- 1Department of Plant Sciences, North Dakota State University, Fargo, ND, United States
- 2Department of Agroenvironmental Sciences, University of Puerto Rico, Mayagüez, Puerto Rico
- 3USDA-ARS, Tropical Agriculture Research Station, Mayagüez, Puerto Rico
Among grain legume crops, common beans (Phaseolus vulgaris L.) are considered to have poor biological nitrogen (N2) fixation (BNF) capabilities although variation in N2 fixing capabilities exists within the species. The availability of genetic panel varying in BNF capacity and a large-scale single nucleotide polymorphism (SNP) data set for common bean provided an opportunity to discover genetic factors associated with N2 fixation among genotypes in the Middle American gene pool. Using nodulation and percentage of N2-derived from atmosphere (%NDFA) data collected from field trials, at least 11 genotypes with higher levels of BNF capacity were identified. Genome-wide association studies (GWASs) detected both major and minor effects that control these traits. A major nodulation interval at Pv06:28.0–28.27 Mbp was discovered. In this interval, the peak SNP was located within a small GTPase that positively regulates cellular polarity and growth of root hair tips. Located 20 kb upstream of this peak SNP is an auxin-responsive factor AUX/indole acetic auxin (IAA)-related gene involved in auxin transportation during root nodulation. For %NDFA, nitrate (NO3−) transporters, NRT1:2 and NRT1.7 (Pv02:8.64), squamosa promoter binding transcriptome factor (Pv08:28.42), and multi-antimicrobial extrusion protein (MATE) efflux family protein (Pv06:10.91) were identified as candidate genes. Three additional QTLs were identified on chromosomes Pv03:5.24, Pv09:25.89, and Pv11: 32.89 Mbp. These key candidate genes from both traits were integrated with previous results on N2 fixation to describe a BNF pathway.
Introduction
Common bean (Phaseolus vulgaris L.) is a major crop of smallholder farmers in Latin America, the Caribbean, and Eastern and Southern Africa. In these regions, common beans are often grown on soils deficient in nitrogen (N2; Fenta et al., 2020) and among grain legumes, the species has a relatively low biological N2 fixation (BNF) capacity (Mnasri et al., 2007). The application of N2 fertilizer can increase bean yields, but the input is often too expensive and/or unavailable to smallholder farmers.
Biological N2 fixation is a process in which rhizobia microorganisms in the soil convert atmospheric N2 to ammonia (NH3). This process generates a usable form of nitrogenous compounds for the plant in an economical and environmentally friendly manner through nodulation (Akter et al., 2018). Nodulation is a symbiotic interaction between rhizobia and the legume plant in which the plant provides carbohydrate to the rhizobial species, and the bacteria convert atmospheric N2 into ammonium for use by the plant (Wang et al., 2018). Although common bean is considered a poor N fixer, genetic diversity for N2 fixation exists within the species. This diversity enables the selection of germplasm with enhanced BNF capacity (Buttery et al., 1987; Barron et al., 1999; Farid et al., 2017; Kamfwa et al., 2019). Pereira et al. (1993) were able to significantly increase the nodule number of common bean lines after three cycles of recurrent selection in a controlled environment. The results demonstrated that nodule number is a heritable trait, and Bliss (1993) suggested that nodule number could be used as a criterion to estimate BNF capacity.
The identification of key genetic factors associated with nodulation and the physiological response in a diverse collection of germplasm may enable the development of molecular markers to select for enhanced levels of N fixation capacity. Previously, genetic mapping (Nodari et al., 1993; Heilig et al., 2017; Kamfwa et al., 2019) and transcriptional regulation studies of the BNF response (Blanco et al., 2009) provided valuable information regarding BNF in common bean. However, few genetic studies to understand the genetic regulation of BNF across a diverse panel of common bean germplasm have been conducted.
Selecting bean lines with greater BNF capacity in breeding nurseries with high N2 fertilizer soil amendments negates the plant’s need for effective BNF capacity. Although quantifying BNF itself is difficult, studying related traits, such as seed yield, photosynthesis, biomass partitioning, root morphology, plasticity, and nodulation, along with environmental effects, can provide a framework for the genetic improvement of BNF in common bean (Diaz et al., 2017; Fenta et al., 2020).
Initially, the lack of a simple, rapid, and economical method to measure BNF capacity limited genetic improvement (Purcell, 2009). The discovery that BNF capacity in common bean is related to root nodule number and weight (Pereira et al., 1993), enabled the development of an effective screening procedure. BNF capacity can also be indirectly quantified by calculating percentage of N2-derived from atmosphere (%NDFA). This procedure compares the amount of 15N (heavy N2 isotope) for a genotype relative to the 15N value of a control genotype that does not have the capacity to nodulate and fix N2. This value, known as δ15N, is obtained from the ratio of 15N: 14N. The abundance of 15N in different parts of a legume plant can be obtained by calculating the relative deviation from the 15N: 14N ratio. In a recent study, the significant genetic variation among recombinant inbred lines (RILs) derived from Middle American genotypes for %NDFA demonstrated that the genetic variation among RILs would be mostly additive (Farid et al., 2017). Furthermore, significant QTL for %NDFA was detected within Andean gene pool (Ramaekers et al., 2013). These significant and repeatable differences between lines in both common bean gene pools suggest additive genetic effects play important role for %NDFA. Previous studies in soybean (Glycine max L. Merr.) confirmed that the differences in δ15N between nodulated roots from different lines or tissues are a robust means for quantifying %NDFA (Wanek and Arndt, 2002). Polania et al. (2016) noted that the natural abundance of 15N estimated using seed tissue would be an appropriate estimate for BNF capacity of common bean.
Given the importance of the nodule numbers and %NDFA in BNF estimation, genetic mapping these two traits may lead to a better understanding of the genetic factors affecting BNF capacity in common bean. In this study, we evaluated a panel specifically developed for abiotic stress tolerance. The aim of this study was to: (1) characterize common bean genotypes with high capacity for N2-fixation in tropical soil conditions and (2) discover the genomic regions and candidate genes associated with nodulation and %NDFA under tropical soil conditions.
Materials and Methods
Bean Abiotic Stress Evaluation (BASE_120) field trials (Oladzad et al., 2019) were conducted at the Isabela Substation of the Agricultural Experiment Station of the University of Puerto Rico. The substation is located on the northwestern coastal plain of Puerto Rico at 18.468 N, −67.042 W at an altitude of 128 m above the sea level. The average annual minimum/maximum temperature at the Isabela Experimental Substation is 22.2/27.8°C, with an average annual rainfall of 1,630 mm. The trial was conducted in a field with Coto Clay soil, a very-fine, kaolinitic, isohyperthermic Typic Eutrustox. Soil subsamples were taken at random from different positions in the fields, where the BASE_120 trials were conducted. The subsamples were mixed to prepare a composite sample for each field. The soil analyses were conducted at AgSource Harris Laboratories in Lincoln, NE (Supplementary Table S1).
Field trials were planted in a randomized complete block design with five replications in four growing seasons; June and November 2015, June 2016, and June 2018. The experimental units were single 3-m rows with 0.76-m spacing between rows. Each BASE_120 trial included 118 common bean and two tepary bean (Phaseolus acutifolius L.) genotypes (Supplementary Table S2). Entries in the BASE_120 trials included elite bean breeding lines and cultivars from Zamorano University in Honduras, the International Center for Tropical Agriculture (CIAT) in Colombia, the University of Puerto Rico, USDA-ARS Tropical Agriculture Research Station in Puerto Rico, and Michigan State University. The BASE_120 genotypes were almost exclusively of race Mesoamerican origin, while including the Andean cultivars “Calima,” “Indeterminate Jamaica Red” (IJR), and “Quimbaya.” The seeding rate was 14 seeds per linear meter. The field trial received supplementary aerial irrigation to avoid drought stress. Weeds were controlled manually. Standard agronomic practices for weed and insect control were used in the trials.
No fertilizer was applied to the BASE_120 trials in order to produce soil conditions that favor nodulation and symbiotic N2 fixation. In the June 2015 trial, seeds were inoculated with a liquid suspension (107 rhizobium cells per milliliter) of two strains of Rhizobium; R. etli (CIAT 632) and R. tropici (CIAT 899). The use of inoculant containing a mixture of more than one strain of Rhizobium is a recommended agronomic practice in common bean (Liu et al., 2020a,b). The inoculant was applied to the seed pre-planting using a sticker, PREMAX (1 ml/kg of seed), and the same CIAT 632 and CIAT 899 inoculant (100 g/kg of seed) by stirring the inoculant and the seed of each genotype in a container. The inoculum was also applied directly to the seeds in the row at planting, and to the base of the seedlings 1 week after planting, using a backpack sprayer. The inoculations for the second, third, and fourth growing seasons were performed using a peat-based inoculant.
Two plants per experimental unit were carefully dug up about 0.75 m from the end of the second row approximately 45 days after planting during flowering. Root nodule numbers were evaluated by assigning values from 1 to 9 using the CIAT scale (Schoonhoven and Pastor-Corrales, 1987), where 1 indicates that the root has >80 nodules, 3 = 41–80 nodules, 5 = 21–40 nodules, 7 = 10–20 nodules, and 9 = less than 10 nodules per plant. Seed samples from three replications of the trials conducted at the Isabela Substation in 2016 and 2018 were used to estimate %NDFA. Approximately five seeds of each sample were dried at 70°C until a constant weight was achieved then ground using a Wiley mini-mill (Thomas Scientific, Swedesboro, New Jersey, United States), and passed through a #40 mesh sieve, resulting in a fine powder. Samples containing 4.2 mg of the seed powder were packaged in 5 × 8 mm tin capsules (D1008, EA Consumables, Pennsauken, NJ, United States) and shipped to the University of California, Davis Stable Isotope Facility in 96-well plates. The 15N natural abundance method (Unkovich et al., 2008) was used to calculate %NDFA. The white bean R-99 was used as the non-N-fixing reference line. %NDFA was calculated as:
where β represents the δ15N of the bean line grown under N-free conditions and relies on BNF for all N requirements. In this study, β was assigned the value of 0 because the R99 reference line does not nodulate. It was assumed that there was no isotopic fractionation in seed harvested from the genotypes grown under N-free conditions. The entries in the BASE_120 trail and the R99 reference bean line trial have similar patterns of phenological development and seed size.
Statistical analyses were performed for nodulation scores and %NDFA estimates using a GLIMMIX model from SAS/STAT14.1 (SAS Institute, Cary, NC, United States) to fit the model, estimate the main effects and interactions, and to compare the least squares (LS) means. Planting dates and bean genotypes were considered fixed effects, whereas replication within planting date was considered a random effect. A 95% probability level was used to establish statistical differences.
A genome-wide association study (GWAS) was performed using the phenotypic data for the BASE_120 genotypes, and their corresponding SNPs. The Base_120 was genotyped with SNPs (n = 2,05,293) generated from genotype-by-sequencing (GBS) reads of 469 Middle American genotypes (Oladzad et al., 2019). Only SNPs with minor allele frequencies ≥5% were extracted from this HapMap for the GWAS analysis using the GEMMA software (Zhou and Stephens, 2012, 2014). The kinship matrix, obtained from the centered relatedness algorithm, was included in the GWAS model as a random effect, and the structure matrix, obtained from the principal component analysis (PCA) using the Prcom function in R3.5, was considered a fixed effect (Price et al., 2006). The smallest number of principle components that accounted for at least 25% of the cumulative variation was used in the mixed GWAS model. Those SNPs whose values of p were in the lower 0.01 and 0.05% tail of the bootstrap distribution were considered significant. Bootstrapping was performed in R with 1,000 resampling. This approach is sensitive to the fact that the more genetic factors affecting a phenotype, the corresponding values of p would be higher. Artificial thresholds work best for traits in which a few genetic factors are involved (such as disease resistance loci). These will typically pass the conservative Bonferroni test. But for other traits, we believe the best way is to define the cut off value based on the trait and the specific population under study. That is why in the current study, 1,000 permutation tests and a p value bootstrap of two cutoff values were used. The amount of phenotypic variation explained by these significant SNPs was estimated by a likelihood-ratio-based (R2LR) analysis in R using the GenABEL package that included population structure and/or relatedness effects (Aulchenko et al., 2007; Sun et al., 2010). While GWAS results are indeed related to LD, it is important to note (and often little appreciated) that LD is a function of recombination. Genomic studies clearly show that recombination is not equal across the genome, especially as it relates to LD. For example, Moghaddam et al. (2016) clearly demonstrated LD must be considered in a regional context, and that regional context is population specific. The regional and population differences mean that LD values averaged across chromosomal regions, across whole chromosomes, and across populations have little value. What is important is the degree of LD in the narrow region, where we find the genetic factors associated with a specific phenotype. The question is what is the solution with regard to selecting a window size for candidate gene selection? The only true solution is to calculate the Mb per cM ratio around each genetic signal discovered by the GWAS analysis and choosing a specific cM distance to define the physical boundaries for candidate gene selection. The challenge though is that we do not know what genetic map to use since each biparental parent will have a different recombination frequency in the region of interest. Therefore, choosing a window size (admittedly somewhat arbitrarily), searching for a gene(s) in that region, and, based on previous analysis of the function of the gene as outlined in referred literature, makes a case for its possibility as a candidate gene. In the current study, candidate genes were identified within a conservative window size of ±50 kb interval of the significant SNPs. The possible effect of SNPs located inside a gene model or its promoter region was investigated using the SNPEff (Cingolani et al., 2012) database for common bean reference genome sequence version 2 (Oladzad et al., 2019). Finally, to check whether significant SNP markers near or within the major candidate genes associated with both traits, exhibit hitchhiking effects due to extensive LD, the pairwise LD in null model, using Tassel v5.0 (Bradbury et al., 2007), as well as in mixed model accounting for both kinship and population structure using R package LDcorSV (Mangin et al., 2012) were estimated.
Results
The BNF capacity of a legume is associated with nodule-mediated nitrate (NO3−) production and general NO3− uptake and mobilization. Integrating nodulation and %NDFA data with genotyping data in a genetic analysis enabled the identification of genomic intervals and candidate genes associated with BNF capacity and N metabolism under tropical soil conditions in common bean.
Phenotypic Analyses of %NDFA Estimates and Nodulation Scores
Significant differences between seasons and among entries were found for %NDFA (Table 1). Although an overall season × entry interaction for %NDFA was not observed, several entries in the BASE_120 trial had significant F tests for the season × entry LS means slice (Supplementary Table S3). LS means for %NDFA from the June 2018 planting were generally lower than the LS means from the June 2016 planting. The range of LS means was comparable to %NDFA estimates for common bean in previous studies based on seed tissue (Polania et al., 2016). The BASE_120 control variety “ICA Pijao” had among the highest estimated %NDFA for both growing seasons.

Table 1. Type III tests of fixed effects for %NDFA and nodulation scores from bean abiotic stress evaluation (BASE) 120 field trials conducted at Isabela, Puerto Rico from 2016 to 2018.
Based on results from previous studies, Polania et al. (2016) estimated that the average %NDFA for common bean was 39%. Several BASE_120 lines including “Beníquez,” FBN 1205-31, FBN 1210-48, “INTA Centro Sur,” “Paraisito,” PR0443-151, PR1418-15′, “Rosetta,” “Sayaxché ML,” SB2-4, and SER 125 had LS means for %NDFA ≥ 50% in 2016 and %NDFA ≥ 40% in 2018 (Supplementary Table S3). In a N-free trial conducted by (Heilig et al., 2017), no significant differences in δ15N were found between flowering plants of “Pueblo 152,” “Zorro,” “Medalist,” and PR0443-151. Polania et al. (2016) used a constant β value for genotypes having type III growth habits based on greenhouse studies conducted by CIAT in Cali, Colombia. The use of a constant β value, however, did not change the ranks of %NDFA estimates among the entries. Unkovich et al. (2008) observed that the size of the β value is less important when %NDFA estimates are low (< 50%).
There were significant differences between seasons, entries, and season × entry interaction for nodulation scores. Nodulation and N2 fixation in common beans are sensitive to higher temperatures (Hungria and Franco, 1993) and soil moisture conditions (Hungria and Vargas, 2000; Polania et al., 2016), which may have contributed to variability in nodulation scores between seasons. The control variety “ICA Pijao” had among the highest nodulation scores across seasons. Other lines that combined superior %NDFA and a greater number of nodules included “Beníquez,” “INTA Centro Sur,” “Paraisito,” PR1418-15, and SB2-4. Among the 112 lines with data for both traits, the Pearson correlation between mean %NDFA and mean nodulation score was highly significant (r = 0.60). Diaz et al. (2017) reported that %NDFA was correlated with seed yield and suggested that enhanced BNF may be beneficial under abiotic stress.
Association Mapping and Identification of Candidate Genes
After filtering for MAF ≥ 0.05, a total of 1,25,745 SNPs out of 2,11,764 were extracted from the original HapMap (Oladzad et al., 2019). Two principal components explained 29.1% of the genetic variation and were used in the mixed linear model analysis. For nodulation score, a block of SNPs in high LD located on Pv06 between 28.19 and 28.25 in the 0.01% cutoff threshold were associated [log10(P) = 7.46] with the trait (Figure 1A). These SNPs explained 11% of the phenotypic variation. When SNPs in the 0.05% cutoff threshold were considered, the interval extended to 28.73 Mbp and cumulatively explained 13% of the phenotypic variation (Tables 2, 3). A total of 21 gene models were detected ±50 kb of this block. Four SNPs in complete LD at Pv06:28.198 Mbp; [−log10 (P) = 7.54] were located inside the gene model Phvul.006G18000, an ortholog of the Arabidopsis RAB GTPase. The auxin-responsive factor AUX/indole acetic auxin (IAA)-related gene model (Phvul.006G181200) was located 20 Kb upstream of RAB GTPase related gene model.
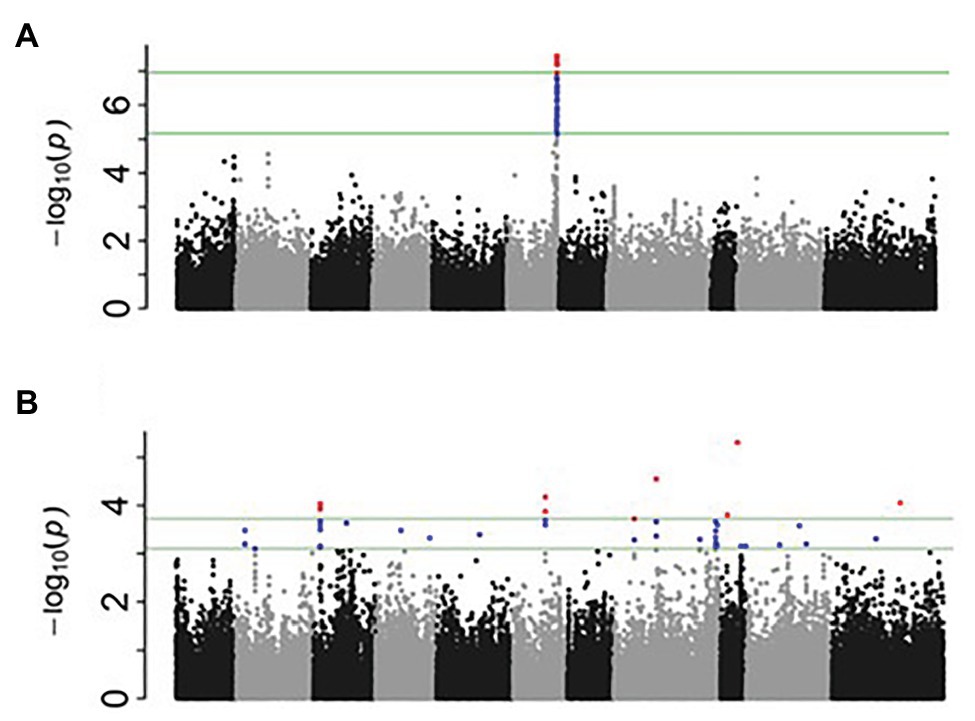
Figure 1. Manhattan plots generated from genome-wide association study (GWAS) analysis for nodulation (A) and percentage of N2-derived from atmosphere (%NDFA; B).
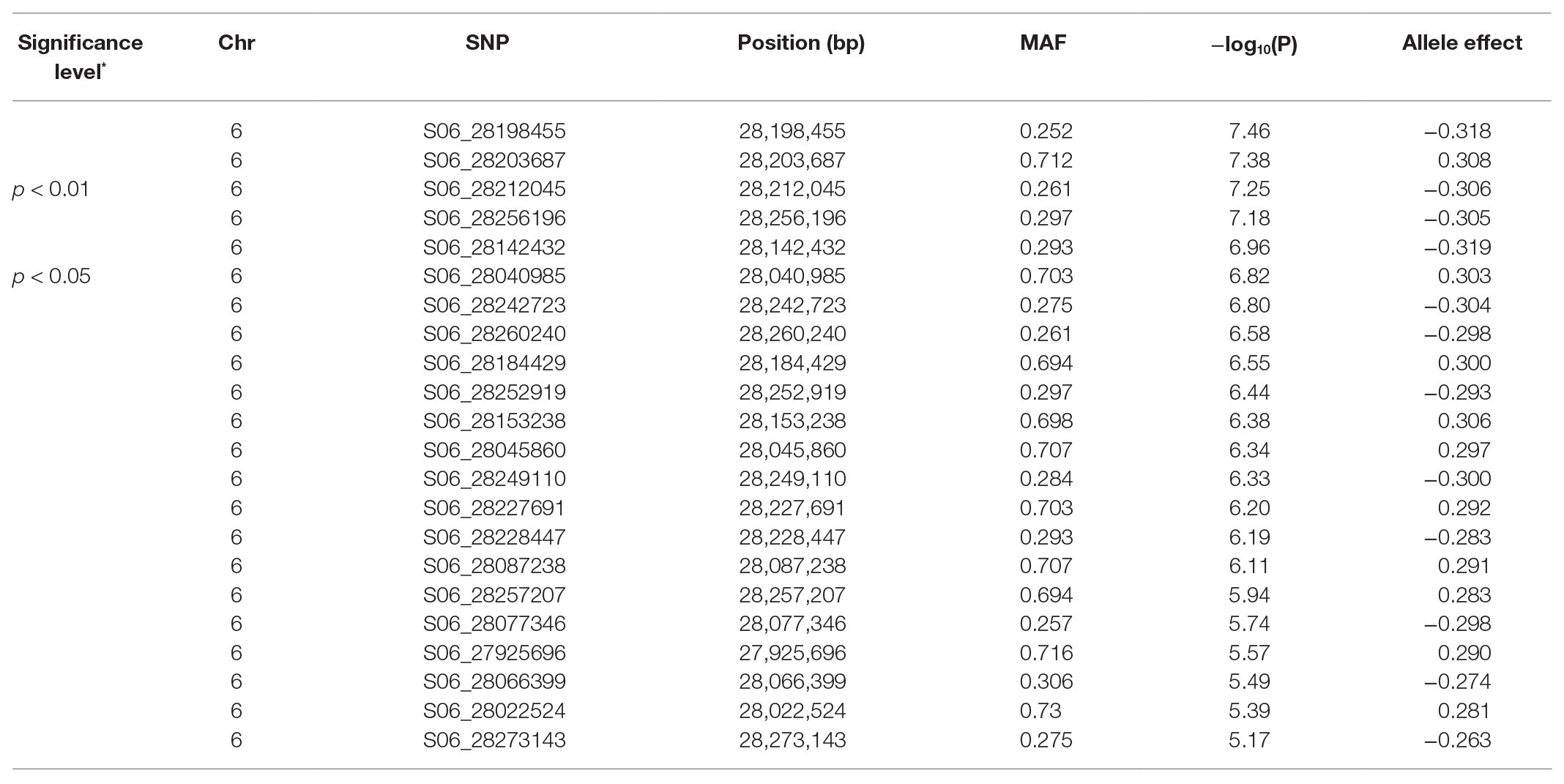
Table 3. Significant SNPs associated with nodulation in Middle American genotypes (redundant markers were removed).
For %NDFA, a total of 25 smaller effect, significant intervals containing 53 SNPs were detected in the 0.05% cutoff threshold (Figure 1B). The peak SNPs among these intervals cumulatively explained 36% of the phenotypic variation in %NDFA (Tables 2, 4). The peak SNP [−log10 (P) > 5.31] was detected at Pv09:25.89 Mbp and was located ~189 kb upstream of another annotated RAB GTPase gene. The second most significant SNP interval was 98 bp in length at Pv08:28.42 Mbp and was located ~2.5 kb upstream region of gene model Phvul.008G157200, an ortholog of a squamosa promoter binding protein-like 8 (SPL8). The SNPEff results revealed that this SNP was placed in the promotor regions of SPL8 and potentially has a modifier effect. A SNP at Pv11:32.89 Mbp was detected 17 Mbp downstream of a small auxin up RNA (SAUR)-like auxin responsive protein family cluster. NO3− transporters, NRT1:7 and NRT1.2 (orthologs to gene models Phvul.002G067700 and Phvul.002G067214, respectively) flanked the Pv02:8.64 Mbp peak SNP within the heterochromatic region of Pv02. Finally, the Pv06:10.91 Mbp SNP interval is located ~40 kb downstream of a MATE efflux protein (gene model; Phvul.006G028701) known to be involved in flavonoid metabolism (Marinova et al., 2007), which is also critical for nodule development (Ng et al., 2015). Despite the importance of these candidate genes potentially involved in N metabolism, due to the quantitative nature of these traits, all the gene models within ±50 kb of the significant intervals were presented in the Supplementary Table S4. Furthermore, for more powerful trait discovery, single GWAS analyses were performed on phenotypic data from each season and year. The results and Manhattan plots are presented in the Supplementary Table S5 and Supplementary Figure S1. The results showed that the significant SNPs on Pv06 for nodulation which was detected from Ls-mean values within a LD block, was also identified in 3 seasons/years (November 2015, June 2018, and June 2015) and interestingly the significant SNP on Pv02; 14.33Mbp was identified in both nodulation and %NDFA in June 2016 and June 2018, respectively. This SNP was upstream of gene model Phvul.002G088100. Furthermore, the most significant SNPs on Pv09, Pv08, Pv06, and Pv11 that were detected from Ls-mean values for %NDFA, were also identified in the single GWAS analysis of June 2016 and June 2018. The pairwise LD values were reported as squared allele frequency correlation in the Supplementary Table S6 for both null model and mixed model accounting for both population structure and relatedness. Based on these values, the significant SNPs are not in high LD specifically when population structure and relatedness are accounted in the model. However, some degree of LD was observed between Pv08:28.42 and two other SNPs on Pv02:8.64 and Pv09:25.89Mbp in the null model and may very well be due to the gene by gene interactions within this pathway. As a final point, for each peak SNP, the allele associated with higher %NDFA and lower nodulation scores (higher number of nodules) were determined, and the mean of each trait of all possible haplotypes was calculated (Table 5). Selecting the genotypes for negative alleles for nodulation and positive alleles for %NDFA revealed that although both nodulation and %NDFA are indicators of BNF capacity, different genetic factors control each of these traits, and selection based on marker data should be independent for each trait (Supplementary Table S7).
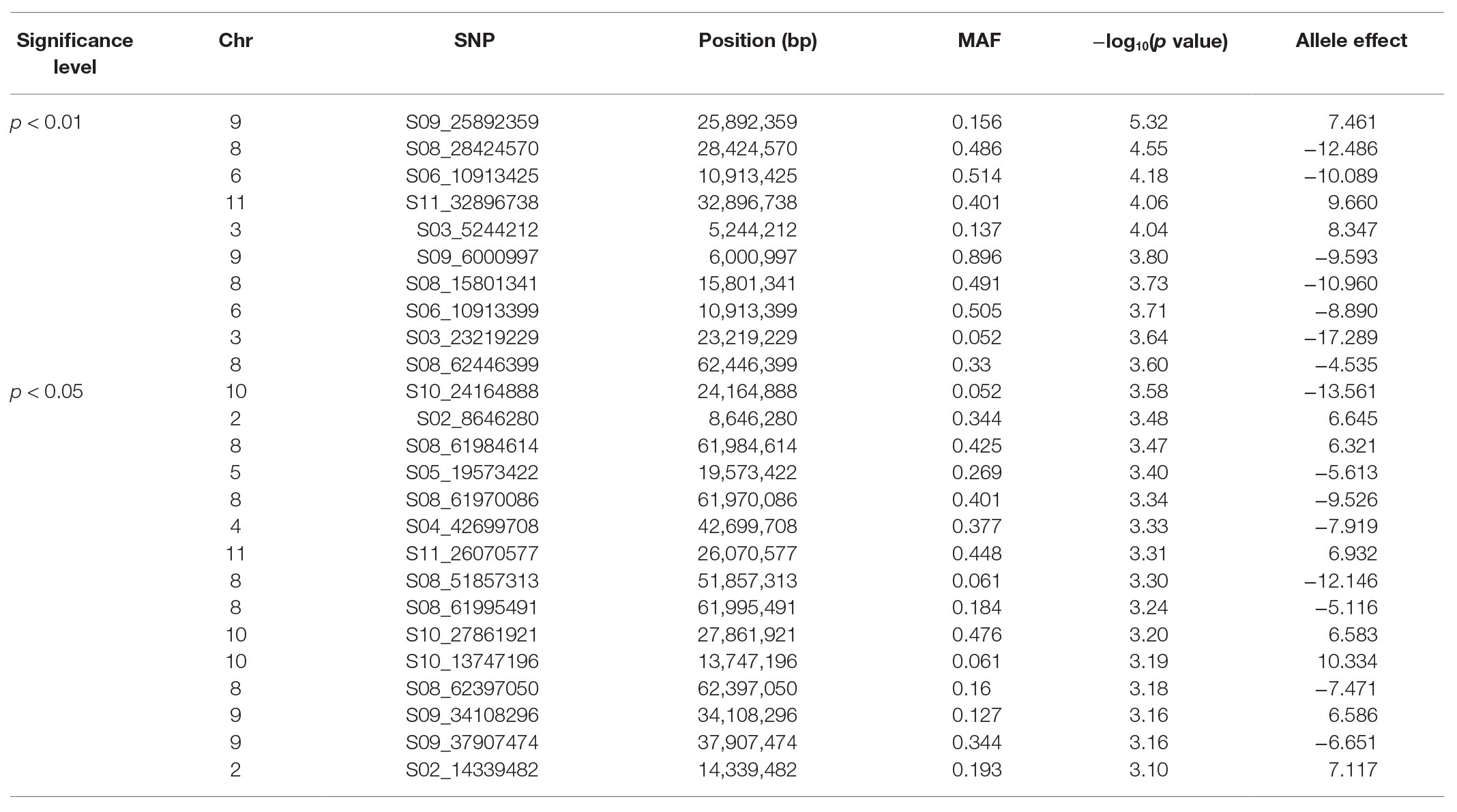
Table 4. Significant SNPs associated with %NDFA in Middle American genotypes (redundant markers were removed).
Discussion
The contribution of BNF to common bean growth and development is key to its performance as a field crop, especially under field stress conditions. While it has been demonstrated previously that degree of nodulation and %NDFA are two key phenotypes related to BNF, the underlying genetic factors that control these traits were not studied before. In this study, a combination of detailed phenotypic data of a population with modern breeding alleles and in-depth genotyping of that population enabled the discovery of key genetic factors for nodulation and %NDFA. The individual genetic analysis for each trait was robust. These genetic analyses also enabled a description of the interactions of a genetic pathway that controls BNF.
The phenotypic analysis confirmed that the range of LS means for seed %NDFA in this common bean population was comparable to previous studies based on seed tissue (Polania et al., 2016). While nodulation and N2 fixation in common beans are sensitive to high temperatures (Hungria and Franco, 1993) and inadequate soil moisture (Hungria and Vargas, 2000; Polania et al., 2016) two conditions that affected the variability in nodulation phenotypes/scores in this study, the consistency of the range of phenotypic when compared with previous studies suggests the data was representative of the modern germplasm and appropriate for performance and genetic analysis. This study identified bean lines capable of obtaining a significant portion of the N needed for growth and development from BNF. The BASE_120 trials were conducted in soils at Isabela, Puerto Rico that have low levels N and P. Therefore, these results should be relevant to small-scale bean producers in the tropics, who often do not have access to fertilizer inputs. Moreover, Heilig et al. (2017) evaluated %NDFA in a RIL population derived from the cross “Puebla 152 x Zorro” (Mesoamerican) at Isabela, Puerto Rico during 2012 and 2013. This study was conducted under conditions similar to the BASE 120 field trials in our study; an unfertilized oxisol (Coto Clay). Significant differences were observed among RILs for %NDFA. Although narrow sense heritability was not estimated, the significant difference among RILs suggests that additive genetic effects were important in the expression of %NDFA in this “Puebla x 152 x Zorro” RIL population.
Associating genetic variation in BNF with specific genomic regions and SNPs offers an important tool for molecular breeding. In this study, we utilized the 200 k SNPs generated previously from a USAID bean project (Oladzad et al., 2019) and identified genomic regions associated with two critical phenotypes associated with BNF in the Middle American gene pool. Within these regions, six important candidate genes previously determined by cellular and physiological analysis of transgenic plants to be associated with N2 fixation (Borg et al., 1997; Jürgens, 2004; Wang, 2004; Blanco et al., 2009; Bloch et al., 2011) were identified. The major SNP for nodulation is located in a gene encoding a small Rab GTPase protein. These classes of proteins were shown to have a positive regulatory role in root hair tip growth (Borg et al., 1997; Meschini et al., 2008; Bloch et al., 2011), a developmental function necessary for the establishment of the bean/rhizobia symbiosis. Gene expression analysis suggested a common bean Rab GTPase protein regulates the intercellular vesicle trafficking in root, which determines cell polarity in growing root hairs (Jürgens, 2004; Wang, 2004; Blanco et al., 2009), and therefore is required for nodulation. Silencing this gene reduced or eliminated nodulation by rhizobia (Blanco et al., 2009). A parallel molecular network involving Rab GTAPase family proteins, reactive oxygen species (ROS), phosphatidylinositide, and Ca+2 signaling pathways establishes the symbiotic interactions when legumes are infected by rhizobacteria (Jürgens, 2004; Wang, 2004; Peleg-Grossman et al., 2007).
The AUX/IAA-related auxin-responsive factor located 20 kb upstream of the Rab GTPase gene is another candidate. Shoot-to-root auxin transport regulates nodule number. Auxin transport was shown to be closely associated with nodule development using biological function, gene expression, and high performance liquid chromatography-immunoassay analyses (Atzorn et al., 1988; Pii et al., 2007; Mathesius, 2008; Barraza et al., 2018; Zou et al., 2019). Upon rhizobia infection, an alteration of the auxin balance is a prerequisite for nodulation (Mathesius, 2008; Jin et al., 2012; Kohlen et al., 2018). Van Noorden et al. (2016) showed that under N2-rich conditions, the number of nodules is reduced because NO3− inhibits the synthesis of flavonoids in the host root (Coronado et al., 1995). Because flavonoids control the accumulation of auxin at the site of nodule formation (Ng et al., 2015), auxin transport consequently the number of nodules can be affected by the presence of NO3−. However, Van Noorden et al. (2016) determined that IAA accumulation at the root-shoot junction of Medicago truncatula was not affected by NO3− 24 h after inoculation. According to Liu and Murray (2016), the flavonoids are the key molecules for biosynthesis of nod factors (NFs), which are the signaling molecules produced by rhizobacteria during interaction with plant. However, it has been shown that flavonoids can also simulate nod expressions even before rhizobia-legume interaction through rhizobial chemotaxis (Hirsch et al., 2001).
Data of %NDFA are used as a proxy for “N15 natural abundance” to quantify the contribution of N2 fixation in common bean to N2 metabolism. The GWAS results of that data identified two candidate genes related to nodulation, SPL8, and SAUR-like auxin responsive protein, and two candidate genes were related to NO3− transport, NRT1:2 and NRT1.7. The SPL gene family is a target gene for miR156 (Yu et al., 2015), which affects the regulation of nodule development in plants (Wang and Wang, 2015). Overexpression of this miRNA downregulates nodulation in legumes by silencing downstream SPL genes. In both common bean and soybean, it has been shown that SPL activates the transcription of miR172, which is highly accumulated in the mature nodules. miR172 binds APETALA2 (AP2), protein positively nodule senescence genes in common bean. In mature nodules, AP2-1 is silenced by miR172 (Íñiguez et al., 2015; Nova-Franco et al., 2015). In soybean, it has been demonstrated that AP2-2 controls the non-symbiotic hemoglobin expression (Hb1), which affects the level of nodulation by regulating nitrogenase activity (Yan et al., 2013). However, it is not clear which subfamily of SPLs in this regulatory network is associated with nodulation in common bean. SAURs were also detected as potential candidate genes. These are the largest families of early auxin response genes (Ren and Gray, 2015), and auxin families are associated with root nodule symbiosis.
Nitrate transporters NRT1:2 and NRT1.7 are both members of the NRT1 NO3− transporter subfamily system (later renamed the NPF family Léran et al., 2014) that transfer a wide range of molecules including auxin and nitrates (Fan et al., 2009). The NPF family uses a proton gradient to transport NO3− from the soil to different parts of the plant (Segonzac et al., 2007). The NRT1:2 mRNA was found in root hairs and in the epidermis of young and mature roots (Huang et al., 1999; Guo et al., 2002), while NRT1.7 mRNA was located in the phloem tissue of older leaves. This suggests a role of NRT1.7 in the remobilization of NO3− from source to sink. This role was further confirmed by analyzing the N15 content of the older and younger leaves in wild (NRT1.7) and mutant (nrt1.7) Arabidopsis (Fan et al., 2009). In wild type genotypes, N15 was remobilized from older leaves to the younger leaves, while in the mutant; higher amount of NO3− was accumulated in older leaves and was not transferred to the younger tissues.
Integrating the information from both nodulation score and %NDFA shows that the SNPs which mapped in or adjacent to candidate genes are associated with BNF in common bean. Figure 2 integrates our genetic results into a N2 metabolism pathway based on previous research to show how the candidate genes interact to provide a high level of N2 fixation in low fertile soil. For this genotype, rhizobacteria infection triggers the expression of the MATE efflux gene involves in flavonoid metabolism. Flavonoids prompt the transcription of genes related to the biosynthesis of the NFs in the soil which are perceived by the LRR domain of plasma membrane-localized receptor-like kinases (RLKs; Ferguson et al., 2010; Kamfwa et al., 2015). This complex would interact with a RAB family GTpase (Choudhury and Pandey, 2016), which alters the hair root cell polarity, resulting in nodule induction. The expression of auxin transporters from shoot to root also triggers flavonoids release, which would also lead to high auxin accumulation in the root resulting in greater lateral root development and consequently greater nodule number. Moreover, upon Rhizobia infection, the expression of SPL would increase miR172 levels until nodulation reaches it maximum accumulation in the mature plant. Higher levels of miR172 also improve root growth which subsequently increases the rhizobia infection and therefore a higher expression of nodulation regulatory-related genes. This leads to further nodule as the plant matures (Nova-Franco et al., 2015). The overall effect of this integrated genetic pathway is an increase in N fixation capacity. The ammonium (NH3+) produced in this process can be converted to NO3− by nitrifying bacteria. The NO3− will then be transported from root to shoot by NRT1.2, and later, remobilized by NRT1.7 from older leaves to the younger leaves. During plant maturation, NO3− will be distributed to the seeds which increases %NDFA (Diaz et al., 2017). As a final point, it should not be rolled out that despite the statistical power of GWAS discovery of the candidate genes for complex traits, still there is potential for missing medium and low-effect associations specifically after filtering for allele frequency which is commonly employed in GWAS analysis. It has been suggested that sequencing the candidate genes specifically in individuals with extreme phenotypes of a quantitative traits may be able to detect other associations but the sample size at the extremes of a quantitative trait is also debatable because it can be affected by small odds ratios (Manolio et al., 2009). However, using GWAS to introduce a breeder “friendly” marker was beyond the scope of this study, rather it aimed using GWAS as a genetic approach to understand the molecular pathways.
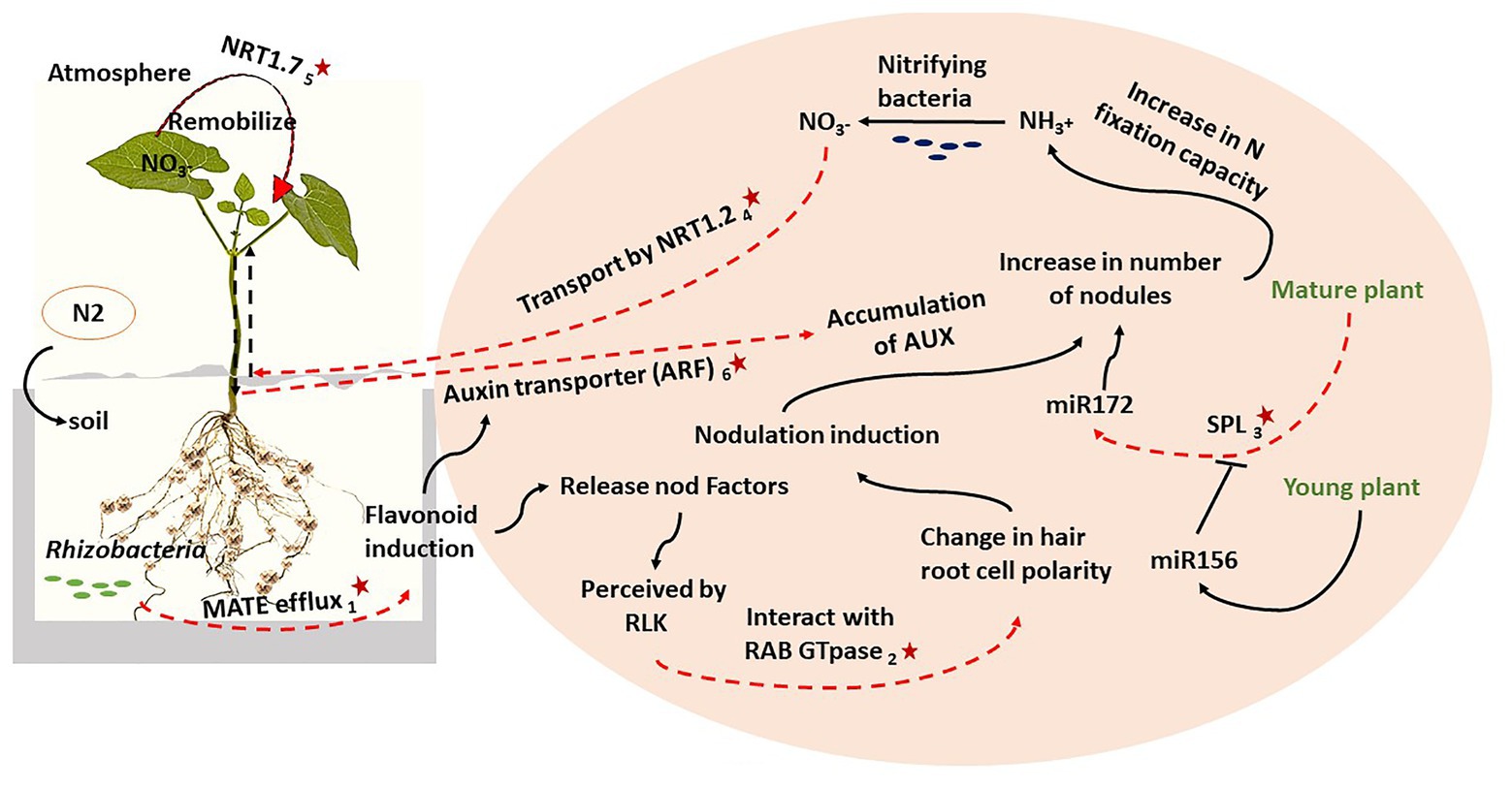
Figure 2. The key candidate genes (red stars) from both traits were integrated with previous results on nitrogen (N2) fixation to describe a biological nitrogen (N2) fixation (BNF) pathway: (1) Phvul.006G028701, (2) Phvul.006G18000, (3) Phvul.008G157200, (4) Phvul.002G067700, (5) Phvul.002G067214, and (6) Phvul.006G181200.
Data Availability Statement
The original contributions presented in the study are included in the article/supplementary material, further inquiries can be directed to the corresponding authors.
Author Contributions
AO, AG, RM, and CJ performed data analysis and JB, TP, and PM supervised the data analysis. JB and TP designed the phenotypic experiment and AO and PM designed the genomics approach for the association mapping. AO, AG, and RM discussed the results and the interpretation of the final data and JB, TP, and PM provided suggestions to improve it. All authors contributed to the article and approved the submitted version.
Funding
This research was supported by USAID Feed the Future Innovation Laboratory for Climate-Resilient Beans through grant USAID OAA-A-13-00077.
Conflict of Interest
The authors declare that the research was conducted in the absence of any commercial or financial relationships that could be construed as a potential conflict of interest.
Acknowledgments
We would like to thank Rian Lee, North Dakota State University, for his lab support.
Supplementary Material
The Supplementary Material for this article can be found online at: https://www.frontiersin.org/articles/10.3389/fpls.2020.576078/full#supplementary-material
References
Akter, Z., Pageni, B., Lupwayi, N. Z., and Balasubramanian, P. (2018). Biological nitrogen fixation by irrigated dry bean (Phaseolus vulgaris L.) genotypes. Can. J. Plant Sci. 98, 1159–1167. doi: 10.1139/cjps-2017-0301
Atzorn, R., Crozier, A., Wheeler, C., and Sandberg, G. (1988). Production of gibberellins and indole-3-acetic acid by rhizobium phaseoli in relation to nodulation of Phaseolus vulgaris roots. Planta 175, 532–538. doi: 10.1007/BF00393076
Aulchenko, Y. S., Ripke, S., Isaacs, A., and Van Duijn, C. M. (2007). GenABEL: an R library for genome-wide association analysis. Bioinformatics 23, 1294–1296. doi: 10.1093/bioinformatics/btm108
Barraza, A., Coss-Navarrete, E. L., Vizuet-De-Rueda, J. C., Martínez-Aguilar, K., Hernández-Chávez, J. L., Ordaz-Ortiz, J. J., et al. (2018). Down-regulation of PvTRX1h increases nodule number and affects auxin, starch, and metabolic fingerprints in the common bean (Phaseolus vulgaris L.). Plant Sci. 274, 45–58. doi: 10.1016/j.plantsci.2018.05.006
Barron, J. E., Pasini, R., Davis, D., Stuthman, D., and Graham, P. (1999). Response to selection for seed yield and nitrogen (N2) fixation in common bean (Phaseolus vulgaris L.). Field Crop Res. 62, 119–128. doi: 10.1016/S0378-4290(99)00009-X
Blanco, F. A., Meschini, E. P., Zanetti, M. E., and Aguilar, O. M. (2009). A small GTPase of the Rab family is required for root hair formation and preinfection stages of the common bean-rhizobium symbiotic association. Plant Cell 21, 2797–2810. doi: 10.1105/tpc.108.063420
Bliss, F. A. (1993). Breeding common bean for improved biological nitrogen fixation. Plant Soil 152, 71–79. doi: 10.1007/BF00016334
Bloch, D., Monshausen, G., Gilroy, S., and Yalovsky, S. (2011). Co-regulation of root hair tip growth by ROP GTPases and nitrogen source modulated pH fluctuations. Plant Signal. Behav. 6, 426–429. doi: 10.4161/psb.6.3.14523
Borg, S., Brandstrup, B., Jensen, T. J., and Poulsen, C. (1997). Identification of new protein species among 33 different small GTP-binding proteins encoded by cDNAs from Lotus japonicus, and expression of corresponding mRNAs in developing root nodules. Plant J. 11, 237–250. doi: 10.1046/j.1365-313X.1997.11020237.x
Bradbury, P. J., Zhang, Z., Kroon, D. E., Casstevens, T. M., Ramdoss, Y., and Buckler, E. S. (2007). TASSEL: software for association mapping of complex traits in diverse samples. Bioinformatics 23, 2633–2635. doi: 10.1093/bioinformatics/btm308
Buttery, B., Park, S., and Findlay, W. (1987). Growth and yield of white bean (Phaseolus vulgaris L.) in response to nitrogen, phosphorus and potassium fertilizer and to inoculation with rhizobium. Can. J. Plant Sci. 67, 425–432. doi: 10.4141/cjps87-061
Choudhury, S. R., and Pandey, S. (2016). Heterotrimeric G-protein complex and its role in regulation of nodule development. Exocytosis Cell Res 27, 29–35.
Cingolani, P., Platts, A., Wang, L. L., Coon, M., Nguyen, T., Wang, L., et al. (2012). A program for annotating and predicting the effects of single nucleotide polymorphisms, SnpEff: SNPs in the genome of Drosophila melanogaster strain w1118; iso-2; iso-3. Fly 6, 80–92. doi: 10.4161/fly.19695
Coronado, C., Zuanazzi, J. S., Sallaud, C., Quirion, J. -C., Esnault, R., Husson, H. -P., et al. (1995). Alfalfa root flavonoid production is nitrogen regulated. Plant Physiol. 108, 533–542. doi: 10.1104/pp.108.2.533
Diaz, L. M., Ricaurte, J., Cajiao, C., Galeano, C. H., Rao, I., Beebe, S., et al. (2017). Phenotypic evaluation and QTL analysis of yield and symbiotic nitrogen fixation in a common bean population grown with two levels of phosphorus supply. Mol. Breed. 37:76. doi: 10.1007/s11032-017-0673-1
Fan, S. -C., Lin, C. -S., Hsu, P. -K., Lin, S. -H., and Tsay, Y. -F. (2009). The Arabidopsis nitrate transporter NRT1. 7, expressed in phloem, is responsible for source-to-sink remobilization of nitrate. Plant Cell 21, 2750–2761. doi: 10.1105/tpc.109.067603
Farid, M., Earl, H. J., Pauls, K. P., and Navabi, A. (2017). Response to selection for improved nitrogen fixation in common bean (Phaseolus vulgaris L.). Euphytica 213:99. doi: 10.1007/s10681-017-1885-5
Fenta, B. A., Beebe, S. E., and Kunert, K. J. (2020). Role of fixing nitrogen in common bean growth under water deficit conditions. Food and Energy Security 9:e183. doi: 10.1002/fes3.183
Ferguson, B. J., Indrasumunar, A., Hayashi, S., Lin, M. H., Lin, Y. H., Reid, D. E., et al. (2010). Molecular analysis of legume nodule development and autoregulation. J. Integr. Plant Biol. 52, 61–76. doi: 10.1111/j.1744-7909.2010.00899.x
Guo, F. Q., Wang, R., and Crawford, N. M. (2002). The Arabidopsis dual-affinity nitrate transporter gene AtNRT1. 1 (CHL1) is regulated by auxin in both shoots and roots. J. Exp. Bot. 53, 835–844. doi: 10.1093/jexbot/53.370.835
Heilig, J. A., Beaver, J. S., Wright, E. M., Song, Q., and Kelly, J. D. (2017). QTL analysis of symbiotic nitrogen fixation in a black bean population. Crop Sci. 57, 118–129. doi: 10.2135/cropsci2016.05.0348
Hirsch, A. M., Lum, M. R., and Downie, J. A. (2001). What makes the rhizobia-legume symbiosis so special? Plant Physiol. 127, 1484–1492. doi: 10.1104/pp.010866
Huang, N. -C., Liu, K. -H., Lo, H -J., and Tsay, Y. -F. (1999). Cloning and functional characterization of an Arabidopsis nitrate transporter gene that encodes a constitutive component of low-affinity uptake. Plant Cell 11, 1381–1392. doi: 10.1105/tpc.11.8.1381
Hungria, M., and Franco, A. A. (1993). Effects of high temperature on nodulation and nitrogen fixation by Phaseolus vulgaris L. Plant Soil 149, 95–102. doi: 10.1007/BF00010766
Hungria, M., and Vargas, M. A. (2000). Environmental factors affecting N2 fixation in grain legumes in the tropics, with an emphasis on Brazil. Field Crop Res. 65, 151–164. doi: 10.1016/S0378-4290(99)00084-2
Íñiguez, L. P., Nova-Franco, B., and Hernández, G. (2015). Novel players in the AP2-miR172 regulatory network for common bean nodulation. Plant Signal. Behav. 10:e1062957. doi: 10.1080/15592324.2015.1062957
Jin, J., Watt, M., and Mathesius, U. (2012). The autoregulation gene SUNN mediates changes in root organ formation in response to nitrogen through alteration of shoot-to-root auxin transport. Plant Physiol. 159, 489–500. doi: 10.1104/pp.112.194993
Jürgens, G. (2004). Membrane trafficking in plants. Annu. Rev. Cell Dev. Biol. 20, 481–504. doi: 10.1146/annurev.cellbio.20.082503.103057
Kamfwa, K., Cichy, K. A., and Kelly, J. D. (2015). Genome-wide association analysis of symbiotic nitrogen fixation in common bean. Theor. Appl. Genet. 128, 1999–2017. doi: 10.1007/s00122-015-2562-5
Kamfwa, K., Cichy, K. A., and Kelly, J. D. (2019). Identification of quantitative trait loci for symbiotic nitrogen fixation in common bean. Theor. Appl. Genet. 132, 1375–1387. doi: 10.1007/s00122-019-03284-6
Kohlen, W., Ng, J. L. P., Deinum, E. E., and Mathesius, U. (2018). Auxin transport, metabolism, and signalling during nodule initiation: indeterminate and determinate nodules. J. Exp. Bot. 69, 229–244. doi: 10.1093/jxb/erx308
Léran, S., Varala, K., Boyer, J. -C., Chiurazzi, M., Crawford, N., Daniel-Vedele, F., et al. (2014). A unified nomenclature of nitrate transporter 1/peptide transporter family members in plants. Trends Plant Sci. 19, 5–9. doi: 10.1016/j.tplants.2013.08.008
Liu, A., Ku, Y. -S., Contador, C. A., and Lam, H. -M. (2020a). The impacts of domestication and agricultural practices on legume nutrient acquisition through symbiosis with rhizobia and arbuscular mycorrhizal fungi. Front. Genet. 11:583954. doi: 10.3389/fgene.2020.583954
Liu, C. W., and Murray, J. D. (2016). The role of flavonoids in nodulation host-range specificity: an update. Plants 5:33. doi: 10.3390/plants5030033
Liu, J., Yu, X., Qin, Q., Dinkins, R. -D., and Zhu, H. (2020b). The impacts of domestication and breeding on nitrogen fixation symbiosis in legumes. Front. Genet. 11:00973. doi: 10.3389/fgene.2020.00973
Mangin, B., Siberchicot, A., Nicolas, S., Doligez, A., This, P., and Cierco-Ayrolles, C. (2012). Novel measures of linkage disequilibrium that correct the bias due to population structure and relatedness. Heredity 108, 285–291. doi: 10.1038/hdy.2011.73
Manolio, T. A., Collins, F. S., Cox, N. J., Goldstein, D. B., Hindorff, L. A., Hunter, D. J., et al. (2009). Finding the missing heritability of complex diseases. Nature 46, 747–753. doi: 10.1038/nature08494
Marinova, K., Pourcel, L., Weder, B., Schwarz, M., Barron, D., Routaboul, J. -M., et al. (2007). The Arabidopsis MATE transporter TT12 acts as a vacuolar flavonoid/H+-antiporter active in proanthocyanidin-accumulating cells of the seed coat. Plant Cell 19, 2023–2038. doi: 10.1105/tpc.106.046029
Mathesius, U. (2008). Goldacre paper: Auxin: at the root of nodule development? Funct. Plant Biol. 35, 651–668. doi: 10.1071/FP08177
Meschini, E. P., Blanco, F. A., Zanetti, M. E., Beker, M. P., Küster, H., Pühler, A., et al. (2008). Host genes involved in nodulation preference in common bean (Phaseolus vulgaris)—rhizobium etli symbiosis revealed by suppressive subtractive hybridization. Mol. Plant-Microbe Interact. 21, 459–468. doi: 10.1094/MPMI-21-4-0459
Mnasri, B., Aouani, M. E., and Mhamdi, R. (2007). Nodulation and growth of common bean (Phaseolus vulgaris) under water deficiency. Soil Biol. Biochem. 39, 1744–1750. doi: 10.1016/j.soilbio.2007.01.030
Moghaddam, S. M., Mamidi, S., Osorno, J. M., Lee, R., Brick, M., Kelly, J., et al. (2016). Genome-wide association study identifies candidate loci underlying agronomic traits in a middle American diversity panel of common bean. Plant Genome 9, 1–21. doi: 10.3835/plantgenome2016.02.0012
Ng, J. L. P., Hassan, S., Truong, T. T., Hocart, C. H., Laffont, C., Frugier, F., et al. (2015). Flavonoids and auxin transport inhibitors rescue symbiotic nodulation in the Medicago truncatula cytokinin perception mutant cre1. Plant Cell 27, 2210–2226. doi: 10.1105/tpc.15.00231
Nodari, R. O., Tsai, S. M., Guzman, P., Gilbertson, R. L., and Gepts, P. (1993). Toward an integrated linkage map of common bean.III. Mapping genetic factors controlling host-bacteria interactions. Genetics 134, 341–350.
Nova-Franco, B., Íñiguez, L. P., Valdés-López, O., Alvarado-Affantranger, X., Leija, A., Fuentes, S. I., et al. (2015). The micro-RNA172c-APETALA2-1 node as a key regulator of the common bean-rhizobium etli nitrogen fixation symbiosis. Plant Physiol. 168, 273–291. doi: 10.1104/pp.114.255547
Oladzad, A., Porch, T., Rosas, J. C., Moghaddam, S. M., Beaver, J., Beebe, S. E., et al. (2019). Single and multi-trait GWAS identify genetic factors associated with production traits in common bean under abiotic stress environments. G3: Genes Genomes, Genetics 9, 1881–1892. doi: 10.1534/g3.119.400072
Peleg-Grossman, S., Volpin, H., and Levine, A. (2007). Root hair curling and rhizobium infection in Medicago truncatula are mediated by phosphatidylinositide-regulated endocytosis and reactive oxygen species. J. Exp. Bot. 58, 1637–1649. doi: 10.1093/jxb/erm013
Pereira, P., Miranda, B., Attewell, J., Kmiecik, K., and Bliss, F. (1993). Selection for increased nodule number in common bean (Phaseolus vulgaris L.). Plant Soil 148, 203–209. doi: 10.1007/BF00012858
Pii, Y., Crimi, M., Cremonese, G., Spena, A., and Pandolfini, T. (2007). Auxin and nitric oxide control indeterminate nodule formation. BMC Plant Biol. 7:21. doi: 10.1186/1471-2229-7-21
Polania, J., Poschenrieder, C., Rao, I., and Beebe, S. (2016). Estimation of phenotypic variability in symbiotic nitrogen fixation ability of common bean under drought stress using 15N natural abundance in grain. Eur. J. Agron. 79, 66–73. doi: 10.1016/j.eja.2016.05.014
Price, A. L., Patterson, N. J., Plenge, R. M., Weinblatt, M. E., Shadick, N. A., and Reich, D. (2006). Principal components analysis corrects for stratification in genome-wide association studies. Nat. Genet. 38, 904–909. doi: 10.1038/ng1847
Purcell, L. C. (2009). Physiological responses of N2 fixation to drought and selecting genotypes for improved N2 fixation. Nitrogen fixation in crop production. 52, 211–238. doi: 10.2134/agronmonogr52.c8
Ramaekers, L., Galeano, C. H., Garzon, N., Vanderleyden, J., and Matthew, W. B. (2013). Identifying quantitative trait loci for symbiotic nitrogen fixation capacity and related traits in common bean. Mol. Breed. 31, 163–180. doi: 10.1007/s11032-012-9780-1
Ren, H., and Gray, W. M. (2015). SAUR proteins as effectors of hormonal and environmental signals in plant growth. Mol. Plant 8, 1153–1164. doi: 10.1016/j.molp.2015.05.003
Schoonhoven, A., and Pastor-Corrales, M. A. (eds.) (1987). Standard system for the evaluation of bean germplasm. Cali, CO: The International Center for Tropical Agriculture (CIAT), 53.
Segonzac, C., Boyer, J. -C., Ipotesi, E., Szponarski, W., Tillard, P., Touraine, B., et al. (2007). Nitrate efflux at the root plasma membrane: identification of an Arabidopsis excretion transporter. Plant Cell 19, 3760–3777. doi: 10.1105/tpc.106.048173
Sun, G., Zhu, C., Kramer, M., Yang, S., Song, W., Piepho, H., et al. (2010). Variation explained in mixed-model association mapping. Heredity 105, 333–340. doi: 10.1038/hdy.2010.11
Unkovich, M., Herridge, D., Peoples, M., Cadisch, G., Boddey, B., Giller, K., et al. (2008). Measuring plant-associated nitrogen fixation in agricultural systems. Canberra, ACT, Australia: Australian Centre for International Agricultural Research (ACIAR).
Van Noorden, G. E., Verbeek, R., Dinh, Q. D., Jin, J., Green, A., Ng, J. L. P., et al. (2016). Molecular signals controlling the inhibition of nodulation by nitrate in Medicago truncatula. Int. J. Mol. Sci. 17:1060. doi: 10.3390/ijms17071060
Wanek, W., and Arndt, S. K. (2002). Difference in δ15N signatures between nodulated roots and shoots of soybean is indicative of the contribution of symbiotic N2 fixation to plant N. J. Exp. Bot. 53, 1109–1118. doi: 10.1093/jexbot/53.371.1109
Wang, X. (2004). Lipid signaling. Curr. Opin. Plant Biol. 7, 329–336. doi: 10.1016/j.pbi.2004.03.012
Wang, Q., Liu, J., and Zhu, H. (2018). Genetic and molecular mechanisms underlying symbiotic specificity in legume-rhizobium interactions. Front. Plant Sci. 9:313. doi: 10.3389/fpls.2018.00313
Wang, H., and Wang, H. (2015). The miR156/SPL module, a regulatory hub and versatile toolbox, gears up crops for enhanced agronomic traits. Mol. Plant 8, 677–688. doi: 10.1016/j.molp.2015.01.008
Yan, Z., Hossain, M. S., Wang, J., Valdés-López, O., Liang, Y., Libault, M., et al. (2013). miR172 regulates soybean nodulation. Mol. Plant-Microbe Interact. 26, 1371–1377. doi: 10.1094/MPMI-04-13-0111-R
Yu, N., Niu, Q. W., Ng, K. H., and Chua, N. H. (2015). The role of miR156/SPL s modules in Arabidopsis lateral root development. Plant J. 83, 673–685. doi: 10.1111/tpj.12919
Zhou, X., and Stephens, M. (2012). Genome-wide efficient mixed-model analysis for association studies. Nat. Genet. 44, 821–824. doi: 10.1038/ng.2310
Zhou, X., and Stephens, M. (2014). Efficient multivariate linear mixed model algorithms for genome-wide association studies. Nat. Methods 11, 407–409. doi: 10.1038/nmeth.2848
Keywords: Phaseolus vulgaris, Rab GTPase, biological nitrogen fixation pathway, NRT1 genes, MATE efflux proteins, genome-wide association study, Aux/IAA family
Citation: Oladzad A, González A, Macchiavelli R, de Jensen CE, Beaver J, Porch T and McClean P (2020) Genetic Factors Associated With Nodulation and Nitrogen Derived From Atmosphere in a Middle American Common Bean Panel. Front. Plant Sci. 11:576078. doi: 10.3389/fpls.2020.576078
Edited by:
Petr Smýkal, Palacký University, CzechiaReviewed by:
Andrés J. Cortés, Colombian Corporation for Agricultural Research (AGROSAVIA), ColombiaPaul Gepts, University of California, United States
Copyright © 2020 Oladzad, González, Macchiavelli, de Jensen, Beaver, Porch and McClean. This is an open-access article distributed under the terms of the Creative Commons Attribution License (CC BY). The use, distribution or reproduction in other forums is permitted, provided the original author(s) and the copyright owner(s) are credited and that the original publication in this journal is cited, in accordance with accepted academic practice. No use, distribution or reproduction is permitted which does not comply with these terms.
*Correspondence: Atena Oladzad, atena.oladzad@ndsu.edu; Phillip McClean, phillip.mcclean@ndsu.edu