- 1Institute of Surface-Earth System Science, School of Earth System Science, Tianjin University, Tianjin, China
- 2Key Laboratory of Recycling and Eco-treatment of Waste Biomass of Zhejiang Province, Zhejiang University of Science and Technology, Hangzhou, China
- 3College of Land and Environment, Shenyang Agriculture University, Shenyang, China
- 4Department of Biology and Environmental Science, Linnaeus University, Kalmar, Sweden
- 5Institute of Environment and Sustainable Development in Agriculture, Chinese Academy of Agricultural Sciences, Beijing, China
- 6College of Urban and Environmental Sciences, Peking University, Peking, China
Silicon (Si) plays an important role in improving soil nutrient availability and plant carbon (C) accumulation and may therefore impact the biogeochemical cycles of C, nitrogen (N), and phosphorus (P) in terrestrial ecosystems profoundly. However, research on this process in grassland ecosystems is scarce, despite the fact that these ecosystems are one of the most significant accumulators of biogenic Si (BSi). In this study, we collected the aboveground parts of four widespread grasses and soil profile samples in northern China and assessed the correlations between Si concentrations and stoichiometry and accumulation of C, N, and P in grasses at the landscape scale. Our results showed that Si concentrations in plants were significantly negatively correlated (p < 0.01) with associated C concentrations. There was no significant correlation between Si and N concentrations. It is worth noting that since the Si concentration increased, the P concentration increased from less than 0.10% to more than 0.20% and therefore C:P and N:P ratios decreased concomitantly. Besides, the soil noncrystalline Si played more important role in C, N, and P accumulation than other environmental factors (e.g., MAT, MAP, and altitude). These findings indicate that Si may facilitate grasses in adjusting the utilization of nutrients (C, N, and P) and may particularly alleviate P deficiency in grasslands. We conclude that Si positively alters the concentrations and accumulation of C, N, and P likely resulting in the variation of ecological stoichiometry in both vegetation and litter decomposition in soils. This study further suggests that the physiological function of Si is an important but overlooked factor in influencing biogeochemical cycles of C and P in grassland ecosystems.
Introduction
Silicon (Si) is the second most abundant element in the Earth’s crust but the beneficial function of this element for plants was overlooked until studies found that Si is actively taken up by many plants (Epstein, 1994; Carey and Fulweiler, 2012; Ma and Yamaji, 2015). After being absorbed by plants dissolved Si is deposited as amorphous SiO2 (or phytoliths) in plant tissues (Ma and Yamaji, 2006; Cooke and Leishman, 2016), while less Si binds to semicellulose of the cell, potentially improving the strength and rigidity of plants (Broadley et al., 2012; He et al., 2015). This physiological function of Si enhances plant resistance against abiotic stresses such as drought and salt environments (Hattori et al., 2005; Liang et al., 2007; Rios et al., 2017) and biotic stresses including plant pathogens and insect pests (Ma, 2004). Si is therefore highly beneficial for plant growth and productivity (Van Bockhaven et al., 2013; Li and Delvaux, 2019).
Interest in the impacts of Si on carbon (C), nitrogen (N), and phosphorus (P) concentrations in plants is increasing (Schoelynck et al., 2010; Schaller et al., 2012a; Neu et al., 2017; Klotzbücher et al., 2018; Li et al., 2018a). Most of these studies have reported that the Si concentration is negatively correlated with that of C in plants (Schaller et al., 2012b; Klotzbücher et al., 2018). However, the application of Si promotes N concentration in non-leguminous plants exposed to N-deficient grasslands (Xu et al., 2018), despite the fact that the N concentration in wetland plants showed negative correlations with the concentration of Si (Schaller et al., 2016). Similarly, P concentration in plants in a P-deficient habitat is also promoted by the application of Si (Schaller et al., 2012b; Kostic et al., 2017; Neu et al., 2017) but is suppressed in an environment of excessive P (Ma and Takahashi, 1990; Hu et al., 2018). This shift between C concentration and nutrient status in response to Si application alters the stoichiometry of C, N, and P in plants, that this has been commonly used to indicate the balance of these elements (Hessen et al., 2004; He et al., 2008; Li et al., 2018b). Furthermore, many studies have observed that Si supply could improve plant biomass production (Eneji et al., 2008; Liang et al., 2015; Neu et al., 2017; Li et al., 2018a; Li and Delvaux, 2019; Li et al., 2019) and could enhance significantly total C, N, and P accumulation in plants (Xu et al., 2015; Li et al., 2018b). However, most of these studies were carried out in farmlands in either pot or field experiments and comprehensive investigations of natural ecosystems are still lacking.
Grassland ecosystems occupy more than 20% of the world’s land surface (Scurlock and Hall, 1998) and store considerable quantities of C to sequester 0.5 Pg C into soil every year (Scurlock and Hall, 1998; Fang et al., 2010; Wu et al., 2014). Additionally, grasslands play an important role in the global terrestrial production of biological silicon (BSi) (Blecker et al., 2006; White et al., 2012; Song et al., 2014a). However, Si is not yet well accepted as an important element in conceptual models of the grassland C biogeochemical cycle or the closely related N and P cycles (Schmidt et al., 2011; Song et al., 2012a; Lehmann and Kleber, 2015). The physiological effects of Si on accumulation and stoichiometry of C, N, and P in grasses are less well known.
We collected aboveground parts of four of the most widespread grasses in our study area in northern China to determine the concentrations of Si and explore the impacts on stoichiometry and accumulation of C, N, and P. Here, we hypothesize that Si accumulation could affect the concentration and stoichiometry of C, N, and P in grass species. The Si could promote N and P absorption in plant, while the C concentration is negatively correlated with Si concentration. We further analyzed the same species of grasses with diverse Si concentrations throughout grasslands in northern China. The study area belongs to agro-pastoral ecotone, where is experiencing degradation at varying degrees because of climate change and human activities (Jiang et al., 2006; Yang et al., 2019). These results facilitated the systematic assessment of the impacts of Si accumulation on stoichiometry and accumulation of C, N, and P in grasslands at landscape scales and further could provide references for grassland management.
Materials and Methods
Study Area and Field Sampling
The study area is situated between the northern part of Hebei Province and the southeastern part of Inner Mongolia in Northern China (Figure 1). The climate may be described as semi-humid and semi-arid temperate, with mean annual precipitations (MAP) and mean annual temperatures (MAT) ranging from 311.9 to 421.3 mm and from 2.1°C to 8.3°C, respectively. The main soil types are kastanozems and arenosols as classified by the Food and Agriculture Organization (FAO). The vegetation type is typical steppe or meadow steppe (Figure 1) (Hou, 1982).
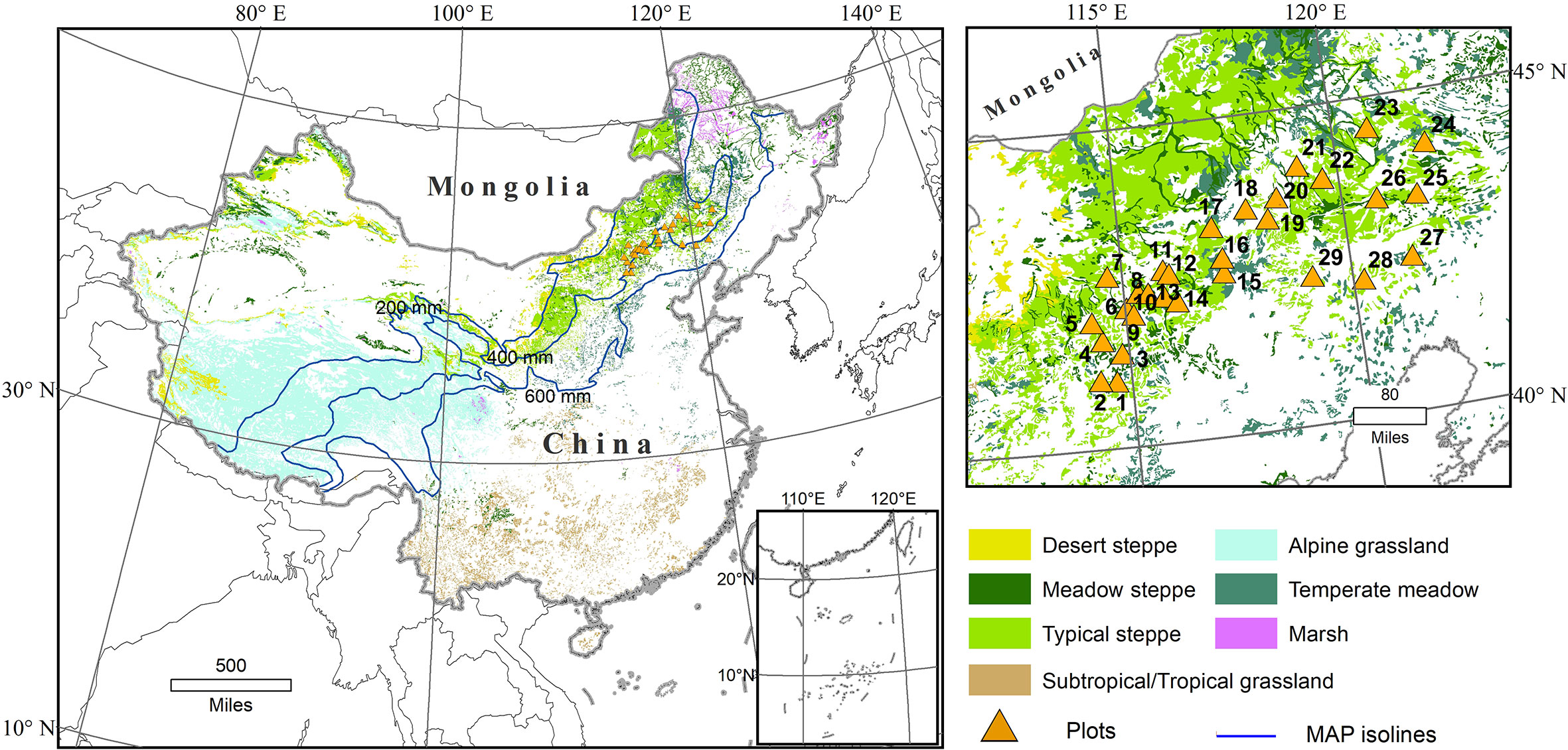
Figure 1 Distribution of grass types in China, with locations of the study region and sampling sites (orange triangles with numbers consistent with Table 1; modified from Yang et al., 2019).
Field investigations and sampling were conducted at 29 sites in July 2017, when grasses reached maturity. At each sampling site, three replicates of 2 m × 2 m plots were randomly set up. Data recorded for plant species included cover, abundance and height. Aboveground parts of all plants in a 1 m × 1 m quadrat within the 2 m × 2 m sample plots were harvested to estimate total aboveground biomass. At the same time, the soil profiles (0–10 cm) of each plot were collected. The detailed method could be referred to Yang et al. (2019).
Four of the most widely distributed grass species were sampled for determining aboveground biomass, including Leymus chinensis, Cleistogenes squarrosa, Agropyron mongolicum and Stipa krylovii (Table 1). Each species had one sample (over 150 g) in one site, but not all the sites contained all the four species (Table 1). A total of 57 samples were collected, including 16 samples of L. chinensis, 23 samples of C. squarrosa, 7 samples of A. mongolicum, and 11 samples of S. krylovii.
Sample Analysis
Plant samples were carefully cleaned with distilled water, dried for 2 hours at 105°C, dried at 65°C to constant mass and subsequently finely powdered. To measure Si and P concentrations, approximately 75 mg of plant samples were fused with Li-metaborate at 950°C and dissolved in dilute nitric acid. Si and P concentrations were determined colorimetrically by the molybdenum blue method (Song et al., 2012a; Li et al., 2013). C and N concentrations (approximately 5-mg sample) were analyzed with the Elementar vario EL III (Elementar Analyzer systeme GmbH, Hanau, Germany) and each analyzed sample had three replicates.
The soil noncrystalline Si were classified into four fractions operationally based on an improved stepwise chemical extraction method (Kurtz et al., 2002; Detmann et al., 2012; Song et al., 2014b).
Data Calculations and Statistical Analysis
At each site, to estimate the aboveground biomass of the four species we used the average importance value (IV) of each species in three repetitive sample plots to represent the percentage of the total biomass. IV of each species was calculated using the following equation:
where RA represents relative abundance, RC represents relative coverage, and RH represents the relative height of the corresponding species (Liu et al., 2008). Aboveground biomass (g m−2) of each species was calculated using the following equation:
where i represents L. chinensis, C. squarrosa, A. mongolicum, or S. krylovii.
The total element (C, N, or P) accumulation (g m−2) for each species was calculated using the following equation (taking C as an example):
where i represents L. chinensis, C. squarrosa, A. mongolicum, or S. krylovii.
One-way analysis of variance (ANOVA) was calculated to determine whether the average values of C, N, P and Si concentrations differed significantly among different species [p < 0.05 with the Least Significant Difference (LSD) test]. Linear regression analysis was applied to analyze the correlation (examined with Pearson’s correlation coefficients) between Si and C, N, or P for each species and all samples. In order to distinguish the independent effect of four explanatory factors (MAT, MAP, altitude, and soil noncrystalline Si) on C, N, and P accumulation in plants, the hierarchical variation partitioning (HP) was calculated for the four kinds of species and all samples in R 3.6.1 (Hao et al., 2020).
Results
Si, C, N, and P Concentrations and Associated Ratios in Grass Species
Si concentrations ranged from 0.23% to 2.27% among the four species (Table 2). Average concentrations of Si in L. chinensis, C. squarrosa, A. mongolicum, and S. krylovii were 1.12%, 1.28%, 0.83%, and 0.96%, respectively, but were not significant (P > 0.05) (Table 2). In addition, the four grasses had similar concentrations of either C, N, or P (Table 2). C concentrations ranged from 41.71% to 45.49% and N from 1.73% to 2.09%. P concentrations were 0.15% ± 0.03%, 0.16% ± 0.05%, 0.15% ± 0.05%, and 0.12% ± 0.04% for the four grass species. The C. squarrosa had significantly higher Si and P concentrations, while S. krylovii had significantly higher C concentration compared with other species (Table 2).

Table 2 Aboveground biomass, concentrations of Si, C, N, and P and stoichiometric ratios for the four grass species (standard deviations are given in parentheses).
C:N, N:P, and C:P ratios stoichiometry for L. chinensis, C. squarrosa, A. mongolicum and S. krylovii are shown in Table 2. The C:N and N:P ratios ranged from 13.62 to 48.30 and 5.22 to 24.45, respectively (Table 2). C:P ratios ranged from 157.34 to 699.58 with an average value of 326.12 ± 118.81.
Relationships Between Si and Other Parameters
Significant negative correlations were found between Si and C concentrations in L. chinensis, C. squarrosa and for all the samples (Figure 2A). In contrast, Si concentrations were positively correlated with P in L. chinensis, A. mongolicum, and all the samples (Figure 2C). However, Si concentrations did not correlate with N concentrations both within and between all species (Figure 2B). Si concentration was negatively correlated with both C:P and N:P ratios for L. chinensis, A. mongolicum, and all the samples (P < 0.05) (Figures 2E, F), and Si concentration was only positively correlated with C/N for S. krylovii (Figure 2D). However, a positive relationship between Si % and C:N occurred only in S. krylovii.
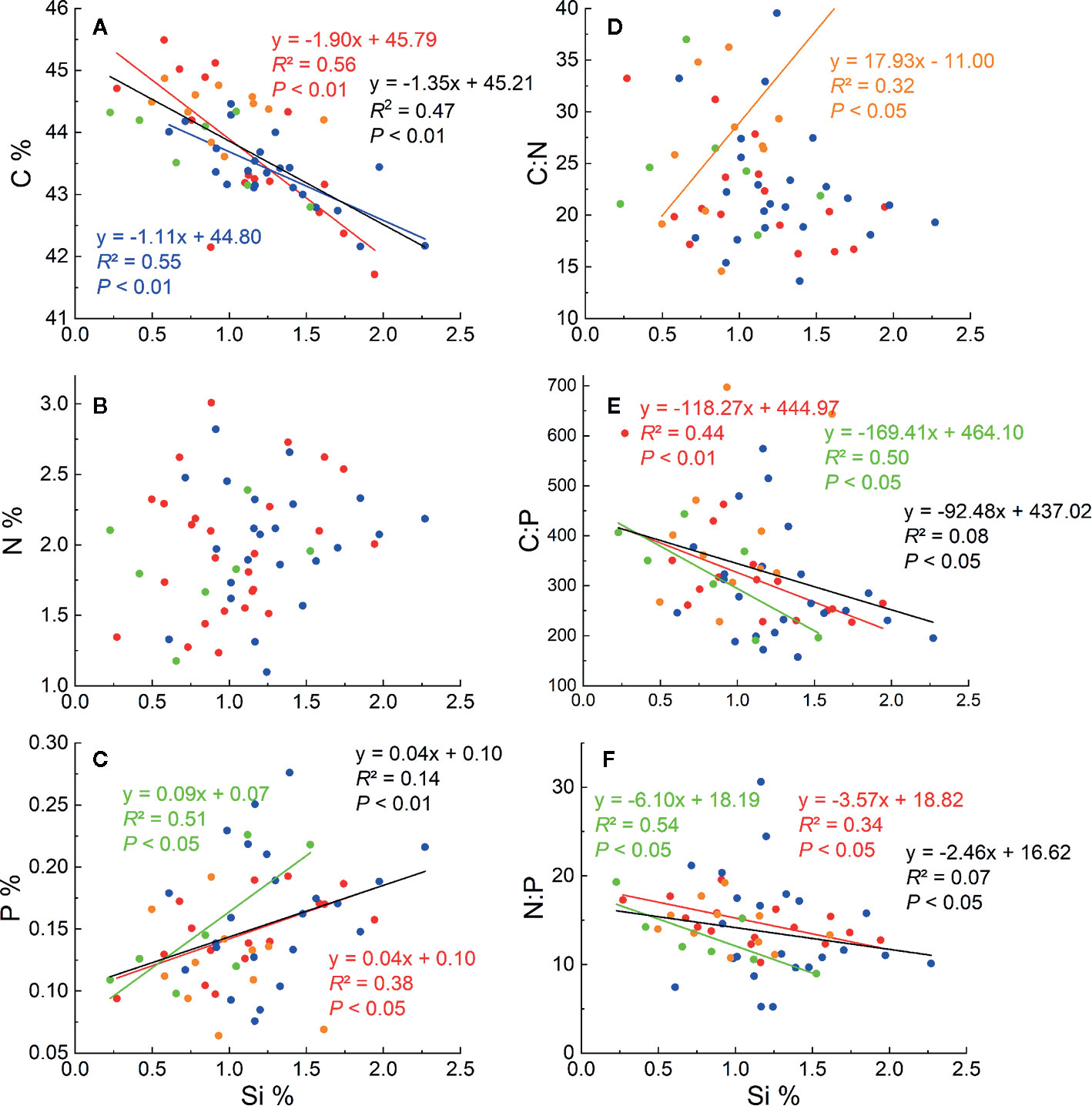
Figure 2 Relationships between Si concentrations and C, N, and P concentrations (A–C), Si concentrations and stoichiometric ratios of N:P, C:P, and C:N (D–F) for these four species. The red dots, lines and text represent Leymus chinensis, blue represent Cleistogenes squarrosa, the green represent Agropyron mongolicum and yellow represent Stipa krylovii. The black dots, lines, and text represent all grass samples.
Strong positive correlations were observed between total C, N, and P accumulations and soil noncrystalline Si (Figure 3), especially for L. chinensis and all samples. The altitude also played an important role in N and P accumulation for L. chinensis (Figures 3G, K). Based on the HP analysis, the soil noncrystalline Si had relatively higher independent effect values on the C, N, and P accumulations for L. chinensis and all the samples compared with MAT, MAP, and altitude (Figure 4). Besides, soil noncrystalline Si concentration was positively correlated with aboveground biomass (Figure 5).
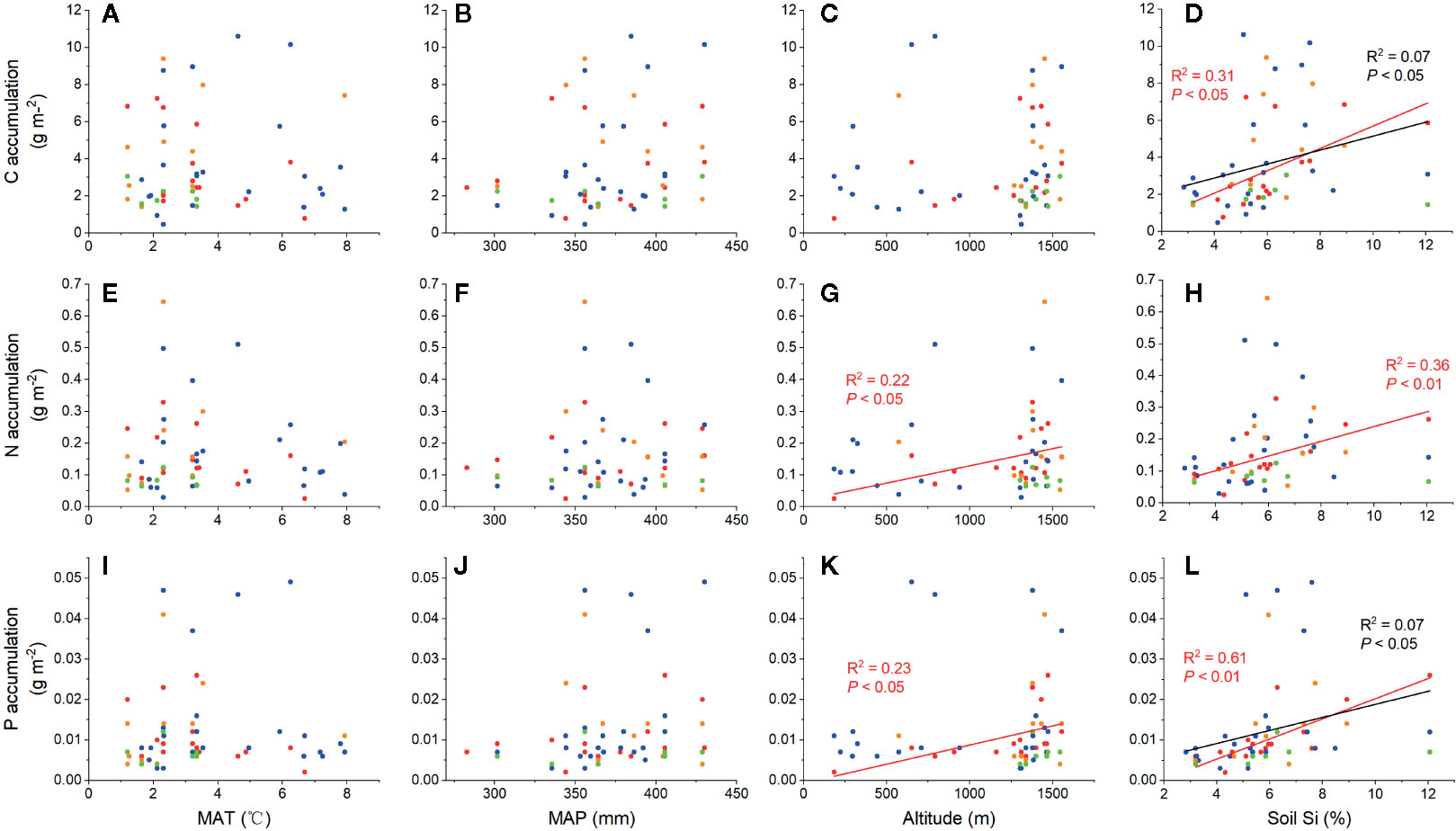
Figure 3 Relationships between environmental factors (MAT, MAP, altitude, and soil noncrystalline Si) and C, N, and P accumulation. The red dots, lines, and text represent L. chinensis, blue represents C. squarrosa, green represents A. mongolicum, and yellow represents S. krylovii. The black dots, lines, and text represent all grass samples. The effects of environmental factors to C accumulation (A–D), N accumulation (E–H) and P accumulation (I–L).
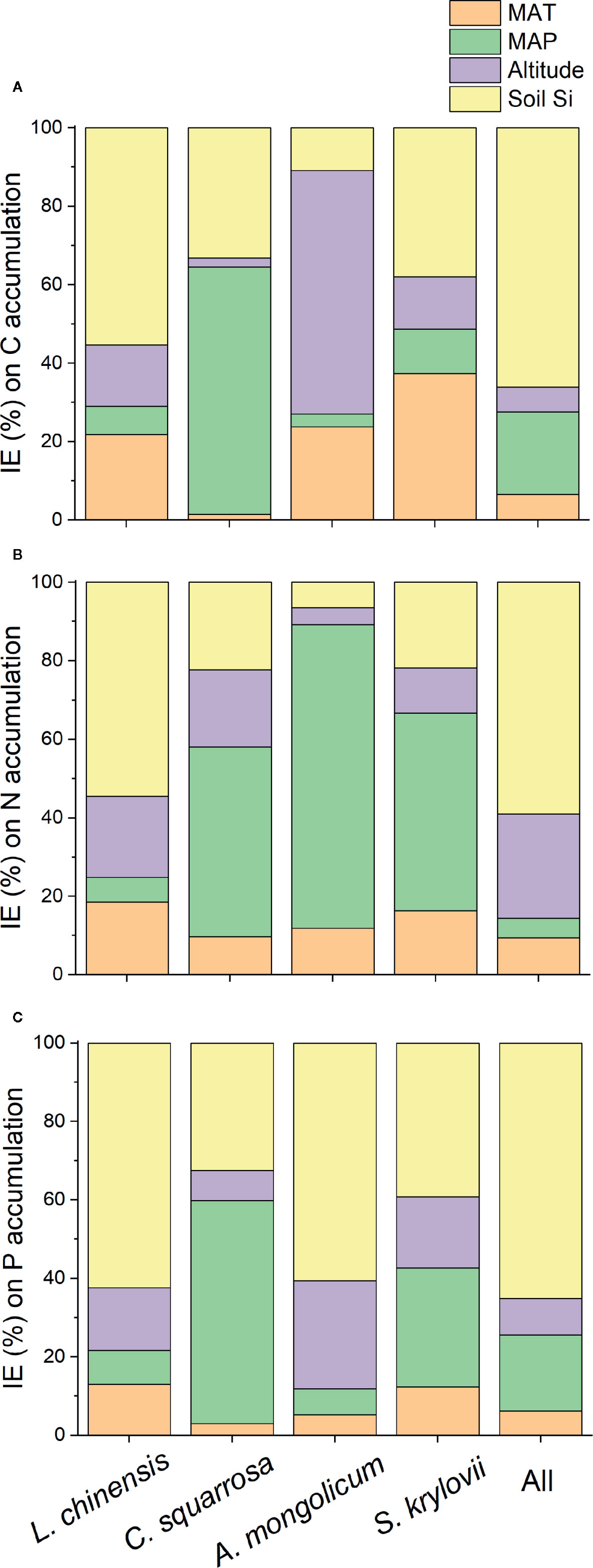
Figure 4 The independent effect (IE; %) of environmental factors (MAT, MAP, altitude, and soil noncrystalline Si) on C (A), N (B), and P (C) accumulation based on hierarchical variation partitioning (HP).
Discussion
Impacts of Si on C, N, and P Concentrations in Grasses
Our data revealed a significant negative correlation between Si and C concentrations in tissues of aboveground parts of the sampled grasses (Figure 2A). Similar results were obtained in studies of reeds (Schaller et al., 2012a; Schaller et al., 2012b), wetlands (Schaller et al., 2016), Si-fertilized winter wheat (Neu et al., 2017), and rice straw in paddies (Klotzbücher et al., 2018). Two possible reasons could explain these findings: i) the accumulation of Si may have a “diluting effect” on the concentrations of C and other elements in plants (Cooke and Leishman, 2012); ii) Si uptake in plants is a “trade-off strategy” between Si and some organic C compounds (Schoelynck et al., 2010; Klotzbücher et al., 2018). In this study, the “diluting effect” is less likely to occur due to a positive correlation between Si and P concentrations (Figure 2C). The Si deposition in tissue works similarly to lignin, causing plants to be resistant to environmental stresses (Epstein, 1994) (Figure 6). Moreover, compared to the synthesis of structural organic compounds, Si deposition is a “low energy cost” strategy for most plants (Raven, 2010; Schoelynck et al., 2010; Schaller et al., 2012a; Klotzbücher et al., 2018).
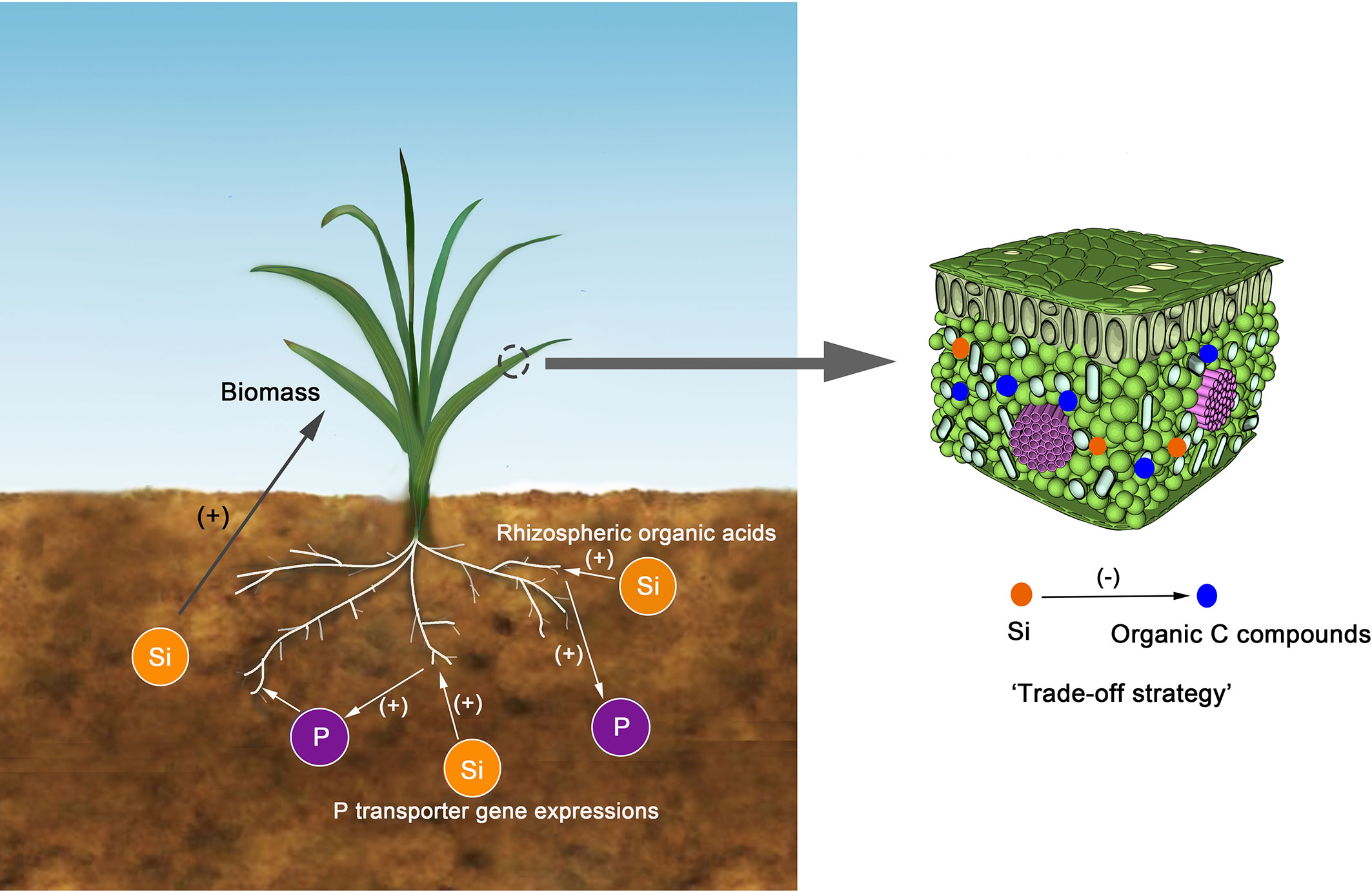
Figure 6 The conceptual model for the relationships between Si and C or P. The Si could substitute come organic carbon compounds, such as lignin. The Si could enhance P bioavailability in soils through regulating P transporter gene expressions or improving rhizospheric organic acids. The soil Si could enhance the biomass of plants and then positively influences the C, N, and P accumulations.
In this study, Si concentration in grass was not correlated with N concentration and this is consistent with the result of rice (Klotzbücher et al., 2018; Li et al., 2018b). This effect may be caused by the offset between the “diluting effect” and the promoting influence that cannot be quantified in this study. However, the Si concentration was positively correlated with P. Similar trends have been reported in other types of grasses (Eneji et al., 2008), reeds (Schaller et al., 2012b), and wheat (Kostic et al., 2017; Neu et al., 2017). This may be related to the interaction between Si uptake and P metabolism in plants (Kostic et al., 2017). Kostic et al. (2017) found that the application of Si could enhance P bioavailability in soils with low P concentrations through up-regulating P transporter gene expressions or improving rhizospheric organic acids (Figure 6).
C:N:P Stoichiometry Regulated by Si
N and P are vital nutrients for plant growth and biosynthesis of organic matter. C:N and C:P stoichiometry can represent the plants’ nutrient status in response to varying environmental conditions (Körner, 1989; Reich and Oleksyn, 2004). Previous studies have highlighted the important roles of N and P status, climate, and phylogeny in controlling C:N:P ecological stoichiometry (Wright et al., 2004; Kerkhoff et al., 2006; He et al., 2008; Olde Venterink and Gusewell, 2010; Song et al., 2014a; Li et al., 2018b). In this study, Si concentrations in the aboveground grass tissues were negatively correlated with grass C but positively with P, resulting in a significant negative correlation between grass Si and the C:P ratio. This may indicate that Si uptake could have a profound impact on the utilization of plant nutrients (Song et al., 2014a; Li et al., 2018b).
Moreover, N:P stoichiometry can be used to assess the nutrient balance of plants and whether N or P limits plant growth at the ecosystem level (Koerselman and Meuleman, 1996; Verhoeven et al., 1996; Elser et al., 2000; Güsewell et al., 2003; Güsewell, 2004). For example, N:P < 14 indicates that N limits the growth of plants, whereas N:P > 16 indicates P limitation (Koerselman and Meuleman, 1996; Aerts and Chapin, 1999). P is considered a major growth-limiting factor in the grasslands of Northern China (Han et al., 2005; He et al., 2008). Our analysis showed that N:P stoichiometry decreased with an increasing Si concentration (Figure 2F), suggesting that Si may partly enable plants to govern the nutrient balance and alleviate P deficiency in the grasslands of northern China. These findings may support the promising potential role of Si in grassland management.
Implications and Limitation of the Study
Although the biogeochemical cycles of C, N, and P influence most ecosystem processes (Chapin, 1980; Chapin et al., 1990; Hessen et al., 2004), Si plays a promising role in regulating the biogeochemical cycles of C, N, and P in grasslands (Conley, 2002; Blecker et al., 2006; Song et al., 2012a; Song et al., 2012b; Song et al., 2014a; Trinh et al., 2017). For example, the element release and CO2 consumption during silicate weathering and the sequestration of organic matter during the formation and accumulation phytolith in plants and soil.
In addition to these mechanisms, Si absorption from soil and deposition in grasses could also affect grassland C, N, and P cycles by controlling the synthesis of structural organic compounds and elemental stoichiometry. Increasing evidence shows that Si uptake by plants can enhance the accumulation of C and nutrient elements (Eneji et al., 2008; Song et al., 2014a; Neu et al., 2017; Li et al., 2018b). For example, Li et al. (2018a) summarized the positive effects of Si on total C accumulation in plants under different stresses in terrestrial ecosystems and found that Si-mediated recovery could potentially lead to a 35% increase in C accumulation. In line with these findings, the soil noncrystalline Si had significantly positively effect on plant C accumulation (Figure 3), which was caused by the significant increased aboveground biomass with total soil non-crystalline Si concentration in our study area (Figure 5). We suggest that the Si could promote the C, N, and P accumulation in plants, while with a certain biomass, C storage in aboveground parts of grassland plants would potentially decrease, and P storage would increase significantly due to Si uptake. However, these phenomena are not consistent among the four species in our study, and this inconsistency may result from different sample numbers and species characteristics. Besides, the insignificant relationships between climate and C, N, and P accumulation in plants might be partly caused by the relatively small differences in precipitation.
Si in soil and Si uptake in plants could not only affect C, N, and P concentrations and accumulations in fresh tissues but also influence the processes of plant litter decay since litter decomposition rates are closely related to the chemical composition of plant tissues, such as lignin concentrations and C, N, and P stoichiometry (Taylor and Parkinson, 1989; Gijsman et al., 1997; Koukoura et al., 2003). In grasslands, microorganisms in soils generally preferentially decompose plant litter with low lignin contents, low C:P ratios and high P concentrations (Zhang et al., 2008; Talbot and Treseder, 2012; Yang et al., 2014). Therefore, the positive effect of Si on the uptake of P over C as observed in the grass species in this study provides additional evidence that litter decomposition rates may increase significantly due to increased Si content (Schaller and Struyf, 2013; Marxen et al., 2016). However, the effects of increased Si incorporation on litter decay are complicated. For example, the protective effects of phytolith on litter may restrict the activity of fungal decomposers in soils (Schaller et al., 2014). Hence further research is needed to examine the relative importance of these opposing effects. Though the possible mechanism for Si regulating the C, N, or P cycles are discussed above, these are mainly based on previous researches of pot or field experiments and more mechanisms on natural ecosystems should be further investigated.
Conclusions
In this study, we assessed the possible impacts of Si on stoichiometry and accumulation of C, N, and P in grasses over large landscape scales in northern China. Results showed that C concentrations in aboveground grass tissues (ranging from 41.71% to 45.49%) were significantly negatively correlated with Si concentrations (0.23% to 2.27%). P concentrations ranged from 0.10% to 0.20%, positively correlating with Si concentrations, while the correlation between Si and N was not significant. Additionally, the C:P and N:P ratios were significantly negatively correlated with Si concentrations. Compared with other environmental factors, soil noncrystalline Si had significant influences on plant C, N, and P accumulations. These results indicate that Si contents in soil and deposition in grasses may influence organic C synthesis and adjust nutrient utilization. We also suggest that Si deposition may promote P absorption and mitigate the limitation of P, considering that the grassland soil in northern China is generally limited in P. Si may also play a promising role in affecting C, N, and P biogeochemical cycles in the grasslands and other terrestrial ecosystems that are dominated by Si-accumulating plants.
Data Availability Statement
All datasets generated for this study are included in the article/supplementary material.
Author Contributions
QH and SY analyzed the data, drew the figures, and wrote the draft of the manuscript. QH and ZS designed the experiment. ZS, ZL, FD, CY, GH, and HL supplied substantial, direct, and intellectual suggestions to the manuscript. All authors contributed to the article and approved the submitted version.
Funding
We acknowledge the support from the National Natural Science Foundation of China (41701049, 41930862 and 41571130042) and the State’s key Project of Research and Development Plan of China (2016YFA0601002 and 2017YFC0212703).
Conflict of Interest
The authors declare that the research was conducted in the absence of any commercial or financial relationships that could be construed as a potential conflict of interest.
References
Aerts, R., Chapin, F. S. (1999). The mineral nutrition of wild plants revisited: A re-evaluation of processes and patterns. Adv. Ecol. Res. 30, 1–67. doi: 10.1016/S0065-2504(08)60016-1
Blecker, S. W., McCulley, R. L., Chadwick, O. A., Kelly, E. F. (2006). Biologic cycling of silica across a grassland bioclimosequence. Global Biogeochem. Cy. 20, GB3023. doi: 10.1029/2006GB002690
Broadley, M., Brown, P., Cakmak, I., Ma, J. F., Rengel, Z., Zhao, F. J. (2012). “Beneficial elements,” in Marschner"s mineral nutrition of higher plants, 3rd ed. Ed. Marschner, P. (Beijing: Science Press), 249–269.
Carey, J. C., Fulweiler, R. W. (2012). The Terrestrial Silica Pump. PLoS One 7, e52932. doi: 10.1371/journal.pone.0052932
Chapin, F. S., Schulze, E. D., Mooney, H. A. (1990). The ecology and economics of storage in plants. Annu. Rev. Ecol. Syst. 21, 423–447. doi: 10.1146/annurev.es.21.110190.002231
Chapin, F. S. (1980). The Mineral Nutrition of Wild Plants. Annu. Rev. Ecol. Syst. 11, 233–260. doi: 10.1146/annurev.es.11.110180.001313
Conley, D. J. (2002). Terrestrial ecosystems and the global biogeochemical silica cycle. Global Biogeochem. Cy. 16, 68-1–68-8. doi: 10.1029/2002GB001894
Cooke, J., Leishman, M. R. (2012). Tradeoffs between foliar silicon and carbon-based defences: evidence from vegetation communities of contrasting soil types. OIKOS 121 (12), 2052–2060. doi: 10.1111/j.1600-0706.2012.20057.x
Cooke, J., Leishman, R. M. (2016). Consistent alleviation of abiotic stress with silicon addition: a meta-analysis. Funct. Ecol. 30, 1340–1357. doi: 10.1111/1365-2435.12713
Detmann, K. C., Araújo, W. L., Martins, S. C. V., Sanglard, L. M. V. P., Reis, J. V., Detmann, E., et al. (2012). Silicon nutrition increases grain yield, which, in turn, exerts a feed-forward stimulation of photosynthetic rates via enhanced mesophyll conductance and alters primary metabolism in rice. New Phytol. 196, 752–762. doi: 10.1111/j.1469-8137.2012.04299.x
Elser, J. J., Fagan, W. F., Denno, R. F., Dobberfuhl, D. R., Folarin, A., Huberty, A., et al. (2000). Nutritional constraints in terrestrial and freshwater food webs. Nature. 408, 578–580. doi: 10.1038/35046058
Eneji, A. E., Inanaga, S., Muranaka, S., Li, J., Hattori, T., An, P., et al. (2008). Growth and Nutrient Use in Four Grasses Under Drought Stress as Mediated by Silicon Fertilizers. J. Plant Nutr. 31, 355–365. doi: 10.1080/01904160801894913
Epstein, E. (1994). The anomaly of silicon in plant biology. Proc. Natl. Acad. Sci. U. S. A. 91, 11–17. doi: 10.1073/pnas.91.1.11
Fang, J. Y., Yang, Y. H., Ma, W. H., Mohammat, A., Shen, H. H. (2010). Ecosystem carbon stocks and their changes in China’s grasslands. Sci. China-Life Sci. 53, 757–765. doi: 10.1007/s11427-010-4029-x
Gijsman, A. J., Alarcón, H. F., Thomas, R. J. (1997). Root decomposition in tropical grasses and legumes, as affected by soil texture and season. Soil Biol. Biochem. 29, 1443–1450. doi: 10.1016/S0038-0717(97)00039-4
Güsewell, S., Koerselman, W., Verhoeven, J. T. A. (2003). Biomass N:P ratios as indicators of nutrient limitation for plant populations in wetlands. Ecol. Monogr. 13, 372–384. doi: 10.1890/1051-0761(2003)013[0372:BNRAIO]2.0.CO;2
Güsewell, S. (2004). N:P ratios in terrestrial plants: variation and functional significance. New Phytol. 164, 243–266. doi: 10.1111/j.1469-8137.2004.01192.x
Han, W. X., Fang, J. Y., Guo, D. L., Zhang, Y. (2005). Leaf nitrogen and phosphorus stoichiometry across 753 terrestrial plant species in China. New Phytol. 168, 377–385. doi: 10.1111/j.1469-8137.2005.01530.x
Hao, Q., Yang, S., Song, Z., Ran, X., Yu, C., Chen, C., et al. (2020). Holocene carbon accumulation in lakes of the current east Asian monsoonal margin: Implications under a changing climate. Sci. Total Environ. 737, 139723. doi: 10.1016/j.scitotenv.2020.139723
Hattori, T., Inanagaa, S., Arakib, H. (2005). Application of silicon enhanced drought tolerance in Sorghum bicolor. Physiol. Plantarum 123, 459–466. doi: 10.1111/j.1399-3054.2005.00481.x
He, J. S., Wang, L., Flynn, D. F. B., Wang, X. P., Ma, W. H., Fang, J. Y. (2008). Leaf nitrogen: phosphorus stoichiometry across Chinese grassland biomes. Oecologia 2008 (155), 301–310. doi: 10.1007/s00442-007-0912-y
He, C. W., Ma, J., Wang, L. J. (2015). A hemicellulose-bound form of silicon with potential to improve the mechanical properties and regeneration of the cell wall of rice. New Phytol. 206, 1051–1062. doi: 10.1111/nph.13282
Hessen, D. O., Agren, G., II, Anderson, T. R., Elser, J. J., Ruiter, P. C. D. (2004). Carbon Sequestration in Ecosystems: The Role of Stoichiometry. Ecology 85, 1179–1192. doi: 10.1890/02-0251
Hou, X. Y. (1982). Vegetation Geography of China and Chemical Composition of its Dominant Plants (Beijing: Science Press of China).
Hu, A. Y., Che, J., Shao, J. F., Yokosho, K., Zhao, X. Q., Shen, R. F., et al. (2018). Silicon accumulated in the shoots results in down-regulation of phosphorus transporter gene expression and decrease of phosphorus uptake in rice. Plant Soil 423, 317–325. doi: 10.1007/s11104-017-3512-6
Jiang, G., Han, X., Wu, J. (2006). Restoration and management of the Inner Mongolia grassland require a sustainable strategy. Ambio. 35 (5), 269–270. doi: 10.1579/06-S-158.1
Kerkhoff, A. J., Fagan, W. F., Elser, J. J., Enquist, B. J. (2006). Phylogenetic and growth form variation in the scaling of nitrogen and phosphorus in the seed plants. Am. Nat. 168, E103–E122. doi: 10.1086/507879
Klotzbücher, T., Klotzbücher, A., Kaiser, K., Vetterlein, D., Jahn, R., Mikutta, R. (2018). Variable silicon accumulation in plants affects terrestrial carbon cycling by controlling lignin synthesis. Global Change Biol. 24, e183–e189. doi: 10.1111/gcb.13845
Koerselman, W., Meuleman, A. F. M. (1996). The vegetation N:P ratio: a new tool to detect the nature of nutrient limitation. J. Appl. Ecol. 33, 1441–1450. doi: 10.2307/2404783
Körner, C. (1989). The nutrient status of plants from high altitudes: a worldwide comparison. Oecologia 81, 379–391. doi: 10.1007/BF00377088
Kostic, L., Nikolic, N., Bosnic, D., Samardzic, J., Nikolic, M. (2017). Silicon increases phosphorus (P) uptake by wheat under low P acid soil conditions. Plant Soil 419, 447–455. doi: 10.1007/s11104-017-3364-0
Koukoura, Z., Mamolos, A. P., Kalburtji, K. L. (2003). Decomposition of dominant plant species litter in a semi-arid grassland. Appl. Soil Ecol. 23, 13–23. doi: 10.1016/s0929-1393(03)00006-4
Kurtz, A. C., Derry, L. A., Chadwick, O. A. (2002). Germanium-silicon fractionation in the weathering environment. Geochim Cosmochim Acta 66, 1525–1537. doi: 10.1016/S0016-7037(01)00869-9
Lehmann, J., Kleber, M. (2015). The contentious nature of soil organic matter. Nature. 528, 60–68. doi: 10.1038/nature16069
Li, Z. M., Delvaux, B. (2019). Phytolith-rich biochar: A potential Si fertilizer in desilicated soils. GCB Bioenergy 11, 1264–1282. doi: 10.1111/gcbb.12635
Li, Z. M., Song, Z. L., Parr, J. F., Wang, H. L. (2013). Occluded C in rice phytoliths: implications to biogeochemical carbon sequestration. Plant Soil 370, 615–623. doi: 10.1007/s11104-013-1661-9
Li, Z. C., Song, Z. L., Yan, Z. F., Hao, Q., Song, A. L., Liu, L. N., et al. (2018a). Silicon enhancement of estimated plant biomass carbon accumulation under abiotic and biotic stresses. A meta-analysis. Agron. Sustain. Dev. 38, 26. doi: 10.1007/s13593-018-0496-4
Li, Z. C., Song, Z. L., Yang, X. M., Song, A. L., Yu, C. X., Tao, W., et al. (2018b). Impacts of silicon on biogeochemical cycles of carbon and nutrients in croplands. J. Integr. Agr. 17, 2182–2195. doi: 10.1016/S2095-3119(18)62018-0
Li, Z. M., Unzué-Belmonte, D., Cornelis, J. T., Vander Linden, C., Struyf, E., Ronsse, F., et al. (2019). Effects of phytolithic rice-straw biochar, soil buffering capacity and pH on silicon bioavailability. Plant Soil. 438, 187–203. doi: 10.1007/s11104-019-04013-0
Liang, Y. C., Sun, W. C., Zhu, Y. G., Christie, P. (2007). Mechanisms of silicon mediated alleviation of abiotic stresses in higher plants: a review. Environ. Pollut. 147, 422–428. doi: 10.1016/j.envpol.2006.06.008
Liang, Y. C., Nikolic, M., Bélanger, R., Gong, H. J., Song, A. L. (2015). Silicon in Agriculture. (Netherlands: Springer). doi: 10.1007/978-94-017-9978-2
Liu, H. Y., Yin, Y., Tian, Y. H., Ren, J., Wang, H. Y. (2008). Climatic and anthropogenic controls of topsoil features in the semi-arid East Asian steppe. Geophys. Res. Lett. 35, 222–256. doi: 10.1029/2007GL032980
Ma, J. F., Takahashi, E. (1990). Effect of silicon on the growth and phosphorus uptake of rice. Plant Soil 126, 115–119. doi: 10.1007/bf00041376
Ma, J. F., Yamaji, N. (2006). Silicon uptake and accumulation in higher plants. Trends Plant Sci. 11, 392–397. doi: 10.1016/j.tplants.2006.06.007
Ma, J. F., Yamaji, N. A. (2015). A cooperative system of silicon transport in plants. Trends Plant Sci. 20, 435–442. doi: 10.1016/j.tplants.2015.04.007
Ma, J. F. (2004). Role of silicon in enhancing the resistance of plants to biotic and abiotic stresses. Soil Sci. Plant Nutr. 50, 11–18. doi: 10.1080/00380768.2004.10408447
Marxen, A., Klotzbucher, T., Jahn, R., Kaiser, K., Nguyen, V. S. (2016). Interaction between silicon cycling and straw decomposition in a silicon deficient rice production system. Plant Soil. 398, 153–163. doi: 10.1007/s11104-015-2645-8
Neu, S., Schaller, J., Dudel, E. G. (2017). Silicon availability modifies nutrient use efficiency and content, C:N:P stoichiometry, and productivity of winter wheat (Triticum aestivum L.). Sci. Rep-UK. 7, 40829. doi: 10.1038/srep40829
Olde Venterink, H., Gusewell, S. (2010). Competitive interactions between two meadow grasses under nitrogen and phosphorus limitation. Funct. Ecol. 24, 877–886. doi: 10.1111/j.1365-2435.2010.01692.x
Raven, J. A. (2010). The transport and function of silicon in plants. Biol. Rev. 58, 179–207. doi: 10.1111/j.1469-185X.1983.tb00385.x
Reich, P. B., Oleksyn, J. (2004). Global patterns of plant leaf N and P in relation to temperature and latitude. Proc. Natl. Acad. Sci. U. S. A. 101, 11001–11006. doi: 10.1073/pnas.0403588101
Rios, J. J., Martinez-Ballesta, M., Ruiz, J. M., Blasco Leòn, B., Carvajal, M. (2017). Silicon-mediated improvement in plant salinity tolerance: the role of aquaporins. Front. Plant Sci. 8, 948. doi: 10.3389/fpls.2017.00948
Schaller, J., Struyf, E. (2013). Silicon controls microbial decay and nutrient release of grass litter during aquatic decomposition. Hydrobiologia. 709, 201–212. doi: 10.1007/s10750-013-1449-1
Schaller, J., Brackhage, C., Dudel, E. G. (2012a). Silicon availability changes structural carbon ratio and phenol content of grasses. Environ. Exp. Bot. 77, 283–287. doi: 10.1016/j.envexpbot.2011.12.009
Schaller, J., Brackhage, C., Gessner, M. O., Bauker, E., Dudel, E. G. (2012b). Silicon supply modifies C:N:P stoichiometry and growth of Phragmites australis. Plant Biol. 14, 392–396. doi: 10.1111/j.1438-8677.2011.00537.x
Schaller, J., Hines, J., Brackhage, C., Bäuker, E., Gessner, M. O. (2014). Silica decouples fungal growth and litter decomposition without changing responses to climate warming and N enrichment. Ecology. 95, 3181–3189. doi: 10.1890/13-2104.1
Schaller, J., Schoelynck, J., Struyf, E., Meire, P. (2016). Silicon Affects Nutrient Content and Ratios of Wetland Plants. Silicon 8, 479–485. doi: 10.1007/s12633-015-9302-y
Schmidt, M. W., II, Torn, M. S., Abiven, S., Dittmar, T., Guggenberger, G., Janssens, I. A., et al. (2011). Persistence of soil organic matter as an ecosystem property. Nature 478, 49–56. doi: 10.1038/nature10386
Schoelynck, J., Bal, K., Backx, H., Okruszko, T., Meire, P., Struyf, E. (2010). Silica uptake in aquatic and wetland macrophytes: a strategic choice between silica, lignin and cellulose? New Phytol. 186, 385–391. doi: 10.1111/j.1469-8137.2009.03176.x
Scurlock, J. M. O., Hall, D. O. (1998). The global carbon sink: A grassland perspective. Global Change Biol. 4, 229–233. doi: 10.1046/j.1365-2486.1998.00151.xt
Song, Z. L., Liu, H. Y., Si, Y., Yin, Y. (2012a). The production of phytoliths in China’s grasslands: implications to the biogeochemical sequestration of atmospheric CO2. Global Change Biol. 18, 3647–3653. doi: 10.1111/gcb.12017
Song, Z. L., Wang, H. L., Strong, P. J., Li, Z. M., Jiang, P. K. (2012b). Plant impact on the coupled terrestrial biogeochemical cycles of silicon and carbon: Implications for biogeochemical carbon sequestration. Earth-Sci Rev. 115, 319–331. doi: 10.1016/j.earscirev.2012.09.006
Song, Z. L., Liu, H. Y., Zhao, F. J., Xu, C. Y. (2014a). Ecological stoichiometry of N: P: Si in China’s grasslands. Plant Soil. 380, 165–179. doi: 10.1007/s11104-014-2084-y
Song, Z. L., Wang, H., Strong, P. J., Shan, S. (2014b). Increase of available soil silicon by Si-rich manure for sustainable rice production. Agron. Sustain Dev. 34, 813–819. doi: 10.1007/s13593-013-0202-5
Talbot, J. M., Treseder, K. K. (2012). Interactions among lignin, cellulose, and nitrogen drive litter chemistry-decay relationships. Ecology. 93, 345–354. doi: 10.1890/11-0843.1
Taylor, B. R., Parkinson, D. (1989). Nitrogen and Lignin Content as Predictors of Litter Decay Rates: A Microcosm Test. Ecology. 70, 97–104. doi: 10.2307/1938416
Trinh, T. K., Nguyen, T. T. H., Nguyen, T. N., Wu, T. Y., Meharg, A. A., Nguyen, M. N. (2017). Characterization and dissolution properties of phytolith occluded phosphorus in rice straw. Soil Till. Res. 171, 19–24. doi: 10.1016/j.still.2017.04.002
Van Bockhaven, J., De Vleesschauwer, D., Höfte, M. (2013). Towards establishing broad-spectrum disease resistance in plants: silicon leads the way. J. Exp. Bot. 64, 1281–1293. doi: 10.1093/jxb/ers329
Verhoeven, J. T. A., Koerselman, W., Meuleman, A. F. M. (1996). Nitrogen or phosphorus-limited growth in herbaceous, wet vegetation: relations with atmospheric inputs and management regimes. Trends Ecol. Evol. 11, 494–497. doi: 10.1016/S0169-5347(96)10055-0
White, A. F., Vivit, D. V., Schulz, M. S., Bullen, T. D., Evett, R. R., Aagarwal, J. (2012). Biogenic and pedogenic controls on Si distributions and cycling in grasslands of the Santa Cruz soil chronosequence, California. Geochim. Cosmochim. Ac. 94, 72–94. doi: 10.1016/j.gca.2012.06.009
Wright, I. J., Reich, P. B., Westoby, M., Ackerly, D. D., Baruch, Z. (2004). The worldwide leaf economics spectrum. Nature 428, 821–827. doi: 10.1038/nature02403
Wu, X., Li, Z. S., Fu, B. J., Zhou, W. M., Liu, H. F., Liu, G. H. (2014). Restoration of ecosystem carbon and nitrogen storage and microbial biomass after grazing exclusion in semi-arid grasslands of Inner Mongolia. Ecol. Eng. 73, 395–403. doi: 10.1016/j.ecoleng.2014.09.077
Xu, D. H., Fang, X. W., Zhang, R. Y., Gao, T. P., Bu, H. Y., Du, G. Z. (2015). Influences of nitrogen, phosphorus and silicon addition on plant productivity and species richness in an alpine meadow. AoB Plants 7, 19–34. doi: 10.1093/aobpla/plv125
Xu, D. H., Gao, X. G., Gao, T. P., Mou, J., Li, J. H., Bu, H. Y., et al. (2018). Interactive effects of nitrogen and silicon addition on growth of five common plant species and structure of plant community in alpine meadow. Catena 169, 80–89. doi: 10.1016/j.catena.2018.05.017
Yang, Y. H., Fang, J. Y., Ji, C. J., Datta, A., Li, P., Ma, W. H., et al. (2014). Stoichiometric shifts in surface soils over broad geographical scales: evidence from China’s grasslands. Global Ecol. Biogeogr. 23, 947–955. doi: 10.1111/geb.12175
Yang, S. L., Hao, Q., Liu, H. Y., Zhang, X. D., Yu, C. X., Yang, X. M., et al. (2019). Impact of grassland degradation on the distribution and bioavailability of soil silicon: Implications for the Si cycle in grasslands. Sci. Total Environ. 657, 811–818. doi: 10.1016/j.scitotenv.2018.12.101
Keywords: carbon cycles, grasslands, silicon, nutrient utilization, stoichiometry, P deficiency
Citation: Hao Q, Yang S, Song Z, Li Z, Ding F, Yu C, Hu G and Liu H (2020) Silicon Affects Plant Stoichiometry and Accumulation of C, N, and P in Grasslands. Front. Plant Sci. 11:1304. doi: 10.3389/fpls.2020.01304
Received: 07 February 2020; Accepted: 11 August 2020;
Published: 27 August 2020.
Edited by:
Jian-Guo Huang, Chinese Academy of Sciences, ChinaReviewed by:
Xiaoan Zuo, Chinese Academy of Sciences, ChinaJian Sun, Chinese Academy of Sciences, China
Xiali Guo, Chinese Academy of Sciences, China
Copyright © 2020 Hao, Yang, Song, Li, Ding, Yu, Hu and Liu. This is an open-access article distributed under the terms of the Creative Commons Attribution License (CC BY). The use, distribution or reproduction in other forums is permitted, provided the original author(s) and the copyright owner(s) are credited and that the original publication in this journal is cited, in accordance with accepted academic practice. No use, distribution or reproduction is permitted which does not comply with these terms.
*Correspondence: Zhaoliang Song, zhaoliang.song@tju.edu.cn
†These authors have contributed equally to this work