis
The segregation of markers in the BC2F2 population was analyzed for goodness-of-fit by chi square (χ2) analysis (Panse and Sukhatme, 1967). To determine the significant variation among the pyramided lines for yield and quality traits, ANOVA was performed online (http://14.139.232.166/opstat/onefactor.htm). To calculate the amount of recurrent parent (Pusa Meghna) genome contribution in pyramided lines, software programme Graphical genotypes (GGTs) version 2.0 was used (van Berloo, 2008).
Results
Parental Polymorphism Survey for Foreground and Background Selection
During parental polymorphism survey using linked markers, the SCAR marker ScOPO-04833 amplified fragments of ~833 bp in the resistant parent (BR-161), but was absent in susceptible parent Pusa Meghna. With respect to the SSR marker BoGMS0624, a band of 350 bp fragment was amplified in BR-2 compared with that of Pusa Meghna in which a fragment of 320 bp was amplified. Thus, resistant lines were distinguished from susceptible one using linked markers. Out of 225 SSR markers used for parental polymorphism survey for background selection, 25 markers indicated polymorphism (11.11%) between Pusa Merghna and BR-161, and 38 (16.88%) markers were polymorphic between Pusa Meghna and BR-2 (Table 2).
Introgression of Black Rot- and Downy Mildew-Resistant Genes Into Pusa Meghna Background
A total of 51 BC1F1 plants were first checked for the presence of the black rot-resistant gene Xca1bo using linked SCAR marker ScOPO-04833. Out of these, 23 plants were found positive for black rot resistance (Xca1boxca1bo), and these plants were checked for the presence of the downy mildew-resistant gene (Ppa3) under heterozygous (Ppa3ppa3) conditions using linked SSR marker BoGMS0624. Finally, five BC1F1 plants were found to be resistant for both black rot and downy mildew diseases (Figure 2). These five plants were compared with the recurrent parent Pusa Meghna in field for morphological traits and, finally, selected for background analysis, and the plants having maximum recurrent parent genome contribution were identified. A total of 65 BC2F1 plants were tested for the presence of black rot-resistant gene, out of which 30 plants were found positive for SCAR marker ScOPO-04833. These lines were then tested for the presence of downy mildew-resistant gene and, finally, 14 plants were found heterozygous for the downy mildew-resistant gene. Out of the 14 plants, five were selected based on their maximum phenotypic similarity with recurrent parent Pusa Meghna.
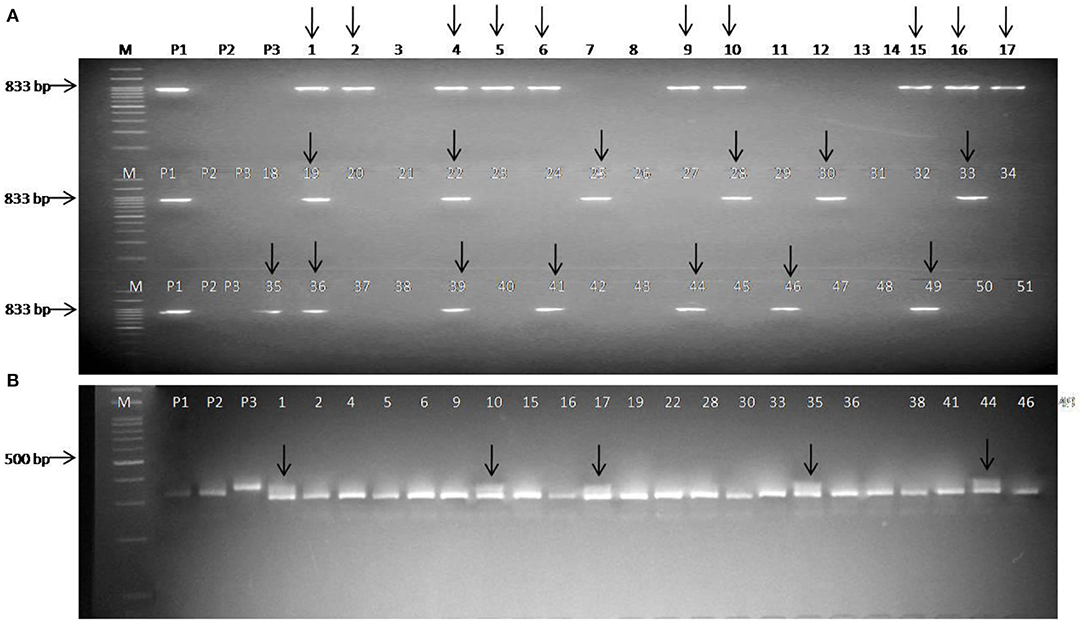
Figure 2. Foreground selection in BC1F1 plants. (A) Screening of plants with the SCAR marker ScOPO-04833 and arrow indicates the plants positive for black rot-resistant gene Xca1bo; (B) screening of Xca1bo-positive plants using Ppa3-linked marker BoGMS0624 and arrow indicates positive for downy mildew-resistant gene Ppa3; Lanes M: 100 bp ladder; P1: donor (BR-161) for black rot-resistant gene; P2: Pusa Meghna (recurrent parent); P3: donor (BR-2) for downy mildew-resistant gene.
A total of 102 BC2F2 plants were screened using the black rot resistant-gene (Xca1bo)-linked SCAR marker ScOPO-04833 to identify plants that are positive for black rot resistance, and, finally, 78 plants were found to show resistant parent specific band. The marker segregated into 3:1 ratio (78 homozygous/heterozygous resistant: 24 homozygous susceptible) with a chi square (λ2) value of.11 (P = 0.7–0.8). Then, the 78 black rot-resistant plants were checked for the downy mildew-resistant gene (Ppa3) using linked SSR marker BoGMS0624. Out of these 78 plants, 21 homozygous-resistant plants, 42 heterozygous-resistant plants, and 15 homozygous-susceptible plants were observed, which segregated in the ratio of 1:2:1 (HoR:HeR:HoS) with (λ2) value of 1.38 (P = 0.5–0.7). Both markers exhibited similarity to the expected marker segregation ratio (3:1/1:2:1) (Table 3).
Phenotypic Evaluation of BC2F2:3 Plants for Black Rot Resistance
In order to identify the plants carrying the black rot resistant-gene under homozygous conditions, a phenotypic screening of BC2F2:3 progenies of 21 BC2F2 plants (carrying the black-rot resistant gene in homozygous/heterozygous form and the downy mildew-resistant gene in homozygous form) was carried out. Out of 21 progeny rows, 16 segregated into resistant and susceptible plants, and five progeny rows were resistant, which were finally selected as homozygous for the black rot-resistant gene (Xca1boXca1bo). The data of black rot resistance of the five homozygous plants showed resistant reaction (Table 4). The susceptible parent Pusa Meghna showed a PDI value of 4, whereas the resistant parent BR-161 showed 0 PDI value. As compared with Pusa Meghna, the pyramided lines showed a PDI value of 0–1.63. During phenotyping, there were very few symptoms or no symptom of the black rot disease on pyramided lines. The lines BC2F2:3-7-16 and BC2F2:3-7-52 showed a PDI value of 0, and BC2F2:3-7-33, BC2F2:3-7-9, and BC2F2:3-7-72 showed a PDI value of 0.28, 0.5, and 1.63, respectively. They also exhibited morphological characteristics similar to those of susceptible parental line Pusa Meghna.
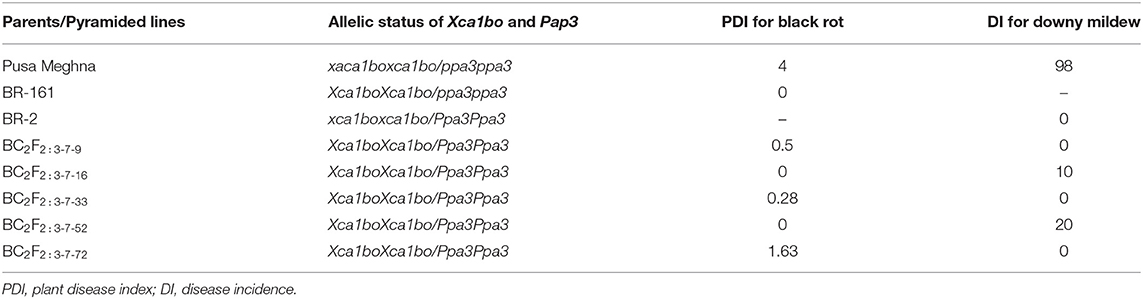
Table 4. Screening of two-gene positive BC2F2:3 plants for resistance against black rot and downy mildew disease.
Phenotypic Evaluation of BC2F2:3 Plants for Downy Mildew Resistance
The phenotyping of 5 BC2F2:3 lines, which were homozygous for downy mildew resistance, were screened phenotypically for downy mildew resistance along with parents as per the protocol described in Materials and methods. The parent Pusa Meghna was highly susceptible to the downy mildew disease with a DI value of 98%, whereas resistant parent BR-2 was free from the disease with a DI value of 0. Among the pyramided lines, all were found to be resistant and DI ranged from 0 to 10 (Table 4) and three lines, viz. BC2F2:3-7-9, BC2F2:3-7-33, and BC2F2:3-7-72 had, 0 DI; whereas two lines, namely BC2F2:3-7-16 and BC2F2:3-7-52, had a DI value of 10 and 20, respectively.
Background Genome Analysis of Backcross Derived Black Rot and Downy Mildew Resistant Lines
The background analysis of the five BC1F1 plants was carried out using 47 parental polymorphic SSR markers. The number of double gene heterozygotes/homozygote plants in the BC1F1, BC2F1, and BC2F2 populations and the maximum recurrent parent genome contribution to the selected plants are provided in Table 5. It was observed that in BC1F1 generation, the genome recovery ranged from 60.56 to 69.7%, and plant number 4 had the maximum recovery. The selected plants were backcrossed for BC2F1 generation. The background analysis of the 5 BC2F1 showed that the extent of genome recovery ranged from 81.78 to 86.5% with maximum genome recovery in plant number 3 (86.5%) followed by plant number 5 (85.69%). The background analysis of five BC2F2 plants with polymorphic SSR markers BoGMS0836 and BoGMS1020 is shown in Figure 3. For SSR marker BoGMS0836, plant numbers 1, 2, and 4 showed Pusa Meghna-specific band. In the case of SSR marker BoGMS1020, individuals 3, 4, and 5 had Pusa Meghna-specific band. The graphical genotyping of the five two-gene homozygous BC2F2 plants using Graphical genotypes (GGTs) version 2.0 is shown in Figures 4, 5. The analysis revealed an average recovery of 85.44% of the Pusa Meghna genome, whereas the recovery of BR-161 and BR-2 genomes was 8.26 with 6.34% residual heterozygosity. Background analysis on the basis of linkage groups revealed that on all the chromosomes, at least some individuals showed short segments from donor parents ranging from 1.1 to 19.4% inheritance (Figure 4, Table 6). Highest genome recovery of 97% from Pusa Meghna was observed on chromosome 6. The genome recovery of five selected lines ranged from 80.9 to 91.7%. Highest genome recovery of 91.7% of Pusa Meghna was observed in individual 4, followed by 86.4 and 84.9% of recovery in individuals 5 and 3, respectively. The chromosome-wise recurrent parent genome recovery of plant number 4 is given in Figure 5. Individual 4 inherited chromosomes 7, 8, and 9 entirely from Pusa Meghna, whereas short segments were derived from donor parents on chromosomes 1, 2, 3, 4, and 6.

Table 5. Number of double resistance gene heterozygotes/homozygous plants identified and estimation of recurrent parent genome recovery.
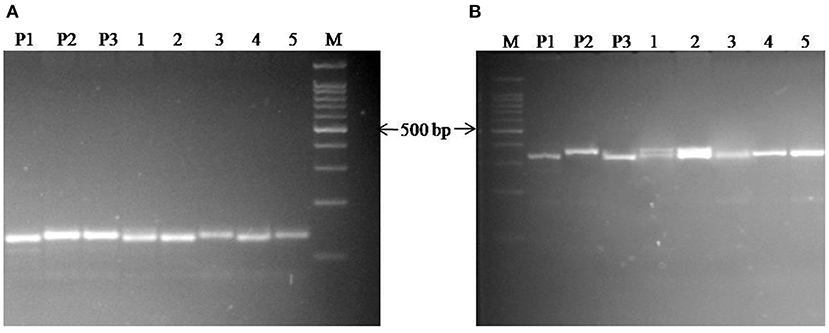
Figure 3. Background analysis of 5 BC2F2 plant with the polymorphic markers (A) for SSR marker BoGMS0836; (B) for SSR marker BoGMS1020; Lanes M: 100 bp ladder; P1: Pusa Meghna (recurrent parent); P2: donor (BR-161) for black rot-resistant gene; P3: donor (BR-2) for downy mildew-resistant gene; Lanes 1–5 are the BC2F2 plants.
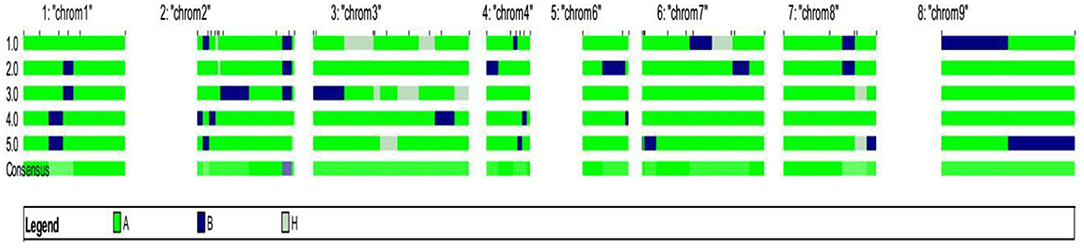
Figure 4. Chromosome-wise recurrent genome recovery of the five selected plants in BC2F2 generation.
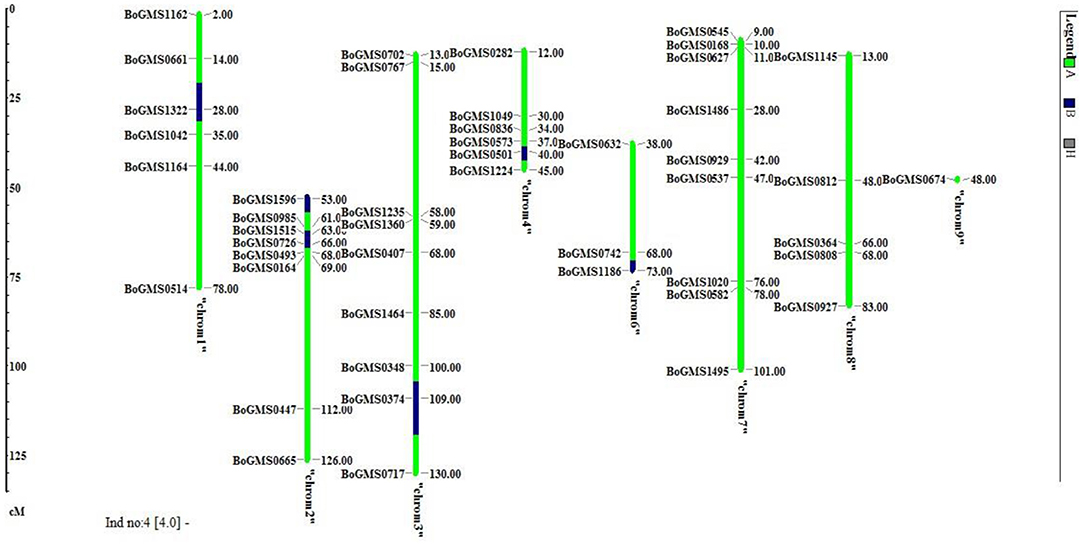
Figure 5. Chromosome-wise recurrent genome recovery of the individual plant number 4 in BC2F2 generation.
Microstructure Analysis of Pyramided Lines
Evanno test denoted the optimum K-value by observing the highest peak for delta K = 2 in the plots of L (K) vs. delta, confirming a likely allocation of the cauliflower pyramided lines into two subgroups. The individual membership coefficient at K = 2 had maximum mean probability of likelihood value of L (K) = −158.84, and the result of the structure analysis classified the lines in two groups. Individuals 1 and 2 were clustered in a group, while individuals 3, 4, and 5 were clustered in the other group (Figure 6). There were no admixed individuals.
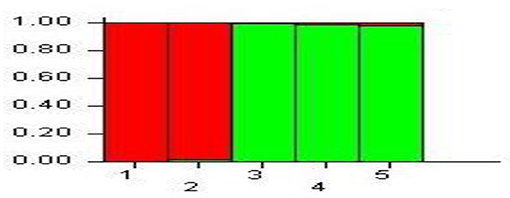
Figure 6. Microstructure analysis of five individual plants of BC2F2 generation (1: individual plant number 1; 2: individual plant number 2; 3: individual plant number 3; 4: individual plant number 4; 5: individual plant number 5.
Agro-Morphological and Quality Traits of Pyramided Lines
After phenotypic screening of the pyramided lines for black rot and downy mildew resistance, five BC3F2:3 lines were evaluated for quality and yield-related parameters for 2 years. The morphological view of susceptible Pusa Meghna and improved Pusa Meghna is shown in Figure 7. The observations were analyzed in RBD, and mean values of 5 lines are presented in Table 7. The pyramided lines did not differ significantly from recurrent parent Pusa Meghna for any of the traits. In both years of evaluation, the pyramided lines BC2F2:3-7-16 and BC2F2:3-7-33 exhibited high yield at par with Pusa Meghna (172.54/176.36 q/ha) with marginal differences. Also, these lines did not differ significantly from Pusa Meghna with respect to curd weight, curd yield, and vitamin C content. The line BC2F2:3-7-33 exhibited higher yield (182.5/182.78 q/ha) compared with Pusa Meghna (172.54 q/ha) in both years. This line also exhibited higher vitamin C content (48.56/47.67 mg/100 g FW) compared with that of recurrent parent Pusa Meghna (45.76/45.34 mg/100 g FW) in both years.
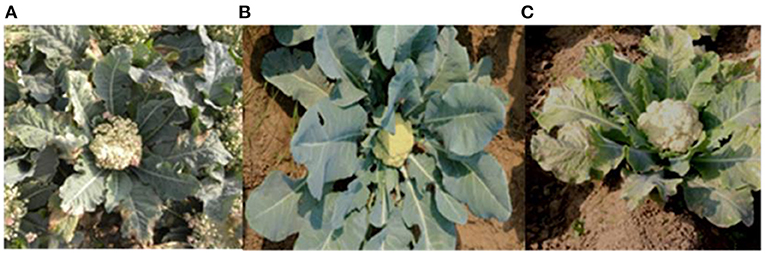
Figure 7. Morphological view of Pusa Meghna (susceptible) and improved Pusa Meghana (resistant to both black rot and downy mildew at curd maturity stage during evaluation of BC2F2:3 lines; (A) black rot-susceptible Pusa Meghna (B) improved Pusa Meghna (BC2F2:3-7-16) (C) downy mildew-susceptible Pusa Meghna.
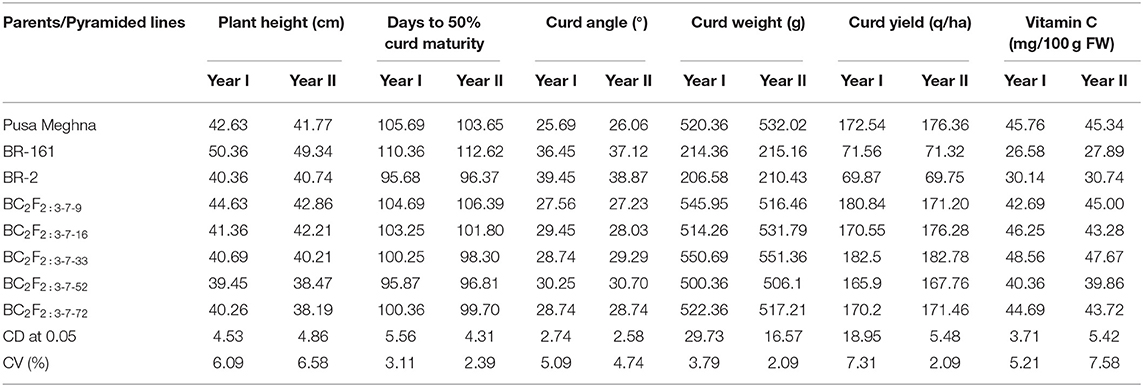
Table 7. Mean values of morphological, yield, and quality traits of two resistant genes pyramided BC2F2:3 lines along with parents.
Discussion
Cauliflower production is hindered by many biotic stresses, among which black rot and downy mildew impose huge yield and quality losses (Singh et al., 2011; Saha et al., 2014, 2020; Verma and Singh, 2018). These challenging issues could be overcome by cultivating resistant variety(s) (Farinhó et al., 2004, 2007; Carlier et al., 2012; Saha et al., 2020). In conventional breeding, introgression of resistant gene(s) involves patho-phenotypic selection. It is a challenging and time- and resource-consuming process. The success of conventional breeding also depends on the accuracy of disease scoring, favorable environmental conditions for disease development, and availability of appropriate virulent pathogenic strain (Sundaram et al., 2014).
Marker-assisted selection is a quick, simple, and effective method for the improvement of susceptible genotypes (Lee J. et al., 2015; Lee Y. G. et al., 2015). However, recurrent parent selection in the backcross breeding programme is vital for marker-assisted breeding (Ye and Smith, 2010). In marker-assisted selection, resistant gene-linked markers help in introgression of the identified gene(s) into recipient cultivars. DNA marker technology can tag novel resistant gene(s) efficiently and provide a straight forward path for identifying and transferring gene(s) into various crops (Gu et al., 2013; Patroti et al., 2019). In previous studies, MAS has been performed to few vegetable crops such as tomato for resistance to tomato leaf curl virus (ToLCV) (Kumar et al., 2014; Prasanna et al., 2014), late blight, and root knot nematode (Kumar et al., 2019); and in pepper for resistance to PVY, TSWV, and PMMoV (Özkaynak et al., 2014). Many QTLs/gene(s) have been reported for both black rot and downy mildew diseases in cauliflower; however, there has been no report of transferring these genes(s)/QTLs through MAS. Therefore, the similar strategy was followed in this study to transfer black rot- and downy mildew-resistant genes in susceptible commercial variety Pusa Meghna along with phenotypic selection for agro-morphological traits.
In the donor parent BR-161, the Xca1bo gene showed complete dominance against Xcc race 1 and in parent BR-2, the Ppa3 gene also showed dominance against the downy mildew disease (Singh et al., 2012; Saha et al., 2014). SCAR marker ScOPO-04833 and SSR marker BoGMS0624 were used to identify double positive (Xca1bo and Ppa3) plants in pyramided lines. The highly polymorphic nature of both markers made it a very effective tool to transfer Xca1bo and Ppa3 resistant genes into susceptible cauliflower variety Pusa Meghna. In the BC2F2 population, both the linked markers segregated in expected Mendelian ratio, which was similar to the results of Tanweer et al. (2015), Miah et al. (2017), and Chukwu et al. (2020). However, the strong linkage of markers with the target gene is the main principle for conducting a successful MAS study (Tanweer et al., 2015).
In this study, polymorphic SSR markers were used for background selection to recover recurrent parent genome in a minimum number of backcross generations. Hence, a set of microsatellite markers that showed polymorphism between the donor and recurrent parents was used in the selection process of the recurrent parent (Pusa Meghna) genome at each backcross generation. The contribution of the recurrent parent genome at each generation of backcrossing was also estimated. In the improved lines, major part of the recurrent parent DNA segment was recovered, but in some of the improved lines, some chromosomal portions could not be recovered. Few heterozygous DNA segments were also found in some of the improved lines. The results are in line with those of Tian et al. (2006) and Hirabayashi et al. (2010). This study was helpful for getting high recovery of the recurrent parent genome and introgression of target genes into Pusa Meghna following two backcrosses and one selfing. Tanweer et al. (2015) described that full recovery of the recurrent parent genome segment is possible only in the BC3F2 generation and not in the BC2F2 generation. During transfer of multiple genes, at least three backcrosses are needed to recover the recurrent parent genome (Shu, 2009).
All the BC2F2:3 lines derived from the BC2F2 population showed strong resistance to both black rot (Xcc race 1) and downy mildew diseases. In India, in the case of the black rot disease, three races (races 1, 4, and 6) have been indentified of which races 1 and 4 are predominant (Singh et al., 2016). In this study, only Xcc race 1 was used, and the pyramided lines showed race-specific resistance. It is important to test the pyramided lines against other isolates/races to assess the spectrum of resistance. There is no report of pathogenic variability for downy mildew causing pathogen H. parasitica in India, and the pyramided lines showed resistance to H. parasitica Delhi isolate. Moreover, the yield level of these lines also did not vary significantly from that of the parent Pusa Meghna, indicating no yield penalty associated with presence of resistant genes. The pyramided lines BC2F2:3-7-16 and BC2F2:3-7-33 were found to be superior based on their high level of resistance to both black rot and downy mildew diseases, high yield, and vitamin C content. Therefore, these improved resistant pyramided lines would offer yield improvement and provide great advantage in areas where these two diseases are highly prevalent. The introgression of resistant genes for both black rot and downy mildew diseases and higher recovery of the genome of the recurrent parent in improved pyramided lines (BC2F2:3-7-33 and BC2F2:3-7-16), and higher yield are the significant achievements of this research, which will go a long way in reducing the use of chemicals, thus protecting the environment from being polluted and reducing pesticide residues from the produce, making it safe for human consumption. Besides, using the target-resistant genes in the foreground selection, the cost as well as the time for recovering the desirable recombinants can also be minimized to a great extent (Tanweer et al., 2015).
A higher recovery of desirable improved plants of the pyramided lines BC2F2:3-7-33 and BC2F2:3-7-16 was obtained because of phenotypic-based selection in each backcross generation. The current strategies of phenotypic and marker-based selection are consistent with the findings of Joseph et al. (2004), Gopalakrishnan et al. (2008), Tanweer et al. (2015), and Patroti et al. (2019), who adopted the phenotypic and marker-assisted selection for the target traits. In this study, the phenotypic-based selection process was adopted from the BC1F1 generation onward for the agro-morphological traits while screening a large number of backcross plants. Improved resistant pyramided lines exhibited an almost identical agro-morphological performance in the field, which was at par with the recurrent parent Pusa Meghna, and even the microstructure analysis classified these lines into two groups. There were no positive or negative interactions observed between both the resistant genes as observed in previous study of Sundaram et al. (2008); Hari et al. (2011), and Balachiranjeevi et al. (2015). The present results proved the efficiency of the phenotypic selection practice. Moreover, BC2F2:3-7-16 (Xca1boXca1boPpa3Ppa3) and BC2F2:3-7-33 (Xca1boXca1boPpa3Ppa3) displayed very compact, white, good quality curds similar to those of the recurrent parent Pusa Meghna. It is, therefore, evident from this study that marker-assisted breeding can be successfully performed for introgressing new genes into popular cauliflower variety. The improved pyramided lines developed in this study can be further evaluated in multi-location trials under All India Coordinated Research Project on Vegetable Crops (AICRP) for release as an improved variety to be grown by Indian farmers for increasing their income and providing chemical free fresh cauliflower curds to consumers. Further, these pyramided lines can be used in their own right as a potential variety(s) and/or donor to breed multiple disease-resistant cauliflower variety(s) and hybrids.
Data Availability Statement
The datasets generated for this study can be found in online repositories. The names of the repository/repositories and accession number(s) can be found in the article.
Author Contributions
PS and PK designed the experiments. PS, CG, NDS, and MS performed the experiments. PS and CG developed and phenotyped the population. CG genotyped the population. CG, NDS, and AV analyzed the data. PS and AV wrote the manuscript. PK edited and improved the manuscript. BST assisted in field facilities and edited the manuscript. All authors contributed to the article and approved the submitted version.
Funding
The authors are thankful to SERB, DST, New Delhi, India for the financial support (SB/YS/LS-163/2014).
Conflict of Interest
The authors declare that the research was conducted in the absence of any commercial or financial relationships that could be construed as a potential conflict of interest.
Publisher's Note
All claims expressed in this article are solely those of the authors and do not necessarily represent those of their affiliated organizations, or those of the publisher, the editors and the reviewers. Any product that may be evaluated in this article, or claim that may be made by its manufacturer, is not guaranteed or endorsed by the publisher.
Acknowledgments
The authors are thankful to Head, Division of Vegetable Science for Providing Field, Laboratory Facilities and Manpower Support, and Dr. Dinesh Singh, Principal Scientist, Division of Plant Pathology for providing the bacterial culture.
Supplementary Material
The Supplementary Material for this article can be found online at: https://www.frontiersin.org/articles/10.3389/fpls.2021.603600/full#supplementary-material
References
Agnola, B., Silue, D., and Boury, S. (2000). “Identification of RAPD markers of downy mildew (Peronospora parasitica) resistance gene in broccoli (Brassica oleracea var. italica),” in Abstr. 3rd Int. Symp. Brassicas, ed G. J. King (Wellesbourne: Horticulture Research International).
Arunakumari, K., Durgarani, C. V., Satturu, V., Sarikonda, K. R., Chittoor, P. D. R., and Vutukuri, B. (2016). Marker-assisted pyramiding of genes conferring resistance against bacterial blight and blast diseases into Indian rice variety MTU1010. Rice Sci. 23, 306–316. doi: 10.1016/j.rsci.2016.04.005
Balachiranjeevi, C. H., Bhaskar, N. S., Abhilash, V., Akanksha, S., Viraktamath, B. C., and Madhav, M. S. (2015). Marker assisted introgression of bacterial blight and blast resistance into DRR17B, an elite, fine-grain type maintainer line of rice. Mol. Breed. 35:15. doi: 10.1007/s11032-015-0348-8
Bernabé, L. I., Madloo, L., Rodríguez, V. M., Francisco, M., and Soengas, P. (2019). Dissecting quantitative resistance to Xanthomonas campestris pv. campestris in leaves of Brassica oleracea by QTL analysis. Sci. Rep. 9:2015. doi: 10.1038/s41598-019-38527-5
Butler, E. J. (1918). Fungi and Diseases in Plants. Pp. vi. + 547. (Calcutta; Simla: Thacker, Spink, and Co.).
Camargo, L. E. A., Williams, P. H., and Osborn, T. C. (1995). Mapping of quantitative trait loci controlling resistance of Brassica oleracea to Xanthomonas campestris pv. campestris in the field and greenhouse. Phytopathology 85, 1296–1300. doi: 10.1094/Phyto-85-1296
Carlier, J. D., Alabac, C. A., Coelho, P. S., Monteiro, A. A., and Leitão, J. M. (2012). The downy mildew resistance locus Pp523 is located on chromosome C8 of Brassica oleracea L. Plant Breed. 131, 170–175. doi: 10.1111/j.1439-0523.2011.01904.x
Carvalho, T., and Monteiro, A. A. (1996). Preliminary study on the inheritance of resistance to downy mildew (Peronospora parasitica) at cotyledon stage in Tronchuda cabbage ‘Algarvia'. Cruciferae Newsl. 18, 104–105.
Chukwu, S. C., Rafii, M. Y., Ramlee, S. I., Ismail, S. I., Oladosu, Y., Kolapo, K., et al. (2020). Marker-assisted introgression of multiple resistance genes confers broad spectrum resistance against bacterial leaf blight and blast diseases in Putra-1 rice variety. Agronomy 10:42. doi: 10.3390/agronomy10010042
Coelho, P. S., and Monteiro, A. (2003). Inheritance of downy mildew resistance in mature broccoli plants. Euphytica 131, 65–69. doi: 10.1023/A:1023008619400
Das, G., and Rao, G. J. N. (2015). Molecular marker assisted gene stacking for biotic and abiotic stress resistance genes in an elite rice cultivar. Front. Plant Sci. 6:698. doi: 10.3389/fpls.2015.00698
Doullah, M., Mohsin, G. M., Ishikawa, K., Hori, H., and Okazaki, K. (2011). Construction of a Linkage Map and QTL analysis for black rot Resistance in Brassica oleracea L. Int. Nat. J. Sci. 11, 1–6. doi: 10.3329/ijns.v1i1.8591
Doyle, J. J., and Doyle, J. L. (1990). A rapid total DNA preparation procedure for fresh plant tissue. Biology 12, 13–15.
Evanno, G., Regnaut, S., and Goudet, J. (2005). Detecting the number of clusters of individuals using the software structure: a simulation study. Molecular Ecology 14, 2611–2620 doi: 10.1111/j.1365-294X.2005.02553.x
FAO (2014). Food and Agriculture Organization. Available online at: http://www.fao.org/statistics/en/
Farinhó, M., Coelho, P., Carlier, J., Svetleva, D., Monteiro, A., and Leitão, J. (2004). Mapping of a locus for adult plant resistance to downy mildew in broccoli (Brassica oleracea convar. italica). Theor. Appl. Genet. 109, 1392–1398. doi: 10.1007/s00122-004-1747-0
Farinhó, M., Coelho, P., Monteiro, A., and Leitão, J. (2007). SCAR and CAPS markers flanking the Brassica oleracea L. Pp523 downy mildew resistance locus demarcate a genomic region syntenic to the top arm end of Arabidopsis thaliana L. chromosome 1. Euphytica 157, 215−221. doi: 10.1007/s10681-007-9414-6
Farinhó, M. J., Coelho, P. S., Monteiro, A. A., and Leitão, J. M. (2000). “RAPD and AFLP markers linked to Peronospora parasitica resistance genes in broccoli,” in Proccedings. 3rd Intl. Symposium. Brassicas (Wellesbourne), 75.
Farnham, M. W., Keinath, A. P., and Smith, J. P. (2001). Characterization of Fusarium yellows resistance in collard. Plant Dis. 85, 890–894. doi: 10.1094/PDIS.2001.85.8.890
Gao, M., Li, G., Yang, B., Farnham, M., and Quiros, C. (2003). “Search for candidate genes for downy mildew resistance in broccoli,” in Abstracts of the Plant and Animal Genomes XI Conference. 476.
Giovannelli, J. L., Farnham, M. W., and Wang, M. (2002). Development of sequence characterized amplified region markers linked to downy mildew resistance in broccoli. J. Am. Soc. Hortic. Sci. 127, 597–601. doi: 10.21273/JASHS.127.4.597
Gopalakrishnan, S., Sharma, R. K., Rajkumar, K. A., Joseph, M., Singh, V. P., Singh, A. K., et al. (2008). Integrating marker assisted background analysis with foreground selection for identification of superior bacterial blight resistant recombinants in Basmati rice. Plant Breed. 127, 131–139. doi: 10.1111/j.1439-0523.2007.01458.x
Gu, J. F., Qiu, M., and Yang, J. C. (2013). Enhanced tolerance to drought in transgenic rice plants overexpressing C4 photosynthesis enzymes. Crop J. 1, 105–114. doi: 10.1016/j.cj.2013.10.002
Hari, Y., Srinivasarao, K., Viraktamath, B. C., Hariprasad, A. S., Laha, G. S., and Ilyas, A. (2011). Marker-assisted improvement of a stable restorer line, KMR-3R and its derived hybrid KRH2 for bacterial blight resistance and grain quality. J. Plant Breed. 30, 608−616. doi: 10.1111/j.1439-0523.2011.01881.x
Hirabayashi, H., Sato, H., Nonoue, Y., Kuno-Takemoto, Y., Takeuchi, Y., Kato, H., et al. (2010). Development of introgression lines derived from Oryza rufipogon and O. glumaepatula in the genetic background of japonica cultivated rice (O. sativa L.) and evaluation of resistance to rice blast. Breed. Sci. 60, 604–612. doi: 10.1270/jsbbs.60.604
Hoser Krauze, J., Łakowska Ryk, E., and Antosik, J. (1995). Inheritance of resistance of some Brassica oleracea L. cultivars and lines to downy mildew—Peronospora parasitica (Pers.) ex. Fr. J. Appl. Genet. 36, 27–33.
Hsu, Y. C., Chiu, C. H., Yap, R., Tseng, Y. C., and Wu, Y. P. (2020). Pyramiding bacterial blight resistance genes in tainung82 for broad-spectrum resistance using marker-assisted selection. Int. J. Mol. Sci. 21:1281. doi: 10.3390/ijms21041281
Jagota, S. K., and Dani, H. M. (1982). A new colorimetric technique for the estimation of vitamin C using Folin phenol reagent. Ana. Biochem. 127, 178–182. doi: 10.1016/0003-2697(82)90162-2
Jamwal, R. S., and Sharma, P. P. (1986). Inheritance of resistance to black rot (Xanthomonas campestris pv. campestris) in cauliflower (Brassica oleracea var. botrytis). Euphytica 35, 941–943. doi: 10.1007/BF00028603
Jensen, B. D., Hockenhull, J., and Munk, L. (1999). Seedling and adult plant resistance to downy mildew (Peronospora parasitica) in cauliflower (Brassica oleracea convar botrytis var botrytis). Plant Pathol. 48, 604–612. doi: 10.1046/j.1365-3059.1999.00388.x
Joseph, M., Gopalakrishnan, S., Sharma, R. K., Singh, V. P., Singh, A. K., Singh, N. K., et al. (2004). Combining bacterial blight resistance and basmati quality characteristics by phenotypic and molecular marker-assisted selection in rice. Mol. Breed. 13, 377–387. doi: 10.1023/B:MOLB.0000034093.63593.4c
Kalia, P., Saha, P., Sharma, M., and Singh, D. (2017). Development of RAPD and ISSR derived SCAR markers linked to Xca1bo gene conferring resistance to black rot disease in cauliflower (Brassica oleracea var. botrytis L.). Euphytica 213:232. doi: 10.1007/s10681-017-2025-y
Kapoor, K. S., Gill, H. S., and Sharma, S. R. (1985). A technique for artificial inoculation of cauliflower seedlings with Sclerotinia sclerotiarum (Lib.) de Bery. J. Phytopathol. 112, 191–192. doi: 10.1111/j.1439-0434.1985.tb04827.x
Kaur, R., Saxena, B., Kanwar, H. S., Dohroo, N. P., Majeed, S., and Sharma, D. R. (2009). Detecting RAPD markers associated with black rot resistance in cabbage (Brassica oleracea var. capitata). Fruit Veg. Cereal Sci. Biotechnol. 3, 12–15.
Kifuji, Y., Hanzaea, H., Terasawa, Y., and Nishio, T. (2013). QTL analysis of black rot resistance in cabbage using newly developed EST-SNP markers. Euphytica 190, 289–295. doi: 10.1007/s10681-012-0847-1
Kumar, A., Jindal, S. K., Dhaliwal, M. S., Sharma, A., Kaur, S., and Jain, S. (2019). Gene pyramiding for elite tomato genotypes against ToLCV (Begomovirus spp.), late blight (Phytophthora infestans) and RKN (Meloidogyne spp.) for northern India farmers. Physiol. Mol. Biol. Plants 25, 1197–1209. doi: 10.1007/s12298-019-00700-5
Kumar, A., Tiwari, K. L., Datta, D., and Singh, M. (2014). Marker assisted gene pyramiding for enhanced Tomato leaf curl virus disease resistance in tomato cultivars. Biol. Plantarum. 58, 792–797. doi: 10.1007/s10535-014-0449-y
Laemmlen, F. F., and Mayberry, K. S. (1984). Broccoli resistance to downy mildew. Calif. Agric. Nov Dec 17131, 65–69.
Lee, J., Izzah, N. K., and Jayakodi, M. (2015). Genome-wide SNP identification and QTL mapping for black rot resistance in cabbage. BMC Plant Biol. 15:32. doi: 10.1186/s12870-015-0424-6
Lee, Y. G., Jeong, N., Kim, J. H., and Lee, L. (2015). Development, validation and genetic analysis of a large soybean SNP genotyping array. Plant J. 81, 625–636. doi: 10.1111/tpj.12755
Li, H., Chen, X., Yang, Y., Xu, J., Gu, J., and Fu, J. (2011). Development and genetic mapping of microsatellite markers from whole genome shotgun sequences in Brassica oleracea. Mol. Breed. 28, 585–596. doi: 10.1007/s11032-010-9509-y
Lowe, A. J., Moule, C, Trick, M, and Edwards, K. J. (2004). Efficient large-scale development of microsatellites for marker and mapping applications in Brassica crop species. Theor. Appl. Genet. 108, 1103–1112. doi: 10.1007/s00122-003-1522-7
Lund, B. M., and Wyatt, G. M. (1978). Post harvest spoilage of cauliflower by downy mildew, Peronospora parasitica. Plant Pathol. 27, 143−144. doi: 10.1111/j.1365-3059.1978.tb01100.x
Mahajan, V., Gill, H. S., and More, T. A. (1995). Inheritance of downy mildew resistance in Indian cauliflower (group III). Euphytica 86, 1–3. doi: 10.1007/BF00035932
McKay, A. G., Floyd, R. M., and Boyd, C. J. (1992). Phosphonic acid controls downy mildew (Peronospora parasitica) in cauliflower curds. Aust. J. Exp. Agric. 32, 127–129. doi: 10.1071/EA9920127
Miah, G., Rafii, M. Y., Ismail, M. R., Puteh, A. B., Rahim, H. A., and Latif, M. A. (2017). Marker-assisted introgression of broad-spectrum blast resistance genes into the cultivated MR219 rice variety. J. Sci. Food Agric. 97, 2810–2818. doi: 10.1002/jsfa.8109
Nguyen, H. T., Vu, Q. H., Mai, T. V., Nguyen, T. T., Vu, L. D., and Nguyen, T. T. (2018). Marker-assisted selection of Xa21 conferring resistance to bacterial leaf blight in Indica rice cultivar LT2. Rice Sci. 25, 52–56. doi: 10.1016/j.rsci.2017.08.004
Özkaynak, E., Devran, Z., Kahveci, E., Doganlar, S., Baskoylu, B., Dogan, F., et al. (2014). Pyramiding multiple genes for resistance to PVY, TSWV and PMMoV in pepper using molecular markers. Eur. J. Hortic. Sci. 79, 233–239.
Pandey, K. K., Pandey, P. K., Singh, B., Kalloo, G., and Kapoor, K. S. (2001). Sources of resistance to downy mildew (Peronospora parasitica) disease in the Asiatic group of cauliflower. Veg. Sci. 28, 55–57.
Panse, V. G., and Sukhatme, P. V. (1967). Statistical Methods for Agricultural Workers. New Delhi: ICAR Publication, New Delhi.
Patroti, P., Vishalakshi, B., Umakanth, B., Suresh, J., Senguttuvel, P., and Madhav, M. S. (2019). Marker-assisted pyramiding of major blast resistance genes in Swarna-Sub1, an elite rice variety (Oryza sativa L.). Euphytica 215:179. doi: 10.1007/s10681-019-2487-1
PPV and FRA (2009). Guidelines for the Conduct of Test for Distinctness, Uniformity, and Stability on Cauliflower (Brassica oleracea var. botrytis L.). Govt. of India, New Delhi. SG.36.2009.
Prasanna, H. C., Sinha, D. P., Rai, G. K., Krishna, R., Kashyap, S. P., Singh, N. K., et al. (2014). Pyramiding Ty-2 and Ty-3 genes for resistance to monopartite and bipartite tomato leaf curl viruses of India. Plant Patholol. 64, 256–264. doi: 10.1111/ppa.12267
Pritchard, J. K., Stephens, M., and Donnelly, P. (2000). Inference of population structure using multilocus genotype data. Genetics. 155, 945–959. doi: 10.1093/genetics/155.2.945
Saha, P., Ghoshal, C., Ray, S., Saha, N. D., Srivastava, M., Kalia, P., et al. (2020). Genetic analysis of downy mildew resistance and identification of molecular markers linked to resistance gene Ppa207 on chromosome 2 in cauliflower. Euphytica 216:183. doi: 10.1007/s10681-020-02696-6
Saha, P., Kalia, P., Sharma, M., and Singh, D. (2016). New source of black rot disease resistance in Brassica oleracea and genetic analysis of resistance. Euphytica 207, 35–48. doi: 10.1007/s10681-015-1524-y
Saha, P., Kalia, P., Sonah, H., and Sharma, T. R. (2014). Molecular mapping of black rot resistance locus Xca1bo on chromosome 3 in Indian cauliflower (Brassica oleracea var. botrytis L.). Plant Breed. 133, 268–274. doi: 10.1111/pbr.12152
Sharma, B. B., Kalia, P., Yadava, D. K., Singh, D., and Sharma, T. R. (2016). Genetics and molecular mapping of black rot resistance locus Xca1bc on chromosome B-7 in Ethiopian mustard (Brassica carinata A. Braun). PLoS ONE 11:e0152290. doi: 10.1371/journal.pone.0152290
Sheng, X. G., Branca, F., Zhao, Z. Q., Wang, J. S., Yu, H. F., Shen, Y. S., et al. (2020). Identification of black rot resistance in a wild Brassica species and its potential transferability to cauliflower. Agronomy 10:1400. doi: 10.3390/agronomy10091400
Shu, Q. Y. (2009). “Induced plant mutations in the genomics era,”. in Proceedings of an International Joint FAO/IAEA Symposium. (Vienna: FAO).
Sidki, S. (1962). Morphology of the curd of cauliflower. Amer. Jour. Bot. 49, 290–297. doi: 10.1002/j.1537-2197.1962.tb14940.x
Singh, D., Rathaur, P. S., and Vicente, J. G. (2016). Characterization, genetic diversity and distribution of Xanthomonas campestris pv. campestris races causing black rot disease in cruciferous crops of India. Plant Pathology Pathol. 65, 1411–1418. doi: 10.1111/ppa.12508
Singh, S., Sharma, S. R., Kalia, P., Deshmukh, R., Katara, J., Sharma, P., et al. (2015). Identification of putative DNA markers for disease resistance breeding in Indian cauliflower (Brassica oleracea var. botrytis L.). Indian J. Biotechnol. 14, 455–460.
Singh, S., Sharma, S. R., Kalia, P., Deshmukh, R., Kumar, V., Sharma, P., et al. (2012). Molecular mapping of the downy mildew resistance gene Ppa3 in cauliflower (Brassica oleracea var. botrytis L.). J. Hortic. Sci. Biotech. 87, 137–143. doi: 10.1080/14620316.2012.11512844
Singh, S., Sharma, S. R., Kalia, P., Sharma, P., Kumar, V., Kumar, R., et al. (2013). Screening of cauliflower (Brassica oleracea L. var. botrytis L.) germplasm for resistance to downy mildew [Hyaloperonospora parasitica Constant (Pers.:Fr) Fr.] and designing appropriate multiple resistance breeding strategies. J. Hortic. Sci. Biotech. 88, 103–109. doi: 10.1080/14620316.2013.11512942
Singh, M., Chaudhuri, I., Mandal, S. K., and Chaudhuri, R. K. (2011). Development of RAPD markers linked to fusarium wilt resistance gene in castor bean (Ricinus communis L.). Genet. Eng. Biotech. J. 28, 1–9.
Soengas, P., Hand, P., Vicente, J. G., Pole, J. M., and Pink, D. A. C. (2007). Identification of quantitative trait loci for resistance to Xanthomonas campestris pv. campestris in Brassica rapa. Theor. Appl. Genet. 114, 637–645. doi: 10.1007/s00122-006-0464-2
Sundaram, R. M., Chatterjee, S., Oliva, R., Laha, G. S., Leach, J. E., and Cruz, C. V. (2014). Update on bacterial blight of rice. fourth international conference on bacterial blight. Rice 7:12. doi: 10.1186/s12284-014-0012-7
Sundaram, R. M., Vishnupriya, M. R., Biradar, S. K., Laha, G. S., Reddy, G. A., Rani, N. S., et al. (2008). Marker assisted introgression of bacterial blight resistance in Samba Mahsuri, an elite indica rice variety. Euphytica 160, 411–422. doi: 10.1007/s10681-007-9564-6
Swarup, V., and Chatterjee, S. S. (1972). Origin and genetic improvement of Indian cauliflower. Eco. Bot. 26, 381–393. doi: 10.1007/BF02860710
Tanweer, F. A., Rafii, M. Y., Sijam, K., Rahim, H. A., Ahmed, F., and Ashkani, S. (2015). Identification of suitable segregating SSR markers for blast resistance in rice using inheritance and disease reaction analysis in backcross families. Australas. Plant Pathol. 44, 619–627. doi: 10.1007/s13313-015-0380-5
Tewari, J. P., Conn, K. L., and Dahiya, J. S. (1987). “Resistance to Alternaria brassicae in Crucifers,” in Procceding 7th Int. Rapeseed Congr (Poznań), 1085–1090.
Thakur, R. P., and Mathur, K. (2002). Downy mildews of India. Crop Prot. 21, 333–345. doi: 10.1016/S0261-2194(01)00097-7
Thamburaj, S., and Singh, N. (2003). “Vegetables, tubercrops and spices,” in Directorate of Information and Publications of Agriculture, (New Delhi: Indian Council of Agricultural Research), 76–97.
Tian, F., Li, D. J., Fu, Q., Zhu, Z. F., Fu, Y. C., Wang, X. K., et al. (2006). Construction of introgression lines carrying wild rice (Oryza rufipogon Griff.) segments in cultivated rice (Oryza sativa L.) background and characterization of introgressed segments associated with yield-related traits. Theor. Appl. Genet. 112, 570–580. doi: 10.1007/s00122-005-0165-2
Tonu, N. N., Doullah, M. A., and Shimizu, M. (2013). Comparison of positions of QTLs conferring resistance to Xanthomonas campestris pv. camperstris in Brassica oleracea. Am. J. Plant Sci. 4, 11–20. doi: 10.4236/ajps.2013.48A002
van Berloo, R. (2008). GGT 2.0: versatile software for visualization and analysis of genetic data. J. Hered. 99, 232–236. doi: 10.1093/jhered/esm109
Vanlalneihi, B., Saha, P., Kalia, P., Jaiswal, S., Kundu, A., Saha, N. D., et al. (2020). Chemometric approach based characterization and selection of mid-early cauliflower for bioactive compounds and antioxidant activity. J. Food Sci. Tech. 57, 293–300. doi: 10.1007/s13197-019-04060-6
Varalakshmi, B., Ganeshan, G., Pushpalatha, A., and Chethana, B. S. (2011). Inheritance of downy mildew resistance in cauliflower (Group I). Veg. Sci. 38, 115–116.
Verma, A., and Singh, Y. (2018). Inheritance of downy mildew resistance and its relationship with biochemical traits in cauliflower (Brassica oleracea L. var. botrytis). Crop Prot. 106, 132–138. doi: 10.1016/j.cropro.2017.12.024
Verma, T. S., and Thakur, P. C. (1989). Comparative field resistance of cabbage collection to downy mildew at seedling stage. Indian J. Plant Prot. 17, 79–80.
Vicente, J. G., Conway, J., Roberts, S. J., and Taylor, J. D. (2001). Identification and origin of Xanthomonas campestris pv. campestris races and related pathovars. Phytopathology 91, 492–499. doi: 10.1094/PHYTO.2001.91.5.492
Vicente, J. G., and Holub, E. B. (2013). Xanthomonas campestris pv. campestris (cause of black rot of crucifers) in the genomic era is still a worldwide threat to brassica crops. Mol. Plant Pathol. 14, 2–18. doi: 10.1111/j.1364-3703.2012.00833.x
Williams, J. G. K., Kubelik, A. R., Livak, J. K., Rafalski, J. A., and Tingey, S. V. (1990). DNA polymorphisms amplified by arbitrary markers are useful as genetic markers. Nucleic Acids Res. 18, 6531–6535. doi: 10.1093/nar/18.22.6531
Keywords: cauliflower, black rot, downy mildew, MAS, resistance
Citation: Saha P, Ghoshal C, Saha ND, Verma A, Srivastava M, Kalia P and Tomar BS (2021) Marker-Assisted Pyramiding of Downy Mildew-Resistant Gene Ppa3 and Black Rot-Resistant Gene Xca1bo in Popular Early Cauliflower Variety Pusa Meghna. Front. Plant Sci. 12:603600. doi: 10.3389/fpls.2021.603600
Received: 07 September 2020; Accepted: 28 June 2021;
Published: 23 August 2021.
Edited by:
Harsh Raman, New South Wales Department of Primary Industries, AustraliaReviewed by:
Joana G. Vicente, Fera Science Ltd., United KingdomMaoteng Li, Huazhong University of Science and Technology, China
Copyright © 2021 Saha, Ghoshal, Saha, Verma, Srivastava, Kalia and Tomar. This is an open-access article distributed under the terms of the Creative Commons Attribution License (CC BY). The use, distribution or reproduction in other forums is permitted, provided the original author(s) and the copyright owner(s) are credited and that the original publication in this journal is cited, in accordance with accepted academic practice. No use, distribution or reproduction is permitted which does not comply with these terms.
*Correspondence: Partha Saha, aG9ydHBhcnRoJiN4MDAwNDA7Z21haWwuY29t
†Present address: Mohita Srivastava, Department of Horticulture, Lovely Professional University, Phagwara, India