- Timiryazev Institute of Plant Physiology, Russian Academy of Sciences, Moscow, Russia
Cytokinins (CKs) were earlier shown to promote potato tuberization. Our study aimed to identify and characterize CK-related genes which constitute CK regulatory system in the core potato (Solanum tuberosum) genome. For that, CK-related genes were retrieved from the sequenced genome of the S. tuberosum doubled monoploid (DM) Phureja group, classified and compared with Arabidopsis orthologs. Analysis of selected gene expression was performed with a transcriptome database for the S. tuberosum heterozygous diploid line RH89-039-16. Genes responsible for CK signaling, biosynthesis, transport, and metabolism were categorized in an organ-specific fashion. According to this database, CK receptors StHK2/3 predominate in leaves and flowers, StHK4 in roots. Among phosphotransmitters, StHP1a expression largely predominates. Surprisingly, two pseudo-phosphotransmitters intended to suppress CK effects are hardly expressed in studied organs. Among B-type RR genes, StRR1b, StRR11, and StRR18a are actively expressed, with StRR1b expressing most uniformly in all organs and StRR11 exhibiting the highest expression in roots. By cluster analysis four types of prevailing CK-signaling chains were identified in (1) leaves and flowers, StHK2/3→StHP1a→StRR1b/+; (2) shoot apical meristems, stolons, and mature tubers, StHK2/4→StHP1a→StRR1b/+; (3) stems and young tubers, StHK2/4→StHP1a→StRR1b/11/18a; and (4) roots and tuber sprouts, StHK4→StHP1a→StRR11/18a. CK synthesis genes StIPT3/5 and StCYP735A are expressed mainly in roots followed by tuber sprouts, but rather weakly in stolons and tubers. By contrast, CK-activation genes StLOGs are active in stolons, and StLOG3b expression is even stolon-confined. Apparently, the main CK effects on tuber initiation are realized via activity of StLOG1/3a/3b/7c/8a genes in stolons. Current advances and future directions in potato research are discussed.
Introduction
Potato tubers (Solanum tuberosum L.) are well known and widespread sources of food, feed, and technical substances (starches). Cytokinins (CKs), classical plant hormones, are known to promote potato tuber formation, at least in conventional in vitro systems (Palmer and Smith, 1970; Aksenova et al., 2000; Romanov et al., 2000; Cheng et al., 2020). CKs are also involved in the formation of artificial “tuberoids” on tobacco and tomato shoots (Guivarc’h et al., 2002; Eviatar-Ribak et al., 2013) as well as tuber-shaped rounded tumors on agrobacteria-infected plants (Dodueva et al., 2020). In addition to stimulating the formation of tubers, CKs contribute to their sprouting (Hartmann et al., 2011; Aksenova et al., 2013). The morphogenic effect of CKs certainly exploits their ability to induce cell divisions (Miller et al., 1955; Sakakibara, 2006; Romanov, 2009; Kieber and Schaller, 2014), although is not limited to this. All the above emphasizes the importance of CK regulatory system for such a valuable tuber-producing crop as potatoes.
Over the past decade, a prominent progress has been achieved in potato research. First of all, this concerns the sequencing of the complete genome of S. tuberosum group Phureja doubled monoploid DM1-3 516 R44 (DM) by the Potato Genome Sequencing Consortium [PGSC] (2011). Thereafter, a set of genes/proteins controlling tuberization was uncovered (Dutt et al., 2017; Hannapel et al., 2017). It became clear that the regulation of tuberization is based on a complex crosstalk between numerous hormonal and non-hormonal factors (Aksenova et al., 2012, 2014). In our research, we focused on the hormonal part of this regulatory network. On the basis of our experimental data (Kolachevskaya et al., 2015, 2017, 2018, 2019a) and data from recent literature, an updated hypothesis of hormonal regulation of potato tuberization was advanced (Kolachevskaya et al., 2017, 2019b), where CKs play an important role, especially at the tuber induction and initiation stages. Furthermore, the DNA sequence coding for CK receptors (sensor histidine kinase) and basic CK-signaling machinery were identified in the sequenced DM genome and analyzed by means of bioinformatics tools (Lomin et al., 2018b). In parallel, a suite of genes encoding sensor histidine kinases from tetraploid cultivar “Désirée” were cloned and expressed, giving rise to individual CK receptors. These receptors were studied in-depth, including their phylogenetics, conserved domains, 3D-structures, ligand-binding properties, organ-specificity of expression and responsiveness to CKs, regulatory cis-elements in their promoters, subcellular localization, homo- and heterodimerization, and mode of interaction with phosphotransmitters (Lomin et al., 2018b, 2020; Arkhipov et al., 2019).
Receptors are considered key proteins in hormone signaling, but they cannot signal by themselves. Regulation by any hormone in planta requires dozens of genes/proteins that function in various aspects of the given hormonal system. Like any other hormonal system, the CK regulatory system can be conventionally divided into a relatively conserved central part (CK signaling, synthesis, metabolism, and transport) and a more variable periphery—mainly CK-responsive regulatory genes (Bhargava et al., 2013; Brenner and Schmülling, 2015). For example, the central part of the model Arabidopsis plant distinguished by small genome size comprises, according to the latest estimates, some 80 genes (Supplementary Figure 1). Among them, 34 genes belong to the so-called two-component system (TCS) and constitute a signaling pathway termed multistep phosphorelay (MSP) from transmembrane receptors to primary response genes in the nucleus (Heyl and Schmülling, 2003; Mizuno, 2005; Kieber and Schaller, 2014, 2018; Pekárová et al., 2016, 2018; Arkhipov et al., 2019; Hallmark and Rashotte, 2019). Other involved genes have been classified as genes for CK synthesis (20 genes in total) (Kamada-Nobusada and Sakakibara, 2009), catabolism, and reversed inactivation (12 genes) (Schmülling et al., 2003; Hoyerová and Hošek, 2020), as well a transport (eight genes) (Liu et al., 2019). The CK regulatory system in potatoes is far less studied than in Arabidopsis. Here we intend to gain insight into the genome-wide composition and functioning of the central part of CK regulatory system in potatoes, with a particular focus on tuber formation. The prospects for using current knowledge to improve potato yield are discussed below.
CK-Related Genes in Potatoes
Current molecular studies of potatoes are based on the sequenced DM genome representing the core genome of this widespread crop. Nevertheless, the size of even this minimal genome (844 Mb) is many times larger than the genome of the model Arabidopsis plant (135 Mb). However, this difference essentially disappears when we compare the numbers of protein-coding genes: 27,029 in Arabidopsis thaliana “Columbia” (Swarbreck et al., 2008) and 39,031 in DM potato (Potato Genome Sequencing Consortium [PGSC], 2011). In this case, the size of the one genome is now only reduced to only 1.44 times that of the other. Consequently, a large dissimilarity in numbers of genes of CK regulatory systems between these two species seems unlikely. Table 1 demonstrates that the core potato genome encodes orthologs of nearly all gene families involved in the central part of the CK system in Arabidopsis. And indeed, the sizes of orthologous gene families are rather close in Arabidopsis and potato (Supplementary Figure 1; Lomin et al., 2018b). For CK synthesis, potatoes possess 16 genes (compare to 20 in Arabidopsis), encoding six isopentenyl adenine transferases (StIPT), one cytochrome P450 monooxygenase StCYP735A, and nine lonely guy (StLOG) orthologs. For CK perception, potatoes have three receptor histidine kinases (StHK2–4) similarly to Arabidopsis; 8, 7, and 2 response regulators of class A, B, and C, respectively, as well as three cytokinin response factor (StCRF) proteins. In this regard, potatoes markedly differ from Arabidopsis in the proportion of TCS-like genes. In potatoes, half (three out of six) of the members of the phosphotransfer protein family (StHP) lack conserved phospho-accepting amino acids and are considered putative inhibitors of the MSP. By contrast, in Arabidopsis only one such member (AHP6, 1 from 6) is a true MSP inhibitor. In Arabidopsis, type B response regulators were divided into three groups, from which only ARR-Bs of the first group (ARR-BI) were proven to participate in CK signaling. Interestingly, ARR-Bs of these groups are not the closest neighbors on the phylogenetic tree but are interspersed with the APRR2 and APRR6 groups of pseudo-RRs. Similarly to Arabidopsis, most (7) of potato RR-B genes are homologous to RR-BI group, whereas three StRR-B genes are beyond this phylogenetic branch (Supplementary Figure 2A). The encoded three StRR-B proteins (StRR25, 27, and 28), along with conserved phospho-accepting aspartate, contain abnormal DD- and K-motifs in their receiver (REC-) domains (Supplementary Figure 2B), which most likely render these proteins inactive. Other potato non-canonical genes are listed in the Supplementary Table 1 as they hardly contribute to CK action. Notably, the potato genome contains genes encoding type C pseudo RRs (PRR type C) which are lacking in Arabidopsis. At least an essential portion of Arabidopsis PRR orthologs harbor CCT motif and take part in the photoperiodic flowering control unrelated to the CK system and to being subject to circadian rhythms (Mizuno, 2005). Any other role of the remaining PRRs (Supplementary Table 1) in CK action cannot be completely excluded but is very questionable.
We included in Table 1 non-CK receptor histidine kinases (CKI1, ETR1) as they may play a role in CK signaling. When one such kinase (AtCKI1) was spontaneously overexpressed, the mutated phenotype mimicked the effect of massive CK treatment (Kakimoto, 1996). The receiver domain of another histidine kinase, ethylene receptor ETR1, also can interact with MSP signaling intermediates acting downstream from CK receptors (Zdarska et al., 2019). The background TCS activity of these proteins seems to be sufficient to rescue the basic phenotype of Arabidopsis triple mutants lacking all three CK receptors and no longer responding to CKs (Higuchi et al., 2004; Nishimura et al., 2004; Riefler et al., 2006; Romanov, 2009). All this indicates the possible role of CKI1 and ETR1 in MSP signaling. In total, the estimated number of genes directly involved in CK signaling (MSP) in DM potatoes is 31.
For CK degradation, potatoes possess seven CKX orthologs (Table 1). Genes for putative CK conjugation are included only in Supplementary Table 1 because potato StUGTs have no direct homology to Arabidopsis UGT genes responsible for CK O- and N-glucosylation (Supplementary Figure 3). Also, genes encoding CK transporters (Liu et al., 2019)—StPUP (10), StENT (4), and StABCG14 (2) [possibly also StABCI19-21 and StAZG1,2 (Kim et al., 2020; Tessi et al., 2020), see Supplementary Table 1]—have active orthologs in Arabidopsis and are obviously of particular importance. The exceptions are gene-orthologs of PUP14 transporters of Arabidopsis that are missing in potatoes (Supplementary Figure 4). Overall, the central part of the CK regulatory system in the core potato genome comprises 70 genes, the number close to that in Arabidopsis (Supplementary Figure 1). Among the potato genes, 28 (40%) are TCS homologs, whereas the remaining genes are not TCS-related.
The Expression Pattern of CK-Related Genes
To elucidate the molecular events underlying potato growth, productivity and stress tolerance, the list of families of paralog genes is a useful but insufficient characteristic. Knowledge of absolute and relative spatiotemporal gene expression is necessary to address this issue. The expression pattern of genes involved in the CK action in the diploid potato line RH89-039-161 is shown in Figure 1. Clearly, most of these genes are expressed differently depending on the organ and stage of development. Among CHK receptors, the expression of StHK2/3 predominates in leaves and flowers, while StHK4 is mostly expressed in roots. In the latter organ, StHK2/4 genes are quite active. Among phosphotransmitters, StHP1a expression predominates in every organ, following by StHP1b, which is also expressed quasi-constitutively but to a much lesser extent. Interestingly, all three genes for the phosphotransfer-like proteins StPHP4b, 4c, 6, which are supposed to suppress CK signaling, are hardly expressed in RH89-039-16 line. As for RR-B transcription factors, StRR1b is expressed most uniformly in all organs, StRR1a acts similarly but much more weakly, and StRR1c is almost not expressed. Among all B-type RR genes, StRR1a, StRR1b, StRR11, and StRR18a are the most strongly expressed. Roots represent the site where almost all RR-B genes are expressed, dominated by StRR11.
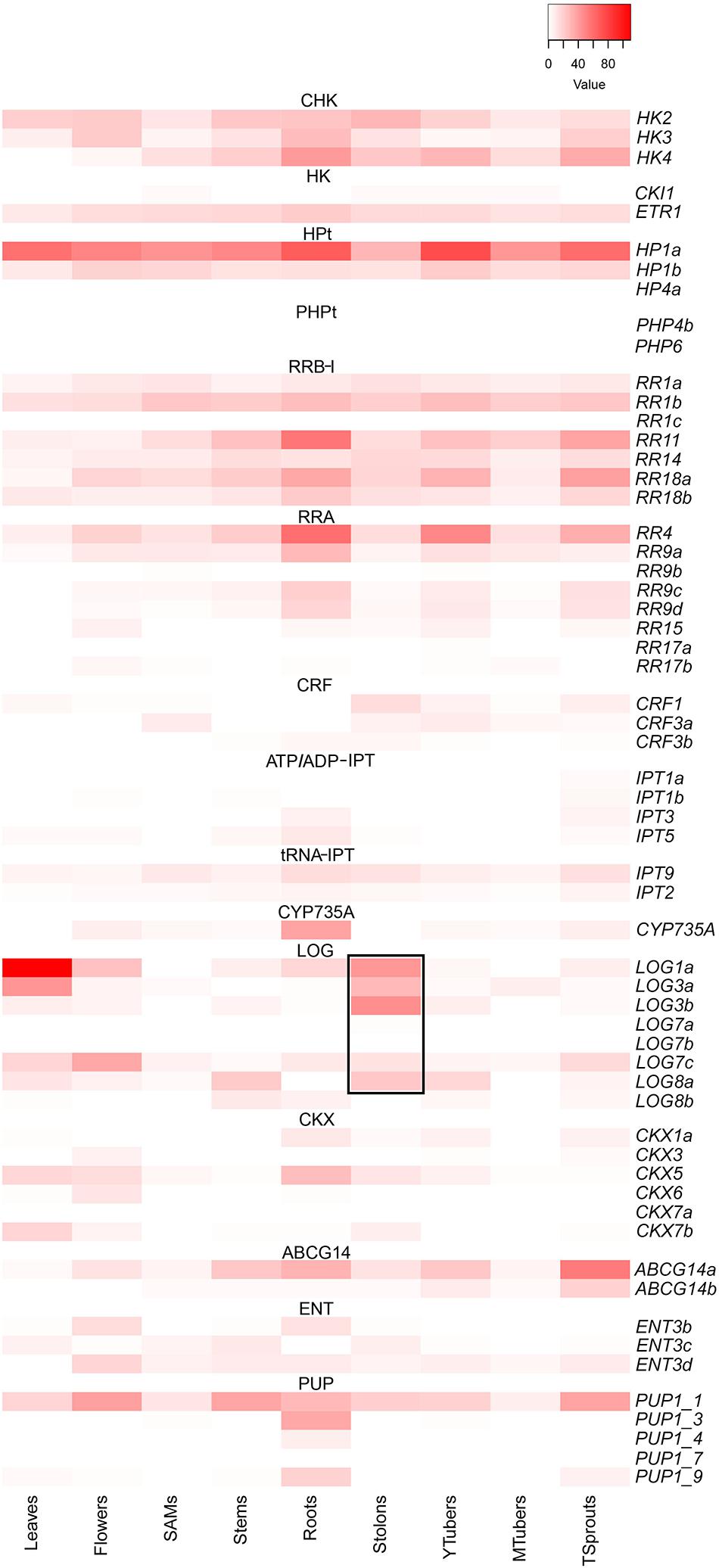
Figure 1. Expression pattern of cytokinin system genes of potato generated with the use of Heatmapper service (http://www.heatmapper.ca/expression/) (Babicki et al., 2016). The LOG gene expression pattern in the stolon is framed. SAMs, shoot apical meristems; YTubers and MTubers, young and mature tubers, respectively; TSprouts, tuber sprouts.
By means of cluster analysis of the organ-specific expression of genes encoding receptors and StRRs type B (Supplementary Figure 5), we identified four types of prevailing signaling chains: (1) in leaves and flowers, StHK2/3→StHP1a→StRR1b/+; (2) in shoot apical meristems, stolons, and mature tubers, StHK2/4→StHP1a→StRR1b/+; (3) in stems and young tubers, StHK2/4→StHP1a→StRR1b/11/18a; and (4) in roots and tuber sprouts, StHK4→StHP1a→StRR11/18a.
Cytokinins synthesis genes StIPT3/5 and StCYP735A are expressed mainly in roots (similarly to Arabidopsis). CK-perception and synthesis genes (StHKs, StIPTs, and StCYP735A) are also actively expressed in tuber sprouts, where StHK4 transcripts prevail over transcripts of other CK receptor genes. A special group of CK-activation genes termed StLOGs are active in stolons, and StLOG3b expression is mainly restricted to this organ (Figure 1). It is noteworthy that organs (leaves, stolons) in which StLOG genes are strongly expressed, are mostly devoid of transcripts of other CK-synthesizing genes (StIPT, StCYP735A) (Figure 1). The above observations, based on the gene expression data1 for the diploid potato RH89-039-16, are generally consistent with organ-specific analysis of the transcriptome in the commercial tetraploid potatoes “Désirée” (Lomin et al., 2018b, and data not shown). However, the organ/tissue patterns of the expressed genes in different potato lines/cultivars (DM, RH89-039-16, “Désirée”) were not strictly identical, indicating some cultivar-specificity of gene functioning in potatoes. For example, RH89-039-16 and “Désirée” share similar organ-dependent expression patterns for StHP1a, StPHP4b, StPHP6, StRR1b, StRR14, StRR18a, StRR9a, StCYP735A, and StABCG14a, although marked differences in these patterns were observed for StRR11, StRR9c, StIPT5, and StCKX3. The expression patterns of remaining CK-related genes coincide moderately.
Discussion
Here we present a global view on the CK regulatory system in potatoes. Generally, each hormonal regulatory system, in particular the cytokinin one (Romanov, 2009), includes complex units ensuring hormone biosynthesis, translocation, perception, and inactivation, as well as the primary response structures to targeted signal transduction. These units consist of corresponding proteins encoded by cognate genes. Here, the CK-related gene sequences were retrieved from the annotated core genome of the doubled monoploid DM1-3 516 R44 potato Phureja (the genes whose role in the CK system is proven or highly probable, Table 1) and thus represent the minimal gene set of the potato CK system. There is no doubt that commercial varieties of potatoes, mostly tetraploids, possess much many genes related to CK system. This is corroborated by data on the tetraploid “Désirée” variety, in which at least six authentic CK receptors have been identified (Lomin et al., 2018b), twice as many (3) CK receptors encoded by the DM genome. However, additional genes are close paralogs of the “core” genes, so the number of gene clades remains unchanged. Other genes that could theoretically acquire the status of CK “core” genes are listed in Supplementary Table 1, but their involvement requires detailed research and seems unlikely at this time.
In terms of nomenclature, we propose to follow the tradition of naming gene/protein according to the best homology to its Arabidopsis counterpart. Thereby, the potato genes were named according to their closest orthologs in Arabidopsis (see Supplementary Figures 2–4) in Supplementary Data. When many potato genes turned out to be orthologous to the same Arabidopsis gene, the former are marked with an additional letter at the end (a, b, c, etc.). Such nomenclature has already been used to designate potato CK-receptors (Lomin et al., 2012; Steklov et al., 2013) and other potato genes/proteins (Lomin et al., 2018b). The exceptions are StPUP genes since their homology to any of Arabidopsis PUPs is not obvious. In any case, this compiled gene set (Table 1) can serve as a convenient basis for studying genes, constituting the basic part of the CK system in potato.
As expected, most of CK-related enzymatic activities are encoded in the core potato genome by small (mostly 2–10 members) gene families. At the moment, 16 such families can be counted, of which eight families correspond to intracellular signal transduction (MSP), totally 31 genes encoding 41 proteins (Table 1). Among these proteins, only three (StHKs) have contact directly with hormonal ligands, while others obviously do not, though this has not been definitely proven. In fact, this part of the global CK system includes 28 authentic TCS homologs, which generate or affect signal transmission through His-Asp phosphorelay. The exceptions are proteins with degenerated TCS homology, which cannot directly participate in MSP because of structural deficiency. These non-functioning in MSP genes and proteins are evidently not bona fide members of the central part of the CK system. Some of them are displayed in Supplementary Table 1 containing not yet excluded but possible candidates for the CK system in potatoes. In fact, CKs almost monopolized the MSP system, using it as a signaling part of their global regulatory circuit. This statement is supported by the fact that every functional phosphotransmitter is promiscuous, i.e., is able to transmit “hot” phosphate from any CK receptor to any RR-B in the nucleus (Hutchison et al., 2006; Dortay et al., 2008; Lomin et al., 2018a; Arkhipov et al., 2019). Other hormones—in particular, ethylene, whose receptors are also TCS homologs—had to switch to a signal transduction pathway other than MSP (Pekárová et al., 2018).
Data on the relative expression of selected genes allowed us to outline the most plausible protein chains transmitting the CK signal from receptors to primary response genes. This corresponds to the activity of the prevailing potato MSP. We suggested four types of main signaling chains, which are delineated above. These chains are partially redundant, especially in relation to the transfer stage from the cytosol to the nucleus, where phosphotransmitter StHP1a predominates in all organs of the diploid potato. However, predominant receptors and B-type response regulators may differ depending on the organ, and this CK-signaling specificity should be taken into account when researchers manipulate the potato genome. To date, we consider the stolon as the most promising organ for engineering potato productivity and early maturation. Apparently, this is where the most important events leading to tuber initiation should take place. The most noticeable feature of the transcriptome of the CK system in stolons is the increased activity of the LOG-genes (StLOG1/3a/3b/7c/8a) without visible changes in the expression of other CK-related gene families. Moreover, the expression of StLOG3b is stolon-specific. In contrast, the activity of other CK synthesis genes, IPTs and CYP735A, was much weaker here than in other organs. Thus, stolons are likely sites where CK precursors (phosphoribosides) are exported and activated to functional CK bases by LOG enzymes. Recently, it was found that three LOG genes (StLOG3a, 7a, and 7c) are activated by the tuberigen StBEL5, most likely through the direct interaction of this transcription factor with the corresponding cis-elements in the promoters of these genes (Sharma et al., 2016). It is noteworthy that the ectopic expression of only one of the tomato LOG genes was sufficient for the formation of tuber-like structures from the lateral meristems in tomato (Eviatar-Ribak et al., 2013). Thus, we assume that CKs are involved in the tuberization process through the activation of LOG genes by the StBEL5 pathway. The contribution of the stolon identity pathway is also possible due to the only partial overlap of LOG expression patterns induced by StBEL5 and inherent in stolons.
Collectively, since CKs were known to be implicated in many aspects of potato growth and productivity, the presented genome-wide data characterizing the CK-regulatory system of potatoes can be useful for the exploration and breeding of this important crop.
Data Availability Statement
Publicly available datasets were analyzed in this study. This data can be found here: http://solanaceae.plantbiology.msu.edu/pgsc_download.shtml.
Author Contributions
SL, YM, OK, IG, ES, SD, and DA presented and analyzed their experimental and/or bioinformatic results. SL prepared main illustrative materials. GR wrote the version of the manuscript. All authors contributed to the manuscript editing, developed the idea and outline of the manuscript, and read and approved the manuscript final version before submission.
Funding
Supported by a grant of the Russian Science Foundation No. 17-74-20181. We also thank the Ministry of Science and Higher Education of the Russian Federation for generous subsidies used to pay for the main Institute facilities as prerequisites for the successful experimental work.
Conflict of Interest
The authors declare that the research was conducted in the absence of any commercial or financial relationships that could be construed as a potential conflict of interest.
Supplementary Material
The Supplementary Material for this article can be found online at: https://www.frontiersin.org/articles/10.3389/fpls.2020.613624/full#supplementary-material
Footnotes
References
Aksenova, N. P., Konstantinova, T. N., Golyanovskaya, S. A., Kossmann, J., Willmitzer, L., and Romanov, G. A. (2000). Transformed potato plants as a model for studying the hormonal and carbohydrate regulation of tuberization. Russ. J. Plant Physiol. 47, 370–379.
Aksenova, N. P., Konstantinova, T. N., Golyanovskaya, S. A., Sergeeva, L. I., and Romanov, G. A. (2012). Hormonal regulation of tuber formation in potato plants. Russ. J. Plant Physiol. 59, 451–466. doi: 10.1134/S1021443712040024
Aksenova, N. P., Sergeeva, L. I., Kolachevskaya, O. O., and Romanov, G. A. (2014). “Hormonal regulation of tuber formation in potato,” in Bulbous Plants: Biotechnology, eds K. Ramavat, and J. Mérillon (Boca Raton, FL: Taylor & Francis Group), 3–36. doi: 10.1201/b16136-3
Aksenova, N. P., Sergeeva, L. I., Konstantinova, T. N., Golyanovskaya, S. A., Kolachevskaya, O. O., and Romanov, G. A. (2013). Regulation of potato tuber dormancy and sprouting. Russ. J. Plant Physiol. 60, 301–312. doi: 10.1134/S1021443713030023
Arkhipov, D. V., Lomin, S. N., Myakushina, Y. A., Savelieva, E. M., Osolodkin, D. I., and Romanov, G. A. (2019). Modeling of protein-protein interactions in cytokinin signal transduction. Int. J. Mol. Sci. 20:2096. doi: 10.3390/ijms20092096
Babicki, S., Arndt, D., Marcu, A., Liang, Y., Grant, J. R., Maciejewski, A., et al. (2016). Heatmapper: web-enabled heat mapping for all. Nucl. Acids Res. 44, W147–W153. doi: 10.1093/nar/gkw419
Bhargava, A., Clabaugh, I., To, J. P., Maxwell, B. B., Chiang, Y. H., Schaller, G. E., et al. (2013). Identification of cytokinin-responsive genes using microarray meta-analysis and RNA-Seq in Arabidopsis. Plant Physiol. 162, 272–294. doi: 10.1104/pp.113.217026
Brenner, W. G., and Schmülling, T. (2015). Summarizing and exploring data of a decade of cytokinin-related transcriptomics. Front. Plant Sci. 6:29. doi: 10.3389/fpls.2015.00029
Cheng, L., Wang, D., Wang, Y., Xue, H., and Zhang, F. (2020). An integrative overview of physiological and proteomic changes of cytokinin-induced potato (Solanum tuberosum L.) tuber development in vitro. Physiol. Plant. 168, 675–693. doi: 10.1111/ppl.13014
Dodueva, I. E., Lebedeva, M. A., Kuznetsova, K. A., Gancheva, M. S., Paponova, S. S., and Lutova, L. A. (2020). Plant tumors: a hundred years of study. Planta 251:82.
Dortay, H., Gruhn, N., Pfeifer, A., Schwerdtner, M., Schmülling, T., and Heyl, A. (2008). Toward an interaction map of the two-component signaling pathway of Arabidopsis thaliana. J. Proteome Res. 7, 3649–3660. doi: 10.1021/pr0703831
Dutt, S., Manjul, A. S., Raigond, P., Singh, B., Siddappa, S., Bhardwaj, V., et al. (2017). Key players associated with tuberization in potato: potential candidates for genetic engineering. Crit. Rev. Biotechnol. 37, 942–957. doi: 10.1080/07388551.2016.1274876
Eviatar-Ribak, T., Shalit-Kaneh, A., Chappell-Maor, L., Amsellem, Z., Eshed, Y., and Lifschitz, E. (2013). A cytokinin-activating enzyme promotes tuber formation in tomato. Curr. Biol. 23, 1057–1064. doi: 10.1016/j.cub.2013.04.061
Guivarc’h, A., Rembur, J., Goetz, M., Roitsch, T., Noin, M., Schmülling, T., et al. (2002). Local expression of the ipt gene in transgenic tobacco (Nicotiana tabacum L. cv. SR1) axillary buds establishes a role for cytokinins in tuberization and sink formation. J. Exp. Bot. 53, 621–629. doi: 10.1093/jexbot/53.369.621
Hallmark, H. T., and Rashotte, A. M. (2019). Review - Cytokinin Response Factors: responding to more than cytokinin. Plant Sci. 289, 110251. doi: 10.1016/j.plantsci.2019.110251
Hannapel, D. J., Sharma, P., Lin, T., and Banerjee, A. K. (2017). The multiple signals that control tuber formation. Plant Physiol. 174, 845–856. doi: 10.1104/pp.17.00272
Hartmann, A., Senning, M., Hedden, P., Sonnewald, U., and Sonnewald, S. (2011). Reactivation of meristem activity and sprout growth in potato tubers require both cytokinin and gibberellin. Plant Physiol. 155, 776–796. doi: 10.1104/pp.110.168252
Heyl, A., and Schmülling, T. (2003). Cytokinin signal perception and transduction. Curr. Opin. Plant Biol. 6, 480–486. doi: 10.1016/s1369-5266(03)00087-6
Higuchi, M., Pischke, M. S., Mähönen, A. P., Miyawaki, K., Hashimoto, Y., Seki, M., et al. (2004). In planta functions of the Arabidopsis cytokinin receptor family. Proc. Nat. Acad. Sci. U.S.A. 101, 8821–8826. doi: 10.1073/pnas.0402887101
Hoyerová, K., and Hošek, P. (2020). New insights into the metabolism and role of cytokinin N-glucosides in plants. Front. Plant Sci. 11:741. doi: 10.3389/fpls.2020.00741
Hutchison, C. E., Li, J., Argueso, C., Gonzalez, M., Lee, E., Lewis, M. W., et al. (2006). The Arabidopsis histidine phosphotransfer proteins are redundant positive regulators of cytokinin signaling. Plant Cell 18, 3073–3087. doi: 10.1105/tpc.106.045674
Kakimoto, T. (1996). CKI1, a histidine kinase homolog implicated in cytokinin signal transduction. Science 274, 982–985. doi: 10.1126/science.274.5289.982
Kamada-Nobusada, T., and Sakakibara, H. (2009). Molecular basis for cytokinin biosynthesis. Phytochem. 70, 444–449. doi: 10.1016/j.phytochem.2009.02.007
Kieber, J. J., and Schaller, G. E. (2014). Cytokinins. Arabidopsis Book 12:e0168. doi: 10.1199/tab.0168
Kieber, J. J., and Schaller, G. E. (2018). Cytokinin signaling in plant development. Development 145:dev149344. doi: 10.1242/dev.149344
Kim, A., Chen, J., Khare, D., Jin, J. Y., Yamaoka, Y., Maeshima, M., et al. (2020). Non-intrinsic ATP-binding cassette proteins ABCI19, ABCI20 and ABCI21 modulate cytokinin response at the endoplasmic reticulum in Arabidopsis thaliana. Plant Cell Rep. 39, 473–487. doi: 10.1007/s00299-019-02503-0
Kolachevskaya, O. O., Alekseeva, V. V., Sergeeva, L. I., Rukavtsova, E. B., Getman, I. A., Vreugdenhil, D., et al. (2015). Expression of auxin synthesis gene tms1 under control of tuber-specific promoter enhances potato tuberization in vitro. J. Integr. Plant Biol. 57, 734–744. doi: 10.1111/jipb.12314
Kolachevskaya, O. O., Lomin, S. N., Arkhipov, D. V., and Romanov, G. A. (2019a). Auxins in potato: molecular aspects and emerging roles in tuber formation and stress resistance. Plant Cell Rep. 38, 681–698. doi: 10.1007/s00299-019-02395-0
Kolachevskaya, O. O., Lomin, S. N., Kojima, M., Getman, I. A., Sergeeva, L. I., Sakakibara, H., et al. (2019b). Tuber-specific expression of two gibberellins oxidase transgenes from Arabidopsis regulates over wide ranges the potato tuber formation. Russ. J. Plant Physiol. 66, 984–991. doi: 10.1134/S1021443720010094
Kolachevskaya, O. O., Sergeeva, L. I., Floková, K., Getman, I. A., Lomin, S. N., Alekseeva, V. V., et al. (2017). Auxin synthesis gene tms1 driven by tuber-specific promoter alters hormonal status of transgenic potato plants and their responses to exogenous phytohormones. Plant Cell Rep. 36, 419–435. doi: 10.1007/s00299-016-2091-y
Kolachevskaya, O. O., Sergeeva, L. I., Getman, I. A., Lomin, S. N., Savelieva, E. M., and Romanov, G. A. (2018). Core features of the hormonal status in in vitro grown potato plants. Plant Signal. Behav. 13:e1467697. doi: 10.1080/15592324.2018.1467697
Liu, C. J., Zhao, Y., and Zhang, K. (2019). Cytokinin transporters: multisite players in cytokinin homeostasis and signal distribution. Front. Plant Sci. 10:693. doi: 10.3389/fpls.2019.00693
Lomin, S. N., Krivosheev, D. M., Steklov, M. Y., Osolodkin, D. I., and Romanov, G. A. (2012). Receptor properties and features of cytokinin signaling. Acta Nat. 4, 31–45. doi: 10.32607/20758251-2012-4-3-31-45
Lomin, S. N., Myakushina, Y. A., Arkhipov, D. V., Leonova, O. G., Popenko, V. I., Schmülling, T., et al. (2018a). Studies of cytokinin receptor–phosphotransmitter interaction provide evidences for the initiation of cytokinin signalling in the endoplasmic reticulum. Funct. Plant Biol. 45, 192–202. doi: 10.1071/FP16292
Lomin, S. N., Savelieva, E. M., Arkhipov, D. V., and Romanov, G. A. (2020). Evidences for preferential localization of cytokinin receptors of potato in the endoplasmic reticulum. Biochem. (Moscow) Suppl. Ser. A Membr. Cell Biol. 14, 146–153. doi: 10.1134/S1990747820010079
Lomin, S. N., Myakushina, Y. A., Kolachevskaya, O. O., Getman, I. A., Arkhipov, D. V., Savelieva, E. M., et al. (2018b). Cytokinin perception in potato: new features of canonical players. J. Exp. Bot. 69, 3839–3853. doi: 10.1093/jxb/ery199
Miller, C. O., Skoog, F., Von Saltza, M. H., and Strong, F. M. (1955). Kinetin, a cell division factor from deoxyribonucleic acid. J. Am. Chem. Soc. 77:1392. doi: 10.1021/ja01610a105
Mizuno, T. (2005). Two-component phosphorelay signal transduction systems in plants: from hormone responses to circadian rhythms. Biosci. Biotechnol. Biochem. 69, 2263–2276. doi: 10.1271/bbb.69.2263
Nishimura, C., Ohashi, Y., Sato, S., Kato, T., Tabata, S., and Ueguchi, C. (2004). Histidine kinase homologs that act as cytokinin receptors possess overlapping functions in the regulation of shoot and root growth in Arabidopsis. Plant Cell 16, 1365–1377. doi: 10.1105/tpc.021477
Palmer, C. E., and Smith, O. E. (1970). Effect of kinetin on tuber formation on isolated stolons of Solanum tuberosum L. cultured in vitro. Plant Cell Physiol. 11, 303–314. doi: 10.1093/oxfordjournals.pcp.a074511
Pekárová, B., Szmitkowska, A., Dopitová, R., Degtjarik, O., Žídek, L., and Hejátko, J. (2016). Structural aspects of multistep phosphorelay-mediated signaling in plants. Mol. Plant 9, 71–85. doi: 10.1016/j.molp.2015.11.008
Pekárová, B., Szmitkowska, A., Houser, J., Wimmerova, M., and Hejátko, J. (2018). “Cytokinin and ethylene signaling,” in Plant Structural Biology: Hormonal Regulations, eds J. Hejátko, and T. Hakoshima (Basel: Springer). doi: 10.1007/978-3-319-91352-0_10
Potato Genome Sequencing Consortium [PGSC] (2011). Genome sequence and analysis of the tuber crop potato. Nature 475, 189–195. doi: 10.1038/nature10158
Riefler, M., Novak, O., Strnad, M., and Schmülling, T. (2006). Arabidopsis cytokinin receptor mutants reveal functions in shoot growth, leaf senescence, seed size, germination, root development, and cytokinin metabolism. Plant Cell 18, 40–54. doi: 10.1105/tpc.105.037796
Romanov, G. A. (2009). How do cytokinins affect the cell? Russ. J. Plant Physiol. 56, 268–290. doi: 10.1134/S1021443709020174
Romanov, G. A., Aksenova, N. P., Konstantinova, T. N., Golyanovskaya, S. A., Kossmann, J., and Willmitzer, L. (2000). Effect of indole-3-acetic acid and kinetin on tuberisation parameters of different cultivars and transgenic lines of potato in vitro. Plant Growth Regul. 32, 245–251. doi: 10.1023/A:1010771510526
Sakakibara, H. (2006). Cytokinins: activity, biosynthesis, and translocation. Annu. Rev. Plant Biol. 57, 431–449. doi: 10.1146/annurev.arplant.57.032905.105231
Schmülling, T., Werner, T., Riefler, M., Krupková, E., and Bartrina y Manns, I. (2003). Structure and function of cytokinin oxidase/dehydrogenase genes of maize, rice, Arabidopsis and other species. J. Plant Res. 116, 241–252. doi: 10.1007/s10265-003-0096-4
Sharma, P., Lin, T., and Hannapel, D. J. (2016). Targets of the StBEL5 transcription factor include the FT ortholog StSP6A. Plant Physiol. 170, 310–324. doi: 10.1104/pp.15.01314
Steklov, M. Y., Lomin, S. N., Osolodkin, D. I., and Romanov, G. A. (2013). Structural basis for cytokinin receptor signaling: an evolutionary approach. Plant Cell Rep. 32, 781–793. doi: 10.1007/s00299-013-1408-3
Swarbreck, D., Wilks, C., Lamesch, P., Berardini, T. Z., Garcia-Hernandez, M., Foerster, H., et al. (2008). The Arabidopsis Information Resource (TAIR): gene structure and function annotation. Nucleic Acids Res. 36, D1009–D1014. doi: 10.1093/nar/gkm965
Tessi, T. M., Brumm, S., Winklbauer, E., Schumacher, B., Pettinari, G., Lescano, I., et al. (2020). Arabidopsis AZG2 transports cytokinins in vivo and regulates lateral root emergence. New Phytol. doi: 10.1111/nph.16943 [Epub ahead of print].
Keywords: potato, cytokinin, two-component system, receptor, response regulator, gene expression, signaling chains, multistep phosphorelay
Citation: Lomin SN, Myakushina YA, Kolachevskaya OO, Getman IA, Savelieva EM, Arkhipov DV, Deigraf SV and Romanov GA (2020) Global View on the Cytokinin Regulatory System in Potato. Front. Plant Sci. 11:613624. doi: 10.3389/fpls.2020.613624
Received: 02 October 2020; Accepted: 19 November 2020;
Published: 21 December 2020.
Edited by:
Wolfram G. Brenner, Universität Leipzig, GermanyCopyright © 2020 Lomin, Myakushina, Kolachevskaya, Getman, Savelieva, Arkhipov, Deigraf and Romanov. This is an open-access article distributed under the terms of the Creative Commons Attribution License (CC BY). The use, distribution or reproduction in other forums is permitted, provided the original author(s) and the copyright owner(s) are credited and that the original publication in this journal is cited, in accordance with accepted academic practice. No use, distribution or reproduction is permitted which does not comply with these terms.
*Correspondence: Georgy A. Romanov, gromanov@yahoo.com