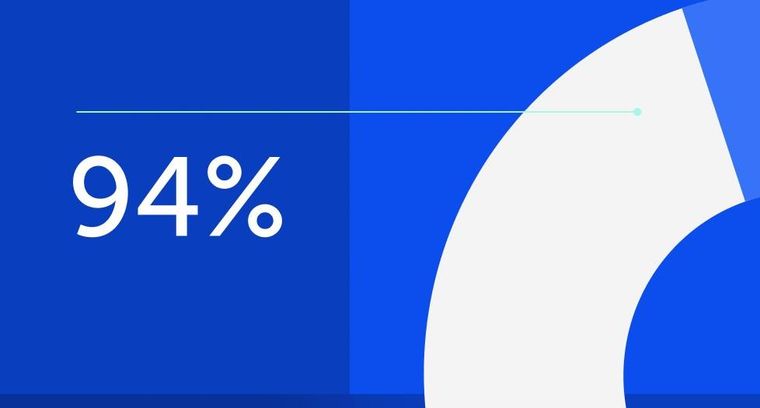
94% of researchers rate our articles as excellent or good
Learn more about the work of our research integrity team to safeguard the quality of each article we publish.
Find out more
ORIGINAL RESEARCH article
Front. Plant Sci., 11 September 2020
Sec. Plant Development and EvoDevo
Volume 11 - 2020 | https://doi.org/10.3389/fpls.2020.564665
MYB transcription factors are involved in many biological processes, including metabolism, stress response and plant development. In our previous work, ZmMYB59 was down-regulated by deep sowing during maize seed germination. However, there are few reports on seed germination regulated by MYB proteins. In this study, to examine its functions during seed germination, Agrobacterium-mediated transformation was exploited to generate ZmMYB59 overexpression (OE) tobacco and rice. In T2 generation transgenic tobacco, germination rate, germination index, vigor index and hypocotyl length were significantly decreased by 25.0–50.9, 34.5–54.4, 57.5–88.3, and 21.9–31.3% compared to wild-type (WT) lines. In T2 generation transgenic rice, above corresponding parameters were notably reduced by 39.1–53.8, 51.4–71.4, 52.5–74.0, and 28.3–41.5%, respectively. On this basis, antioxidant capacity and endogenous hormones were determined. The activities of catalase, peroxidase, superoxide dismutase, ascorbate peroxidase of OE lines were significantly lower than those of WT, suggesting that ZmMYB59 reduced their oxidation resistance. As well, ZmMYB59 overexpression extremely inhibited the synthesis of gibberellin A1 (GA1) and cytokinin (CTK), and promoted the synthesis of abscisic acid (ABA) concurrently. Taken together, it proposed that ZmMYB59 was a negative regulator during seed germination in tobacco and rice, which also contributes to illuminate the molecular mechanisms regulated by MYB transcription factors.
Seed germination is a crucial stage in plant development (Mazer, 1999), in which three progress were included, a phase of fast absorbing water, a dynamic equilibrium of water potential and a stage of rapid combination water for radical elongation (Narbona et al., 2013; Xie et al., 2014; Gu et al., 2016). For most plants, the germination stages are the most delicate to biotic and abiotic stresses. Abiotic stress could cause the accumulation of Reactive oxygen species (ROS), which might initiate destructive oxidative processes, such as lipid peroxidation (inflected by MDA content), chlorophyll and protein oxidation (Sarker and Oba, 2018). ROS with low-concentration can break dormitory to promote seed germination, high-concentration will inhibit germination and activate plant antioxidant system (Bailly et al., 2008; El-Maarouf-Bouteau and Bailly, 2008). ROS scavenging system is an essential mechanism to delay senescence, including superoxide dismutase (SOD), peroxidase (POD), catalase (CAT), ascorbic acid peroxidase (APX) (Foyer et al., 1994). SOD catalyzes the dismutation of O2-, CAT, POD and APX mainly scavenge H2O2 (Mittler et al., 2004).
Previously, there were a few reports on transcription factors regulating plant growth (Riechmann et al., 2000). Nonetheless, the contributions of MYB transcription factors during seed germination have not yet been functionally characterized. MYB is the largest transcription factor family in plants, which is widely distributed in both monocotyledons and dicotyledons (Dubos et al., 2010; Feller et al., 2011). The C-terminal region of MYBs alters strikingly, thereby allowing MYB superfamily to perform a considerable assortment of structures and functions (Stracke et al., 2001; Kim et al., 2015). Depending on the number of conserved motifs, the superfamily is divided into four classes: R1-MYB, R2R3-MYB, R1R2R3-MYB, and 4R-MYB (Liu et al., 2019). The functions of MYB proteins have been probed in plentiful plant species such as Arabidopsis, maize, rice, petunia, snapdragon, grapevine, poplar, and apple (Dubos et al., 2010), involving the regulation of cell differentiation, plant development, organ morphogenesis, hormone response, stress tolerance, secondary metabolism (Du et al., 2012; Pu et al., 2020).
In Arabidopsis, AtMYB7 was found to positively regulate seed germination by blocking the expression of ABI5, which was a crucial transcription factor involved in ABA mediated germination inhibition (Kim et al., 2015). RSM1, an Arabidopsis MYB protein could modulate seed germination in response to ABA and salinity (Yang et al., 2018). LcMYB2 increased root growth to enhance drought tolerance during seed germination (Zhao et al., 2019). However, there are relatively few reports on seed germination regulated by MYB proteins and the explicit mechanisms remain unidentified.
In our previous study, a new MYB gene named ZmMYB59, was cloned from the B73 inbred line. Real-Time PCR showed the expression of ZmMYB59 in maize mesocotyl was down-regulated by deep sowing and exogenous GA during seed germination (Du et al., 2017). In this study, ZmMYB59 overexpression tobacco and rice were produced by genetic transformation. Afterwards, germination experiment, antioxidant capacity, cellular morphology, and endogenous hormone content were measured. The objective of this study was to further investigate the functions of ZmMYB59 during seed germination in ZmMYB59 exogenous expressed tobacco and rice, which will also contribute to elucidate the regulatory mechanisms by MYB transcription factors affecting seed germination.
T2 generation ZmMYB59 transgenic seeds of tobacco (Nicotiana tabacum L.), rice (Oryza sativa L. ssp. Japonica) were provided by Hangzhou Biogle Co., Ltd by Agrobacterium-mediated transformation. The embryogenic callus from wild-type plants was inoculated with agrobacterium tumefaciens strain EHA105 and expression vector plasmid pCAMBIA3301-Bar-ZmMYB59 to generate ZmMYB59 transgenic tobacco, rice. In each crop, three independent ZmMYB59 transgenic lines were used in this study.
Genomic DNA was isolated using CTAB method (Porebski et al., 1997). Total RNA was extracted using All-in-one DNA/RNA Mini-preps kit (B618203, Sangon Biotech, shanghai, China) according to the manufacturer’s instructions. CDNA (20 µl) was synthesized from 1,000 ng of total RNA using PrimerScript TM RT regent Kit with gDNA Eraser (RR047, Takara, Beijing, China). qRT-PCR was performed in the CFX Connect ™ Real-Time System (BIO-RAD, Singapore) using TB Green Premix Ex Taq II (Tli RNaseH Plus) (RR820A/B, Takara). Each PCR mixture (20 µl) contained 5 µl of diluted cDNA (about 250 ng), 10 µl of 2 × TB Green Premix Ex Taq II, 1 µl forward primer, 1 µl reverse primer, 1.5 µl DMSO, and 1.5 µl ddH2O. All reactions were performed in three replications and the 2 -△△Ct method was used to calculate relative expression values. Primers were designed by Primer Premier 5.0 software and showed in Table 1.
Germination experiment was conducted on three replicates and twenty seeds for each replicate. Wild-type and ZmMYB59 overexpressing seeds were evenly floored in the germination boxes. Culture conditions were set up at 20°C dark for tobacco and 25°C dark for rice. The number of germinated seeds was recorded from day 2 to 14 days. Germination rate, germination index, vigor index were determined according to following formulas:
Germination rate = n1 within 14 days/n2 × 100%. Here n1 is the number of germinated seeds; n2 is the number of tested seeds.
Vigor index = ∑ (Gt/Dt) × S. Here Gt is corresponding number of seeds germinated in the t day; Dt is time corresponding to Gt in days; S is the average length of 10 seedlings.
After incubation for 14 days, the seedlings of ZmMYB59 overexpressed tobacco or rice and their WT lines were used for extraction of genomic DNA, total RNA, and determination of antioxidant capacity, hormones content. There were three replicates for each index, and 8 tobacco seedlings or 12 rice seedlings for each replicate.
Malondialdehyde (MDA) content was measured by using 2-thiobarbituric acid (TBA) (Sarker and Oba, 2018) with slight modifications. Fresh seedlings 0.5 g were ground with 5 ml 0.6% TBA in 10% trichloroacetic acid. The mixture was heated at 100°C for 15 min and then cooled in ice bath. Finally, the OD values at 450, 532, and 600 nm were recorded. computational formula was as follows: µmol MDA g−1 fresh weight (FW) = 6.45 (OD532 − OD600) − 0.56 OD450.
The activities of catalase (CAT), peroxidase (POD), superoxide dismutase (SOD), ascorbate peroxidase (APX) were measured by employing 0.5 g seedlings in 5 ml extraction buffer containing 0.05 M phosphate buffer (Li et al., 2018). CAT was determined spectrophotometrically based on the decrease in absorbance of H2O2 at 240 nm. POD was measured as the absorbance at 470 nm. SOD was assayed by measuring the ability of the enzyme extract to inhibit the photochemical reduction of nitroblue tetrazolium (NBT). APX was assayed from the decrease in absorbance at 290 nm (Yoshimura et al., 2000).
After 14 days of incubation, hypocotyl in tobacco/mesocotyl in rice both wild-type and OE seedlings was cut longitudinally, and the cell sections were made to determine the changes of cell length and cell number. The middle position of hypocotyl/mesocotyl was cut and fixed with 25% glutaraldehyde stationary solution initially. Five visual fields were randomly selected for observation of cell morphology. Cell length was measured by calibrated eyepiece and cell number was counted by photographing.
The concentration of the endogenous phytohormones involving gibberellin A1 (GA1), gibberellin A3 (GA3), gibberellins A4 (GA4), cytokinin (CTK), abscisic acid (ABA), indole-3-acetic acid (IAA) during seed germination was determined by LC-MS/MS system (Qin et al., 2019; Wang et al., 2019) with slight modifications. Each sample was transferred into a 2 mL LC/MS glass vial for LC-MS/MS analysis. MS system: ion spray voltage -4,500 V, temperature 550°C, Ion source Gas 1:50/Gas 2:50. Chromatographic system: HSS T3 liquid chromatography column (100 ≤ 2.1 mm, 1.8 µm), mobile phase A (0.1% formic acid-aqueous solution), mobile phase B (0.1% formic acid-acetonitrile). Multiple reaction monitoring detection method was used for the quantification of all analytes. Each sample was extracted three times.
Analysis of variance (ANOVA) was carried out with SPSS 19.0 (IBM SPSS Statistics, Chicago, USA). Duncan’s multiple range test or Student’s t test was employed to determine if there were significant differences between the determination indicators of transgenic and wild-type lines at p < 0.05.
The expression vector pCAMBIA3301-Bar-ZmMYB59 construct was transferred into immature embryos to gain ZmMYB59 overexpression tobacco and rice. The regeneration of somatic embryos and their conversion into plants was attempted to each transgenic line. In this study, transgenic plants were obtained through the following procedure: callus induction, subculture, Agrobacterium transformation, co-cultivation, resistance screening, differentiation, rooting and transplantation. T0 generation transgenic plants were continually self-pollinated until T2 generation. T2 generation transgenic tobacco and rice were used for genotype identification by PCR amplification, wild-type lines was as negative control, vector pCAMBIA3301-Bar-ZmMYB59 was as positive control. In tobacco, the result of electrophoresis showed that 18 of 22 transgenic lines were consistent with the positive control (Additional file: Figure S1). In rice, the results suggested that 16 of 19 lines were successfully transformed (Additional file: Figure S2). During all electrophoretic bands, three overexpression lines were selected as materials in this study (OE1, OE2, OE3 in tobacco, OE2, OE4, OE6 in rice), which was showed in Figures 1A, B. Additionally, semi-quantitative RT-PCR and qRT-PCR were used for detecting the ZmMYB59 gene expression level in three overexpression lines. The results showed that ZmMYB59 expression in OE1, OE2, OE3 in transgenic tobacco was increased by 25.68, 31.84, and 20.51-fold compared to the wild-type, respectively (Figures 2A, B). In transgenic rice, compared to the wild-type ZmMYB59 expression in OE2, OE4, OE6 was enhanced by 3.64, 2.99, and 4.98, respectively (Figures 2D, E).
Figure 1 Electrophoretic results to confirm the presence of the ZmMYB59 gene in T2 generation transgenic tobacco and rice in genomic level. (A) T2 generation transgenic tobacco. M: DL10000 DNA Marker, 1: positive control, 2: negative control, OE1~OE3: ZmMYB59 transgenic tobacco lines. (B) T2 generation transgenic rice. M: DL2000 DNA Marker, 1: negative control, 2: positive control, OE2~OE6: ZmMYB59 transgenic rice lines. Seedlings of 14 day-old were used for DNA extraction.
Figure 2 ZmMYB59 inhibited seedling growth in tobacco and rice. (A, B) The expression of ZmMYB59 overexpressed tobacco was detected by semi-quantitative RT-PCR (A) or real time PCR (B). RNA was extracted from T2 generation transgenic tobacco. 1: WT; 2: vector used for transformation; OE-1, 2, 3: different lines of ZmMYB59 overexpressed tobacco. The expression of Ntactin7 was used as internal control. Values are means ± SD; n = 3. ***P < 0.001 (Student’s t-test). (C) Phenotype of ZmMYB59 overexpressed tobacco. The white scale bar represents 5 cm. (D, E) The expression of ZmMYB59 overexpressed rice was detected by semi-quantitative RT-PCR (D) or real time PCR (E). RNA was extracted from T2 generation transgenic rice. 1: WT; 2: vector used for transformation; OE-2, 4, 6: different lines of ZmMYB59 overexpressed rice. The expression of Osactin was used as internal control. Values are means ± SD; n = 3. ***P < 0.001 (Student’s t-test). (F) Phenotype of ZmMYB59 overexpressed rice. The white scale bar represents 5 cm.
The results showed that ZmMYB59 overexpression significantly inhibited seed germination among three independent homozygous transgenic lines. In T2 generation transgenic tobacco, germination rate, germination index, vigor index and hypocotyl length were decreased by 25.0–50.9, 34.5–54.4, 57.5–88.3, and 21.9–31.3% compared to WT lines (Table 2, Figure 2C). In T2 generation transgenic rice, the corresponding indexes were reduced by 39.1–53.8, 51.4–71.4, 52.5–74.0, and 28.3–41.5%, respectively (Table 2, Figure 2F). The above results suggested that ZmMYB59 played a negative regulatory role in the process of seed germination in both transgenic tobacco and rice.
To investigate whether ZmMYB59 expression influenced antioxidant capacity, the contents of malondialdehyde (MDA) and the activities of CAT, POD, SOD and APX were measured (Table 3). In T2 generation transgenic tobacco, MDA content was enhanced by 5.7–21.5% compared to WT lines. In T2 generation transgenic rice, MDA content was increased by 4.3–8.0% compared to WT lines. Moreover, the activities of CAT, POD, SOD, and APX of transgenic tobacco were significantly decreased by 32.3–46.2, 18.0–25.3, 9.8–18.9, 19.8–29.0%, respectively. In transgenic rice, the above enzymatic activities were decreased by 8.3–12.8, 9.0–19.4, 24.8–43.5, 36.5–59.6%, respectively. It could be documented that ZmMYB59 could decrease antioxidant capacity of transgenic tobacco and rice, which was generally consistent with the results of germination experiment.
Considering that ZmMYB59 reduced hypocotyl/mesocotyl length, cellular morphology of hypocotyl/mesocotyl in tobacco and rice was observed in this experiment to determine whether and how ZmMYB59 affects cell proliferation and elongation. This is indeed the case (Figure 3, Table 4). In T2 generation transgenic tobacco, cell number and cell length of hypocotyl were significantly decreased by 12.8–22.2 and 21.7–42.7% compared to WT lines (Figures 3A, C). In T2 generation transgenic rice, cell number and cell length of mesocotyl were significantly reduced by 20.0–28.2 and 10.8–17.6% (Figures 3B, D). The results suggested the low hypocotyl/mesocotyl length caused by ZmMYB59 might be attributed to the inhibition of cell growth including cell number and cell length.
Figure 3 Microstructure of hypocotyl in tobacco (A, C) /mesocotyl in rice (B, D) cells of wild-type and ZmMYB59 transgenic plants. A-Wild-type tobacco. C-T2 generation transgenic tobacco. B-Wild-type rice. D-T2 generation transgenic rice. The image is a 4 × fluorescence microscope, and the arrow refers to the longitudinal boundary position of the cell.
Endogenous phytohormones play important roles during seed germination, thus ABA, IAA, GA1, GA3, GA4, CTK was determined. In T2 generation transgenic tobacco, compared to WT lines, the contents of endogenous GA1, GA3, GA4, IAA, CTK were reduced by 21.1–39.2, 18.7–29.9, 3.3–15.4, 3.4–7.5 and 27.9–44.8%, whereas the content of ABA was increased by 23.8–43.9% (Table 5). In T2 generation transgenic rice, the contents of endogenous GA1, GA3, GA4, IAA and CTK were decreased by 29.4–47.6, 14.9–22.3, 15.4–24.3, 5.7–10.0, and 15.7–37.8%, whereas the content of ABA was increased by 17.9–26.9% (Table 5). Among them, the changes of endogenous GA1, CTK, and ABA reached significant level while there were no significant changes in those of endogenous GA3, GA4, and IAA. These results indicated that the inhibiting effect of ZmMYB59 might be ascribed to the promotion of endogenous GA1 and CTK synthesis and the inhibition of endogenous ABA synthesis.
Seed germination is directly related to field emergence and crop yield. The function MYB family in plant growth and biotic/abiotic stresses were extensively reported (Naing and Kim, 2018; Li et al., 2019), but the roles of MYB on hypocotyl/mesocotyl elongation during seed germination was rarely studied. It was well known that elongation of the mesocotyl and the first internode were helpful for seed germination (Zhao and Wang, 2008; Ohno et al., 2018). In previous report, CIR1, an MYB-related genes, could inhibit hypocotyl elongation and seed germination (Zhang et al., 2007). During seed germination, the expression ZmMYB59 in maize mesocotyl was highly inhibited by the increase of sowing depth (Du et al., 2017). In same manner, AtMYB30 was highly expressed in brassinosteroid pathway to manipulate hypocotyl cell prolongation during seed germination (Li et al., 2009). In this study, hypocotyl/mesocotyl length of ZmMYB59 transgenic tobacco and rice was significantly lower than wild-type lines.
To further detect the effect of ZmMYB59 expression on hypocotyl/mesocotyl elongation, cell morphology of hypocotyl/mesocotyl was observed. AtMYB59 was reported to negatively regulate cell cycle progression of root tips, and inhibited root growth by extending the metaphase of mitotic cells (Mu et al., 2009). Here, we found ZmMYB59, which had 53.65% similarity to AtMYB59 in the sequence of amino acid. Moreover, our results showed that ZmMYB59 could suppress hypocotyl/mesocotyl elongation in phenotype, which might contributed to negatively control cell growth in cell level.
MYB transcription factors were thought to involve in plant development mediated by phytohormone. AtMYB60 and AtMYB96 could synergistically control stomatal aperture, drought, and disease resistance by ABA signal pathway (Dubos et al., 2010). GAMYB expression in the first internode was substantially increased by GA3 application in wheat (Chen et al., 2001). AtMYB7 negatively regulated ABA-induced inhibition of seed germination by blocking the expression of a bZIP transcription factor ABI5 (Kim et al., 2015). Overexpression of OsMYBR1 conferred improved drought tolerance and decreased ABA sensitivity in rice (Yin et al., 2017). CLAU was a MYB transcription factor that modulated leaf morphogenesis by constraining the morphogenetic potential, in part due to attenuation of CTK signaling (Bar et al., 2016).
In our previous study, GA and MYB were thought to involve in mesocotyl elongation by combining Affymetrix GeneChip analysis and Real-time PCR in maize (Zhao et al., 2010). Further analysis showed that the expression of ZmMYB59 was inhibited by exogenous GA treatment in maize mesocotyl (Du et al., 2017). In this study, GA1 content was decreased in ZmMYB59 overexpressed tobacco and rice. Cytokinin (CTK) was positively regulated cell division to control plant growth (Schaller et al., 2014). Here, we found the content of CTK and cell number were reduced in ZmMYB59 overexpressed tobacco and rice (Figure 3; Table 4).
In Arabidopsis, GA was found to interact with ATHB5 and increase cell expansion to promote middle and upper hypocotyl elongation (Stamm et al., 2017). CTK could promote the elongation of hypocotyl in the light when ethylene signal pathway is blocked (Smets et al., 2005). Here, we found the content of GA1 and CTK was decreased in ZmMYB59 overexpressed rice and tobacco (Table 5). During germination, ROS was found to inhibit cell growth by regulating expression of 4EBP and S6K (Toshniwal et al., 2019). The accumulation of ROS could be induced by ABA in Arabidopsis (Postiglione and Muday, 2020). In Physcomitrella patens, ppabi1a/b double mutant, in which ABA signaling was constitutively active, exhibited server growth retardation (Komatsu et al., 2013). In another report, ABA was found to inhibit hypocotyl elongation in early seedling growth in Arabidopsis (Belin et al., 2009). Further study showed that ABA could inhibit hypocotyl elongation by dephosphorylating H+-ATPase in Arabidopsis (Hayashi et al., 2014). In this study, we found the contents of ABA and ROS were accumulated in ZmMYB59 overexpressed rice and tobacco, in which the growth of hypocotyl/mesocotyl was inhibited (Table 2). These means that ABA might suppress elongation of hypocotyl/mesocotyl by different pathways. Taken together, ZmMYB59 may inhibit mesocotyl elongation during seed germination by regulating GA, CTK, and ABA signaling pathways (Figure 4).
Figure 4 The regulatory mechanisms of seed germination regulated by ZmMYB59 in tobacco and rice. GA1, CTK ABA represent gibberellin A1, cytokinin, abscisic acid, respectively. CAT, POD, SOD, APX represent catalase, peroxidase, superoxide dismutase, ascorbate peroxidase, respectively.
In summary, the possible model regulated by ZmMYB59 gene during seed germination of tobacco and rice was elucidated in Figure 4. The model in Figure 4 suggested that ZmMYB59 gene was a negative regulatory factor during seed germination in tobacco and rice. In future, genetic transformation of ZmMYB59 gene in maize will be performed to further validate its functions. Gene knockout is advised as an effective strategy to breeding new maize varieties, which improve seed germination.
The results reported here demonstrated that ZmMYB59 heterogenous expression in tobacco and rice had a negative effect on seed germination by inhibiting the synthesis of GA1, CTK and IAA and promoting the synthesis of ABA. Meanwhile, the decrease of GA and CTK might have a negative effect on cell growth, while high ABA could promote the ROS accumulation and suppress the antioxidant enzyme activity. Above negative factors (high ABA, ROS and low GA, CTK) may have a joint influence on hypocotyl/mesocotyl elongation during seed germination. Collectively, our findings suggest that ZmMYB59 plays a negatively regulatory role in tobacco and rice, which will contribute to elucidate the mechanisms of seed germination regulated by MYB transcription factors, and also provides a key gene affecting seed germination.
All datasets generated for this study are included in the article/Supplementary Material.
GZ designed, supervised the study, and analyzed the data. KZ wrote the manuscript and analyzed the data. HJ performed the experiments of rice. CS and JR performed the experiments of tobacco. All authors contributed to the article and approved the submitted version.
This research was supported by Natural Science Foundation of Zhejiang province (LY18C130001, LY13C130011), National Natural Science Foundation of China (31371712), Zhejiang Key Project for New Variety Breeding of Agriculture (Grain) (2016C02050-9-5), The National Key Research and Development Program of China (2018YFD0100900), Special Fund for Agro-scientific Research in the Public Interest of China (201303002), The Key Research and Development Program of Zhejiang (2019C02013). The funder bodies have no role in the design of the study and collection, analysis, and interpretation of data and writing the manuscript.
The authors declare that the research was conducted in the absence of any commercial or financial relationships that could be construed as a potential conflict of interest.
We acknowledge all the members of the research team for their assistance in the field and laboratory work. Meanwhile, we thank Hangzhou Biogle Co., Ltd. for assistance with genetic transformation, seed propagation and providing the pictures of genetic transformation. Finally, this manuscript has been released as a pre-print at [ResearchSquare] (Zhai et al., 2020).
The Supplementary Material for this article can be found online at: https://www.frontiersin.org/articles/10.3389/fpls.2020.564665/full#supplementary-material
Bailly, C., El-Maarouf-Bouteau, H., Corbineau, F. (2008). From intracellular signaling networks to cell death: the dual role of reactive oxygen species in seed physiology. Comptes Rendus Biologies 331, 806–814. doi: 10.1016/j.crvi.2008.07.022
Bar, M., Israeli, A., Levy, M., Ben Gera, H., Jimenez-Gomez, J. M., Kouril, S., et al. (2016). CLAUSA Is a MYB Transcription Factor That Promotes Leaf Differentiation by Attenuating Cytokinin Signaling. Plant Cell 28, 1602–1615. doi: 10.1105/tpc.16.00211
Belin, C., Megies, C., Hauserova, E., Lopez-Molina, L. (2009). Abscisic acid represses growth of the Arabidopsis embryonic axis after germination by enhancing auxin signaling. Plant Cell. 21, 2253–2268. doi: 10.1105/tpc.109.067702
Chen, L., Nishizawa, T., Higashitani, A., Suge, H., Wakui, Y., Takeda, K., et al. (2001). A variety of wheat tolerant to deep-seeding conditions: elongation of the first internode depends on the response to gibberellin and potassium. Plant Cell Environ. 24, 469–476. doi: 10.1046/j.1365-3040.2001.00688.x
Du, H., Feng, B.-R., Yang, S.-S., Huang, Y.-B., Tang, Y.-X. (2012). The R2R3-MYB Transcription Factor Gene Family in Maize. PloS One 7, e37463. doi: 10.1371/journal.pone.0037463
Du, L., Jiang, H., Zhao, G., Ren, J. (2017). Gene cloning of ZmMYB59 transcription factor in maize and its expression during seed germination in response to deep-sowing and exogenous hormones. Plant Breed. 136, 834–844. doi: 10.1111/pbr.12550
Dubos, C., Stracke, R., Grotewold, E., Weisshaar, B., Martin, C., Lepiniec, L. (2010). MYB transcription factors in Arabidopsis. Trends Plant Sci. 15, 573–581. doi: 10.1016/j.tplants.2010.06.005
El-Maarouf-Bouteau, H., Bailly, C. (2008). Oxidative signaling in seed germination and dormancy. Plant Signaling Behav. 3, 175–182. doi: 10.4161/psb.3.3.5539
Feller, A., Machemer, K., Braun, E. L., Grotewold, E. (2011). Evolutionary and comparative analysis of MYB and bHLH plant transcription factors. Plant J. 66, 94–116. doi: 10.1111/j.1365-313X.2010.04459.x
Foyer, C. H., Lelandais, M., Kunert, K. J. (1994). Photooxidative stress in plants. Physiologia Plantarum 92, 696–717. doi: 10.1111/j.1399-3054.1994.tb03042.x
Gu, J. W., Chao, H. B., Gan, L., Guo, L. X., Zhang, K., Li, Y. H., et al. (2016). Proteomic Dissection of Seed Germination and Seedling Establishment in Brassica napus. Front. Plant Sci. 7, 1482. doi: 10.3389/fpls.2016.01482
Hayashi, Y., Takahashi, K., Inoue, S., Kinoshita, T. (2014). Abscisic acid suppresses hypocotyl elongation by dephosphorylating plasma membrane H(+)-ATPase in Arabidopsis thaliana. Plant Cell Physiol. 55, 845–853. doi: 10.1093/pcp/pcu028
Kim, J. H., Hyun, W. Y., Nguyen, H. N., Jeong, C. Y., Xiong, L., Hong, S. W., et al. (2015). AtMyb7, a subgroup 4 R2R3 Myb, negatively regulates ABA-induced inhibition of seed germination by blocking the expression of the bZIP transcription factor ABI5. Plant Cell Environ. 38, 559–571. doi: 10.1111/pce.12415
Komatsu, K., Suzuki, N., Kuwamura, M., Nishikawa, Y., Nakatani, M., Ohtawa, H., et al. (2013). Group A PP2Cs evolved in land plants as key regulators of intrinsic desiccation tolerance. Nat. communications. 4, 2219. doi: 10.1038/ncomms3219
Li, L., Yu, X., Thompson, A., Guo, M., Yoshida, S., Asami, T., et al. (2009). Arabidopsis MYB30 is a direct target of BES1 and cooperates with BES1 to regulate brassinosteroid-induced gene expression. Plant J. 58, 275–286. doi: 10.1111/j.1365-313X.2008.03778.x
Li, F., Zhang, H., Zhao, H., Gao, T., Song, A., Jiang, J., et al. (2018). Chrysanthemum CmHSFA4 gene positively regulates salt stress tolerance in transgenic chrysanthemum. Plant Biotechnol. J. 16, 1311–1321. doi: 10.1111/pbi.12871
Li, M., Lin, L., Zhang, Y., Sui, N. (2019). ZmMYB31, a R2R3-MYB transcription factor in maize, positively regulates the expression of CBF genes and enhances resistance to chilling and oxidative stress. Mol. Biol. Rep. 46, 3937–3944. doi: 10.1007/s11033-019-04840-5
Liu, H., Xiong, J.-s., Jiang, Y.-t., Wang, L., Cheng, Z.-m. (2019). Evolution of the R2R3-MYB gene family in six Rosaceae species and expression in woodland strawberry. J. Integr. Agric. 18, 2753–2770. doi: 10.1016/s2095-3119(19)62818-2
Mazer, S. J. (1999). Seeds - Ecology, biogeography, and evolution of dormancy and germination. Science 283, 334–334. doi: 10.1126/science.283.5400.334
Mittler, R., Vanderauwera, S., Gollery, M., Van Breusegem, F. (2004). Reactive oxygen gene network of plants. Trends Plant Sci. 9, 490–498. doi: 10.1016/j.tplants.2004.08.009
Mu, R.-L., Cao, Y.-R., Liu, Y.-F., Lei, G., Zou, H.-F., Liao, Y., et al. (2009). An R2R3-type transcription factor gene AtMYB59 regulates root growth and cell cycle progression in Arabidopsis. Cell Res. 19, 1291–1304. doi: 10.1038/cr.2009.83
Naing, A. H., Kim, C. K. (2018). Roles of R2R3-MYB transcription factors in transcriptional regulation of anthocyanin biosynthesis in horticultural plants. Plant Mol. Biol. 98, 1–18. doi: 10.1007/s11103-018-0771-4
Narbona, E., Delgado, A., Encina, F., Miguez, M., Buide, M. L. (2013). Seed germination and seedling establishment of the rare Carex helodeLink depend on the proximity to water. Aquat. Bot. 110, 55–60. doi: 10.1016/j.aquabot.2013.05.005
Ohno, H., Banayo, N. P. M. C., Bueno, C. S., Kashiwagi, J.-i., Nakashima, T., Corales, A. M., et al. (2018). Longer mesocotyl contributes to quick seedling establishment, improved root anchorage, and early vigor of deep-sown rice. Field Crops Res. 228, 84–92. doi: 10.1016/j.fcr.2018.08.015
Porebski, S., Bailey, L. G., Baum, B. R. (1997). Modification of a CTAB DNA extraction protocol for plants containing high polysaccharide and polyphenol components. Plant Mol. Biol. Rep. 15, 8–15. doi: 10.1007/BF02772108
Postiglione, A. E., Muday, G. K. (2020). The Role of ROS Homeostasis in ABA-Induced Guard Cell Signaling. Front. Plant science. 11, 968. doi: 10.3389/fpls.2020.00968
Pu, X., Yang, L., Liu, L., Dong, X., Chen, S., Chen, Z., et al. (2020). Genome-Wide Analysis of the MYB Transcription Factor Superfamily in Physcomitrella patens. Int. J. Mol. Sci. 21, 975. doi: 10.3390/ijms21030975
Qin, S. S., Chen, X. Y., Jiang, C., Li, M. J., Yuan, Y., Yang, J., et al. (2019). Pruning induced yield and quality variations and the correlated gene expression and phytohormone changes in Lonicera japonica. Ind. Crops Products 132, 386–395. doi: 10.1016/j.indcrop.2019.02.048
Riechmann, J. L., Heard, J., Martin, G., Reuber, L., Jiang, C. Z., Keddie, J., et al. (2000). Arabidopsis transcription factors: Genome-wide comparative analysis among eukaryotes. Science 290, 2105–2110. doi: 10.1126/science.290.5499.2105
Sarker, U., Oba, S. (2018). Drought Stress Effects on Growth, ROS Markers, Compatible Solutes, Phenolics, Flavonoids, and Antioxidant Activity in Amaranthus tricolor. Appl. Biochem. Biotechnol. 186, 999–1016. doi: 10.1007/s12010-018-2784-5
Schaller, G. E., Street, I. H., Kieber, J. J. (2014). Cytokinin and the cell cycle. Curr. Opin. Plant Biol. 21, 7–15. doi: 10.1016/j.pbi.2014.05.015
Smets, R., Le, J., Prinsen, E., Verbelen, J. P., Van Onckelen, H. A. (2005). Cytokinin-induced hypocotyl elongation in light-grown Arabidopsis plants with inhibited ethylene action or indole-3-acetic acid transport. Planta 221, 39–47. doi: 10.1007/s00425-004-1421-4
Stamm, P., Topham, A. T., Mukhtar, N. K., Jackson, M. D., Tome, D. F., Beynon, J. L., et al. (2017). The Transcription Factor ATHB5 Affects GA-Mediated Plasticity in Hypocotyl Cell Growth during Seed Germination. Plant Physiol. 173, 907–917. doi: 10.1104/pp.16.01099
Stracke, R., Werber, M., Weisshaar, B. (2001). The R2R3-MYB gene family in Arabidopsis thaliana. Curr. Opin. Plant Biol. 4, 447–456. doi: 10.1016/s1369-5266(00)00199-0
Toshniwal, A. G., Gupta, S., Mandal, L., Mandal, S. (2019). ROS Inhibits Cell Growth by Regulating 4EBP and S6K, Independent of TOR, during Development. Dev. Cell. 49, 473–489. doi: 10.1016/j.devcel.2019.04.008
Wang, Y., Htwe, Y. M., Li, J., Shi, P., Zhang, D., Zhao, Z., et al. (2019). Integrative omics analysis on phytohormones involved in oil palm seed germination. BMC Plant Biol. 19, 363–363. doi: 10.1186/s12870-019-1970-0
Xie, L. X., Tan, Z. W., Zhou, Y., Xu, R. B., Feng, L. B., Xing, Y. Z., et al. (2014). Identification and fine mapping of quantitative trait loci for seed vigor in germination and seedling establishment in rice. J. Integr. Plant Biol. 56, 749–759. doi: 10.1111/jipb.12190
Yang, B. C., Song, Z. H., Li, C. N., Jiang, J. H., Zhou, Y. Y., Wang, R. P., et al. (2018). RSM1, an Arabidopsis MYB protein, interacts with HY5/HYH to modulate seed germination and seedling development in response to abscisic acid and salinity. PloS Genet. 14, e1007839. doi: 10.1371/journal.pgen.1007839
Yin, X., Cui, Y., Wang, M., Xia, X. (2017). Overexpression of a novel MYB-related transcription factor, OsMYBR1, confers improved drought tolerance and decreased ABA sensitivity in rice. Biochem. Biophys. Res. Commun. 490, 1355–1361. doi: 10.1016/j.bbrc.2017.07.029
Yoshimura, K., Yabuta, Y., Ishikawa, T., Shigeoka, S. (2000). Expression of spinach ascorbate peroxidase isoenzymes in response to oxidative stresses. Plant Physiol. 123, 223–233. doi: 10.1104/pp.123.1.223
Zhai, K., Zhao, G., Jiang, H., Sun, C., Ren, J. (2020). Expression of MYB transcription factor gene ZmMYB59 affects seed germination in Nicotiana tabacum and Oryza sativa. ResearchSquare. doi: 10.21203/rs.3.rs-19878/v2
Zhang, X., Chen, Y., Wang, Z. Y., Chen, Z., Gu, H., Qu, L. J. (2007). Constitutive expression of CIR1 (RVE2) affects several circadian-regulated processes and seed germination in Arabidopsis. Plant J. Cell Mol. Biol. 51, 512–525. doi: 10.1111/j.1365-313X.2007.03156.x
Zhao, G., Wang, J. (2008). Effect of Gibberellin and Uniconazole on Mesocotyl Elongation of Dark-Grown Maize under Different Seeding Depths. Plant Production Sci. 11, 423–429. doi: 10.1626/pps.11.423
Zhao, G., Fu, J., Wang, G., Ma, P., Wu, L., Wang, J. (2010). Gibberellin-induced mesocotyl elongation in deep-sowing tolerant maize inbred line 3681-4. Plant Breed. 129, 87–91. doi: 10.1111/j.1439-0523.2009.01658.x
Keywords: MYB transcription factor, overexpression, ZmMYB59, negative regulation, seed germination
Citation: Zhai K, Zhao G, Jiang H, Sun C and Ren J (2020) Overexpression of Maize ZmMYB59 Gene Plays a Negative Regulatory Role in Seed Germination in Nicotiana tabacum and Oryza sativa. Front. Plant Sci. 11:564665. doi: 10.3389/fpls.2020.564665
Received: 02 June 2020; Accepted: 17 August 2020;
Published: 11 September 2020.
Edited by:
Enamul Huq, University of Texas at Austin, United StatesReviewed by:
Min Ni, University of Minnesota Twin Cities, United StatesCopyright © 2020 Zhai, Zhao, Jiang, Sun and Ren. This is an open-access article distributed under the terms of the Creative Commons Attribution License (CC BY). The use, distribution or reproduction in other forums is permitted, provided the original author(s) and the copyright owner(s) are credited and that the original publication in this journal is cited, in accordance with accepted academic practice. No use, distribution or reproduction is permitted which does not comply with these terms.
*Correspondence: Guangwu Zhao, Z3d1emhhb0AxMjYuY29t
Disclaimer: All claims expressed in this article are solely those of the authors and do not necessarily represent those of their affiliated organizations, or those of the publisher, the editors and the reviewers. Any product that may be evaluated in this article or claim that may be made by its manufacturer is not guaranteed or endorsed by the publisher.
Research integrity at Frontiers
Learn more about the work of our research integrity team to safeguard the quality of each article we publish.