- 1College of Agronomy, Northwest A&F University/State Key Laboratory of Crop Stress Biology for Arid Areas, Yangling, China
- 2Institute of Crop Science, Chinese Academy of Agricultural Sciences (CAAS)/National Key Facility for Crop Gene Resources and Genetic Improvement, Key Laboratory of Biology and Genetic Improvement of Triticeae Crops, Ministry of Agriculture, Beijing, China
- 3College of Agriculture, Yangtze University, Jingzhou, China
Ankyrin repeat (ANK) proteins are essential in cell growth, development, and response to hormones and environmental stresses. In the present study, 226 ANK genes were identified and classified into nine subfamilies according to conserved domains in the soybean genome (Glycine max L.). Among them, the GmANK114 was highly induced by drought, salt, and abscisic acid. The GmANK114 encodes a protein that belongs to the ANK-RF subfamily containing a RING finger (RF) domain in addition to the ankyrin repeats. Heterologous overexpression of GmANK114 in transgenic Arabidopsis improved the germination rate under drought and salt treatments compared to wild-type. Homologous overexpression of GmANK114 improved the survival rate under drought and salt stresses in transgenic soybean hairy roots. In response to drought or salt stress, GmANK114 overexpression in soybean hairy root showed higher proline and lower malondialdehyde contents, and lower H2O2 and O2– contents compared control plants. Besides, GmANK114 activated transcription of several abiotic stress-related genes, including WRKY13, NAC11, DREB2, MYB84, and bZIP44 under drought and salt stresses in soybean. These results provide new insights for functional analysis of soybean ANK proteins and will be helpful for further understanding how ANK proteins in plants adapt to abiotic stress.
Introduction
In recent years, various abiotic stresses, especially drought and salt, became more and more frequent with global climate change. Abiotic stresses change the physiological traits and metabolism of plants, affect the growth and development processes, and eventually lead to decreased yield and quality of crops (Veatch-Blohm, 2007; Hasanuzzaman et al., 2012). To adapt to the changing environments, plants induce the expression of stress-related genes by receiving external signals and undergoing a series of complex signal transduction pathways and diverse response mechanisms, thereby reducing the damage of abiotic stresses on their growth and development (Chen and Murata, 2011; Wang et al., 2015; Zhu, 2016; Qi et al., 2018; Yang and Guo, 2018).
The ankyrin repeat (ANK) proteins, with at least four consecutive ANK motifs (Michaely and Bennett, 1992; Mosavi et al., 2004), are distributed in diverse organisms ranging from viruses to plants (Sedgwick and Smerdon, 1999), and are very crucial in different functions, including cell cycle regulation, transcriptional regulation, cytoskeleton interaction, and signal transduction. In humans and animals, ANK proteins are directly involved in the development of cancer. The human VBARP is thought to be involved with cellular apoptosis (anti-apoptotic) and cell survival pathways (Miles et al., 2005).
Similarly, plant ANK proteins were involved in various important biological processes. AKR, the first reported ANK protein with five ankyrin repeats in Arabidopsis, played a regulatory role in cell differentiation and development (Zhang et al., 1992). EMB506, similar to AKR with five ankyrin repeats, is an essential chloroplast protein for normal development of Arabidopsis embryos (Albert et al., 1999). Subsequently, the EMB506 was proved interact with the AKR protein and caused to lose its function, thereby resulted in an embryo-defective phenotype. It was demonstrated that EMB506 and AKR are involved in crucially and tightly controlled events in plastid differentiation linked to cell differentiation, morphogenesis, and organogenesis during the plant life cycle (Garcion et al., 2006). AtACD6, a regulator and an effector of salicylic acid (SA) signaling, is a dose-dependent activator of the defense responses against virulent bacteria and can activate SA-dependent cell death (Lu et al., 2003, 2005). The interaction of the tobacco ANK protein NEIP2 with ethylene receptor NTHK1 improved plant growth and performance under salt and oxidative stresses (Cao et al., 2015).
In previous studies, ANK proteins were divided into multiple subfamilies according to the conserved domains (Becerra et al., 2004). The ANK-RF subgroup proteins contain the RING finger (RF) domain, which was first identified in the Really Interesting New Gene (Freemont et al., 1991). The RF domain, belonging to the zinc finger domain protein family, could bind to RNA, protein or lipid substrates (Elenbaas et al., 1996). A large number of RF-containing proteins have an E3 ligase role in ubiquitination reactions (Stone et al., 2005; Berrocal-Lobo et al., 2010) and the C3HC4 motif of RF domain is essential for conferring E3 ligase activity (Lorick et al., 1999). Rice XA21-binding protein 3 (XB3), an ANK-RF E3 ubiquitin ligase, is a substrate for the XA21 kinase and interacts with the XA21 kinase domain for full accumulation of the XA21 protein and for XA21-mediated immunity. Therefore, XB3 contributes to the stability of XA21 and is required for full accumulation of the XA21 protein in XA21-mediated rice immunity (Wang et al., 2006).
ANK-RF proteins functions in various biological processes in plant growth and development. The Arabidopsis ANK-RF protein AtXBAT32, an ubiquitin ligase, positively affected ethylene biosynthesis by degradation of the ethylene biosynthetic enzyme1-aminocyclopropane-1-carboxylate synthase 7 (Lyzenga et al., 2012), resulting in regulation of lateral root initiation (Nodzon et al., 2004; Prasad et al., 2010). Another ANK-RF protein AtXBAT35 participated in ubiquitin-mediated protein degradation, and played an important role in negatively regulating ethylene-mediated apical hook curvature in Arabidopsis (Carvalho et al., 2012). The overexpression of LIANK, a lily ANK-RF protein, resulted in pollen tube growing abnormally and its silencing impaired pollen germination and tube growth (Huang et al., 2006).
Recently, ANK-RF subfamily genes were proven to play diverse roles in the stress responses. A RING zinc finger ankyrin protein gene AdZFP1 was isolated from drought-tolerant Artemisia desertorum and the transcript level of AdZFP1 was strongly induced by drought, salt, cold, heat, and exogenous abscisic acid (ABA) treatments. Besides, overexpression of the AdZFP1 gene enhanced drought tolerance in transgenic tobacco (Yang et al., 2008). The pepper ANK-RF gene CaKR1 participates in various biotic and abiotic stresses, such as NaCl, cold, SA, ethylene, and pathogens infection. Overexpression of CaKR1 enhances resistance to salt and oxidative stresses in tomato (Seong et al., 2007a). The potato ANK-RF gene Star, a putative E3 ubiquitin ligase gene, is involved in late blight resistance and organ development (Wu et al., 2009). The rice ANK-RF protein OsXB3 plays a role in resistance against Xanthomonas oryzae pv. Oryzae (Wang et al., 2006).
Soybean (Glycine max L.), an important crop providing plant protein and oil, suffers from drought and salt damage with global climate change, especially in China. Previous studies characterized the ANK gene families in various plants (Becerra et al., 2004; Huang et al., 2009; Jiang et al., 2013; Yuan et al., 2013; Lopez-Ortiz et al., 2020). In this study, we identified 226 non-redundant soybean ANK genes, which were classified into nine subfamilies. An ANK-RF subfamily gene GmANK114 was significantly induced by drought, salt, and exogenous ABA. We further investigated stress tolerance conferred by GmANK114 in both Arabidopsis and soybean. The functional identification of GmANK114 will be very helpful for further understanding how ANK proteins in soybean adapt to abiotic stresses.
Materials and Methods
Identification of Soybean ANK Genes
We obtained the Hidden Markov Model (HMM) profile of the ANK domain (PF00023) from Pfam v29.0.1 BLAST was used to identify putative GmANK with the ANK domain (PF00023) as a query against the Phytozome database (v12.1)2 of soybean. All hits with expected values less than 1.0 were retrieved and redundant sequences were removed using BLASTclust.3 Then, all candidate sequences that met the standards were analyzed manually in the Pfam database once more and were checked using the SMART program (Letunic et al., 2009) for the purposes of eliminating any sequences not containing the ANK domain (Liu et al., 2016a). The ExPASy website was used to predict physio-chemical parameters of ANK proteins such as molecular weights (Mw) and theoretical isoelectric points (pI) (Artimo et al., 2012).
Phylogenetic Relationships and Classification of GmANKs
The phylogenetic relationships among ANKs were inferred using Clustal X with a gap opening penalty of 10 and a gap extension penalty of 0.1 (Thompson et al., 1997). A phylogenetic tree was constructed using the neighbor-joining method in MEGA 7 and bootstrap analysis was conducted using 1,000 replications (Tamura et al., 2011). Database tools in SMART were used to analyze their typical functional structure domains. The sample protein structures of each subfamily were drawn manually.
Gene Structure Prediction, Motif Analysis and Promoter Analysis
An exon-intron substructure map was produced by tools online GSDS 2.0 (Gene Structure Display Sever)4 (Guo et al., 2007). The conserved domain motifs analyze of soybean ANK proteins were conducted by TBtools (Chen et al., 2020), according to the analyzed result from MEME Suite 5.1.1.5 The motifs number were set for 10. The 2,000 bp region upstream of the initiation codon as the promoter region of the GmANK-RFs were selected to identify the cis-acting elements by submitting the promoter sequence to the PLACE6 and PlantCARE7 databases (Li et al., 2019).
Physical Mapping and Gene Duplication
All non-redundant ANKs were mapped on the 20 chromosomes on the basis of information in the soybean database using the MG2C website (MapGene2Chrom web v2)8 (Liu et al., 2016b). Segmental and tandem duplication events were determined as previously described (Wang et al., 2016; Fan et al., 2019). Gene segmental duplications events were analyzed and visualized by Multiple Collinearity Scan toolkit (MCScanx)9 and Circos-0.67 program10 respectively, with a E-value for 10–10. The tandem duplications were characterized as adjacent genes with a distance of less than 200 kb within neighboring intergenic region (Holub, 2001). Tandem duplications were manually marked on the physical map.
Gene Ontology Annotation
The functional annotation for the GmANK genes were conducted by Blast2GO11 software according to the previous description, including the subsequent analysis of annotation results (Conesa and Gotz, 2008).
Expression Analysis of Soybean ANK-RF Genes
Transcriptome data was obtained from the Phytozome database to investigate the expression profile of 17 ANK-RF genes in different soybean tissues, including root, root hairs, stem, leaves, nodules, flower, and seed of soybean. These transcriptome data were obtained under normal growing conditions and were not subjected to any stress. RNA-seq data of various abiotic stresses were extracted from our previous research to study the expression of ANK-RF genes under drought, NaCl and ABA treatments (Shi et al., 2018). The RNA-seq data were obtained from three biological replicates. Tophat and cufflinks were used to analyze the RNA-seq expression, and the gene expressions were uniformed in fragments per kilobase million (FPKM). The expression of ANK-RF genes was extracted from the total expression data. The FPKM values of soybean ANK-RF genes in different tissues and under abiotic stress treatments (drought, NaCl and ABA) were shown in Supplementary Tables 2, 3. TBtools software was used to generate the heatmap (Chen et al., 2020).
Plant Materials and Stresses Treatment
Seeds of soybean variety Williams 82 were grown in a greenhouse at 28/20°C day/night temperatures, with a photoperiod of 14 h light/10 h dark and 60% relative humidity. For drought treatment, 16-day-old seedlings were placed on filter paper for the induction of rapid drought for 0, 1, 2, 4, 8, 12, and 24 h. For NaCl treatment, 16-day-old seedlings in soil watered with 200 mM NaCl solution. For ABA treatments, the seedlings were exposed to 100 μM ABA. Unstressed plants were maintained as control. After stress treatments, the seedlings were carefully harvested and immediately frozen with liquid nitrogen, and stored at −80°C until RNA isolation.
Wild-type (WT) and T3 transgenic seeds were used to evaluate the drought and salt tolerance. For germination assay, seeds were cultured in the medium with 6 or 9% (w/v) PEG 6000 and 75, 100, and 125 mM NaCl. After 3 days of vernalization, the seeds were transferred to normal conditions for germination. Seeds were considered to be germinated when radicles emerged from the seed coats. The percentage of germinated seeds was calculated based on the number of seedlings at 1, 2, 3, 4, 5, and 6 day. All the experiments were repeated three times.
Agrobacterium rhizogenes-Mediated Transformation of Soybean Hairy Roots
The open reading frame (ORF) of GmANK114 was amplified and ligated into plant transformation vector pCAMBIA3301 driven by the CaMV35S promoter to generate the pCAMBIA3301-GmANK114 overexpression vector. The recombinant vectors were introduced into Agrobacterium rhizogenes strain K599, and that was used to infect soybean cultivar Williams 82 cotyledonary node by injection as described previously (Kereszt et al., 2007). All experiments have three biological repetitions at least.
Measurements of Proline, MDA, H2O2, and O2– Contents
Before measurements, the transgenic soybean hairy roots were subjected to drought or 200 mM NaCl stress for 5 days, after which the proline (Pro), malondialdehyde (MDA), H2O2, and O2– contents of leaves were measured using the corresponding assay kit (Solarbio, Beijing, China) in accordance with the manufacturer’s protocol. All measurements were repeated three times.
RNA Extraction and Quantitative Real-Time PCR
Total RNA was isolated using Trizol reagent (TaKaRa, Japan) and treated with RNase-free DNase I (TaKaRa, Japan) to remove genomic DNA contamination. The cDNA synthesis and reverse transcription-PCR were conducted using TransScript All-in One First-Strand cDNA Synthesis SuperMix for qPCR (TRANSGEN, China). Quantitative real-time PCR (qRT-PCR) was performed in three technical replicates using Super Real PreMix Plus (SYBR Green) (TIANGEN, China) with an Applied Biosystems® 7500 Real-Time PCR System. The soybean CYP2 (Glyma.12g024700) gene was used for normalization (Zhang et al., 2019). The primers used for qRT-PCR are listed in Supplementary Table 4.
DAB and NBT Staining
The detached leaves of transgenic soybean were stained separately after stress treatment. For 3,3-diaminobenzidine (DAB) and nitroblue tetrazolium (NBT) staining, the samples were immersed in DAB or NBT solution (Solarbio, China) for 12 h and then transferred to 75% ethanol for decoloring until the leaves became white (Du et al., 2018).
Statistical Analysis
All experiments above were replicated three times independently. The values are shown as mean ± standard deviation (SD). ANOVA test was used for statistical analyses, and the signifcance was labeled ∗P < 0.05; and ∗∗P < 0.01.
Results
Identification of Soybean ANKs
The ANK domain (PF00023) was used as a query and searched in several databases including Phytozome, NCBI, Pfam, and SMART, a total of 226 genes encoding ANK proteins were identified in soybean. Almost all the GmANKs contained introns. The number of introns in these genes ranged from 0 (GmANK9, GmANK12 and GmANK14) to 21 (GmANK53) (Supplementary Figure 1). These results suggested that members of the GmANK family might be active and constantly evolving.
The size and physicochemical properties of GmANKs varied substantially. The length of GmANK proteins ranged from 133 (GmANK7) to 1,637 (GmANK122) amino acids. The molecular weights of GmANK proteins changed from 14,747.23 Da (GmANK7) to 179,852.44 Da (GmANK122). The characteristic features of GmANK protein sequences are summarized in Supplementary Table 1.
Chromosomal Location and Duplication Events Analysis
To determine the genomic distribution of ANK genes, chromosomal localization maps were constructed in soybean. The precise position (in bp) of each GmANK gene on soybean chromosomes is detailed in Supplementary Table 1. In total, 223 of the 226 GmANKs on chromosomes revealed an uneven distribution in the genome. As shown in Figure 1, the number of genes per chromosome ranged from 6 to 18. Chromosomes 6 and 9 had the largest number of GmANK genes (18 members), followed by 16 GmANK genes on each of chromosomes 13 and 15, while low densities of GmANK genes were observed in chromosome 16 (six members).
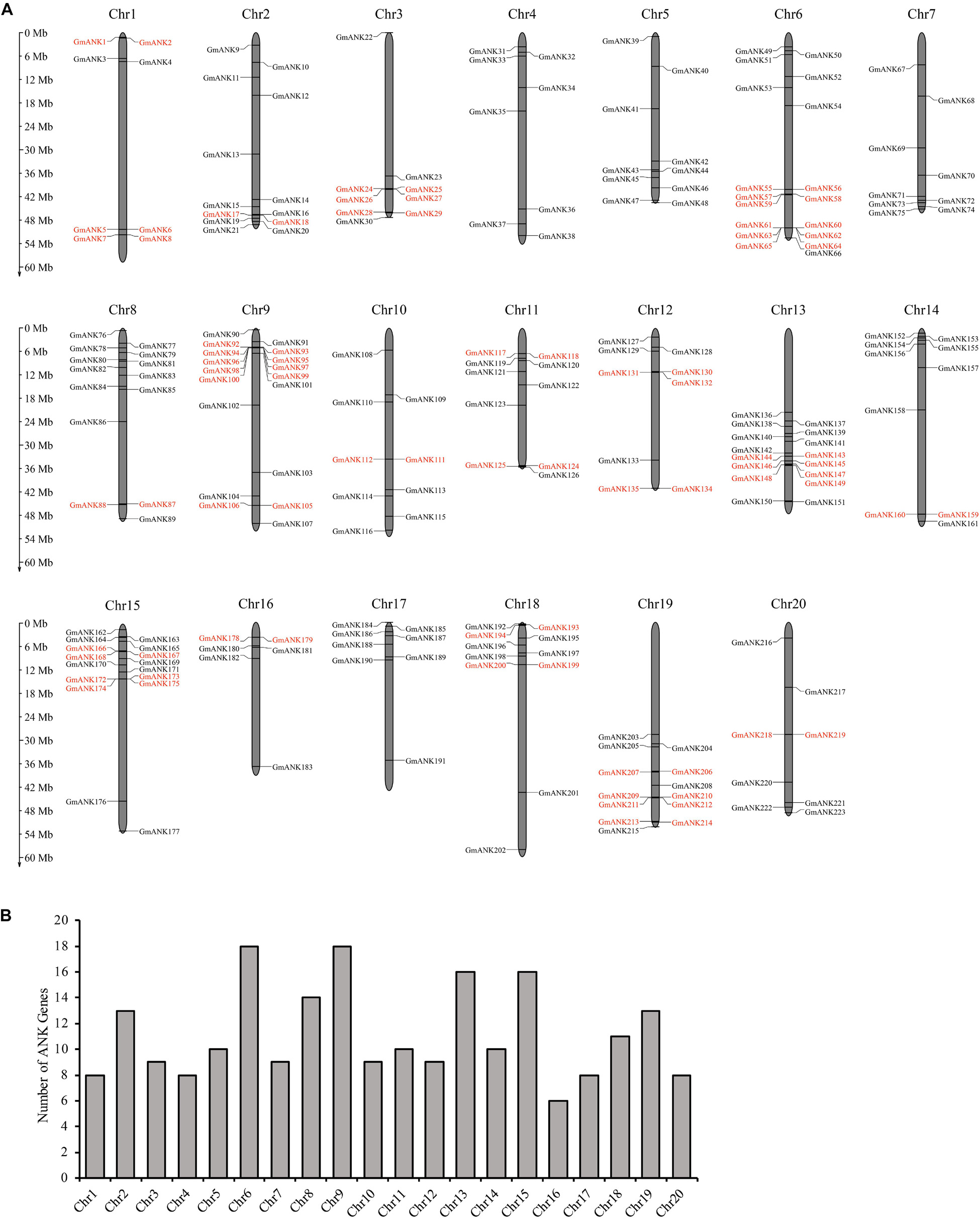
Figure 1. Distribution of 223 GmANK genes on the soybean chromosomes. (A) The physical location of each member in soybean. The scale on the left is in megabases (Mb). The chromosome number (Chr1–Chr20) is indicated at the top of each chromosome. The tandem duplicated gene clusters are marked by the red rectangle. (B) The numbers of ANK genes were distributed on 20 chromosomes.
To elucidate the conceivable mechanism of evolution of GmANKs, tandem and segmental duplication events were analyzed. A total of 81 GmANK genes were involved in tandem duplications consisting of 34 clusters (Figure 1). The distance between these genes ranged from 2.8 to 180.4 kb and five tandemly duplicated GmANK sets contained more than three genes. Among them, two tandemly duplicated GmANK sets contained three members (GmANK57/58/59, GmANK166/167/168), one tandemly duplicated GmANK set contained four members (GmANK172/173/174/175), one tandemly duplicated GmANK set contained six members (GmANK57/58/59/166/167/168), the maximum tandemly duplicated set contained nine members (GmANK92/93/94/95/96/97/98/99/100) and the other tandem duplications contained only two genes. Besides tandem duplication events, we further observed that up to 46% (104 out of 226) of GmANK genes were involved in segmental duplication and 36 genes were duplicated more than twice (Figure 2). In general, these results show that tandem and segmental duplication may be one of the main contributing factors for the large expansion of ANK genes in the soybean genome.
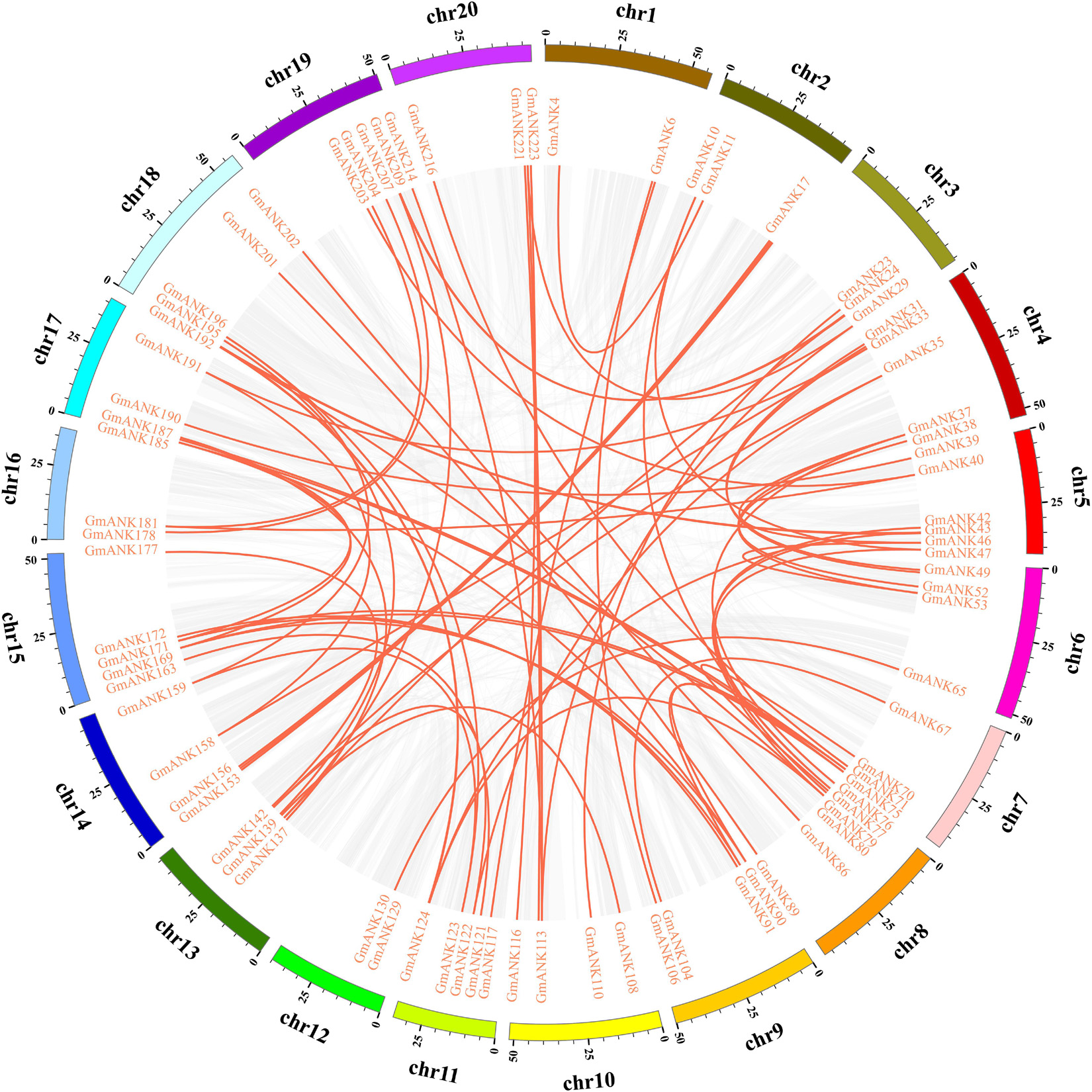
Figure 2. Distribution of segmentally duplication ANK genes on soybean chromosomes. Gray lines indicate all synteny blocks in whole soybean genome, and red lines indicate duplicated ANK gene pairs.
Classification of GmANKs
Based on the domain compositions (Huang et al., 2009), the 226 GmANK proteins were classified into nine subfamilies according to the results of SMART searches (Figure 3). In total, 78 members that contained only one ANK domain belonged to subfamily ANK-M, while the remaining 148 proteins contained additional typical domains. Transmembrane domains were found in 78 GmANKs, which were identified as the ANK-TM subfamily. Seventeen members were confirmed as subfamily ANK-RF (ring finger). ANK-BTB subfamily (four members) had broad-complex, tramtrack and bric a brac domains. The other subfamilies included seven members with BAR, PH and ArfGap domains (ANK-BPA subfamily), two with tetratricopeptide repeat domains (ANK-TPR subfamily), 13 with zinc-finger domain (ANK-ZnF), and 11 contained conserved CG-1 (Calmodulin-binding Transcription Activator) and IQ (Calmodulin-binding motif) domains (ANK-IQ subfamily). ANK-O subfamily (16 members) contained other domains including FYVE, AAA, cNMP and CHROMO (Table 1).
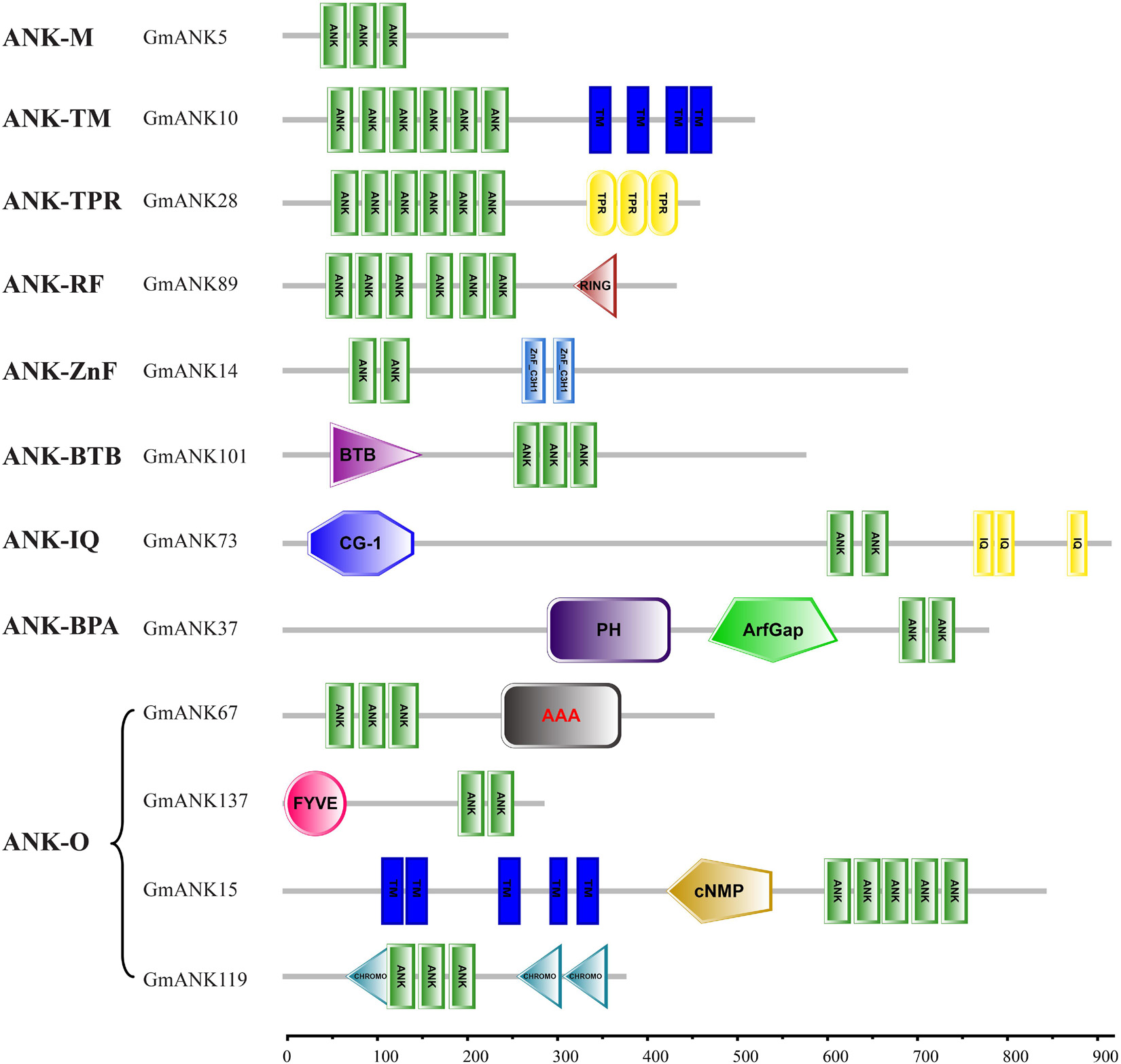
Figure 3. Domain compositions of representative GmANK proteins from each subfamily. The subfamily name of each corresponding protein and gene ID of a representative protein of the family are given on the left. Different domains are indicated with different colors and abbreviations. The length, order and number of domains represent the actual situation in each protein. Domain abbreviations are: ANK, ankyrin repeat domain; TM, transmembrane; TPR, tetratricopeptide repeat domain; RING, ring finger domain; ZnF-C3H1, zinc finger; BTB, BTB/POZ domain; CG-1, calmodulin-binding transcription activator; IQ, calmodulin-binding domain; PH, pleckstrin homology domain; ArfGap, putative GTP-ase activating proteins for the small GTPase; AAA, ATPase family associated with various cellular activities; FYVE, FYVE zinc finger; cNMP, cyclic nucleotide-binding domain; CHROMO, chromatin organization modifier domain.
Phylogenetic Analysis of GmANK Proteins and Conserved Motif Analysis
To understand the evolutionary relationships of GmANKs, an unrooted phylogenetic tree was constructed using the neighbor-joining method. The phylogenetic analysis categorized all GmANKs into six discrete groups (Group I–VI) comprising 41, 52, 50, 28, 16, and 39 proteins, respectively (Figure 4). Classification based on the phylogenetic tree and domain composition were consistent in general but not quite, such as for GmANK122 and GmANK142. Phylogenetic analysis reveals well-supported bootstrap values between GmANK122 and GmANK142 (Group III). However, GmANK122 has a RING domain (ANK-RF) but GmANK142 belongs to the ANK-M subfamily.
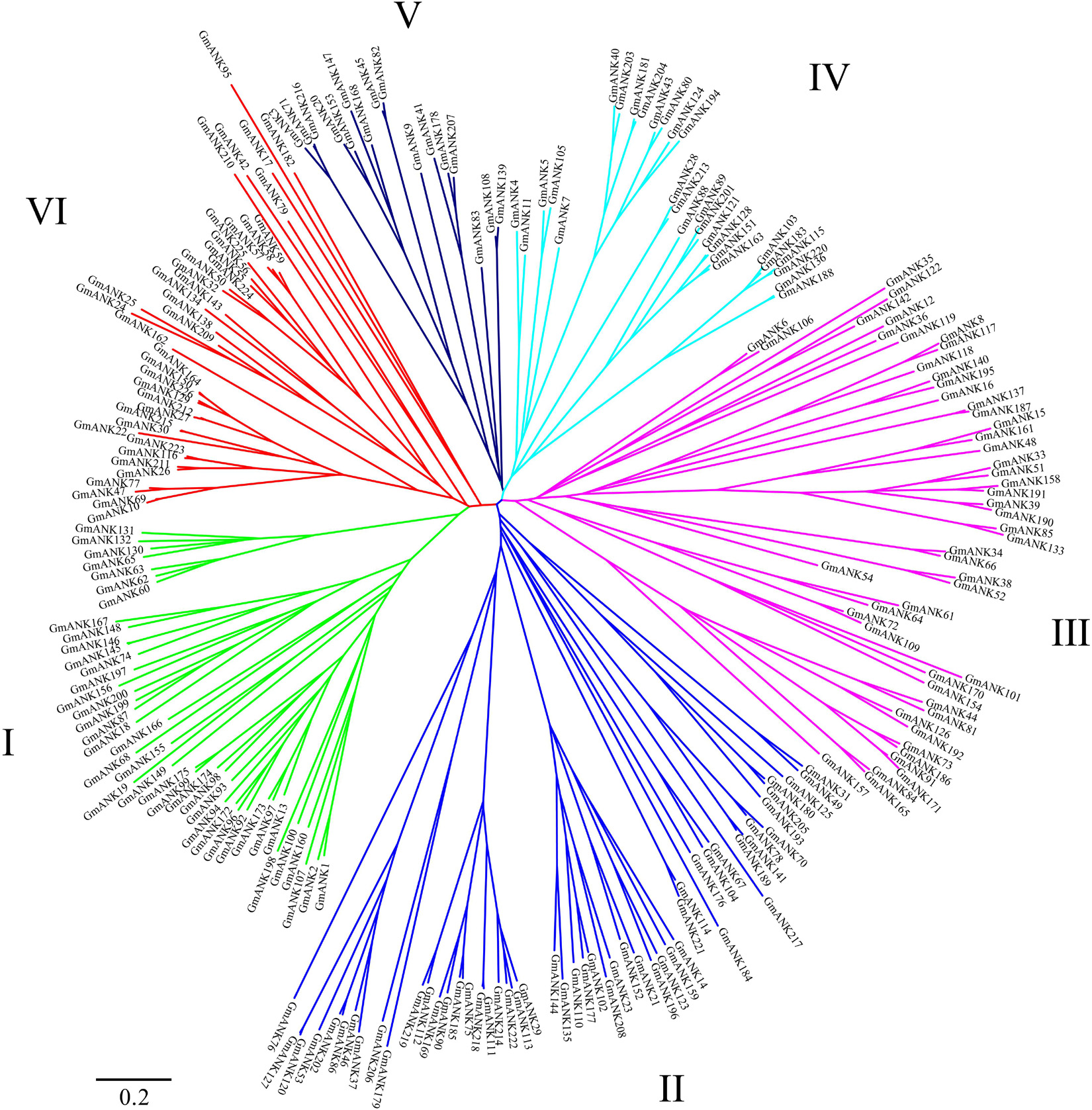
Figure 4. Phylogenetic analyses of ANK proteins in soybean. The complete amino acid sequences of the 226 GmANK proteins were aligned via Clustal X and were manually corrected. The phylogenetic tree was constructed with MEGA 7 in conjunction with the neighbor-joining method. This tree is drawn to scale with branch lengths in the same units as those of the evolutionary distances used to infer the phylogenetic tree. Six discrete groups (Cluster I–VI) were highlighted in different colors.
To better understand the function of each GmANKs subgroups, the motifs analysis of GmANKs family were conducted using MEME and TBtools software with default parameters (Supplementary Figure 2). The sizes of these conserved motifs ranged from 15 to 50 amino acids (Table 2). In general, the motifs structures in each subgroup of GmANK family were highly consistent and different combination of motifs represented different subgroups of GmANK family according to the analysis results of Supplementary Figure 2, which may account for the special biological functions of each groups and further confirmed their phylogenetic relationships.
Gene Ontology Annotation
To better understand the possible biological function of GmANK genes, Blast2GO software were used to performed the Gene Ontology (GO) annotation (Supplementary Figure 3). According to the GO annotation results, there were a total of 62 GmANK proteins were annotated in various biological processes, including signal transduction, regulation of membrane potential, positive regulation of transcription from RNA polymerase II promoter, regulation of proteolysis and S-adenosylmethionine metabolic process. There were 59 GmANK proteins were predicted to be related to molecular functions, such as transcriptional activator activity, RNA polymerase II core promoter proximal region sequence-specific binding, voltage-gated potassium channel activity, ubiquitin protein ligase binding, zinc ion binding and metal ion binding. Finally, 138 GmANK proteins were belonged to cellular components, which were located to membrane, integral component of membrane, cytoplasm, integral component of plasma membrane and ubiquitin ligase complex respectively. With the help of GO analysis results, we could primarily confirm the functional characteristics of GmANK proteins, like biological processes, molecular functions and cellular components, and will be very useful for its future functional researches in soybean.
GmANK-RFs Promoters Contain Various Stress-Responsive Elements
Promoter sequences were analyzed by PlantCARE and some cis-acting elements were revealed in all ANK-RFs promotor regions, such as ABRE (response to ABA), MYB (response to drought), MYB-like (response to drought), MBS (involvement in drought-inducibility), MYC (response to drought and salt) and LTR (response to low-temperature). In addition, an auxin-responsive element (TGA-element) and a MeJA-responsive element (CGTCA-motif and TGACG-motif) were also identified (Table 3). This result indicated that the GmANK-RFs genes may be involved in abiotic stresses, especially in drought.
Tissue-Specific Expression Patterns of GmANK-RF Genes
The expression abundance among different tissues and development stages may show diversity of different genes to adapt to various biological processes. To gain insights into the expression of ANK-RF genes in seven soybean tissues and organs, gene chip data were downloaded using publicly available RNA-seq data from the soybean genome database (Supplementary Table 2). The heatmap showed that 17 members of the ANK-RF subfamily were expressed in root, root hairs, stem, leaves, nodules, flowers and seeds (Figure 5). Among these ANK-RF genes, GmANK89 and GmANK201 had the highest expression in all tissues and organs, while GmANK88 was only weakly expressed in root and GmANK121 was slightly expressed in root hairs. Additionally, the expression patterns were different between ANK-RF genes in the same tissues. For example, the expression of GmANK151 and GmANK63 were at the highest level in stem, but GmANK220 was expressed most strongly in flowers; GmANK136 transcription had higher enrichment in root and GmANK163 had stronger accumulation in root hairs. These transcriptional patterns suggested that the expression of these genes might be governed by diverse and potentially tissue-dependent regulatory mechanisms. GmANK89 and GmANK201 had extremely high expression in all tissues indicating that they may play specific roles in growth and development of soybean.
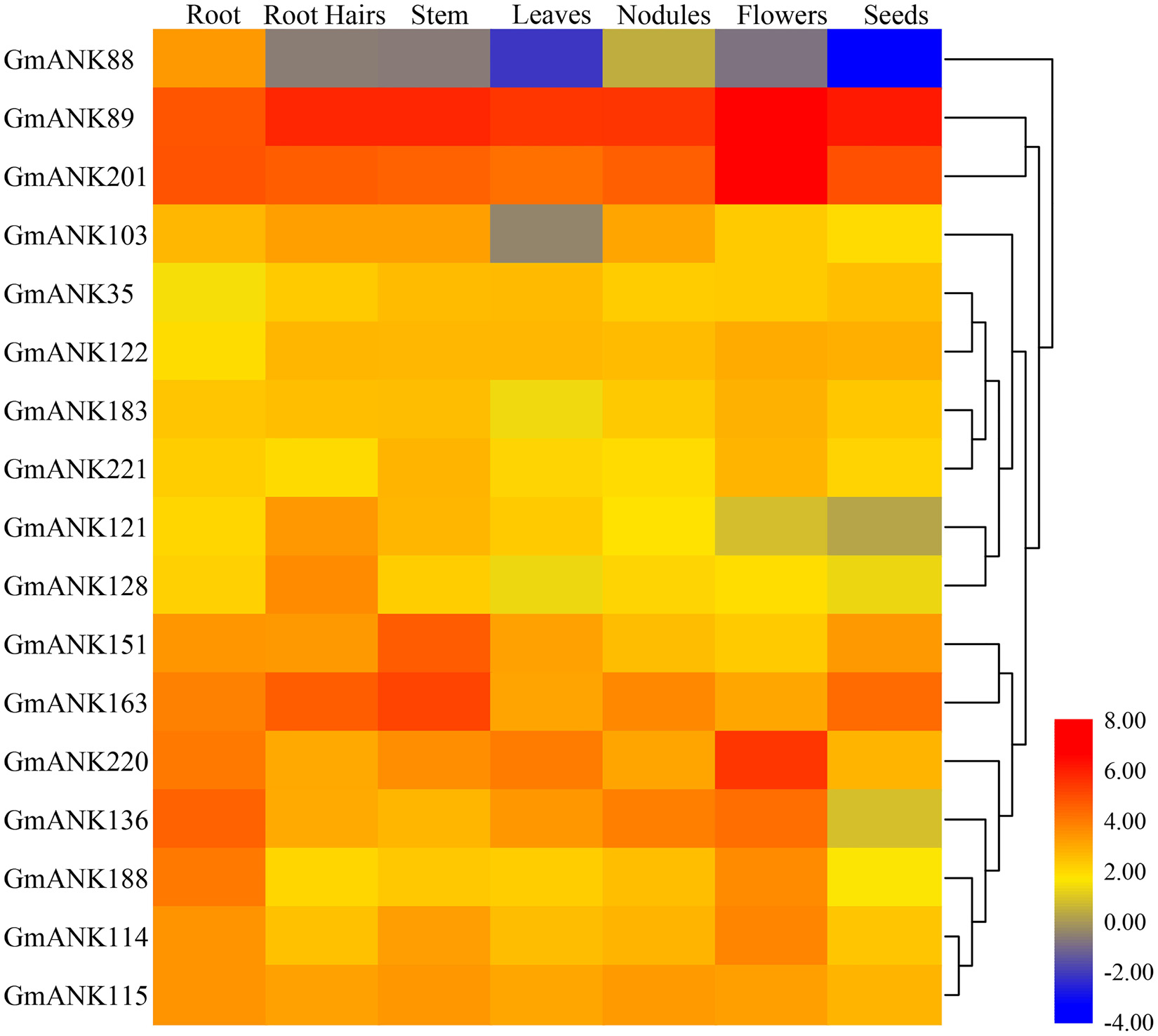
Figure 5. Heat map of expression profiles (in log2-based FPKM) of soybean ANK-RF subgroup genes in different tissues (root, root hairs, stem, leaf, nodules, flower and seed). The gene names are on the left and the tissue names are on the top of the figure, and the expression abundance of each transcript is represented by the color bar: Red, higher expression; blue, lower expression.
GmANK-RF Genes Were Involved in Various Abiotic Stresses
Extensive studies have shown that ANK-RF subfamily genes may be involved in responding to various abiotic stresses in some species (Nodzon et al., 2004; Seong et al., 2007a, b; Yang et al., 2008). According to our previous research, RNA-seq was performed using soybean seedlings under drought, NaCl and ABA treatments (Shi et al., 2018). RNA-seq data indicated that 17 GmANK-RFs responded differently to drought, NaCl, and ABA treatments (Figure 6). Among them, several genes induced expressed under drought stress, such as GmANK103, GmANK114, GmANK136, and GmANK220. GmANK114 were also specifically induced by NaCl. Under ABA stress, GmANK183 and GmANK188 showed down-regulated expression.
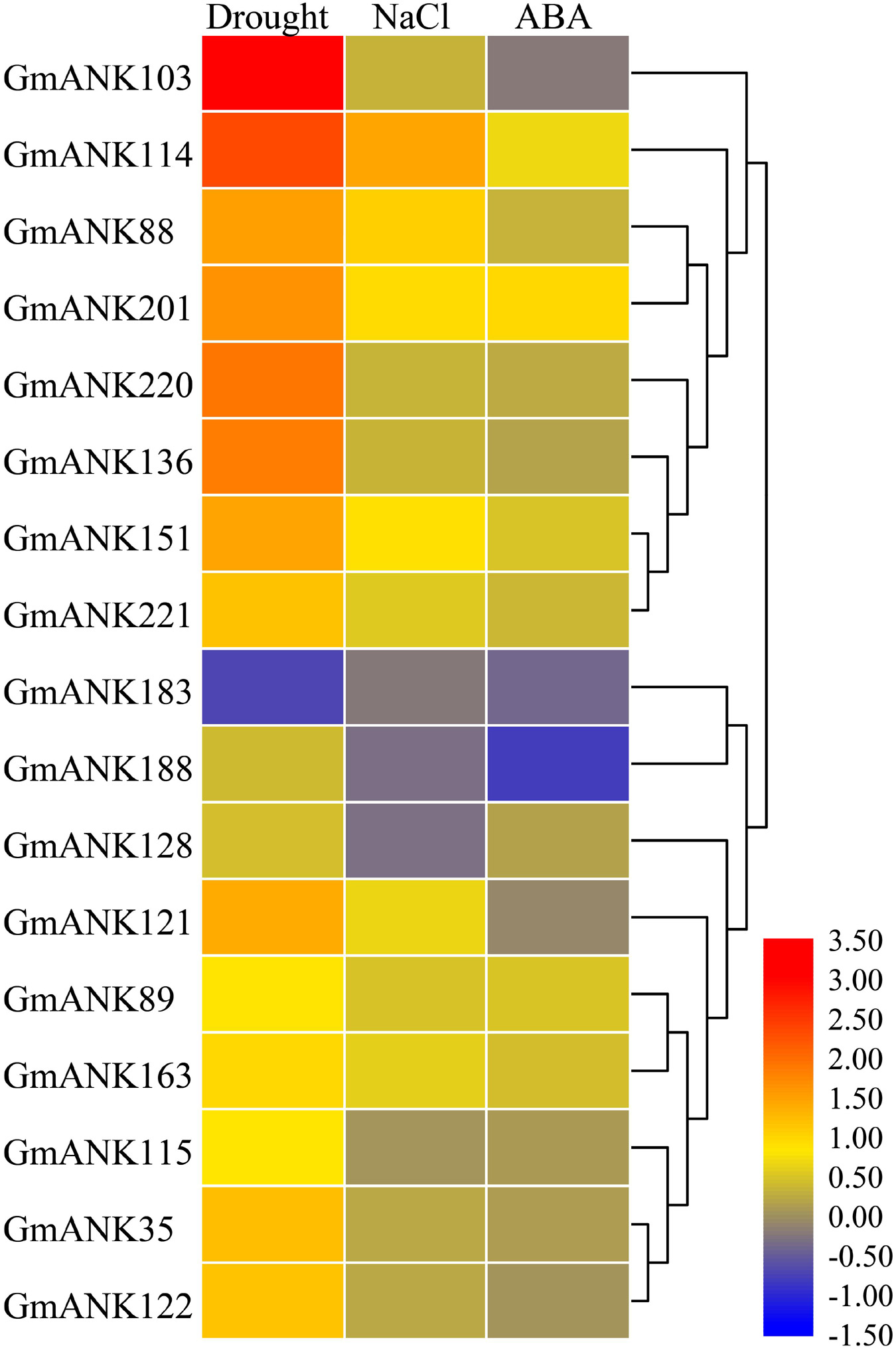
Figure 6. Transcriptome analysis-based soybean RNA sequencing data under drought, NaCl, and ABA. The expression abundance of each transcript is represented by the color bar: Red, higher expression; blue, lower expression.
Based on the results of RNA-seq, we examined the expression patterns of 17 GmANK-RF genes during various abiotic stresses and phytohormone treatments by qRT-PCR (Figure 7). Quantitative real-time PCR results were roughly consistent with RNA-seq data. The expression levels of candidate genes varied in response to drought, NaCl, and ABA for 1, 2, 4, 8, 12, 24 h compared to untreated control samples. Under drought treatment, the expression of all GmANK-RF genes was increased, especially GmANK103, GmANK114, GmANK201, and GmANK221, which were induced more than 30-fold. For salt treatment, the peaks of GmANK114, GmANK151, and GmANK163 transcription were increased to 15-fold. GmANK89, GmANK114, GmANK151, GmANK163, and GmANK221 were also induced by ABA, however, the level of GmANK115, GmANK121, GmANK128, and GmANK220 barely changed compared with 0 h. Through our transcriptional analysis of GmANK-RF genes, we found that the expression of GmANK114 was significantly up-regulated under drought, salt, and ABA stress conditions (Figure 7). Therefore, GmANK114 was selected for further verification.
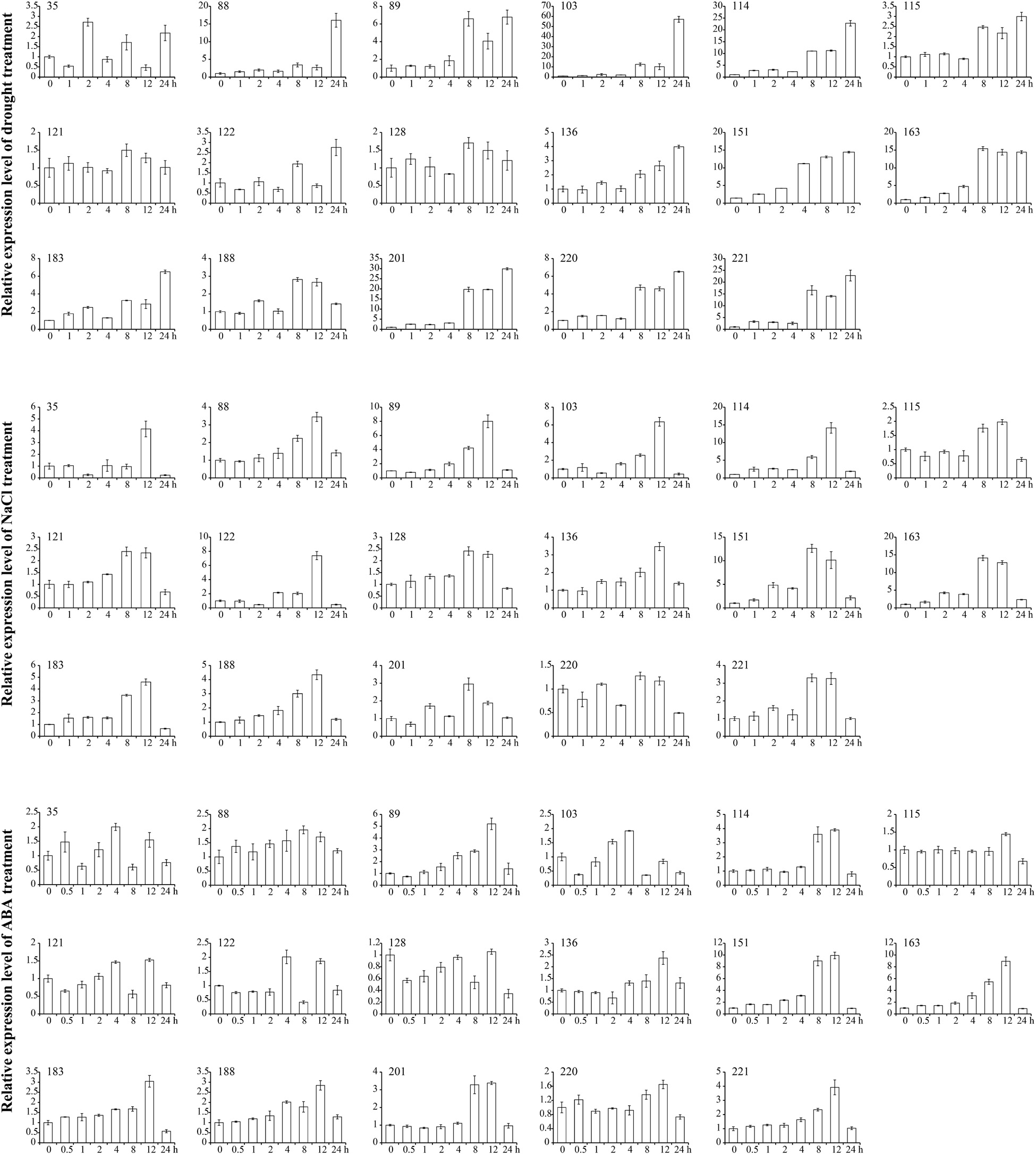
Figure 7. qRT-PCR analysis of 17 soybean ANK-RF genes under drought, NaCl and ABA treatments. The expression levels were normalized to that of CYP2. The mean and SD calculated from three biological replicates.
GmANK114 Conferred Drought and Salt Tolerance in Arabidopsis
GmANK114 under the control of CaMV35S were transformed into Arabidopsis plants and T3 transgenic seeds were selected for tolerance identification. For drought treatment, the transgenic and WT seeds were germinated on Murashige and Skoog (MS) medium containing various concentrations of PEG6000 (Figure 8A). The transgenic and WT plants had no significant differences under the standard conditions in growth and morphology (Figure 8B), suggesting that over-expression of GmANK114 genes might not affect plant growth and development under normal conditions. However, the germination percentage of transgenic plants was higher than the wild type in the presence of 6 and 9% PEG (Figures 8C,D). In growth medium with 9% PEG, 51.7, 48.9, and 46.2% of seeds germinated from three transgenic lines whereas only 40.1% of wild type germinated within 3 days (Figure 8D).
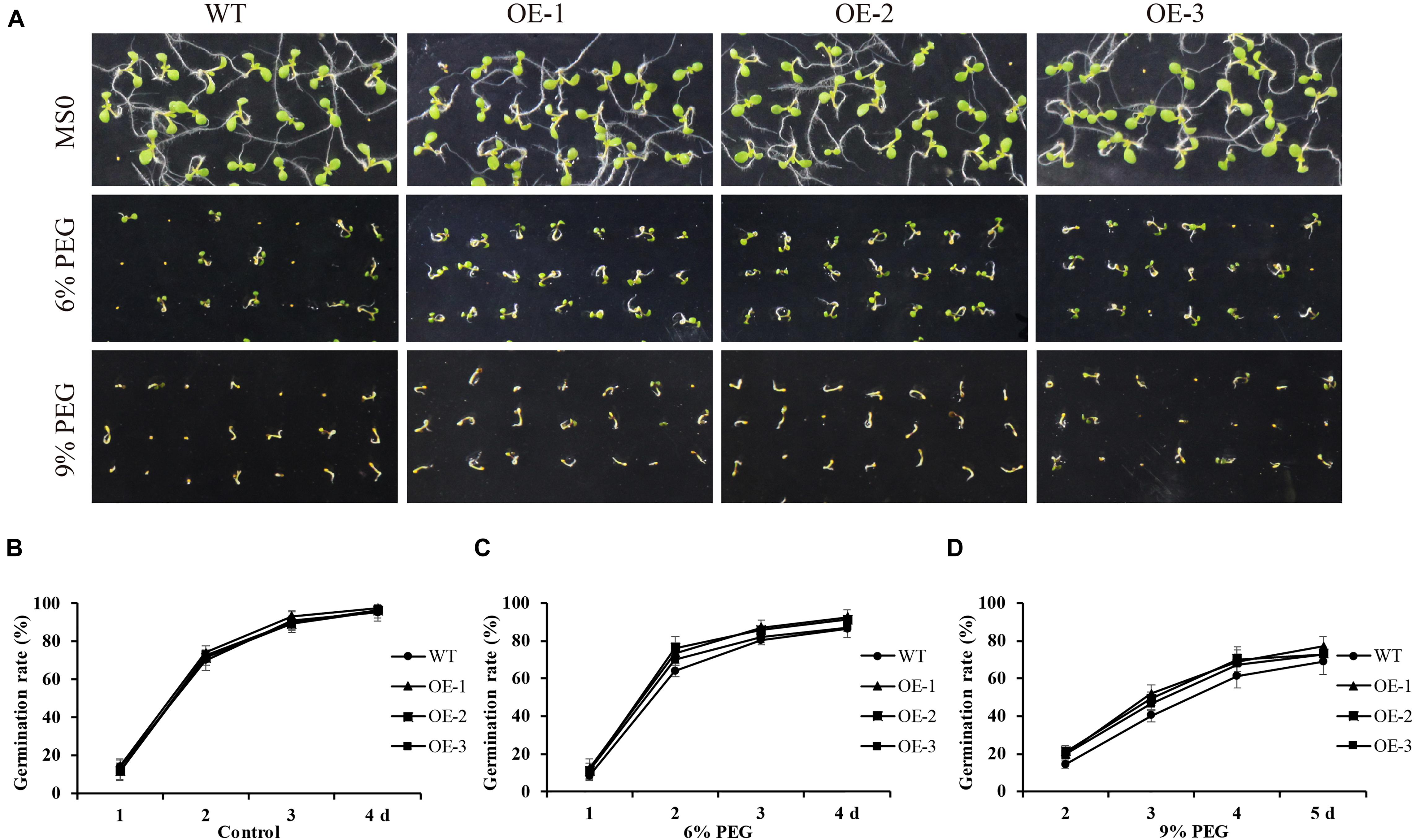
Figure 8. Overexpression of GmANK114 enhanced seed germination rates under PEG6000 treatment. (A) Seed germination analysis of different lines seeds under 6% and 9% PEG6000 to simulated drought treatment. (B–D) Seed germination rates of WT and GmANK114 transgenic Arabidopsis seeds at different time points. Date for each time point are means of three independent replicates.
To further investigate the role of GmANK114 under high salt, we observed the germination of overexpression GmANK114 transgenic Arabidopsis seeds grown on medium containing various salt concentrations. For the germination assay, seeds of transgenic lines and WT were germinated on 75, 100, and 125 mM NaCl (Figure 9A), and the germination rates of both WT and transgenic seeds were determined. There was no difference in germination rate between transgenic lines and WT seeds in MS medium (Figure 9B), but the germination time and the germination rate in transgenic lines was earlier and higher than that in WT when treated with NaCl, especially for 125 mM NaCl (Figures 9C–E).
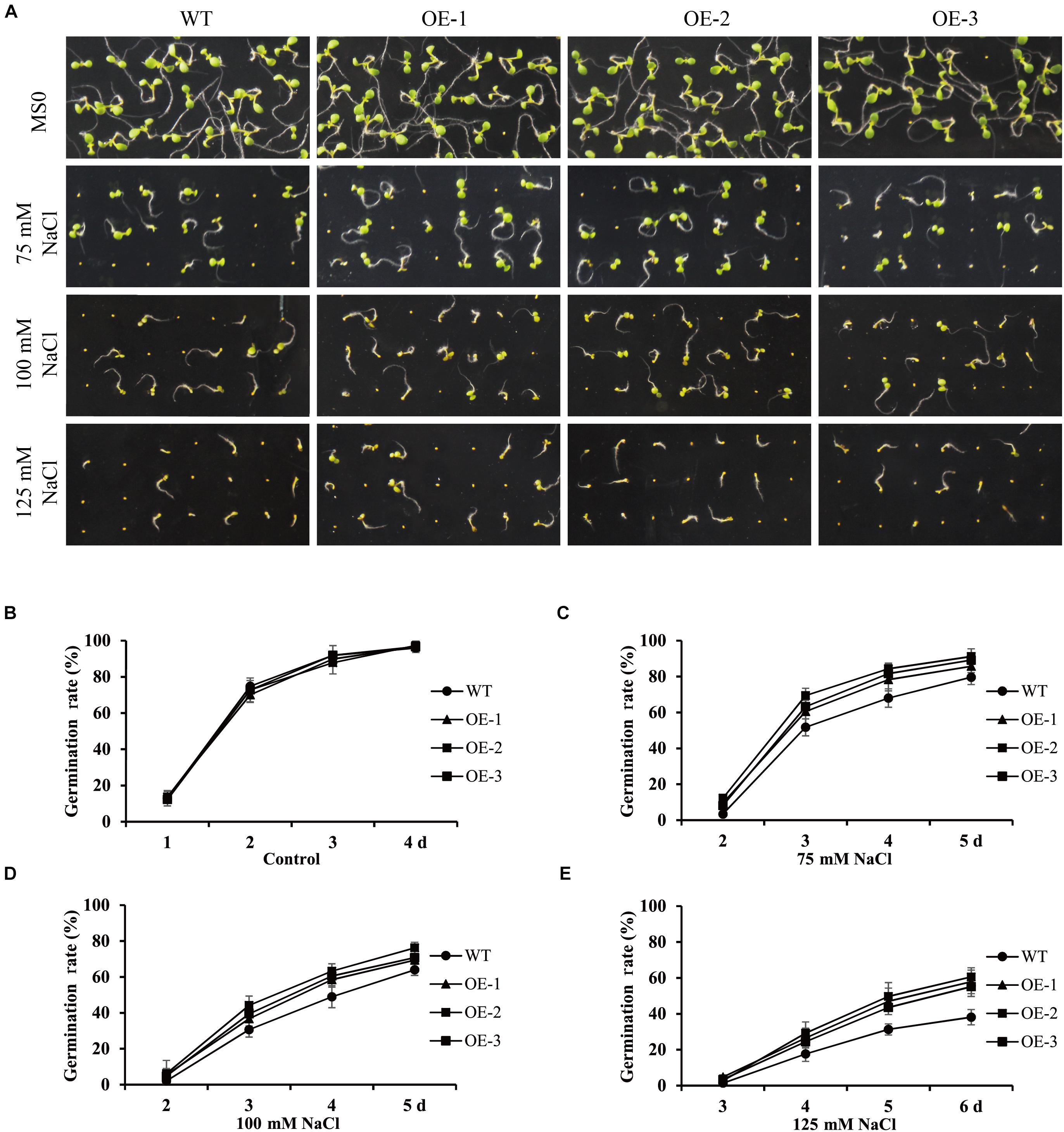
Figure 9. Germination rates of seeds in the presence or absence of NaCl in transgenic Arabidopsis. Values are means from three experiments. (A) Seed germination analysis of different lines seeds under 75, 100 and 125 mM NaCl to simulated salt treatment. (B–E) Seed germination rates of WT and GmANK114 transgenic Arabidopsis seeds at different time points. Date for each time point are means of three independent replicates. The error bars indicate SD.
GmANK114 Improved Drought and Salt Tolerance in Transgenic Soybean Hairy Roots
The function of GmANK114 in drought and salt tolerance was further investigated by performing similar abiotic stress assays in Agrobacterium rhizogenes-mediated soybean hairy roots. Quantitative real-time PCR analysis showed that the expression level of GmANK114 was significantly higher in hairy roots overexpressing GmANK114 (OE) compared with the empty vector (3301) control (Figure 10C). For drought treatment, all hairy root seedlings including control grew in soil with no water for 2 weeks, and then rewatered for one week. Morphologically, no significant differences were observed for all experimental plants under normal growth conditions. Drought treatment caused obvious differences in growth and physiology between GmANK114 overexpression lines and 3301-control. The leaves of all hairy root plants gradually yellowed and wilted with the drought treatment going on, but the control was more sensitive compared with the plants carrying the GmANK114 under drought treatment after 7 days (Figure 10A). After rewatered for 7 days, 66.7% of the transgenic plants were still alive, while the control plants had a survival rate of only 22.2% (Figure 10D). The same phenomenon occurred with NaCl treatment and the survival rate in transgenic hairy roots was higher than that in control (Figures 11A,C).
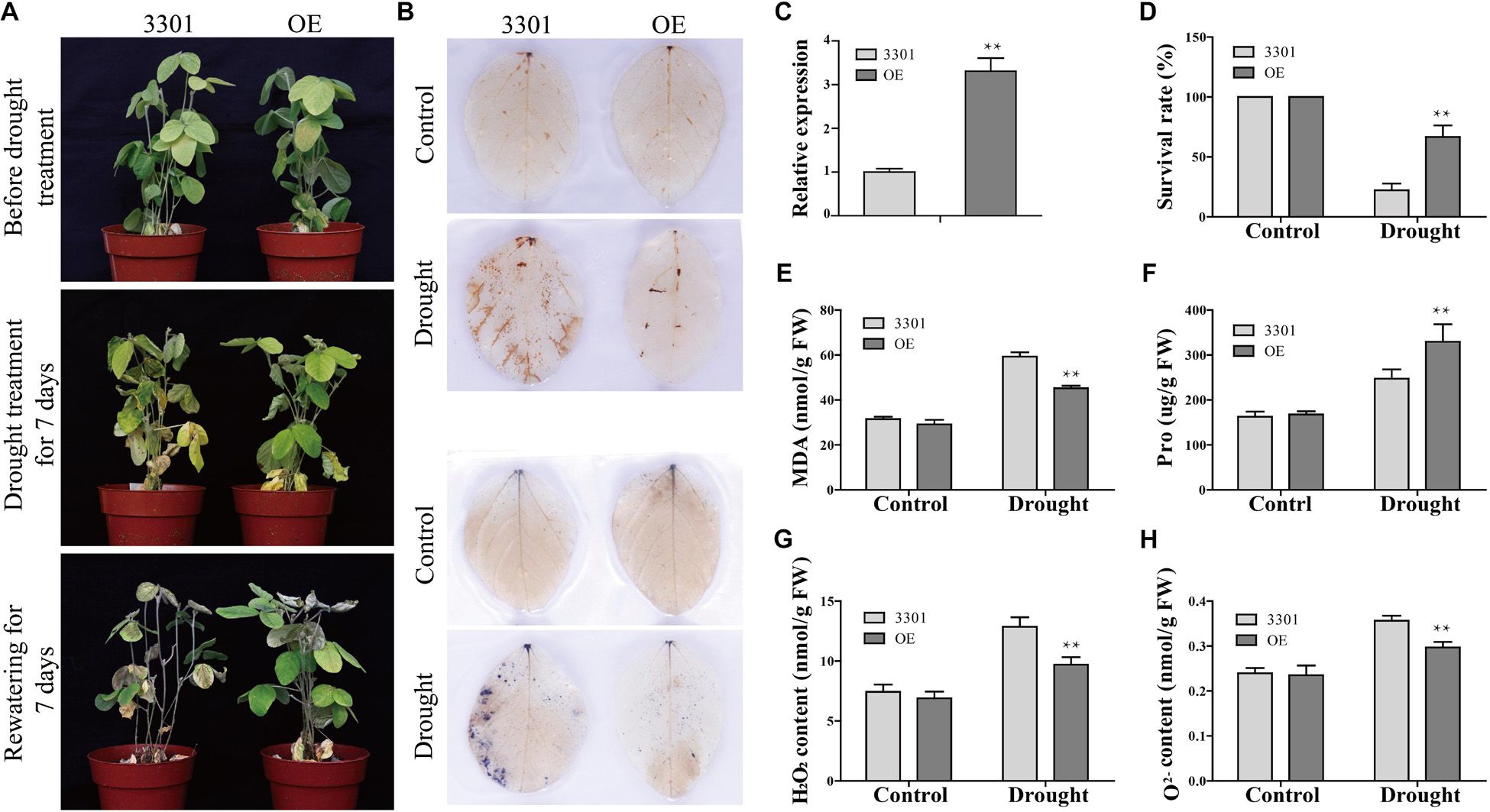
Figure 10. GmANK114 improves drought stress tolerance in transgenic soybean hairy roots. (A) Phenotypes of GmANK114-overexpression and 3301-control transgenic soybean plants under before and after drought treatment. (B) DAB (top) and NBT (bottom) staining of OE and 3301 plant leaves under drought treatments. (C) Relative GmANK114 expression in hairy roots of overexpressing GmANK114 and control as shown by qRT-PCR. (D) Survival rate of normal and drought-stressed plants. (E) MDA and (F) proline content were detected in OE and 3301 plants under drought or normal growth condition. (G)The content of H2O2 and (H) O2– in the leaves of GmANK114-OE and 3301-control plants after drought or normal condition for one week. The data were means ± SDs of three experiments. ANOVA test demonstrated that OE were significant differences (*P < 0.05 and **P < 0.01) compared with the corresponding controls.
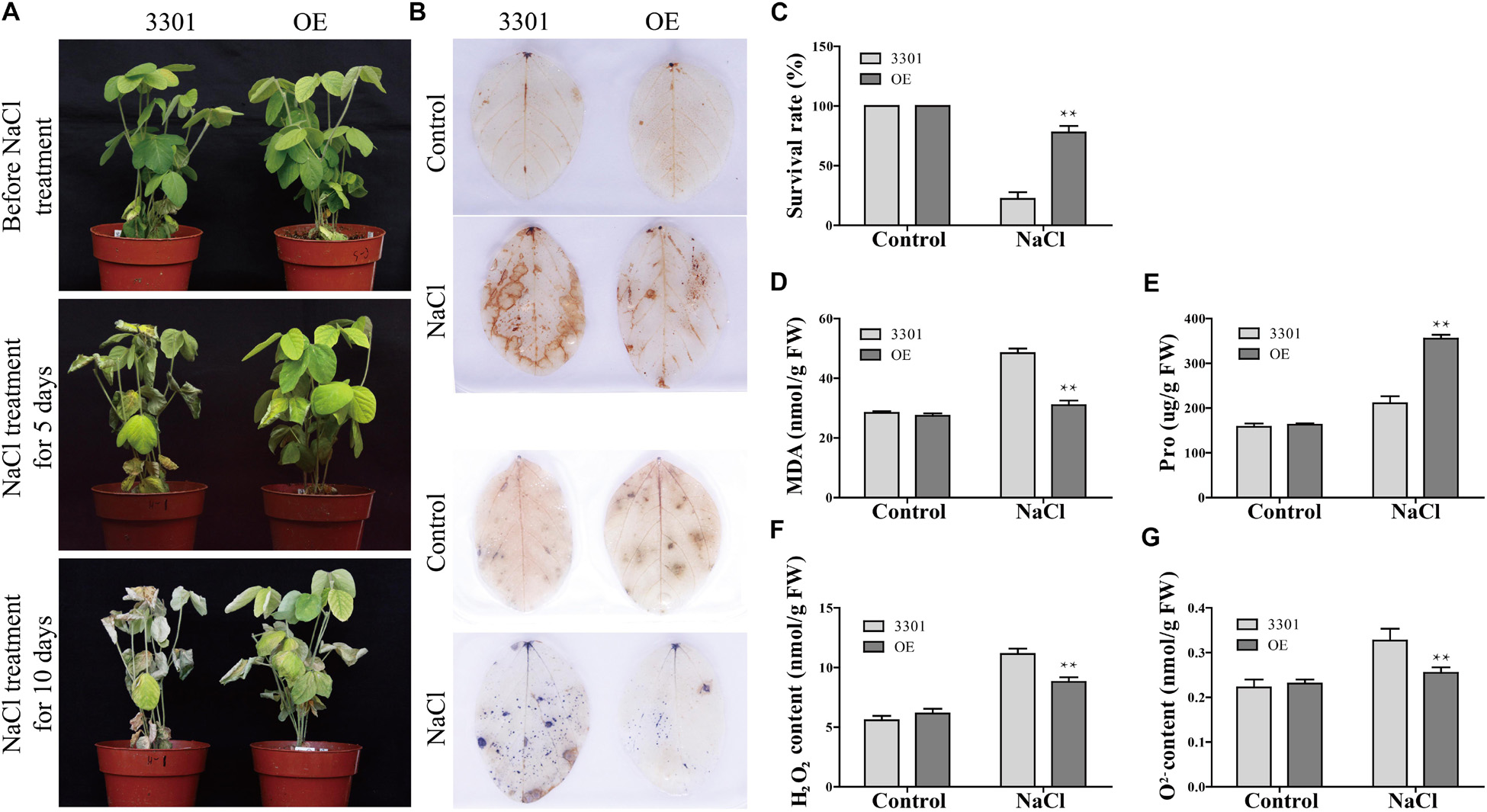
Figure 11. Phenotype and physiological indexes analysis of GmANK114 transgenic soybean hairy roots under salt stress. (A) Phenotypes was evaluated in transgenic soybean plants after NaCl treatment. (B) DAB (top) and NBT (bottom) staining of leaves of OE and 3301 plants under NaCl treatments. (C) Survival rate of soybean hairy roots grown with salt treatment. (D–G) MDA, proline, H2O2 and O2– contents in leaves of plants with transgenic hairy roots were measured under salt treatment for 7 days. All values are represented means for three biological replicates (n = 18). The error bars indicate SD. Significant differences (*P < 0.05 and **P < 0.01) are indicated by asterisks above the columns.
In order to analyze the mechanism in improving drought resistance of GmANK114, MDA and Pro contents were determined under normal growth and stress conditions. Under normal growth conditions, the MDA content, which were 31.5 and 29.2 nmol/g in transgenic lines and 3301, respectively, did not differ among all plants. While under drought conditions, the MDA content in transgenic soybean was 45.2 nmol/g, significantly lower than that in control (59.3 nmol/g) (Figure 10E). The same results were obtained with salt treatment: the MDA contents in transgenic hairy root and CK were 30.9 and 48.4 nmol/g, respectively (Figure 11D). Under both drought and salt treatments, the Pro content in transgenic lines was higher than that in CK. By contrast, there was no significant difference in the Pro contents between transgenic lines and control under normal condition (Figures 10F, 11E).
Abiotic stress leads to the accumulation of reactive oxygen species (ROS), which can affect plant growth and development. Soybean leaves were stained with DAB and NBT to detect hydrogen peroxide (H2O2) and superoxide (O2–) contents in 3301 and OE. Under normal growth condition, no significant difference was observed in all plant leaves between the 3301-control and GmANK114-OE. However, the color depth of the transgenic hairy root leaves was significantly lower than that of the 3301 plants under drought and salt treatments (Figures 10B, 11B). The contents of O2– and H2O2 in leaves were also measured and the results were consistent with the staining of DAB and NBT. Meanwhile, we found that the leaves of overexpressed GmANK114 plants produced lower levels of free oxygen radicals with drought and NaCl treatments (Figures 10G,H, 11F,G). These results indicated that GmANK114 positively regulated tolerance to drought and salt stresses in transgenic soybean hairy roots.
GmANK114 Activated Transcripts of Stress-Responsive Genes in Soybean
To investigate the possible tolerance mechanisms, several drought- and salt-related genes were analyzed in transgenic soybean hairy roots under normal and stress conditions (Figure 12). When grown in normal conditions, we found that there were no significant differences in the expression level of these genes between 3301 and GmANK114 OE lines by qRT-PCR. Under drought condition, the expression of bZIP1 (Gao et al., 2011) had no clear difference, whereas transcript levels of the other genes in OE lines were higher than that in the 3301 control, including WRKY13 (Zhou et al., 2008), NAC11 (Hao et al., 2011), DREB2 (Chen et al., 2007), MYB84 (Wang et al., 2017), and bZIP44 (Liao et al., 2008). Similarly, overexpression of GmANK114 regulated transcripts of WRKY13, NAC11, DREB2, MYB84, and bZIP44 under salt stress. These results indicated that GmANK114 may be a key signaling molecule regulating plant drought and salt responses.
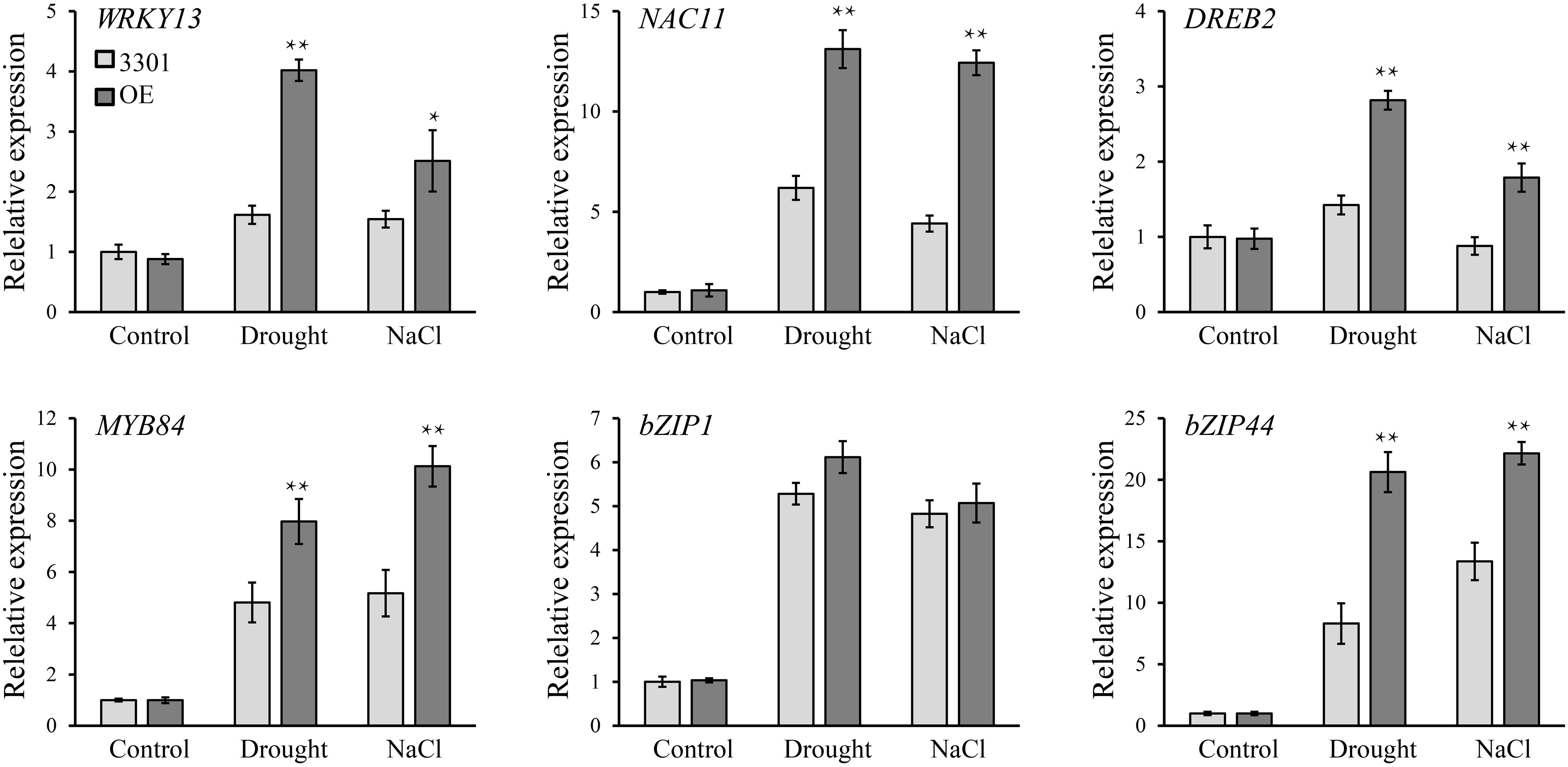
Figure 12. The relative transcript levels of WRKY13, NAC11, DREB3, MYB84, bZIP1 and bZIP44 in transgenic GmANK114 and 3301 soybeans. qRT-PCR was used to detect expression levels under normal and stress treatment and GmCYP2 was used as an internal control. Values are means and SD obtained from three biological replicates. The asterisks indicate a statistical significance (*P < 0.05 and **P < 0.01) compared with the corresponding controls.
Discussion
Drought and salt are the important factors that seriously affect the production and quality for soybean, especially for China which possess a population base. The ANK protein containing several conserved ankyrin repeats (2 to over 20) are important connexins protein that links membrane proteins and signaling molecules to the components of the underlying cytoskeleton (Li and Chye, 2004; Voronin and Kiseleva, 2008; Cunha and Mohler, 2009). ANK protein plays a key role in various cellular processes, participates in plant growth, development, intracellular protein transport, signal transduction, and stress responses (Wu et al., 2009; Brestic et al., 2015; Popescu et al., 2017). The study on the function of ANK protein will help to elucidate the mechanism that plants respond to environmental stresses, and provide the theoretical basis for environmental adaptation in plants.
Recently, the number of ANK proteins have been found is 105, 175, 130, and 71 in Arabidopsis, rice, tomato, and maize, respectively (Becerra et al., 2004; Huang et al., 2009; Jiang et al., 2013; Yuan et al., 2013). Although, it has been previously reported that 162 ankyrin repeats genes were found in soybean (Zhang et al., 2016), we identified 226 ANK genes in soybean and classified them into nine subfamilies. This difference maybe resulted from constantly updated in soybean genome database in recent years. The same subfamilies were found in Arabidopsis, rice, maize and soybean based on the domain compositions (Table 1), we inferred that these kinds of subfamilies were relatively conserved and they will take the similar functions in plants. The ANK-GPCR subfamily (protein with GPCR-chaperone1 domain) only found in tomato, but not in Arabidopsis, rice, maize and soybeans. Due to the differences in morphological structure, lifestyle and growth environment of plants evolution, some conserved subfamily genes have been retained to exercise the common physiological functions of plants, and specific genes have been developed to meet their own functional needs. ANK-GPCR subfamily genes may perform specific functions in tomato.
Several ANK genes may participate in responses to biotic and abiotic stresses. The expression of tobacco NEIP2 was induced in the first few hours by treatment with salt and ethylene and further enhances plant performance under salt and oxidative stresses (Cao et al., 2015). ANK domain are crucial for YrU1 resistance to stripe rust in wheat (Wang et al., 2020). Based on this evidence, it was speculated that the soybean ANK genes probably involved in biotic and abiotic stress responses.
When plants are subjected to environmental stress, the second messenger perceives the stimulus in the environment and then modulates intracellular Ca2+ levels. The change of Ca2+ concentration often initiates a protein phosphorylation cascade that finally transfers to transcription factors controlling specific stress-regulated genes (Xiong et al., 2002). Transcription factors regulate the expression of target genes by binding to downstream gene promoters, thereby enabling plants to adapt to changes in the external environment. ANK-RFs were previously reported to be related to pathogen defense, plant growth and development, such as XA21 and XB3 (Wang et al., 2006). In this study, multiple cis-elements were found in the promoter region of GmANK-RF genes, including MeJA-responsive element (CGTCA-motif and TGACG-motif), which showed that GmANK-RF genes could function in defense responses. In addition, a number of stress response elements were also identified in the ANK-RF genes promoter region, such as drought response element MYB, MYB-like, MBS, salt response element MYC, low temperature element LTR, and ABA response element ABRE (Table 3). This indicates that GmANK-RFs might participate in multiple abiotic and biotic stress responses.
In the present study, the soybean ANK-RF gene GmANK114 was shown to improve drought and salt tolerance in plants (Figures 10, 11). Proline functions in lowering the cellular osmotic potential and restoring intracellular solute concentrations, which prevents water loss from cells (Cui et al., 2018). MDA can inhibit the activity of cellular protective enzymes and reduce antioxidant content (Del Rio et al., 2005). When the enzyme and membrane system of plant tissues are destroyed, MDA content will be greatly increased (Tsikas, 2017). Therefore, MDA reflects the antioxidant capacity of plant tissues and can also be used as an indicator to measure the resistance of plants against external stress (Yang et al., 2019). Under drought and salt stresses, the differences of Pro and MDA contents between transgenic lines and controls showed that GmANK114 could confer tolerance of soybean to drought and salinity. The intracellular ROS content affects plant growth and development. H2O2 and O2– are the main sources of ROS, and can lead to the oxidative destruction of cells (Mittler et al., 2004). The contents of H2O2 and O2– in leaves of transgenic soybean were significantly lower than that in control. This indicates that GmANK114 gives soybean the ability to remove ROS. These results show that the GmANK114 gene is involved in abiotic stresses and can scavenge ROS.
Plants have developed flexibly molecular and cellular mechanisms to fight against various abiotic stresses. GmANK114 was induced by drought, NaCl, and ABA. To further explain the molecular mechanism of GmANK114 participating in abiotic stresses, the expression levels of drought- and salt-related marker genes were tested by qRT-PCR. Transcription factor DREB2 specifically binds DRE element containing core sequence -CCGAC- that is involved in rapid expression of salt and drought stress responsive genes in Arabidopsis (Sakuma et al., 2006). bZIP proteins are well known for responding to ABA and stresses signaling. Several bZIP proteins can bind to ABRE (–ACGT–) elements and activate expression of ABRE-driven reporter genes, including GmbZIP1 and GmbZIP44 which are induced by drought, NaCl, cold, and ABA (Liao et al., 2008; Gao et al., 2011). Besides, WRKY13, NAC11, and MYB84 are also involved in stress responses. Compared with controls, the expression profiles of stresses target genes have obvious differences in GmANK114 transgenic hairy roots under drought and salt stresses, especially for WRKY13, NAC11, DREB2, MYB84, and bZIP44 which were significantly up-regulated (Figure 12). This result suggest that GmANK114 could change the expression of drought- and salt-related marker genes. That’s probably because ANK114 indirectly affects the expression of these genes by affecting the function of other genes. However, it is not clear how ANK114 affects the function of other genes to enhance stress resistance in soybean. Therefore, further studies are necessary to clarify the mechanism of the GmANK114 participating in abiotic stresses.
Conclusion
A total of 226 ANK genes were identified from the soybean genome. A member of the ANK-RF subfamily, GmANK114, improved tolerance against drought and salt stresses. This study provides important clues for future functional analysis of ANK-RFs in regulating of drought- and salt-related signal pathways in soybean.
Data Availability Statement
The datasets presented in this study can be found in online repositories. The names of the repository/repositories and accession number(s) can be found in the article/Supplementary Material.
Author Contributions
Z-SX and D-HM coordinated the project, conceived and designed the experiments, and edited the manuscript. J-YZ performed the experiments and wrote the first draft. Z-WL and YS conducted the bioinformatic work and performed the experiments. Z-WF, JC, Y-BZ, and MC contributed with valuable discussions. Y-ZM coordinated the project. All authors have read and agreed to the published version of the manuscript.
Funding
This research was financially supported by the National Natural Science Foundation of China (31871624), the National Transgenic Key Project of the Chinese Ministry of Agriculture (2016ZX08002002-010), the Fundamental Research Funds for Central Non-Profit Institute of Crop Sciences, Chinese Academy of Agricultural Sciences (Y2020PT12 and S2020ZC02), and the Programme of Introducing Talents of Innovative Discipline to Universities (Project 111) from the State Administration of Foreign Experts Affairs (#B18042) “Crop breeding for disease resistance and genetic improvement”.
Conflict of Interest
The authors declare that the research was conducted in the absence of any commercial or financial relationships that could be construed as a potential conflict of interest.
Acknowledgments
We are grateful to Dr. Lijuan Qiu from Crop Science, Chinese Academy of Agricultural Sciences for providing the soybean seeds.
Supplementary Material
The Supplementary Material for this article can be found online at: https://www.frontiersin.org/articles/10.3389/fpls.2020.584167/full#supplementary-material
Supplementary Figure 1 | Gene structure analysis of the 226 GmANK genes. Untranslated regions (upstream/downstream) are displayed by blue box. Introns and exons are shown by black lines and yellow boxes, respectively. The lengths of introns and exons of each gene were displayed proportionally.
Supplementary Figure 2 | Putative motifs of each soybean ANK proteins.
Supplementary Figure 3 | Gene Ontology (GO) annotation for the GmANK proteins. BP: biological processes; MF: molecular functions; CC: cellular components.
Supplementary Figure 4 | Sequence alignment of ANK-RF proteins in soybean.
Supplementary Figure 5 | Germination rates of seeds in transgenic Arabidopsis under PEG6000 and NaCl treatments.
Supplementary Table 1 | Description of soybean ANK genes.
Supplementary Table 2 | FPKM values of different tissues of soybean ANK-RF genes.
Supplementary Table 3 | FPKM values under drought, NaCl, and ABA treatment of soybean ANK-RF genes.
Supplementary Table 4 | Primers used in this study.
Supplementary Table 5 | Gene Ontology (GO) annotation for the GmANK proteins.
Footnotes
- ^ http://pfam.sanger.ac.uk
- ^ https://phytozome.jgi.doe.gov/pz/portal.html
- ^ http://toolkit.tuebingen.mpg.de/blastclust
- ^ http://gsds.cbi.pku.edu.cn/
- ^ http://meme-suite.org/tools/meme
- ^ http://www.dna.affrc.go.jp/PLACE/
- ^ http://bioinformatics.psb.ugent.be/webtools/plantcare/html/
- ^ http://mg2c.iask.in/mg2c_v2.0/
- ^ http://chibba.pgml.uga.edu/mcscan2/
- ^ http://circos.ca/
- ^ http://www.blast2go.com
References
Albert, S., Després, B., Guilleminot, J., Bechtold, N., Pelletier, G., Delseny, M., et al. (1999). The EMB506 gene encodes a novel ankyrin repeat containing protein that is essential for the normal development of Arabidopsis embryos. Plant J. 17, 169–179. doi: 10.1046/j.1365-313x.1999.00361.x
Artimo, P., Jonnalagedda, M., Arnold, K., Baratin, D., Csardi, G., de Castro, E., et al. (2012). ExPASy: SIB bioinformatics resource portal. Nucleic Acids Res. 40, W597–W603. doi: 10.1093/nar/gks400
Becerra, C., Jahrmann, T., Puigdomenech, P., and Vicient, C. M. (2004). Ankyrin repeat-containing proteins in Arabidopsis: characterization of a novel and abundant group of genes coding ankyrin-transmembrane proteins. Gene 340, 111–121. doi: 10.1016/j.gene.2004.06.006
Berrocal-Lobo, M., Stone, S. L., Yang, X., Antico, J., Callis, J., Ramonell, K. M., et al. (2010). ATL9, a RING zinc finger protein with E3 ubiquitin ligase activity implicated in chitin- and NADPH oxidase-mediated defense responses. PLoS One 5:e14426. doi: 10.1371/journal.pone.0014426
Brestic, M., Zivcak, M., Kunderlikova, K., Sytar, O., Shao, H., Kalaji, H. M., et al. (2015). Low PSI content limits the photoprotection of PSI and PSII in early growth stages of chlorophyll b-deficient wheat mutant lines. Photosynth. Res. 125, 151–166. doi: 10.1007/s11120-015-0093-1
Cao, Y. R., Chen, H. W., Li, Z. G., Tao, J. J., Ma, B., Zhang, W. K., et al. (2015). Tobacco ankyrin protein NEIP2 interacts with ethylene receptor NTHK1 and regulates plant growth and stress responses. Plant Cell Physiol. 56, 803–818. doi: 10.1093/pcp/pcv009
Carvalho, S. D., Saraiva, R., Maia, T. M., Abreu, I. A., and Duque, P. (2012). XBAT35, a novel Arabidopsis RING E3 ligase exhibiting dual targeting of its splice isoforms, is involved in ethylene-mediated regulation of apical hook curvature. Mol. Plant 5, 1295–1309. doi: 10.1093/mp/sss048
Chen, C., Chen, H., Zhang, Y., Thomas, H. R., Frank, M. H., He, Y., et al. (2020). TBtools – an integrative toolkit developed for interactive analyses of big biological data. Mol. Plant 13:116. doi: 10.1016/j.molp.2020.06.009
Chen, M., Wang, Q. Y., Cheng, X. G., Xu, Z. S., Li, L. C., Ye, X. G., et al. (2007). GmDREB2, a soybean DRE-binding transcription factor, conferred drought and high-salt tolerance in transgenic plants. Biochem. Biophys. Res. Commun. 353, 299–305. doi: 10.1016/j.bbrc.2006.12.027
Chen, T. H., and Murata, N. (2011). Glycinebetaine protects plants against abiotic stress: mechanisms and biotechnological applications. Plant Cell Environ. 34, 1–20. doi: 10.1111/j.1365-3040.2010.02232.x
Conesa, A., and Gotz, S. (2008). Blast2GO: a comprehensive suite for functional analysis in plant genomics. Int. J. Plant Genomics 2008:619832. doi: 10.1155/2008/619832
Cui, X. Y., Du, Y. T., Fu, J. D., Yu, T. F., Wang, C. T., Chen, M., et al. (2018). Wheat CBL-interacting protein kinase 23 positively regulates drought stress and ABA responses. BMC Plant Biol. 18:93. doi: 10.1186/s12870-018-1306-5
Cunha, S. R., and Mohler, P. J. (2009). Ankyrin protein networks in membrane formation and stabilization. J. Cell. Mol. Med. 13, 4364–4376. doi: 10.1111/j.1582-4934.2009.00943.x
Del Rio, D., Stewart, A. J., and Pellegrini, N. (2005). A review of recent studies on malondialdehyde as toxic molecule and biological marker of oxidative stress. Nutr. Metab. Cardiovasc. Dis. 15, 316–328. doi: 10.1016/j.numecd.2005.05.003
Du, Y. T., Zhao, M. J., Wang, C. T., Gao, Y., Wang, Y. X., Liu, Y. W., et al. (2018). Identification and characterization of GmMYB118 responses to drought and salt stress. BMC Plant Biol. 18:320. doi: 10.1186/s12870-018-1551-7
Elenbaas, B., Dobbelstein, M., Roth, J., Shenk, T., and Levine, A. J. (1996). The MDM2 oncoprotein binds specifically to RNA through its RING finger domain. Mol. Med. 2, 439–451. doi: 10.1007/bf03401903
Fan, K., Yuan, S., Chen, J., Chen, Y., Li, Z., Lin, W., et al. (2019). Molecular evolution and lineage-specific expansion of the PP2C family in Zea mays. Planta 250, 1521–1538. doi: 10.1007/s00425-019-03243-x
Freemont, P. S., Hanson, I. M., and Trowsdale, J. (1991). A novel gysteine-rich sequence motif. Cell 64, 483–484. doi: 10.1016/0092-8674(91)90229-R
Gao, S. Q., Chen, M., Xu, Z. S., Zhao, C. P., Li, L., Xu, H. J., et al. (2011). The soybean GmbZIP1 transcription factor enhances multiple abiotic stress tolerances in transgenic plants. Plant Mol. Biol. 75, 537–553. doi: 10.1007/s11103-011-9738-4
Garcion, C., Guilleminot, J., Kroj, T., Parcy, F., Giraudat, J., and Devic, M. (2006). AKRP and EMB506 are two ankyrin repeat proteins essential for plastid differentiation and plant development in Arabidopsis. Plant J. 48, 895–906. doi: 10.1111/j.1365-313X.2006.02922.x
Guo, A.-Y., Zhu, Q.-H., Chen, X., and Luo, J.-C. (2007). GSDS: a gene structure display server. Yi Chuan 29, 1023–1026. doi: 10.1360/yc-007-1023
Hao, Y. J., Wei, W., Song, Q. X., Chen, H. W., Zhang, Y. Q., Wang, F., et al. (2011). Soybean NAC transcription factors promote abiotic stress tolerance and lateral root formation in transgenic plants. Plant J. 68, 302–313. doi: 10.1111/j.1365-313X.2011.04687.x
Hasanuzzaman, M., Hossain, M. A., da Silva, J. T., and Fujita, M. (2012). “Plant response and tolerance to abiotic oxidative stress: antioxidant defense is a key factor,” in Crop Stress and its Management: Perspectives and Strategies, eds B. Venkateswarlu, A. Shanker, C. Shanker, and M. Maheswari (Dordrecht: Springer), 261–315. doi: 10.1007/978-94-007-2220-0_8
Holub, E. B. (2001). The arms race is ancient history in Arabidopsis, the wildflower. Nat. Rev. Genet. 2, 516–527. doi: 10.1038/35080508
Huang, J., Chen, F., Del Casino, C., Autino, A., Shen, M., Yuan, S., et al. (2006). An ankyrin repeat-containing protein, characterized as a ubiquitin ligase, is closely associated with membrane-enclosed organelles and required for pollen germination and pollen tube growth in lily. Plant Physiol. 140, 1374–1383. doi: 10.1104/pp.105.074922
Huang, J., Zhao, X., Yu, H., Ouyang, Y., Wang, L., and Zhang, Q. (2009). The ankyrin repeat gene family in rice: genome-wide identification, classification and expression profiling. Plant Mol. Biol. 71, 207–226. doi: 10.1007/s11103-009-9518-6
Jiang, H., Wu, Q., Jin, J., Sheng, L., Yan, H., Cheng, B., et al. (2013). Genome-wide identification and expression profiling of ankyrin-repeat gene family in maize. Dev. Genes Evol. 223, 303–318. doi: 10.1007/s00427-013-0447-7
Kereszt, A., Li, D., Indrasumunar, A., Nguyen, C. T., Nontachaiyapoom, S., Kinkema, M., et al. (2007). Agrobacterium rhizogenes-mediated transformation of soybean to study root biology. Nat. Protoc. 2, 948–952. doi: 10.1038/nprot.2007.141
Letunic, I., Doerks, T., and Bork, P. (2009). SMART 6: recent updates and new developments. Nucleic Acids Res. 37, D229–D232. doi: 10.1093/nar/gkn808
Li, B., Liu, Y., Cui, X. Y., Fu, J. D., Zhou, Y. B., Zheng, W. J., et al. (2019). Genome-wide characterization and expression analysis of soybean TGA transcription factors identified a novel TGA gene involved in drought and salt tolerance. Front. Plant Sci. 10:549. doi: 10.3389/fpls.2019.00549
Li, H.-Y., and Chye, M.-L. (2004). Arabidopsis Acyl-CoA-binding protein ACBP2 interacts with an ethylene-responsive element-binding protein, AtEBP, via its ankyrin repeats. Plant Mol. Biol. 54, 233–243. doi: 10.1023/b:plan.0000028790.75090.ab
Liao, Y., Zou, H. F., Wei, W., Hao, Y. J., Tian, A. G., Huang, J., et al. (2008). Soybean GmbZIP44, GmbZIP62 and GmbZIP78 genes function as negative regulator of ABA signaling and confer salt and freezing tolerance in transgenic Arabidopsis. Planta 228, 225–240. doi: 10.1007/s00425-008-0731-3
Liu, J. M., Xu, Z. S., Lu, P. P., Li, W. W., Chen, M., Guo, C. H., et al. (2016a). Genome-wide investigation and expression analyses of the pentatricopeptide repeat protein gene family in foxtail millet. BMC Genomics 17:840. doi: 10.1186/s12864-016-3184-2
Liu, J. M., Zhao, J. Y., Lu, P. P., Chen, M., Guo, C. H., Xu, Z. S., et al. (2016b). The E-subgroup pentatricopeptide repeat protein family in Arabidopsis thaliana and confirmation of the responsiveness PPR96 to abiotic stresses. Front. Plant Sci. 7:1825. doi: 10.3389/fpls.2016.01825
Lopez-Ortiz, C., Pena-Garcia, Y., Natarajan, P., Bhandari, M., Abburi, V., Dutta, S. K., et al. (2020). The ankyrin repeat gene family in capsicum spp: genome-wide survey, characterization and gene expression profile. Sci. Rep. 10:4044. doi: 10.1038/s41598-020-61057-4
Lorick, K. L., Jensen, J. P., Fang, S., Ong, A. M., Hatakeyama, S., and Weissman, A. M. (1999). RING fingers mediate ubiquitin-conjugating enzyme (E2)-dependent ubiquitination. Proc. Natl. Acad. Sci. U.S.A. 96, 11364–11369. doi: 10.1073/pnas.96.20.11364
Lu, H., Liu, Y., and Greenberg, J. T. (2005). Structure-function analysis of the plasma membrane- localized Arabidopsis defense component ACD6. Plant J. 44, 798–809. doi: 10.1111/j.1365-313X.2005.02567.x
Lu, H., Rate, D. N., Song, J. T., and Greenberg, J. T. (2003). ACD6, a novel ankyrin protein, is a regulator and an effector of salicylic acid signaling in the Arabidopsis defense response. Plant Cell 15, 2408–2420. doi: 10.1105/tpc.015412
Lyzenga, W. J., Booth, J. K., and Stone, S. L. (2012). The Arabidopsis RING-type E3 ligase XBAT32 mediates the proteasomal degradation of the ethylene biosynthetic enzyme, 1-aminocyclopropane-1-carboxylate synthase 7. Plant J. 71, 23–34. doi: 10.1111/j.1365-313X.2012.04965.x
Michaely, P., and Bennett, V. (1992). The ANK repeat: a ubiquitous motif involved in macromolecular recognition. Trends Cell Biol. 2, 127–129. doi: 10.1016/0962-8924(92)90084-z
Miles, M. C., Janket, M. L., Wheeler, E. D., Chattopadhyay, A., Majumder, B., Dericco, J., et al. (2005). Molecular and functional characterization of a novel splice variant of ANKHD1 that lacks the KH domain and its role in cell survival and apoptosis. FEBS J. 272, 4091–4102. doi: 10.1111/j.1742-4658.2005.04821.x
Mittler, R., Vanderauwera, S., Gollery, M., and Van Breusegem, F. (2004). Reactive oxygen gene network of plants. Trends Plant Sci. 9, 490–498. doi: 10.1016/j.tplants.2004.08.009
Mosavi, L. K., Cammett, T. J., Desrosiers, D. C., and Peng, Z.-y (2004). The ankyrin repeat as molecular architecture for protein recognition. Protein Sci. 13, 1435–1448. doi: 10.1110/ps.03554604
Nodzon, L. A., Xu, W. H., Wang, Y., Pi, L. Y., Chakrabarty, P. K., and Song, W. Y. (2004). The ubiquitin ligase XBAT32 regulates lateral root development in Arabidopsis. Plant J. 40, 996–1006. doi: 10.1111/j.1365-313X.2004.02266.x
Popescu, S. C., Brauer, E. K., Dimlioglu, G., and Popescu, G. V. (2017). Insights into the structure, function, and ion-mediated signaling pathways transduced by plant integrin-linked kinases. Front. Plant Sci. 8:376. doi: 10.3389/fpls.2017.00376
Prasad, M. E., Schofield, A., Lyzenga, W., Liu, H., and Stone, S. L. (2010). Arabidopsis RING E3 ligase XBAT32 regulates lateral root production through its role in ethylene biosynthesis. Plant Physiol. 153, 1587–1596. doi: 10.1104/pp.110.156976
Qi, J., Song, C. P., Wang, B., Zhou, J., Kangasjarvi, J., Zhu, J. K., et al. (2018). Reactive oxygen species signaling and stomatal movement in plant responses to drought stress and pathogen attack. J. Integr. Plant Biol. 60, 805–826. doi: 10.1111/jipb.12654
Sakuma, Y., Maruyama, K., Osakabe, Y., Qin, F., Seki, M., Shinozaki, K., et al. (2006). Functional analysis of an Arabidopsis transcription factor, DREB2A, involved in drought-responsive gene expression. Plant Cell 18, 1292–1309. doi: 10.1105/tpc.105.035881
Sedgwick, S. G., and Smerdon, S. J. (1999). The ankyrin repeat: a diversity of interactions on a common structural framework. Trends Biochem. Sci. 24, 311–316. doi: 10.1016/s0968-0004(99)01426-7
Seong, E. S., Cho, H. S., Choi, D., Joung, Y. H., Lim, C. K., Hur, J. H., et al. (2007a). Tomato plants overexpressing CaKR1 enhanced tolerance to salt and oxidative stress. Biochem. Biophys. Res. Commun. 363, 983–998. doi: 10.1016/j.bbrc.2007.09.104
Seong, E. S., Choi, D., Cho, H. S., Lim, C. K., Cho, H. J., and Wang, M. H. (2007b). Characterization of a stress-responsive ankyrin repeat containing zinc finger protein of capsicum annuum (CaKR1). J. Biolchem. Mol. Biol. 40, 952–958. doi: 10.5483/bmbrep.2007.40.6.952
Shi, W. Y., Du, Y. T., Ma, J., Min, D. H., Jin, L. G., Chen, J., et al. (2018). The WRKY transcription factor GmWRKY12 confers drought and salt tolerance in soybean. Int. J. Mol. Sci. 19:4087. doi: 10.3390/ijms19124087
Stone, S. L., Hauksdottir, H., Troy, A., Herschleb, J., Kraft, E., and Callis, J. (2005). Functional analysis of the RING-type ubiquitin ligase family of Arabidopsis. Plant Physiol. 137, 13–30. doi: 10.1104/pp.104.052423
Tamura, K., Peterson, D., Peterson, N., Stecher, G., Nei, M., and Kumar, S. (2011). MEGA5: molecular evolutionary genetics analysis using maximum likelihood, evolutionary distance, and maximum parsimony methods. Mol. Biol. Evol. 28, 2731–2739. doi: 10.1093/molbev/msr121
Thompson, J. D., Gibson, T. J., Plewniak, F., Jeanmougin, F., and Higgins, D. G. (1997). The CLUSTAL_X windows interface: flexible strategies for multiple sequence alignment aided by quality analysis tools. Nucleic Acids Res. 25, 4876–4882. doi: 10.1093/nar/25.24.4876
Tsikas, D. (2017). Assessment of lipid peroxidation by measuring malondialdehyde (MDA) and relatives in biological samples: analytical and biological challenges. Anal. Biochem. 524, 13–30. doi: 10.1016/j.ab.2016.10.021
Veatch-Blohm, M. E. (2007). Principles of plant genetics and breeding. Crop Sci. 47:385. doi: 10.2135/cropsci2007.05.0002br
Voronin, D. A., and Kiseleva, E. V. (2008). Functional role of proteins containing ankyrin repeats. Cell Tissue Biol. 2, 1–12. doi: 10.1134/s1990519x0801001x
Wang, F., Chen, H. W., Li, Q. T., Wei, W., Li, W., Zhang, W. K., et al. (2015). GmWRKY27 interacts with GmMYB174 to reduce expression of GmNAC29 for stress tolerance in soybean plants. Plant J. 83, 224–236. doi: 10.1111/tpj.12879
Wang, H., Zou, S., Li, Y., Lin, F., and Tang, D. (2020). An ankyrin-repeat and WRKY-domain-containing immune receptor confers stripe rust resistance in wheat. Nat. Commun. 11:1353. doi: 10.1038/s41467-020-15139-6
Wang, M., Yue, H., Feng, K., Deng, P., Song, W., and Nie, X. (2016). Genome-wide identification, phylogeny and expressional profiles of mitogen activated protein kinase kinase kinase (MAPKKK) gene family in bread wheat (Triticum aestivum L.). BMC Genomics 17:668. doi: 10.1186/s12864-016-2993-7
Wang, N., Zhang, W., Qin, M., Li, S., Qiao, M., Liu, Z., et al. (2017). Drought tolerance conferred in soybean (Glycine max. L) by GmMYB84, a novel R2R3-MYB transcription factor. Plant Cell Physiol. 58, 1764–1776. doi: 10.1093/pcp/pcx111
Wang, Y.-S., Pi, L.-Y., Chen, X., Chakrabarty, P. K., Jiang, J., De Leon, A. L., et al. (2006). Rice XA21 binding protein 3 is a ubiquitin ligase required for full Xa21-mediated disease resistance. Plant Cell 18, 3635–3646. doi: 10.1105/tpc.106.046730
Wu, T., Tian, Z., Liu, J., Yao, C., and Xie, C. (2009). A novel ankyrin repeat-rich gene in potato, Star, involved in response to late blight. Biochem. Genet. 47, 439–450. doi: 10.1007/s10528-009-9238-2
Xiong, L., Schumaker, K. S., and Zhu, J. K. (2002). Cell signaling during cold, drought, and salt stress. Plant Cell 14, S165–S183. doi: 10.1105/tpc.000596
Yang, R., Chen, M., Sun, J. C., Yu, Y., Min, D. H., Chen, J., et al. (2019). Genome-wide analysis of LIM family genes in foxtail millet (Setaria italica L.) and characterization of the role of SiWLIM2b in drought tolerance. Int. J. Mol. Sci. 20:1303. doi: 10.3390/ijms20061303
Yang, X., Sun, C., Hu, Y., and Lin, Z. (2008). Molecular cloning and characterization of a gene encoding RING zinc finger ankyrin protein from drought-tolerant Artemisia desertorum. J. Biosci. 33, 103–112. doi: 10.1007/s12038-008-0026-7
Yang, Y., and Guo, Y. (2018). Unraveling salt stress signaling in plants. J. Integr. Plant Biol. 60, 796–804. doi: 10.1111/jipb.12689
Yuan, X., Zhang, S., Qing, X., Sun, M., Liu, S., Su, H., et al. (2013). Superfamily of ankyrin repeat proteins in tomato. Gene 523, 126–136. doi: 10.1016/j.gene.2013.03.122
Zhang, D., Wan, Q., He, X., Ning, L., Huang, Y., Xu, Z., et al. (2016). Genome-wide characterization of the ankyrin repeats gene family under salt stress in soybean. Sci. Total Environ. 568, 899–909. doi: 10.1016/j.scitotenv.2016.06.078
Zhang, H., Scheirer, D. C., Fowle, W. H., and Goodman, H. M. (1992). Expression of antisense or sense RNA of an ankyrin repeat-containing gene blocks chloroplast differentiation in Arabidopsis. Plant Cell 4, 1575–1588. doi: 10.1105/tpc.4.12.1575
Zhang, X. Z., Zheng, W. J., Cao, X. Y., Cui, X. Y., Zhao, S. P., Yu, T. F., et al. (2019). Genomic analysis of stress associated proteins in soybean and the role of GmSAP16 in abiotic stress responses in Arabidopsis and soybean. Front. Plant Sci. 10:1453. doi: 10.3389/fpls.2019.01453
Zhou, Q. Y., Tian, A. G., Zou, H. F., Xie, Z. M., Lei, G., Huang, J., et al. (2008). Soybean WRKY-type transcription factor genes, GmWRKY13, GmWRKY21, and GmWRKY54, confer differential tolerance to abiotic stresses in transgenic Arabidopsis plants. Plant Biotechnol. J. 6, 486–503. doi: 10.1111/j.1467-7652.2008.00336.x
Keywords: ankyrin repeat protein, genome-wide analysis, responsive mechanism, drought and salt tolerance, soybean
Citation: Zhao J-Y, Lu Z-W, Sun Y, Fang Z-W, Chen J, Zhou Y-B, Chen M, Ma Y-Z, Xu Z-S and Min D-H (2020) The Ankyrin-Repeat Gene GmANK114 Confers Drought and Salt Tolerance in Arabidopsis and Soybean. Front. Plant Sci. 11:584167. doi: 10.3389/fpls.2020.584167
Received: 16 July 2020; Accepted: 06 October 2020;
Published: 29 October 2020.
Edited by:
Zhi Chang Chen, Fujian Agriculture and Forestry University, ChinaReviewed by:
Dezhi Wu, Zhejiang University, ChinaUmesh K. Reddy, West Virginia State University, United States
Copyright © 2020 Zhao, Lu, Sun, Fang, Chen, Zhou, Chen, Ma, Xu and Min. This is an open-access article distributed under the terms of the Creative Commons Attribution License (CC BY). The use, distribution or reproduction in other forums is permitted, provided the original author(s) and the copyright owner(s) are credited and that the original publication in this journal is cited, in accordance with accepted academic practice. No use, distribution or reproduction is permitted which does not comply with these terms.
*Correspondence: Zhao-Shi Xu, xuzhaoshi@caas.cn; Dong-Hong Min, mdh2493@126.com
†These authors have contributed equally to this work
‡Present address: Zhi-Wei Lu, Zhanjiang City Key Laboratory for Tropical Crops Genetic Improvement, South Subtropical Crops Institute, Chinese Academy of Tropical Agricultural Sciences, Zhanjiang, China