- 1College of Life Sciences, Qufu Normal University, Qufu, China
- 2College of Life and Environmental Sciences, University of Exeter, Exeter, United Kingdom
Although various seed-marking methods have been developed for seed dispersal, it remains difficult to track the actual patterns of seed dispersal and seedling recruitment. Thus, new labeling methods that accurately track seedling establishment along with seed movement would help us better understand seed dispersal. Here, we developed a new nondestructive method using 15N xylem injection to track seed dispersal and seedling recruitment based on the enriched isotopic signals in the mature seeds. Our results first showed that xylem injection of 15N successfully enriched 15N both in the acorns and seedlings of Quercus variabilis. By marking acorns and seedlings with 15N stable isotopes, we successfully tracked seedlings established from acorns dispersed by seed-eating animals in the field. Our xylem 15N injection caused little alteration to seeds and showed no significant effects on seed selection by seed-eating animals as well as seed germination and seedling establishment, verifying the validity of the 15N xylem injection method to track seedling establishment. Our xylem 15N injection method is expected to be a powerful tool for tracking seed dispersal and seedling recruitment mediated by seed-eating animals in seed dispersal ecology.
Introduction
Understanding the factors affecting the composition and abundance of tree regeneration is critical to the natural succession of various forest ecosystems (Lichti et al., 2014; Montoro Girona et al., 2018). Natural regeneration of natural forests is mainly dependent on plant–animal interactions (Steele et al., 2018), in which seed dispersal mediated by seed-eating animals is vital in successional patterns of structure and composition of forests (Yang et al., 2020).
Seed dispersal, seed movement away from mother trees, shows prominent effects on the genetic structures, population dynamics, and community ecology of all ecosystems (Levine and Murrell, 2003; Yi et al., 2011). Seed dispersers play a critical role in dispersing seeds of many plant species in various ecosystems (Moore et al., 2007; Yi et al., 2011). However, to accurately estimate success of dispersed seedlings remains a challenging field research problem, because it is often difficult to determine seed movements mediated by seed-eating animals (Davis et al., 2011; Muñoz and Bonal, 2011; Hirsch et al., 2012a,b). These difficulties are largely attributed to methodological limitations to identify where the seeds germinate after seed dispersal (Galetti et al., 2010; Yi and Wang, 2015), which hampers better understanding of the ecological and evolutionary consequence of seed dispersal. Therefore, a better assessment of seed dispersal impacts on community ecology and population dynamics is highly dependent on developing new approaches to reveal where the seeds are deposited by seed-eating animals and whether they successfully escape predation and establish in the suitable conditions (Hirsch et al., 2012b; Yi et al., 2014).
During the past several decades, several methods have been developed for tracking seed dispersal. Inserting metal or magnets into large seeds and detecting with metal detectors appear to be a pioneering attempt (Alverson and Díaz, 1989; Den Ouden et al., 2005; Moore et al., 2007; Lichti et al., 2014). Later, some researchers attempted to dye seeds with fluorescent microspheres or powders for tracking (Lemke et al., 2010; Wróbel and Zwolak, 2013). Labeling seeds with threads or tags has been widely accepted and used in various studies (Yi and Zhang, 2008; Deng et al., 2020; Yang et al., 2020). In recent years, passive integrated transponders (PITs) and radio transmitters were used in seed dispersal ecology despite their high technical requirements (Steele et al., 2011; Hirsch et al., 2012b; Suselbeek et al., 2013). Most recently, Gu et al. (2017) and White et al. (2017) investigated the relationships between rodents and seeds at the individual level combining infrared radiation camera tracking and seed tagging. Although these methods have been used in many ecosystems for tracking seed dispersal, the main shortcoming is the influence of seed alteration on either seed choice by animals or the following seedling establishment (Levey and Sargent, 2000; Godoy and Jordano, 2001; Tewksbury et al., 2002; Hirsch et al., 2012a,b; Yi and Wang, 2015; Niu et al., 2020). Xiao and Zhang (2003) have well compared a majority of tracking methods, pointing out their advantages and limitations and potential implications in seed dispersal ecology.
Stable isotope labeling has been regarded as a powerful quantitative technique widely used in ecological research (Schmidt and Scrimgeour, 2001; Putz et al., 2011). Carlo and Norris (2012) first showed that petal surfaces can effectively capture and quickly allocate nitrogen ions into ovaries and developing seeds. Carlo et al. (2009) for the first time tracked seed distribution of small herbaceous plants (Solanum americanum and Capsicum annuum) based on labeling seeds or fruits by foliar-spraying with a solution of 15N-urea during the flowering stage. Later, Castellano and Gorchov (2013) and Forster and Herrmann (2014) verified the feasibility of foliar application of 15N-labeled urea to enrich 15N in seeds and seedlings of shrubby Lonicera maackii and herbaceous Eupatorium glaucescens and Sericocarpus tortifolius. Although these methods cause little alteration to seeds, they are highly limited to plants small enough to adequately and uniformly be covered with the urea-15N spray. Therefore, it is not broadly applicable for woody plants with high trunks and large canopies. Recently, Yi et al. (2014) and Yi and Wang (2015) further developed the stable isotope technique to track seed dispersal of tree species by soaking seeds with 15N-urea solutions. This approach is highly efficient for tracking seedling establishment in the field based on the 15N isotopic clues in seeds and seedlings. However, directly soaking seeds with 15N isotopic solution may alter seed palatability and odor and then render feeding discrimination of seed-eating animals. Therefore, developing a simple and reliable seed-tracking method is highly needed to facilitate better understanding of seed dispersal ecology (Bologna et al., 2017).
It has been evidenced that biomass enriched in 15N can be produced by direct injections of 15N-labeled fertilizers into the plant vascular system (Swanston and Myrold, 1998; Proe et al., 2000; Nair et al., 2014). Horwath et al. (1992) first utilized an injection technique to apply 15N-enriched compounds into plants via a purpose-drilled hole reaching the cambium and xylems where the solution was taken up via a Venturi effect (Nair et al., 2014). This procedure consists of a reservoir of injection substrate introduced into the tree trunks either passively (Proe et al., 2000) or under pressure (Horwath et al., 1992; Swanston and Myrold, 1998). Although this method has been used to trace the fate of injected elements, to our knowledge, it has never been used with the primary purpose of creating 15N-labeled propagules to track seed dispersal and seedling establishment. Here, we developed a novel approach to overcome previous methodological limitations by tracking seed dispersal and seedling recruitment relying on 15N enrichment in seeds via xylem injections. In this study, 15NH4Cl and K15NO3 were used for the injections because both of their constituent ions are transported in the xylem stream (Marschner and Marschner, 2012). We expected that the 15NH4Cl and K15NO3 will be transported along the xylems of Chinese cork oak Quercus variabilis and finally incorporated into their acorns, generating 15N-enriched seeds. Our aim was to develop a new method to track seed dispersal using isotopic clues injected via xylems to seeds. We specifically addressed the following questions: (1) Can xylem injection of 15NH4Cl and K15NO3 produce 15N-enriched seeds and seedlings? (2) Given that the previous tracking methods usually show effects on seed handling by animals, we would like to answer whether xylem injection of 15NH4Cl and K15NO3 influences seed choice of seed-eating animals. (3) Existing literature has shown that tracking methods cause negative impacts on or even mortality to seeds and seedlings, and we also wanted to know if xylem injection of 15NH4Cl and K15NO3 influences seed germination and seedling establishment. (4) Can xylem injection of 15NH4Cl and K15NO3 be a reliable new approach to track seedling recruitment in the field?
Materials and Methods
Injection of 15N Isotope Into the Xylems
In early July 2018 when the tiny fruits were setting, fruiting individuals of Q. variabilis were selected for 15N injection in a primary forest stand in Sichuan provinces in southwest China (Supplementary Figure 1, also see detailed information in Yang et al., 2020). We selected Q. variabilis trees with DBH (diameter at breast height) ranging from 30 to 40 cm > 100 m apart each other scattered in a sub-tropical forest for xylem 15N injection. Considering the limited area of the primary forest, we selected seven focal trees to avoid potential interference between their seedlings originated in the next year. The passive injection system was used to inject 60 mmol/L 15NH4Cl and K15NO3 (enriched to 10 atom% 15N, dissolved in 1,500 ml of distilled water) into the xylem with the aid of a 1,500-ml plastic infusion bag (Guoguang, Sichuan, China), which was inversely mounted on the trunk 2 m from the ground (Proe et al., 2000; Supplementary Figure 2). First, we removed an area (less than 15 cm2) of bark on the two opposite sides of the stem at the breast height. Then, two small opposite holes measuring 4 mm in diameter and 4 cm in depth were drilled using an electric hand drill with an auger bit (4 mm in diameter). Once drilled, two plastic tubes of 4 mm diameter were led through the bag top into the two holes on each tree stem. Immediately, we used a commercially available waterproof silicone sealant to coat the sides of holes to avoid potential cavitation (see Nair et al., 2014). The concentration of 60 mmol/L was intentionally selected because our previous attempts indicate that 40 mmol/L is not high enough to obtain 15N enriched seeds comparable to natural ones (Supplementary Figure 3). We checked every day to ensure all trees to successfully uptake 15N solution without obvious evidence of leaks. The solutions were taken up by all trees within 10 days. Once the solution was completely taken up by a given tree, the holes were refilled with silicon sealant.
Measurement of 15N Isotopes in Acorns
In late September 2018 when fruits of Q. variabilis were ripened, we collected acorns under the canopy of each tree. To see if injection of 15NH4Cl and K15NO3 through xylem resulted in 15N enrichment in acorns, 30 acorns were randomly selected from each tree. For comparison, acorns from three to five control trees without 15N injection were collected around each focal tree in the same way for isotope measurement.
Measurement of Nutrition in Acorns
Acorns were air-dried to constant weight in an oven at 70°C for 48 h to test if 15N injection significantly changed nutrition level of acorns. Crude protein content was determined by Kjeldahl method with digestion of sulfuric acid–hydrogen peroxide (Wang et al., 2014). Soxhlet extraction was used for determination of crude fat content (Gu et al., 2009). Total starch was determined by acidolysis-DNS (Lu and Li, 2002). The chlorine content was determined using silver nitrate titration method (Maines and Bruch, 2012). All samples were sent to the Cavenix Test Technology Co., Ltd (Nanjing, China) for analyses.
Measurement of 15N Isotopes in Seedlings
To test if 15N injection showed any positive or negative effects on seed germination and seedling establishment, we randomly selected 50 15N-enriched acorns from 15N enriched Q. variabilis trees for germination experiments. For comparison, 50 acorns were selected from a composite sample of control Q. variabilis trees, respectively. Acorns were individually planted 1 cm deep into the plastic flower pots (diameter × height: 8 cm × 15 cm) containing fine sand free of nutrition. To compare germination rates, 50 pots were evenly assigned into 10 groups both for the control and 15N-enriched acorns. Plant containers were kept at room temperature under 600–800 μmol⋅m–2⋅s–1 radiation of fluorescent lamps, regularly watered, and randomly arranged in space. After 60 days’ cultivation, seed germination and seedling dry mass were measured for each tree species to see if xylem injection of 15N affects seed germination and seedling establishment. To see if xylem injection of 15NH4Cl and K15NO3 successfully produced 15N-enriched seedlings, one fully developed leaf, representing a mature leaf at the age of 60 days, was collected from each seedling. Previous study has shown that the newly assimilated N and remobilized N from reserves have rather different 15N signatures for a seedling (Yang et al., 2019; Cui et al., 2020). In addition, 15N will be diluted in old leaves (Yi and Wang, 2015); we therefore selected mature leaves to create sub-samples for both the 15N-enriched and control seedlings for isotope measurements, as N assimilation from soil and N remobilization from reserves are expected to reach a state of balance (Yi and Ju, 2020).
Tracking Seedling Recruitment Using Isotopic Signals in the Field
To investigate whether xylem injection of 15N was reliable for tracking seed dispersal kernel and seedling establishment, we conducted area-constrained searches defined by a 30-m radius to locate 1-year seedlings of Q. variabilis around the seven injected trees in the next spring. Locating seedlings involved a systematic, comprehensive search of eight radial belts (2 m wide, 30 m long) around each focal tree. Distance between each seedling to the nearest focal tree was measured. Then, like the indoor procedures, one fully developed leaf was sampled from each seedling for later identification by using 15N isotope analysis. We collected leaves from 811 seedlings for 15N isotope analysis.
Stable Isotope Analysis
All samples for isotope analyses were air-dried to constant weight in an oven at 70°C for 48 h. Then, they were ground finely and placed in an isotope ratio spectrometer for isotopic analysis using elemental analyzer/continuous flow isotope ratio mass spectrometry following the method of Yi et al. (2014). Samples were analyzed for stable nitrogen isotope abundance and nitrogen concentration at the Institute of Applied Ecology, Chinese Academy of Sciences (Shenyang, China). Interface between element-analysis meter and spectrometer was Flash EA1112 HT (Thermo Finnigan, United States). Operation condition: oxidizing furnace temperature was 900°C, reducing furnace was 680°C, and pillar temperature was 40°C. The resulting N2 was purified in a vacuum line and injected in a Finnigan MAT 235 (dual) spectrometer (Thermo Fisher Scientific, Inc., United States) fitted with double inlet and collector systems. The results are expressed in δ15N relative to the standards in the conventional δ per mil notation with a standard deviation of 0.2 per mil:
δ15N = [(15N/14N) sample/(15N/14N) standard − 1] × 1000
Where 15N/14N are the isotopic ratios of sample (seeds or leaves) and standard (atmospheric nitrogen).
Seed Selection and Pilferage by Seed-Caching Animals in Enclosures
Enrichment of 15N may change seed nutrition and seed odor, which may also influence seed handling (e.g., eating, caching and pilfering) by seed-eating animals. To test whether seed-eating animals selectively consumed and cached the control and 15N-enriched seeds, we provided 20 paired control and 15N enriched acorns of Q. variabilis to each individual of scatter-hoarding rodent Edward’s long-tailed rat (Leopoldamys edwardsi) caged in the separate enclosures (10 × 10 × 1.5 m) supplied with bedding materials, nests, and drinking water. Five females and five males were used to see if there was difference in acorn preference between genders. The ground of each enclosure was covered with bricks to create an 8 × 8 grid of 64 evenly spaced shallow pits (length × width × depth: 24 cm × 12 cm × 6 cm) each separated by 1 m. The shallow pits were filled with fine sand to allow seed burial by L. edwardsi. A seed station (0.5 m2) was established at the center of each enclosure for presentation of acorns. The 15N-enriched and control acorns of Q. variabilis were marked with different colors for easy identification. Ten hours after seed placement and animal introduction, we carefully searched the enclosures to record the seed fates: intact in situ (IIS), eaten in situ (EIS), eaten after removal (EAR), intact after removal (IAR), scatter-hoarded (SH), and LH (larder-hoarded). Seed fate of each category was compared to see if L. edwardsi showed preference for the control or 15N-enriched acorns of Q. variabilis.
To test if xylem injection of N isotope affects cache pilferage by scatter-hoarding animals, we established 32 artificial caches containing 16 paired control and 15N-enriched acorns in each enclosure and allowed individual animal to pilfer freely for 10 h. In total, 10 L. edwardsi were tested individually in the enclosures. In this study, we used L. edwardsi for testing because this species has been regarded as the predominant scatter-hoarding rodents in the study area (Xiao et al., 2008; Zhang et al., 2008), and scatter-hoarding rodents are better pilferers than larder-hoarders (Wang et al., 2018). The remaining rodent species (Apodemus draco, A. chevrieri, Niviventer confucianus, and N. fulvescens) are predominant larder-hoarders and may contribute little to seed dispersal and seedling establishment (Chang and Zhang, 2011). We did not conduct paired-seed selection experiments in the field because it was impossible to exclude the background effects of abundant natural acorns and probably the ecological role of an avian seed disperser Garrulus glandarius in the study area.
Data Analysis
We conducted all statistical analyses using SPSS 16.0. An independent t-test was used to test the effects of xylem 15N injection on δ15N values in acorns and seedlings of Q. variabilis. Differences in acorn nutrients (protein, fat, and starch) and minerals (Cl and N) between the control and 15N-enriched acorns were tested using independent t-tests. The same procedure was used to test if xylem injection of 15N affected seed germination and seedling performance. We used paired samples t-test to test if xylem injection of 15N affected seed selection and cache pilferage by seed-eating animals in the artificial enclosures. In this study, seedlings with δ15N values 10 order of magnitudes higher than those of the control seedlings were considered from the 15N-enriched seeds in the field (Yi and Wang, 2015).
Results
15N Enrichment in Seeds and Seedlings
Xylem injection using 15NH4Cl and K15NO3 caused significant 15N enrichment in seeds of Q. variabilis (t = −14.312, df = 9, P < 0.001) (Figure 1A). Correspondingly, 15N was observed significantly enriched in seedlings of Q. variabilis (t = −21.549, df = 4, P < 0.001) (Figure 1B). However, crude fat, starch, and chlorine in Q. variabilis acorns were not significantly affected by xylem 15N injection (t = 0.010, df = 4, P = 0.992; t = −0.961, df = 4, P = 0.391; t = −1.217, df = 4, P = 0.290), while the content of crude protein and N were slightly increased by xylem 15N injection (t = −2.803, df = 4, P = 0.049; t = −2.332, df = 9, P = 0.045) (Figures 2A,B).
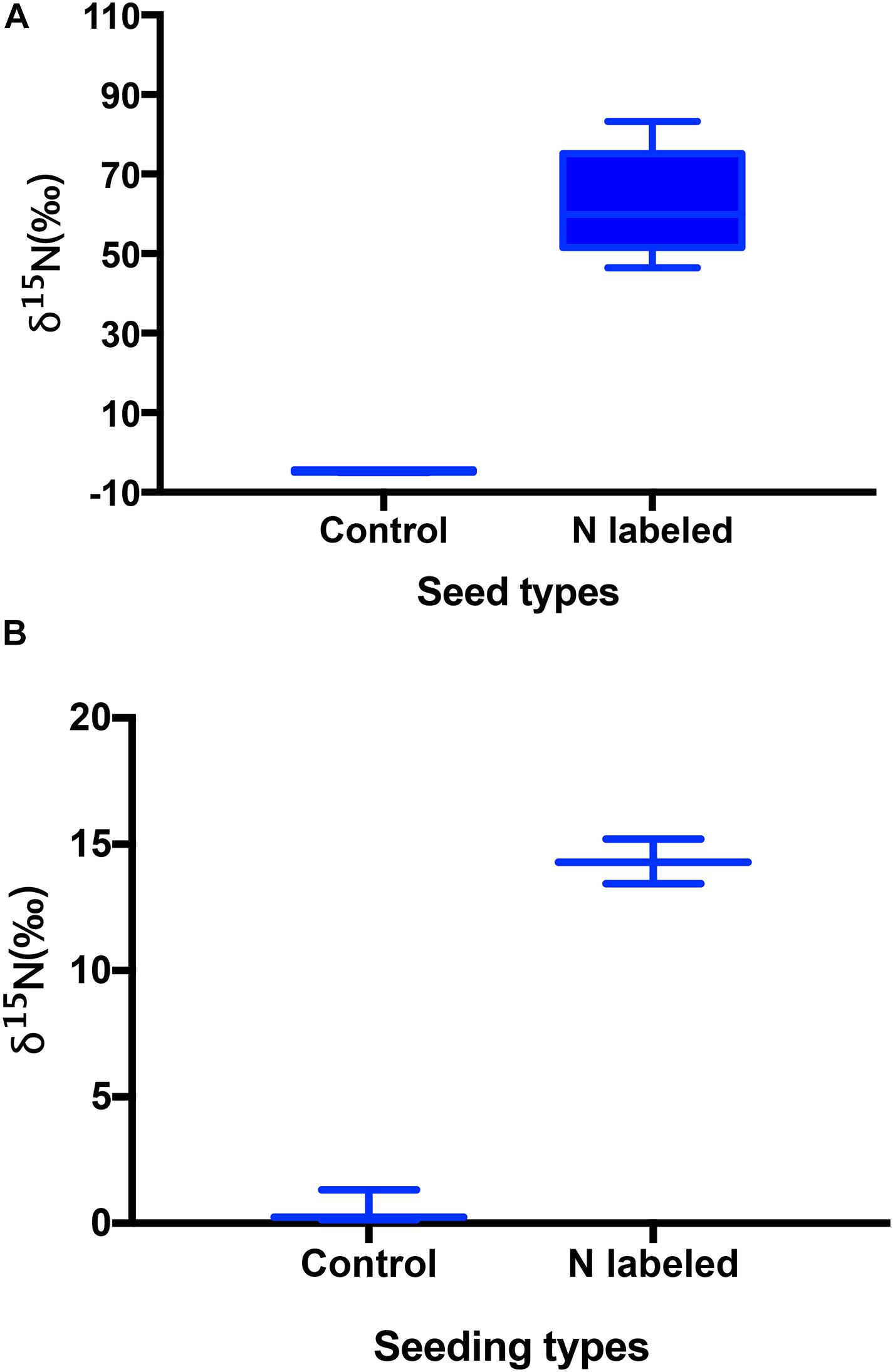
Figure 1. Effects of xylem injection of 15NH4Cl and K15NO3 on 15N enrichment of acorns (A) and seedlings (B). Box plots show the median, upper (25%), and lower quartile (25%) of data and whiskers indicate the maximum and minimum values, with the exception of outliers, and the same below.
Effects of Xylem 15N Injection on Seed Germination and Seedling Establishment
Xylem 15N injection had no significant effect on seed germination rates of Q. variabilis (t = 0.849, df = 18, P = 0.407) (Figure 3A). Moreover, we detected no significant effects of xylem 15N injection on shoot and root dry mass of Q. variabilis seedlings (t = −1.474, df = 1, P = 0.114 t = 1.594, df = 87, P = 0.114; Figure 3B).
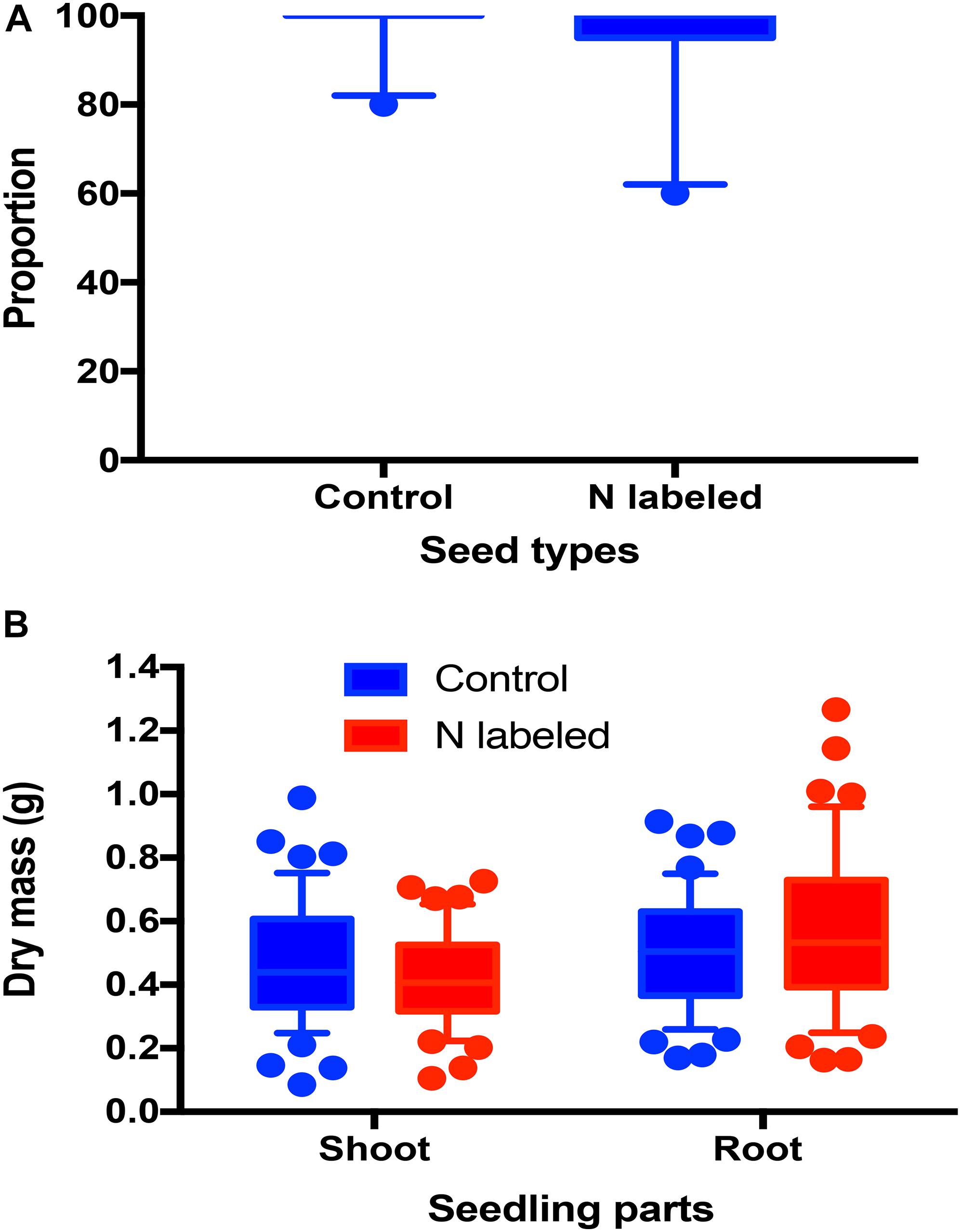
Figure 3. Effects of xylem injection of 15NH4Cl and K15NO3 on acorn germination rate (A) and shoot and root dry masses (B).
Effects of Xylem 15N Injection on Seed Selection by L. edwardsi
Overall, xylem 15N injection did not affect seed selection by L. edwardsi. In detail, acorns remaining intact in situ (IIS) were not different between the control and 15N-enriched groups after removed by L. edwardsi (t = −1.747, df = 9, P = 0.115) (Figure 4A). Similarly, no significant differences were found in acorns eaten in situ (EIS) (t = 2.023, df = 9, P = 0.074) and eaten after removal (EAR) (t = 1.060, df = 9, P = 0.317) (Figure 4A). Moreover, xylem 15N injection did not affect both scatter-hoarding (SH) and larder-hoarding by L. edwardsi (t = 1.300, df = 9, P = 0.226; t = −0.275, df = 9, P = 0.790) (Figure 4A). Xylem 15N injection showed no effect on cache pilferage by L. edwardsi (t = 1.450, df = 9, P = 0.181) (Figure 4B). Neither male nor female of L. edwardsi showed any preference to scatter-hoard the paired acorns (t = −0.250, df = 4, P = 0.815; t = −0.152, df = 4, P = 0.887). The same patterns were found in acorns eaten in situ, eaten after removal, larder-hoarded, and cache pilfering (all P > 0.05).
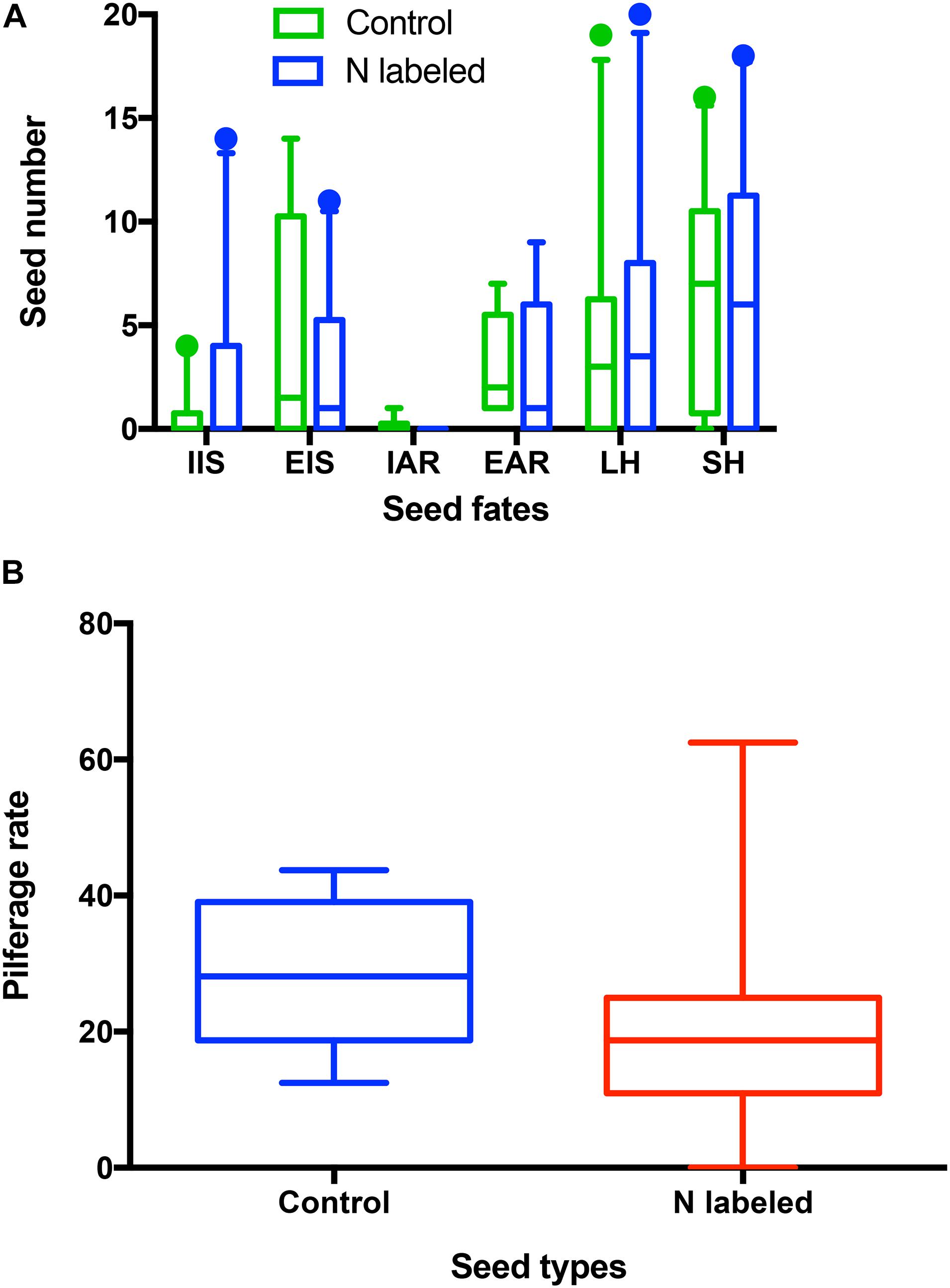
Figure 4. Effects of xylem injection of 15NH4Cl and K15NO3 on seed fates (A) and pilferage rate (B) of Quercus variabilis manipulated by Leopoldamys edwardsi in the enclosures. IIS, EIS, EAR, IAR, and SH stand for seeds intact in situ, eaten in situ, eaten after removal, intact after removal, and scatter-hoarded, respectively.
Seedling Recruitment Based on Isotopic Signals in Seeds
In spring 2019, we totally located 811 1-year seedlings of Q. variabilis around the seven focal trees through area-constrained searches by three people for 10 days (Figure 5A). Based on the isotope analyses, 234 seedlings of Q. variabilis showed δ15N values higher than 15‰ (average 23.01‰) and were regarded to be originated from the seven focal parent trees that received 15N xylem injection (Supplementary Figure 4). The remaining seedlings (577, 71.1%), however, exhibited extreme low δ15N values (average 1.45‰) and were considered as natural seedlings originated from the untreated Q. variabilis trees. The frequency distribution curve of Q. variabilis seedlings near the parent trees appeared right-skewed (Figure 5B), generating an average distance between the 15N-enriched seedlings and the corresponding trees of Q. variabilis estimated at 5.6 m.
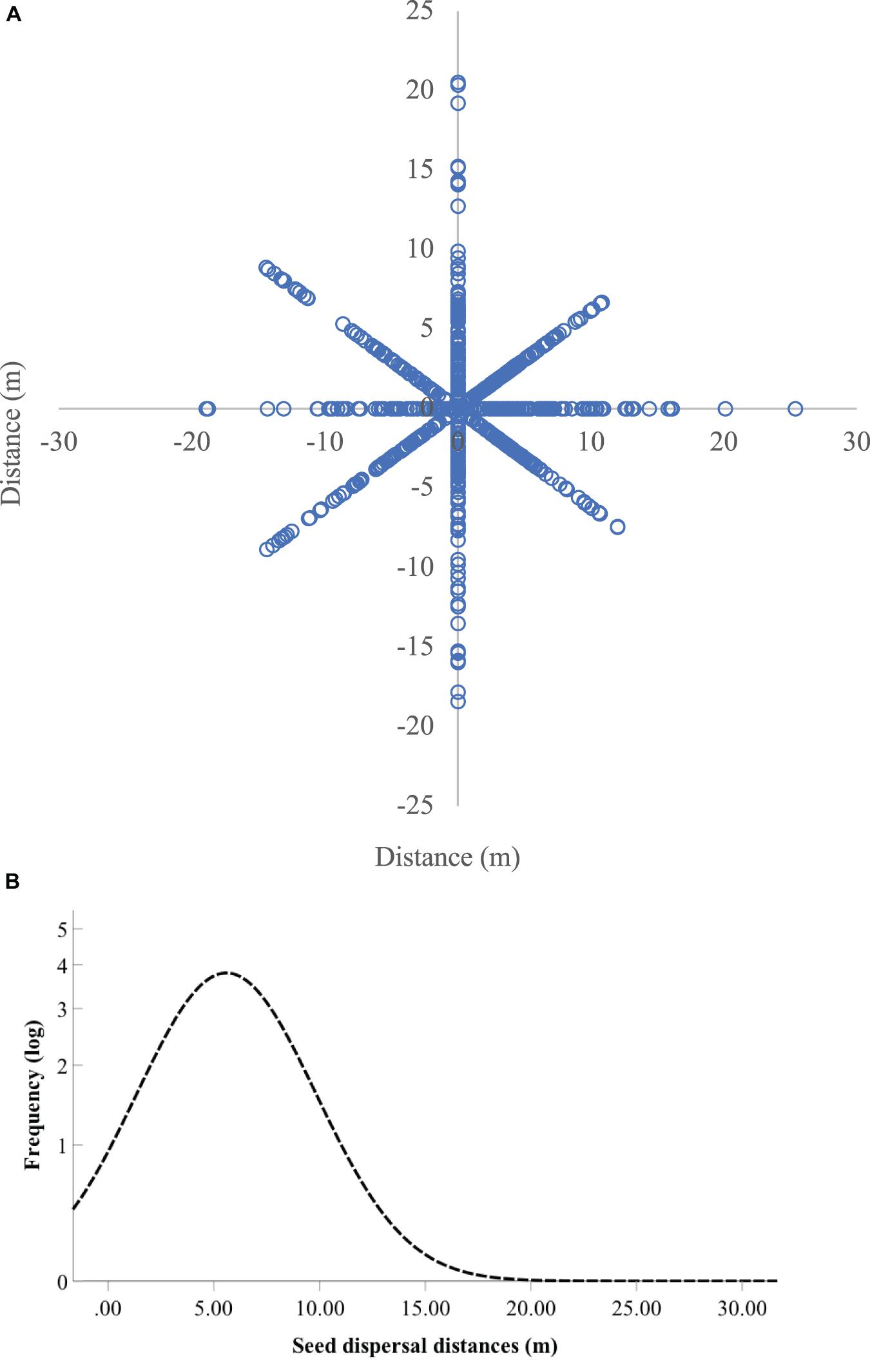
Figure 5. The spatial distribution pattern (A) and frequency distribution curve of observed dispersal distances (B) of Quercus variabilis seedlings in the field. In total, 811 seedlings were surveyed, in which 234 seedlings showed δ15N values higher than the natural ones.
Discussion
Our experiments clearly showed that xylem 15N injection of 60 mmol/L 15NH4Cl and K15NO3 successfully resulted in 15N enrichment in acorns of Q. variabilis. Most importantly, 15N enrichment was also successfully detected in the 1-year seedlings of Q. variabilis receiving xylem 15N injection. The apparent differences in δ15N values between the control and 15N-enriched seedlings helped us easily track seedling recruitment in the field (Supplementary Figure 4). Compared to the δ15N values of the natural seedlings, we successfully tracked seedlings produced by the 15N-enriched seeds of Q. variabilis in the field, verifying the reliability of xylem injection of 15NH4Cl and K15NO3 to mark seeds for tracking seedling recruitment. The shape of the dispersal kernel, i.e., the probability distribution of dispersal distances, has great influence on the ultimate distribution of genetic diversity within populations. However, in contrast to the highly left-skewed (fat tail) distributions reported for many empirical studies (Goto et al., 2006; Morales and Carlo, 2006; Hardy, 2009), we find that the dispersal distribution in Q. variabilis near the parent trees was thin-tailed and right-skewed (Figure 5B). The thin-tailed and right-skewed shape of the Q. variabilis seedling distribution does imply that long-distance dispersal is rare in this species (Barlow et al., 2013). In many species, seedling mortality decreases with increasing distance from the mother plants (Peters, 2003). However, acorns of Q. variabilis exhibit no dormancy and germinate rapidly after seed fall, generating robust taproots penetrating deep in the soil. Moreover, the robust taproots alone, even without support of cotyledon reserves, show amazing capability to regenerate into normal seedlings (Xiang et al., 2019). Therefore, we predict that the germination schedule and rapid colonization of taproots are highly responsible for the thin-tailed and right-skewed shape of the Q. variabilis seedling distribution than in those species exhibiting fat-tailed distributions.
We found that 15N enrichment in seeds can be achieved regardless of tree size of Q. variabilis. This implies that trees entering their flowering stage can successfully transport and distribute nitrogen isotope to their seeds via xylem systems. The high efficiency of transport and incorporation of 15N into seeds via xylem is well beyond our current knowledge (Horwath et al., 1992; Proe et al., 2000; Nair et al., 2014). We also noticed that 15N enrichment in seeds was dependent on the tree size under the same dosage of 15N solution. However, the tree size-dependent feature of isotope enrichment in seeds may allow for a comparison of response variables from several enriched focal trees that scattered in one landscape (Carlo et al., 2009). Unfortunately, we failed to explore the relation between 15N enrichment in seeds and tree size due to small sample size in this study, though it could be an interesting point in future work. The application of this technique using stable nitrogen isotope is making possible the exact identification of within-population seedlings established at long distances. Because 15N was successfully allocated into the mature seeds, we suggest that xylem 15N injection could be applied to a variety of tree species bearing large-sized seeds for tracking seed dispersal and seedling recruitment in different forest ecosystems. Therefore, our experiments with these plant species demonstrate that xylem 15N injection method can be a valuable tool for studies of seed dispersal and recruitment.
Swanston and Myrold (1998) found that both 15 NO and 15 NH at levels approaching 1% of crown N were effective in labeling trees. Horwath et al. (1992) reported that adding greater than 5–10% of the crown N through stem injection resulted in toxic effects on trees. In our study, however, only 2.7 g N was injected into each tree, much less than 1% of the crown N. Our pilot study has shown that there is a positive relationship between seed δ15N and injected 15N. Due to the fact that 15N will be diluted with the development of seedlings (Carlo et al., 2009; Yi et al., 2014), we strongly recommend xylem injection of 0.2–0.3 g 15N to a single tree with DBH > 10 cm for future studies, which will ensure higher levels of 15N enrichment in both seeds and seedlings and benefit seedling tracking in the field. Most vascular plants acquire the main available forms of nitrogen (N) as NH or NO by taking up through root absorption and then undergo xylem transport within plant parts (Oh et al., 2008); therefore, 15NH415NO3 can be an alternative compound for xylem injection (Nair et al., 2014).
Seed manipulation of previous tracking methods has been found to cause seed death, fungal contamination, or even low germination rate (Den Ouden et al., 2005; Iida, 2006; Canner and Spence, 2011; Hirsch et al., 2012a,b; Suselbeek et al., 2013; Yi and Wang, 2015), which makes it a little bit difficult to track the real patterns of seedling recruitment in the field. However, our xylem 15N injection method caused no such problems because xylem injection did not involve any surgical alterations to 15N enriched seeds that resembled the natural counterparts, which allows tracking for actual seed dispersal kernel. Previous investigations have shown that seed manipulation by marking also affects seed consumption and predation by seed-eating animals (Alverson and Díaz, 1989; Winn, 1989; Den Ouden et al., 2005; Pons and Pausas, 2007; Yi and Zhang, 2008; Yi et al., 2011). On the contrary, our in vivo xylem 15N injection showed no significant effects on seed selection by seed-eating animals as well as seed germination and seedling development. Although the N and protein contents of acorns were slightly enhanced by xylem 15N injection, no significant changes were detected in seed hoarding and pilfering of L. edwardsi, suggesting that xylem 15N injection is unable to cause detectable changes in seed odor and palatability of Q. variabilis and then to affect actual patterns of seed dispersal. We are unable to guarantee that seed selection in the enclosures matches the seed dispersal patterns in the field; however, data collected from the enclosures usually reflect the true patterns (Xiao et al., 2008). Injecting isotopic signals into seeds through xylem appear to be an undetectable clue to seed-eating animals and have no negative effects on seed germination and seedling development. Despite lacking experimental evidence, it is not possible that xylem injection of 2.7 g N into a tree with DBH of 20–30 cm will cause significant variations of seed production. Making this judgment is based on the fact that a supplement of 15 g m–2 year–1 of nitrogen shows reliable sign of increase in acorn production (Bogdziewicz et al., 2017). Even if N xylem injection will potentially increase seed production, high level of seed availability has been shown to promote seed dispersal (Vander Wall, 2002; Jansen et al., 2004). Perhaps the main benefit of the xylem injection method is that it labels the seeds without directly affecting them. Moreover, our xylem 15N injection represents a passive injection system that not only avoids multiple administration of nitrogen solution onto the plant parts (Carlo et al., 2009; Putz et al., 2011; Castellano and Gorchov, 2013; Forster and Herrmann, 2014), but also benefits 15N enrichment in seeds along with their development. In addition, we found that drilling small shallow holes in the tree trunks for xylem 15N injection was unlikely to cause severe damage to trees that has already entered into their flowering stages. Therefore, the xylem 15N injection method is expected to be powerful to track seed dispersal and seedling recruitment mediated by hoarding animals.
Similar to the 15N-urea foliar-spraying methods and seed-soaking approaches (Carlo et al., 2009; Castellano and Gorchov, 2013; Forster and Herrmann, 2014; Yi et al., 2014; Yi and Wang, 2015), the xylem 15N injection method requires much effort to locate potential seedlings over a wide range after seeds are dispersed. However, different from other seed-labeling approaches (Wang et al., 2013), our xylem 15N injection method appears to be labor-saving as the multiple-step seed movements by animals were ignored. This deployment can be well accepted because seedling recruitment is regarded as the ultimate outcome of seed dispersal ecology, and most seed shadows have been estimated from spatial patterns of seedlings (Vander Wall, 1990). Some may doubt that xylem 15N injection will not be applicable for plants bearing small seeds (Longland and Clements, 1995). Recent studies have verified that isotopic signals can be transferred from 15N-enriched seeds to their seedlings for small-seeded or wind-dispersed tree species (Castellano and Gorchov, 2013; Forster and Herrmann, 2014; Wang et al., 2016). Moreover, we can collect seedlings for analysis at early stages to compensate for isotope dilution in samples (Yi et al., 2014), which facilitates us to locate and identify the focal seedlings. Moreover, our xylem 15N injection method permits seed removal directly from the canopies either by wind or by avian frugivores participating in long-distance dispersal, which has been verified by Carlo et al. (2009). Although tracking long-distance dispersal using this method appears to be hard, previous seed tracking methods also face this problem (Hirsch et al., 2012a). Despite this, we believe that our xylem 15N injection method could broaden the study of dispersal ecology and may provide a powerful tool to estimate long-distance seed dispersal. Our xylem 15N injection method also allows tracking seedling recruitment from many individuals within the same site by deliberately altering the 15N dosage levels (Carlo et al., 2009; Yi et al., 2014) or selecting trees with different DBH or crown size.
We found that acorns of Q. variabilis were successfully 15N enriched in this study, and seed-eating animals showed no significant caching and pilfering preference, implying that our xylem 15N injection method can be applied to a variety of tree species. Our previous 15N injection attempts on Melia azedarach, Elaeocarpus decipiens, Juglans mandshurica, Q. mongolica, Q. fabri, and Q. variabilis from different genera have successfully resulted in enriched 15N in their seeds or nuts, though low 15N abundance in seeds was obtained (Supplementary Figure 2), which is mainly due to the low concentration of 15NH4Cl and K15NO3 (40 mmol/L) administrated. Therefore, our xylem 15N injection method makes it possible to track seedling recruitment of plants regardless of seed size, which will play an important role in the seed dispersal ecology.
Conclusion and Management Implications
In summary, we showed that xylem injection of 15N successfully enriched 15N in both the acorns and seedlings of Q. variabilis. We also successfully tracked seedlings established from acorns dispersed by seed-eating animals in the field 15N stable isotopes, verifying the validity of the 15N xylem injection method to track seedling establishment. We therefore suggest that xylem 15N injection, combined with foliar application of 15N, is a reliable, labor-saving and user-friendly isotopic method for seed dispersal biology. We believe that our xylem 15N injection method will provide new perspectives and some novel approaches to tracking seed dispersal and seedling recruitment mediated by seed-eating animals in seed dispersal ecology. Given that seed dispersal mediated by seed-eating animals and natural regeneration have been widely recognized as key processes within forest ecosystems, our tracking method using 15N xylem injection will be promising in better understanding the actual patterns of seed dispersal and seedling recruitment, and natural succession of forest ecosystems. In addition, the 15N tracking method is powerful to demonstrate the actual pattern of spatial dispersal and therefore to deepen our understanding of seed dispersal ecology, highlighting the importance of seed dispersal ecology for natural regeneration and forest management practices.
Data Availability Statement
The datasets presented in this study are publicly available. This data can be found here: Figshare, doi: 10.6084/m9.figshare.14390918.
Ethics Statement
The animal study was reviewed and approved by the Ethics Committee of Qufu Normal University.
Author Contributions
XY designed the study. SY, MW, and MJ carried out the experiments. SY performed the data analyses. XY and SY wrote the first draft of the manuscript. All authors contributed substantially to revisions.
Funding
Funding for this study was supported by the National Natural Science Foundation of China (32070447 and 31760156) and Youth Talent Introduction and Education Program of Shandong Province (20190601).
Conflict of Interest
The authors declare that the research was conducted in the absence of any commercial or financial relationships that could be construed as a potential conflict of interest.
Acknowledgments
We thank Yuan Li, Yinhua Deng, and Youbi Shen for their field help.
Supplementary Material
The Supplementary Material for this article can be found online at: https://www.frontiersin.org/articles/10.3389/fpls.2021.582530/full#supplementary-material
Supplementary Figure 1 | A map with the study area.
Supplementary Figure 2 | An illustration of xylem injection of 15NH4Cl and K15NO3 into Quercus variabilis in the field.
Supplementary Figure 3 | Isotopic values of seeds of several tree species xylem injected with 40 mmol/L 15NH4Cl and K15NO3 compared to the control.
Supplementary Figure 4 | Difference in δ15N of enriched and natural seedlings of Quercus variabilis collected in the field.
References
Alverson, W. S., and Díaz, A. G. (1989). Measurement of the dispersal of large seeds and fruits with a magnetic locator. Biotropica 21, 61–63. doi: 10.2307/2388443
Barlow, E. J., Daunt, F., Wanless, S., Reid, J. M., and Frederiksen, M. (2013). Estimating dispersal distributions at multiple scales: within-colony and among-colony dispersal rates, distances and directions in European shags Phalacrocorax aristotelis. Ibis 155, 762–778. doi: 10.1111/ibi.12060
Bogdziewicz, M., Crone, E. E., Steele, M. A., and Zwolak, R. (2017). Effects of nitrogen deposition on reproduction in a masting tree: benefits of higher seed production are trumped by negative biotic interactions. J. Ecol. 105, 310–320. doi: 10.1111/1365-2745.12673
Bologna, A., Toffin, E., Detrain, C., and Campo, A. (2017). An automated method for large-scale monitoring of seed dispersal by ants. Sci. Rep. 7:40143.
Canner, J. E., and Spence, M. (2011). A new technique using metal tags to track small seeds over short distances. Ecol. Res. 26, 233–236. doi: 10.1007/s11284-010-0761-8
Carlo, T. A., and Norris, A. E. W. (2012). Direct nitrogen intake by petals. Oikos 121, 1953–1958. doi: 10.1111/j.1600-0706.2012.20527.x
Carlo, T. A., Tewksbury, J., and del Rio, C. M. (2009). A new method to track seed dispersal and recruitment using 15N isotope enrichment. Ecology 90, 3516–3525. doi: 10.1890/08-1313.1
Castellano, S. M., and Gorchov, D. L. (2013). Using a stable isotope to label seeds and seedlings of an invasive shrub, Lonicera maackii. Invasive Plant Sci. Manage. 6, 112–117. doi: 10.1614/ipsm-d-12-00053.1
Chang, G., and Zhang, Z. (2011). Differences in hoarding behaviors among six sympatric rodent species on seeds of oil tea (Camellia oleifera) in southwest China. Acta Oecol. 37, 165–169. doi: 10.1016/j.actao.2011.01.009
Cui, J., Lamade, E., Fourel, F., and Tcherkez, G. (2020). δ15N values in plants are determined by both nitrate assimilation and circulation. New Phytol. 226, 1696–1707. doi: 10.1111/nph.16480
Davis, A. S., Daedlow, D., Schutte, B. J., and Westerman, P. R. (2011). Temporal scaling of episodic point estimates of seed predation to long-term predation rates. Methods Ecol. Evol. 2, 682–890. doi: 10.1111/j.2041-210x.2011.00119.x
Den Ouden, J., Jansen, P. A., and Smit, R. (2005). “Jays, mice and oaks: predation and dispersal of Quercus robur and Q. petraea in north-western Europe,” in Seed Fate: Predation, Dispersal and Seedling Establishment, eds P. M. Forget, J. E. Lambert, P. E. Hulme, and S. B. Vander Wall (Wallingford: CABI), 223–240. doi: 10.1079/9780851998060.0223
Deng, Y., Ju, M., and Yi, X. (2020). Exclusion of interspecific competition reduces scatter-hoarding of Siberian chipmunk Tamias sibiricus: a field study. Integr. Zool. 15, 127–134. doi: 10.1111/1749-4877.12416
Forster, C., and Herrmann, J. D. (2014). Tracking wind-dispersed seeds using 15N-isotope enrichment. Plant Biol. 16, 1145–1148.
Galetti, M., Donatti, C. I., Steffler, C., Genini, J., Bovendorp, R. S., and Fleury, M. (2010). The role of seed mass on the caching decision by agoutis, Dasyprocta leporina (Rodentia: Agoutidae). Zoologia 27, 472–476. doi: 10.1590/s1984-46702010000300022
Godoy, J. A., and Jordano, P. (2001). Seed dispersal by animals: exact identification of source trees with endocarp DNA microsatellites. Mol. Ecol. 10, 2275–2283. doi: 10.1046/j.0962-1083.2001.01342.x
Goto, S., Shimatani, K., Yoshimaru, H., and Takahashi, Y. (2006). Fat-tailed gene flow in the dioecious canopy tree species Fraxinus mandshurica var. japonica revealed by microsatellites. Mol. Ecol. 15, 2985–2996. doi: 10.1111/j.1365-294x.2006.02976.x
Gu, H., Zhao, Q., and Zhang, Z. (2017). Does scatter-hoarding of seeds benefit cache owners or pilferers? Integr. Zool. 12, 477–488. doi: 10.1111/1749-4877.12274
Gu, Z., Wu, B., Wu, L., and Hang, Y. (2009). Analysis of total lipid contents and fatty acids composition of three species of euphorbia in Jiangsu province. Chem. Industry For. Prod. 29, 63–66.
Hirsch, B. T., Kays, R., and Jansen, P. A. (2012a). A telemetric thread tag for tracking seed dispersal by scatter-hoarding rodents. Plant Ecol. 213, 933–943. doi: 10.1007/s11258-012-0054-0
Hirsch, B. T., Visser, M. D., Kays, R., and Jansen, P. A. (2012b). Quantifying seed dispersal kernels from truncated seed-tracking data. Methods Ecol. Evol. 3, 595–602. doi: 10.1111/j.2041-210x.2011.00183.x
Horwath, W., Paul, E., and Pregitzer, K. (1992). Injection of nitrogen-15 into trees to study nitrogen cycling in soil. Soil Sci. Soc. Am. J. 58, 316–319. doi: 10.2136/sssaj1992.03615995005600010051x
Iida, S. (2006). Dispersal of Quercus serrata acorns by wood mice in and around canopy gaps in a temperate forest. For. Ecol. Manage. 227, 71–78. doi: 10.1016/j.foreco.2006.02.010
Jansen, P. A., Bongers, F., and Hemerik, L. (2004). Seed mass and mast seeding enhance dispersal by a neotropical scatter-hoarding rodent. Ecol. Monogr. 74, 569–589. doi: 10.1890/03-4042
Lemke, A., Lippe, M. V. D., and Kowarik, I. (2010). New opportunities for an old method: using fluorescent colours to measure seed dispersal. J. Appl. Ecol. 46, 1122–1128. doi: 10.1111/j.1365-2664.2009.01691.x
Levey, D. J., and Sargent, S. (2000). A simple method for tracking vertebrate- dispersed seed. Ecology 81, 267–274. doi: 10.1890/0012-9658(2000)081[0267:asmftv]2.0.co;2
Levine, J. M., and Murrell, D. J. (2003). The community-level consequences of seed dispersal patterns. Annu. Rev. Ecol. Syst. 34, 549–574. doi: 10.1146/annurev.ecolsys.34.011802.132400
Lichti, N. I., Steele, M. A., Zhang, H., and Swihart, R. K. (2014). Mast species composition alters seed fate in North American rodent-dispersed hardwoods. Ecology 95, 1746–1758. doi: 10.1890/13-1657.1
Longland, W. S., and Clements, C. (1995). Use of fluorescent 23 pigments in studies of seed caching by rodents. J. Mammal. 76, 1260–1266. doi: 10.2307/1382621
Lu, G., and Li, X. (2002). Quick analysis of starch content of sweet potato by HCl hydrolysis-DNS method. J. Chin. Cereals Oils Assoc. 17, 25–28.
Maines, L. L., and Bruch, M. D. (2012). Identification of unknown chloride salts using a combination of qualitative analysis and titration with silver nitrate: a general chemistry laboratory. J. Chem. Educ. 89, 933–935. doi: 10.1021/ed200518w
Marschner, H., and Marschner, P. (2012). Mineral Nutrition of Higher Plants. London: Academic Press.
Montoro Girona, M., Lussier, J. M., Morin, H., and Thiffault, N. (2018). Conifer regeneration after experimental shelterwood and seed-tree treatments in boreal forests: finding silvicultural alternatives. Front. Plant Sci. 9:1145.
Moore, J., McEuen, A. B., Swihart, R. K., Contreras, T. A., and Steele, M. A. (2007). Determinants of seed removal distance by scatter-hoarding rodents in deciduous forests. Ecology 88, 2529–2540. doi: 10.1890/07-0247.1
Morales, J. M., and Carlo, T. A. (2006). The effects of plant distribution and frugivore density on the scale and shape of dispersal kernels. Ecology 87, 1489–1496. doi: 10.1890/0012-9658(2006)87[1489:teopda]2.0.co;2
Muñoz, A., and Bonal, R. (2011). Linking seed dispersal to cache protection strategies. J. Ecol. 99, 1016–1025. doi: 10.1111/j.1365-2745.2011.01818.x
Nair, R., Weatherall, A., Perks, M., and Mencuccini, M. (2014). Stem injection of 15N-NH4NO3 into mature Sitka spruce (Picea sitchensis). Tree Physiol. 34, 1130–1140. doi: 10.1093/treephys/tpu084
Niu, H., Zhang, J., Wang, Z., Huang, G., and Zhang, H. (2020). Context-dependent responses of food-hoarding to competitors in Apodemus peninsulae: implications for coexistence among asymmetrical species. Integr. Zool. 15, 115–126. doi: 10.1111/1749-4877.12425
Oh, K., Kato, T., and Xu, H. L. (2008). Transport of nitrogen assimilation in xylem vessels of green tea plants fed with NH4-N and NO3-N. Pedosphere 18, 222–226. doi: 10.1016/S1002-0160(08)60010-7
Peters, H. A. (2003). Neighbour-regulated mortality: the influence of positive and negative density dependence on tree populations in species-rich tropical forests. Ecol. Lett. 6, 757–765. doi: 10.1046/j.1461-0248.2003.00492.x
Pons, J., and Pausas, J. G. (2007). Acorn dispersal estimated by radio tracking. Oecologia 153, 903–911. doi: 10.1007/s00442-007-0788-x
Proe, M. F., Mead, D. J., and Byrne, D. (2000). Effect of pruning on nitrogen dynamics within crowns of Pinus radiata. Tree Physiol. 20, 653–661. doi: 10.1093/treephys/20.10.653
Putz, B., Drapela, T., Wanek, W., Schmidt, O., Frank, T., and Zaller, J. G. (2011). A simple method for in situ-labelling with 15N and 13C of grassland plant species by foliar brushing. Methods Ecol. Evol. 2, 326–332. doi: 10.1111/j.2041-210x.2010.00072.x
Schmidt, O., and Scrimgeour, C. M. (2001). A simple urea leaf-feeding method for the production of 13C and 15N labelled plant material. Plant Soil 229, 197–202.
Steele, M. A., Bugdal, M., Yuan, A. W., Bartlow, A., Buzalewski, J., Lichti, N., et al. (2011). Cache placement, pilfering, and a recovery advantage in a seed-dispersing rodent: could predation of scatter hoarders contribute to seedling establishment? Acta Oecol. 37, 554–560. doi: 10.1016/j.actao.2011.05.002
Steele, M. A., Yi, X., and Zhang, H. (2018). Plant-animal interactions: patterns and mechanisms in terrestrial ecosystems. Integr. Zool. 13, 225–227. doi: 10.1111/1749-4877.12320
Suselbeek, L., Jansen, P. A., Prins, H. H. T., and Steele, M. A. (2013). Tracking rodent-dispersed large seeds with passive integrated transponder (PIT) tags. Methods Ecol. Evol. 4, 513–519. doi: 10.1111/2041-210x.12027
Swanston, C., and Myrold, D. (1998). Evaluation of the stem injection technique and subsequent 15N partitioning in red alder crowns. Plant Soil 198, 63–69.
Tewksbury, J. J., Levey, D. J., Haddad, N. M., Sargent, S., Orrock, J. L., Weldon, A., et al. (2002). Corridors affect plants, animals, and their interactions in fragmented landscapes. Proce. Natl. Acad. Sci. U.S.A. 99, 12923–12926. doi: 10.1073/pnas.202242699
Vander Wall, S. B. (2002). Masting in animal-dispersed pines facilitates seed dispersal. Ecology 83, 3508–3516. doi: 10.1890/0012-9658(2002)083[3508:miadpf]2.0.co;2
Wang, B., Ye, C., Cannon, C. H., and Chen, J. (2013). Dissecting the decision making process of scatter-hoarding rodents. Oikos 122, 1027–1034. doi: 10.1111/j.1600-0706.2012.20823.x
Wang, G., Song, Y., Yan, Q., and Zhang, J. (2016). Accumulation responses of seeds and seedlings to N isotope for two typical broad-leaved trees in northeast China. Chin. J. Appl. Ecol. 27, 2379–2389.
Wang, Q., Xie, Y., Zhao, W., Li, P., Qian, H., and Wang, X. (2014). Rapid microchip-based faims determination of trimethylamine, an indicator of pork deterioration. Anal. Methods 6:2965. doi: 10.1039/c3ay41941j
Wang, Z., Wang, B., Yi, X., Yan, C., Cao, L., and Zhang, Z. (2018). Scatter-hoarding rodents are better pilferers than larder-hoarders. Anim. Behav. 141, 151–159. doi: 10.1016/j.anbehav.2018.05.017
White, J. D., Bronner, G. N., and Midgley, J. J. (2017). Camera-trapping and seed-labelling reveals widespread granivory and scatter-hoarding of nuts by rodents in the fynbos biome. Zool. Africana 52, 31–41. doi: 10.1080/15627020.2017.1292861
Winn, A. A. (1989). Using radionuclide labels to determine the post- dispersal fate of seeds. Trends Ecol. Evol. 4, 1–2. doi: 10.1016/0169-5347(89)90002-5
Wróbel, A., and Zwolak, R. (2013). The choice of seed tracking method influenced fate of beech seeds dispersed by rodents. Plant Ecol. 214, 471–475. doi: 10.1007/s11258-013-0183-0
Xiang, J., Li, X., and Yi, X. (2019). One acorn produces two seedlings in Chinese cork oak Quercus variabilis. Plant Signal. Behav. 14:e1654817. doi: 10.1080/15592324.2019.1654817
Xiao, Z., Chang, G., and Zhang, Z. (2008). Testing the high-tannin hypothesis with scatter-hoarding rodents: experimental and field evidence. Anim. Behav. 75, 1235–1241. doi: 10.1016/j.anbehav.2007.08.017
Xiao, Z., and Zhang, Z. (2003). How to trace seeds and fruits dispersed by frugivorous animals: a review. Biodivers. Sci. 11, 248–255. In Chinese with English Abstract.
Yang, F., Schufele, R., Liu, H. T., Ostler, U., and Gong, X. Y. (2019). Gross and net nitrogen export from leaves of a vegetative C4 grass. J. Plant Physiol. 244:153093. doi: 10.1016/j.jplph.2019.153093
Yang, X., Yan, C., Gu, H., and Zhang, Z. (2020). Interspecific synchrony of seed rain shapes rodent-mediated indirect seed–seed interactions of sympatric tree species in a subtropical forest. Ecol. Lett. 23, 45–54. doi: 10.1111/ele.13405
Yi, X., and Ju, M. (2020). Soil nitrogen assimilation of 1-year oak seedlings: implication for forest fertilization and management. For. Ecol. Manage. 456:117703. doi: 10.1016/j.foreco.2019.117703
Yi, X., Liu, G., Zhang, M., Dong, Z., and Yang, Y. (2014). A new approach for tracking seed dispersal of large plants: soaking seeds with 15N-urea. Ann. For. Sci. 71, 43–49. doi: 10.1007/s13595-013-0331-7
Yi, X., and Wang, Z. (2015). Tracking animal-mediated seedling establishment from dispersed acorns with the aid of the attached cotyledons. Mamm. Res. 60, 149–154. doi: 10.1007/s13364-015-0214-4
Yi, X., Yang, Y., and Zhang, Z. (2011). Different interspecific effects of mast seeding on seed removal of two sympatric Corylus species. Plant Ecol. 212, 785–793. doi: 10.1007/s11258-010-9861-3
Yi, X., and Zhang, Z. (2008). Seed predation and dispersal of glabrous filbert (Corylus heterophylla) and pilose filbert (Corylus mandshurica) by small mammals in a temperate forest, northeast China. Plant Ecol. 196, 135–142. doi: 10.1007/s11258-007-9340-7
Keywords: seed dispersal, isotopic clue, 15N labeling, xylem injection, seedling recruitment
Citation: Wang M, Yi S, Ju M and Yi X (2021) Tracking Animal-Dispersed Seedlings Using 15N Xylem Injection Method. Front. Plant Sci. 12:582530. doi: 10.3389/fpls.2021.582530
Received: 15 July 2020; Accepted: 16 March 2021;
Published: 30 April 2021.
Edited by:
Sebastian Leuzinger, Auckland University of Technology, New ZealandReviewed by:
Miguel Montoro Girona, Université du Québec en Abitibi-Témiscamingue, CanadaMark Duxbury, Auckland University of Technology, New Zealand
Copyright © 2021 Wang, Yi, Ju and Yi. This is an open-access article distributed under the terms of the Creative Commons Attribution License (CC BY). The use, distribution or reproduction in other forums is permitted, provided the original author(s) and the copyright owner(s) are credited and that the original publication in this journal is cited, in accordance with accepted academic practice. No use, distribution or reproduction is permitted which does not comply with these terms.
*Correspondence: Xianfeng Yi, eW1wY2xvbmdAMTYzLmNvbQ==