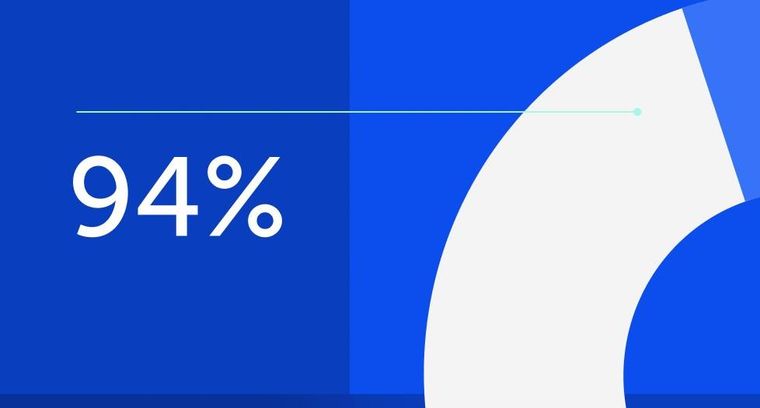
94% of researchers rate our articles as excellent or good
Learn more about the work of our research integrity team to safeguard the quality of each article we publish.
Find out more
ORIGINAL RESEARCH article
Front. Plant Sci., 20 January 2021
Sec. Plant Development and EvoDevo
Volume 11 - 2020 | https://doi.org/10.3389/fpls.2020.621338
This article is part of the Research TopicMolecular Mechanisms of Flowering Plant ReproductionView all 19 articles
Magnesium (Mg) is an abundant and important cation in cells. Plants rely on Mg transporters to take up Mg from the soil, and then Mg is transported to anthers and other organs. Here, we showed that MGT6+/− plants display reduced fertility, while mgt6 plants are fertile. MGT6 is expressed in the anther at the early stages. Pollen mitosis and intine formation are impaired in aborted pollen grains (PGs) of MGT6+/− plants, which is similar to the defective pollen observed in mgt5 and mgt9 mutants. These results suggest that Mg deficiency leads to pollen abortion in MGT6+/− plants. Our data showed that mgt6 organs including buds develop significantly slower and mgt6 stamens accumulate a higher level of Mg, compared with wild-type (WT) and MGT6+/− plants. These results indicate that slower bud development allows mgt6 to accumulate sufficient amounts of Mg in the pollen, explaining why mgt6 is fertile. Furthermore, we found that mgt6 can restore fertility of mgt5, which has been reported to be male sterile due to defects in Mg transport from the tapetum to microspores and that an additional Mg supply can restore its fertility. Interestingly, mgt5 fertility is recovered when grown under short photoperiod conditions, which is a well-known factor regulating plant fertility. Taken together, these results demonstrate that slow development is a general mechanism to restore mgts fertility, which allows other redundant magnesium transporter (MGT) members to transport sufficient Mg for pollen formation.
Magnesium (Mg) is one of the most abundant and essential divalent metal cations in prokaryotic and eukaryotic cells. The most important function of Mg is to act as a cofactor of many enzymes. For example, Mg is essential for DNA and RNA polymerases, aminoacyl-tRNA synthetases, and ATPase. Mg-dependent enzymes participate in many metabolic and developmental processes, such as protein and nucleic acid synthesis, lipid and carbohydrate metabolism, and membrane stability (Rissler et al., 2002; Ador et al., 2004; Dudev and Lim, 2013; Gao and Yang, 2016; Li et al., 2016; Apell et al., 2017). In humans, disturbance of Mg metabolism leads to various diseases, such as heart disease, diabetes mellitus, Parkinson’s disease, Alzheimer’s disease, cancer, and growth retardation (Glasdam et al., 2016). In plants, a lack of Mg affects normal photosynthesis, growth development, and fertility (Rissler et al., 2002; Gebert et al., 2009; Xu et al., 2015).
For animals, it is easy to intake sufficient amounts of Mg by consuming Mg-containing foods such as leaves, seeds, and seafood (Glasdam et al., 2016). However, land plants obtain Mg from the soil only by their roots. Mg uptake and the subsequent transport to different organs rely on Mg transporters, which have been widely identified in both prokaryotes and eukaryotes (Snavely et al., 1989; Schmitz et al., 2003). In Arabidopsis, the magnesium transporter (MGT) family includes nine members (Schock et al., 2000; Li et al., 2001). MGT1, MGT2, MGT3, MGT6, and MGT7 are expressed in the roots and are involved in Mg uptake and distribution. The mgt1mgt2mgt3, mgt6, and mgt7 mutants display growth retardation under low-Mg conditions (Gebert et al., 2009; Lenz et al., 2013; Mao et al., 2014; Oda et al., 2016). MGT10 is expressed in the leaves and is essential for chloroplast development and photosynthesis (Sun et al., 2017).
In flowering plants, the stamen is a distal organ located far from the root. Pollen develops in the anther, and mature pollen is released after anther dehiscence (Xu et al., 2019). The anther consists of four microsporangiums, which are connected with vascular bundle of filament by the connective cells (Scott et al., 2004). Each microsporangium is surrounded by a layer of tapetum which provides various nutrients and materials such as sporopollenin for pollen development (Ariizumi and Toriyama, 2011; Wang et al., 2018). Mg is transported through filaments and connective cells to the four-lobed structure of anthers. In each locule, the tapetum secretes Mg to support pollen formation (Xu et al., 2015). Of the MGT family, MGT4, MGT5, and MGT9 have been reported to be involved in pollen formation and plant fertility. Pollen of mgt4, mgt5, and mgt9 show similar defects in intine formation and pollen mitosis, suggesting insufficient amounts of Mg in the pollen of these plants (Li et al., 2015; Xu et al., 2015). Both MGT4 and MGT9 are highly expressed in pollen. MGT4 is located in the endoplasmic reticulum and expressed in pollen from the bicellular to mature pollen stage (Li et al., 2015). MGT9 is located on the plasma membrane of microspores (MSps) and is involved in the absorption of Mg from locules (Chen et al., 2009; Xu et al., 2015). MGT5 is expressed in the tapetum and MSps. It has dual localization, occurring in both mitochondria and the plasma membrane. In the tapetum, MGT5 is located in the plasma membrane and is important for Mg transport from the tapetum to the locule. Moreover, MGT5 is also essential for mitochondrial Mg homeostasis in pollen (Li et al., 2008; Xu et al., 2015). mgt5 plants are male sterile. Under high-Mg conditions, the fertility of these plants is fully restored, suggesting that other MGTs are also involved in Mg transport (Xu et al., 2015). MGT6 is expressed in the root and flower (Gebert et al., 2009) and is localized in the plasma membrane (Mao et al., 2014). MGT6 is important for Mg uptake and transport, as its reduced expression in roots results in growth retardation (Mao et al., 2014; Yan et al., 2018). However, its function in anthers is unclear.
Male sterility is an important trait in agriculture. Thermosensitive genic male sterility (TGMS) and photoperiod-sensitive genic male sterility (PGMS) have been widely used in the two-line system hybrid rice breeding. In rice, several P/TGMS genes have been cloned (Chen and Liu, 2014). Our group has reported a general mechanism of fertility restoration in Arabidopsis (Zhu et al., 2020). Recently, the TGMS line reversible male sterile (rvms), which encodes a GDSL lipase, was cloned (Zhu et al., 2020). Additionally, several pollen wall-related Arabidopsis male-sterile lines were identified as TGMS lines. Their fertility was restored under low-temperature conditions. Analysis of the underlying mechanisms revealed that slow development is a general mechanism for the fertility restoration of these TGMS lines (Zhu et al., 2020). The fertility of these lines can also be restored under short days or low-light conditions. Therefore, slow development was proposed to be a general mechanism for fertility restoration of PGMS lines (Zhang et al., 2020). In this study, the partial male sterility of a heterozygous mutant of MGT6 (MGT6+/−) and a fertile homozygous line (mgt6) were further analyzed. We dissected the complex relationship among environmental factors (light and temperature), Mg transport in the anther, plant growth and plant fertility. The results show that MGT6 plays redundant roles with other MGT members in the transport of Mg for anther development and pollen formation. Slow development is also a general mechanism to restore the fertility of mgt male-sterile lines.
Arabidopsis ecotype Columbia-0 was the source of both the WT and mutant plants. T-DNA insertion mutants of mgt5 (SALK_037061C) and mgt6 (Salk_203866C) were obtained from the ABRC.1 The dyt1, tdf1, ams, myb80, and tek mutants were preserved at the laboratory of Z.N.Y. The genotypes were confirmed by PCR. The plants were grown under long photoperiod (16 h of light/8 h of darkness) or short days (8 h of light/16 h of darkness) at approximately 22°C and 80% relative humidity. The soil was vermiculite and was fertilized with 0.1% chemical fertilizer (consisting of 100 μM Mg; Shanghai Yongtong Chemical Co., Ltd., China).
The hydroponic cultivation of Arabidopsis was performed as described previously (Xu et al., 2015). The seeds were placed on the surface of sponges soaked with plant nitrate solution (PNS). The sponges were placed in a large opaque container that contained 4 L of PNS and that was covered with a transparent lid. The container was then transferred to a growth chamber (AR-41 L2, Percival, China). The light intensity was 100 μmol photons m−2 s−1. The bottom of the sponge was submerged in the PNS to prevent desiccation. After 10 days, healthy seedlings were retained from each sponge. After approximately 20 days, the seedlings were transferred to a larger opaque container. This container contained 5 L of PNS with various concentrations of Mg.
The plants were imaged with a Cybershot T-20 digital camera (Sony, Japan). Silique images were acquired under a dissecting microscope equipped with a DP70 digital camera (Olympus, Japan). Alexander staining solution (Alexander, 1969) was prepared containing 10 ml of ethanol, 1 ml of 1% malachite green in 95% ethanol, 50 ml of distilled H2O, 25 ml of glycerol, 5 g of phenol, 5 g of chloral hydrate, 5 ml of 1% acid fuchsine in H2O, 0.5 ml of 1% orange G in H2O, and 1–4 ml of glacial acetic acid. The anthers were stained overnight at room temperature. The flower buds for semithin sections were prepared and embedded in Spurr’s resin. The semithin sections were prepared at a thickness of 1 μm and incubated in a 0.01% toluidine blue/sodium borate solution for 5 min at 45°C before being washed with H2O. The sections were subsequently observed under bright-field microscopy.
For SEM, fresh pollen grains (PGs) were coated with 8 nm of gold and observed under a JSM-840 microscope (JEOL, Japan). For TEM, the flower buds were fixed in 0.1 M phosphate buffer (pH 7.2) comprising 2.5% glutaraldehyde (v/v) and then washed several times before gradient dehydration. Finally, the samples were embedded in Spurr’s resin and polymerized for 48 h at 60°C. The resulting ultrathin sections were examined via transmission electron microscopy (JEOL, Japan).
The MGT6 genomic region was cloned using primers (CMGT6-F/R) to complement the mgt6 and MGT6+/− mutants. The fragment was amplified using KOD polymerase (Takara Biotechnology, http://www.takarabio.com) and cloned into a pCAMBIA1300 binary vector (Cambia, Australia). The CRISPR/Cas9 system was used for gene editing of MGT6. MGT6-Cas9-F/R primers were used to generate MGT6-gRNA in the backbone vector: pEarlyGate100 which was modified with Arabidopsis YAO promoter-driven Cas9 (Yan et al., 2015). The plasmids were transformed into Agrobacterium tumefaciens GV3101 and screened using 50 mg/ml kanamycin, 40 mg/ml gentamicin, and 50 mg/ml rifampicin. The agrobacteria containing the plasmid constructs were introduced into the plants. Transformed mgt6 and MGT6+/− lines carrying the MGT6 complement fragment were selected using 20 mg/L hygromycin. PCR was performed to verify the backgrounds of the transgenic lines. The primers MGT6ID-F/R, MGT6IDF/CMGT6IDR, and SalkLB1.3/MGT6IDR were used to identify the genotype of the T-DNA insertion. The primer MGT6IDF/1300P2 was used to confirm the insertion of the complement fragment of the MGT6 genome. Transformed WT plants carrying the pYAOCRISPR/Cas9-MGT6-gRNA construct was selected using 0.1% Basta. The editing sites in the transgenic lines were verified by sequencing of the gene specific PCR product. The sequences of the primers used are listed in Supplementary Table S2.
RNA was extracted from the inflorescences of the wild-type (WT) and mutant plants using a TRIzol kit (Invitrogen, United States). Reverse transcription PCR (RT-PCR) was performed for 28 cycles to analyze the expression of MGT6, using the primers rtMGT6-F/R. Quantitative reverse transcription PCR (qRT-PCR) was performed on an ABI PRISM 7300 detection system (Applied Biosystems, USA) in conjunction with SYBR Green I master mix (Toyobo, Japan). The expression level of MGT6 was detected using primers qMGT6-F/R. The qRT-PCR results were shown as the relative expression levels normalized to those of TUBULIN BETA 8 (Tubulin), which served as the positive control (Tub-F/R). The primers used for RT-PCR and qRT-PCR are listed in Supplementary Table S2.
For RNA in situ hybridization, the protocol was performed as described previously (Xu et al., 2015). Here, the flower samples were fixed in FAA solution (RNase free), dehydrated in an ethanol gradient, and embedded in wax. The sections were prepared using a rotary microtome (MR2; RMC, United States). For RNA in situ hybridization, a 108 bp MGT6 probe (inMGT6-F/R) was synthesized with a DIG RNA Labeling Kit (Roche, United States). The sections were incubated with xylene and rehydrated in an ethanol series. Proteinase K (Roche)-treated slides were hybridized with the probe at 55°C overnight. The slides were blocked with 1% blocking reagent (Roche) in a solution consisting of 100 mM Tris (pH 7.5) and 150 mM NaCl. An anti-DIG antibody (Roche) was used for immunological detection, and an NBT/BCIP solution (Roche) was diluted 1:100 in a solution consisting of 100 mM Tris (pH 9.5), 100 mM NaCl, and 50 mM MgCl2 for colorimetric detection at room temperature. The sections were ultimately imaged under an Olympus BX51 microscope.
The construction of Arabidopsis lines carrying promoterMGT6::GUS fusion construct is described in a previously published report (Gebert et al., 2009). Here, flowers were collected and incubated in β-glucuronidase (GUS) staining solution at 37°C for 12 h. The samples were then decolorized in a gradient of ethanol solutions (30, 50, 60, and 70%) or further dehydrated in an ethanol gradient (80, 90, 95, and 100%), after which they were embedded in wax before sectioning. The flower samples were imaged under an Olympus SZX12 microscope. The sections of anther samples were imaged under an Olympus BX51 microscope.
The Mg2+ concentrations in the stamens of Arabidopsis WT, MGT6+/− and mgt6 plants grown in the same pot containing approximately 100 μM Mg2+ were measured. After 6 weeks, the fresh stamens during anther stages 5–9 were collected and weighed (>10 mg). During stage 5–9, the bude diameters ranged from 400 to 650 μm. The anther stages were determined by semi-thin section (Sanders et al., 1999). The stamen samples were first digested with HNO3 and maintained at 98°C for 3 h, and then ultrapure H2O of the same volume was added. The concentration of Mg2+ was measured via inductively coupled plasma optical emission spectrometry (ICP-OES; Vista-MPX, America) at the 285.2 nm wavelength. The Mg2+ content in ultrapure H2O used in this assay was 0.005814 mg/L.
To investigate the role of MGT6 in plant reproduction, a T-DNA insertion line, Salk_203866C, was obtained from the Arabidopsis Biological Resource Center (ABRC). PCR-based analysis and sequencing confirmed that the T-DNA insertion was present in the third exon of the MGT6 gene in this line (Supplementary Figures S1A,B). The leaves of mgt6 are smaller than those of the wild type (WT) under low-Mg conditions, though this phenotype was rescued under high-Mg conditions (Supplementary Figure S2). This is consistent with the findings of previous studies (Mao et al., 2014; Oda et al., 2016; Yan et al., 2018). mgt6 displayed a dwarf phenotype and the same fertility as WT (Figure 1A). Then we obtained heterozygous T-DNA insertion plants (MGT6+/−) from cross between mgt6 and WT. In contrast, the MGT6+/− showed no observable defects in vegetative growth, but abnormal fertility, as indicated by its relatively short siliques (Figure 1B; Supplementary Figure S3). Alexander staining revealed that, like that of the WT, the pollen fertility of mgt6 was normal. However, the majority of PGs were aborted in MGT6+/− anthers (Figures 1C–E). To further confirm the phenotype of MGT6+/−, we generated another heterozygous mutant of MGT6 by the use of the CRISPR/Cas9 system (cas9MGT6+/−). Like MGT6+/−, the cas9MGT6+/− mutant exhibited severe sterility and reduced pollen fertility (Supplementary Figure S3). Statistical analysis showed that there were approximately 400 pollen grains in each WT anther. In each mgt6 anther, the number of pollen grains was similar to that in the WT anther. However, the amount of pollen grains in the anthers from MGT6+/− was only approximately 25% of that in the WT anthers (Figure 1F). Furthermore, the number of seeds was severely decreased in MGT6+/− plants, compared with the WT and mgt6 plants (Figure 1G). These suggest that MGT6+/− affects normal pollen development and plant fertility.
Figure 1. Heterozygous MGT6+/− mutants exhibit severe sterility. (A) Phenotypes of the wild-type (WT) and homozygous mutant of MGT6 (mgt6). (B) Phenotypes of the WT and heterozygous mutant of MGT6 (MGT6+/−). Bars, 1.5 cm. (C–E) Alexander staining of anthers of WT (C), mgt6 (D), and MGT6+/− (D). The mature pollen is purple, but the aborted pollen is green. Bars, 1 mm. (F,G) Number of pollens (F) and seeds (G) in each anther and silique from WT, mgt6, and MGT6+/− plants. The means are shown with ±SDs, n > 30. A two-sample t-test was used to evaluate statistical significance compared with the WT (** p < 0.01).
To complement the mgt6 and MGT6+/− mutant phenotype, the 3.1 kb genomic fragment of the MGT6 gene was cloned from the WT and transferred to mgt6 and MGT6+/− plants. In the T2 generation, 22/22 transgenic lines in the mgt6 background showed normal vegetative growth and fertility. Moreover, 5/5 transgenic lines in the MGT6+/− background showed normal fertility (Supplementary Figures S1C–E). These results demonstrated that the T-DNA insertion in MGT6 affects the normal function of this gene during plant reproduction. RT-PCR indicated that the expression of MGT6 was completely absent in the mgt6 mutant. qRT-PCR showed that the amount of MGT6 transcripts was half as low in the MGT6+/− flowers than in the WT flowers. Furthermore, the expression of MGT6 in the inflorescences was not affected when the plants were grown under different Mg conditions (Supplementary Figure S4). These results suggest that MGT6 is involved in male fertility.
Cytological analyses were employed to understand the defects of anther development in the MGT6+/− plants. Semithin sections revealed that anther development is normal in the MGT6+/− mutant. No significant difference was observed during anther stages 4–8 (Sanders et al., 1999) between MGT6+/− and WT. At later stages, no significant developmental defects were found in the vascular, connective, or tapetal cells. However, most MGT6+/− microspores began degenerating at stage 10 and had aborted at stage 11 (Figure 2A). DAPI staining indicated that meiosis is normal in MGT6+/− plants. However, some of the microspores displayed diffuse staining in MGT6+/− cells at stage 10. At stage 12, normal pollen with two sperm cells and one vegetative cell was formed, but no signal was observed in the aborted pollen in MGT6+/− (Figure 2B). Ultrathin sections revealed that the intine was absent in aborted pollen, while the exine was not affected in the MGT6+/− pollen (Figure 2C). These results show that normal mitosis and intine development are defective in aborted pollen from the MGT6+/− mutant. The developmental defects in the aborted pollen from MGT6+/− are similar to those in pollen from mgt4, mgt5, and mgt9 (Li et al., 2015; Xu et al., 2015). Thus, it is possible that insufficient amounts of Mg lead to pollen abortion in MGT6+/− plants.
Figure 2. Pollen development defects in MGT6+/− mutants and MGT6 expression patterns in anthers. (A) Semithin sections of anthers of WT and MGT6+/− mutants showing pollen development at the anther stages 9–11. Bars, 20 μm. The anther developmental stages refer to previous report (Sanders et al., 1999). (B) DAPI staining indicates male gametophytic mitosis within normal and aborted pollen of MGT6+/− plants. (C) TEM of anthers of MGT6+/− plants. The image shows that the intine development of aborted microspores (MSps) is prevented in the MGT6+/− mutant. Bar, 1 μm. DPG, degenerated pollen grain; DMSp, degenerated microspore; MSp, microspore; and PG, pollen grain. (D–G) GUS expression in inflorescences of transgenic plants harboring the promoterMGT6::GUS construct. The black arrow indicates the expression of GUS in the anthers of young buds (D,E). Paraffin sections of GUS-stained anthers. GUS expression in transverse sections of anthers transformed with promoterMGT6::GUS at stage 5 (F) and stage 7 (G). (H–L) RNA in situ hybridization in which a MGT6 antisense probe was used to detect MGT6 expression in WT anthers at stages 6–8. (K,L) A MGT6 sense probe was used as a control at stage 5 (K) and 7 (L). At least 30 anthers were examined in each stage under the microscope. C, connective cell; V, vascular region; T, tapetum; Tds, tetrads; and MMc, microspore mother cell. Bars, 20 μm.
During anther development, the tapetum secretes Mg into the locules, where microspores take it up for pollen formation. It has been shown that MGT4 and MGT9 mainly function in pollen (Chen et al., 2009; Li et al., 2015), whereas MGT5 functions in both pollen and the tapetum (Xu et al., 2015). To understand whether MGT6 functions in sporophytes and/or gametophytes, a transmissibility assay was performed. WT plants were crossed with MGT6+/− plants. The reciprocal cross experiments revealed that the transmission of MGT6+/+ and MGT6+/− alleles is close to 1:1 in the F1 generation, suggesting that gametophytes with T-DNA insertion have normal fertility (Supplementary Table S1). Furthermore, the MGT6+/+ (normal), MGT6+/− (partial male sterile), and mgt6 (dwarf) plants of population from MGT6+/− segregated with the ratio of 1:2:1 (n > 200). These results indicate that MGT6 functions in sporophytic tissue to supply Mg for microspore development. To address the expression pattern of MGT6 in flowers, we obtained the promoterMGT6::GUS transgenic plants that had been previously generated (Gebert et al., 2009). GUS signals were observed in the anthers of young buds (Figures 2D,E) and were consistently expressed in the receptacle (Supplementary Figure S5). Paraffin sections showed that GUS signals occurred in the vascular region and connective, tapetal and male gametophytic cells during anther stages 5–7 (Figures 2F,G). Furthermore, RNA in situ hybridization assays showed that MGT6 was expressed in male gametophytic cells and surrounding sporophytic cells during anther stages 5–7 (Figures 2H–L), which is consistent with the GUS staining. These results suggest that MGT6 mainly functions in Mg transport in sporophytic tissues of anthers during the early stage. In anther, the tapetum is one of the important sporophytic cells for pollen development. There are five transcription factors, DYT1, TDF1, AMS, MYB80, and TEK are essential for tapetum development and function (Lou et al., 2014). The MGT5 is under the control of DYT1-TDF1-AMS pathway in tapetum (Xu et al., 2015). To understand whether this genetic pathway also regulates MGT6, we analyzed the expression level of MGT6 in these mutants. The qRT-PCR indicated that the expression of MGT6 was not affected in these mutant inflorescences (Supplementary Figure S5). This suggest that the MGT6 may be independent of this pathway in the tapetum.
Our previous results have shown that slow development restores the fertility of P/TGMS lines (Zhang et al., 2020; Zhu et al., 2020). The absence of MGT6 function can result in developmental retardation (Mao et al., 2014). We analyzed the overall developmental status of mgt6 and MGT6+/− mutants under the same growth conditions. The measurement of fresh weight indicated that the growth speed of mgt6 was much slower than that of WT and MGT6+/− (Figure 3A). The MGT6+/− fertility was dramatically reduced compared with that of WT and mgt6 (Figure 1). Previous investigations have shown that low-temperature treatment is effective at the reproductive stage (Zhu et al., 2020). We further analyzed the developmental speed of the flower buds of MGT6+/− and mgt6. The young floral buds were marked, and the time needed to grow from stage 5 to 13 was recorded. Quantitative analysis showed that the flower bud development of mgt6 was prolonged compared with that of WT and MGT6+/− (Figure 3B), indicating that the flower bud development of mgt6 is slower than that of WT and MGT6+/−. Taken together, these results suggest that slow development may contribute to fertility restoration in mgt6.
Figure 3. Flower bud development time and the magnesium (Mg) concentration in the stamen. (A) Fresh weight of WT, MGT6+/−, and mgt6 plants. The plants were grown under long photoperiod and supplied with 100 μM Mg. The error bars indicate SDs and were calculated from three replicates. (B) Quantitative analyses of floral bud development time for WT, MGT6+/−, and mgt6 plants under long photoperiod. Measurement of the development time of buds during anther stages 5 to 13. The means are shown as ±SDs of three biological repeats, n > 30. Bars, 200 μm. A two-sample t-test was used to evaluate statistical significance compared with the MGT6+/− (** p < 0.01). (C,D) Mg concentrations of stamens in the flower buds of the WT, MGT6+/−, and mgt6 plants. Fresh stamens at anther stages 5–9 were isolated from buds (C). The stamens were weighed to determine the Mg concentration via inductively coupled plasma optical emission spectrometry (ICP-OES; D). The means are shown with ±SEs of three technical repeats. A two-sample t-test was used to evaluate statistical significance compared with the MGT6+/− (** p < 0.01).
Magnesium is essential for microspore development and pollen formation (Xu et al., 2015). We further analyzed whether mgt6 plants accumulated sufficient amounts of Mg for pollen formation. MGT6 is expressed in anther tissues at early stages (Figure 2). We isolated fresh stamens from young buds (anther stages 5–9) of WT, MGT6+/−, and mgt6. ICP-OES was employed to measure the Mg concentrations in the fresh stamens (Figure 3C). The ICP-OES analysis results showed that the MGT6+/− stamens contain Mg in amounts similar to those in the WT stamens. However, the mgt6 stamens contained more Mg than the WT and MGT6+/− stamens (Figure 3D). Therefore, these results suggest that slow development facilitates Mg accumulation in pollen, which leads to fertility restoration in mgt6.
Slow development under low temperature and/or short photoperiod restores the fertility of Arabidopsis P/TGMS lines (Zhang et al., 2020; Zhu et al., 2020). Compared with the WT, the mgt6 mutant is slower in buds development and contains high Mg accumulation in anther (Figure 3). We crossed mgt6 with mgt5 to obtained mgt5mgt6 double mutants. mgt5 plants show severe male sterility under low Mg condition (Xu et al., 2015). Like mgt6, the mgt5mgt6 mutant showed overall developmental retardation but normal fertility (Figures 4A–C). Pollen development was restored in the mgt5mgt6 mutant compared with the mgt5 mutant under the same developmental conditions (Figures 4D–G), indicating that pollen development of mgt5 was restored by mgt6. The bud development of the mgt5mgt6 plants was slower than that of the WT and mgt5 plants (Figure 4H). Previous work has shown that MGT5 is important for Mg transport from the tapetum to pollen. The mgt5 pollen can restore fertility under high Mg conditions (Xu et al., 2015). These findings indicate that slow development in mgt6 also facilitates Mg accumulation in mgt5 pollen.
Figure 4. Phenotypes of mgt5 and mgt5mgt6 under long-photoperiod conditions. (A–C) Phenotypes of WT (A), mgt5 (B), and mgt5mgt6 (C) under long-day conditions. The short siliques indicate that mgt5 is sterile, while mgt5mgt6 shows normal fertility. Bars, 1.5 cm. (D–F) Alexander staining of the anthers from WT (D), mgt5 (E), and mgt5mgt6 (F) plants grown under long-photoperiod conditions. Bars, 1 mm. (G) The numbers of pollen in each anther from WT, mgt5, and mgt5mgt6. The means are shown with ±SDs, n > 30. (H) Quantitative analyses of flower bud development time for WT, mgt5, and mgt5mgt6 plants under long-photoperiod conditions. The means are shown as ±SDs of three biological repeats, n > 30. A two-sample t-test was used to evaluate statistical significance compared with the mgt5 (** p < 0.01).
The mgt5 mutant shows severe male sterility, but its fertility was restored under high-Mg conditions (Xu et al., 2015). Fertility restoration of P/TGMS lines under low temperature and/or short photoperiod has been widely applied in two-line rice breeding systems (Chen and Liu, 2014). We analyzed whether these environmental factors also affect the fertility of mgt5. To this end, we grew WT and mgt5 plants under different photoperiod and temperature conditions as reported previously (Zhang et al., 2020; Zhu et al., 2020). The buds development speed of these plants under short-photoperiod or low-temperature conditions was greatly reduced. Interestingly, we found that mgt5 showed normal fertility under short-photoperiod conditions, but not under low-temperature conditions. Alexander staining indicated that the mgt5 pollen formed under short-photoperiod conditions but not under low-temperature conditions (Figures 5A,B; Supplementary Figure S6). The growth speeds of WT and mgt5 plants were greatly reduced under short-photoperiod conditions, and the flower bud development time was greatly prolonged (Figure 5C). These results suggest that a short photoperiod leading to slow bud development can satisfy Mg requirement for pollen development.
Figure 5. Phenotypes of mgt5 under different photoperiod conditions. (A,B) Fertility of mgt5 under short-photoperiod and long-photoperiod conditions in the presence of 100 μM Mg. Alexander staining of the anthers from these plants. (C) Quantitative analyses of flower bud development time for WT and mgt5 under long-photoperiod and short-photoperiod conditions. The means are shown as ±SDs of three biological repeats, n > 30.
Magnesium is essential for vegetative growth and male reproduction (Gebert et al., 2009; Mao et al., 2014; Xu et al., 2015). Mg uptake and transport mainly rely on members of the MGT family in Arabidopsis (Gebert et al., 2009). Several MGT members have been reported to be involved in pollen formation. In this study, we reveal that MGT6 is also involved in Mg transport in the anthers for pollen formation (Figure 1). The defects in pollen intine and mitosis in MGT6+/− (Figure 2) are quite similar to those in mgt5 and mgt9 (Xu et al., 2015). This suggests that the amount of Mg is not sufficient to support pollen formation in MGT6+/−. Many MGT members are expressed in the anthers (Gebert et al., 2009), which are extremely complex reproductive organs in plants (Xu et al., 2019). The sporophytic tissues in anthers must transport sufficient amounts of Mg for pollen formation during plant reproduction. The transport of nutrients to pollen depends on the coordination of different types of sporophytic cells (Scott et al., 2004). MGT5 is expressed in the tapetum and microspores during anther stages 6–7 (Xu et al., 2015). MGT9 expression clearly occurs in the tapetum and microspores during anther stages 7–9 (Chen et al., 2009). Therefore, these two genes play important roles in Mg transport from the tapetum to microspores during anther stages 6–9. Genetic analysis indicated that MGT6 plays a sporophytic role in pollen formation (Supplementary Table S1). In the anthers, MGT6 is expressed in the vascular region, connective cells, tapetum, and microspores during stages 5–7 (Figure 2). These results suggest that MGT6 mainly functions in Mg transport from the filament to the tapetum. We thus propose a model to summarize Mg transport in the anthers: MGT6 is responsible for Mg transport from the filaments to the tapetum; MGT5 is involved in Mg transport from the tapetum to the locule; MGT9 is essential for microspores to obtain Mg from the locules; and finally, MGT4 and MGT5 maintain Mg homeostasis in the pollen (Figure 6A). All MGT family members function in Mg transport, and protein-protein interactions between different members are highly permissive (Schmitz et al., 2013). Both MGT5 and MGT6 are localized in the plasma membrane (Mao et al., 2014; Xu et al., 2015), and they are expressed in tapetum at stages 6–7 (Figure 2; Xu et al., 2015). Hence, MGT6 may interact with MGT5 to facilitate Mg transportation from tapetum to locule so that pollen/microspore can obtain sufficient Mg under different conditions. The mgt6 showed normal pollen fertility, and its stamen contains higher Mg than WT and MGT6+/− (Figures 1, 3), suggesting that higher Mg in stamens can overcome the Mg transportation defects in mgt6 anther. The fertility restoration of mgt5 and mgt6 under different conditions suggests that other MGT members are also involved in Mg transport in the stamen.
Figure 6. Model of Mg transport in anthers and schematic diagram of the mechanism by which slow development restores fertility. (A) Model of the Mg transport pathway in anthers. MGT6 is responsible for Mg transport from the filaments to the tapetum. MGT5 and MGT6 are involved in Mg transport from the tapetum to the locules. MGT9 is essential for pollen absorption of Mg from the locules. Both MGT5 and MGT4 are important for Mg homeostasis in pollen. (B) Schematic diagram of the mechanism by which slow development functions in fertility restoration, modified from our previous report (Zhang et al., 2020). The rvms, acos5-2, cyp703a2, cals5, rpg1, and npu show male sterility and defective pollen development. Temperature and photoperiod are important for the fertility restoration of these T/photoperiod-sensitive genic male sterility (PGMS) lines (Zhang et al., 2020; Zhu et al., 2020). Under restrictive conditions, pollen development is also affected in the mgt5 and MGT6+/− mutants (left). However, Mg accumulation can be restored by slow development in mgt6 and under short-photoperiod conditions in mgt5 (right).
Male sterility and fertility restoration are important not only for understanding plant development and reproduction but also for plant breeding with respect to agriculture (Chen and Liu, 2014). The formation of fine exine pattern plays an important role in determining male fertility (Guan et al., 2008; Chang et al., 2012; Huang et al., 2013; Xiong et al., 2016; Peng et al., 2020; Xue et al., 2020). A recent investigation revealed that rvms and other exine-defect Arabidopsis mutants are TGMS lines, as their fertility could be restored under low temperature; mechanism analysis revealed that slow development under low temperature restores the fertility of these TGMS lines (Zhu et al., 2020). Plants grow slowly under short photoperiod or low light; these conditions also restored the fertility of these TGMS lines (Zhang et al., 2020). Therefore, slow development is a general mechanism for fertility restoration of P/TGMS lines under low temperature and/or short photoperiod. In this work, the slow growth of mgt6 led to fertility, while MGT6+/− remained male sterile (Figure 1), and mgt6 restored the fertility of mgt5 (Figure 4). These results demonstrate that slow development is also a general mechanism to restore the fertility of mgt male-sterile lines (Figure 6B). Both low temperature and/or short photoperiod slow plant development and restore P/TGMS fertility in Arabidopsis (Zhang et al., 2020; Zhu et al., 2020). However, the fertility of mgt5 could be restored only under short photoperiod (Figure 5). We found that low-temperature conditions were unable to restore the fertility of mgt5 plants (Supplementary Figure S6). It has been reported that Mg uptake and transport are sensitive to low temperature (Tanoi et al., 2014). It is likely that Mg accumulation under slow growth of low temperature is not sufficient to support microspore development or pollen formation in mgt5 plants. MGT6+/− cannot restore its fertility under short-photoperiod. We observed that the buds development of MGT6+/− under short-photoperiod was not prolonged (Figure 3; Supplementary Figure S7). This further supports that slow development facilitates Mg accumulation in pollen. Under high Mg conditions, the MGT6+/− plants showed normal growth, but its fertility also remained partial sterile (Supplementary Figure S8). It was reported that MGT6 controls plant Mg homeostasis in both root and shoot tissues (Yan et al., 2018). These suggest that the MGT6+/− plant may not obtain extra Mg under high Mg conditions. In rvms, cals5, and other P/TGMS lines, slow development overcomes cellular defects and produces functional pollen (Zhang et al., 2020; Zhu et al., 2020). Members of the MGT family play a redundant role in Mg transport in plant development. Several other MGT family members have been reported to be expressed in the anthers (Gebert et al., 2009). The mgt6 and mgt5mgt6 plants under normal growth conditions and mgt5 plants under short photoperiod grew slowly and were fertile (Figures 4, 5). Slow development may allow other redundant MGT members to transport sufficient Mg for pollen formation.
The original contributions presented in the study are included in the article/Supplementary Material, further inquiries can be directed to the corresponding author.
Z-NY led the project. Z-NY and X-FX designed the experiments. X-FX, X-XQ, K-QW, Y-HY, Y-YG, BW, XZ, and N-YY performed the experiments. Z-NY, J-RH, and X-FX wrote the manuscript. All authors contributed to the article and approved the submitted version.
This work was supported by grants from the National Natural Science Foundation of China (31900260 and 31930009), the Shanghai Municipal Education Commission (2019-01-07-00-02-E00006), and the Science and Technology Commission of Shanghai Municipality (18DZ2260500 and 17DZ2252700).
The authors declare that the research was conducted in the absence of any commercial or financial relationships that could be construed as a potential conflict of interest.
We thank Prof. Volker Knoop from the University of Bonn (Germany) for providing the pMGT6::GUS seeds.
The Supplementary Material for this article can be found online at: https://www.frontiersin.org/articles/10.3389/fpls.2020.621338/full#supplementary-material
Ador, L., Jaeger, S., Geslain, R., Martin, F., Cavarelli, J., and Eriani, G. (2004). Mutation and evolution of the magnesium-binding site of a class II aminoacyl-tRNA synthetase. Biochemistry 43, 7028–7037. doi: 10.1021/bi049617+
Alexander, M. P. (1969). Differential staining of aborted and nonaborted pollen. Stain. Technol. 44, 117–122. doi: 10.3109/10520296909063335
Apell, H. J., Hitzler, T., and Schreiber, G. (2017). Modulation of the Na, K-ATPase by magnesium ions. Biochemistry 56, 1005–1016. doi: 10.1021/acs.biochem.6b01243
Ariizumi, T., and Toriyama, K. (2011). Genetic regulation of sporopollenin synthesis and pollen exine development. Annu. Rev. Plant Biol. 62, 437–460. doi: 10.1146/annurev-arplant-042809-112312
Chang, H. S., Zhang, C., Chang, Y. H., Zhu, J., Xu, X. F., Shi, Z. H., et al. (2012). No primexine and plasma membrane undulation is essential for primexine deposition and plasma membrane undulation during microsporogenesis in Arabidopsis. Plant Physiol. 158, 264–272. doi: 10.1104/pp.111.184853
Chen, J., Li, L. G., Liu, Z. H., Yuan, Y. J., Guo, L. L., Mao, D. D., et al. (2009). Magnesium transporter AtMGT9 is essential for pollen development in Arabidopsis. Cell Res. 19, 887–898. doi: 10.1038/cr.2009.58
Chen, L., and Liu, Y. G. (2014). Male sterility and fertility restoration in crops. Annu. Rev. Plant Biol. 65, 579–606. doi: 10.1146/annurev-arplant-050213-040119
Dudev, T., and Lim, C. (2013). Importance of metal hydration on the selectivity of Mg2+ versus Ca2+ in magnesium ion channels. J. Am. Chem. Soc. 135, 17200–17208. doi: 10.1021/ja4087769
Gao, Y., and Yang, W. (2016). Capture of a third Mg2+ is essential for catalyzing DNA synthesis. Science 352, 1334–1337. doi: 10.1126/science.aad9633
Gebert, M., Meschenmoser, K., Svidova, S., Weghuber, J., Schweyen, R., Eifler, K., et al. (2009). A root-expressed magnesium transporter of the MRS2/MGT gene family in Arabidopsis thaliana allows for growth in low-Mg2+ environments. Plant Cell 21, 4018–4030. doi: 10.1105/tpc.109.070557
Glasdam, S. M., Glasdam, S., and Peters, G. H. (2016). The importance of magnesium in the human body: a systematic literature review. Adv. Clin. Chem. 73, 169–193. doi: 10.1016/bs.acc.2015.10.002
Guan, Y. F., Huang, X. Y., Zhu, J., Gao, J. F., Zhang, H. X., and Yang, Z. N. (2008). RUPTURED POLLEN GRAIN1, a member of the MtN3/saliva gene family, is crucial for exine pattern formation and cell integrity of microspores in Arabidopsis. Plant Physiol. 147, 852–863. doi: 10.1104/pp.108.118026
Huang, X. Y., Niu, J., Sun, M. X., Zhu, J., Gao, J. F., Yang, J., et al. (2013). CYCLIN-DEPENDENT KINASE G1 is associated with the spliceosome to regulate CALLOSE SYNTHASE5 splicing and pollen wall formation in Arabidopsis. Plant Cell 25, 637–648. doi: 10.1105/tpc.112.107896
Lenz, H., Dombinov, V., Dreistein, J., Reinhard, M. R., Gebert, M., and Knoop, V. (2013). Magnesium deficiency phenotypes upon multiple knockout of Arabidopsis thaliana MRS2 clade B genes can be ameliorated by concomitantly reduced calcium supply. Plant Cell Physiol. 54, 1118–1131. doi: 10.1093/pcp/pct062
Li, J., Huang, Y., Tan, H., Yang, X., Tian, L., Luan, S., et al. (2015). An endoplasmic reticulum magnesium transporter is essential for pollen development in Arabidopsis. Plant Sci. 231, 212–220. doi: 10.1016/j.plantsci.2014.12.008
Li, Z., Lau, C., and Lu, J. (2016). Effect of the concentration difference between magnesium ions and Total Ribonucleotide triphosphates in governing the specificity of T7 RNA polymerase-based rolling circle transcription for quantitative detection. Anal. Chem. 88, 6078–6083. doi: 10.1021/acs.analchem.6b01460
Li, L. G., Sokolov, L. N., Yang, Y. H., Li, D. P., Ting, J., Pandy, G. K., et al. (2008). A mitochondrial magnesium transporter functions in Arabidopsis pollen development. Mol. Plant 1, 675–685. doi: 10.1093/mp/ssn031
Li, L., Tutone, A. F., Drummond, R. S., Gardner, R. C., and Luan, S. (2001). A novel family of magnesium transport genes in Arabidopsis. Plant Cell 13, 2761–2775. doi: 10.1105/tpc.010352
Lou, Y., Xu, X. F., Zhu, J., Gu, J. N., Blackmore, S., and Yang, Z. N. (2014). The tapetal AHL family protein TEK determines nexine formation in the pollen wall. Nat. Commun. 5:3855. doi: 10.1038/ncomms4855
Mao, D., Chen, J., Tian, L., Liu, Z., Yang, L., Tang, R., et al. (2014). Arabidopsis transporter MGT6 mediates magnesium uptake and is required for growth under magnesium limitation. Plant Cell 26, 2234–2248. doi: 10.1105/tpc.114.124628
Oda, K., Kamiya, T., Shikanai, Y., Shigenobu, S., Yamaguchi, K., and Fujiwara, T. (2016). The Arabidopsis Mg transporter, MRS2-4, is essential for Mg homeostasis under both low and high Mg conditions. Plant Cell Physiol. 57, 754–763. doi: 10.1093/pcp/pcv196
Peng, X., Wang, M., Li, Y., Yan, W., Chang, Z., Chen, Z., et al. (2020). Lectin receptor kinase OsLecRK-S.7 is required for pollen development and male fertility. J. Integr. Plant Biol. 62, 1227–1245. doi: 10.1111/jipb.12897
Rissler, H. M., Collakova, E., DellaPenna, D., Whelan, J., and Pogson, B. J. (2002). Chlorophyll biosynthesis. Expression of a second chl I gene of magnesium chelatase in Arabidopsis supports only limited chlorophyll synthesis. Plant Physiol. 128, 770–779. doi: 10.1104/pp.010625
Sanders, P. M., Bui, A. Q., Weterings, K., McIntire, K. N., Hsu, Y. -C., Lee, P. Y., et al. (1999). Anther developmental defects in Arabidopsis thaliana male-sterile mutants. Sex. Plant Reprod. 11, 297–322.
Schmitz, C., Perraud, A. L., Johnson, C. O., Inabe, K., Smith, M. K., Penner, R., et al. (2003). Regulation of vertebrate cellular Mg2+ homeostasis by TRPM7. Cell 114, 191–200. doi: 10.1016/s0092-8674(03)00556-7
Schmitz, J., Tierbach, A., Lenz, H., Meschenmoser, K., and Knoop, V. (2013). Membrane protein interactions between different Arabidopsis thaliana MRS2-type magnesium transporters are highly permissive. Biochim. Biophys. Acta 1828, 2032–2040. doi: 10.1016/j.bbamem.2013.05.019
Schock, I., Gregan, J., Steinhauser, S., Schweyen, R., Brennicke, A., and Knoop, V. (2000). A member of a novel Arabidopsis thaliana gene family of candidate Mg2+ ion transporters complements a yeast mitochondrial group II intron-splicing mutant. Plant J. 24, 489–501. doi: 10.1046/j.1365-313x.2000.00895.x
Scott, R. J., Spielman, M., and Dickinson, H. G. (2004). Stamen structure and function. Plant Cell 16, S46–S60. doi: 10.1105/tpc.017012
Snavely, M. D., Florer, J. B., Miller, C. G., and Maguire, M. E. (1989). Magnesium transport in Salmonella typhimurium: 28 Mg2+ transport by the CorA, MgtA, and MgtB systems. J. Bacteriol. 171, 4761–4766.
Sun, Y., Yang, R., Li, L., and Huang, J. (2017). The magnesium transporter MGT10 is essential for chloroplast development and photosynthesis in Arabidopsis thaliana. Mol. Plant 10, 1584–1587. doi: 10.1016/j.molp.2017.09.017
Tanoi, K., Kobayashi, N. I., Saito, T., Iwata, N., Kamada, R., Iwata, R., et al. (2014). Effects of magnesium deficiency on magnesium uptake activity of rice root, evaluated using 28Mg as a tracer. Plant Soil 384, 69–77. doi: 10.1007/s11104-014-2197-3
Wang, K., Guo, Z. L., Zhou, W. T., Zhang, C., Zhang, Z. Y., Lou, Y., et al. (2018). The regulation of Sporopollenin biosynthesis genes for rapid Pollen Wall formation. Plant Physiol. 178, 283–294. doi: 10.1104/pp.18.00219
Xiong, S. X., Lu, J. Y., Lou, Y., Teng, X. D., Gu, J. N., Zhang, C., et al. (2016). The transcription factors MS188 and AMS form a complex to activate the expression of CYP703A2 for sporopollenin biosynthesis in Arabidopsis thaliana. Plant J. 88, 936–946. doi: 10.1111/tpj.13284
Xu, X. F., Wang, B., Feng, Y. F., Xue, J. S., Qian, X. X., Liu, S. Q., et al. (2019). AUXIN RESPONSE FACTOR17 directly regulates MYB108 for anther dehiscence. Plant Physiol. 181, 645–655. doi: 10.1104/pp.19.00576
Xu, X. F., Wang, B., Lou, Y., Han, W. J., Lu, J. Y., Li, D. D., et al. (2015). Magnesium transporter 5 plays an important role in Mg transport for male gametophyte development in Arabidopsis. Plant J. 84, 925–936. doi: 10.1111/tpj.13054
Xue, J. S., Zhang, B., Zhan, H., Lv, Y. L., Jia, X. L., Wang, T., et al. (2020). Phenylpropanoid derivatives are essential components of Sporopollenin in vascular plants. Mol. Plant 13, 1644–1653. doi: 10.1016/j.molp.2020.08.005
Yan, Y. W., Mao, D. D., Yang, L., Qi, J. L., Zhang, X. X., Tang, Q. L., et al. (2018). Magnesium transporter MGT6 plays an essential role in maintaining magnesium homeostasis and regulating high magnesium tolerance in Arabidopsis. Front. Plant Sci. 9:274. doi: 10.3389/fpls.2018.00274
Yan, L., Wei, S., Wu, Y., Hu, R., Li, H., Yang, W., et al. (2015). High-efficiency genome editing in Arabidopsis using YAO promoter-driven CRISPR/Cas9 system. Mol. Plant 8, 1820–1823. doi: 10.1016/j.molp.2015.10.004
Zhang, C., Xu, T., Ren, M. Y., Zhu, J., Shi, Q. S., Zhang, Y. F., et al. (2020). Slow development restores the fertility of photoperiod-sensitive male-sterile plant lines. Plant Physiol. 184, 923–932. doi: 10.1104/pp.20.00951
Keywords: Mg2+ transporter, male fertility, Arabidopsis, MGT5, MGT6
Citation: Xu X-F, Qian X-X, Wang K-Q, Yu Y-H, Guo Y-Y, Zhao X, Wang B, Yang N-Y, Huang J-R and Yang Z-N (2021) Slowing Development Facilitates Arabidopsis mgt Mutants to Accumulate Enough Magnesium for Pollen Formation and Fertility Restoration. Front. Plant Sci. 11:621338. doi: 10.3389/fpls.2020.621338
Received: 28 October 2020; Accepted: 28 December 2020;
Published: 20 January 2021.
Edited by:
David Smyth, Monash University, AustraliaReviewed by:
Zheng Yuan, Shanghai Jiao Tong University, ChinaCopyright © 2021 Xu, Qian, Wang, Yu, Guo, Zhao, Wang, Yang, Huang and Yang. This is an open-access article distributed under the terms of the Creative Commons Attribution License (CC BY). The use, distribution or reproduction in other forums is permitted, provided the original author(s) and the copyright owner(s) are credited and that the original publication in this journal is cited, in accordance with accepted academic practice. No use, distribution or reproduction is permitted which does not comply with these terms.
*Correspondence: Zhong-Nan Yang, em55YW5nQHNobnUuZWR1LmNu
†These authors have contributed equally to this work
Disclaimer: All claims expressed in this article are solely those of the authors and do not necessarily represent those of their affiliated organizations, or those of the publisher, the editors and the reviewers. Any product that may be evaluated in this article or claim that may be made by its manufacturer is not guaranteed or endorsed by the publisher.
Research integrity at Frontiers
Learn more about the work of our research integrity team to safeguard the quality of each article we publish.