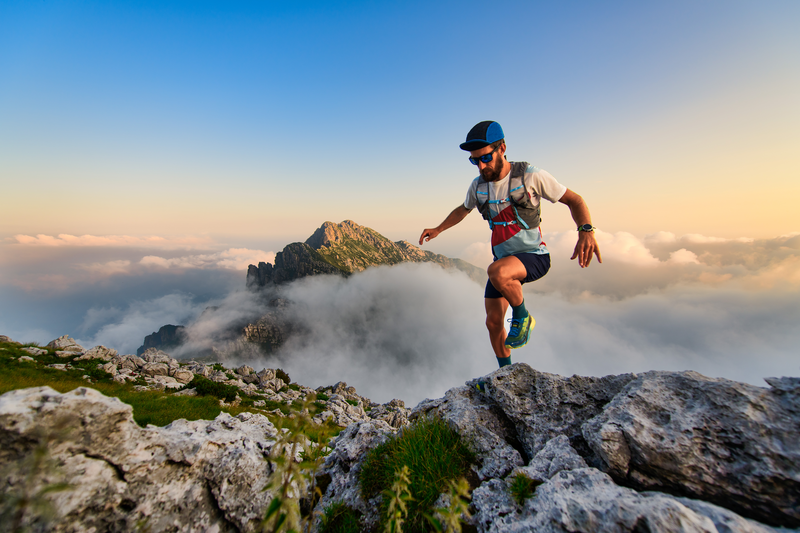
94% of researchers rate our articles as excellent or good
Learn more about the work of our research integrity team to safeguard the quality of each article we publish.
Find out more
PERSPECTIVE article
Front. Plant Sci. , 20 October 2020
Sec. Plant Breeding
Volume 11 - 2020 | https://doi.org/10.3389/fpls.2020.575412
This article is part of the Research Topic Reproductive Barriers and Gene Introgression in Rice Species View all 16 articles
In crosses between genetically divergent parents, traits such as weakness and sterility often segregate in later generations. This hybrid breakdown functions as a reproductive barrier and reduces selection efficiency in crossbreeding. Here, I provide an overview of hybrid breakdown in rice crosses and discuss ways to avoid and mitigate the effects of hybrid breakdown on rice crossbreeding, including genomics-assisted breeding.
Breeders and researchers alike have been interested in hybridization and introgression between divergent genotypes as the potential driver leading to the ecological divergence of progeny (Rieseberg et al., 2007; Arnold et al., 2012). Conversely, hybridization can sometimes involve a reduced hybrid fitness, such as weakness and/or sterility in F1 and later generations, even in cases of hybridization between members of the same species.
Reduced hybrid viability and/or fertility segregating in F2 or later generations are referred to as hybrid breakdown (HB), in which recessive alleles are necessarily associated. This reproductive barrier has been observed for a long time in both plants and animals (Dobzhansky, 1970; Grant, 1971). Many of the genetic analyses of this barrier have revealed that it is accomplished by a complementary effect between and/or among loci with differentiated alleles, commonly called the Bateson–Dobzhansky–Muller (BDM) incompatibility (Rieseberg and Willis, 2007). HB necessarily involves intrinsic postzygotic reproductive barriers, such as hybrid inviability (including weakness, necrosis, and chlorosis) and hybrid sterility (in the male, female, or both gametes); therefore, some researchers may not distinguish HB from inviability and sterility in the F1 progeny. Nevertheless, I believe that HB is a convenient classification for reproductive barriers because it implies their underlying genetic basis (i.e., the involvement of recessive alleles).
Recent studies using the Arabidopsis model plant species have provided a better understanding of HB regarding its molecular mechanisms (Vaid and Laitinen, 2019). Conversely, although rice (Oryza species) is a model crop, the current understanding of the genetic basis of HB remains limited in this species, probably because HB is not a reproductive barrier in F1 hybrids, in which a higher grain production is expected compared with the parental inbred lines, and probably because inferior plants that segregate in F2 and later generations can be easily selected out based on the phenotype from the breeding population. The difficulty in genetic mapping caused by recessive inheritance can also be behind this limitation.
Here, I outline HB in rice crosses while referring to information provided by Arabidopsis studies, and discuss how HB is handled in rice breeding.
It is considered that cultivated rice, O. sativa japonica and indica, forms a species complex with their putative progenitor (O. rufipogon) and wild species; however, multiple reproductive barriers, including HB, are observed in crosses among them (Oka, 1988; Vaughan et al., 2003).
To the best of my knowledge, Dr. Oka was the first to describe the genetic basis of HB in intersubspecific crosses between japonica and indica, which was accounted for by two complementary genes (Oka, 1957). However, the responsible genes were not mapped on chromosomes, because molecular markers were not available at that time.
In rice hybrids, HB has often been described in intersubspecific (O. sativa ssp. japonica × ssp. indica) and interspecific (O. sativa × O. nivara and O. sativa × O. glumaepatula) crosses (Oka, 1957; Sato and Morishima, 1988; Wu et al., 1995; Li et al., 1997; Fukuoka et al., 1998, 2005; Kubo and Yoshimura, 2002, 2005; Matsubara et al., 2007a,b, 2015; Yamamoto et al., 2007; Ichitani et al., 2012;for intersubspecific crosses; Sobrizal et al., 2001; Miura et al., 2008 for interspecific crosses) (Table 1). Most of these HB cases were caused by two-locus BDM incompatibility and recessive alleles, whereby 1/16 of the F2 progeny that was homozygous for recessive alleles at both loci showed the HB phenotype; however, 4/16 of the progeny that was heterozygous at only one locus showed the HB phenotype, depending on the cross combination (Table 1 and Figure 1A). In the backcross hybrids, one locus was already fixed with the alleles from the recurrent parent; therefore, 1/4 of the BCnF2 progeny exhibited the HB phenotype (Table 1 and Figure 1B).
Figure 1. The genetic basis of hybrid breakdown (HB). (A) Schematic representation of the HB genotype that segregates in the F2 progeny. When the parental genotypes are AAbb and aaBB, 1/16 of the F2 progeny without dominant allele, which is indicated by gray character (i.e., aabb), show reduced viability and/or fertility, although the other genotypes are normal. In some rice crosses, the F2 progeny with only one dominant allele (i.e., Aabb, aaBb) also show reduced viability and/or fertility. (B) Schematic representation of the HB genotype that segregates in the BCnF2 progeny. Further, 1/4 of the BCnF2 progeny shows reduced viability and/or fertility. (C) Chromosomal location of genes underlying HB in rice crosses. The genes are roughly mapped based on the results of gene mapping in each study. A set of complementary genes are connected by the dotted line. Complementary genes with hbd1 and hwf1 have not been reported. The example of the functional nucleotide polymorphism causing HB published by Yamamoto et al. (2010) is shown, and this information will allow us to conduct a survey by SNP array or amplicon sequencing.
The HB phenotype in rice hybrids varies according to the cross combination. For example, the hbd2/hbd2 hbd3/hbd3 genotype shows weakness, but no obvious seed sterility. However, the hbd4/hbd4 hbd5/hbd5 genotype exhibits both weakness and seed sterility (Matsubara et al., 2007a, 2015; Yamamoto et al., 2007; Table 1). There are also complicated cases in which HB showing both weakness and seed sterility are observed (hwe1/hwe1 hwe2/hwe2 genotype) or HB showing only seed sterility (hsa1/hsa1 hsa2/hsa2 hsa3/hsa3) is found, despite the same cross combination, i.e., Asominori × IR24 cross (Kubo and Yoshimura, 2002, 2005; Table 1). As these HBs have independent genetic bases, at least 5/64 of F2 progeny would show the HB phenotype in this cross.
To date, several sets of loci responsible for HB have been mapped to particular genomic regions using DNA markers in crosses between the japonica and indica rice varieties (Chrs 7 and 10, Fukuoka et al., 1998; Chrs 1 and 12, Wu et al., 1995; Kubo and Yoshimura, 2002; Matsubara et al., 2015; Chrs 6 and 11, Fukuoka et al., 2005; Chrs 8, 9, and 12, Kubo and Yoshimura, 2005; Chrs 2 and 11, Matsubara et al., 2007a; Yamamoto et al., 2007, 2010; Chrs 11 and 12, Ichitani et al., 2012; Table 1 and Figure 1C). The results of these studies revealed that loci underlying rice HB are shared in some crosses, but differ in other crosses, which is suggestive of their diversification in rice genomes. For example, in some cases the HB allele carried by O. nivara, a species related closely to incica varieties, share the same locus with an indica variety; however, the HB allele carried by O. glumaepatula, a species closely related to cultivated varieties, does not share the locus with any other variety (Table 1 and Figure 1C). It should be noted that the HB cases described above are caused by a set of genes with major effects; however, there are also cases caused by a set of genes with minor effects, e.g., slightly reduced seed fertility, which cannot be overlooked in rice breeding, that have not yet been detected.
In Arabidopsis hybrids, several molecular mechanisms underlying the BDM type of HB have been experimentally demonstrated, e.g., autoimmune response (Bomblies et al., 2007; Alcázar et al., 2009) and reciprocal silencing of duplicated genes (Bikard et al., 2009; Vlad et al., 2010; Agorio et al., 2017; Blevins et al., 2017).
Autoimmune response: Bomblies et al. (2007) reported first that the autoimmune response that an NB-LRR disease-resistance gene or R gene is associated with Arabidopsis HB in intraspecific crosses, although this autoimmune response is mainly expressed as necrosis in F1 plants, and HB in F2 progeny seemed to be conditioned by temperature. In Arabidopsis hybrids, several lines of evidence of HB caused by the autoimmune response have been described (Alcázar et al., 2009, 2010). The involvement of NB-LRR genes in HB suggests that multiple genomic regions can be associated with the Arabidopsis HB; in fact, the extensive survey carried out by Chae et al. (2014) supports this idea.
Also in rice hybrids, HB caused by an autoimmune response has been reported in a japonica × indica cross (Yamamoto et al., 2010). In this case, HB occurs in F2 plants when the hybrid breakdown 2 (hbd2) gene, which encodes casein kinase I and is carried by the indica variety is combined with a cluster of R genes carried by the japonica variety. As these causative alleles are likely to behave as partially recessive ones, the gene products can be involved (Matsubara et al., 2007a; Yamamoto et al., 2007, 2010).
Reciprocal silencing of duplicated genes: this notion was first theoretically proposed as a genetic basis for hybrid incompatibility (Werth and Windham, 1991; Lynch and Force, 2000). In the model, one population loses function at one locus and retains it at the other, whereas the other population experiences the opposite effect. Consequently, 1/16 of the F2 zygotes do not have functional genes (Lynch and Force, 2000). The HB in Arabidopsis hybrids described by Bikard et al. (2009) and Vlad et al. (2010) is a good example of this phenomenon. In this scenario, cases involving epigenetic silencing have also been reported (Agorio et al., 2017; Blevins et al., 2017).
As rice has experienced both whole-genome and segmental duplication (Wang et al., 2007; Guo et al., 2019), reciprocal silencing could be prevalent as a cause of hybrid incompatibility. However, to date, there is no evidence of HB caused by reciprocal silencing of duplicated genes in rice.
Independent of the autoimmune response and reciprocal silencing, an interaction between a DEAD-box RNA helicase 18 and another gene (MORPHEUS MOLECULE 1 as a likely candidate) that cause HB in Arabidopsis hybrids has been identified (Plötner et al., 2017; Vaid et al., 2020). Interestingly, the HB phenotype reduces between the F3 and F4 generations, implying epigenetic regulation of gene expression.
In rice hybrids, among the three hybrid sterility-a (hsa) loci, Kubo et al. (2016) recently showed that the hsa1 locus consists of two genes, and that these genes encode a DUF1618 protein and an uncharacterized protein with some similarity to a nucleotide-binding protein, respectively. The molecular features of the remaining complementary genes, hsa2 and hsa3, have not been reported.
For decades, the bulk-population method was widely employed in conventional rice breeding. In this method, after crossing, the F4 or F5 population is raised by self-fertilization in bulk (rather than by the single-seed descent method) without artificial selection, but the early generation population is subjected to natural and viability selection (Allard, 1960; Ikehashi and Fujimaki, 1980 for details). In the bulk-population method, many weak and/or sterile genotypes can be expected to be eliminated from the population before the establishment of an advanced-generation population; therefore, this method may provide limited information about HB.
For the introgression or accumulation of desirable traits from donor(s) to a variety, rice breeders have often performed backcrossing or multiple parental crossing, followed by the bulk-population method. Empirically, it has long been known that these crossing methods allow the mitigation of the loss of selection candidates by reproductive barriers, because these methods often reduce the segregation of disruptive combinations of alleles associated with HB in a hybrid progeny. However, the mitigation of reproductive barriers by these crossing methods is inevitably dependent on the HB genotype of the parents.
The publication of reference crop genome sequences and the development of next-generation sequencing technologies have accelerated the progress of the molecular breeding of crops (Kole et al., 2015). In this context, genomics-assisted breeding, such as genomic selection based on genotypes of genome-wide DNA markers, has been considered in crop breeding (Spindel and Iwata, 2018).
In genomics-assisted breeding of self-pollinated crops, advanced-generation populations, such as recombinant inbred lines, are often used as reference populations, from which genome-wide genotype and phenotype data are obtained. Subsequently, selection based only on marker genotypes is carried out in the progeny of early generations (e.g., F2). In this selection scheme, it should be noted that the reference population does not usually provide the information of genomic region for HB, because weak and/or sterile progeny should have been eliminated in the early generations after crossing. Therefore, if such selection scheme is adopted, we will have to abandon some important selection candidates. Alternatively, we may select undesirable candidates such that HB becomes apparent in later generations.
Despite the limited number of studies on this subject, it seems that the loci underlying HB in rice crosses are diversified rather than shared (Table 1 and Figure 1). As described above, the information on the distribution of HB-associated alleles among cross parents should be a prerequisite for rice breeding, particularly for maximizing the effectiveness of genomics-assisted breeding. Therefore, the additional detection of HB in rice crosses and the mapping of responsible genes in the rice genome are needed. In Arabidopsis hybrids, such data about the R genes and their interacting genes has been extensively surveyed (Alcázar et al., 2010, 2014; Chae et al., 2014). The reporting of the hbd2 gene by Yamamoto et al. (2010) in a rice hybrid is a good example of an extensive survey performed using SNP arrays or amplicon sequencing (Figure 1C). The hbd3 and hsa1 genes are also candidates for this type of survey. Eventually, the development of criteria that allow us to predict HB based on the genomic information of the parental lines will serve as an important tool for genomics-assisted breeding. Even if a causal factor has not been identified as a single gene, closely linked markers, such as single-nucleotide polymorphisms, will be effective for classifying HB-associated haplotypes, because the linkage disequilibrium of cultivated rice has been estimated to extend to 100–200 kb, although that of wild species (such as O. rufipogon) may extend over several tens of kb (Mather et al., 2007; McNally et al., 2009; Huang et al., 2010). This information will enable the design of a more efficient and effective cross combination.
Bulked segregant analysis followed by next-generation sequencing can be useful for the mapping of HB-associated loci, as well as conventional linkage mapping (such as quantitative trait locus analysis), because HB segregants often show distinguishable features (about traits such as plant height, tiller number, and fertility) from normal growth segregants in each cross population. The acquisition of imaging data using a digital camera and drone loading may also play an important role in phenotyping in the laboratory and the paddy field. These efforts will provide valuable information not only to rice breeders, but also to evolutionary biologists.
Furthermore, if an HB-associated allele is identified, gene disruption through ion beam or genome editing may help overcome HB, as exemplified by F1 hybrid sterility in rice (Koide et al., 2018; Xie et al., 2019).
All datasets presented in this study are included in the article/supplementary material.
The author confirms being the sole contributor of this work and has approved it for publication.
The authors declare that the research was conducted in the absence of any commercial or financial relationships that could be construed as a potential conflict of interest.
I would like to dedicate this paper to the memory of Dr. Darshan Brar. He was an eminent rice breeder who vigorously introduced useful genes carried by wild species into cultivars beyond reproductive barriers.
Agorio, A., Durand, S., Fiume, E., Brousse, C., Gy, I., Simon, M., et al. (2017). An Arabidopsis natural epiallele maintained by a feed-forward silencing loop between histone and DNA. PLoS Genet. 13:e1006551. doi: 10.1371/journal.pgen.1006551
Alcázar, R., García, A. V., Kronholm, I., de Meaux, J., Koornneef, M., Parker, J. E., et al. (2010). Natural variation at strubbelig receptor kinase 3 drives immune-triggered incompatibilities between Arabidopsis thaliana accessions. Nat. Genet. 42, 1135–1139. doi: 10.1038/ng.704
Alcázar, R., García, A. V., Parker, J. E., and Reymond, M. (2009). Incremental steps toward incompatibility revealed by Arabidopsis epistatic interactions modulating salicylic acid pathway activation. Proc. Natl. Acad. Sci. U.S.A. 106, 334–339. doi: 10.1073/pnas.0811734106
Alcázar, R., von Reth, M., Bautor, J., Chae, E., Weigel, D., Koornneef, M., et al. (2014). Analysis of a plant complex resistance gene locus underlying immune-related hybrid incompatibility and its occurrence in nature. PLoS Genet. 10:e1004848. doi: 10.1371/journal.pgen.1004848
Arnold, M. L., Ballerini, E. S., and Brothers, A. N. (2012). Hybrid fitness, adaptation and evolutionary diversification: lessons learned from Louisiana Irises. Heredity 108, 159–166. doi: 10.1038/hdy.2011.65
Bikard, D., Patel, D., Le Metté, C., Giorgi, V., Camilleri, C., Bennett, M. J., et al. (2009). Divergent evolution of duplicate genes leads to genetic incompatibilities within A. thaliana. Science 323, 623–626. doi: 10.1126/science.1165917
Blevins, T., Wang, J., Pflieger, D., Pontvianne, F., and Pikaard, C. S. (2017). Hybrid incompatibility caused by an epiallele. Proc. Natl. Acad. Sci. U.S.A. 114, 3702–3707. doi: 10.1073/pnas.1700368114
Bomblies, K., Lempe, J., Epple, P., Warthmann, N., Lanz, C., Dangl, J. L., et al. (2007). Autoimmune response as a mechanism for a Dobzhansky-Muller-type incompatibility syndrome in plants. PLoS Biol. 5:e236. doi: 10.1371/journal.pbio.0050236
Chae, E., Bomblies, K., Kim, S. T., Karelina, D., Zaidem, M., Ossowski, S., et al. (2014). Species-wide genetic incompatibility analysis identifies immune genes as hot spots of deleterious epistasis. Cell 159, 1341–1351. doi: 10.1016/j.cell.2014.10.049
Dobzhansky, T. (1970). Genetics of the Evolutionary Process. New York, NY: Columbia University Press.
Fukuoka, S., Namai, H., and Okuno, K. (1998). RFLP mapping of the genes controlling hybrid breakdown in rice (Oryza sativa L.). Theor. Appl. Genet. 97, 446–449. doi: 10.1007/s001220050915
Fukuoka, S., Newingham, M. C. V., Ishtiaq, M., Nagamine, T., Kawase, M., and Okuno, K. (2005). Identification and mapping of two new loci for hybrid breakdown in cultivated rice. Rice Genet. Newslett. 22, 29–31.
Guo, H., Jiao, Y., Tan, X., Wang, X., Huang, X., Jin, H., et al. (2019). Gene duplication and genetic innovation in cereal genomes. Genome Res. 29, 261–269. doi: 10.1101/gr.237511.118
Huang, X., Wei, X., Sang, T., Zhao, Q., Feng, Q., Zhao, Y., et al. (2010). Genome-wide association studies of 14 agronomic traits in rice landraces. Nat. Genet. 42, 961–967. doi: 10.1038/ng.695
Ichitani, K., Takemoto, Y., Iiyama, K., Taura, S., and Sato, M. (2012). Chromosomal location of HCA1 and HCA2, hybrid chlorosis genes in rice. Int. J. Plant Genomics 2012:649081. doi: 10.1155/2012/649081
Ikehashi, H., and Fujimaki, H. (1980). “Modified bulk population method for rice breeding,” in Proceedings of the International Rice Research Conference on Innovative approaches to rice breeding, (Los Baños, PA: International Rice Research Institute), 163–182.
Koide, Y., Ogino, A., Yoshikawa, T., Kitashima, Y., Saito, N., Kanaoka, Y., et al. (2018). Lineage-specific gene acquisition or loss is involved in interspecific hybrid sterility in rice. Proc. Natl. Acad. Sci. U.S.A. 115, E1995–E1962. doi: 10.1073/pnas.1711656115
Kole, C., Muthamilarasan, M., Henry, R., Edwards, D., Sharma, R., Abberton, M., et al. (2015). Application of genomics-assisted breeding for generation of climate resilient crops: progress and prospects. Front. Plant Sci. 6:563. doi: 10.3389/fpls.2015.00563
Kubo, T., Takashi, T., Ashikari, M., Yoshimura, A., and Kurata, N. (2016). Two tightly linked genes at the hsa1 locus cause both F1 and F2 hybrid sterility in rice. Mol. Plant 9, 221–232. doi: 10.1016/j.molp.2015.09.014
Kubo, T., and Yoshimura, A. (2002). Genetic basis of hybrid breakdown in a Japonica/Indica cross of rice, Oryza sativa L. Theor. Appl. Genet. 105, 906–911. doi: 10.1007/s00122-002-1059-1
Kubo, T., and Yoshimura, A. (2005). Epistasis underlying female sterility detected in hybrid breakdown in a Japonica-Indica cross of rice (Oryza sativa L.). Theor. Appl. Genet. 110, 346–355. doi: 10.1007/s00122-004-1846-y
Li, Z., Pinson, S. R. M., Paterson, A. H., Park, W. D., and Stansel, J. W. (1997). Genetics of hybrid sterility and hybrid breakdown in an intersubspecific rice (Oryza sativa L.) population. Genetics 145, 1139–1148. doi: 10.1142/9789812814289_0039
Lynch, M., and Force, A. G. (2000). The origin of interspecific genomic incompatibility via gene duplication. Am. Nat. 156, 590–605. doi: 10.1086/316992
Mather, K. A., Caicedo, A. L., Polato, N. R., Olsen, K. M., McCouch, S., Purugganan, M. D., et al. (2007). The extent of linkage disequilibrium in rice (Oryza sativa L.). Genetics 177, 2223–2232. doi: 10.1534/genetics.107.079616
Matsubara, K., Ando, T., Mizubayashi, T., Ito, S., and Yano, M. (2007a). Identification and linkage mapping of complementary recessive genes causing hybrid breakdown in an intraspecific rice cross. Theor. Appl. Genet. 115, 179–186. doi: 10.1007/s00122-007-0553-x
Matsubara, K., Ito, S., Nonoue, Y., Ando, T., and Yano, M. (2007b). A novel gene responsible for hybrid breakdown found in a cross between japonica and indica cultivars in rice. Rice Genet. Newslett. 23, 11–13.
Matsubara, K., Yamamoto, E., Mizobuchi, R., Yonemaru, J.-I., Yamamoto, T., Kato, H., et al. (2015). Hybrid breakdown caused by epistasis-based recessive incompatibility in a cross of rice (Oryza sativa L.). J. Hered. 106, 113–122. doi: 10.1093/jhered/esu065
McNally, K. L., Childs, K. L., Bohnert, R., Davidson, R. M., Zhao, K., Ulat, V. J., et al. (2009). Genomewide SNP variation reveals relationships among landraces and modern varieties of rice. Proc. Natl. Acad. Sci. U.S.A. 106, 12273–12278. doi: 10.1073/pnas.0900992106
Miura, K., Yamamoto, E., Morinaka, Y., Takashi, T., Kitano, H., Matsuoka, M., et al. (2008). The hybrid breakdown 1(t) locus induces interspecific hybrid breakdown between rice Oryza sativa cv. Koshihikari and its wild relative O. nivara. Breed. Sci. 58, 99–105. doi: 10.1270/jsbbs.58.99
Oka, H.-I. (1957). Phylogenetic differentiation lethal of cultivated rice. XV. Complementary lethal genes in rice. Jpn. J. Genet. 32, 83–87. doi: 10.1266/jjg.32.83
Plötner, B., Nurmi, M., Fischer, A., Watanabe, M., Schneeberger, K., Holm, S., et al. (2017). Chlorosis caused by two recessively interacting genes reveals a role of RNA helicase in hybrid breakdown in Arabidopsis thaliana. Plant J. 91, 251–262. doi: 10.1111/tpj.13560
Rieseberg, L. H., Kim, S. C., Randell, R. A., Whitney, K. D., Gross, B. L., Lexer, C., et al. (2007). Hybridization and the colonization of novel habitats by annual sunflowers. Genetica 129, 149–165. doi: 10.1007/s10709-006-9011-y
Rieseberg, L. H., and Willis, J. H. (2007). Plant speciation. Science 317, 910–914. doi: 10.1126/science.1137729
Sato, Y. I., and Morishima, H. (1988). Distribution of the genes causing F2 chlorosis in rice cultivars of the Indica and Japonica types. Theor. Appl. Genet. 75, 723–727. doi: 10.1007/BF00265594
Sobrizal, T. C., Yasui, H., and Yoshimura, A. (2001). Mapping of a gene for F2 weakness on chromosome 4 of rice. Rice Genet. Newslett. 18, 61–62.
Spindel, J., and Iwata, H. (2018). “Genomic selection in rice breeding,” in Rice Genomics, Genetics and Breeding, eds T. Sasaki and M. Ashikari (Singapore: Springer).
Vaid, N., Ishihara, H., Plötner, B., Sageman-Furnas, K., Wiszniewski, A., and Laitinen, R. A. E. (2020). Leaf chlorosis in Arabidopsis thaliana hybrids is associated with transgenerational decline and imbalanced ribosome number. New Phytol. [Epub ahead of print] doi: 10.1111/nph.16752
Vaid, N., and Laitinen, R. A. E. (2019). Diverse paths to hybrid incompatibility in Arabidopsis. Plant J. 97, 199–213. doi: 10.1111/tpj.14061
Vaughan, D. A., Morishima, H., and Kadowaki, K. (2003). Diversity in the Oryza genus. Curr. Opin. Plant Biol. 6, 139–146. doi: 10.1016/S1369-5266(03)00009-8
Vlad, D., Rappaport, F., Simon, M., and Loudet, O. (2010). Gene transposition causing natural variation for growth in Arabidopsis thaliana. PLoS Genet. 6:21. doi: 10.1371/journal.pgen.1000945
Wang, X., Tang, H., Bowers, J. E., Feltus, F. A., and Paterson, A. H. (2007). Extensive concerted evolution of rice paralogs and the road to regaining independence. Genetics 177, 1753–1763. doi: 10.1534/genetics.107.073197
Werth, C. R., and Windham, M. D. (1991). A model for divergent, allopatric speciation of polyploid pteridophytes resulting from silencing of duplicate-gene expression. Am. Nat. 137, 515–526. doi: 10.1086/285180
Wu, P., Zhang, G., Huang, N., and Ladha, J. K. (1995). Non-allelic interaction conditioning spikelet sterility in an F2 population of indica/japonica cross in rice. Theor. Appl. Genet. 91, 825–829. doi: 10.1007/BF00223887
Xie, Y., Tang, J., Xie, X., Li, X., Huang, J., Fei, Y., et al. (2019). An asymmetric allelic interaction drives allele transmission bias in interspecific rice hybrids. Nat. Commun. 10:2501. doi: 10.1038/s41467-019-10488-3
Yamamoto, E., Takashi, T., Morinaka, Y., Lin, S., Kitano, H., Matsuoka, M., et al. (2007). Interaction of two recessive genes, hbd2 and hbd3, induces hybrid breakdown in rice. Theor. Appl. Genet. 115, 187–194. doi: 10.1007/s00122-007-0554-9
Keywords: genomics-assisted breeding, Oryza, reproductive barrier, sterility, weakness
Citation: Matsubara K (2020) How Hybrid Breakdown Can Be Handled in Rice Crossbreeding? Front. Plant Sci. 11:575412. doi: 10.3389/fpls.2020.575412
Received: 23 June 2020; Accepted: 30 September 2020;
Published: 20 October 2020.
Edited by:
Ryo Fujimoto, Kobe University, JapanReviewed by:
Ling Jiang, Nanjing Agricultural University, ChinaCopyright © 2020 Matsubara. This is an open-access article distributed under the terms of the Creative Commons Attribution License (CC BY). The use, distribution or reproduction in other forums is permitted, provided the original author(s) and the copyright owner(s) are credited and that the original publication in this journal is cited, in accordance with accepted academic practice. No use, distribution or reproduction is permitted which does not comply with these terms.
*Correspondence: Kazuki Matsubara, dmFndWVAYWZmcmMuZ28uanA=
Disclaimer: All claims expressed in this article are solely those of the authors and do not necessarily represent those of their affiliated organizations, or those of the publisher, the editors and the reviewers. Any product that may be evaluated in this article or claim that may be made by its manufacturer is not guaranteed or endorsed by the publisher.
Research integrity at Frontiers
Learn more about the work of our research integrity team to safeguard the quality of each article we publish.