- 1Research Institute for Nature and Forest, Geraardsbergen, Belgium
- 2Research Institute for Nature and Forest, Brussels, Belgium
- 3Public Waste Agency of Flanders, Mechelen, Belgium
Exotic Populus taxa pose a threat to the success of riparian forest restoration in floodplain areas. We evaluated the impact of exotic Populus taxa on softwood riparian forest development along the river Common Meuse after introducing native Populus nigra and after the re-establishment of the natural river dynamics. We sampled 154 poplar seedlings that spontaneously colonized restored habitat and assessed their taxonomy based on diagnostic chloroplast and nuclear microsatellite markers. Furthermore, by using a paternity analysis on 72 seedlings resulting from six open pollinated P. nigra females, we investigated natural hybridization between frequently planted cultivated poplars and native P. nigra. The majority of the poplar seedlings from the gravel banks analyzed where identified as P. nigra; only 2% of the sampled seedlings exhibited genes of exotic poplar species. Similarly, the majority of the seedlings from the open pollinated progenies were identified as P. nigra. For three seedlings (4%), paternity was assigned to a cultivar of P. × canadensis. Almost two decades after reintroducing P. nigra, the constitution of the seed and pollen pools changed in the study area in favor of reproduction of the native species and at the expense of the exotic poplar species. This study indicates that, although significant gene flow form exotic poplars is observed in European floodplains, restoration programs of the native P. nigra can vigorously outcompete the exotic gene flows and strongly reduce the impact of exotic Populus taxa on the softwood riparian forest development.
Introduction
The restoration and protection of riparian forests is one of the key priorities in biodiversity conservation and climate change adaptation strategies (e.g., EU Biodiversity Strategy 2030, EU Floods Directive 2007/60/EC). Riparian forests are biodiversity hotspots and provide a range of ecosystem services including flood protection, prevention of bank erosion, thermal regulation by forest canopy cover, and water quality protection (Van Looy et al., 2013). They are therefore necessary for the ecological functioning of riparian corridors and are recognized as an important part of the world’s natural capital.
After decades of ecological degradation caused by human activities, including impoundment and straightening of rivers, cutting off meanders, drainage of floodplains and deforestation for agriculture and plantations, several European countries have undertaken actions to restore natural river dynamics (e.g., Schindler et al., 2016; Mansourian et al., 2019), and the European Commission proposed a Biodiversity Strategy planning to restore at least 25,000 km of free-flowing rivers (UE COM Biodiversity Strategy 20/05/2020). Generally, restoration activities involve dyke relocation, lowering of river banks and floodplains to restore the floodplain habitat heterogeneity, an essential feature for the regeneration of riparian forests. However, many restoration projects are poorly documented and written records on the outcome of restoration projects are generally lacking (Palmer et al., 2007).
Revegetation of river margins is a widely applied technique for riparian forest restoration (Palmer et al., 2007). However, exotic, invasive alien species can significantly undermine efforts to protect and restore natural floodplain forests (Palmer et al., 2007). Exotic Populus species have been introduced into Europe as well as into the United States and Canada for wood production in riparian landscapes and pose a threat to native Populus species. Poplar cultivars are frequently planted in monoclonal plantations in the vicinity of wild relatives and may contribute to a large extent to pollen and seed pools, thereby competing with the native relatives in colonizing restored habitat. Furthermore, intercrossability among Populus species is well-known (Eckenwalder, 1984, 1996). In Europe, the native European black poplar (Populus nigra L.; hereafter referred to as black poplar) is threatened by Euramerican (P. × canadensis Moench.), interamerican (P. × generosa Henry) hybrid poplar and black poplar varieties such as the male Lombardy poplar (Populus nigra cv. Italica Du Roi; Cagelli and Lefèvre, 1995; Arens et al., 1998; Fossati et al., 2005; Ziegenhagen et al., 2008; Smulders et al., 2008a; Chenault et al., 2011; Debeljak et al., 2015). Gene flow between cultivated and native poplar species may result in the reduction of the effective population size, genetic swamping, and ultimately the replacement of populations of the native species (Arnold et al., 2001).
As a pioneer species, black poplar plays a key-role in the development of softwood forests in Europe. In the lower parts of the floodplain, black poplar colonizes bare moist soil and river banks and is highly adapted to water dynamics and sediment movement (Van Looy, 2006; Debeljak et al., 2015). Due to their rapid growth and strong flow resistance, black poplar together with species of Salicaceae (Salix alba and S. viminalis), contributes to raising bar and island levels by retaining sand and gravel (Van Looy, 2006). Consequently, these softwood forest species pave the way for the development of the next forest succession stage, the hardwood forests. Unfortunately, the European black poplar is one of the most threatened tree species in Europe, mainly because of the loss of its natural alluvial habitats especially sand and gravel banks that allow for successful reproduction, and because of competition and introgression with exotic poplar species (Fossati et al., 2004; Vanden Broeck et al., 2005; Ziegenhagen et al., 2008; Meyer et al., 2018).
Here, we report on the impact of exotic Populus taxa at the initial stages of softwood riparian forest development of the river Meuse on the Dutch-Belgian border almost two decades after introducing black poplar and after the re-establishment of the natural river dynamics. To our knowledge, this is the first study reporting on the effect of re-introducing a native Populus species on gene flow of exotic relative species. The specific objectives of this study are (i) to study the taxonomy of the poplar seedlings that spontaneously colonized the river banks of the Common Meuse and (ii) to determine the frequency of natural hybridization events between male cultivated poplars (including P. nigra cv. Italica) and the female native black poplars in the study area. We hereto use a combination of diagnostic chloroplast and nuclear molecular markers that have been proven useful in identifying the taxonomy of Populus seedlings (Heinze, 1998; Imbert and Lefèvre, 2003; Ziegenhagen et al., 2008). We compare the results with a similar study performed in 1999–2001 at the same site and before the restoration actions took place (Vanden Broeck et al., 2004). Finally, we discuss the implications for the restoration and conservation of softwood riparian forests with black poplar along European rivers.
Materials and Methods
Study Site
The area of interest was the riverside of the Common Meuse, a free-flowing middle course of the river Meuse, which forms for 45 km the border between Belgium and Netherlands (Figure 1). Shipping and flow regulation are absent on this river stretch. The Common Meuse is a rain-fed river with a gravel-bed, a strong longitudinal gradient (0.45 m/km) and a wide alluvial plain (Van Looy et al., 2005). The Common Meuse valley consists of a gravel underground with a loamy alluvial cover. Traditionally, meadows were created in the floodplains. Cultivated poplars are frequently planted at the study site (Vanden Broeck et al., 2004). Large parts of the alluvial plain have been excavated for gravel mining, leaving large gravel pits or lowered floodplain zones (Van Looy et al., 2005). The extreme high water levels and floodings of 1993 and 1995, were the start of the development of a large scale, transboundary river restoration project for the Common Meuse; called “Living River Strategy.” The concept of this plan was to restore hydrodynamics and morphodynamics and related ecological characteristics in the primary river channel (Van Looy, 2006). The restoration activities were performed during the period 2005–2008 and included removal of dykes, excavation of the floodplain, channel widening, and bank lowering. The restoration of the morphological activity (erosion/sedimentation rates) of the river reach resulted in a strong revitalization of the elevation of bars and islands, creating suitable habitat for seedling recruitment and the restoration of softwood riparian forest with willow, poplar and ash communities (Van Braeckel, 2007; Van Looy et al., 2008).
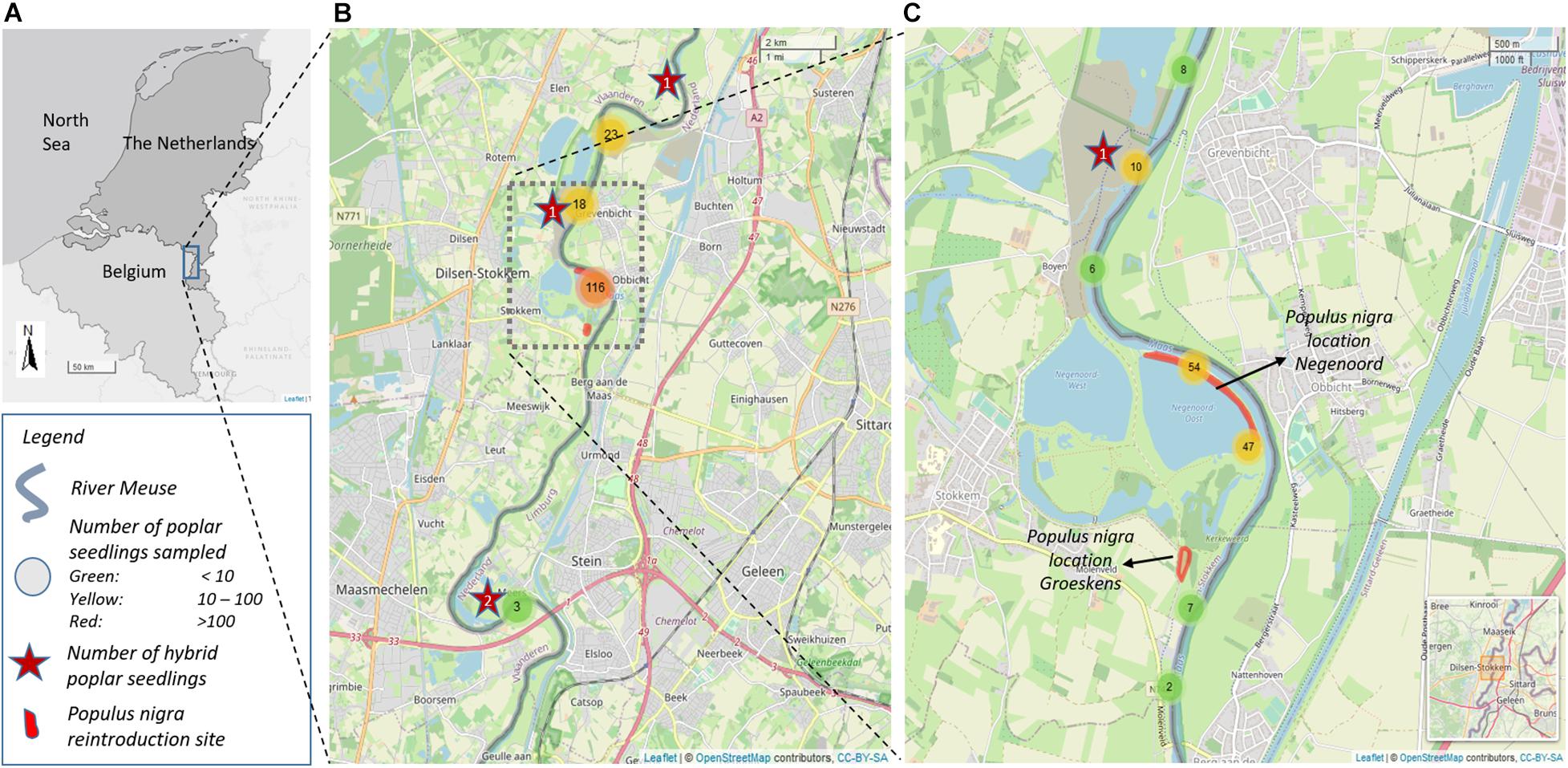
Figure 1. (A) Location of the river Common Meuse. (B) Map of the study site and sampling locations. Nearby sampled poplar seedlings are clustered and the total number of clustered samples is given in the circles. Stars indicate the position of hybrid poplar seedlings sampled. (C) Location of the introduced Populus nigra.
Reinforcement of the Black Poplar Population
A reintroduction project of P. nigra was performed in the period 2002–2005. The aim was to create seed sources and thereby to promote the colonization of native P. nigra in newly restored habitat. This was the follow-up of a study performed in 1999–2000 on the restoration potential and constraints of the development on softwood riparian forests along the Common Meuse (Vanden Broeck et al., 2002, 2004). This former study emphasized the need for re-planting native P. nigra since natural populations were almost gone extinct in the study area and >150 km further upstream in Northeast France (Vanden Broeck, 2004). A few isolated relict trees of P. nigra in the study area suggested the presence of natural populations in the past.
In contrast to the native poplar species, plantations of exotic poplars of P. × canadensis, P. × generosa, P. trichocarpa, and P. nigra cv. Italica were common within the study area (Vanden Broeck et al., 2004). Since 1970, monocultures of the female P. trichocarpa cultivar Fritzi Pauley and of the male cultivars Columbia River and Trichobel, were established in Belgium and Netherlands (van Broekhuizen, 1970; Steenackers and Van Slycken, 1982). Also, the P. × generosa cultivars; Unal, Beaupré, Boelare, Raspalje, Hunnegem, and the P. × canadensis cultivars; Primo, Gibecq, Ghoy, Gaver, Ogy, and Isières, were frequently planted. These cultivars, also known as the Unal-clones, were tested and selected at the Poplar Research Station in Geraardsbergen (Belgium) and widely made available to poplar planters in Belgium and Netherlands since 1970 (Steenackers and Van Slycken, 1982).
Populus nigra was successfully planted on two locations; location Groeskens (Dilsen-Stokkem, 51°01′01N, 05°46′02E) and location Negenoord (Dilsen-Stokkem, 51°01′36 N, 05°46′16E) on 0.77 ha and 2 ha, respectively, (Figure 1). In total, 275 2-year old bare-root P. nigra trees were planted, with plant distances of 9 m × 9 m and 4 m × 8 m, in Groeskens and Negenoord, respectively. The distance from the center of the plantings to the river bank was about 140 m for Groeskens and 5 m for Negenoord. The selection of the plant locations was based on the close connection to the river and on locations indicated close to potential sites for riparian forest development by a habitat model developed for the Common Meuse by Van Looy et al. (2005). The plant material originated form the national P. nigra gene bank collections at the Research Institute for Nature and Forest (INBO, Belgium; 57 genets) and at Alterra (Netherlands; 21 genets) supplemented with 23 genets from a natural population located along the river Rhine in Germany (location Kühkopf, FA Groß-Gerau, 48° 484 56,62 N, 8° 7′ 38,60 O) and about 90 seedlings of controlled crosses performed at INBO with genotypes of P. nigra from the gene bank collection. All the plant material, was identified as pure P. nigra by molecular markers (Storme et al., 2004; Smulders et al., 2008b). The sex was known for the plant material originating from the gene bank collections (60% of the trees planted) of which 28% were males. The number of plants per genet ranged from 1 to 21 with an overall mean of 2 plants per genet. Trees started to produce pollen and seeds, ten to 15 years after planting.
Plant Material Sampled
Poplar Seedlings From the Gravel Banks
Poplar seedlings that naturally colonized the gravel banks of the natural floodplains of the river Common Meuse were sampled on the banks along a 28 km-river section. We collected leaf samples of 52 and 102 poplar seedlings in late summer of 2018 and 2019, respectively. The geographical location of each sampled seedling was recorded. In 2018, poplar seedlings were sampled randomly within the 28 km-section. In 2019, a more systematic survey was performed within part of the same route, of about 9 km along the riverside; each gravel bank was inspected by walking along the river. In case rejuvenation was present on a site, one to twenty-three seedlings were sampled across the rejuvenation site (mean: 1.6), depending on the area of the colonized location. Only individuals that most likely originated from seed germination and not from vegetative reproduction by root suckers were sampled. One young leaf was collected from each seedling and dried in silica gel for DNA-analyses. The estimated height of the majority of the sampled individuals was between 10 and 50 cm, corresponding with the seedling or establishing stage (2–3 years) of riparian forest development (Van Looy et al., 2005). The location along the river of the seedlings sampled is given in Figure 1. The poplar seedlings were visually identified by their leaf morphology.
Open Pollinated Progenies of P. nigra
Populus species are predominantly dioecious and thus obligatory outcrossers. Seeds of adult open pollinated (OP) European black poplar females located in the study area were collected in two successive years. In June 2018, seeds were collected at the location Groeskens from the lower branches of the crown of six European black poplars. In June 2019, seeds were collected on in total nine European black poplar females; six trees from location Groeskens and three trees from location Negenoord. Seeds were collected from mature catkins, sown in trays in the greenhouse (50% white peat/50% black peat) within 1 week after collection. For the seeds collected in 2019, germination percentage was determined. One young leaf was collected from each seedling and dried in silica gel for DNA-analyses. The number of seeds collected per open-pollinated P. nigra female is given in Table 1.
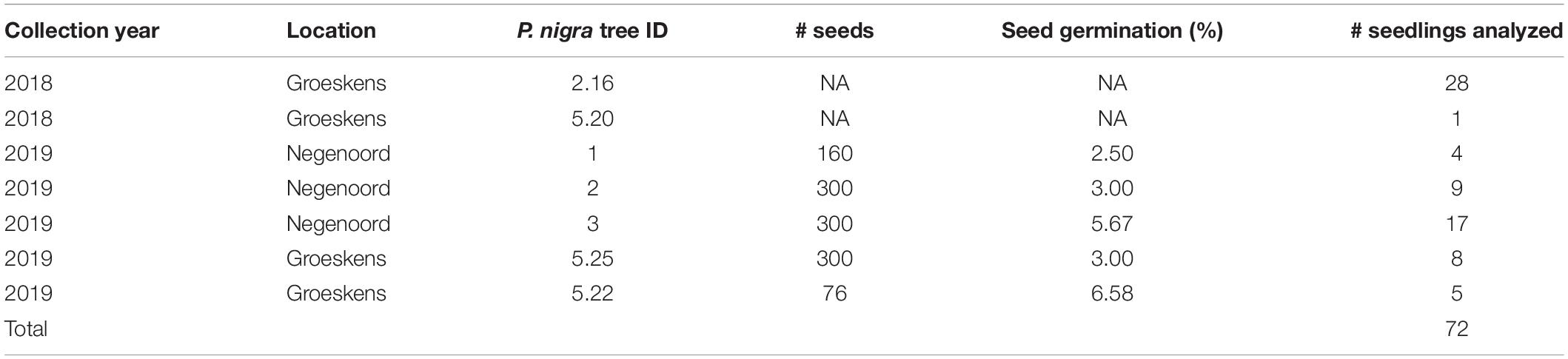
Table 1. The number of seeds collected, seed germination percentage and the number of seedlings analyzed per open pollinated Populus nigra female.
Reference Material
In Europe, poplar species that are frequently used in breeding programs in order to produce hybrids are the European P. nigra, the North American cottonwoods P. deltoides W. Bartram ex Marshall. and P. trichocarpa Torr. & A. Gray., and to a lower extent P. maximowiczii Henry native to northeast Asia. The unit of cultivation and breeding in poplars is a clone, and individual cultivars are normally represented by a single clone. We included 75 reference samples with known taxonomic identity of frequently used pure poplar species and hybrids as positive controls for verifying the species-specificity of the molecular markers. Furthermore, reference samples of P. nigra and of frequently planted commercial male poplar cultivars were included as potential fathers in the paternity-analyses. Reference material was obtained from the clone collection of INBO located in Geraardsbergen, Belgium. The following taxa were included: section Aigeiros with P. deltoides (4 samples), P. nigra (18 samples), P. × canadensis (P. deltoides × P. nigra; 37 samples), section Tacamahaca with P. trichocarpa (3 samples), P. trichocarpa × P. maximowiczii (3 samples) and the intersectional hybrid; P. × generosa (P. trichocarpa × P. deltoides; 10 samples). The list of reference material is given in Supplementary Table 1.
Identification of Hybrids
DNA Extraction
Total genomic DNA was extracted from the sampled leaves with the QuickPick Plant DNA kit (Bio-Nobile) using the MagRo 96-M robotic workstation (Bio-Nobile). For a subset of the samples (10%), the integrity of the DNA was assessed on 1% agarose gels. DNA quantification was performed with the Quant-iT PicoGreen dsDNA Assay Kit (Thermo Fisher Scientific, MA, United States) using a Synergy HT plate reader (BioTek, Vermont, United States).
Chloroplast DNA Marker
The chloroplast DNA (cpDNA) locus trnDT shows interspecific variation between P. nigra, P. deltoides, and P. trichocarpa (Heinze, 1998; Meyer et al., 2018). Because of its maternal inheritance and the female contribution of P. deltoides to the poplar clones frequently planted in Europe, this marker offers a tool to identify the maternal contribution of P. deltoides/P. × canadensis, P. nigra, and P. trichocarpa in the poplar seedlings collected from the gravel banks (Heinze, 1997; Ziegenhagen et al., 2008; Csencsics and Holderegger, 2016). We used the conserved PCR primer pair trnD/trnT (Demesure et al., 1995) in combination with the restriction enzyme HinfI. Genomic DNA (∼20–50 ng) was used as template in the PCR reactions. The PCR reaction conditions and restriction reactions were as described by Heinze (1998). Reaction products and a 100 bp ladder were analyzed by agarose gel electrophoresis.
Microsatellite Analysis
We selected nine microsatellite loci that were found useful in former studies for the analysis of natural hybridization events between cultivated poplars and their wild relative P. nigra (van der Schoot et al., 2000; Smulders et al., 2001; Liesebach et al., 2010), and that were found reliable for clonal fingerprinting in several species and hybrids of the sections Aigeiros and Tacamahaca (Dayanandan et al., 1998; Rahman and Rajora, 2002; Liesebach et al., 2010). All markers are unlinked (Cervera et al., 2001; Gaudet et al., 2008). According to former studies, five loci produce species-diagnostic alleles for P. deltoides and/or P. trichocarpa: PMGC14 (Fossati et al., 2003; Liesebach et al., 2010), PMGC456 (Liesebach et al., 2010), PMGC2163 (Liesebach et al., 2010), WPMS09 (Fossati et al., 2003; Liesebach et al., 2010), and WPMS20 (Liesebach et al., 2010). These diagnostic loci are useful to identify offspring of the frequently planted cultivars of P. × canadensis, P. × generosa, and P. trichocarpa. SSR analysis was performed on the OP progenies, the seedlings from the gravel banks and the reference samples as described by Smulders et al. (2001) and van der Schoot et al. (2000). Ten samples were replicated twice starting from a second DNA-extract to calculate the genotyping error rate. PCR products were run on an ABI3500 Genetic Analyzer (Applied Biosystems) and genotypes were analyzed with the GeneMapper v.6.0 software package. Samples with fewer than 8 scored loci were discarded from the data analysis. Details on the microsatellite loci are given in Table 2.
Data Analysis
The maternal origin of the poplar seedlings sampled on the gravel banks was investigated using the cpDNA locus trnDT. The poplar seedlings from the gravel banks and the OP progenies were analyzed for the presence of diagnostic alleles of P. deltoides and P. trichocarpa on the five diagnostic SSR loci. A diagnostic allele for P. deltoides in P. nigra maternal offspring indicates a backcrossed individual with P. × canadensis paternity as a result of a natural hybridization event between a cultivar of P. × canadensis and a native P. nigra.
We also performed a paternity analysis on the OP progenies using the microsatellite data to determine pollination events of frequently planted male poplar cultivars included in the reference samples. We used a likelihood-based approach implemented in the program CERVUS 3.0.7 (Marshall et al., 1998) with the reference samples described above (48; females excluded) as potential fathers, given a known mother. The paternity assignment technique used by CERVUS requires the knowledge of the total number of candidate males in the population and the proportion of the candidate males sampled for the simulation-based approach to assess the confidence of the assignments. For the simulation, we used the LOD distribution and we assumed 50 candidate fathers and a proportion of 0.3 candidate fathers sampled, allowing an error rate of 0.01 and allowing a mismatch between parent and offspring on one locus taking into account somatic mutations and null alleles.
Results
Diagnostic Microsatellite Alleles
The percentage missing data for the total microsatellite data was 0.07%. Replicated samples resulted in identical multilocus genotypes. For the seedlings sampled from the gravel banks, two multilocus genotypes were shared by two and three sampled seedlings, respectively. It is possible that some individuals were sampled twice, in 2018 and 2019. Only one genotype among these replicates was kept in the final dataset. The microsatellite data confirm the presence of species-diagnostic alleles reported in former studies by Liesebach et al. (2010) and Fossati et al. (2003) for PMGC14, PMGC456, PMGC2163, and WPMS09 but not for WPMS20. For PMGC14, the alleles 192 and 198 were typical for all reference clones of P. deltoides and for P. × canadensis, and the allele 200 was typical for all reference clones of P. trichocarpa. For WPMS09, allele 232 was present in the reference clones of P. deltoides and of P. × canadensis, except for 16 P. × canadensis reference clones that were homozygous for this locus. This can be explained by their genetic origin (i.e., F2 or back-cross clones) or by the presence of a null allele. For WPMS20, the characteristic allele for P. deltoides (allele 200) also frequently occurred in P. nigra, which was also reported by Liesebach et al. (2010). Furthermore, species-specific alleles were present at locus PMGC2163 (P. deltoides: allele 185, P. trichocarpa: alleles 199, 200) and locus PMGC456 (P. deltoides: allele 82, P. trichocarpa: alleles 93, 109, P. nigra: allele 75).
Poplar Seedlings From the Gravel Banks
From the 154 seedlings sampled on the gravel banks, 135 showed a clear banding pattern for the cpDNA locus trnDT. From the latter, 133 (98.5%) poplar seedlings showed a P. nigra-specific trnDT marker-fragment (∼880 bp). These seedlings also showed a P. nigra-like leaf morphology. The typical gene variant of P. nigra observed on the maternal inherited diagnostic cpDNA locus trnDT indicated that they originated from a female P. nigra. Furthermore, two (1.5%) seedlings showed a P. trichocarpa-specific cpDNA fragment (∼1,000 bp). These two samples with a P. trichocarpa-like leaf morphology were collected on an upstream distance of 6 km from the nearest P. nigra. No seedlings were detected with a fragment length specific of P. deltoides (∼1,135 bp). This suggests the absence of progeny originating from female cultivars of P. × canadensis within the samples collected on the gravel banks. The results of the diagnostic microsatellite alleles confirmed the presence of species-specific P. trichocarpa alleles at loci PMGC147, PMGC2163, and PMGC456 for the two seedlings with a P. trichocarpa-specific trnDT marker-fragment. For one of these two seedlings, species-specific alleles of P. trichocarpa were homozygous, suggesting that this seedling could have originated from two P. trichocarpa or P. × generosa cultivars. Furthermore, two more seedlings sampled in the field showed species-specific alleles of P. deltoides in heterozygous state at three and four diagnostic loci, respectively. Given their P. nigra-specific trnDT marker-fragment, they likely originated from a P. nigra × P. × canadensis back-cross. Summarized, four (2.6%) out of the 154 young poplars analyzed from the gravel banks exhibited genes of exotic, cultivated poplar species.
Identification of Hybrids in Open Pollinated Progenies
Viable seedlings were obtained from six and five black poplar trees in 2018 and 2019, respectively, (Table 1). The mother trees presented only two different genets. The mean germination percentage for the 1492 seeds sown in 2019 was low (2.9%; range of 0 to 6.6%). In total, we obtained 74 viable seedlings of which 72 were successfully genotyped. We detected no alleles species-specific to P. deltoides or P. trichocarpa in the 72 analyzed seedlings from the OP P. nigra progenies, suggesting the absence of backcrossed or trihybrid individuals with P. × canadensis or P. × generosa as potential fathers.
However, the paternity analysis revealed P. x canadensis a highly likely father for three seedlings from the OP progeny from seed harvested in 2018 on one mother tree (ID 2.16) at location Groeskens. Paternity was determined with 95% confidence for 27 (38%) seedlings. When using a relaxed confidence level of 80%, paternity was assigned to 48 (67%) seedlings. For 24 (33%) seedlings, the paternity remained unresolved. For one seedling paternity was assigned to P. × canadensis cv. Serotina de Champagne using a relaxed confidence level and with no paternity-offspring mismatches. Also for a second seedling from the same mother tree, P. × canadensis cv. Serotina de Champagne was the most likely father with a mismatch for only one locus (PMGC14), possibly due to a somatic mutation. A third seeding from the same OP progeny showed P. × canadensis cv. Serotina as the most likely father, although mismatch was observed at two loci (PMGC14, PTR07). We repeated the SSR analysis for the latter two seedlings which confirmed the observed mismatches. The other determined paternities were assigned to trees of P. nigra. No offspring was assigned to the male cultivar P. nigra cv. Italica, nor to the nearby planted male P. × canadensis cv. Robusta or to the reference cultivars of P. × generosa.
Discussion
The results of this study illustrate the importance of in situ reinforcement of black poplar to reduce the potential impact of exotic poplar cultivars in European floodplains in case natural river dynamic processes are restored and adult black poplar trees are scarce. The numbers of young poplar seedlings that colonized the gravel banks of the river Meuse exceeded 100 fold the initial number of seedlings observed by Vanden Broeck et al. (2004). Additionally the number of seeds and seedlings with exotic poplar genes were diminished to a very low proportion compared to the situation before the restoration.
Limited Interspecific Matings
We assessed the taxonomy of poplar seedlings that spontaneously colonized the gravel banks of the river Meuse based on diagnostic chloroplast and nuclear microsatellite markers. Furthermore, by using nuclear microsatellite markers and a paternity analysis on seedlings resulting from open pollinated P. nigra females, we investigated natural hybridization between frequently planted male cultivated poplars and female native P. nigra.
The majority (150, 97.4%) of the poplar seedlings from the gravel banks analyzed where identified as “pure” P. nigra seedlings; the typical chloroplast gene variant of P. nigra observed in the maternal inherited diagnostic cpDNA locus trnDT indicated that they originated from a female P. nigra. They could not originate from cultivated first-generation (F1) P. × canadensis hybrids, as cultivated clones of P. × canadensis are the result from artificial crosses between a female P. deltoides and a male P. nigra, thus carrying the P. deltoides haplotype (Zsuffa, 1974; Heinze, 1998). Furthermore, the absence of microsatellite alleles of P. deltoides or P. trichocarpa suggests that they were fathered by P. nigra. Only a small number (four; 2.6%) of young poplars analyzed from the gravel banks exhibited nuclear microsatellite genes of exotic, cultivated poplars. Of the latter, two seedlings showed P. trichocarpa genes while the other two resulted from a first-generation back-cross (BC1) of a male P. × canadensis and a female P. nigra.
No gene variants of exotic poplar species were detected in the OP progenies. This was largely confirmed by the paternity analyses, except for three seedlings (4.1%) of which two were likely fathered by P. × canadensis cv. Serotina de Champagne and one seedling by P. × canadensis cv. Serotina. It must be noted that these two cultivars are clearly different and not related, although their names suggest otherwise. They show different alleles at seven out of the nine microsatellite loci genotyped and also show differences in leaf morphology as was noted by Broekhuizen (1960). No paternity was assigned to the male cultivar P. nigra cv. Italica (Lombardy poplar), one of the most planted poplar cultivars. In Belgium, the Lombardy poplar generally flowers before the majority of the native black poplars (Vanden Broeck et al., 2003a), which may not be the case in more Southern regions (Chenault et al., 2011). Absence of flowering synchrony may explain why no paternity was assigned to this cultivar or to the nearby planted male P. × canadensis cv. Robusta in this study.
The probability to detect gene variants of P. deltoides using four diagnostic nuclear microsatellite loci and according to Mendelian rules in first-backcross generations (BC1) is 93.75%, but decreases to 68.36% for second back-cross generations (BC2; Bialozyt et al., 2012). This could explain why gene variants of P. deltoides remained undetected in three seedlings from the OP progenies to which a cultivar of P. × canadensis was assigned as the most likely father. In this study, the paternity analysis increased the reliability to identify further generation back-cross events in the OP progenies. However, the power of a paternity analysis depends on the potential male parents included in the analysis and the assumptions made on the proportion of potential fathers sampled. It is possible that some second back-cross events remained undetected because of these sampling limitations. Furthermore, the number of seedlings analyzed from the open pollinated P. nigra progenies was small due to low seed germination percentages which limited the statistical power to estimate the frequency of rare hybridization events. There are several possible explanations for the low seed germination percentages observed including; the relative young age of the black poplar stand resulting in low pollen and seed production compared to older stands, the sub-optimal weather conditions during fertilization and/or seed ripening, and post-zygotic incompatibility barriers reducing the number of ovules in the embryo sac that can develop to viable embryos as a result from exotic pollen fertilization.
Pollen and Seed Pools Affecting Exotic Gene Flow
The contrast in the number of interspecific mating events before and after the river and vegetation restoration activities is remarkable. In 1999–2001, the majority of the seedlings sampled on the gravel banks (27/29; 93%) showed a P. trichocarpa fragment at the cpDNA locus trnDT and/or species-specific alleles of P. deltoides (Vanden Broeck et al., 2004). In contrast to this study, gene variants of exotic poplar species were also detected in the majority of the seedlings (32/34; 94.1%) of the OP progenies of an isolated female P. nigra in the study area in 1999–2001 (Vanden Broeck et al., 2004). At that time, P. nigra was extremely rare in the study area as a result of habitat reduction and fragmentation; the native species was only represented by a few old trees, in contrast with the widely planted poplar cultivars (Vanden Broeck et al., 2004). The strong decrease in population size combined with the habitat fragmentation of the native P. nigra increased the opportunities for contact with cultivated poplar clones and provided at that time excellent opportunities for the production of hybrid seeds and seedlings. Poplars are prolific seed producers; one tree can produce over 50 million of seeds in a single season (Oecd, 2000). Nevertheless, poplar hybrids are generally characterized by reduced fertility relative to parental species, with significant lower pollen production and seed viability in F1-hybrids (Stettler et al., 1996). It is therefore likely that the seed production and seed viability of P. nigra outperformed those of the cultivated poplars. Also, pollen load composition and size could have been an important process in limiting the production of hybrid progeny. The low frequencies of interspecific mating relative to intraspecific mating using a pollen mixture of different taxa in controlled crosses suggest a greater competitive ability for conspecific pollen (Gaget et al., 1989; Rajora, 1989; Benetka et al., 2002; Vanden Broeck et al., 2003b). As a result, the relative fertilization success of a pollen tree depends upon the taxon constitution of the pollen mix and thus on the number and taxon of the producing pollen trees in the study area.
In this study, the few seedlings showing exotic poplar genes were located at distances of >1 km from the nearest reintroduction sites of black poplar. This indicates that hybrid poplars still reproduce in the study area, in particular on sites located further away from the reforestation sites of black poplar. Establishing more seed sources of black poplar over the river stretch could further reduce hybrid reproductive success along the Common Meuse. Although conspecific siring of female P. × canadensis is enhanced by the presence of pollen of black poplar (Vanden-Broeck et al., 2012), the higher local density of male and female black poplars appears to guarantee the establishment of mainly pure black poplar seedlings nearby the parental stands. Considering the possibility of long distance gene flow (Imbert and Lefèvre, 2003), investigations of interspecific mating events would ideally cover the whole river stretch where natural habitat is restored.
Conservation Implications
The results of this study illustrate the importance of in situ reinforcement of black poplar populations to reduce the potential impact of exotic poplar cultivars along European floodplains where natural river dynamic processes are restored and adult black poplar trees are scarce. The potential risks for gene introgression from exotic poplars into black poplar is indeed highly variable between geographic locations and river sites, largely depending on habitat fragmentation, flowering synchrony and the composition of the pollen and seed pool at the specific site. While interspecific gene flow seems to be absent in large natural populations of black poplar, like in France along the rivers Loire, Garonne and Drôme (Imbert and Lefèvre, 2003) significant gene flow from exotic poplars in riparian floodplains is reported along the rivers Rhine and the Elbe in Germany (Ziegenhagen et al., 2008; Meyer et al., 2018), the Morava River in the Czech Republic (Pospiskova and Salkova, 2006), the Ticino river in Italy (Fossati et al., 2003), the Rhine river in Netherlands (Arens et al., 1998; Smulders et al., 2008a) and the rivers Thur and Reuss in Switzerland (Csencsics and Holderegger, 2016). This case study along the Common Meuse, demonstrates that reintroduction and reinforcement of native black poplar populations can reverse the situation from high to low risk of introgression with cultivated poplars on a relatively short time scale. By changing the composition of the seed and pollen pool, black poplar nowadays has a competitive advantage compared to the exotic poplars in colonizing the restored river banks along the Common Meuse.
Hundreds of young black poplar seedlings colonized the gravel banks. With an estimated age for the majority of the seedlings of 2 to 3 years old, they survived the germination stage and currently present the establishment stage of softwood forest development with the development of dense thickets (Van Looy, 2006). The survival of poplar seedlings depends on the combination of many factors broadly related to the availability of water and oxygen (Hughes et al., 2001). As a result of the critical conditions required by seedlings, successful establishment only occurs in some years, and within well-defined elevational bands (Hughes et al., 2001). Furthermore, established seedlings at lower river levels are frequently subject to later removal or damage by drought, flooding, sediment burial, or mechanical disturbance (Hughes et al., 2001). Seedling survival should therefore be monitored over larger time scales to evaluate the success of this restoration project and to predict riparian forest development along the river Common Meuse. Management and conservation plans should include further monitoring of the seedling colonization and survival, the effective population size and the introgression of foreign genes in black poplar across multiple generations. Also, the vegetative spread of cultivated hybrids, which was not the focus of this study, should be taken into consideration in predicting the development of riparian forest ecosystems. The data can then be used for the construction of habitat models to identify occupied or potential habitat and to predict riparian forest development in the restored floodplain area (Van Looy et al., 2005; Debeljak et al., 2015).
Conclusion
Almost two decades after the reintroduction of black poplar along the river Common Meuse, the constitution of the seed and pollen pool changed in the study area thereby benefiting the reproduction of the native species at the expense of the reproduction capacity of the exotic poplar species. Increasing the density of male and female black poplars appears to guarantee the establishment of mainly pure black poplar seedlings nearby the parental stands. Nevertheless, exotic poplar species still reproduce at larger distances from the revegetation sites and colonize restored habitat thereby competing for resources with the native species. Therefore, increasing the number of native black poplar seed sources along the river stretch will help the native black poplar to maintain its competitive advantage over the exotic poplars and will contribute considerably to the success of riparian forest development along the Common Meuse.
Data Availability Statement
The data supporting the conclusions of this article are available at DRYAD; doi: 10.5061/dryad.n2z34tmvn.
Author Contributions
AV and KV: conceptualization. AV: methodology, formal analysis, and writing – original draft. KC, AV, and KV: writing – review and editing. SN and ND: methodology and resources. All authors have read and approved the final version.
Funding
De Vlaamse Waterweg nv provided financial support for the conduct of the research. The funding source was not involved in study design, also not in the collection, analysis and interpretation of data, or in the decision to submit the article for publication.
Conflict of Interest
The authors declare that the research was conducted in the absence of any commercial or financial relationships that could be construed as a potential conflict of interest.
Acknowledgments
The authors gratefully acknowledge the river managers Herman Gielen and Joke Verstraelen for their enthusiasm and support. The authors also thank Wim De Clercq and Jemp Peeters for the field assistance, and Marc Schouppe for the technical assistance in the greenhouse. A preprint of this study was posted on bioRxiv: https://biorxiv.org/cgi/content/short/2020.07.02.183699v1.
Supplementary Material
The Supplementary Material for this article can be found online at: https://www.frontiersin.org/articles/10.3389/fpls.2020.580653/full#supplementary-material
Supplementary Table 1 | List of Populus species and poplar cultivars with known taxonomic identity used as reference samples. References to the cultivars were obtained from the International Register of Poplar Cultivars (IPC – FAO).
References
Arens, P., Coops, H., Jansen, J., and Vosman, B. (1998). Molecular genetic analysis of black poplar (Populus nigra L.) along dutch rivers. Mol. Ecol. 7, 11–18. doi: 10.1046/j.1365-294x.1998.00316.x
Arnold, M. L., Kentner, E. K., Johnston, J. A., Cornman, S., and Bouck, A. C. (2001). Natural hybridisation and fitness. Taxon 50, 93–104. doi: 10.2307/1224513
Benetka, V., Vacková, K., Bartáková, I., Pospíšková, M., and Rasl, M. (2002). Introgression in black poplar (Populus nigra L. ssp. P. nigra) and its transmission. J. Forest Sci. 48, 115–120. doi: 10.17221/11863-jfs
Bialozyt, R., Rathmacher, G., Niggemann, M., and Ziegenhagen, B. (2012). Reconstructing explicit mating schemes in poplar hybrids - a case study in the Populus nigra L. - Populus x canadensis moench complex. Silvae Genet. 61, 157–167. doi: 10.1515/sg-2012-0020
Broekhuizen, J. T. M. (1960). Herkomst en nomenclatuur van enige populierencultivars. Nederlands Bosbouw Tijdschrift 32, 153–159.
Cagelli, L., and Lefèvre, F. (1995). The conservation of Populus nigra L. and gene flow with cultivated poplars in europe. Forest Genet. 2, 135–144.
Cervera, M.-T., Storme, V., Ivens, B., Gusmao, J., Liu Ben, H., Hostyn, V., et al. (2001). Dense geneticlinkage maps of three Populus Species (Populus deltoides, P. nigra and P. trichocarpa) based on AFLP and microsatellite markers. Genetics 158, 787–809.
Chenault, N., Arnaud-Haond, S., Juteau, M., Valade, R., Almeida, J.-L., Villar, M., et al. (2011). SSR-based analysis of clonality, spatial genetic structure and introgression from the Lombardy poplar into a natural population of Populus nigra L. along the Loire River. Tree Genet. Genom. 7, 1249–1262. doi: 10.1007/s11295-011-0410-6
Csencsics, D., and Holderegger, R. (2016). Origin of natural regeneration of black poplar (Populus nigra L.) and co-occurrence with hybrid poplar offspring. Flora 222, 20–26. doi: 10.1016/j.flora.2016.03.009
Dayanandan, S., Rajora, O. P., and Bawa, K. S. (1998). Isolation and characterization of microsatellites in trembling aspen (Populus tremuloides). Theor. Appl. Genet. 96, 950–956. doi: 10.1007/s001220050825
Debeljak, M., Ficko, A., and Brus, R. (2015). The use of habitat and dispersal models in protecting european black poplar (Populus nigra L.) from genetic introgression in slovenia. Biol. Conser. 184, 310–319. doi: 10.1016/j.biocon.2015.02.004
Demesure, B., Sodzi, N., and Petit, R. J. (1995). A Set of universal primers for amplification of polymorphic noncoding regions of mitochondrial and chloroplast dna in plants. Mol. Ecol. 4, 129–131. doi: 10.1111/j.1365-294x.1995.tb00201.x
Eckenwalder, J. E. (1984). Natural intersectional hybridization between north american species of populus (salicaceae) in sections aigeiros and tacamahaca. III. paleobotany and evolution. Can. J. Bot. 62, 336–342. doi: 10.1139/b84-052
Eckenwalder, J. E. (1996). ““Systematics and evolution of Populus”,” in Biology of Populus, eds R. F. Stettler, H. D. Bradshaw, and P. E. Heilman Jr., and T. M. Hinckley (Ottawa, Canada: National Research Council), 7–32.
Fossati, T., Grassi, F., Sala, F., and Castiglione, S. (2003). Molecular analysis of natural populations of Populus nigra L. intermingled with cultivated hybrids. Mol. Ecol. 12, 2033–2043. doi: 10.1046/j.1365-294x.2003.01885.x
Fossati, T., Patrignani, G., Zapelli, I., Sabatti, M., Sala, F., Castiglione, S., et al. (2004). Development of molecular markers to assess the level of introgression of Populus tremula into P. alba natural populations. Plant Breed. 123, 382–385. doi: 10.1111/j.1439-0523.2004.00979.x
Fossati, T., Zapelli, I., Bisoffi, S., Micheletti, A., Vietto, L., Sala, F., et al. (2005). Genetic relationships and clonal identity in a collection of commercially relevant poplar cultivars assessed by AFLP and SSR. Tree Genet. Genom. 1, 11–19. doi: 10.1007/s11295-004-0002-9
Gaget, M., Villar, M., and Dumas, C. (1989). The mentor pollen phenomenon in poplars: a new concept. Theor. Appl. Genet. 78, 129–135. doi: 10.1007/bf00299765
Gaudet, M., Jorge, V., Paolucci, I., Beritognolo, I., Mugnozza, G. S., Sabatti, M. et al. (2008). Genetic linkage maps of Populus nigra L. including AFLPs, SSRs, SNPs, and sex trait. Tree Genet. Genom. 4, 25–36. doi: 10.1007/s11295-007-0085-1
Heinze, B. (1997). A PCR Marker for a populus deltoides allele and its use in studying introgression with native european populus nigra belgian. J. Bot. 129, 123–130.
Heinze, B. (1998). PCR-Based chloroplast DNA assays for the identification of native Populus nigra and introduced poplar hybrids in Europe. Forest Genet. 5, 31–38.
Hughes, F. M. R., Adams, W. M., Muller, E., Nilsson, C., Richards, K. S., Barsoum, N., et al. (2001). The importance of different scale processes for the restoration of floodplain woodlands. Reulated Rivers Res. Manage. 17, 325–345. doi: 10.1002/rrr.656
Imbert, E., and Lefèvre, F. O. (2003). Dispersal and gene flow of Populus nigra (Salicaceae) along a dynamic river system. J. Ecol. 91, 447–456. doi: 10.1046/j.1365-2745.2003.00772.x
Liesebach, H., Schneck, V., and Ewald, E. (2010). Clonal fingerprinting in the genus populus L. by nuclear microsatellite loci regarding differences between sections, species and hybrids. Tree Genet. Genom. 6, 259–269. doi: 10.1007/s11295-009-0246-5
Mansourian, S., Doncheva, N., Valchev, K., and Vallauri, D. (2019). Lessons learnt from 20 years of floodplain forest restoration: the lower danube landscape. France: WWF.
Marshall, T. C., Slate, J., Kruuk, L. E. B., and Pemberton, J. M. (1998). Statistical confidence for likelihood-based paternity inference in natural populations. Mol. Ecol. 7, 639–655. doi: 10.1046/j.1365-294x.1998.00374.x
Meyer, M., Zacharias, M., Morgenstern, K., Krabel, D., and Liesebach, H. (2018). Variable genotypes at the cpDNA marker locus trnDT in spontaneous rejuvenation of the species complex around the European black poplar (Populus nigra L.) and its relatives collected in Germany. Landbauforschung 68, 93–102. doi: 10.3220/lbf1544605045000
Oecd. (2000). ”Consensus document on the biology of Populus L. (Poplars)”. Paris, France: Organisation for Economic Co-operation and Development.
Palmer, M., Allan, J. D., Meyer, J., and Bernhardt, E. S. (2007). River restoration in the twenty-first century: Data and experiential future efforts. Restor. Ecol. 15, 472–481. doi: 10.1111/j.1526-100X.2007.00243.x
Pospiskova, M., and Salkova, I. (2006). Population structure and parentage analysis of black poplar along the morava river. Can. J. Forest Res. Revue. 36, 1067–1076. doi: 10.1139/x06-003
Rahman, M. H., and Rajora, O. P. (2002). Microsatellite DNA fingerprinting, differentiation, and genetic relationships of clones, cultivars, and varieties of six poplar species from three sections of the genus Populus. Genome 45, 1083–1094. doi: 10.1139/g02-077
Rajora, O. P. (1989). Pollen competition among Populus deltoides Marsh., P. nigra L. and P. maximowiczii Henry in fertilizing P. deltoides ovules and siring its seed crop. Sex. Plant Reprod. 2, 90–96.
Schindler, S., O’Neill, F. H., Biró, M., Damm, C., Gasso, V., Kanka, R., et al. (2016). Multifunctional floodplain management and biodiversity effects: a knowledge synthesis for six European countries. Biodiv. Conserv. 25, 1349–1382. doi: 10.1007/s10531-016-1129-3
Smulders, M. J. M., Beringen, R., Volosyanchuk, R., Broeck, A. V., van der Schoot, J., Arens, P., et al. (2008a). Natural hybridisation between Populus nigra L. and P. x canadensis Moench. Hybrid offspring competes for niches along the rhine river in the netherlands. Tree Genet. Genom. 4, 663–675. doi: 10.1007/s11295-008-0141-5
Smulders, M. J. M., Cottrell, J. E., Lefevre, F., van der Schoot, J., Arens, P., Vosman, B., et al. (2008b). Structure of the genetic diversity in black poplar (Populus nigra L.) populations across European river systems: Consequences for conservation and restoration. Forest Ecol. Manage. 255, 1388–1399. doi: 10.1016/j.foreco.2007.10.063
Smulders, M. J. M., van der Schoot, J., Arens, P., and Vosman, B. (2001). Trinucleotide repeat microsatellite markers for black poplar (Populus nigra L.). Mol. Ecol. Notes 1, 188–190. doi: 10.1046/j.1471-8278.2001.00071.x
Steenackers, V., and Van Slycken, J. (1982). De Unal-populierenklonen: enkele eerste resultaten. Commun. Res. Inst. Nat. Forest 1:113.
Stettler, R. F., Zsuffa, L., and Wu, R. (1996). ““The role of hybridization in the genetic manipulation of Populus,”,” in Biology of Populus, eds H. D. Stettler, J. H. E. Bradshaw, P. E. Heilman, and T. M. Hinckley (Ottawa, Canada: NRC Research Press), 87–112.
Storme, V., Vanden Broeck, A., Ivens, B., Van Slycken, J., Castiglione, S., et al. (2004). Ex-situ conservation of Black poplar in Europe: genetic diversity in nine gene bank collections and their value for nature development. Theor. Appl. Genet. 108, 969–981. doi: 10.1007/s00122-003-1523-6
Van Braeckel, A. V. L. (2007). River restoration along the Border Meuse: evaluation of scenarios for measures and management with an integrated model. Water J. Integ. Water Manage. 30, 87–94.
van der Schoot, J., Posp!‘sková, M., and Vosman, B. (2000). Development and characterization of microsatellite markers in black poplar (Populus nigra L.). Oretical Appl. Genet. 101, 317–322. doi: 10.1007/s001220051485
Van Looy, K. (2006). River Restoration & Biodiversity Conservation. A Disorder Approach. Brussels: Research Institute for Nature and Forest.
Van Looy, K., Meire, P., and Wasson, J.-G. (2008). Including riparian vegetation in the definition of morphologic reference conditions for large rivers: a case study for Europe’s Western Plains. Environ. Manage. 41, 625–639. doi: 10.1007/s00267-008-9083-9
Van Looy, K., Severyns, J., Jochems, H., and Smedt, F. (2005). Predicting patterns of riparian forest restoration. Archiv für Hydrobiologie 155, 373–390. doi: 10.1127/lr/15/2003/373
Van Looy, K., Tormos, T., Ferreol, M., Villeneuve, B., Valette, L., Chandesris, A., et al. (2013). Benefits of riparian forest for the aquatic ecosystem assessed at a large geographic scale. Knowl. Manage. Aquat. Ecosyst. 408:14. doi: 10.1051/kmae/2013041
Vanden Broeck, A. (2004). Potential gene flow between native European black poplar (Populus nigra L.) and cultivated poplars in Belgium. Belgium: University Ghent.
Vanden Broeck, A., Cox, K., Quataert, P., Van Bockstaele, E., and Van Slycken, J. (2003a). Flowering phenology of Populus nigra L., P. nigra cv. Italica and P. x canadensis Moench. and the potential for natural hybridisation in Belgium. Silvae Genet. 52, 280–283.
Vanden Broeck, A., Cox, K., Van Braeckel, A., Neyrinck, S., De Regge, N., Van Looy, K., et al. (2020). Reintroduced native Populus nigra in restored floodplain reduces spread of exotic poplar species. bioRxiv 2020:183699. doi: 10.1101/2020.07.02.183699
Vanden Broeck, A., Cox, K., Van Braeckel, A., Neyrinck, S., De Regge, N., Van Looy, K., et al. (2020). Data from: Reintroduced native Populus nigra in restored floodplain reduces spread of exotic poplar species, Dryad, Dataset. Belgium: Research Institute for Forest and Nature.
Vanden Broeck, A., Jochems, H., Storme, V., and Van Looy, K. (2002). Vlaams impulsprogramma natuurontwikkeling : mogelijkheden tot herstel van levensvatbare populaties zwarte populier (populus nigra L.) langsheen de Grensmaas. Brussel: Instituut voor Bosbouw en Wildbeheer.
Vanden Broeck, A., Quataert, P., Roldan-Ruiz, I., Van Bockstaele, E., and Van Slycken, J. (2003b). Pollen competition in Populus nigra females revealed by microsatellite markers. Forest genetics 10, 219–227.
Vanden Broeck, A., Storme, V., Cottrell, J. E., Boerjan, W., Van Bockstaele, E., Quataert, P., et al. (2004). Gene flow between cultivated poplars and native black poplar (Populus nigra L.): a case study along the river meuse on the dutch-belgian border. Forest Ecol. Manage. 197, 307–310. doi: 10.1016/j.foreco.2004.05.021
Vanden Broeck, A., Villar, M., Van Bockstaele, E., and VanSlycken, J. (2005). Natural hybridization between cultivated poplars and their wild relatives: evidence and consequences for native poplar populations. Annal. Forest Sci. 62, 601–613. doi: 10.1051/forest:2005072
Vanden-Broeck, A., Cox, K., Michiels, B., Verschelde, P., and Villar, M. (2012). With a little help from my friends: hybrid fertility of exotic Populus x canadensis enhanced by related native Populus nigra. Biol. Invasions 14, 1683–1696. doi: 10.1007/s10530-012-0180-6
Ziegenhagen, B., Gneuss, S., Rathmacher, G., Leyer, I., Bialozyt, R., Heinze, B., et al. (2008). A fast and simple genetic survey reveals the spread of poplar hybrids at a natural Elbe river site. Conserv. Genet. 9, 373–379. doi: 10.1007/s10592-007-9349-4
Keywords: European black poplar, floodplain ecosystem, hybridization, interspecific mating, introgression, riparian forests
Citation: Vanden Broeck A, Cox K, Van Braeckel A, Neyrinck S, De Regge N and Van Looy K (2021) Reintroduced Native Populus nigra in Restored Floodplain Reduces Spread of Exotic Poplar Species. Front. Plant Sci. 11:580653. doi: 10.3389/fpls.2020.580653
Received: 06 July 2020; Accepted: 22 December 2020;
Published: 15 January 2021.
Edited by:
Ronald Bialozyt, Northwest German Forest Research Institute, GermanyReviewed by:
Dušan Gömöry, Technical University of Zvolen, SlovakiaMarinus J. M. Smulders, Wageningen University and Research, Netherlands
Copyright © 2021 Vanden Broeck, Cox, Van Braeckel, Neyrinck, De Regge and Van Looy. This is an open-access article distributed under the terms of the Creative Commons Attribution License (CC BY). The use, distribution or reproduction in other forums is permitted, provided the original author(s) and the copyright owner(s) are credited and that the original publication in this journal is cited, in accordance with accepted academic practice. No use, distribution or reproduction is permitted which does not comply with these terms.
*Correspondence: An Vanden Broeck, An.vandenbroeck@inbo.be