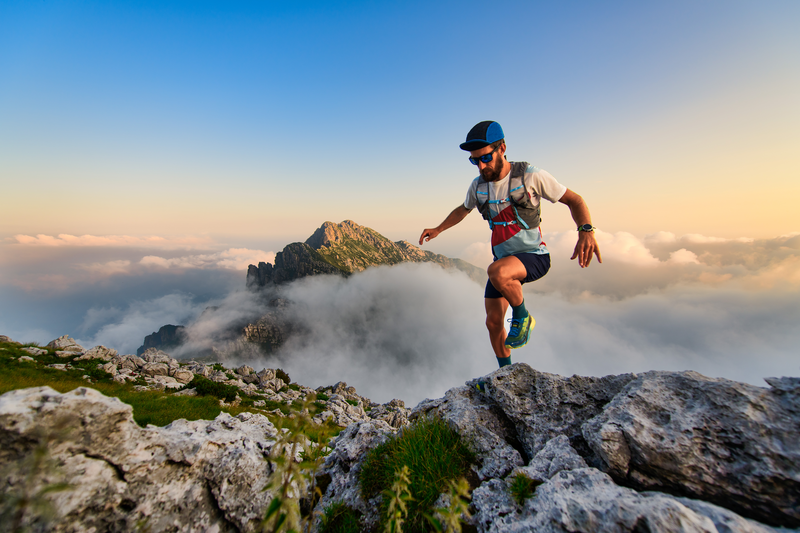
94% of researchers rate our articles as excellent or good
Learn more about the work of our research integrity team to safeguard the quality of each article we publish.
Find out more
ORIGINAL RESEARCH article
Front. Plant Sci. , 11 December 2020
Sec. Plant Abiotic Stress
Volume 11 - 2020 | https://doi.org/10.3389/fpls.2020.614144
This article is part of the Research Topic Identification and Characterization of Contrasting Genotypes/Cultivars to Discover Novel Players in Crop Responses to Abiotic/Biotic Stresses View all 46 articles
The identification of drought-tolerant olive tree genotypes has become an urgent requirement to develop sustainable agriculture in dry lands. However, physiological markers linking drought tolerance with mechanistic effects operating at the cellular level are still lacking, in particular under severe stress, despite the urgent need to develop these tools in the current frame of global change. In this context, 1-year-old olive plants growing in the greenhouse and with a high intra-specific variability (using various genotypes obtained either from cuttings or seeds) were evaluated for drought tolerance under severe stress. Growth, plant water status, net photosynthesis rates, chlorophyll contents and the extent of photo- and antioxidant defenses (including the de-epoxidation state of the xanthophyll cycle, and the contents of carotenoids and vitamin E) were evaluated under well-watered conditions and severe stress (by withholding water for 60 days). Plants were able to continue photosynthesizing under severe stress, even at very low leaf water potential of −4 to −6 MPa. This ability was achieved, at least in part, by the activation of photo- and antioxidant mechanisms, including not only increased xanthophyll cycle de-epoxidation, but also enhanced α-tocopherol contents. “Zarrazi” (obtained from seeds) and “Chemlali” (obtained from cuttings) showed better performance under severe water stress compared to the other genotypes, which was associated to their ability to trigger a higher antioxidant protection. It is concluded that (i) drought tolerance among the various genotypes tested is associated with antioxidant protection in olive trees, (ii) the extent of xanthophyll cycle de-epoxidation is strongly inversely related to photosynthetic rates, and (iii) vitamin E accumulation is sharply induced upon severe chlorophyll degradation.
Olive tree (Olea europaea L.) is one of the most widespread crops in Mediterranean-type agroecosystems, with high economic and social importance. In semi-arid areas of the Mediterranean basin, the scarcity of precipitation combined with extreme climatic conditions such as high temperatures increase water stress in olive trees. It is particularly in this context that serious concerns have been raised about the impact of climate change on olive agricultural productivity. According to De Ollas et al. (2019), the Mediterranean region is expected to witness a decrease in the total amount of precipitation and an increase of the spread and intensity of drought. Among various countries from the Mediterranean basin, Tunisia is considered to be one of countries most exposed to climate change. During the drought episode of 1999–2002, the decrease of olive production reached 90%, which adversely affected farmers income (Gargouri et al., 2012). Considering this situation, much attention has recently been drawn to the valorization and conservation of plant genetic resources to achieve relatively high yields under severe drought. New approaches including the selection of drought-tolerant cultivars and rootstocks have been considered to reduce the effects of water stress. Several researchers have demonstrated that combinations of rootstocks and scions decrease the depressive effects of water stress and increase resistance to drought (Trifilo et al., 2007; Therios, 2009).
Understanding the mechanisms that olive trees have evolved to withstand drought stress is important for selecting drought-tolerant genotypes and improving olive yield and oil quality (Sofo et al., 2004). Under water stress conditions, olive trees regulate leaf water status by morphological, anatomical and physiological adaptations (Guerfel et al., 2009). Indeed, water deficit affects leaf water relations and gas exchange mechanisms of olive cultivars (Trabelsi et al., 2019). Some authors attributed the decrease of photosynthetic rate in response to water stress to stomatal conductance limitation (Perez-Martin et al., 2014; Ben Hamed et al., 2016). Stomatal control is an effective mechanism that decreases water loss through transpiration in drought conditions (Perez-Martin et al., 2014). When environmental stress factors become severe for plants, the mesophyll conductance and biochemical limitations further contribute to the decrease of photosynthetic activity (Flexas and Medrano, 2002).
The limitation of CO2 assimilation in water-stressed olive trees leads to an over-reduction of the photosynthetic electron chain (Sofo et al., 2004). Since chloroplasts cannot dissipate the excess light energy, there is a redirection of photon energy into processes that favor the production of reactive oxygen species (ROS) (Hasanuzzaman et al., 2017). High ROS concentration can seriously disrupt the metabolism of the plant causing enzyme inhibition, protein oxidation and membrane lipid peroxidation, and plants are endowed with antioxidant defense systems to protect the photosynthetic apparatus against oxidative damage (Sircelj et al., 2007). Several researchers have been interested in the enzymatic activity against oxidative stress in olive trees (Sofo et al., 2004; Ben Ahmed et al., 2009, Ben Abdallah et al., 2017), but little is known about the role of non-enzymatic antioxidants on the tolerance of olive trees under drought stress conditions. Among them, carotenoids and tocopherols constitute the main protection system against ROS, including their role in quenching the singlet oxygen (1O2) and in the case of tocopherols additionally preventing lipid peroxidation in thylakoid membranes (Muñoz and Munné-Bosch, 2019). The increase in carotenoids contents is one of the mechanisms evolved by the olive tree to protect the photosynthetic apparatus against photooxidation (Bacelar et al., 2007; Ben Abdallah et al., 2017). However, the identification of particular carotenoids involved in photo- and antioxidant protection under water deficit conditions in olive trees has been poorly investigated to date. The excess excitation energy is harmlessly dissipated in the antennae complexes of PSII as heat through a process which involves the xanthophyll cycle (Demmig-Adams and Adams III, 1996). Likewise, enhanced non-enzymatic antioxidant defense systems have been reported to improve plant abiotic stress tolerance in different studies (Munné-Bosch and Alegre, 2000; Hasanuzzaman et al., 2017). Some non-enzymatic antioxidant mechanisms, such as the increase in the content of phenolic compounds and glutathione, have been described to play a role in olive trees subjected to contrasting water availability regimes (Bacelar et al., 2006, 2007; Petridis et al., 2012), but to our knowledge no studies have been performed at the level of the xanthophyll cycle and α-tocopherol in olive trees, and even less these photo- and antioxidant mechanisms tested for their potential use in genotype selection.
Tunisian olive germplasm is characterized by a large number of varieties with about 200 cultivars. The main olive cultivars in Tunisia are Chemlali in the south and the center of the country and Chetoui in the north. These varieties account for 95% of the total olive trees and contribute more than 90% of the national production of olive oil. Chemlali is well known for its ability to cope with arid conditions (rainfall <200 mm per year). By taking advantage of the variability associated to the genotypic diversity of various young olive trees, we aimed here to unravel the link, if any, between leaf water potential, photosynthesis rates and chlorophyll loss with mechanisms of photo- and antioxidant protection in juvenile olive trees subjected to severe drought. Furthermore, it is discussed how the development of new photo- and antioxidant markers may be useful in order to select and develop new drought-tolerant olive tree cultivars for oil production in semi-arid regions. Due to the difficulty of performing studies in mature olive trees in field trials, we used here trees at an early stage of development. The juvenile phase of trees usually represents the most abiotic stress sensitive stage in woody plants (Shannon et al., 1994); therefore, young genotypes represented here may be considered a proper and valuable material for the detection of drought-tolerant genotypes. Furthermore, another important goal of our study was to establish new photo- and antioxidant markers that may be useful in order to select and develop new drought-tolerant olive tree cultivars in semi-arid regions. Selection methods including field trials testing several genotypes are generally limited, more specifically comparing rooted cutting with seedlings obtained from seeds (Laczko and Aghazarm, 2009). We aimed to fill this knowledge gap by testing the drought stress response of several olive trees at the juvenile stage under semi-controlled conditions to offer a rapid alternative (in comparison to field trials) in genotype selection for crop improvement.
One-year-old olive trees (Olea europaea L.) were grown under natural daylight conditions with a mean maximum daily photosynthetically active radiation (PAR) of 1017 μmol m–2 s–1 in a plastic greenhouse at the Olive Tree Institute of Sfax. Plants were obtained from cuttings and/or from germination of seeds. Rooted cuttings were obtained from the two main Tunisian olive cultivars “Chemlali” and “Chetoui,” while seedlings were regenerated from the seeds of wild olive tree “Oleaster” and from three olive cultivars “Chetoui,” “Chemlali” and “Zarrazi” which are cultivated, respectively, in the north, the center and the south of Tunisia. All seeds were collected from the experimental station “Taous” of the Olive Tree Institute (central Tunisia, 34°56′38″N, 10°36′50″E), except for the wild olive tree “Oleaster,” whose seeds were collected from the National Park of Ichkeul (north Tunisia, 37°10′00″N, 9°40′00″E).
After germination, seedlings were selected for homogeneity and grown for 3 months on an MS medium (Murashigue and Skoog, 1962) supplemented with 8 g⋅L–1 agar and 30 g⋅L–1 sucrose. Both seedlings and rooted cuttings were transplanted in 1-L plastic pots filled with sand and peat (2:1, v/v) and then irrigated twice a week with half-strength Hoagland”s solution (Hoagland and Arnon, 1950) for 8 months. During this period, mean daily maximum and minimum temperatures and relative humidity in the greenhouse ranged from 20 to 30°C, and between 40 and 50%, respectively. Before stress application, soil field capacity was calculated. Indeed, all the plants were saturated with water and left to drain freely until drainage became negligible. After 24 h, the weight recorded for each individual pot (W1) was considered as the soil field capacity. Soil water content was monitored by weighing all the pots every 2 days (W2) and the amount of water transpired was determined and restored by irrigation. The weight difference (W1–W2) allowed a calculation of the amount of water lost by transpiration and used for irrigation. Drought stress was imposed by a gradual reduction of 20% of the total transpired water every 2 days until full irrigation withdrawal. Well-watered (control) plants were irrigated with 100% of soil field capacity throughout the experiment.
Plants were arranged in two completely randomized blocks design. Each block represents a water regime: irrigated and stressed. A total number of 24 seedlings and 3 plants per cultivar were selected for the irrigated water regime. For the contrasting water regime, a total number of 50 seedlings and 8 plants per cultivar were used. Measurements were performed on plants exposed to severe stress after 60 days of starting treatments, including leaf water potential, leaf gas exchange and mechanisms of photo- and antioxidant protection. Growth parameters were estimated both after 30 and 60 days of starting treatments, For biochemical analyses, fully-expanded young leaves exposed to sunlight were sampled between 10 a.m. and 11 a.m. (local time), immediately frozen in liquid nitrogen and stored at −20°C until analyses.
Stem elongation (cm) and the number of leaves per plant were measured for each plant at the beginning of stress (Hi, Li), at 30 days (H30, L30) and at 60 days (H60, L60) of drought. Stem growth rate (SGR) and leaf production rate (LPR) were estimated from these parameters according to the equations described by Sun et al. (2019) and Rodrigues de Lima et al. (2019).
Leaf water potential (ΨL) was measured using a Scholander pressure chamber model SKPM 1400 (Skye Instruments, Powys, United Kingdom) between 9:00 and 11:00 local time. Net photosynthesis rate (An), stomatal conductance (gs), transpiration (E), water use efficiency (A/E) and intercellular to ambient carbon dioxide concentration ratio (Ci/Ca) were determined between 9:00 and 11:00 using a portable gas exchange system (Cl-340 Handheld Photosynthesis System). During leaf gas exchange measurements, photosynthetically-active radiation was set at 1250 μmol⋅m–2⋅s–1, leaf temperature at 35°C, CO2 concentration at 430 ppm and vapor pressure deficit at 5 KPa. All measurements were carried out on fully-expanded young leaves taken from the median part of the shoot. Leaf water potential determination was performed using the whole leaf with the petiole, taking care the latter remained intact during measurements.
The extraction and HPLC analysis of chlorophylls and carotenoids were performed as described by Munné-Bosch and Alegre (2000). In short, leaves were ground in liquid nitrogen and pigments extracted with cold methanol using ultrasonication. After centrifuging at 1000 × g for 10 min at 4°C, the supernatant was collected, and the pellet re-extracted with the same solvent until it turned colorless. Then, supernatants were pooled and filtered with a 0.22-μm pore size. Pigments were separated by high-performance liquid chromatography (HPLC) on a Dupont non-end capped Zorbax ODS-5-μm column (250 mm long, 4.6 mm i.d.; 20% carbon, Teknokroma, Sant Cugat, Spain) at 30°C at a flow rate of 1 ml⋅min–1 for 38 min. The solvent mixture for the gradient, detection at 445 nm (diode array detector, HP1100 Series HPLC System, Agilent Technologies, Santa Clara, CA, United States) and calculation of pmol/are ratios for quantification of chlorophyll a, chlorophyll b, neoxanthin, violaxanthin, antheraxanthin, zeaxanthin, lutein, and β-carotene were performed as described by Munné-Bosch and Alegre (2000). The de-epoxidation state of the xanthophyll cycle (DPS) was calculated as DPS = (Z + 0.5A)/(V + Z + A), where V, A, and Z are violaxanthin, antheraxanthin and zeaxanthin, respectively.
The same methanolic extract was used for the determination of tocopherols, which were separated isocratically on a normal-phase HPLC system (JASCO, Tokyo, Japan) and quantified using a fluorescent detector as described by Amaral et al. (2005). Detection was carried out at an excitation of 295 nm and emission at 330 nm. α-Tocopherol was quantified by co-elution with an authentic standard (Sigma–Aldrich, Steinheim, Germany) and quantified using a calibration curve. From all vitamin E homologs measured (α-, β-, γ-, and δ-tocopherol), only the α-tocopherol form accumulated in olive tree leaves.
Statistical analyses were carried out with the SPSS Base 20.0 software (Chicago, IL, United States). A two-way analysis of variance (ANOVA) was used to examine cultivar and treatment effects. Duncan”s multiple range tests were used for mean comparison at p ≤ 0.05. Correlation between variables was determined according to Pearson tests.
Drought and cultivar effects on growth were observed (Figure 1A). Leaf production rate (LPR) dropped severely in all olive trees exposed to drought stress, with the seedlings “Chemlali” (“Chemlali S”) and “Oleaster” (“Oleaster S”) being the most affected already at 30 days of drought (Figure 1B). Increasing drought stress up to 60 days resulted in a further leaf production decrease with a response that depended on the cultivar, as evidenced by a strong cultivar × water regime interaction (p < 0.001) (Figure 1B).
Figure 1. Stem and leaf growth in olive trees subjected to mild and severe drought stress. Effects of drought stress periods (30 and 60 days after start of treatments) on (A) stem growth and (B) leaf production rate in olive trees obtained from cuttings (cv. “Chemlali” and “Chetoui”) and obtained from seeds (“Chemlali S,” “Chetoui S,” “Zarrazi S” and “Oleaster S”). Values represent means ± S.E. of n ≥ 3 for irrigated plants and n ≥ 8 for water-stressed plants. A two-way analysis of variance (ANOVA) was used to examine if the effects of treatment (water regime) and genotype (cultivar) were statistically significant and to look at the significance of interaction effects. Different letters indicate significant differences between genotypes in each condition using Duncan post hoc tests (p ≤ 0.05).
In irrigated conditions, ΨL showed the lowest values (−1.4 MPa) in both “Chetoui” rooted cuttings and seedlings, which significantly differ to other genotypes except “Chemlali” (Figure 2A). The highest values (−0.8 MPa) were observed for “Zarrazi” seedlings. For the stressed treatment, ΨL decreased significantly (p < 0.001) in all olive trees compared to control plants (Figure 2A). The response to water stress depended on the cultivar as evidenced by cultivar × water regime interaction (p < 0.001). The reduction of ΨL ranged from 71 to 88%.
Figure 2. Water status and leaf gas exchange in olive trees subjected to severe drought stress. (A) Leaf water potential (ΨL), (B) net photosynthesis rate (An), (C) stomatal conductance (gs), (D) transpiration (E), (E) water use efficiency (A/E) and (F) intercellular to ambient carbon dioxide concentration ratio (Ci/Ca) in olive trees obtained from cuttings (cv. “Chemlali” and “Chetoui”) and obtained from seeds (“Chemlali S,” “Chetoui S,” “Zarrazi S” and “Oleaster S”) after 60 days of drought stress. Values represent means ± S.E. of n = 3. A two-way analysis of variance (ANOVA) was used to examine if the effects of treatment (water regime) and genotype (cultivar) were statistically significant and to look at the significance of water regime × cultivar interaction effects. Means having the same letter are not significantly different according to Duncan post hoc test at p ≤ 0.05.
An decreased significantly after 60 days of drought stress in all genotypes, irrespective of whether the plants were obtained from cuttings or seeds (Figure 2B). As compared to the control plants, “Zarrazi” seedlings (“Zarrazi S”), followed by “Chemlali” rooted cuttings, were the less affected by water deficit. For the rest of olive trees, more severe reductions due to water deficit were observed, ranging from 87 to 93% relative to their respective controls.
Except for “Oleaster” seedlings, the high decrease of An coincided with the decrease in gs and E. Stressed olive plants exhibited severe decreases in gs and E, as compared to well-watered plants. However, for the “Oleaster S,” despite the decrease in photosynthetic activity (more than 92%), no significant difference was observed in gs and E between irrigated and stressed plants (Figures 2C,D). Water use efficiency (calculated as A/E) did not show significant differences between irrigated and stressed olive trees, with the exception of “Oleaster S,” which showed a severe reduction in this stress marker. By contrast, “Zarrazi S” was the most efficient in water use (Figure 2E). The intercellular to ambient carbon dioxide concentration ratio (Ci/Ca) kept nearly constant in response to drought with values close to 1 for all genotypes (Figure 2F).
A significant interaction between cultivar and water regime (p = 0.001) was recorded on leaf chlorophyll (Chl a + b) content after 60 days of drought stress (Table 1). Compared to control plants, withholding irrigation induced a significant decrease in Chl a + b, with the exception of “Zarrazi S” and both rooted cuttings “Chemlali” and “Chetoui” in which no significant changes were observed. The highest decrease of Chl a + b was recorded in “Oleaster S” which was about 36.4%. Also, no significant change in terms of chlorophyll a/b ratio (Chl a/b) was observed between irrigated and stressed olive trees, with the exception of “Chemlali S” and “Chetoui” rooted cuttings for which significant decreases of 12.2 and 10.2%, respectively, were obtained (Table 1).
Table 1. Contents of chlorophyll (Chl a + b mg⋅g–1 DW), chlorophyll a/b ratio (Chl a/b), xanthophyll cycle pool per unit of Chl (VZA/Chl mmol⋅mol–1), de-epoxidation state of the xanthophyll cycle (DPS), lutein per Chl ratio (Lut/Chl mmol⋅mol–1), β-carotene per Chl ratio (β-car/Chl mmol⋅mol–1), and α-tocopherol per unit of Chl (α-Toc/Chl mmol⋅mol–1) in olive trees under irrigated and water stress conditions for 60 days.
Drought stress increased significantly the xanthophyll cycle pool expressed per unit of chlorophyll (VZA/Chl) and the de-epoxidation state of the xanthophyll cycle (DPS) in olive trees compared to control plants (p < 0.001). The increase in VZA/Chl ranged in all genotypes between 1.3 and 1.9-fold in stressed plants compared to their respective controls. The highest VZA/Chl ratio was recorded in “Zarrazi S,” followed by “Chemlali” rooted cuttings, whereas the lowest one was obtained for “Oleaster S” (Table 1). An effect of water regime, but not of cultivar, was also observed in DPS (p < 0.001). The stress-related increase in DPS ranged between 1.2 and 1.5-fold relative to controls, respectively, in “Chetoui” seedlings (“Chetoui S”) and “Zarrazi S.” In contrast, the β-carotene per unit of chlorophyll (β-car/Chl) decreased significantly under stress conditions in all olive trees with “Zarrazi S” showing the lowest decrease (27.2%). However, the lutein to Chl ratio (Lut/Chl) increased significantly with stress in “Chemlali S “and remained unchanged in the rest of the olive trees compared to control plants (Table 1).
Under water stress conditions, α-tocopherol per unit of chlorophyll (α-Toc/Chl) increased significantly in stressed olive trees compared to control plants, with the exception of “Chemlali S,” “Oleaster S” and “Chetoui” rooted cuttings, in which no significant changes were observed (Table 1). This increase ranged between 2 and 6-fold relative to controls in “Chemlali” rooted cuttings and “Chetoui S,” respectively.
De-epoxidation state of the xanthophyll cycle and VZA levels were closely related to leaf water potential (Figure 3), photosynthetic activity (Figure 4) and leaf chlorophyll content (Figure 5). In fact, results showed negative correlations between either DPS or VZA with ΨL (r = −0.85 and r = −0.74, respectively), An (r = −0.73 and r = −0.62, respectively), Chl a + b (r = −0.46 and r = −0.43, respectively). It appears that a linear and significant increase of DPS and VZA levels occurred when the ΨL decreased below −4 MPa and An was reduced below 5 μmol CO2⋅m–2⋅ s–1. Moreover, a significant correlation was observed between ΨL and α-Toc/Chl, and between Chl a + b and α-Toc/Chl (r = −0.51 and r = −0.48, respectively, Figures 3–5).
Figure 3. Relationship between leaf water status and photo- and antioxidant protection in olive trees. Relationship between leaf water potential and the de-epoxidation state of the xanthophyll cycle (A), the contents of the xanthophyll cycle pool (B), lutein (C), β-carotene (D), and α-tocopherol (E) per unit of chlorophyll of olive trees after 60 days of treatments. Correlation was determined according to Pearson test. p values and corresponding correlation coefficient (r) are shown.
Figure 4. Relationship between photosynthesis and photo- and antioxidant protection in olive trees. Relationship between net photosynthesis rates and the de-epoxidation state of the xanthophyll cycle (A), the contents of the xanthophyll cycle pool (B), lutein (C), β-carotene (D), and α-tocopherol (E) per unit of chlorophyll of olive trees after 60 days of treatments. Correlation was determined according to Pearson test. p values and corresponding correlation coefficient (r) are shown.
Figure 5. Relationship between chlorophyll loss and photo- and antioxidant protection in olive trees. Relationship between chlorophyll contents and the de-epoxidation state of the xanthophyll cycle (A), the contents of the xanthophyll cycle pool (B), lutein (C), β-carotene (D), and α-tocopherol (E) per unit of chlorophyll of olive trees after 60 days of treatments. Correlation was determined according to Pearson test. p values and corresponding correlation coefficient (r) are shown.
The effects of water stress on growth may be considered the first line of defense, as it has been previously reported on olive trees (Trabelsi et al., 2019). This is essentially due to the inhibition of cell elongation by the interruption of water flow from the xylem to the surrounding cells (Nonami, 1998) and serves to reduce the amount to total water transpired by the plant under drought conditions. In the present study, growth measurements revealed genotypic variability in response to drought stress. Using stem and leaf growth parameters as indicators of tolerance, “Chemlali” rooted cuttings and “Zarrazi S” can be considered the genotypes more tolerant to drought. In contrast, “Chemlali S,” “Oleaster S” and “Chetoui S” can be considered the most susceptible to drought stress because these seedlings showed the highest reduction in LPR. The superior performance of “Zarrazi S” to drought stress could be explained by the genotypic adaptation to the local environment of this variety, which is natural from Medenine - southern Tunisia, in which the average annual rainfall does not exceed 180 mm. In our study, two irrigation treatments and 60 days of drought stress were selected for measurements of leaf water potential, net photosynthesis and photo- and antioxidant protection, which due to the high tolerance of some of the genotypes tested led to an adequate comparison between genotypes under well-watered and severe water deficit conditions. No information is available in the literature on selection methods including photoprotection and chloroplastic antioxidant markers such as carotenoids and tocopherols, and even less comparing olive tree rooted cuttings with seedlings (obtained from seeds). We filled here this knowledge gap by testing the drought stress response of several olive trees at the juvenile stage under semi-controlled conditions to offer a rapid alternative (in comparison to field trials) in genotype selection for crop improvement. Furthermore, found a link between both water potential and net photosynthesis rates with various photo- and antioxidant markers in olive trees subjected to severe water deficit, which helps us better understand the response of a very tolerant xerophyte plant, such as olive trees, to severe drought.
Plant growth decrease is mainly due to the loss of turgor pressure (Nonami, 1998). Indeed, exposure of plants to severe drought stress (60 days without irrigation) decreased significantly the olive trees water status attaining leaf water potential values between −6 and −4 MPa, thus indicating severe water deficit. For olive trees (cv. Koroneiki), 30 days without watering decreased significantly the ΨL to achieve −6.5 MPa (Boussadia et al., 2008). Similarly, for pistachio, Behboudian et al. (1986) revealed very low leaf water potential (−6 MPa), which is not completely unusual for woody plants growing under severe drought conditions. Furthermore, our results indicated that ΨL were differently affected by drought stress depending on the cultivar. Contrary to “Zarrazi S,” “Chetoui S” showed the less reduction rate (71.4%) of ΨL. This difference is probably associated with the different mechanisms of osmotic adjustment adopted by the olive trees under water stress conditions, as previously reported by Dichio et al. (2005). In olive trees, damage caused by drought stress is mainly associated with a decrease of photosynthesis activity. The net photosynthesis rate showed a significant decrease (between 58 and 93%) after a long period of drought stress (60 days). These differences in gas exchange responses between olive trees could be used as a selection factor for olive trees grown under drought conditions (Ben Ahmed et al., 2009; Guerfel et al., 2009). In fact, the lowest decrease of An was recorded in “Zarrazi S” and, in a second degree, in “Chemlali” rooted cuttings, which allows them to withstand water stress more effectively than the other tested olive trees. These results are in accordance with those found by Guerfel et al. (2009), who have indicated a small decrease in An in “Chemlali” classified as a drought-tolerant cultivar. However, “Oleaster S” can be considered the most sensitive to water stress as they showed the highest depressive effect of water stress on An (92.8% reduction compared to control). In susceptibility to water stress, “Oleaster S” was followed by “Chemlali S,” “Chetoui S” and “Chetoui” rooted cuttings with a mean of 88.4% reduction on An compared to its respective control. Moreover, the increase in water use efficiency (calculated here as the A/E ratio) could be considered as a drought tolerance index (Jimenez et al., 2013;, Santana-Vieira et al. 2016). As compared to the rest of olive trees, “Zarrazi S” were able to maintain the highest An value even with a minimum ΨL of about −6.5 MPa and was more efficient in water use under severe drought conditions. Plants with a high A/E ratio may be suitable for cultivation in semi-arid regions (Santana-Vieira et al., 2016). It should be noted that cultivated varieties “Chemlali” and “Chetoui” are at the origin of selected genotypes propagated vegetatively. The “Chemlali” variety is known for its tolerance to drought, hence the predominance of its cultivation in central and southern Tunisia in arid and semi-arid conditions, whereas the “Chetoui” variety with less pronounced tolerance to drought predominates in the north with climatic conditions where rainfalls are more abundant.
It is interesting to note that despite the very low values of −6 MPa achieved, some photosynthetic activity was maintained in some cultivars of olive trees. At leaf water potentials below −4 MPa, net photosynthesis rate ranged between 0.45 and 3.57 μmol CO2⋅m–2⋅s–1. The ability to maintain some photosynthetic activity at very low leaf water potential was reported in other fruity tree species such as pistachio (Behboudian et al., 1986). Indeed, these authors showed that although photosynthesis declined with decreasing leaf water potential, plants continued to photosynthesize until a leaf water potential of as low as −5 MPa was reached which is a typical response of xerophytic plants. Likewise, in the case of olive tree (cv. Koroneiki), Boussadia et al. (2008) recorded a net CO2 assimilation rate of 1 μmol CO2⋅m–2⋅s–1 under severe drought stress in which the leaf water potential dropped to very low values of −6.5 MPa. It appears from the present study that stressed young olive plants maintained some photosynthetic activity at low leaf water potential, which might allow them help surviving the severe water stress conditions. It is noteworthy that conditions imposed to plants during leaf gas exchange measurements were very severe, including high temperatures (35°C) and a high vapor pressure deficit (5 KPa), which explains the low stomatal conductance and photosynthesis rates together with the high Ci/Ca values observed in well-watered plants. Similar stomatal conductance, net photosynthesis rates and Ci/Ca ratios were observed for anti-sense Rubisco transgenic tobacco plants (Von Caemmerer et al., 2004). Leaf gas exchange results obtained in our study suggest that there was a stomatal and non-stomatal limitation of photosynthesis under well-watered conditions, and that both of them increased under severe water deficit, given that not only stomatal conductance and net photosynthesis were reduced but also the Ci/Ca ratio kept constant in water-stressed plants. It is very likely that the severe stomatal closure in severely water-stressed plants did not result in an increased Ci/Ca ratio because Rubisco and non-stomatal limitations of photosynthesis impaired the correct functioning of the Calvin cycle, which might indeed led to a oversaturation of the photosynthetic electron transport and excess excitation energy in chloroplasts. To avoid the potential deleterious effects of oxidative damage induced by water stress, plants have evolved both enzymatic and non-enzymatic antioxidant defense systems. The role of photosynthetic pigments (total carotenoids) and enzymatic antioxidant systems as mechanisms of water stress tolerance in olive trees have been already investigated previously (Sofo et al., 2005, 2008; Bacelar et al., 2006, 2007; Brito et al., 2009; Ben Abdallah et al., 2017; reviewed by Brito et al., 2019). Hence, we focused here on the study of specific photosynthetic pigments with a function in photoprotection (such as individual xanthophylls and carotenes) and other non-enzymatic chloroplastic antioxidants (such as tocopherols) Among those molecules, carotenoids and tocopherols are well known to play an important role in preventing photo-oxidative stress in several species, including other xerophytes such as rosemary plants (Munné-Bosch and Alegre, 2000). Our results showed an increase in the xanthophyll cycle pool (VZA) and the de-epoxidation state of the xanthophyll cycle (DPS) levels in stressed olive trees. The contribution of VZA to olive drought tolerance mechanisms is clearly demonstrated in “Zarrazi S” and “Chemlali” rooted cuttings that accumulated the largest amount of VZA/Chl (399 and 383 mmol⋅mol–1, respectively). The lowest VZA/Chl level (302 mmol⋅mol–1) was recorded in “Oleaster S.” Indeed, water stress is known to induce the activation of the xanthophyll cycle, in which violaxanthin is de-epoxidized to antheraxanthin and then zeaxanthin, enabling excess light energy to be dissipated as heat (Demmig-Adams and Adams III, 1996). The increase observed in DPS and VZA/Chl levels was associated with the decrease in ΨL and An (Figures 3, 4). It appears therefore that the antioxidant defense mechanisms can be efficiently developed even when the ΨL decreased below −4 MPa and An turned less than 5 μmol CO2⋅m–2⋅s–1. Indeed, loss of leaf turgor and photosynthetic limitation might be involved in triggering the activation of photoprotective mechanisms in young olive trees under severe water stress. β-carotene/Chl ratio was significantly decreased under drought conditions. A similar response was reported by Sircelj et al. (1999), who described an increase in the zeaxanthin level at the expense of β-carotene in field-grown apple trees subjected to water stress. Reduced β-carotene associated with increased zeaxanthin contents, as occurred in our study, may be indicative of an enhanced conversion of β-carotene to xanthophyll cycle components for excess energy dissipation, as well as an increased oxidation of β-carotene by singlet oxygen in light harvesting complexes and thylakoid membranes (Burton and Ingold, 1984; D’Alessandro and Havaux, 2019). Furthermore, changes in α-tocopherol content under water stress conditions were also observed. In fact, ΨL decreases paralleled an accumulation of α-Toc/Chl in leaves in all studied cultivars, although significantly only for “Chemlali” rooted cuttings, “Chetoui S” and “Zarrazi S.” Differences observed in α-Toc/Chl level could be related to genotypic differences in antioxidant capacity among olive trees. α-Tocopherol is involved in the elimination of 1O2 (Fahrenholtz et al., 1974; Muñoz and Munné-Bosch, 2019) and among other functions, α-tocopherol is known to inhibit the propagation of lipid peroxidation in thylakoid membranes by reducing the accumulation of lipid peroxyl radicals in chloroplasts (Munné-Bosch and Alegre, 2002; Muñoz and Munné-Bosch, 2019). α-Toc/Chl ratio was also associated with the decrease in Chl a + b, which may be related to the fact that this antioxidant can be synthesized using the phytol recycling pathway from chlorophyll degradation (in the so-called phytol recycling pathway, see Muñoz and Munné-Bosch, 2019). The accumulation of photoprotective molecules together with a reduction in chlorophyll content may be an effective strategy to survive severe drought conditions, as it has been observed in other highly drought-tolerant plants (Kyparissis et al., 2000; Munné-Bosch and Alegre, 2000).
A summary of the differences in the response to severe water stress among olive trees are shown in Figure 6. Our data suggest that “Zarrazi S” may cope better with drought conditions which exhibited a lower decrease in growth and net photosynthesis rates, as well as a higher accumulation of photoprotective molecules. “Chemlali” rooted cuttings showed an acclimation response close to that of “Zarrazi S.” “Oleaster S” can be considered the most sensitive to these water stress conditions as it was the genotype showing the highest depressive effect of water stress on growth and net photosynthesis rates and lower photoprotective capacity. In susceptibility to water stress, “Oleaster S” were followed by “Chemlali S,” “Chetoui S” and “Chetoui” rooted cuttings (Figure 6). To our knowledge, this is the first time in which the activation of photo- and antioxidant protection mechanisms is linked to leaf water status, net photosynthetic rates and chlorophyll loss in olive trees, showing that mechanisms of photo- and antioxidant protection can still be active at very low water potentials in this plant species and that the better adapted genotypes show their photo- and antioxidant protection capacity intact, despite suffering from severe drought. In general, “Chemlali” rooted cuttings were more drought tolerant than “Chemlali S”, and “Chetoui” root cuttings and seedlings did respond similarly, thus indicating that vegetative propagation in these two genotypes, and most particularly in Chemlali, may be effective in large-scale propagation for field cultivation in arid or semi-arid areas. Further research is, however, needed to unravel whether the same occurs in “Zarrazi” genotype, which was the genotype showing the highest drought stress tolerance among all the genotypes tested.
Figure 6. Tolerance to severe water stress of olive genotypes. The color gradation ranges from dark red to dark green to indicate progressively increased stress tolerance. Three levels of susceptibility and three levels of tolerance were given for each of the parameters in red and green, respectively, and the mean of tolerance was then calculated considering all parameters measured after 60 days of treatments. SGR, Stem growth rate; LPR, Leaf production rate; ΨL, Leaf water potential; An, Net photosynthesis rate; gs, stomatal conductance; E, transpiration; A/E, water use efficiency; Ci/Ca, intercellular to air carbon dioxide concentration ratio; Chl a + b, Total chlorophyll; Chl a/b, chlorophyll a/b ratio; VZA/Chl, xanthophyll cycle pool per unit of Chl; DPS, de-epoxidation state of the xanthophyll cycle; Lut/Chl, lutein per Chl ratio; β-car/Chl, β-carotene per Chl ratio; α-Toc/Chl, α-tocopherol per unit of Chl. Genotypes: Rooted cuttings, “Chemlali” and “Chetoui”; Seedlings, “Chemlali S,” “Chetoui S,” “Zarrazi S” and “Oleaster S.”
In conclusion, withholding irrigation for 60 days induced a considerable decrease in growth, leaf water potential and net photosynthesis rates in 1-year-old olive trees. This might lead to the activation of photoprotective mechanisms, including, increases in xanthophyll cycle de-epoxidation, and accumulation of α-tocopherol as chlorophyll loss progressed in severely stressed plants. These increases may contribute to the survival of young olive trees under severe water stress conditions. The study also revealed that “Zarrazi” seedlings may serve as drought-tolerant rootstock which exhibited a lower decrease in growth and net photosynthesis rate, as well as a higher antioxidant activity. “Oleaster,” “Chemlali” and “Chetoui” seedlings could be comparatively considered in this order as more drought-sensitive rootstocks. “Chemlali” rooted cuttings showed the tolerance behavior closer to that of “Zarrazi” seedlings, thus, “Chemlali” rooted cuttings are interesting to create vegetatively propagated drought-tolerant rootstocks and/or cultivars used for breeding programs.
The raw data supporting the conclusions of this article will be made available by the authors, without undue reservation.
SB, AC-R, and SM-B conceived and designed the study. SB, EF, and MM performed the experiments. SB, OE, and SM-B analyzed the data and wrote the manuscript, while other authors performed a critical revision of the manuscript. AC-R, FBA, LF, and SM-B supervised the work. All authors approved the final manuscript.
This research was financially supported by the Tunisian Ministry of Higher Education and Scientific Research and the Tunisian Ministry of Agriculture (grant number L22019204063).
The authors declare that the research was conducted in the absence of any commercial or financial relationships that could be construed as a potential conflict of interest.
We are thankful to Mrs. Zina Ben Salem, Mrs. Hajera Chatti, and Mr. Fouad Labidi for their technical assistance.
Ψ L, leaf water potential; 1O2, singlet oxygen; An, net photosynthesis rate; Chl, chlorophyll; DPS, de-epoxidation state of the xanthophyll cycle; DW, dry weight; LPR, leaf production rate; PSII, photosystem II; ROS, reactive oxygen species; SGR, stem growth rate; VZA, xanthophyll cycle pool.
Amaral, J. S., Casal, S., Torres, D., Seabra, R. M., and Oliveira, B. P. P. (2005). Simultaneous determination of tocopherols and tocotrienols in hazelnuts by a normal phase liquid chromatographic method. Anal. Sci. 21, 1545–1548. doi: 10.2116/analsci.21.1545
Bacelar, E. A., Santos, D. L., Moutinho-Pereira, J. M., Gonçalves, B. C., Ferreira, H. F., and Correia, C. M. (2006). Immediate responses and adaptative strategies of three olive cultivars under contrasting water availability regimes: changes on structure and chemical composition of foliage and oxidative damage. Plant Sci. 170, 596–605. doi: 10.1016/j.plantsci.2005.10.014
Bacelar, E. A., Santos, D. L., Moutinho-Pereira, J. M., Lopes, J. L., Goncalves, B. C., Ferreira, T. C., et al. (2007). Physiological behaviour, oxidative damage and antioxidative protection of olive trees grown under different irrigation regimes. Plant Soil 292:1. doi: 10.1007/s11104-006-9088-1
Behboudian, M. H., Walker, R. R., and Törökfalvy, E. (1986). Effects of water stress and salinity on photosynthesis of pistachio. Sci. Hort. 29, 251–261. doi: 10.1016/0304-4238(86)90068-3
Ben Abdallah, M., Methenni, K., Nouairi, I., Zarrouk, M., and BenYoussef, N. (2017). Drought priming improves subsequent more severe drought in a drought-sensitive cultivar of olive cv. Chétoui. Sci. Hort. 221, 43–52. doi: 10.1016/j.scienta.2017.04.021
Ben Ahmed, Ch, Ben Rouina, B., Sensoy, S., Boukhris, M., and Ben Abdallah, F. (2009). Changes in gas exchange, proline accumulation and antioxidative enzyme activities in three olive cultivars under severe water availability stress. Environ. Exp. Bot. 67, 345–352. doi: 10.1016/j.envexpbot.2009.07.006
Ben Hamed, S., Lefi, E., and Chaieb, M. (2016). Physiological responses of Pistacia vera L. versus Pistacia atlantica Desf. to water stress conditions under arid bioclimate in Tunisia. Sci. Hort. 203, 224–230. doi: 10.1016/j.scienta.2016.03.019
Boussadia, O., Ben Mariem, F., Mechri, B., Boussetta, W., Braham, M., and Ben El Hadj, S. (2008). Response to drought of two olive tree cultivars (cv Koroneki and Meski). Sci. Hort. 116, 388–393. doi: 10.1016/j.scienta.2008.02.016
Brito, C., Dinis, L.-T., Moutinho-Pereira, J., and Correia, C. M. (2019). Drought stress effects and olive tree acclimation under a changing climate. Plants 8:232. doi: 10.3390/plants8070232
Brito, G., Costa, A., Coelho, C., and Santos, C. (2009). Large-scale field acclimatization of Olea maderensis micropropagated plants: morphological and physiological survey. Trees 23, 1019–1031. doi: 10.1007/s00468-009-0344-x
Burton, G. W., and Ingold, K. U. (1984). beta-Carotene: an unusual type of lipid antioxidant. Science 224, 569–573. doi: 10.1126/science.6710156
D’Alessandro, S., and Havaux, M. (2019). Sensing β-carotene oxidation in photosystem II to master plant stress tolerance. New Phytol. 223, 1776–1783. doi: 10.1111/nph.15924
De Ollas, C., Morillón, R., Fotopoulos, V., Puértolas, J., Ollitrault, P., Gómez-Cadenas, A., et al. (2019). Facing climate change: biotechnology of iconic Mediterranean woody crops. Front. Plant Sci. 10:427. doi: 10.3389/fpls.2019.00427
Demmig-Adams, B., and Adams III, W. W. (1996). Xanthophyll cycle and light stress in nature: uniform response to excess direct sunlight among higher plant species. Planta 198, 460–470. doi: 10.1007/BF00620064
Dichio, B., Xiloyannis, C., Sofo, A., and Montanaro, G. (2005). Osmotic regulation in leaves and roots of olive trees during a water deficit and rewatering. Tree Physiol. 26, 179–185. doi: 10.1093/treephys/26.2.179
Fahrenholtz, S. R., Doleiden, F. H., Trozzolo, A. M., and Lamola, A. A. (1974). On the quenching of singlet oxygen by alpha-tocopherol. Photo. Chem. Photobiol. 20, 505–509. doi: 10.1111/j.1751-1097.1974.tb06610.x
Flexas, J., and Medrano, H. (2002). Drought-inhibition of photosynthesis in C3 plants: stomatal and non-stomatal limitations revisited. Ann. Bot. 89, 183–189. doi: 10.1093/aob/mcf027
Gargouri, K., Bentaher, H., and Rhouma, A. (2012). A novel method to assess drought stress of olive tree. Agron. Sustain. Dev. 32, 735–745. doi: 10.1007/s13593-011-0078-1
Guerfel, M., Baccouri, O., Boujnah, D., Chaïbi, W., and Zarrouk, M. (2009). Impacts of water stress on gas exchange, water relations, chlorophyll content and leaf structure in the two main Tunisian olive (Olea europaea L.) cultivars. Sci. Hort. 119, 257–263. doi: 10.1016/j.scienta.2008.08.006
Hasanuzzaman, M., Nahar, K., Hossain, M. S., Al Mahmud, J., Rahman, A., Inafuku, M., et al. (2017). Coordinated actions of glyoxalase and antioxidant defense systems in conferring abiotic stress tolerance in plants. Int. J. Mol. Sci. 8:200. doi: 10.3390/ijms18010200
Hoagland, D. R., and Arnon, D. I. (1950). The Water Culture Method for Growing Plants Without Soil. Berkeley, CA: California Agricultural Experiment Station Circular, 347.
Jimenez, S., Dridi, J., Gutiérrez, D., Moret, D., Irigoyen, J. J., Moreno, M. A., et al. (2013). Physiological, biochemical and molecular responses in four Prunus rootstocks submitted to drought stress. Tree Physiol. 33, 1061–1075. doi: 10.1093/treephys/tpt074
Kyparissis, A., Drilias, P., and Manetas, Y. (2000). Seasonal fluctuations in photoprotective (xanthophyll cycle) and photoselective (chlorophylls) capacity in eight Mediterranean plant species belonging to two different growth forms. Aust. J. Plant Physiol. 27, 265–272. doi: 10.1071/PP99037
Laczko, F., and Aghazarm, C. (2009). Migration, Environment and Climate Change: Assessing the Evidence. Grand-Saconnex: International Organization for Migration.
Munné-Bosch, S., and Alegre, L. (2000). Changes in carotenoids, tocopherols and diterpenes during drought and recovery, and the biological significance of chlorophyll loss in Rosmarinus officinalis plants. Planta 210, 925–931. doi: 10.1007/s004250050699
Munné-Bosch, S., and Alegre, L. (2002). Interplay between ascorbic acid and lipophilic antioxidant defences in chloroplasts of water-stressed Arabidopsis plants. FEBS Lett. 524, 145–148. doi: 10.1016/S0014-5793(02)03041-7
Muñoz, P., and Munné-Bosch, S. (2019). Vitamin E in plants: biosynthesis, transport, and function. Trends Plant Sci. 24, 1040–1051. doi: 10.1016/j.tplants.2019.08.006
Murashigue, T., and Skoog, F. (1962). A revised medium for rapid growth and bioassays with tobacco tissue cultures. Physiol. Plant 15, 473–479. doi: 10.1111/j.1399-3054.1962.tb08052.x
Nonami, H. (1998). Plant water relations and control of cell elongation at low water potentials. J. Plant Res. 111, 373–382. doi: 10.1007/BF02507801
Perez-Martin, A., Michelazzo, C., Torres-Ruiz, J. M., Flexas, J., Fernández, J. E., Sebastiani, L., et al. (2014). Regulation of photosynthesis and stomatal and mesophyll conductance under water stress and recovery in olive trees: correlation with gene expression of carbonic anhydrase and aquaporins. J. Exp. Bot. 65, 3143–3156. doi: 10.1093/jxb/eru160
Petridis, A., Therios, I., Samouris, G., Koundouras, S., and Giannakoula, A. (2012). Effect of water deficit on leaf phenolic composition, gas exchange, oxidative damage and antioxidant activity of four Greek olive (Olea europaea L.) cultivars. Plant Physiol. Biochem. 60, 1–11. doi: 10.1016/j.plaphy.2012.07.014
Rodrigues de Lima, K., Brandão de Carvalho, C. A., Vidal Azevedo, F. H., de Campos, F. P., et al. (2019). Morphogenesis and forage accumulation of Urochloa ruziziensis under nitrogen and potassium fertilization management. Semina Ciências Agrárias 40, 1605–1618. doi: 10.5433/1679-0359.2019v40n4p1605
Santana-Vieira, D. D. S., Freschi, L., Almeida, L. A., Moraes, D. H., Neves, D. M., Santos, L. M., et al. (2016). Survival strategies of citrus rootstocks subjected to drought. Sci. Rep. 6:38775. doi: 10.1038/srep38775
Shannon, M. C., Grieve, C. M., and Francois, L. E. (1994). “Whole-plant response to salinity,” in Plant–Environment Interactions, ed. R. E. Wilkinson (New York, NY: Marcel Dekker), 199–244.
Sircelj, H., Batic, F., and Stampar, F. (1999). Effects of drought stress on pigment, ascorbic acid and free amino acids content in leaves of two apple tree cultivars. Phyton 39, 97–100.
Sircelj, H., Tausz, M., Grill, D., and Batic, F. (2007). Detecting different levels of drought stress in apple trees (Malus domestica Borkh.) with selected biochemical and physiological parameters. Sci. Hort. 113, 362–369. doi: 10.1016/j.scienta.2007.04.012
Sofo, A., Dichio, B., Xiloyannis, C., and Masia, A. (2004). Lipoxygenase activity and proline accumulation in leaves and roots of olive trees in response to drought stress. Physiol. Plant 121, 58–65. doi: 10.1111/j.0031-9317.2004.00294.x
Sofo, A., Dichio, B., Xiloyannis, C., and Masia, A. (2005). Antioxidant defences in olive trees during drought stress: changes in activity of some antioxidant enzymes. Func. Plant Biol. 32, 45–53. doi: 10.1071/FP04003
Sofo, A., Manfreda, S., Fiorentino, M., and Dichio, B. (2008). The olive tree: a paradigm for drought tolerance in Mediterranean climates. Hydrol. Earth Syst. Sci. 12, 293–301. doi: 10.5194/hess-12-293-2008
Sun, J., Wang, M., Lyu, M., Niklas, K. J., Zhong, Q., Li, M., et al. (2019). Stem and leaf growth rates define the leaf size vs. number trade-off. AoB Plants 11:plz063. doi: 10.1093/aobpla/plz063
Therios, I. N. (2009). Olives, Crop Production Science in Horticulture 18. Wallingford: CABI Publishing.
Trabelsi, L., Gargouri, K., Ben Hassena, A., Mbadra, C., Ghrab, M., Ncubec, B., et al. (2019). Impact of drought and salinity on olive water status and physiological performance in an arid climate. Agric. Water Manag. 213, 749–759. doi: 10.1016/j.agwat.2018.11.025
Trifilo, P., Lo Gullo, M. A., Nardini, A., Pernice, F., and Salleo, S. (2007). Rootstock effects on xylem conduit dimensions and vulnerability to cavitation of Olea europaea L. Trees 21, 549–556. doi: 10.1007/s00468-007-0148-9
Keywords: drought tolerance, olive germplasm, photoprotection, α-tocopherol, xanthophyll cycle
Citation: Baccari S, Elloumi O, Chaari-Rkhis A, Fenollosa E, Morales M, Drira N, Ben Abdallah F, Fki L and Munné-Bosch S (2020) Linking Leaf Water Potential, Photosynthesis and Chlorophyll Loss With Mechanisms of Photo- and Antioxidant Protection in Juvenile Olive Trees Subjected to Severe Drought. Front. Plant Sci. 11:614144. doi: 10.3389/fpls.2020.614144
Received: 05 October 2020; Accepted: 23 November 2020;
Published: 11 December 2020.
Edited by:
Felipe Klein Ricachenevsky, Federal University of Rio Grande, BrazilReviewed by:
José Manuel Moutinho-Pereira, University of Trás-os-Montes and Alto Douro, PortugalCopyright © 2020 Baccari, Elloumi, Chaari-Rkhis, Fenollosa, Morales, Drira, Ben Abdallah, Fki and Munné-Bosch. This is an open-access article distributed under the terms of the Creative Commons Attribution License (CC BY). The use, distribution or reproduction in other forums is permitted, provided the original author(s) and the copyright owner(s) are credited and that the original publication in this journal is cited, in accordance with accepted academic practice. No use, distribution or reproduction is permitted which does not comply with these terms.
*Correspondence: Sergi Munné-Bosch, c211bm5lQHViLmVkdQ==
Disclaimer: All claims expressed in this article are solely those of the authors and do not necessarily represent those of their affiliated organizations, or those of the publisher, the editors and the reviewers. Any product that may be evaluated in this article or claim that may be made by its manufacturer is not guaranteed or endorsed by the publisher.
Research integrity at Frontiers
Learn more about the work of our research integrity team to safeguard the quality of each article we publish.