- 1Division of Agricultural Chemicals, ICAR-Indian Agricultural Research Institute, New Delhi, India
- 2Division of Nematology, ICAR-Indian Agricultural Research Institute, New Delhi, India
- 3Division of Design of Experiments, ICAR-Indian Agricultural Statistical Research Institute, New Delhi, India
Nematicidal potential of essential oils (EOs) has been widely reported. Terpenoids present in most of the essential oils have been reported responsible for their bioactivity though very less is known about their modes of action. In the present study, an in vitro screening of nine Eos, namely, Citrus sinensis (OEO), Myrtus communis (MTEO), Eucalyptus citriodora (CEO), Melaleuca alternifolia (TEO), Acorus calamus (AEO), Commiphora myrrha (MREO), Cymbopogon nardus (CNEO), Artemisia absinthium (WEO), and Pogostemon cablin (PEO) against Meloidogyne incognita revealed OEO, CNEO, and TEO as most effective with LC50 39.37, 43.22, and 76.28 μg ml–1 respectively. EOs had varying compositions of mono- and sesquiterpenes determined by gas chromatography-mass spectrometry (GC-MS) analysis. The in silico molecular interactions screening of major EO constituents and the seven selected target proteins of the nematode indicated highest binding affinity of geraniol-ODR1 (odorant response gene 1) complex (ΔG = -36.9 kcal mol–1), due to extensive H-bonding, hydrophobic and π-alkyl interactions. The relative binding affinity followed the order: geraniol-ODR1 > β-terpineol-ODR1 > citronellal-ODR1 > l-limonene-ODR1 > γ-terpinene-ODR1. Taken together, the cumulative in vitro and computational bioefficacy analysis related to the chemoprofiles of EOs provides useful leads on harnessing the potential of EOs as bionematicides. The insight on biochemical ligand–target protein interactions described in the present work will be helpful in logical selection of biomolecules and essential oils for development of practically viable bionematicidal products.
Introduction
Root knot nematodes, pose a major challenge to the global pest management programs due to devastating crop losses caused by these organisms (Sidhu et al., 2017). Among the root knot nematodes, Meloidogyne incognita is most abundant in tropical soils, barely sparing any crop family, and the most challenging part is to control its population below economic damage levels (Collange et al., 2011; de Freitas Silva et al., 2020). Synthetic recommended nematicides like carbofuran, fluopyram owing to their associated detrimental effects on the environment, non-target organisms besides phytotoxicity, necessitate safer approaches for nematode management in cropping systems (Westphal, 2011; Jones et al., 2017). To develop ecofriendly alternatives, a wide spectrum of plant metabolites with nematostatic and nematicidal actions has extensively been reported (Atolani and Fabiyi, 2020).
Phytochemicals have been extensively reported as potential sources of bioactive ingredients for the development of natural nematicides (Oka, 2001). Long-chain hydrocarbons, sulfur compounds, alkenes, furans, acetogenins, phenolics, saponins, etc., have been reported to be effective against various phytoparasitic nematodes (Aissani et al., 2018). Most of the phytochemicals have served as models for the identification of a lead molecule with potential commercial applications (Cantrell et al., 2012). Volatile organic compounds of botanical origin, most commonly found in essential oils, have particularly been recognized as highly effective against M. incognita (Aissani et al., 2015; Silva et al., 2018; Pedroso et al., 2019).
Plant essential oils (EOs) are complex mixtures of terpenoids and their oxygenated derivatives, produced by isoprenoid pathways (Tetali, 2019). Only ∼10% of the reported plant species produce EOs (Kalemba and Kunicka, 2003). Stored in secretory glands in epidermic cells, secretory hair, glandular trichomes, EOs play a key role in plant defense against biotic stresses (Bakkali et al., 2008). Known for their bioactive potential against diverse agriculturally important pests, EOs in numerous reports have been mentioned as very effective against M. incognita (Caboni et al., 2013). EOs of Acorus calamus and Pogostemon cablin have been tested for nematicidal activities (Perrett and Whitfield, 1995; Lee et al., 2009). EO from Eucalyptus citriodora was found highly toxic to M. incognita at 500 μl ml–1 by Pandey et al. (2000). Citrus sinensis EO was reported effective against the phytonematodes, M. incognita, Pratylenchus vulnus, and Xiphinema index (Avato et al., 2017). Similarly, EO of Myrtus communis was reported to kill 100% of M. incognita juveniles at 4,000 μl ml–1 concentration (Ardakani et al., 2013). Another study reported that EO of Melaleuca alternifolia was highly active against the larvae of Anisakis simplex at a concentration of 7 μl mL–1 (Andrés et al., 2012). The EO of Cymbopogon nardus tested against M. incognita exhibited moderate effectiveness in a study reported by Sinha et al. (2006). Similarly, the toxicity of Artemisia absinthium EO has been documented against M. incognita on the tomato plant (Amora et al., 2017). Promising nematicidal action of sesquiterpenes rich in the EO of Commiphora myrrha against juveniles of M. incognita was reported by Kong et al. (2006) and Ardakani et al. (2013).
A review of literature clearly showed that EO bioactivity evaluation against nematodes largely remained restricted so far to the evaluation of EOs against different plant parasitic nematodes. Emphasis on the correlation of their anti-nemic activity with chemical compositions and mechanism of interaction at molecular level with the possible target sites of action has remained lacking. Therefore, the present study was performed to characterize the chemical composition of the selected EOs, evaluate their bio-efficacy in vitro against M. incognita, and subject the most effective EOs to in silico analysis for a likely mode of action, using molecular docking and modeling approach.
Materials and Methods
Essential Oils
Commercially available EOs (99% purity) of different plants, namely, OEO (Citrus sinensis (L.) Osbeck; family Rutaceae, orange essential oil), MTEO (Myrtus communis L.; family Myrtaceae, myrtle essential oil), CEO (Eucalyptus citriodora L.; family Myrtaceae, citriodora essential oil), TEO (Melaleuca alternifolia L.; family Myrtaceae, tea tree oil), AEO (Acorus calamus L.; family Acoraceae, calamus essential oil), MREO [Commiphora myrrha (Nees) Engl; family Burseraceae, myrrh essential oil], CNEO (Cymbopogon nardus L. Rendle.; family Poaceae, citronella oil), WEO (Artemisia absinthium L.; family Asteraceae, wormwood essential oil), and PEO [Pogostemon cablin (Blanco) Benth.; family Lamiaceae, patchouli essential oil] were purchased from CDH Fine Chemicals (New Delhi, India) and Merck®, (New Delhi, India) and used without further purification.
Chemicals and Reagents
All the solvents used were of AR grade, purchased from Merck®, (New Delhi, India). Surfactants, Atlas G5002 and Triton X-100 were procured from Croda India Company Pvt. Ltd. (Navi Mumbai, India) and Loba Chemie Pvt., Ltd. (Mumbai, India), respectively. For GC-MS analysis, helium (He) gas of high purity (99%) was used.
Gas Chromatography-Mass Spectrometry Analysis
Volatile constituents of EOs were analyzed in GC-MS in a 7890A GC instrument (Agilent Technologies®, United States) equipped with an HP-5MS column (30 m × 0.25 μm;/0.25 μm, Agilent Co., United States) as stationary phase, which was directly connected to a triple axis HED-EM 5975C mass spectrometer (Agilent Co., United States). The injection volume was 1 μl with flow mode in split control. Helium was used as carrier gas at a head pressure of 10 psi, and flow was set at 1 ml min–1. The GC-MS condition was programmed with the oven temperature initially held at 40°C for 1 min, thereafter increased with a gradient of 3°C min–1, until the temperature reached to 120°C and held constant for 2 min. The temperature was raised again with a gradient of 5°C min–1 up to 220°C and held constant for 1 min and finally raised to 280°C with an increment of 4°C min–1. The total run time of the analysis was 65 min. The MS acquisition parameters were ion source temperature 180°C, electron ionization 70 eV, full scan mode (50–550 AMU), transfer line temperature 280°C, solvent delay 3 min, and E.M voltage 1,380 V. Compounds were identified by matching their mass spectra and fragmentation pattern using NIST (National Institute of Standards and Technologies) Mass Spectra Library. Further rentention indices (RI) have been calculated following Kovats (1978):
RI = 100 ∗ n + [log(RTcompound - v) - log(RT - v)]/[log(RTlarger alkane - v) - log(RTsmaller alkane - v)]
where n = the number of C in the smaller alkane, RTcompound = the retention time of the compound, v = the column void time, RTlarger alkane = the retention time of the larger alkane, and RTsmaller alkane = the retention time of the smaller alkane.
Nematicidal Assay
Collection of Nematodes
Nematode culture was maintained on infected tomato plants (var. Pusa Ruby) under greenhouse conditions. Second instar juveniles (J2s) of M. incognita were collected from roots of 21-day-old infected tomato seedlings. Nematode-infested soil was screened through water screening method following Cobb’s sieving and decanting technique (Cobb, 1918). Further, nematode egg masses were picked up from the sterilized infected roots of tomato seedlings, transferred to fresh distilled water in Petri plates, and allowed to hatch under ambient condition of 27 ± 1°C for 5 days. The hatched nematode juveniles travel through soft wet tissue placed on the wire of the Petri plate on the surface water (Julio et al., 2017). Nematode J2s suspensions were combined and counted under light microscope.
Preparation of Essential Oil Emulsions
Primary stock emulsions (10,000 μg ml–1) of all EOs except PEO were prepared using Atlas G5002 surfactant (2% w/w). In the case of PEO, Triton X-100 (2% w/w) was used. Each primary stock emulsion was diluted serially with surfactant solution to prepare secondary test emulsions of varying strengths (1,000–10 μg ml–1).
Nematicidal Activity
Nematicidal assay was conducted under in vitro condition to assess the activity of the EOs against M. incognita following a known method with slight modifications (Kundu et al., 2016). Treatments comprised of nine Eos, namely, OEO, MTEO, CEO, TEO, AEO, MREO, CNEO, WEO, and PEO. Aqueous suspension (1 μl) containing 25 J2s of M. incognita was added to each well of multiwell plates (15.6-mm diameter), each containing EO emulsion (2 ml) of a particular test strength (1,000–10 μg ml–1). Surfactant solutions used to dissolve EOs were taken as corresponding negative controls. Each treatment was replicated thrice. Multiwell plates were incubated at 27 ± 1°C and examined using a stereoscopic microscope at 24, 48, and 72-h intervals. The numbers of dead vs alive juveniles in each treatment was recorded. Motionless nematodes with straight bodies were counted. The revival test was done as described by Choi et al. (2007). Briefly, the motionless nematodes were teased with a needle followed by transfer to fresh wells containing deionized water. One drop of sodium hydroxide (1M) solution was added to check any movement. Mortality (%) and corrected mortality (%) of J2s was calculated considering the mortality of juveniles in negative control.
Molecular Docking and Simulation
Based on the results of in vitro nematicidal assay and GC-MS analysis, major volatile constituents of OEO, CNEO, and TEO were selected out of nine EOs, for in silico ligand target protein interaction analysis.
Selection of Protein
Seven target proteins, namely, cytochrome c oxidase subunit 1, AChE, Hsp90, ODR1, ODR3, neuropeptide GPCR, CLAVATA3/ESR (CLE)-related protein of M. incognita were selected as target receptors for the molecular docking studies. Cytochrome c oxidase subunit 1 is involved in the oxidative phosphorylation pathway, which is part of the energy metabolism. AChE regulates synaptic transmission and locomotion processes. The full functional activity of Hsp90 is gained in coordination with other co-chaperones, playing an important role in the folding of newly synthesized proteins, stabilization and refolding of denatured proteins during stress. ODR1 and ODR3 regulate chemosensory functions. Neuropeptide GPCR is associated in the regulation of movement of the parasite toward (or within) its host. CLAVATA3/ESR (CLE)-related protein plays an important role in the differentiation or division of feeding cells (syncytia) induced in plant roots during infection. (Ref. for each).
Protein Preparation
The hypothetical protein sequences were taken from the NCBI and UNIPROT database (Table 1). The BLAST servers1 ,2 were used to search and annotate the molecular and biological functions of the query sequences. The NCBI Blast tool and the PDB database were together used to identify the templates for modeling the secondary structures of the query sequences. Further homology modeling of the proteins was carried out using Modeller v 9.24.
Ligand and Receptor Preparation
The molecular structures of the chemical constituents, referred hereafter as “ligands,” of OEO, CNEO, and TEO were downloaded as.sdf file from PUBCHEM database3. The ligand structures were minimized using MM2 forcefield in Chem Draw Ultra 11.0 software [Cambridge Soft Corp., Cambridge, MA, United States (2009)] and used for molecular modeling studies. The ligand molecules were customized for docking using the Dock prep tool of Autodock Vina. Hydrogen molecules were added, and the incomplete side chains were replaced using Dunbrack rotamer library (Dunbrack, 2006). Charges were computed using ANTECHAMBER. AMBER ff14SB and Gasteiger charges were allotted to standard residues and to other residue types, respectively. Similarly, receptor molecules were prepared using the same tools except that the ANTECHAMBER was not employed. All the prepared ligand files were saved in the Mol2 format and the receptor files in the.pdb format.
Molecular Docking Simulation
The customized ligand and receptor molecules were used for docking in ICM Molsoft v. 2.8.ICM software, which performed adaptable ligand docking through global optimization of the energy function (Abagyan et al., 1994). The energy functions incorporated the internal energy of the ligand in view of the ECEPP/3 drive field, and van der Waals, hydrogen-holding, electrostatic and hydrophobic ligand/receptor association terms pre-ascertained on the lattice for computational proficiency (Bursulaya et al., 2003). Flexible ligand docking with the ICM software used Monte Carlo simulations to globally optimize a set of ligand internal coordinates in the space of grid potential maps calculated for the protein pocket (Neves et al., 2012). Discovery Studio v. 4.1 Client was used to study the docked receptor–ligand interactions. The most favored docking conformation interactions of ODR1 with geraniol, β-terpineol, citronellal, l-limonene, and γ-terpinene were analyzed on the basis of docking score, binding affinity, and interacting residues. The active site residues were identified, and depictions of all possible interactions in 3D and 2D poses were prepared using DS Visualiser v. 4.1.
In order to avoid affinity-based selection and optimization of larger ligands, the emphasis was given to compounds that most effectively utilized their atoms. In an attempt to measure the compound effectiveness, Hopkins et al. (2014) suggested an estimation of binding affinity of molecule, in terms of ligand efficiency (LE):
where, ΔG is free-binding energy and HA is the number of ligand non-hydrogen atoms. LE is related to the amount and effectiveness of heavy atoms in a molecule toward complex formation. The average affinity contribution per atom was taken into consideration instead of considering the affinity of the whole compound. This enabled measuring the affinity of the corrected molecules with their size. In drug discovery modules, candidate molecules with LE values ≥ 0.3 kcal per mole per heavy atom usually are taken ahead as lead molecule (Hopkins et al., 2014).
Statistical Analysis
The bioassay experiments were done in triplicate. The significance of the differences between variables was tested using one-way ANOVA. The means were compared using Duncan’s multiple range test. Statistical significance was determined at p < 0.05. Percent mortality data were subjected to probit analysis using Polo Plus software to determine lethal concentrations (LC50 and LC90, expressed in μg ml–1).
Results
Essential Oil Composition
The compositions of EOs of OEO, MTEO, CEO, TEO, AEO, MREO, CNEO, WEO, and PEO were determined by comparing their mass spectra with data library, corresponding retention indices, and mass fragmentation patterns. The identified chemical constituents of the oils are listed in Table 2. The aromatic profile of most of the EOs showed dominance of one or two major constituents. Individually, l-limonene (93.2 ± 2.30%) was found to be most abundant in OEO, along with β-myrcene (2.2 ± 0.2%), α-pinene (1.6 ± 0.1%), sabinene (0.5 ± 0.1%), limonene oxide (0.2 ± 0.0%), and decanal (0.2 ± 0.0%). l-Limonene was confirmed based on its fragmentation pattern with characteristic daughter ion peaks of m/z 136.2, 108.2, and 71.2, generated due to sequential loss of methyl and ethyl moieties (Figure 1). Interestingly, OEO was found to contain only monoterpenes. The monoterpenic constituents of MTEO were identified as α-pinene (42.3 ± 1.1%), 1,8-cineol (30.3 ± 1.3%), linalool (7.6 ± 0.9%), and linalyl acetate (6.6 ± 0.5%). GC-MS analysis of TEO showed several peaks corresponding to 27 mono and sesquiterpenoids, comprising 93.3% of the total oil. Monoterpenes (43.5%) and their oxygenated derivatives (42.6%) were found to be the most abundant. Among monoterpenes, β-terpineol (35.7 ± 1.2%) was identified as the major constituent followed by γ-terpinene (17.5 ± 1.1%), α-terpinene (8.7 ± 0.6%), p-cymene (4.8 ± 0.2%), α-pinene (3.2 ± 0.2%), p-menth-8-en-2-ol (3.2 ± 0.5%), and 1,8-cineol (3.1 ± 0.4%). Besides, α-gurjunene (2.1 ± 0.1%) and δ-cadinene (1.6 ± 0.2%) were identified as the major sesquiterpenes.
Analysis of volatiles of CEO and CNEO revealed the presence of various terpenes, representing 93.5% and 95.8% of the total oil composition, respectively. These oils were characterized by the presence of predominant acyclic monoterpene aldehyde and citronellal with its respective contents of 81.9 ± 1.1% and 31.5 ± 1,1%, in two oils. Both CEO and CNEO showed higher content of oxygenated compounds, in which the former attributed an appreciably higher content primarily of 91.3% oxygenated monoterpenes. Similarly, CNEO mainly contained oxygenated terpenoids (81.9%) and hydrocarbons (13.9%). Except citronellal, other constituents of CEO were citronellol (5.8 ± 0.7%) and fenchone (2.3 ± 0.3%), while CNEO contained geraniol (30.6 ± 1.3%), citronellol (9.6 ± 0.3%), l-limonene (5.7 ± 0.5%), β-elemene (3.3 ± 0.1%), and neryl acetate (2.1 ± 0.2%).
GC-MS analysis of AEO showed identification of 16 mono and sesquiterpenes, accounting for 98.2% of the total oil. Oxygenated terpenes were the major constituents (94.1%) of the oil with the β-asarone being the highest contributor (85.4 ± 1.1%). Methyl isoeugenol (3.1 ± 0.2%), caryophyllene (2.4 ± 0.3%), α-asarone (1.9 ± 0.3%), spathulenol (1.7 ± 0.1%), caryophyllene oxide (1.4 ± 0.1%), and γ-muurolene (1.2 ± 0.2%) were also detected. Sesquiterpene content was found relatively higher in AEO (3.7%), whereas monoterpene content was meager (0.4%). Volatile composition of MREO showed abundance of furanoeudesm (41.9%) and curcerene (23.9%), considered as marker components of MREO. Besides these, other sesquiterpenoids such as β-elemene (4.8%), δ-elemene (2.1%), germacrene D (1.2%), elemol acetate (1.2%), and β-cudesmol (1.1%) were also identified.
Total ion chromatogram (TIC) of WEO in GC-MS analysis exhibited characteristic peaks corresponding to 29 mono- and sesquiterpenes, contributing 97.7% of the oil. Oxygenated terpenoids (69.7%) formed the major share of the composition; borneol acetate (26.6 ± 1.7%) and β-terpineol (16.2 ± 0.9%) being the most dominant ones. 1,8-Cineol (10.6 ± 0.5%), linalool (6.4 ± 0.4%), sabinene (5.6 ± 0.3%), o-cymene (5.1 ± 0.2%), camphor (3.8 ± 0.5%), and α-pinene (2.7 ± 0.2%) were other important terpenes identified in WEO. Sesquiterpenoids detected in WEO were β-caryophyllene (8.2 ± 1.0%), farnesol (3.0 ± 0.2%), and δ-elemene (2.0 ± 0.3%). Volatile composition of PEO showed various peaks in TIC of GC-MS, representing 15 constituents contributing 97.1% of the oil. Sesquiterpene constituents were highly abundant. Among these, α-guaiene (40.6 ± 1.3%) was the major compound followed by α-bulnesene (14.6 ± 0.9%), α-patchoulene (10.7 ± 0.5%), patchoulol (6.7 ± 0.3%), and β-patchoulene (5.8 ± 0.5%). A comprehensive profile of the chemical composition of the EOs, number of identified compounds, and their group-wise classification is presented in Table 3, which described the number of compounds identified along with their content based on functional groups.
Nematicidal Activity of Essential Oils
All the test EOs immobilized more than 50% of juveniles of M. incognita at different test concentrations. Antinemic activity of the EOs is depicted in Table 4. CNEO exhibited LC50 of 325.41, 87.27, and 43.22 μg ml–1 concentration after 24, 48, and 72-h exposure, respectively. However, CEO containing a high amount of citronellal showed moderate activity with an LC50 of 124.50 μg ml–1 after 72 h. OEO rich in l-limonene was found to exhibit a comparatively higher nematode toxicity with a lethal concentration LC50 of 353.20 μg ml–1 within 24 h of J2 exposure. The nematicidal activity of OEO enhanced with the exposure time, and the highest activity was recorded at an LC50 of 79.35 and 39.37 μg ml–1 after 48 and 72 h, respectively (Table 4).
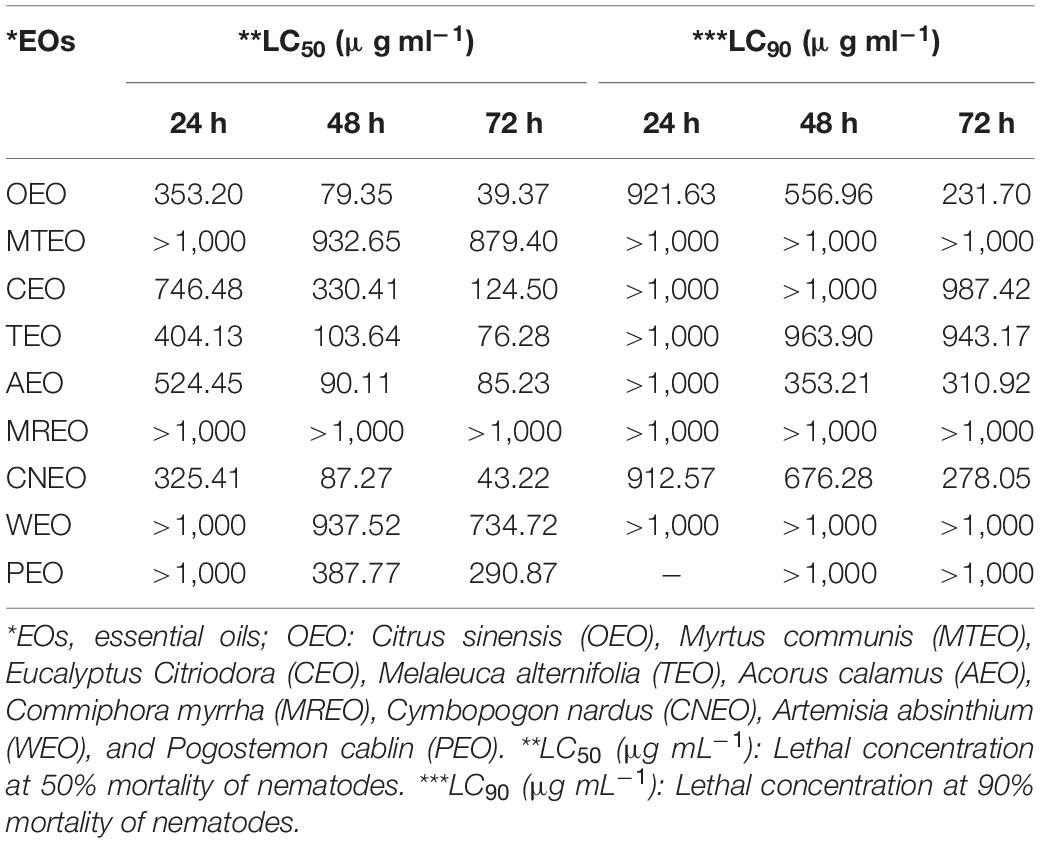
Table 4. LC50 and LC90 values (μg ml–1) of EOs against M. incognita, calculated for three exposure periods in test solutions.
In this study, TEO and AEO were found effective with an LC50 of 76.28 and 85.23 μg ml–1 within 72 h, whereas CEO exhibited an LC50 of 124.50 μg ml–1. MTEO, however, exerted moderate action with an LC50 of 879.40 μg ml–1. The first three Eos, i.e., OEO, CNEO, and TEO, with an LC50 (72 h) below 50 μg ml–1 except TEO, were subjected to molecular docking analysis, to understand their possible interaction with proteins for nematicidal action.
Molecular Docking Study
Seven receptor proteins (putative target proteins) of M. incognita were screened against the biomolecules of OEO, CNEO, and TEO, the three most effective EOs in the present study. Gibb’s free energy of binding and other docking parameters of the screened targets are presented in Table 5. Bioactivity of OEO, TEO, and CNEO against M. incognita J2s was best explained by the in silico inhibition of the odorant response gene 1 (ODR1). The binding pocket of the ODR1 allosteric site is composed of 45 amino acid residues. Screening of the compounds present in the three EOs against the ODR1 gave significantly low binding free energy values ranging from -36.9 to 15.3 kcal mol–1, suggestive of formation of stable protein–ligand complexes.
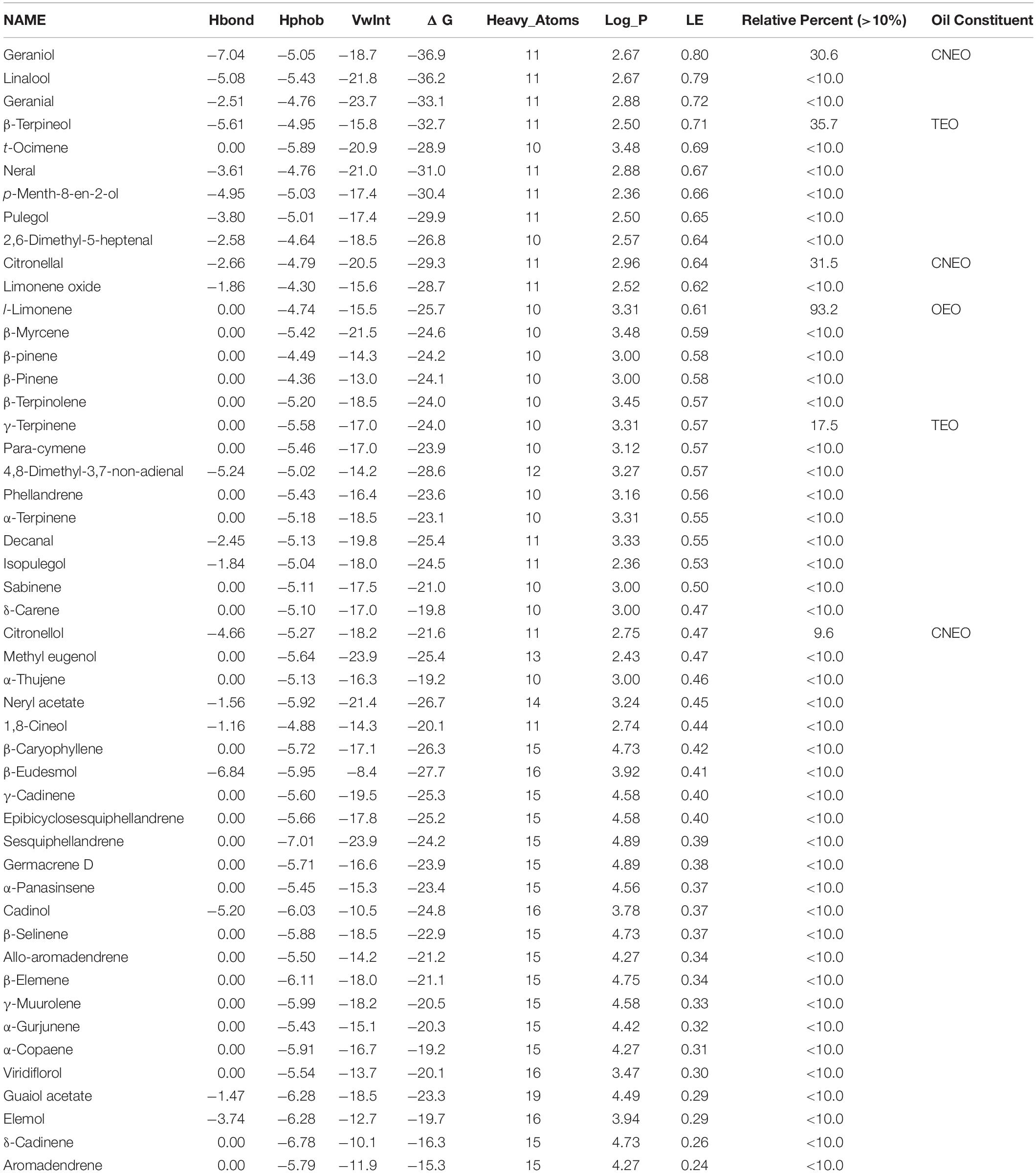
Table 5. In silico nematicidal activity of OEO, TEO, and CNEO oil constituents against M. incognita ODR1.
The relative stability of the docked complexes of 49 ligands (major compounds, >10% present in the OEO, TEO, and CNEO oils) with the ODR1 was computed in terms of ligand efficiency (Table 5). It can be seen that the lowest binding energy value for the geraniol–ODR1 complex (-36.9 kcal mol–1), as depicted in Table 6 may be attributed to the three conventional H-bonds with relatively shorter bond distances (∼2 Å). Additionally, it appeared that the three hydrophobic interactions of π-alkyl type led to further stabilization of the geraniol–ODR1 complex (Table 7). Geraniol was bound specifically to the guanylate cyclase catalytic domain of the ODR1 receptor (Figure 2).
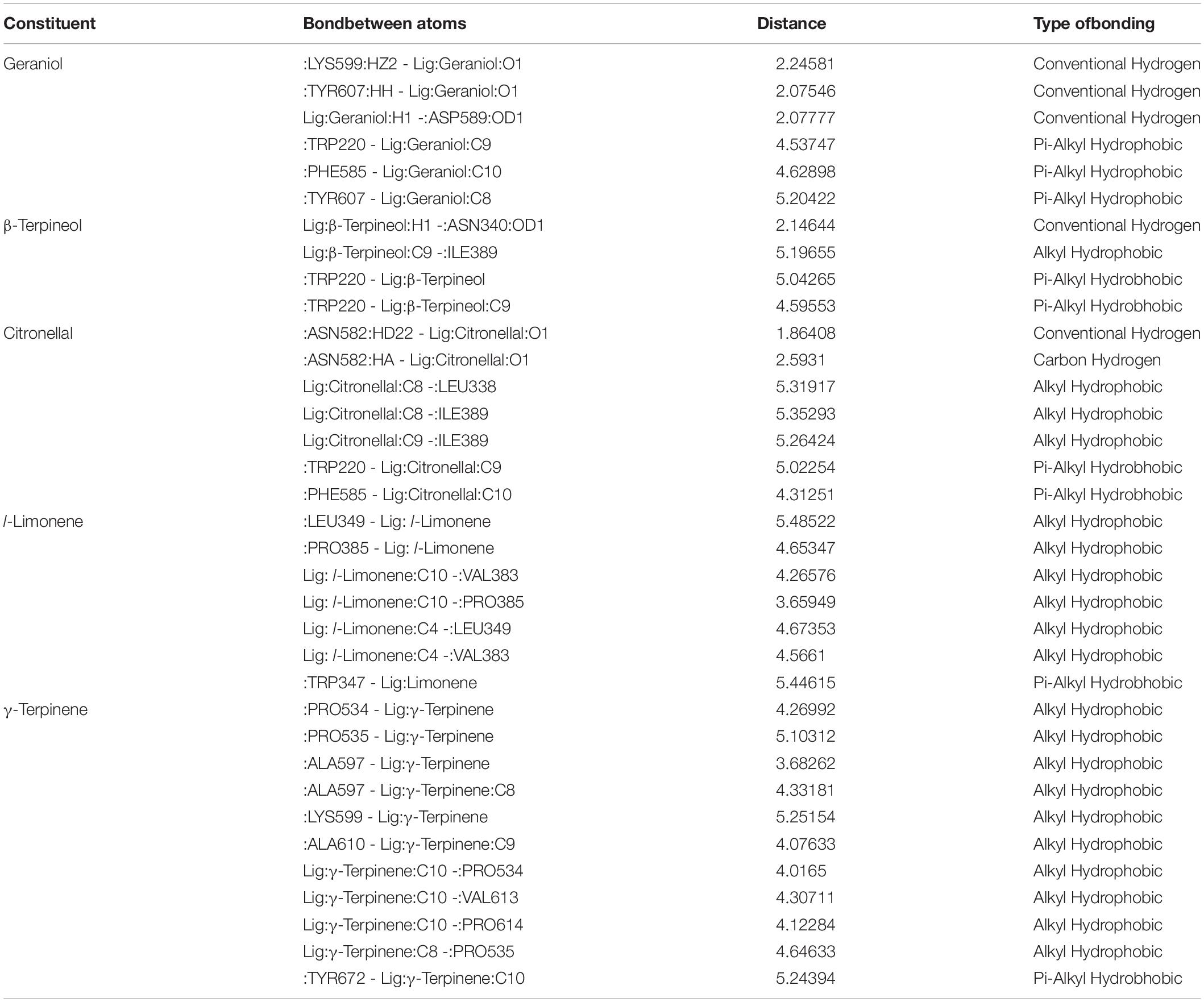
Table 7. Molecular interaction details of flexible ligand docking of major oil constituents (the top 5) with the ODR1 receptor.
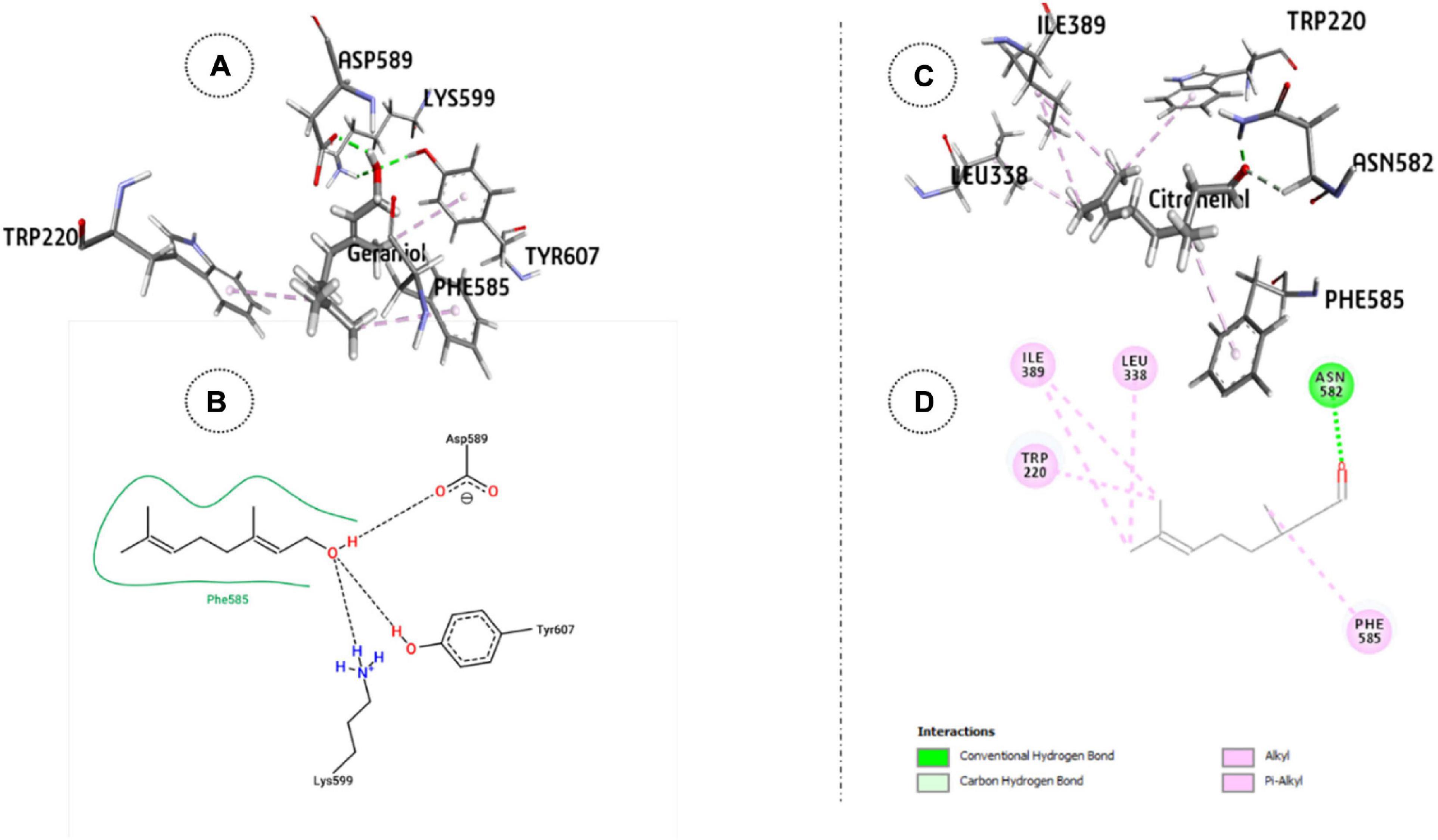
Figure 2. ODR1 bound major CNEO oil constituents: (A) 3-D representation of geraniol. (B) 2-D representation of geraniol. (C) 3-D representation of citronellal and (D) citronellal.
The citronellal–ODR1 complex with two H bonds (one conventional and one C–H type) and five hydrophobic bonds (three alkyl and two π-alkyl types) (Figure 2), exhibited ΔG-29.3 kcal mol–1. In this case, the amino acid residues responsible for the ligand binding interactions belonged to both guanylate cyclase and tyrosine/serine/threonine kinase catalytic domains.
The β-terpineol–ODR1 complex, emerged as the next strongest one with -32.7 kcal mol–1 binding energy. One conventional H bond and three hydrophobic bonds (one alkyl type and two π-alkyl types) attributed to complex formation (Figure 3). Here, the specific binding site was tyrosine and serine/threonine kinase catalytic domain.
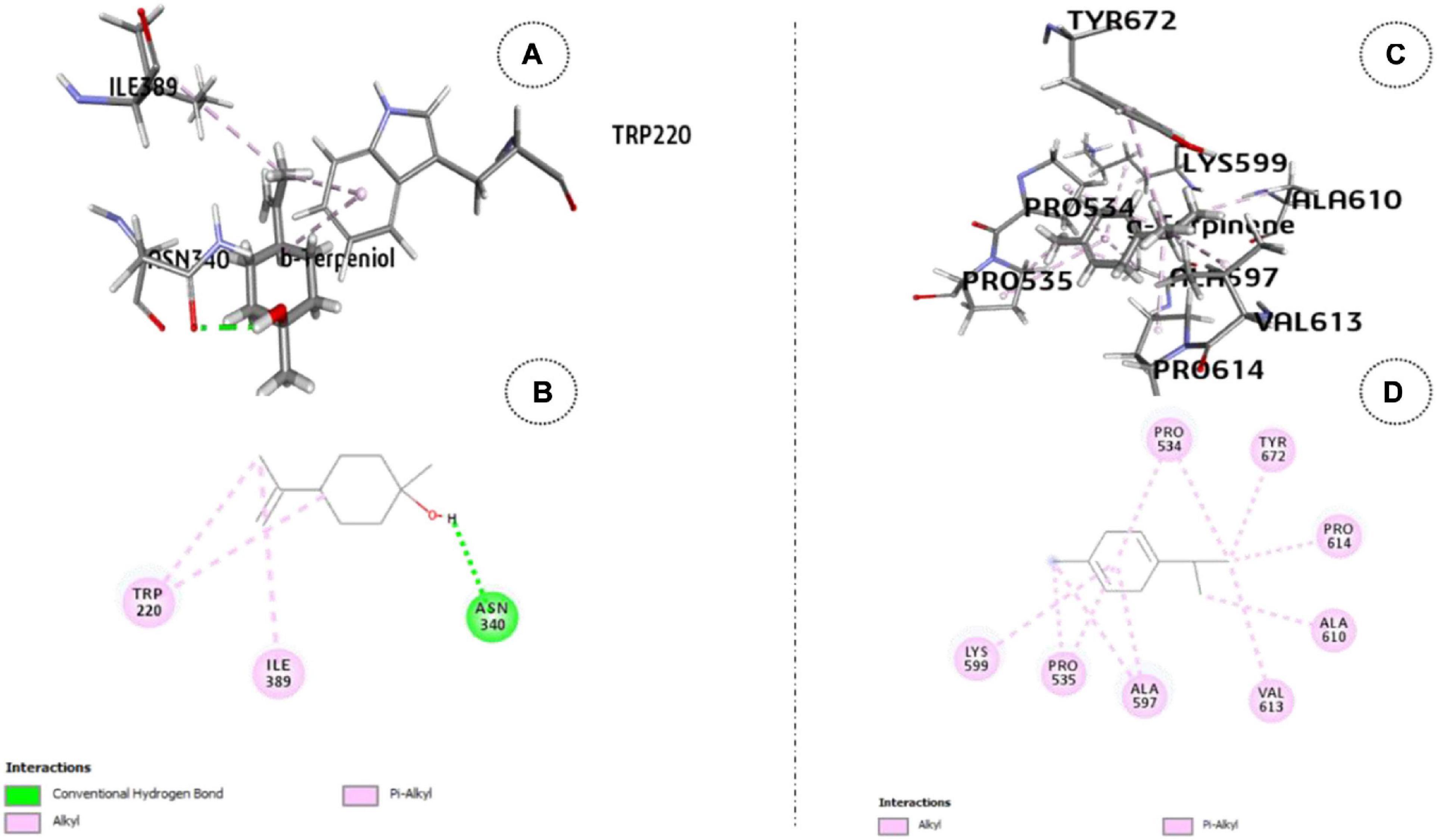
Figure 3. ODR1 bound major TEO oil constituents: (A) 3-D representation of β-terpineol. (B) 2-D representation of β-terpineol. (C) 3-D representation of γ-terpinene and (D) 2-D representation of γ-terpinene.
l-Limonene, the major constituent (93.2% w/w) in OEO showed significant inhibition of the ODR1 gene (ΔG = -25.7 kcal mol–1). The l-limonene–ODR1 complex exhibited seven hydrophobic interactions (six alkyl and one π-alkyl type) in between the protein and the ligand (Figure 4). Apparently, it was bound to the tyrosine/serine/threonine kinase catalytic domain.
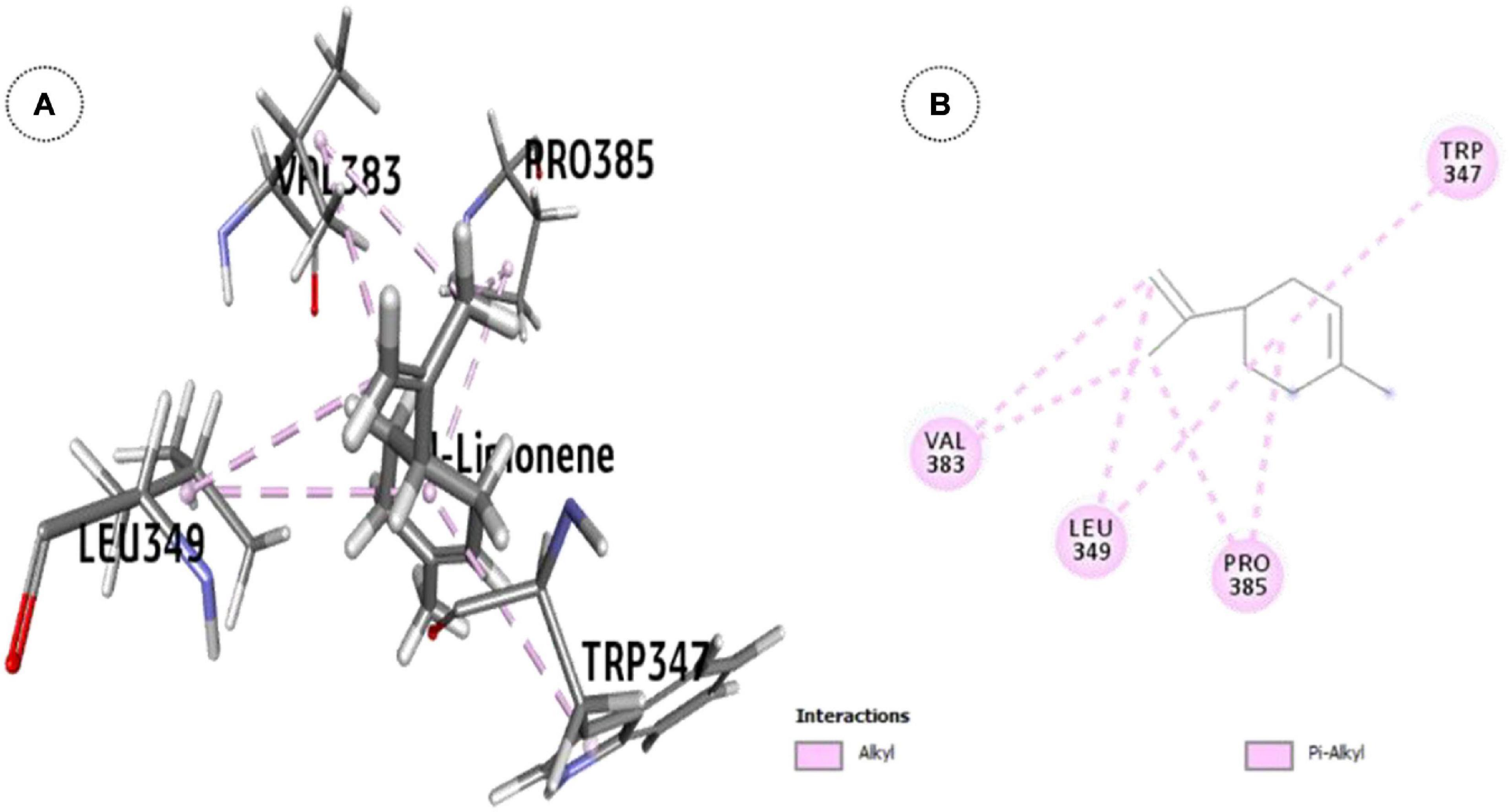
Figure 4. ODR1 bound major OEO oil constituent: (A) 3-D representation of l-limonene and (B) 2-D representation of l-limonene.
The next major EO constituent showing significantly low free energy of binding was γ-terpinene (ΔG = −24 kcal mol–1, 17.5% in TEO). The γ-terpinene–ODR1 complex showed 10 hydrophobic interactions (nine alkyl and one π-alkyl type). The γ-terpinene molecule bound to the HNOBA and guanylate cyclase catalytic domains.
Based on the observed relative ΔG values of ligand receptor complexes, l-limonene ranked fourth (geraniol–ODR1 complex > β-terpineol–ODR1 complex > citronellal–ODR1 complex > l-limonene–ODR1 complex > γ-terpinene–ODR1 complex). Inspite of this, the highest observed in vitro nematicidal activity of the OEO oil with l-limonene could be possible due to the exceptionally high content of l-limonene (93.7%). The major constituents in other active EOs was up to about 36% only (Table 6).
In order to compare the efficiency of smaller ligands with larger ligands in a non-biased manner, ligand efficiency (LE) calculated for the 49 phytochemical constituents of the three oils varied in the range of 0.8–0.24 kcal mol–1 HA–1. Ninety-one percent of the compounds had an LE above the threshold value of 0.3 kcal mol–1 HA–1, establishing the discovery of natural leads targeting the ODR1 gene in M. incognita. This is the first report on the quantitative binding affinity of the EO constituents toward the ODR1 gene of the root-knot nematode, M. incognita, to the best of our information.
Discussion
In the present study, we performed comprehensive chemo-profiling of EOs in order to understand their possible interactions with the target sites of M. incognita. The previously investigated reports on OEO suggested the most prominent monoterpene, l-limonene, with a range of 32–98% (Zhang et al., 2019; Matuka et al., 2020). Dejam and Farahmand (2017) described MTEO as primarily composed of monoterpenes such as 1,8-cineol, α-pinene, and linalool, which was further confirmed in our study. However, Tunisian MTEO have been reported to be rich in α-pinene (Jamoussi et al., 2005). In our study, α-pinene has been found to be a major component of MTEO. Bioactive terpenic compositions of CEO make it worthy to study on volatile constituents for diverse biological properties. Contrastingly, the oil contains a high amount of citronellal, citronellol, and isopulegol (Singh et al., 2012; Siddique et al., 2013). An earlier report by Madalosso et al. (2017) and Raymond et al. (2017) described the volatile composition of TEO rich in terpinenes, terpinen-4-ol, and methyl eugenol. Our analysis too revealed that TEO comprised of β-terpineol and terpinene. Methyl eugenol, however, was not detected. Our findings on AEO predominantly containing β-asarone have been corroborated by Deepalakshmi et al. (2016). The present study suggested that industrially important MREO, CNEO, WEO, and PEO contained a higher amount of furanoeudesm 1,3 diene, geraniol, myrcene, camphor, and patchoulol, respectively, as reported previously (Buré and Sellier, 2004; Nguyen et al., 2018; Kalaiselvi et al., 2019). Reported variation in chemical profiles of these EOs could be attributed to the plant sources related to locational, seasonal, and climatic factors.
Plant EOs have been described as having great potential in nematode control (Andrés et al., 2012). Oxygenated monoterpenes particularly aldehydes and alcohols have particularly been found effective against M. incognita (Echeverrigaray et al., 2010). A similar trend in activity was demonstrated in the case of CNEO comprising an abundance of citronellal and geraniol (Choi et al., 2007). The activity increased both with increasing concentration of EOs and treatment time. Literature also confirmed that EOs containing higher amounts of l-limonene usually showed excellent nematicidal potential (Duschatzky et al., 2004). The relative order of nematicidal activity exhibited by the test EOs after a 72-h incubation period, was OEO > CNEO > TEO > AEO > CEO > PEO > WEO > MTEO > MREO.
Conclusion
The present study employs analytical and molecular modeling tools to relate the nematicidal activity of potential essential oils and the interactions of their chemical constituents with the target site proteins of the organism. Among the nine essential oils screened against M. incognita in vitro, the orange (OEO) and citronella (CNEO) oils were identified in the present work as most effective for immobilization and killing of nematodes. In silico analysis suggested a higher binding capacity of geraniol, β-terpineol, citronellal, l-limonene, γ-terpinene, to the selected target proteins. Molecular docking-based understanding of the bioactivity of aromatic oils is a novel attempt toward logic-driven selection of natural materials and discovery of biopesticidal leads. The present findings will be further confirmed through wet lab molecular studies and utilized in bionematicide product development.
Data Availability Statement
The original contributions presented in the study are included in the article/supplementary materials, further inquiries can be directed to the corresponding author/s.
Author Contributions
AS, AdK, AD, and AM conceptualized the study. AdK, AM, AnK, and SD validated the study. AdK, AD, AM, LN, RP, MM, JA, NP, and PS conducted the investigation. AS provided the resources. AdK, AM, AD, and SM wrote and prepared the original draft. AdK, AM, AD, SS, and RK wrote, reviewed, and edited the manuscript. AS and UR supervised the study. AS acquired the funding. All authors contributed to the article and approved the submitted version.
Funding
This work was supported by the Indian Council of Agricultural Research-Niche Area of Excellence Programme (ICAR-NAE) (Grant number: Edn. 5(17)/2017-EP&HS), Ministry of Agriculture, New Delhi, India. Though there was no funds from the funding agency for article processing for open access publication fees, authors will pay themselves. Thus, all the authors requested if the charges can be discounted at the maximum.
Conflict of Interest
The authors declare that the research was conducted in the absence of any commercial or financial relationships that could be construed as a potential conflict of interest.
Acknowledgments
The authors wish to thank the Director, Indian Council of Agricultural Research-Indian Agricultural Research Institute (ICAR-IARI), New Delhi, India.
Footnotes
- ^ http://blast.ncbi.nlm.nih.gov
- ^ https://parasite.wormbase.org//Tools/Blast
- ^ pubchem.ncbi.nlm.nih.gov
References
Abagyan, R., Totrov, M., and Kuznetsov, D. (1994). ICM—a new method for protein modeling and design: applications to docking and structure prediction from the distorted native conformation. J. Comput. Chem. 15, 488–506. doi: 10.1002/jcc.540150503
Aissani, N., Balti, R., and Sebai, H. (2018). Potent nematicidal activity of phenolic derivatives on Meloidogyne incognita. J. Helminthol. 92, 668–673. doi: 10.1017/s0022149x17000918
Aissani, N., Urgeghe, P. P., Oplos, C., Saba, M., Tocco, G., Petretto, G. L., et al. (2015). Nematicidal activity of the volatilome of Eruca sativa on Meloidogyne incognita. J. Agricult. Food Chem. 63, 6120–6125.
Amora, D. X., de Podesta, G. S., Nasu, E. D. G. C., de Figueiredo, L. D., Ferreira, F. C., Lopes, E. A., et al. (2017). Effect of essential oils on the root-knot nematode management. Agri-Environ. Sci. 3, 15–23.
Andrés, M. F., González-Coloma, A., Sanz, J., Burillo, J., and Sainz, P. (2012). Nematicidal activity of essential oils: a review. Phytochem. Rev. 11, 371–390. doi: 10.1007/s11101-012-9263-3
Ardakani, A. S., Hosyninejad, S. A., and Pourshirzad, A. (2013). Killing effects of Myrtus communis L. essential oil on Meloidogyne incognita. Int. J. Agric. Crop Sci. 5, 806–810.
Atolani, O., and Fabiyi, O. A. (2020). “Plant parasitic nematodes management through natural products: current progress and challenges,” in Management of Phytonematodes: Recent Advances and Future Challenges, eds R. Ansari, R. Rizvi, and I. Mahmood (Singapore: Springer), 297–315. doi: 10.1007/978-981-15-4087-5_13
Avato, P., Laquale, S., Argentieri, M. P., Lamiri, A., Radicci, V., and D’Addabbo, T. (2017). Nematicidal activity of essential oils from aromatic plants of Morocco. J. Pest Sci. 90, 711–722. doi: 10.1007/s10340-016-0805-0
Bakkali, F., Averbeck, S., Averbeck, D., and Idaomar, M. (2008). Biological effects of essential oils – a review. Food Chem. Toxicol. 46, 446–475.
Buré, C. M., and Sellier, N. M. (2004). Analysis of the essential oil of Indonesian patchouli (Pogostemon cablin Benth.) using C/MS (EI/CI). J. Essent. Oil Rese. 16, 17–19. doi: 10.1080/10412905.2004.9698638
Bursulaya, B. D., Totrov, M., Abagyan, R., and Brooks, C. L. (2003). Comparative study of several algorithms for flexible ligand docking. J. Comput. Aided Mol. Des. 17, 755–763. doi: 10.1023/b:jcam.0000017496.76572.6f
Caboni, P., Saba, M., Tocco, G., Casu, L., Murgia, A., Maxia, A., et al. (2013). Nematicidal activity of mint aqueous extracts against the root-knot nematode Meloidogyne incognita. J. Agric. Food Chem. 61, 9784–9788. doi: 10.1021/jf403684h
Cantrell, C. L., Dayan, F. E., and Duke, S. O. (2012). Natural products as sources for new pesticides. J. Nat. Prod. 75, 1231–1242. doi: 10.1021/np300024u
Choi, I., Kim, J., Shin, S., and Park, I. (2007). Nematicidal activity of monoterpenoids against the pine wood nematode (Bursaphelenchus xylophilus). Russian J. Nematol. 15, 35–40.
Cobb, N. A. (1918). Estimating the Nema Population of Soil, with Special Reference to the Sugar-beet and Root-gall Nemas, Heterodera schachtii Schmidt and Heterodera radicicola (Greef) Müller: and with a Description of Tylencholaimus Aequalis n. sp. Washington, D.C: US Government Printing Office.
Collange, B., Navarrete, M., Peyre, G., Mateille, T., and Tchamitchian, M. (2011). Root-knot nematode (Meloidogyne) management in vegetable crop production: the challenge of an agronomic system analysis. Crop Protec. 30, 1251–1262. doi: 10.1016/j.cropro.2011.04.016
de Freitas Silva, M., Campos, V. P., Barros, A. F., da Silva, J. C. P., Pedroso, M. P., de Jesus Silva, F., et al. (2020). Medicinal plant volatiles applied against the root-knot nematode Meloidogyne incognita. Crop Prot. 130:105057. doi: 10.1016/j.cropro.2019.105057
Deepalakshmi, P. D., Odgerel, K., Thirugnanasambantham, P., Yungeree, O., Khorolragchaa, A., and Senthil, K. (2016). Metabolite profiling of in vitro cultured and field grown rhizomes of Acorus calamus from Mongolia using GC–MS. Chromatographia 79, 1359–1371. doi: 10.1007/s10337-016-3152-7
Dejam, M., and Farahmand, Y. (2017). Essential oil content and composition of myrtle (Myrtus communis L.) leaves from South of Iran. J. Essent. Oil Bearing Plants 20, 869–872. doi: 10.1080/0972060x.2014.981599
Dunbrack, R. L. Jr. (2006). Sequence comparison and protein structure prediction. Curr. Opin. Struct. Biol. 16, 374–384. doi: 10.1016/j.sbi.2006.05.006
Duschatzky, C. B., Martinez, A. N., Almeida, N. V., and Bonivardo, S. L. (2004). Nematicidal activity of the essential oils of several Argentina plants against the root-knot nematode. J. Essent. Oil Res. 16, 626–628. doi: 10.1080/10412905.2004.9698812
Echeverrigaray, S., Zacaria, J., and Beltrão, R. (2010). Nematicidal activity of monoterpenoids against the root-knot nematode Meloidogyne incognita. Phytopathol 100, 199–203. doi: 10.1094/phyto-100-2-0199
Hopkins, A. L., Keserü, G. M., Leeson, P. D., Rees, D. C., and Reynolds, C. H. (2014). The role of ligand efficiency metrics in drug discovery. Nat. Rev. Drug Discov. 13, 105–121. doi: 10.1038/nrd4163
Jamoussi, B., Romdhane, M., Abderraba, A., Hassine, B. B., and Gadri, A. E. (2005). Effect of harvest time on the yield and composition of Tunisian myrtle oils. Flavour Frag. J. 20, 274–277. doi: 10.1002/ffj.1453
Jones, J. G., Kleczewski, N. M., Desaeger, J., Meyer, S. L., and Johnson, G. C. (2017). Evaluation of nematicides for southern root-knot nematode management in lima bean. Crop Prot. 96, 151–157. doi: 10.1016/j.cropro.2017.02.015
Julio, L. F., González-Coloma, A., Burillo, J., Diaz, C. E., and Andrés, M. F. (2017). Nematicidal activity of the hydrolate byproduct from the semi industrial vapor pressure extraction of domesticated Artemisia absinthium against Meloidogyne javanica. Crop Prot. 94, 33–37. doi: 10.1016/j.cropro.2016.12.002
Kalaiselvi, D., Mohankumar, A., Shanmugam, G., Thiruppathi, G., Nivitha, S., and Sundararaj, P. (2019). Altitude-related changes in the phytochemical profile of essential oils extracted from Artemisia nilagirica and their nematicidal activity against Meloidogyne incognita. Ind. Crop Prod. 139:111472. doi: 10.1016/j.indcrop.2019.111472
Kalemba, D., and Kunicka, A. (2003). Antibacterial and antifungal properties of essential oils. Curr. Med. Chem. 10, 813–829. doi: 10.2174/0929867033457719
Kong, J. O., Lee, S. M., Moon, Y. S., Lee, S. G., and Ahn, Y. J. (2006). Nematicidal activity of plant essential oils against Bursaphelenchus xylophilus (Nematoda: Aphelenchoididae). J. Asia-Pac. Entomol. 9, 173–178. doi: 10.1016/s1226-8615(08)60289-7
Kundu, A., Saha, S., Walia, S., and Dutta, T. K. (2016). Antinemic potentiality of chemical constituents of Eupatorium adenophorum Spreng leaves against Meloidogyne incognita. Nat. Acad. Sci. Lett. 39, 145–149. doi: 10.1007/s40009-016-0439-z
Lee, Y. S., Kim, J., Lee, S. G., Oh, E., Shin, S. C., and Park, I. K. (2009). Effects of plant essential oils and components from Oriental sweetgum (Liquidambar orientalis) on growth and morphogenesis of three phytopathogenic fungi. Pestic. Biochem. Physiol. 93, 138–143. doi: 10.1016/j.pestbp.2009.02.002
Madalosso, R. G., Verdi, C. M., Souza, M. E., Cunha, J. A., Santos, P. S., Balardin, R. S., et al. (2017). In vitro and in vivo effects of Melaleuca alternifolia nanoparticles in Meloidogyne sp. J. Asia-Pac. Entomol. 20, 1344–1349. doi: 10.1016/j.aspen.2017.10.002
Matuka, T., Oyedeji, O., Gondwe, M., and Oyedeji, A. (2020). Chemical composition and in vivo anti-inflammatory activity of essential oils from Citrus sinensis (L.) osbeck growing in South Africa. J. Essent. Oil Bear. Plants 23, 638–647. doi: 10.1080/0972060x.2020.1819885
Neves, M. A., Totrov, M., and Abagyan, R. (2012). Docking and scoring with ICM: the benchmarking results and strategies for improvement. J. Comput. Aided Mol. Des. 26, 675–686. doi: 10.1007/s10822-012-9547-0
Nguyen, H. T., Radácsi, P., Gosztola, B., Rajhárt, P., and Németh, ÉZ. (2018). Accumulation and composition of essential oil due to plant development and organs in wormwood (Artemisia absinthium L.). Ind. Crop Prod. 123, 232–237. doi: 10.1016/j.indcrop.2018.06.076
Oka, Y. (2001). Nematicidal activity of essential oil components against the root-knot nematode Meloidogyne javanica. Nematol. 3, 159–164. doi: 10.1163/156854101750236286
Pandey, R., Kalra, A., Tandon, S., Mehrotra, N., Singh, H. N., and Kumar, S. (2000). Essential oils as potent source of nematicidal compounds. J. Phytopathol. 148, 501–502. doi: 10.1046/j.1439-0434.2000.00493.x
Pedroso, L. A., Campos, V. P., Pedroso, M. P., Barros, A. F., Freire, E. S., and Resende, F. M. (2019). Volatile organic compounds produced by castor bean cake incorporated into the soil exhibit toxic activity against Meloidogyne incognita. Pest Manag. Sci. 75, 476–483. doi: 10.1002/ps.5142
Perrett, S., and Whitfield, P. J. (1995). Anthelmintic and pesticidal activity of Acorus gramineus (Araceae) is associated with phenylpropanoid asarones. Phytother. Res. 9, 405–409. doi: 10.1002/ptr.2650090604
Raymond, C. A., Davies, N. W., and Larkman, T. (2017). GC-MS method validation and levels of methyl eugenol in a diverse range of tea tree (Melaleuca alternifolia) oils. Anal. Bioanal. Chem. 409, 1779–1787. doi: 10.1007/s00216-016-0134-4
Sidhu, H. S., Kumar, V., and Madhu, M. R. (2017). Eco-Friendly management of root-knot nematode, Meloidogyne javanica in okra (Abelmoschus esculentus) crop. Int. J. Pure Appl. Biosci. 5, 569–574. doi: 10.18782/2320-7051.2507
Siddique, Y. H., Mujtaba, S. F., Jyoti, S., and Naz, F. (2013). GC-MS analysis of Eucalyptus citriodora leaf extract and its role on the dietary supplementation in transgenic Drosophila model of Parkinson’s disease. Food Chem. Toxicol. 55, 29–35. doi: 10.1016/j.fct.2012.12.028
Silva, J. C. P., Campos, V. P., Barros, A. F., Pedroso, M. P., Terra, W. C., Lopez, L. E., et al. (2018). Plant volatiles reduce the viability of the root-knot nematode Meloidogyne incognita either directly or when retained in water. Plant Dis. 102, 2170–2179. doi: 10.1094/pdis-01-18-0143-re
Singh, H. P., Kaur, S., Negi, K., Kumari, S., Saini, V., Batish, D. R., et al. (2012). Assessment of in vitro antioxidant activity of essential oil of Eucalyptus citriodora (lemon-scented Eucalypt; Myrtaceae) and its major constituents. LWT Food Sci. Technol. 48, 237–241. doi: 10.1016/j.lwt.2012.03.019
Sinha, A., Maheshwari, R. C., Dureja, P., and Mojumder, V. (2006). Nematicidal activity of essential oils and their components against the root-knot nematode Meloidogyne incognita. Ind. J. Nematol. 36, 109–114.
Tetali, S. D. (2019). Terpenes and isoprenoids: a wealth of compounds for global use. Planta 249, 1–8. doi: 10.1007/s00425-018-3056-x
Westphal, A. (2011). Sustainable approaches to the management of plant-parasitic nematodes and disease complexes. J. Nematol. 43, 122–125.
Keywords: volatile oils, gas chromatography-mass spectrometry analysis, Meloidogyne incognita, molecular docking, odorant response gene 1
Citation: Kundu A, Dutta A, Mandal A, Negi L, Malik M, Puramchatwad R, Antil J, Singh A, Rao U, Saha S, Kumar R, Patanjali N, Manna S, Kumar A, Dash S and Singh PK (2021) A Comprehensive in vitro and in silico Analysis of Nematicidal Action of Essential Oils. Front. Plant Sci. 11:614143. doi: 10.3389/fpls.2020.614143
Received: 05 October 2020; Accepted: 20 November 2020;
Published: 08 January 2021.
Edited by:
Rachid Lahlali, Ecole Nationale d’Agriculture de Meknès, MoroccoReviewed by:
Pinarosa Avato, University of Bari Aldo Moro, ItalyFouad Mokrini, National Institute for Agricultural Research (Morocco), Morocco
Copyright © 2021 Kundu, Dutta, Mandal, Negi, Malik, Puramchatwad, Antil, Singh, Rao, Saha, Kumar, Patanjali, Manna, Kumar, Dash and Singh. This is an open-access article distributed under the terms of the Creative Commons Attribution License (CC BY). The use, distribution or reproduction in other forums is permitted, provided the original author(s) and the copyright owner(s) are credited and that the original publication in this journal is cited, in accordance with accepted academic practice. No use, distribution or reproduction is permitted which does not comply with these terms.
*Correspondence: Anupama Singh, anupamanil2000@gmail.com; Aditi Kundu, chem.aditi@gmail.com