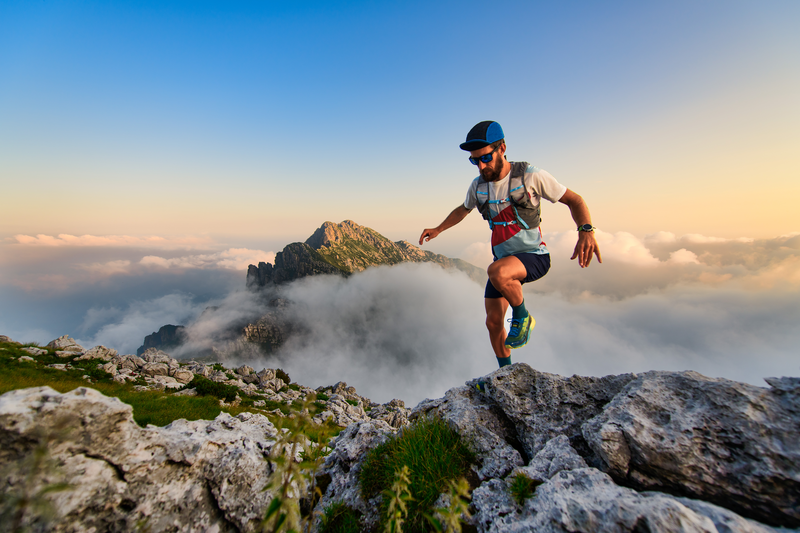
94% of researchers rate our articles as excellent or good
Learn more about the work of our research integrity team to safeguard the quality of each article we publish.
Find out more
ORIGINAL RESEARCH article
Front. Plant Sci. , 05 November 2020
Sec. Plant Systematics and Evolution
Volume 11 - 2020 | https://doi.org/10.3389/fpls.2020.594272
Dendroseris D. Don comprises 11 species endemic to the Juan Fernández islands in Chile. They demonstrate spectacular and unusual growth forms of rosette trees with extremely variable morphology and occupy wide ecological ranges on the islands. These unique plants are now highly threatened with extinction with very small population sizes, typically consisting of 10 or fewer individuals in wild. Despite morphological and ecological divergence among species of Dendroseris, their monophyly has been supported in previous studies, but with little resolution among subgeneric groups. We assembled seven complete plastome sequences from seven species of Dendroseris, including representatives from three subgenera, and carried out comparative phylogenomic analyses. The plastomes are highly conserved in gene content and order, with size ranging from 152,199 to 152,619 bp and containing 130 genes (87 coding genes, 6 rRNA genes, and 37 tRNA genes). Plastid phylogenomic analyses based on both the complete plastome sequences and 81 concatenated coding genes only show Dendroseris nested within Sonchus sensu lato, and also that inter-subgeneric relationships are fully resolved. Subg. Phoenicoseris is resolved as sister to the remaining species of the genus and a sister relationship between the two subgenera Dendroseris and Rea. Ten mutation hotspots from LSC and SSC regions and variable SSRs are identified as potential chloroplast markers for future phylogenetic and phylogeographic studies of Sonchus and related groups.
The currently circumscribed Sonchus subg. Dendroseris (D. Don) S.-C. Kim & Mejías (tribe Cichorieae; Asteraceae) includes 12 highly threatened island endemics to the Juan Fernández and the Desventuradas Islands in the Pacific Ocean. The Juan Fernández archipelago is composed of three volcanic islands, i.e., Robinson Crusoe (also known as Masatierra, located about 667 km west of continental Chile; 48 km2), Alejandro Selkirk (also known as Masafuera, 181 km further westward, 50 km2), and Santa Clara (close to Robinson Crusoe, only 2 km2). Located 750 km north of the Juan Fernández archipelago, the Desventuradas Islands consist of the small islands of San Ambrosio and San Félix, along with several small islets.
For several decades, these 12 species in subg. Dendroseris have been recognized at the generic rank (Dendroseris D. Don and Thamnoseris F. Phil.) in subtribe Dendroseridinae Benth. (Stebbins, 1953; Bremer, 1994). However, recent molecular phylogenetic studies of Sonchus and related genera revealed that Dendroseris (comprising 11 species on the Juan Fernández archipelago) is deeply embedded within the genus Sonchus (Kim et al., 1996a, b, 2007). As a consequence, Dendroseris and Thamnoseris were merged in Sonchus as one of four recognized subgenera in a newly delimited subtribe Hyoseridinae Less (Lack, 2007; Kilian et al., 2009; Mejías and Kim, 2012). The genus Sonchus is an ideal group to study patterns and processes of plant evolution given its diverse morphological and life history traits (e.g., weedy annuals/biennials, herbaceous perennials, and woody perennials) and peculiar geographic distribution (e.g., Atlantic and Pacific Oceans, Africa, and cosmopolitan).
Regardless of its taxonomic rank, the Dendroseris lineage has attracted numerous taxonomists and evolutionary biologists for nearly a century. Hereafter, while the lineage is nested within Sonchus, we treat Dendroseris in the traditional sense as a distinc genus. Within the typically herbaceous family Asteraceae, Dendroseris shows spectacular and unusual tree-like growth-forms with extremely variable morphology and occupies wide ecological ranges on the islands (Figure 1 and Table 1). The spectrum of life form ranges from palmiform rosette trees to sparsely branched rosette trees and succulent rosette shrubs (Carlquist, 1967). Initially, this diversity in growth forms led Skottsberg (1953) to recognize four segregate genera (Dendroseris, Rea, Hesperoseris, and Phoenicoseris), but recognition of one cohesive genus is now followed by most workers (e.g., Wodehouse, 1935; Stebbins, 1953). Anatomical and molecular evidence provides support for a species-level classification that concur with the three traditionally recognized subgenera within Dendroseris (Carlquist, 1967; Sang et al., 1994). Subsequent treatments have recognized three subgenera; (1) subg. Dendroseris Skottsb., comprising the four species D. litoralis Skottsb., D. macrantha (Bertero ex Decne.) Skottsb., D. macrophylla D. Don and D. marginata Hook. & Arn., (2) subg. Phoenicoseris Skottsb., with the three species D. berteroana (Decne.) Hook. & Arn., D. pinnata (Bertero ex Decne.) Hook. & Arn., and D. regia Skottsb., and (3) subg. Rea (Bertero ex Decne.) Skottsb., consisting of the four species D. gigantea Johow, D. micrantha Hook. & Arn., D. neriifolia Hook. & Arn., and D. pruinata (Johow) Skottsb. (Stuessy et al., 1984; Sanders et al., 1987; Crawford et al., 1992, 1998). These unique plants are now highly threatened with extinction, as they are exceedingly rare with very small population sizes in the wild (almost always fewer than 10 plants) and widely scattered (Skottsberg, 1953; Stuessy et al., 1998). Faced with the threats of extinction, all 11 Dendroseris species on the Juan Fernández archipelago have been categorized as Critically Endangered in the wild, CE BI +2c, on the IUCN Red List of Threatened Species (Walter and Gillett, 1998).
Figure 1. Species of Dendroseris from Robinson Crusoe Island in the Juan Fernández Archipelago, showing divergent morphological features. (A,B) D. litoralis (subg. Dendroseris); (C,D) D. micrantha (subg. Rea); (E,F) D. berteroana (subg. Phoenicoseris). Photo Credit: Tod F. Stuessy (Ohio State University, Columbus).
Table 1. Morphological and ecological characteristics of seven Dendroseris species sequenced and analyzed in this study.
Dendroseris on the Juan Fernández Islands has been the subject of numerous systematic and biogeographic studies over several decades as one of the most striking examples of adaptive radiation on oceanic islands in the Pacific Ocean (e.g., Skottsberg, 1953, 1956; Carlquist, 1967; Sanders et al., 1983, 1987; Crawford et al., 1987, 1992; Sang et al., 1994; Anderson et al., 2001; Bernardello et al., 2001). Despite the morphological, anatomical, and ecological divergence, Dendroseris has been strongly supported as a monophyletic group by several molecular markers (Sanders et al., 1983, 1987; Crawford et al., 1987, 1992; Spooner et al., 1987; Pacheco et al., 1991; Sang et al., 1994; Kim et al., 1996a, b; Esselman et al., 2000). Although the monophyly of Dendroseris was well-established, phylogenetic relationships among the three traditionally recognized taxonomic groups and species remained uncertain probably due to their rapid speciation. In addition, the phylogenetic position of Dendroseris relative to other Sonchus groups has been elusive (Sang et al., 1994; Kim et al., 1996a, b, 1999, 2007). While the two subgenera Dendroseris and Phoenicoseris were resolved as monophyletic, subg. Rea was not and relationships among the three subgenera were unresolved based on chloroplast (cpDNA) restriction fragment length polymorphisms (RFLPs), nuclear ribosomal DNA (nrDNA) internal transcribed spacer (ITS) sequences (Crawford et al., 1992; Sang et al., 1994), and allozymes (Crawford et al., 1998; Esselman et al., 2000). The neighbor-joining (NJ) tree based on randomly amplified polymorphic DNAs (RAPDs) showed the monophyly of each three subgenera, without further resolutions among them (Esselman et al., 2000). Lastly, the phylogenetic position of Dendroseris within the Sonchus group could not be determined confidently (Kim et al., 1999, 2007). In particular, the partial use of several coding and non-coding plastid regions in previous studies have been insufficient to provide robust phylogenetic relationships among Dendroseris and other closely related Sonchus groups (Sang et al., 1994; Kim et al., 1996a, b, 1999, 2007; Lee et al., 2005).
Fortunately, massive amounts of data have now become available with the advent of high-throughput sequencing technologies of next-generation sequencing (NGS) has revealed considerable genome-wide variation in sequences and structures of entire plastid genomes. The benefits of genome-wide data have increased phylogenetic resolution and significantly enhanced our understanding of plant evolution and diversity in the field of plastid genetics and genomics (Daniell et al., 2016). Whole plastome sequencing is now an efficient option for increasing phylogenetic resolution at lower taxonomic levels that are currently hindered by limited sequence variation due to recent divergence, rapid radiation and conservative genome evolution of plastomes (Parks et al., 2009).
In this study, we sequenced and assembled the whole plastid genomes of seven species of Dendroseris, representing three subgenera (Dendroseris, Rea, and Phoenicoseris) on the Juan Fernández Islands. Based on the complete plastome sequences, we tested the previous phylogenetic hypotheses proposed by various molecular markers, specifically focusing on inter-subgeneric relationships within the genus. We also performed comparative plastome analyses based on the phylogenetic framework to determine the structure, gene content, and rearrangements in the plastid genomes. Furthermore, we wanted to identify highly variable plastid regions and microsatellites or simple sequence repeats (SSRs), which could be utilized as useful markers for further population genetic or phylogeograhic studies of Dendroseris.
Plant materials of seven Dendroseris species were collected previously in the field during four expeditions of the Universidad de Concepción, Chile and the Ohio State University, United States to the Juan Fernández Islands. As acknowleged in the previous studies (Crawford et al., 1987, 1992; Pacheco et al., 1991), the CONAF (Corporación National Forestal) of Chile issued the permission to collect in the Robinson Crusoe National Park. Our current samples of Dendroseris are representatives of those previous collections. For outgroup Reichardia ligulata, we used the same material as previously studied (Kim et al., 1996b, 2007). The fresh leaves were either dried (placed in sealable plastic bags with silica gel) or placed on ice and retained at 4°C until extracted in the laboratory at The Ohio State University, Columbus, OH, United States. Total genomic DNAs were extracted using the CTAB technique of Doyle and Doyle (1987), and purified in CsCl/ethidium bromide gradient.
Illumina paired-end (PE) genomic libraries with fragment size of 550 bp were prepared and sequenced using the Illumina HiSeq platform (Illumina, Inc., San Diego, CA, United States) at Macrogen Corporation (Seoul, South Korea). The sequence contigs were assembled by the de novo genomic assembler, Velvet 1.2.10 (Zerbino and Birney, 2008) at coverage ranging from 429 to 1489x. Annotation was performed using the Dual Organellar GenoMe Annotator (Wyman et al., 2004), ARAGORN v1.2.36 (Laslett and Canback, 2004), and RNAmmer 1.2 Server (Lagesen et al., 2007). Using Geneious v8.1.6 (Biomatters, Ltd., Auckland, New Zealand), the draft annotation was inspected and corrected manually, performing blast search by comparison with homologous genes in Lactuca sativa (DQ383816), S. canariensis (NC042381), S. acaulis (NC042382), and S. webbii (NC042383) from the GenBank database at the National Center for Biotechnology Information (NCBI) as references. The complete plastome sequences were registered in GenBank under the accession numbers MN893255 (R. ligulata from Spain, Collection # TE); MK371014 (D. berteroana, Collection # 12125); MK371011 (D. litoralis, Collection # 6435); MT157218 (D. marginata, Collection # 12068); MK371008 (D. micrantha, Collection # 11944); MT157219 (D. pinnata, Collection # 11132); and MK371005 (D. pruinata, Collection # 5108). OGDRAW (Lohse et al., 2013) was used to draw circular plastid genome maps (Figure 2).
Figure 2. Gene maps of the plastid genomes of seven Dendroseris and Reichardia ligulata sequenced and analyzed in this study. The other six Dendroseris plastomes were consistent with D. litoralis in the structures and gene contents, but differed in their total length, having 152,263 bp for D. macrantha (MT157217), 152,261 bp for D. marginata (MT157218), 152,290 bp for D. pinnata (MT157219), 152,327 bp for D. micrantha (MK371008), 152,199 bp for D. berteroana (MK371014), and 152,348 bp for D. pruinata (MK371005). The genes inside and outside of the circle are transcribed in the clockwise and counterclockwise directions, respectively. Genes belonging to different functional groups are shown in different colors. The thick lines indicate the extent of the inverted repeats that separate the genomes into small single copy (SSC) and large single copy (LSC) regions.
We performed several comparative plastome analyses among Dendroseris and other closely related Sonchus species on the Canary Islands, Atlantic Ocean. The analyses also included two related Sonchus species (S. asper and S. canariensis). Sonchus asper is a globally distributed herbaceous weed and S. canariensis is an arborescent shrub endemic to the Canary Islands. Codon usage frequency was calculated by using MEGA7 (Kumar et al., 2016) with relative synonymous codon usage (RSCU) value, which is the relative frequency of occurrence of the synonymous codon for a specific amino acid. The online program predictive RNA editor for plants (PREP) suite (Mower, 2009) was used to predict the possible RNA editing sites for annotated protein-coding genes with 35 reference genes available with known edit sites, based on a cutoff value of 0.8 (suggested as optimal for PREP-Cp). Overall sequence divergence was estimated using the LAGAN alignment mode (Brudno et al., 2003) in mVISTA (Frazer et al., 2004). Nucleotide diversity (Pi) was calculated using the sliding window analysis (window length = 1000 bp and step size = 200 bp excluding sites with alignment gaps) to detect the most divergent regions (i.e., mutation hotspots) in DnaSP (Librado and Rozas, 2009).
Two types of repeat sequences were identified in the eight plastid genomes of seven species of Dendroseris and R. ligulata. REPuter (Kurtz et al., 2001) was used to detect the various types of repetitive sequences with search parameters set to: maximum computed repeats = 50, minimum repeat size = 8 bp, and hamming distance = 1. SSRs were identified using MISA web1 with search parameters of 1–15 (unit size-minimum repeats, i.e., mono-nucleotide motifs with 15 minimum numbers of repetition), 2–5, 3–3, 4–3, 5–3, and 6–3 with 100 interruption (maximum difference for two SSRs).
Phylogenetic relationships of the newly sequenced accessions of Dendroseris were investigated with other closely related Sonchus species using R. ligulata as the outgroup. Seven representative complete plastid sequences belonging to the major lineages of Sonchus were obtained from GenBank, including the woody Sonchus alliance species (Sonchus subg. Dendrosonchus) in Macaronesian Islands and globally occurring herbaceous weedy species (Sonchus subg. Sonchus). In total, full sequences of 15 plastid genomes, including an outgroup taxon, R. ligulata, were aligned using MAFFT v.7 (Katoh and Standley, 2013). Maximum likelihood trees based on both the complete plastid genome sequences and the concatenated sequences of 81 coding genes (excluding six repeated in IR) were produced with 1000 replicate bootstrap (BS) analyses by IQ-TREE (Nguyen et al., 2014). The best fit evolutionary model was chosen as TVM + F + I, which was scored according to the Bayesian information criterion (BIC) scores and weights by testing 88 DNA models of ModelFinder (Kalyaanamoorthy et al., 2017) implemented in IQ-TREE.
Despite the great morphological and ecological differences among them, the seven plastomes of Dendroseris species and one outgroup taxon, R. ligulata were highly conserved in gene content and arrangement, displaying 99.3% pairwise similarity in sequences (99.8% among seven Dendroseris species only) (Figures 2, 3). Within Dendroseris, the total length of seven plastomes ranged from 152,199 (D. berteroana; subg. Dendroseris) to 152,348 (D. pruinata; subg. Rea) base pairs (bp), and consisted of four typical regions: large single copy (LSC), small single copy (SSC), and a pair of inverted repeat (IR) regions. The overall guanine-cytosine (GC) content of each plastid genome was 37.6%, with LSC, SSC, and IR regions having 35.7–35.8, 31.1–31.3, and 43.1% GC contents, respectively (Table 2). Each of the eight cp genomes contained 130 genes, including 87 protein-coding genes (excluding pseudogenes), six rRNA genes, and 37 tRNA genes (Table 3). Eighteen genes contained introns, including seven tRNA genes. Three genes of clpP, rps12, and ycf3 exhibited two introns. The trnK-UUU tRNA gene harbored the largest intron, which contained the matK gene in between. In total, 17 genes were duplicated in the IR regions, including seven tRNAs, three rRNAs, and seven protein genes. The trans-splicing gene rps12, consisting of three exons, was located in the LSC region for exon 1, but exon 2 and exon 3 of the gene were imbedded in the IR regions. Part of ycf1 and rps19 duplicated in IR region were annotated as pseudogenes in all cp genomes sequenced in this study.
Figure 3. Comparison of the border positions of the large single copy (LSC), small single copy (SSC), and inverted repeat (IR) regions among seven Dendroseris and Reichardia ligulata plastid genomes. Gene names are indicated in boxes, and their lengths in the corresponding regions are displayed above the boxes. Ψ indicates a pseudogene.
Table 2. Genomic features of the complete plastid genomes of seven Dendroseris species and Reichardia ligulata sequenced and analyzed in this study.
Table 3. Genes present in the complete plastid genomes of seven Dendroseris species and R. ligulata sequenced in this study.
The frequency of codon usage was calculated based on the sequences of protein coding genes, of which RSCU (the relative frequencies of occurrence of the synonymous codon usages for a specific amino acid) values were reported in Supplementary Figure 1 (see Supplementary Table 1 for details). The 10 plastomes including seven Dendroseris, two Sonchus species (S. asper and S. canariensis), and outgroup taxon, R. ligulata showed very similar frequencies of codon usage despite morphological and evolutionary divergence among them. We found that all possible codons for all amino acids were used in their plastomes as specified in Supplementary Table 1. The highest RSCU value was in the usage of AGA codon for arginine (1.88) followed by UUA for leucine (1.81–1.82), while the lowest one, AGC for serine (0.34–0.35) followed by CUG for leucine (0.37–0.39) among all of them in common.
The total number of possible RNA editing sites predicted among 10 plastomes ranged from 93 to 104 sites in 35 protein-coding genes. Compared to the herbaceous species S. asper (98 sites) and R. ligulata (93 sites), most woody species of Dendroseris species and S. canariensis showed relatively more numbers of RNA edited sites (102–104 sites) except for D. berteroana (99 sites). The numbers of potential editing sites were not correlated with gene length; the highest numbers of potential editing sites were found in the psaB gene (10–12 sites), followed by the ndhB gene (9–10 sites). Only minor sites were predicted for longer genes, i.e., two sites for the longest gene rpoC2 and one for the second longest rpoB. Any potential RNA edited sites were not predicted for seven genes of atpF, petD, petG, petL, psaI, psbB, and psbF consistently from all analyzed plastomes. R. ligulata differed at two more codon position in the psaB gene, from serine (S) to leucine (L), and from leucine (L) to phenylalanine (F), while S. asper changed one more codon position in the ndhB gene, from leucine (L) to phenylalanine (F) when compared with the rest of the species of Dendroseris and Sonchus. Most editing sites were distributed at the 2nd and 1st codon positions (Supplementary Table 2). The highest conversions in editing frequencies of codons associated with the corresponding amino acid changes were represented by the changes from proline (P) to leucine (L) (average score of 21.606) followed by serine (S) to leucine (L) (average score of 19.12) (Supplementary Figure 2).
The divergence level of nucleotide diversity was compared using DnaSP in both ways among seven Dendroseris plastomes only vs. among 10 plastomes including reference plastomes of Sonchus species (S. asper and S. canariensis) and the outgroup species R. ligulata. Overall nucleotide diversity value (Pi) among 10 plastomes (average value of 0.00283, ranging from 0 to 0.01593) was much higher than the one comparing seven Dendroseris plastid genomes (average value of 0.00061, ranging from 0 to 0.0039). The SSC regions, where the most variable gene, ycf1 was located, showed the highest nucleotide diversity in both analyses (0.00573 for 10 plastomes vs. 0.00121 for seven of Dendroseris), while the lowest value was in the IR boundary regions (0.00083 vs. 0.0003). Ten divergence hotspots among 10 plastomes were suggested as potential plastid markers for phylogenetic studies of Dendroseris species and closely related Sonchus groups. Eight intergenic regions (trnS-trnC, trnC-petN, trnT-trnL, trnL-trnF, ndhC-trnC, psbE-petL, ycf1-rps15, and rpl32-ndhF), one intron region (rpl16 intron), and one protein coding region (ycf1) were found in LSC and SSC regions (Figure 4). Additionally, seven hot spots identified among Dendroseris plastomes were found in LSC and SSC regions (Supplementary Figure 3).
Figure 4. Ten most hotspot regions found in seven Dendroseris, two Sonchus (S. asper, and S. canariensis), and Reichardia ligulata.
The result of mVISTA plotted against R. ligulata also exhibited a high degree of synteny and gene order conservation among the plastomes of Dendroseris and Sonchus species (Figure 5). A total of 1,873 polymorphic sites, which were identified in the DnaSP analysis, were visualized in mVISTA graph from mostly non-coding and intron regions, but also from several protein coding regions. The divergent coding genes of Dendroseris and Sonchus species against R. ligulata were atpA, accD, rpoA, ycf2, ycf1, and ndhF.
Figure 5. Comparison of the plastid genomes of seven Dendroseris and two Sonchus species, against R. ligulata by mVISTA. Gray arrows indicate genes with their orientation and position. Genome regions are color-coded as blue blocks for the conserved coding genes (exon), aqua blue blocks for introns, and orange blocks for the conserved non-coding sequences in intergenic regions (CNS). Thick lines below the alignment indicate the quadripartite regions of genomes; LSC region is in beige, IR regions, in pink, and SSC region, in light blue.
All of the eight plastid genomes of Dendroseris and R. ligulata contained very similar numbers and distribution patterns of repeated sequences. There were 71–74 SSRs detected by MISA based on search parameters set for 1–15 (mono-nucleotide motifs with 15 minimum numbers of repetition), 2–5, 3–3, 4–3, 5–3, and 6–3. The vast majority of the SSRs were tri-nucleotide motifs (63–64 SSRs, 87%), while other repeat types were relatively fewer; one to two mono-nucleotide motifs (3%), three to four di-nucleotide motifs (5%), and three to four tetra-nucleotide motifs (5%) (Figure 6A). The most abundant repeat motif was ‘AAT/ATT’ (30%) followed by ‘AAG/CTT’ (29%) in all eight genomes (Figure 6B and Supplementary Table 3). Interestingly, SSRs were distributed most frequently in the coding regions (53%), followed by intergenic regions (42%), with much lower numbers found in the non-coding introns (5%) in each cp genome (Supplementary Table 4). The coding regions with highest number of SSRs were ycf genes; 10 SSRs (five duplicated in each IR) in ycf2 and two in ycf1 (in SSC). Considering the quadripartite regional occupancy of SSRs, the IR and SSC regions were lower in overall SSR frequency compared with the LSC region; 15% from the SSC region and 18% from each of both IR regions versus 62% from the LSC region (Supplementary Table 4). Additionally, we found 50 pairs of large repeats in each cp genome (excluding duplicated IR region) using the parameters of maximum computed repeats = 50, minimum repeat size = 8 bp, and hamming distance = 1 by REPuter. They contained 19–22 forward, 8–10 reverse, and 19–23 palindromic matches of repeats (Figure 7A). Most of these large repeats were present in the intergenic spacers, but five repeat matches were found within the ycf coding genes, two in ycf1 and three in ycf2. Lengths of 21–25 repeats were the most frequent (37%) followed by lengths of 19–20 repeats (35%), while longer repeats of 26–30 (17%) and 39–58 (11%) were rarer than shorter ones (Figure 7B).
Figure 6. Simple sequence repeat (SSR) number per distribution and repeat type of the plastid genomes of seven Dendroseris and Reichardia ligulata. (A) Variation in the numbers of SSRs per distribution pattern. (B) Number of SSR motifs in different repeat motifs.
Figure 7. Repeat numbers per repeat type and repeat length of eight plastid genomes of seven Dendroseris and Reichardia ligulata. (A) Variation in the distribution of forward, reverse, complement, and palindromic repeats. (B) Number of different repeat lengths.
The complete plastome sequences provide good resolution of inter-subgeneric and interspecifc relationships within Dendroseris as well as resolving relationships among three major lineages within Sonchus sensu lato (Figure 8). Two maximum likelihood (ML) trees based on the complete sequences and the protein-coding genes only confirmed the monophyly of Dendrosonchus, Dendroseris, and Sonchus (100% BS) (Figure 8). However, phylogenetic relationships among three lineages within Sonchus sensu lato and within Dendroseris were unresolved between two ML trees. The complete plastome sequences suggested sister relationship between Dendroseris and Sonchus (92% BS; Figure 8A), while protein-coding genes showed sister relationships between Dendroseris and Dendrosonchus (56%; Figure 8B). In terms of relationships within Dendrosonchus from the Canary Islands, both trees showed that herbaceous perennial species with tuberous roots, S. webbii, diverged first within the group and also that pachycaulous S. acaulis is sister to the tree species S. canariensis (Figure 8). Within Sonchus, the newly described herbaceous perennial species S. boulosii from Morocco is sister to the cosmopolitan herbaceous weedy species S. asper and S. oleraceus.
Figure 8. Maximum likelihood tree of Dendroseris and closely related Sonchus based on the complete plastome sequences (A) and 81 concatenated protein-coding genes (B). Numbers above nodes are bootstrap values with 1000 replicates. The double slash (“//”) indicates that the branch lengths between outgroup and ingroup are shortened by one third size to improve readability of trees. Newly sequenced eight plastid genomes in this study are marked with an asterisk (*).
The whole plastid genomes of Dendroseris species reported for the first time in this study were highly conserved structurally, sharing most common genomic features such as sequence similarity, gene content and numbers, and distributions of repeated sequences despite their morphological and ecological divergences. Interestingly, their genomic features are also very similar to other Sonchus groups with drastically different habits or geographical distribution (i.e., the woody Sonchus alliance of Dendrosonchus in the Macaronesian Islands, Atlantic Ocean and the globally distributed weedy sow thistles of Sonchus sensu stricto). Minor differences were found only in the total length of the herbaceous weedy species (Sonchus s. str.), which were shorter (151,808 ∼ 151,849 bp) than the woody shrubs and trees of Dendrosonchus (152,071 ∼ 152,194 bp) and Dendroseris (152,199 ∼ 152,620 bp), the differences due mainly to the SSC and LSC sizes (Cho et al., 2019a, b). Generally, the length of the plasid genome and its quadripartite regions varies among plant lineages due to the contraction and expansion of the inverted repeat regions. Evaluating their contraction and expansion by comparing the location of the boundaries among the four regions can provide some insights into plastid evolution (Menezes et al., 2018). The lengths of inverted repeats in the plastids of Dendroseris, Dendrosonchus, and Sonchus s. str. were very similar as a result of the complete duplication of: the genes rpl2, rpl23, ycf2, ycf15, ndhB, rps7; exons 1 and 2 of rps12; all three rRNA genes (5S, 16S, and 23S); and seven tRNA genes (trnLCAA, trnMCAU, trnVGAC, trnAUGC, trnEUUC, trnRACG, and trnNGUU). Furthermore, the boundaries among the four regions IRs, LSC and SSC were fairly conserved, sharing exactly the same genes and similar gene contents at all junctions. The SSC region has been shown to exist in two equimolar states within individual plants differing only in the relative orientation of their single copy sequences (Palmer, 1983). Since the orientation of the SSC region is not standardized in GenBank (Walker et al., 2014, 2015), we reported one particular orientation of the SSC region for the comparative analysis in Dendroseris, Dendrosonchus, and Sonchus. All of them contained the functional protein coding gene of ycf1ψ at IR/SSC with its pseudogene copy, ycf1ψ at SSC/IR, and functional rps19 at LSC/IR with pseudogene copy rps19Ψ at IR/LSC endpoints (Figure 3). Such a high conservation of plastid organization further corroborates that they share the most recent common ancestors, which has been previously suggested (Kim et al., 1996a, b, 2007; Kilian et al., 2009).
The frequency of codon usage vary by factors in species-specific ways, showing different preferences for the codons used to encode specific amino acids, probably as the result of evolution in the presence of mutational biases, selection for translation rate and accuracy, and possibly other factors (Orešiè and Shalloway, 1998). The patterns of frequently used codons were the same among Dendroseris, Dendrosonchus, and Sonchus, and only showed slightly different RSCU (the relative frequencies of occurrence of the synonymous codon usages for a specific amino acid) values. RNA editing alters plastid transcripts by converting specific cytidines to uridines, which results in a change in the amino acid sequence of the translated protein (Mower, 2009). In this study, several protein-coding genes showed variances in RNA editing prediction. The herbaceous weedy S. asper displayed less RNA editing at a total of 98 sites, especially for the genes rpl20 and matK, compared to the woody Dendrosonchus species, S. canariensis (104 sites), and Dendroseris species (ranging from 99 to 104 sites). The dissimilarity in the RNA editing of the cosmopolitan weedy S. asper may originate from its different growth habit, but this needs further confirmation based on wider sampling because the woody species of Dendroseris on the Juan Fernández Islands exhibited quite diverse RNA editing prediction including D. berteroana (99 sites) with very similar RNA editing as herbaceous S. asper. As for SSRs and large repeat sequences, Dendroseris showed patterns comparable to Dendrosonchus and Sonchus in the numbers and frequencies of repeat type (Cho et al., 2019a, b). In addition, all showed an abundance of tri-nucleotide SSRs (over 80%), which is also consistent with other weedy Asteraceae species such as Ambrosia trifida (Sablok et al., 2019), and the highest frequencies of SSRs from the LSC region. Furthermore, the similar distribution pattern of large repeats was also observed for majority in forward (F) and palindromic (P) matches from Dendroseris (F = 19∼22, P = 19∼23 out of a total of 50 pairs) and herbaceous weedy Sonchus (F = 21, P = 21 out of total 49 pairs) (Cho et al., 2019b).
The nucleotide diversity (Pi value) was quite low within Dendroseris (average 0.00061) and Dendrosonchus (0.00090), and increased to 0.00117 for Dendrosonchus and Sonchus s. str., and to 0.00283 for Dendroseris, Dendrosonchus, and Sonchus (Cho et al., 2019a, b). Given the highly conserved nature of the plastid genome in most angiosperms and the relatively recent origin of Dendroseris species on the Juan Fernández Islands (estimate ranging from 800,000 to 2.6 million years) (Crawford et al., 1992; Sang et al., 1994; Daniell et al., 2016), the low nucleotide diversity within Dendroseris is not surprising. Further, the overall patterns for highly variable regions were similar among the taxa. The most divergent hot spot was the ycf1 region followed by rpl32-ndhF, trnT-trnL-trnF, and psbE-petL, which were suggested as potential cp markers for phylogenetic studies of Sonchus and closely related groups (Figure 4 and Supplementary Figure 3). Of 10 highly variable regions based on complete plastid genomes across diverse angiosperm lineages (Shaw et al., 2014), only three regions, i.e., rpl32-trnL, psbE-petL, and ndhF-rpl32, were found to be also highly variable in Dendroseris. In this study, we identified variable molecular markers including SSRs and highly variable regions from plastid genomes, which will increase the efficiency and feasibility for species identification and phylogenetic reconstruction within Sonchus. The ML tree constructed from the concatenated sequences of 10 mutation hotspot regions (12,879 bp in length) demonstrated their effectiveness as potential molecular markers, and for resolving with high support values inter-subgeneric relationships within Dendroseris (except subg. Rea) as well as their relationships to other Sonchus groups (Supplementary Figure 4).
Considering the overall high conservation among the plastomes of Sonchus and closely related species, it is not surprising that previous studies based on relatively few sequences from the plastid genomes provided limited resolution of relationships. Although Dendroseris was confirmed to be monophyletic in a matK phylogeny, its relationships to other Sonchus groups and inter-subgeneric relationships within Dendroseris were not well-resolved (Kim et al., 2007). Here, the phylogenomic analyses of entire plastid sequences revealed better resolution among the three lineages sampled (i.e., Dendrosonchus, Dendroseris, and Sonchus). In agreement with earlier results, in the present study Dendroseris was nested deeply within the genus Sonchus sensu lato on both ML trees (Figures 8A,B). Therefore, Dendroseris was most likely derived from the Sonchus group, but its closest relatives or progenitors remain to be determined based on broader sampling of plastid sequences. Especially, to be desired are other Pacific Island endemics, and additional taxa of subg. Sonchus sections Maritimi and Arvenses because results of a prior study showed that species from these groups share the most recent common ancestor with Dendroseris (Kim et al., 2007). Since all Dendroseris have a diploid chromosome number of 36 (Sanders et al., 1983) and there is no evidence of multivalent formation (Sanders et al., 1983), suggestive of an autopolyploid (Jackson, 1982; Ramsey and Schemske, 2002), it can be considered allotetraploid. Given the higher frequency of polyploid Sonchus species in the Pacific Ocean compared to typically diploid ones in the Atlantic Ocean and Old World, the geographical origin of Dendroseris is likely somewhere in the Pacific Ocean (e.g., Australia, New Zealand, etc.) and adjacent regions (Kim et al., 2007). However, its origin is still enigmatic; it remains to be determined which lineages of Sonchus contributed to allotetraploid origin of Dendroseris.
Unlike the uncertain position of Dendroseris relative to other Sonchus groups, this study provides strong evidence of relationships among the three subgenera Dendroseris, Rea, and Phoenicoseris. Previous plastid phylogenies based on the matK gene, the psbA-trnH intergenic spacer and restriction site mutations showed unresolved inter-subgeneric relationships, presumably the result of rapid radiation and speciation following the arrival of the common ancestor (Crawford et al., 1992; Kim et al., 1999, 2007). Other types of molecular markers, such as RAPDs (Esselman et al., 2000), ITS sequences (Sang et al., 1994), and allozymes (Crawford et al., 1998) failed to resolve relationships among the three subgenera. The initial divergence of subg. Dendroseris (D. litoralis, D. marginata, and D. macrantha) in the maximum likelihood tree based on ITS sequences is not significant, leaving inter-subgeneric relationships unresolved. However, species relationships within each subgenus have been strongly and consistently recognized regardless of different marker types. For example, a sister relationship between D. litoralis and D. marginata of subg. Dendroseris has been consistently recognized. Despite morphological and molecular similarities due to common ancestry, the species are ecologically and altitudinally well-differentiated: D. litoralis occurs in coastal lower elevations and D. marginata is found on higher elevation exposed cliffs (Stuessy et al., 1998). The second species pair of subg. Rea, D. pruinata and D. micrantha, also shows marked ecological and altitudinal differentiation: the former species commonly in middle elevation forests and the latter one in open lower coastal areas or open higher elevation windy cliffs (Sanders et al., 1987). Lastly, the species pair of subg. Phoenicoseris, D. beteroana and D. pinnata, are morphologically and ecologically quite divergent despite sharing the most recent common ancestor: the former in high altitude tree/fern forests and the latter in open wind-swept ridges at higher elevations. Therefore, all these species pairs suggest that spatial/ecological/altitudinal factors likely promoted the divergence and speciation of this largest and fascinating group on the Juan Fernández Islands.
The current whole plastid phylogenomic study shows the unresolved relationship of D. marginata within subg. Dendroseris. The phylogenetic tree suggests that D. marginata is either sister to the clade containing the subgenera Dendroseris and Rea (complete plastome sequence tree; Figure 8A) or is sister to the clade of D. litoralis and D. macrantha of subg. Dendroseris (protein coding genes only tree; Figure 8B). Given that the subg. Dendroseris is monophyletic based on various molecular markers and morphology, the tree based on protein coding genes only most likely represents the most plausible hypothesis of species relationships. In addition, our current study suggested that D. litoralis is more closely related to D. macrantha than to D. marginata. It has been demonstrated that, based on morphological traits, D. macrantha is most closely related to D. macrophylla on the geologically younger island Masafuera (Alejandro Selkirk), while flavonoid profiles provided no specific insights (Pacheco et al., 1991). The current study strongly suggests a sister relationship between D. litoralis and D. macrantha (100% BS). However, this relationship contrasts with morphological traits: D. litoralis has shorter morphological distance with D. marginata (two features) compared to the distance with D. macrantha (four features) (Sanders et al., 1987; Pacheco et al., 1991). It is probable that the close relationship of D. litoralis and D. macrantha based on the plastid genomes could be the result of past gene flow since D. macrantha is from cultivated material in the village and also morphology, especially leaf margin, is suggestive of gene flow event (TF Stuessy, personal observation).
Based on fully resolved inter-subgeneric relationships inferred in this study, we can hypothesize some processes with regard to the divergence and speciation of Dendroseris on the Juan Fernández Islands (Figure 8B). Upon arrival of the common ancestor on the older Robinson Crusoe island, two major lineages might have diverged; one lineage (subg. Phoenicoseris) toward higher elevation and the other lineage containing the two subgenera Dendroseris and Rea toward middle and lower elevations. Subgenus Phoenicoseris was considered to be highly derived within the genus based on morphology, with the characteristics of compound pinnatifid leaves, single-stemmed habit and being monocarpic (Sanders et al., 1987). In addition, subg. Rea was regarded as the most primitive subgenus based on morphological features and occurrence in middle elevation forests, and more open, arid habitats (Sanders et al., 1987). The current study, however, does not necessarily support the hypothesis that Phoenicoseris is highly derived, which was based on morphological features. Rather, the complete plastid sequences strongly suggest that after initial divergence of the two major lineages, the subg. Phoenicoseris lineage speciated at higher elevations with modification in some life history traits (e.g., monocarpic). A sister relationship between Dendroseris and Rea has never been postulated based on various molecular markers and, given their ecological preferences (Dendroseris in lower elevations along drier seacoasts and cliffs in full sun versus Rea in middle elevation in the edges of the cooler forests), it is plausible that the evolution of these two subgenera progressed from lower to middle elevations of the island.
We characterized the first complete plastid sequences of seven species of Dendroseris, the largest endemic genus on the Juan Fernández Islands. As in most angiosperms, we found highly conserved plastomes at the generic level, including gene order and content. Despite the recent origin of Dendroseris species on the islands and the low rate of plastome evolution, we achieved the first fully resolved phylogeny for the genus. Especially, noteworthy was the complete resolution with strong support of relationships among the three subgenera. The plastid phylogenomics strongly suggest early divergence of two major lineages, one consisting of subg. Phoenicoseris and the other the clade comprised of subgenera Dendroseris and Rea. Although we achieved full resolution within the genus, questions remain such as the geographical origin within several lineages of Sonchus in the Pacific Ocean, the monophyly of subg. Rea, and the timing of the origin and radiation of the lineage in the archipelago. Our thorough characterization and comparative analyses among the plastid genomes have led us to discover several informative mutation hot spots and variable SSR regions, which can be used to identify and characterize each individual in these highly threatened and nearly extinct species of Dendroseris.
The datasets presented in this study can be found in online repositories. The names of the repository/repositories and accession number(s) can be found below: https://www.ncbi.nlm.nih.gov/genbank/, MN893255; https:// www.ncbi.nlm.nih.gov/genbank/, MK371011; https://www.ncbi. nlm.nih.gov/genbank/, MT157217; https://www.ncbi.nlm. nih.gov/genbank/, MT157218; https://www.ncbi.nlm.nih.gov/ genbank/, MK371005; https://www.ncbi.nlm.nih.gov/genbank/, MK371008; https://www.ncbi.nlm.nih.gov/genbank/, MK371014; https://www.ncbi.nlm.nih.gov/genbank/, MT157219.
M-SC and S-CK designed the experiment. DC, TS, and PL-S collected the samples. M-SC, S-HK, and JY performed the experiments and analyzed the data. M-SC drafted the manuscript. DC, TS, and S-CK revised the manuscript. All authors approved the final version of the manuscript.
This project was supported by the Basic Science Research Program through the National Research Foundation of Korea (NRF) (NRF-2019R1A2C2009841) to S-CK.
The authors declare that the research was conducted in the absence of any commercial or financial relationships that could be construed as a potential conflict of interest.
We thank the herbarium of the Ohio State University (OS) for the permission to take leaf samples from specimens.
The Supplementary Material for this article can be found online at: https://www.frontiersin.org/articles/10.3389/fpls.2020.594272/full#supplementary-material
Anderson, G. J., Bernardello, G., Stuessy, T. F., and Crawford, D. J. (2001). Breeding system and pollination of selected plants endemic to Juan Fernández Islands. Am. J. Bot. 88, 220–233. doi: 10.2307/2657013
Bernardello, G., Anderson, G. J., Stuessy, T. F., and Crawford, D. J. (2001). A survey of floral traits, breeding systems, floral visitors, and pollination systems of the angiosperms of the Juan Fernández Islands (Chile). Bot. Rev. 67, 255–308. doi: 10.1007/bf02858097
Brudno, M., Do, C. B., Cooper, G. M., Kim, M. F., Davydov, E., Green, E. D., et al. (2003). NISC comparative sequencing program. LAGAN and multi-LAGAN: efficient tools for large-scale multiple alignment of genomic DNA. Genome Res. 13, 721–731. doi: 10.1101/gr.926603
Carlquist, S. J. (1967). Anatomy and systematics of Dendroseris (sensu lato). Brittonia 19, 99–121. doi: 10.2307/2805268
Cho, M. S., Kim, J. H., Kim, C. S., Mejías, J. A., and Kim, S.-C. (2019b). Sow thistle chloroplast genomes: insights into the plastome evolution and relationship of two weedy species, Sonchus asper and Sonchus oleraceus (Asteraceae). Genes 10:881. doi: 10.3390/genes10110881
Cho, M. S., Yang, J. Y., Yang, T. J., and Kim, S.-C. (2019a). Evolutionary comparison of the chloroplast genome in the woody Sonchus Alliance (Asteraceae) on the Canary Islands. Genes 10:217. doi: 10.3390/genes10030217
Crawford, D. J., Sang, T., Stuessy, T. F., Kim, S.-C., and Silva, M. O. (1998). “Dendroseris (Asteraceae: Lactuceae) and Robinsonia (Asteraceae: Senecioneae) on the Juan Fernandez islands: similarities and differences in biology and phylogeny,” in Evolution and Speciation of Island Plants, eds T. Stuessy and M. Ono (New York, NY: Cambridge University Press), 97–119. doi: 10.1017/cbo9780511721823.007
Crawford, D. J., Stuessy, T. F., Cosner, M. B., Haines, D. W., Silva, M. O., and Baeza, M. (1992). Evolution of the genus Dendroseris (Asteraceae: Lactuceae) on the Juan-Fernandez Islands: evidence from chloroplast and ribosomal DNA. Syst. Bot. 17, 676–682. doi: 10.2307/2419735
Crawford, D. J., Stuessy, T. F., and Silva, M. O. (1987). Allozyme divergence and the evolution of Dendroseris (Compositae: Lactuceae) on the Juan Fernandez Islands. Syst. Bot. 12, 435–443. doi: 10.2307/2419268
Daniell, H., Lin, C. S., Yu, M., and Chang, W. J. (2016). Chloroplast genomes: diversity, evolution, and applications in genetic engineering. Genome Biol. 17:134.
Doyle, J. J., and Doyle, J. L. (1987). A rapid DNA isolation procedure for small quantities of fresh leaf tissue. Phytochem. Bull. 19, 11–15.
Esselman, E. J., Crawford, D. J., Brauner, S., Stuessy, T. F., Anderson, G. J., and Silva, M. O. (2000). RAPD marker diversity within and divergence among species of Dendroseris (Asteraceae: Lactuceae). Am. J. Bot. 87, 591–596. doi: 10.2307/2656603
Frazer, K. A., Pachter, L., Poliakov, A., Rubin, E. M., and Dubchak, I. (2004). VISTA: computational tools for comparative genomics. Nucleic Acids Res. 32, W273–W279.
Jackson, R. C. (1982). Polyploidy and diploidy: new perspectives on chromosome pairing and its evolutionary implications. Am. J. Bot. 69, 1512–1523. doi: 10.1002/j.1537-2197.1982.tb13400.x
Kalyaanamoorthy, S., Minh, B. Q., Wong, T. K., von Haeseler, A., and Jermiin, L. S. (2017). ModelFinder: fast model selection for accurate phylogenetic estimates. Nat. Methods 14, 587–589. doi: 10.1038/nmeth.4285
Katoh, K., and Standley, D. M. (2013). MAFFT multiple sequence alignment software version 7: improvements in performance and usability. Mol. Biol. Evol. 30, 772–780. doi: 10.1093/molbev/mst010
Kilian, N., Gemeinholzer, B., and Lack, W. L. (2009). “Cichorieae,” in Systematics, Evolution and Biogeography of Compositae, eds V. A. Funk, A. Susanna, T. F. Stuessy, and R. J. Bayer (Vienna: International Association for Plant Taxonomy), 343–383.
Kim, S.-C., Crawford, D. J., Francisco-Ortega, J., and Santos-Guerra, A. (1996a). A common origin for woody Sonchus and five related genera in the Macaronesian islands: molecular evidence for extensive radiation. Proc. Natl. Acad. Sci. U.S.A. 93, 7743–7748. doi: 10.1073/pnas.93.15.7743
Kim, S.-C., Crawford, D. J., and Jansen, R. K. (1996b). Phylogenetic relationships among the genera of the subtribe Sonchinae (Asteraceae): evidence from ITS sequences. Syst. Bot. 21, 417–432. doi: 10.2307/2419668
Kim, S.-C., Crawford, D. J., Jansen, R. K., and Santos-Guerra, A. (1999). The use of a non-coding region of chloroplast DNA in phylogenetic studies of the subtribe Sonchinae (Asteraceae: Lactuceae). Plant Syst. Evol. 215, 85–99.
Kim, S.-C., Lee, C., and Mejías, J. A. (2007). Phylogenetic analysis of chloroplast DNA matK gene and ITS of nrDNA sequences reveals polyphyly of the genus Sonchus and new relationships among the subtribe Sonchinae (Asteraceae: Cichorieae). Mol. Phylogenet. Evol. 44, 578–597. doi: 10.1016/j.ympev.2007.03.014
Kumar, S., Stecher, G., and Tamura, K. (2016). MEGA7: molecular evolutionary genetics analysis version 7.0 for bigger datasets. Mol. Biol. Evol. 33, 1870–1874. doi: 10.1093/molbev/msw054
Kurtz, S., Choudhuri, J. V., Ohlebusch, E., Schleiermacher, C., Stoye, J., and Giegerich, R. (2001). REPuter: the manifold applications of repeat analysis on a genomic scale. Nucleic Acids Res. 29, 4633–4642. doi: 10.1093/nar/29.22.4633
Lack, H. W. (2007). “Cichorieae,” in The Families and Genera of Vascular Plants, vol. 8, Flowering Plants, Eudicots, Asterales, eds J. W. Kadereit and C. Jeffrey (Berlin: Springer), 180–199.
Lagesen, K., Hallin, P., Rødland, E. A., Stærfeldt, H. H., Rognes, T., and Ussery, D. W. (2007). RNammer: consistent annotation of rRNA genes in genomic sequences. Nucleic Acids Res. 35, 3100–3108. doi: 10.1093/nar/gkm160
Laslett, D., and Canback, B. (2004). ARAGORN, a program to detect tRNA genes and tmRNA genes in nucleotide sequences. Nucleic Acids Res. 32, 11–16. doi: 10.1093/nar/gkh152
Lee, C., Kim, S.-C., Lundy, K., and Santos−Guerra, A. (2005). Chloroplast DNA phylogeny of the woody Sonchus alliance (Asteraceae: Sonchinae) in the Macaronesian Islands. Am. J. Bot. 92, 2072–2085. doi: 10.3732/ajb.92.12.2072
Librado, P., and Rozas, J. (2009). DnaSP v5: a software for comprehensive analysis of DNA polymorphism data. Bioinformatics 25, 1451–1452. doi: 10.1093/bioinformatics/btp187
Lohse, M., Drechsel, O., Kahlau, S., and Bock, R. (2013). OrganellarGenomeDRAW—A suite of tools for generating physical maps of plastid and mitochondrial genomes and visualizing expression data sets. Nucleic Acids Res. 41, W575–W581.
Mejías, J. A., and Kim, S.-C. (2012). Taxonomic treatment of Cichorieae (Asteraceae) endemic to the Juan Fernández and Desventuradas Islands (SE Pacific). Ann. Bot. Fenn. 49, 171–178. doi: 10.5735/085.049.0303
Menezes, A. P. A., Resende-Moreira, L. C., Buzatti, R. S. O., Nazareno, A. G., Carlsen, M., Lobo, F. P., et al. (2018). Chloroplast genomes of Byrsonima species (Malpighiaceae): comparative analysis and screening of high divergence sequences. Sci. Rep. 8, 1–12.
Mower, J. P. (2009). The PREP suite: predictive RNA editors for plant mitochondrial genes, chloroplast genes and user-defined alignments. Nucleic Acids Res. 37, W253–W259. doi: 10.1016/0168-9452(90)90180-v
Nguyen, L. T., Schmidt, H. A., von Haeseler, A., and Minh, B. Q. (2014). IQ-TREE: a fast and effective stochastic algorithm for estimating maximum-likelihood phylogenies. Mol. Biol. Evol. 32, 268–274. doi: 10.1093/molbev/msu300
Orešiè, M., and Shalloway, D. (1998). Specific correlations between relative synonymous codon usage and protein secondary structure. J. Mol. Biol. 281, 31–48. doi: 10.1006/jmbi.1998.1921
Pacheco, P., Crawford, D. J., Stuessy, T. F., and Silva, M. O. (1991). Flavonoid evolution in Dendroseris (Compositae, Lactuceae) from the Juan Fernandez Islands, Chile. Am. J. Bot. 78, 534–543. doi: 10.1002/j.1537-2197.1991.tb15220.x
Palmer, J. D. (1983). Chloroplast DNA exists in two orientations. Nature 301, 92–93. doi: 10.1038/301092a0
Parks, M., Cronn, R., and Liston, A. (2009). Increasing phylogenetic resolution at low taxonomic levels using massively parallel sequencing of chloroplast genomes. BMC Biol. 7:84. doi: 10.1186/1741-7007-7-84
Ramsey, J., and Schemske, D. W. (2002). Neopolyploidy in flowering plants. Annu. Rev. Ecol. Evol. Syst. 33, 589–639. doi: 10.1146/annurev.ecolsys.33.010802.150437
Sablok, G., Amiryousefi, A., He, X., Hyvönen, J., and Poczai, P. (2019). Sequencing the plastid genome of giant ragweed (Ambrosia trifida, Asteraceae) from a herbarium specimen. Front. Plant Sci. 10:218. doi: 10.3389/fpls.2019.00218
Sanders, R. W., Stuessy, T. F., Marticorena, C., and Silva, M. O. (1987). Phytogeography and evolution of Dendroseris and Robinsonia, tree-Compositae of the Juan Fernandez Islands. Opera Bot. 92, 195–215.
Sanders, R. W., Stuessy, T. F., and Rodriguez, R. (1983). Chromosome numbers from the flora of the Juan Fernandez Islands. Am. J. Bot. 70, 799–810. doi: 10.1002/j.1537-2197.1983.tb06415.x
Sang, T., Crawford, D. J., Kim, S.-C., and Stuessy, T. F. (1994). Radiation of the endemic genus Dendroseris (Asteraceae) on the Juan Fernandez Islands: evidence from sequences of the ITS regions of nuclear ribosomal DNA. Am. J. Bot. 81, 1494–1501. doi: 10.1002/j.1537-2197.1994.tb15635.x
Shaw, J., Shafer, H. L., Leonard, O. R., Kovach, M. J., Schorr, M., and Morris, A. B. (2014). Chloroplast DNA sequence utility for the lowest phylogenetic and phylogeographic inferences in angiosperms: the tortoise and the hare IV. Am. J. Bot. 101, 1987–2004. doi: 10.3732/ajb.1400398
Skottsberg, C. (1953). “The vegetation of the Juan Fernandez Islands,” in The Natural History of Juan Fernandez and Easter Island V.2, ed. C. Skottsberg (Uppsala: Almqvist and Wiksells boktryckeri), 793–960.
Skottsberg, C. (1956). “Derivation of the Flora and Fauna of Juan Fernandez and Easter Island,” in The Natural History of Juan Fernandez and Easter Island V.1, ed. C. Skottsberg (Uppsala: Almqvist and Wiksells boktryckeri), 193–427.
Spooner, D. M., Stuessy, T. F., Crawford, D. J., and Silva, M. O. (1987). Chromosome numbers from the flora of the Juan Fernandez Islands. II. Rhodora 89, 351–356.
Stebbins, G. L. (1953). A new classification of the tribe Cichorieae, family Compositae. Madroño 12, 65–81.
Stuessy, T. F., Sanders, R. W., and Silva, M. O. (1984). “Phytogeography and evolution of the Juan Fernandez Islands: a progress report,” in Pacific basin biogeography, eds F. J. Radovsky, P. H. Raven, and S. H. Sohmer (Lawrence, KS: B. P. Bishop Museum), 55–69.
Stuessy, T. F., Swenson, U., Crawford, D. J., and Anderson, G. (1998). Plant conservation in the Juan Fernandez archipelago, Chile. Aliso 16, 89–101. doi: 10.5642/aliso.19971602.04
Walker, J. F., Zanis, M. J., and Emery, N. C. (2014). Comparative analysis of complete chloroplast genome sequence and inversion variation in Lasthenia burkei (Madieae, Asteraceae). Am. J. Bot. 101, 722–729. doi: 10.3732/ajb.1400049
Walker, J. F., Zanis, M. J., and Emery, N. C. (2015). Correction to “Comparative analysis of complete chloroplast genome sequence and inversion variation in Lasthenia burkei (Madieae, Asteraceae)”. Am. J. Bot. 102, 1008–1008. doi: 10.3732/ajb.1500990
Walter, K. S., and Gillett, H. J. (1998). 1997 IUCN Red List of Threatened Plants. Gland: IUCN-The World Conservation Union.
Wodehouse, R. P. (1935). Pollen Grains, Their Structure, Identification and Significance in Science and Medicine. London: McGraw-Hill Book Company, Inc.
Wyman, S. K., Jansen, R. K., and Boore, J. L. (2004). Automatic annotation of organellar genomes with DOGMA. Bioinformatics 20, 3252–3255. doi: 10.1093/bioinformatics/bth352
Keywords: Asteraceae, adaptive radiation, plastome evolution, insular woodiness, Juan Fernández Islands, critically endangered
Citation: Cho M-S, Kim S-H, Yang JY, Crawford DJ, Stuessy TF, López-Sepúlveda P and Kim S-C (2020) Plastid Phylogenomics of Dendroseris (Cichorieae; Asteraceae): Insights Into Structural Organization and Molecular Evolution of an Endemic Lineage From the Juan Fernández Islands. Front. Plant Sci. 11:594272. doi: 10.3389/fpls.2020.594272
Received: 12 August 2020; Accepted: 14 October 2020;
Published: 05 November 2020.
Edited by:
Stefan Wanke, Technische Universität Dresden, GermanyReviewed by:
Sònia Garcia, Botanical Institute of Barcelona, SpainCopyright © 2020 Cho, Kim, Yang, Crawford, Stuessy, López-Sepúlveda and Kim. This is an open-access article distributed under the terms of the Creative Commons Attribution License (CC BY). The use, distribution or reproduction in other forums is permitted, provided the original author(s) and the copyright owner(s) are credited and that the original publication in this journal is cited, in accordance with accepted academic practice. No use, distribution or reproduction is permitted which does not comply with these terms.
*Correspondence: Seung-Chul Kim, c29uY2h1czk2QHNra3UuZWR1; c29uY2h1czIwMDlAZ21haWwuY29t
Disclaimer: All claims expressed in this article are solely those of the authors and do not necessarily represent those of their affiliated organizations, or those of the publisher, the editors and the reviewers. Any product that may be evaluated in this article or claim that may be made by its manufacturer is not guaranteed or endorsed by the publisher.
Research integrity at Frontiers
Learn more about the work of our research integrity team to safeguard the quality of each article we publish.