- 1Sustainable Agricultural Systems Laboratory, The Henry A. Wallace Beltsville Agricultural Research Center, United States Department of Agriculture-ARS, Beltsville, MD, United States
- 2Soybean Genomics and Improvement Laboratory, The Henry A. Wallace Beltsville Agricultural Research Center, United States Department of Agriculture-ARS, Beltsville, MD, United States
Heat shock proteins (HSPs) are ubiquitous and highly conserved in nature. Heat stress upregulates their gene expression and now it is known that they are also developmentally regulated. We have studied regulation of small HSP genes during ripening of tomato fruit. In this study, we identify two small HSP genes, SlHSP17.7A and SlHSP17.7B, localized on tomato Chr.6 and Chr.9, respectively. Each gene encodes proteins constituting 154 amino acids and has characteristic domains as in other sHSP genes. We found that SlHSP17.7A and SlHSP17.7B gene expression is low in the vegetative tissues as compared to that in the fruit. These sHSP genes are characteristically expressed in a fruit-ripening fashion, being upregulated during the ripening transition of mature green to breaker stage. Their expression patterns mirror that of the rate-limiting ethylene biosynthesis gene ACC (1-aminocyclopropane-1-carboxylic acid) synthase, SlACS2, and its regulator SlMADS-RIN. Exogenous application of ethylene to either mature green tomato fruit or tomato leaves suppressed the expression of both the SlHSP17.7A, B genes. Notably and characteristically, a transgenic tomato line silenced for SlACS2 gene and whose fruits produce ~50% less ethylene in vivo, had higher expression of both the sHSP genes at the fruit ripening transition stages [breaker (BR) and BR+3] than the control fruit. Moreover, differential gene expression of SlHSP17.7A versus SlHSP17.7B gene was apparent in the tomato ripening mutants—rin/rin, nor/nor, and Nr/Nr, with the expression of SlHSP17.7A being significantly reduced but that of SlHSP17.7B significantly upregulated as compared to the wild type (WT). These data indicate that ethylene negatively regulates transcriptional abundance of both these sHSPs. Transient overexpression of the ripening regulator SlMADS-RIN in WT and ACS2-AS mature green tomato fruits suppressed the expression of SlHSP17.7A but not that of SlHSP17.7B. Thus, ethylene directly or in tune with SlMADS-RIN regulates the transcript abundance of both these sHSP genes.
Introduction
Heat shock proteins (HSPs) are ubiquitous in nature and highly conserved in living organisms (Plesofsky-Vig et al., 1992; Waters and Vierling, 1999; Kappé et al., 2002; Franck et al., 2004; Fu et al., 2006; Aevermann and Waters, 2008; Waters, 2013). They are prominent in cells/tissues exposed to elevated temperatures (Sun et al., 2002; Waters, 2013; Zhang et al., 2015) as well as to chilling temperatures (Ré et al., 2016). HSPs are classified based on their molecular weight, namely, HSP100s, HSP90s, HSP70s, HSP60s, HSP20s and small HSPs (sHSPs) (Waters, 2013). sHSPs constitute low molecular weight proteins that function as molecular chaperones critical for protein folding and prevention of irreversible protein aggregation (Becker and Craig, 1994; Hartl, 1996; Liberek et al., 2008; Giorno et al., 2010; Tyedmers et al., 2010; Waters, 2013). In addition to sHSPs being prominently expressed during heat shock response in plants, it is now known that some are expressed in unstressed cells as well and are, therefore, involved in processes other than heat stress (Tyedmers et al., 2010; Waters, 2013). For example, in plants, they are upregulated during ripening initiation of tomato fruit (Goyal et al., 2012; Shukla et al., 2017) and may also protect ripe tomato fruits against chilling injury (Ré et al., 2016).
Ethylene regulates plant processes such as fruit ripening independently as well as in conjunction with other hormones and molecules (Fluhr and Mattoo, 1996; Giovannoni et al., 2017; Mattoo and Upadhyay, 2019). Ethylene directly or indirectly promotes transcription/translation of numerous ripening-related genes, including those associated with cell wall breakdown, carotenoid biosynthesis, aroma development, pigment accumulation, fruit softening, and flavor (Gray et al., 1994; Barry and Giovannoni, 2007; Klee and Giovannoni, 2011). Tomato is one of the models for dissection of ethylene-mediated regulation of genes during fruit ripening, facilitated by the availability of nonripening tomato lines, such as ripening-inhibitor (rin/rin), nonripening (nor/nor), and never-ripe (Nr/Nr), in which ethylene production is compromised (Mattoo and Vickery, 1977; Oeller et al., 1991; Picton et al., 1993; Wilkinson et al., 1995; Hackett et al., 2000; Alexander and Grierson, 2002; Moore et al., 2002; Vrebalov, 2002; Giovannoni, 2007; Razdan and Mattoo, 2007). Of these mutants, ripening-inhibitor (rin) mutation encodes a MADS-box transcription factor SlMADS-RIN that regulates genes involved in fruit ripening (Ng and Yanofsky, 2001; Vrebalov, 2002; Hileman et al., 2006; Martel et al., 2011; Fujisawa et al., 2013).
Tomato genome harbors five members of class I sHSP genes (Goyal et al., 2012; Yu et al., 2016; Shukla et al., 2017). The involvement of sHSPs in fruit biology became apparent by earlier studies which demonstrated VISCOSITY 1 (VIS1) as a regulator of pectin depolymerization affecting juice viscosity of tomato fruit (Ramakrishna et al., 2003) while HSP21 was shown to stabilize photosystem II of photosynthesis against oxidative stress in addition to promoting color change during tomato fruit ripening (Neta-Sharir et al., 2005). More recently, a unique intron-less cluster of three sHSP chaperone genes, SlHSP17.6, SlHSP20.0 and SlHSP20.1, was shown to be resident on the short arm of chromosome 6 and found differentially expressed during tomato fruit ripening (Goyal et al., 2012). Further, it was shown that ethylene suppresses the transcription of the latter tomato sHSP gene cluster during the transition of mature green stage to ripening initiation and involves SlMADS-RIN box protein (Shukla et al., 2017).
Here we identify, characterize and present transcriptional regulation of two novel duplicated members of class I sHSPs in tomato, SlHSP17.7A and SlHSP17.7B, during fruit ripening. Further, expression of SlHSP17.7A gene was found minimal in the isogenic ripening mutants of Alisa Craig—rin/rin, nor/nor, and Nr/Nr, while that of SlHSP17.7B was higher in these mutants. We also utilized an ethylene-deficient tomato line to demonstrate that ethylene biosynthesis directly and/or indirectly regulates the expression of sHSP genes in tomato.
Materials and Methods
Plant Materials, Transgenic Tomato Lines, and Sample Collection
Wild-type tomato (Solanum lycopersicum cv. Ailsa Craig) and its near isogenic mutant lines—ripening-inhibitor (rin/rin), nonripening (nor/nor) and never-ripe (Nr/Nr)—and previously characterized ACC synthase 2 (ACS2)-silenced transgenic line (ACS2-AS) together with its azygous/wild-type control tomato (WT-Ohio8245) (Mehta et al., 2002; Sobolev et al., 2014) were employed for the studies presented here. sHSP gene expression was quantified at distinct ripening stages: mature green (-5BR), breaker (BR), pink (breaker+3), and red ripe (breaker+7). All these genotypes were grown in a temperature-controlled greenhouse under natural light conditions. Vegetative tissues, leaf, stem, flower, and roots, were collected from 1-month-old wild-type Ailsa Craig tomato plants. For seedling collection, 8- to 10-day-old 50-germinated seeds in triplicates with two cotyledons were immediately frozen in liquid nitrogen and stored at −80°C until used. For development studies, fruits tagged at anthesis (DPA: days post anthesis) were collected at 8 DPA (developmental stage 1), 15 DPA (developmental stage 2), and 22 DPA (developmental stage 3). For ripening studies, tomato fruits at 5 days before breaker (-)5BR—equivalent to mature green (MG) stage, breaker (BR) and red ripe stage [8 days after breaker (BR+8)] were collected. Fruits from the WT-Ohio8245 were harvested at (-)5BR, (MG), BR, and BR+8 stages. Pericarp tissue excised from harvested fruits was immediately frozen in liquid nitrogen and stored at −80°C until used (Mehta et al., 2002). A minimum of 3 biological replicates were used for each experiment.
Identification, Sequence Alignment and Phylogenetic Analysis of SlHSP17.7A and SlHSP17.7B
Two novel ripening-specific genes SlHSP17.7A (NM_001279116.2/Solyc06g076520.1) and SlHSP17.7B (XM_015231817.1/Solyc09g015020.1) were identified by utilizing prior information on the following genes: SlHSP17.6 (AY150039/Solyc06g076540.1.1), SlHSP20.0 (AJ225048/Solyc06g076570.1.1), and SlHSP20.1 (AJ225046/Solyc06g076560.1.1) (Goyal et al., 2012; Shukla et al., 2017). The latter tomato HSPs were used as query sequences for BLAST P search in gene bank (https://blast.ncbi.nlm.nih.gov) as well as with BLAST P program in tomato genome [International Tomato Genome Sequencing Consortium (SGN; solgenomics.net) database, version ITAG 2.4] to look for similar sequences. ExPASy bioinformatics resource (https://www.expasy.org) portal was used for the prediction of molecular weight and isoelectric point (PI). Individual domains in the protein sequences were identified and manually highlighted. Multiple sequence alignment was performed using MUSCLE program (http://www.ebi.ac.uk/Tools/msa) and primers were designed using Primer3 program (http://bioinfo.ut.ee/primer3-0.4.0/). Forty-two similar sequences were extracted from tomato genome database to generate the phylogeny among tomato sHSPs (Goyal et al., 2012; Paul et al., 2016; Yu et al., 2016; Shukla et al., 2017; Supplementary Table 1). The phylogenetic tree was constructed by maximum likelihood method based on JTT matrix model with 1000 boot strap values using Mega 7 program (Kumar et al., 2016). The analysis involved 42 amino acid sequences. All positions with less than 95% site coverage were eliminated. For qRT-PCR analysis, forward primer was made from 3′ coding DNA sequence while reverse primer was designed from 3′ UTR due to sequence degeneracy within the five closely related class I tomato HSP members. Primer sequences used in qRT-PCR along with their gene bank accessions and SGN identity numbers are listed in Supplementary Table 2.
Exogenous Ethylene and 1-Methylcyclopropene Treatments
Mature green [MG/(-)5BR] tomato fruits from WT-Ohio8245 control and ACS2-AS transgenic lines were treated with 25 ppm ethylene or 2 ppm 1-Methylcyclopropene (1-MCP) (AgroFresh, Goyal et al., 2012 Collegeville, PA, USA) in triplicate from three independent biological replicates (Shukla et al., 2017). A third set of fruits left in the open air was considered as a control for the experiment. Treated and control fruit were collected at 0, 12, and 24 h of treatment. Ethylene (25 ppm) treatment of 8–10 fully expanded leaves from mature WT-Ailsa Craig plants was carried out for 0, 24, 48, 72, and 96 h in the dark at 25°C. Samples were removed at the indicated time points and immediately flash frozen at −80°C until used.
Total RNA Extraction, cDNA Synthesis, and Quantitative Real-Time PCR (qRT-PCR)
Total RNA was extracted from 100 mg of each sample using Plant RNeasy kit according to manufacturer’s instructions (Qiagen). Isolated RNA was first subjected to RNase-free DNase (Qiagen) treatment to eliminate genomic DNA contamination and then purified using a RNeasy Mini Kit (Qiagen). RNA samples with an A260/280 ratio of 1.8–2 were electrophoresed on agarose gels to ensure the presence of intact rRNA bands (Goyal et al., 2012). Methods for cDNA synthesis and qRT-PCR were essentially as described previously (Bustin et al., 2009; Shukla et al., 2017; Upadhyay et al., 2017). Relative gene expression was quantified according to 2−ΔΔCT method (Livak and Schmittgen, 2001). Tomato genes SlTIP41 and SlUBI3 were used as standard housekeeping genes to normalize the expression of target genes (Expósito-Rodríguez et al., 2008; Mascia et al., 2010). For relative expression data, the threshold cycle (CT) values for all the genes of interest (CT of GOI) were normalized to the geometric mean CT value obtained from the two tomato reference genes (CT tom Refs) as ΔCT = (CT of GOI) − (geometric mean CT of SlTIP41 and SlUBI3) (Vandesompele et al., 2002). For fruit ripening studies, mature green/(-)5BR was taken as a calibrator to calculate the 2−ΔΔCT for relative gene expression values. Primer efficiency was calculated for each primer pair as: efficiency=10^(-1/slope). Primer pairs with primer efficiency above 90% were used for the study. qRT-PCR data represent average ± standard deviation from a minimum of three independent biological replicates for each gene.
In Silico Analysis of SlHSP17.7A and SlHSP17.7B Promoters for Predicting cis-Elements
International Tomato Genome Sequencing Consortium (SGN; solgenomics.net) database (version ITAG 2.4) was used to extract promoter region sequences (≈ 2 kb of the 5′ upstream region of the start codon) of SlHSP17.7A and SlHSP17.7B genes. Plant CARE relational database (Lescot et al., 2002) and PLACE (the plant-cis-acting regulatory DNA elements) database (Higo et al., 1999) were used for plant cis-element searches in both the gene promoters (Supplementary Tables 3 and 4).
Overexpression, Construct Preparation, and Agro-Injection of Tomato Fruits for Transient Expression Analysis
For overexpression, SlMADS-RIN coding sequence was amplified from breaker stage cDNA and then cloned into the pENTR/D-TOPO donor vector (Invitrogen). It was further subcloned into the pK2GW7 gateway plant destination vector to generate a plasmid-designated as pK2GW7-SlMADS-RIN-OE, and then transformed into agrobacterium GV3101pmp90RK strains. Mature green fruits from wild-type and ethylene-deficient genotypes were chosen for agroinfiltration and subsequent expression analysis. Agrobacterium culture carrying pK2GW7-SlMADS-RIN-OE was grown overnight at 28°C in Luria-Bertani (Sigma) medium with selective antibiotics (gentamycin, rifampicin and kanamycin). Primary culture (500 μl) was then transferred to a 50-ml modified induction medium (2 mM MgSO4, 20 mM acetosyringone, 10 mM MES, pH 5.6) plus antibiotics, and grown overnight until optical density reached 1.0. The culture was centrifuged at 8,000 rpm for 5 min, resuspended in the infiltration medium (10 mM MgCl2, 10 mM MES, 200 mM acetosyringone, pH 5.6), and incubated at room temperature with gentle agitation (50 rpm) for a minimum of 2 h. Tomato agro-injection was done as described earlier (Orzaez et al., 2006). Briefly, 3–4 mature green tomato fruits from each genotype were infiltrated using a 2-ml syringe with needle (BD Biosciences). Needle was introduced to a depth of 3 to 4 mm into the fruit tissue through the stylar apex, and the infiltrated solution was gently injected into the fruit. The total volume of solution injected varied with the size of the mature green tomato fruit, from 600 μl to 1 ml. The completely infiltrated fruits were used for the experiments. Thereafter, fruits were harvested after 72–96 h of infiltration. RNA from agro-injected fruit samples was isolated and used to make cDNA as described previously (Shukla et al., 2017). cDNA was diluted 10-fold for qRT-PCR analysis. pK2GW7-SlMADS-RIN infiltrated fruits and control fruits infiltrated with infiltration medium were analyzed for the accumulation of SlMADS-RIN, SlACS2 and both sHSP gene transcripts.
Data Analysis and Statistics
Statistical analysis was carried out using Graph Pad (version Prism 8.0) and P values < 0.05 treated as statistically significant as described previously (Upadhyay and Mattoo, 2018). Significant differences between fruit ripening stages were calculated by separately comparing mature green/(-)5BR stage with breaker (BR), breaker+3 (BR+3), and breaker+8 (BR+8) stages for each gene. Similarly, significant differences between wild-type and ripening stages of mutants were calculated by comparing BR, BR+3 and BR+8 stages of AC/AC with respective stages of rin/rin, nor/nor, and Nr/Nr. Significant changes for SlHSP17.7 transcripts in the in vitro ethylene suppression experiment were calculated by comparing air and ethylene treated samples at 12 and 24 h.
Results
Identification and Phylogenetic Analysis of Tomato SlHSP17.7A, B Class I HSP Genes
Bioinformatics analysis carried out as described in the Materials and Methods section revealed two novel class I intron-less sHSP genes which are organized similarly to a cluster of three sHSP genes described previously (Goyal et al., 2012). The two novel proteins were named SlHSP17.7A (Solyc06g076520.1.1) and SlHSP17.7B (Solyc09g015020.1.1), respectively. While this work was in progress, two genome-wide studies identified HSP20-related gene family in tomato (Paul et al., 2016; Yu et al., 2016). These studies indicated that SlHSP17.7A was housed on chromosome 6, and its close relative was identified as SlHSP17.7B housed on chromosome 9 of tomato. SlHSP17.7A gene (NM_001279116.2) constitutes 798 nucleotides with a coding DNA sequence (CDS) of 495 nucleotides with 127 nucleotides of 5′ UTR and 206 nucleotides of 3′ UTR. It encodes a functional protein of 154 amino acids, with a predicted molecular weight of 17,735.12 Daltons and PI 5.84 (Figure 1A). Like the SlHSP17.7A gene, the SlHSP17.7B gene (XM_015231817.1) constitutes 813 nucleotides with a coding DNA sequence (CDS) of 495 nucleotides along with 176 nucleotides of 5′ UTR and 172 nucleotides of 3′ UTR. The SlHSP17.7B CDS encodes a functional protein of 154 amino acids, with a predicted molecular weight of 17,662.01 Daltons and PI 5.84 (Figure 1A). The gene bank sequences for SlHSP17.7A (NM_001279116.2) and SlHSP17.7B (XM_015231817.1) were annotated with both untranslated regions (UTRs) while SGN sequence for SlHSP17.7A (Solyc06g076520.1) has yet to be annotated for positioning the 5′ and 3′ UTRs. Further, neither SlHSP17.7A (NM_001279116.2), (submitted in gene bank, Fray et al., 1990), nor SlHSP17.7 B (XM_015231817.1) have been so far characterized.
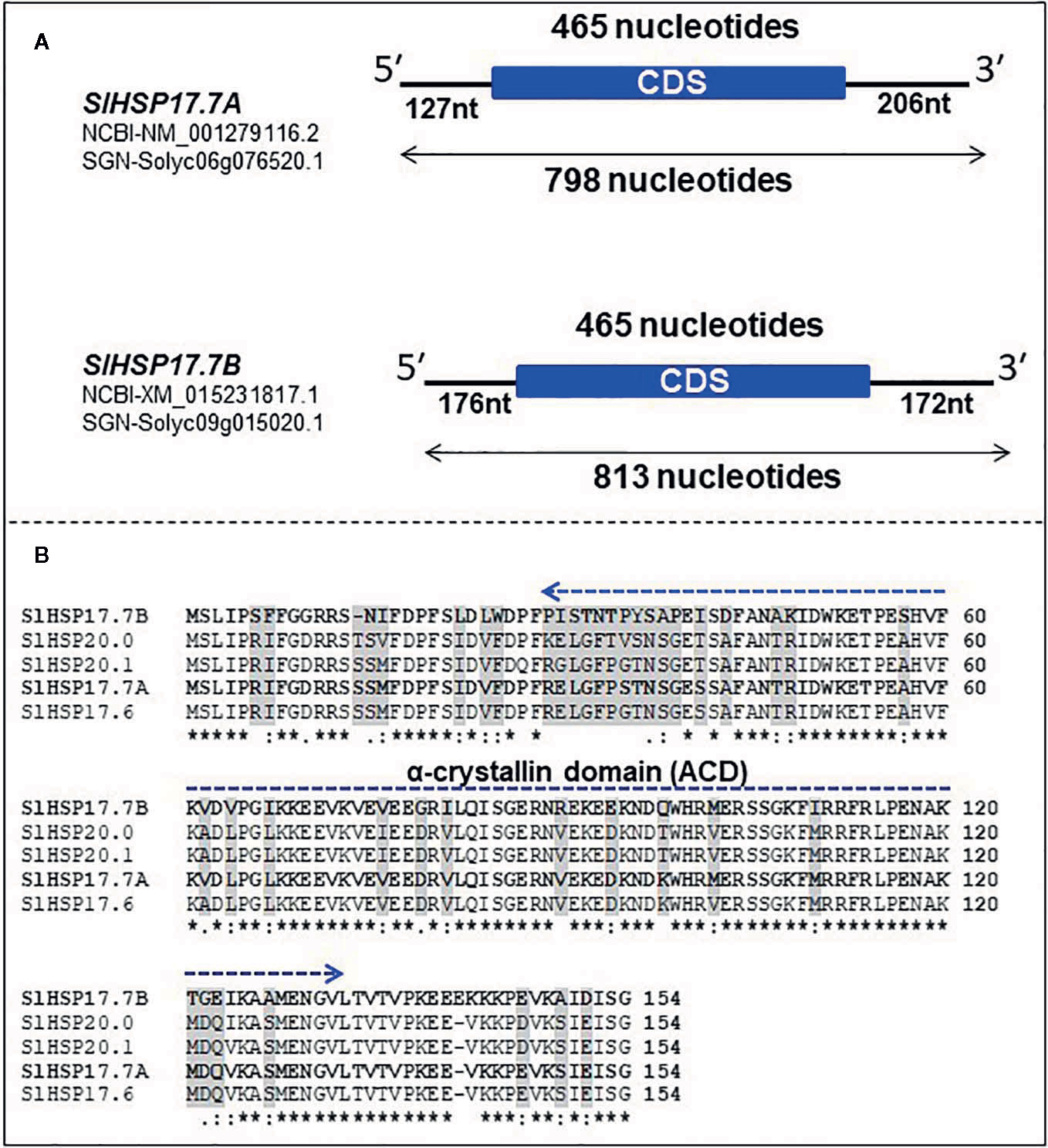
Figure 1 Organization, alignment and phylogeny of SlHSP17.7A and SlHSP17.7B genes. (A) Schematic representation of heat shock protein (HSP) genes with coding DNA sequence (CDS) and untranslated regions (UTRs). SlHSP17.7A gene (NM_001279116.2/Solyc06g076520.1) is made of 798 nucleotides with a CDS of 465 nucleotides, and 127 nucleotides of 5′ UTR, and 206 nucleotides of 3′ UTR. The SlHSP17.7 CDS encodes a functional protein of 154 amino acids. Similarly, SlHSP17.7B gene (XM_015231817.1/Solyc09g015020.1) is made of 813 nucleotides with CDS of 465 nucleotides, and 176 nucleotides of 5′ UTR and 172 nucleotides of 3′ UTR. The SlHSP17.7B CDS encodes a functional protein of 154 amino acids. (B) Alignment of SlHSP17.7A and SlHSP17.7B to their closest homologs SlHSP17.6, SlHSP20.0 and SlHSP20.1—different key conserved amino acid positions are shown. The characteristic conserved α-crystallin domain (ACD) is highlighted by dark blue line. Conserved residues are denoted by asterisks.
Alignment of protein sequences revealed that both the SlHSP17.7A and SlHAP17.7B proteins differ from the other three close homologs, SlHSP17.6, SlHSP20.0 and SlHSP20.1, at 14 amino acid positions (namely, 14, 16, 27, 29, 34, 35, 36, 41, 62, 76, 98, 102, 124, and 146) (N→C) (Figure 1B). To decode evolutionary relationship among tomato sHSPs, a total of 42 protein sequences were extracted from SGN database and were exclusively annotated as small HSP proteins in tomato genome (Goyal et al., 2012; Yu et al., 2016) to construct a phylogenetic tree using MEGA7 program (Figure 2). The sequences separated into 3 major groups/clades. Both the SlHSP17.7A and SlHAP17.7B proteins constitute to clade I along with other members of this cluster, viz., SlHSP17.6, SlHSP20.0 and SlHSP21.0 (Shukla et al., 2017).
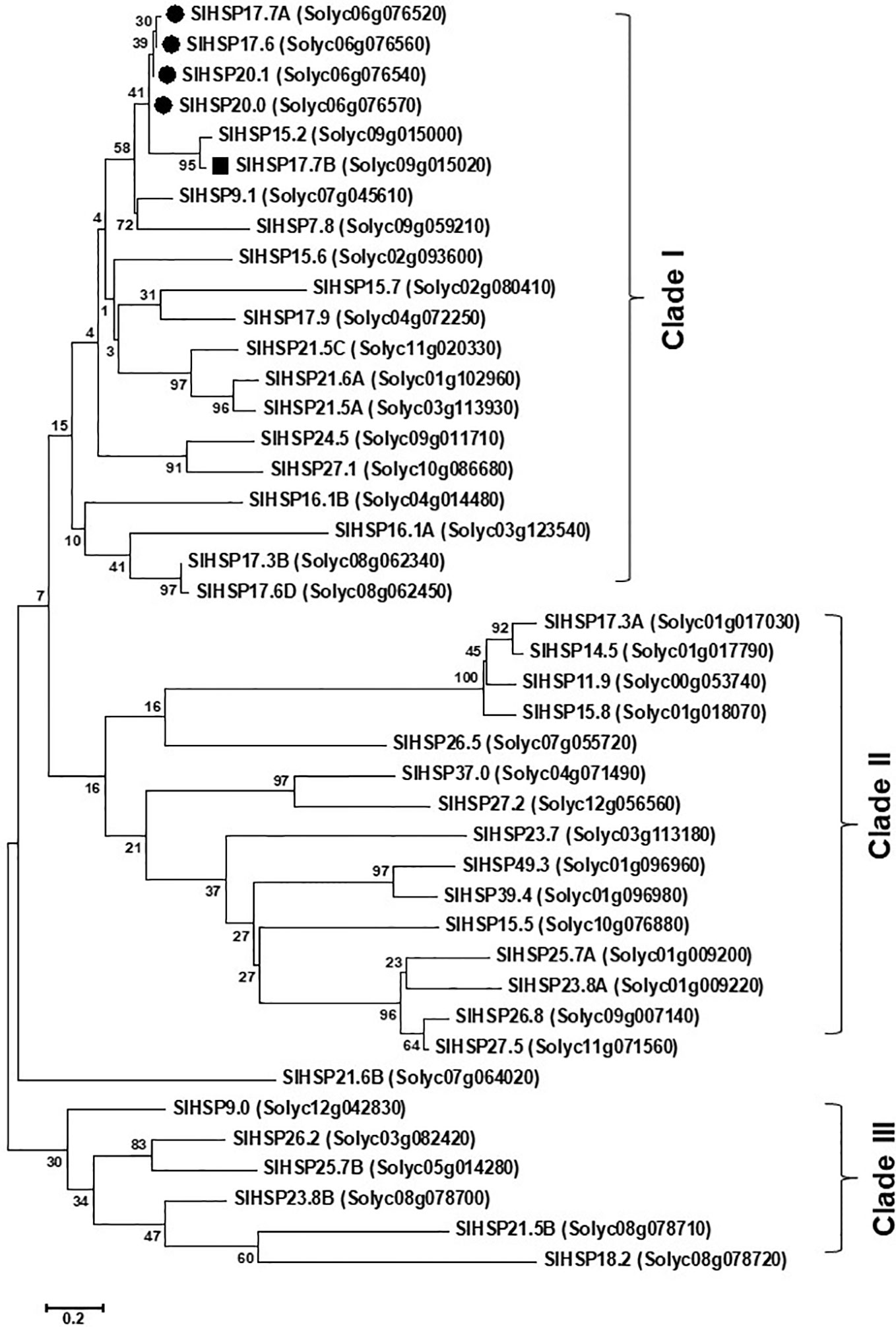
Figure 2 Phylogeny relatedness of SlHSP17.7A and SlHSP17.7B with other known proteins. The phylogenetic tree was constructed by maximum likelihood method with 1,000 boot strap values. Poisson model with complete deletion was employed during construction of the tree. The tree is drawn to scale, with branch lengths measured in the number of substitutions per site (Kumar et al., 2016). Forty two small heat shock proteins (HSPs) from tomato fell into two major groups, I and II. Group I was further segregated into “A” and “B” subgroups while group II was segregated into “C” and “D” subclades. SlHSP21.5C did not fall in any of the major groups. Subclade ‘A’ of group I consisted of small class I heat shock proteins namely, SlHSP17.6, 20.0, and 20.1 (Goyal et al., 2012; Shukla et al., 2017) and the newly identified SlHSP17.7A and SlHSP17.7B.
Fruit Ripening-Specific Expression of SlHSP17.7A and SlHSP17.7B Is Synergistic With Upregulation of SlMADS-RIN and SlACS2 Gene Transcripts
We extracted available transcriptome data (S. lycopersicum cv. Heinz) from International Tomato Genome Sequencing Consortium (SGN; solgenomics.net) database (version ITAG 2.4) in order to determine the relative transcript abundances for SlHSP17.7A, SlHSP17.7B, SlMADS-RIN and SlACS2 during plant development and tomato fruit ripening (Figure 3A). Gene transcripts of all these four are upregulated during ripening with the abundance of each gene transcripts increasing from MG/(-)5BR to BR stage [5.8-fold for SlHSP17.7A, 5.7-fold for SlHSP17.7B, 10.7-fold for SlACS2, and 49.3-fold for SlMADS-RIN] and yet more abundant at the BR+10 stage [8.9-fold for SlHSP17.7A, 9.4-fold for SlHSP17.7B, 24.1-fold for SlACS2, and 102.9-fold for SlMADS-RIN] (Figure 3B). Their RPKM counts followed an increasing trend with SlHSP17.7A > SlHSP17.7B > SlMADS-RIN > SlACS2, which indicates that the relative expression of SlHSP17.7A was highest among the four genes tested. In terms of fold change from mature green to ripening stages, SlMADS-RIN transcripts were highly expressed, followed by SlACS2 > SlHSP17.7B > SlHSP17.7A. The transcriptome data for SlHSP17.7 genes expression was validated by qRT-PCR analysis of RNA from vegetative tissues (root, stem, leaf, flower and seedling) (Figure 4A) and fruit developmental/ripening stages of Ailsa Craig (Figures 4B, C). Overall, the expression of SlHSP17.7A and B genes was found low in the vegetative tissues and during fruit development as compared to that during fruit ripening progression. Both sHSPs were similarly expressed in the stem while in leaf, flower and seedling tissues expression of SlHSP17.7B was significantly lower (P < 0.001) compared to that of SlHSP17.7A(Figure 4A).
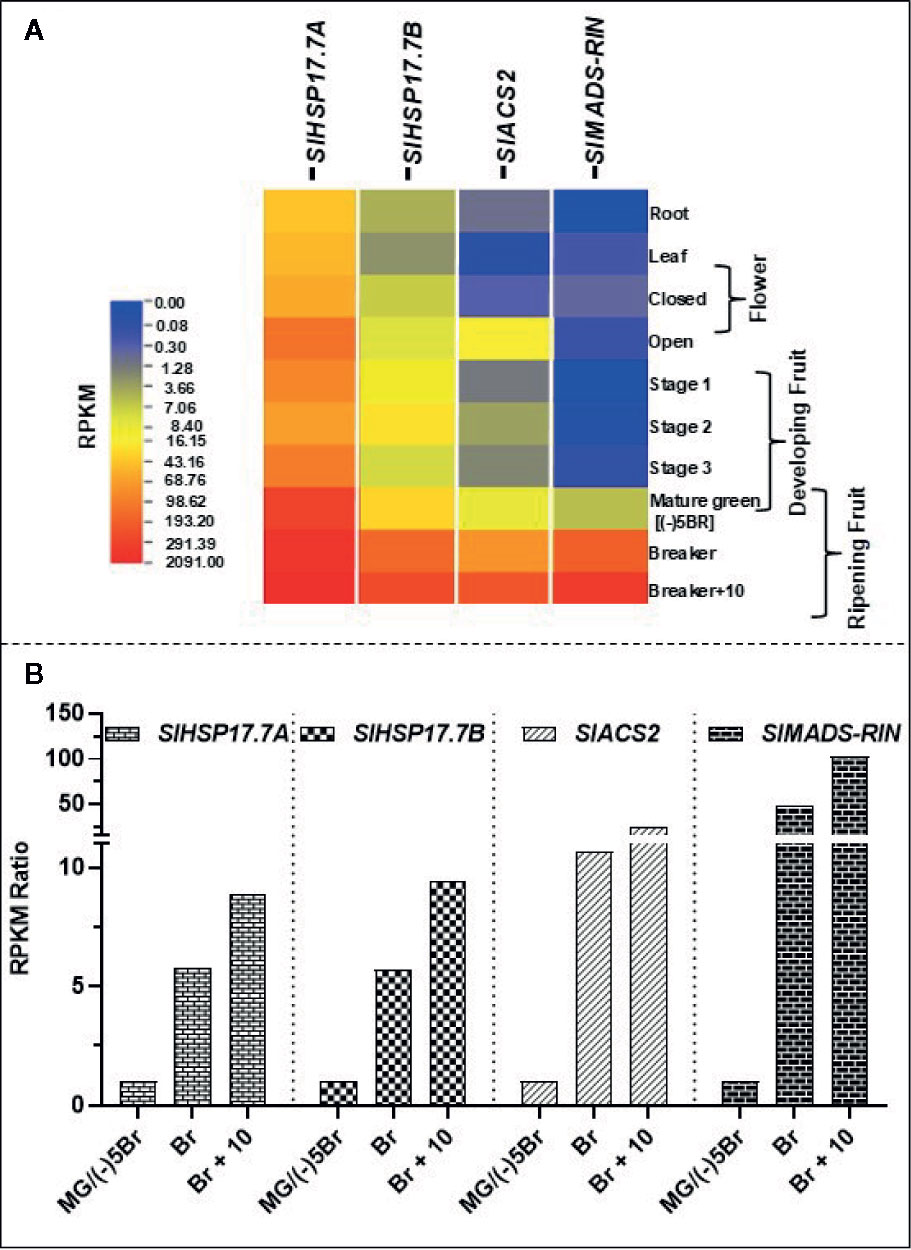
Figure 3 Transcriptome analysis of SlHSP17.7 transcripts in wild-type S. lycopersicum cv. Heinz tomato during fruit ripening. (A) RPKM values for the SlHSP17.7A, SlHSP17.7B SlACT2, and SlRIN genes in tomato during plant growth, devepolment and fruit ripening [root, leaf, bud, flower, 3 fruit developmental stages, mature green (MG), breaker (BR), and red ripe (BR+10)] were derived from RNA-seq data from the SGN database (S. lycopersicum cv. Heinz) as described in the materials and methods section. (B) Mature green stage/(-)5BR was used as calibrator to reveal ripening-specific changes in gene expression. Fold change for each gene was calculated by dividing the RPKM values of (-)5BR stage to BR and BR +8 stages. Ripening-induced gene expression seen for SlHSP17.7A and SlHSP17.7B was corroborated with the expression of climacteric ethylene regulator SlACS2 and SlMADS-RIN.
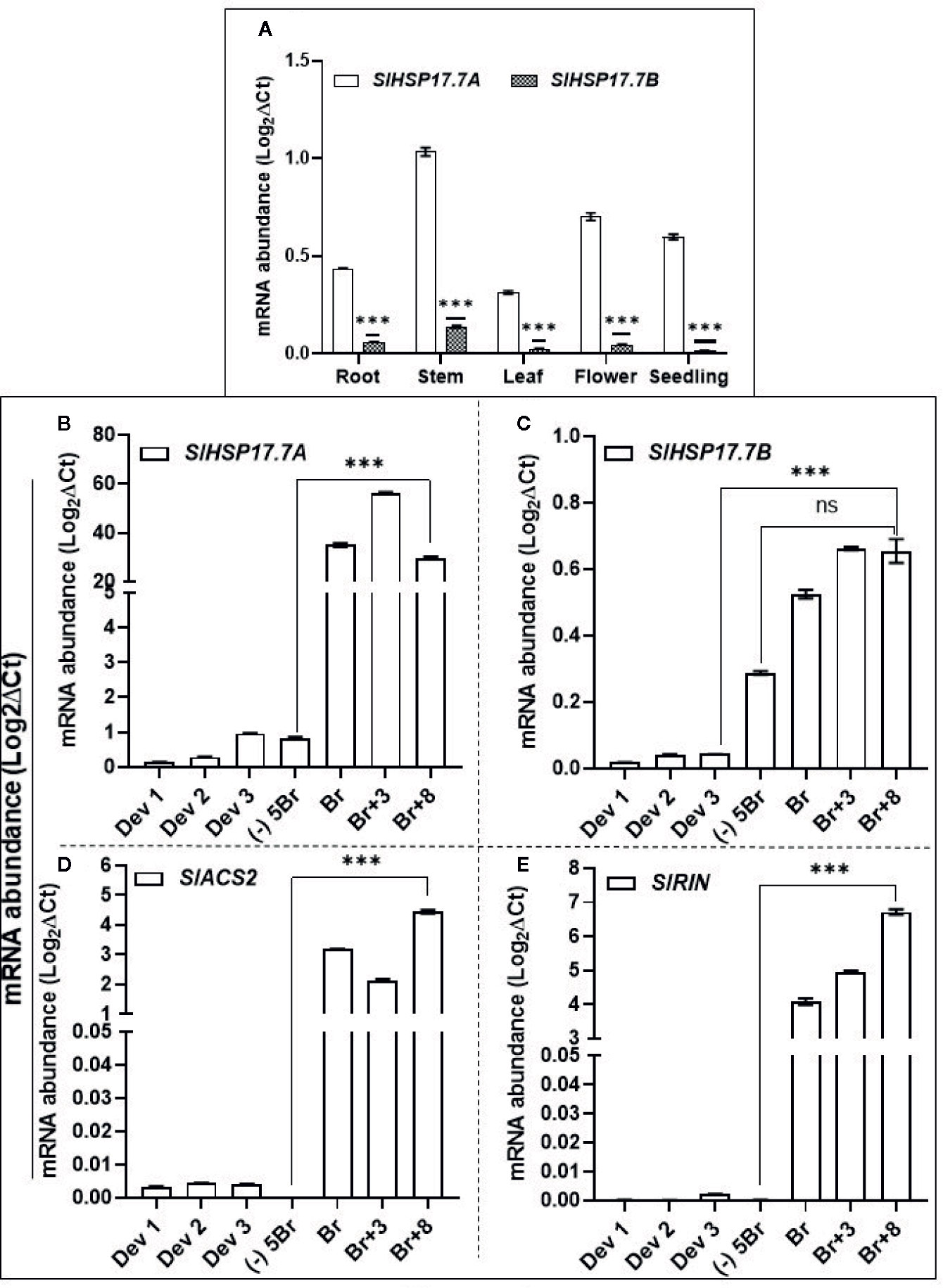
Figure 4 Quantitative real-time PCR (qRT-PCR) of SlHSP17.7A, SlHSP17.7B, SlACS2 and SlMADS-RIN genes in different tissues of wild-type S. lycopersicum cv. Ailsa Craig. RNA was isolated from root, stem, leaf, flower, seedling tissue and from fruit developmental and ripening stages. Leaf and stem samples were from 1-month old tomato plants; flowers were sampled from 3-month old plant; young seedlings were harvested from 8- to 10-day-old germinated seeds while for fruit harvesting stages Ailsa Craig plants were tagged at anthesis and fruits harvested at 8DPA (DPA = days post anthesis) (Dev 1), 15DPA (Dev 2), 22DPA (Dev 3) and ripening stages [(-)5BR, BR, BR+3, BR+8]. (A) Expression of SlHSP17.7A and SlHSP17.7B genes in vegetative tissue; (B) expression of SlHSP17.7A and (C) SlHSP17.7B during fruit development and ripening; (D) expression of SlACS2 and (E) SlMADS-RIN during fruit development and ripening. SlTIP41 and SlUBI3 genes were used to normalize the expression of the target genes (Expósito-Rodríguez et al., 2008; Mascia et al., 2010). Error bars indicate standard deviation from a minimum of three biological replicates. Asterisks indicate statistically significant differences, *P < 0.05, **P < 0.01, ***P < 0.001 and ****P < 0.0001 (see Materials and Methods). ns, not significant.
During fruit ripening both the sHSP genes were upregulated as is known for the ripening regulators SlACS2 and SlMADS-RIN. In Ailsa Craig, SlHSP17.7A expression was 40.63-fold higher at BR stage, increasing significantly (P < 0.001) to 61.56-fold at BR+3 stage, and decreased thereafter to 35-fold as compared to MG (-)5BR fruit (Figure 4B). In comparison, SlHSP17.7B expression was 1.8-fold higher at BR stage (P > 0.01), increasing to 2.3-fold (P < .001) at BR+3 and 2.27-fold (P < .01) at BR+8 stage compared to the MG/(-)5BR fruit (Figure 4C). Notably, SlACS2 expression in Ailsa Craig fruit increased from 18 thousand-fold at the BR stage to 25 thousand-fold (P <0.001) at the BR+8 stage as compared to the MG/(-)5BR fruit (Figure 4D). Similarly, SlMADS-RIN expression increased from 26 thousand-fold (P <0.001) at the BR stage to 43 thousand-fold (P <0.001) at the BR+8 stage as compared to the MG/(-)5BR fruit (Figure 4E). Similar high expression dynamics of SlACS2 and SlMADS-RIN genes are known (Martel et al., 2011).
Differential Accumulation of SlHSP17.7A and SlHSP17.7B Gene Transcripts in Alisa Craig Versus Ohio 8245 During Ripening
Ripening-regulated expression of SlHSP17.7A and SlHSP17.7B genes in Ailsa Craig was notably higher than in the Ohio 8245 variety (Figures 5A, B). Expression of SlHSP17.7A in Ailsa Craig fruit was 40.97-fold higher at BR stage, further increased by 64.87-fold at BR+3, and decreased thereafter to 35.65-fold at BR+8 compared to that at MG/(-)5BR. In comparison, SlHSP17.7A transcript expression in Ohio8245 genotype was notably much lower with a 3.7-fold increase at the BR stage, increasing further to 7.3-fold at BR+3 and 4.5-fold at BR+8 relative to that at MG/(-)5BR stage (Figure 5A). The expression of sHSP17.7B in Ailsa Craig and Ohio8245 fruits during ripening was not as dramatically different as that found for SlHSP17.7A gene above. In Ailsa Craig fruit stages, expression of sHSP17.7B was 1.63-fold at BR, 2.18-fold at BR+3 and 2.20-fold at BR+8 stages as compared to that in the MG/(-)5BR fruit. Likewise, in Ohio 8245 fruit stages, a moderate sequential increase in SlHSP17.7 transcript expression was 1.26-fold at BR, 1.5-fold at BR+3 and 3.6-fold at BR+8 stages as compared to MG/(-)5BR stage (Figure 5B). Thus, the expression levels of these sHSP genes vary to different degrees in the two tomato varieties; however, in both varieties, the two genes are upregulated during fruit ripening.
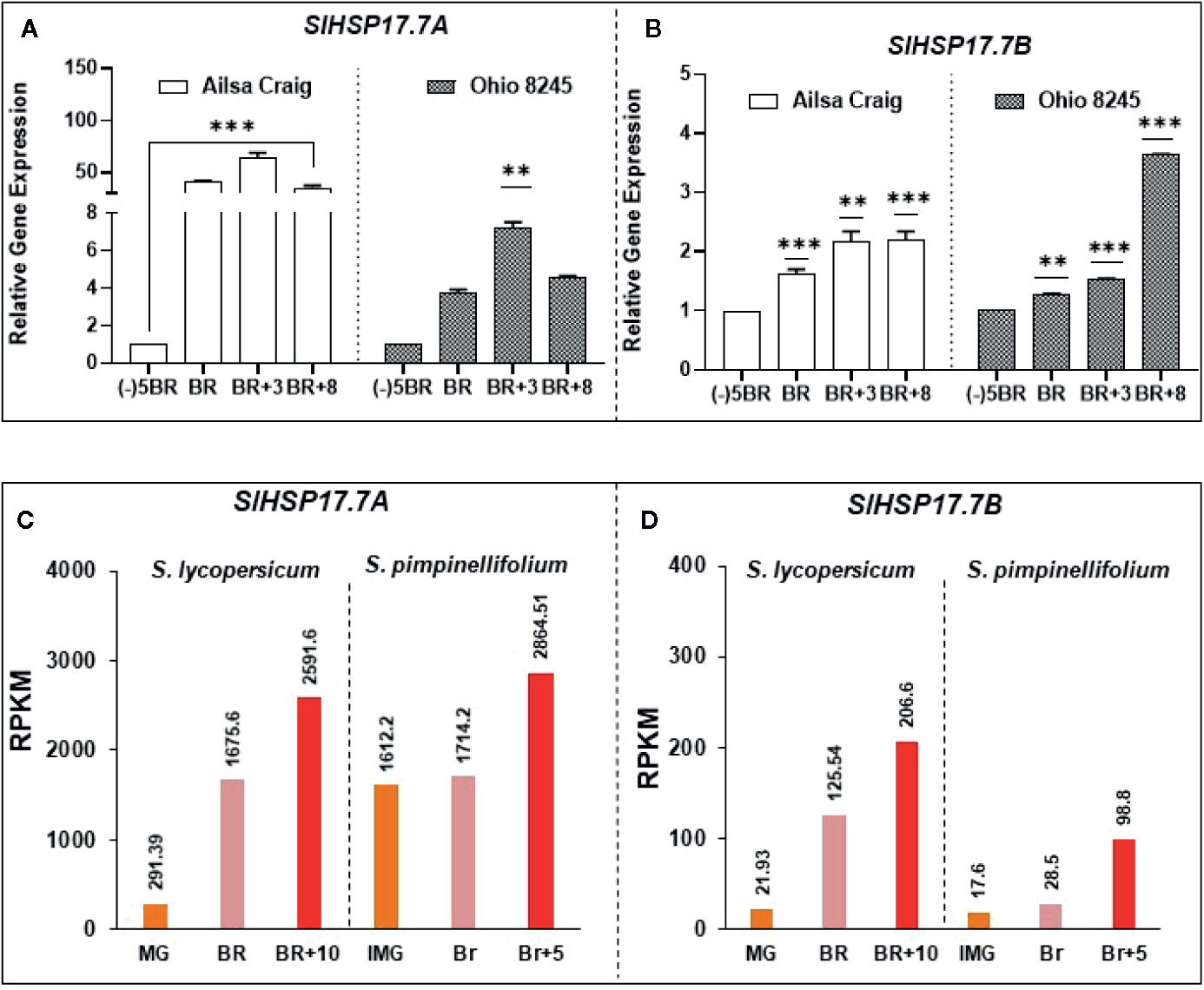
Figure 5 Differential expression of SlHSP17.7A and SlHSP17.7B genes in two modern tomato varieties as well as in two different genotypes. Tomato (S. lycopersicum) var. Ailsa Craig and Ohio 8245 fruits from various ripening stages were harvested as described in the Materials and Methods section. Both (A) SlHSP17.7A and (B) SlHSP17.7B genes showed differential expression during ripening. Variety-based abundance was also determined. Calibrator used for quantitative real-time PCR (qRT-PCR) calculations was (-)5BR stage. SlTIP41 and SlUBI3 genes were used to normalize the expression of target genes as described in the legend to Figure 3. Three biological replicates were used from each variety. A minimum of three fruits (n=3) were used for RNA isolation for each biological replicate. Transcriptome data for Solanum lycopersicum Heinz and Solanum pimpinellifolium fruit ripening stages for SlHSP17.7A and B, obtained from tomato expression database, show fold difference variation among genotypes with a functionally conserved ripening-induced nature of both HSPs (C, D).
Transcriptome data for Solanum lycopersicum Heinz and Solanum pimpinellifolium from tomato expression database also indicated that HSP expression greatly depends on the tomato genotype. However, a consistent ripening-induced expression of SlHSP17.7A, B genes is observed irrespective of genotypes (Figures 5C, D). This also reflects a functional conservation of these genes during evolution irrespective of gain in fruit size between S. lycopersicum vs. S. pimpinellifolium.
Ethylene Inhibits SlHSP17.7A, B Expression in a Time-Dependent Manner in Fruit and Leaf Tissues
To discern if ethylene treatment of fruit modulates the expression of these two sHSP genes, we held the Ohio8245 MG/(-)5BR fruits separately in either air (control), ethylene or in the presence of 1-MCP an inhibitor of ethylene signaling (Figure 6). Fruits held in ethylene atmosphere for 12 and 24 h were found to be suppressed for the expression of both SlHSP17.7A and SlHSP17.7B genes (P < 0.001). However, it took 24 h before the fruits held in 1-MCP were found to be suppressed in SlHSP17.7A expression (Figure 6). Notably, 1-MCP-treated tomato fruit had higher expression levels of SlHSP17.7B gene at 12 and 24 h as compared to air control. We also determined if ethylene-mediated suppression of these two sHSPs occurs also in leaves. Both the gene transcripts were found suppressed for a longer duration in tomato leaves incubated in the ethylene atmosphere (Supplementary Figure 1). This demonstrates that ethylene mediated transient suppression of both these sHSP transcripts occurs in both fruit and leaf tissues.
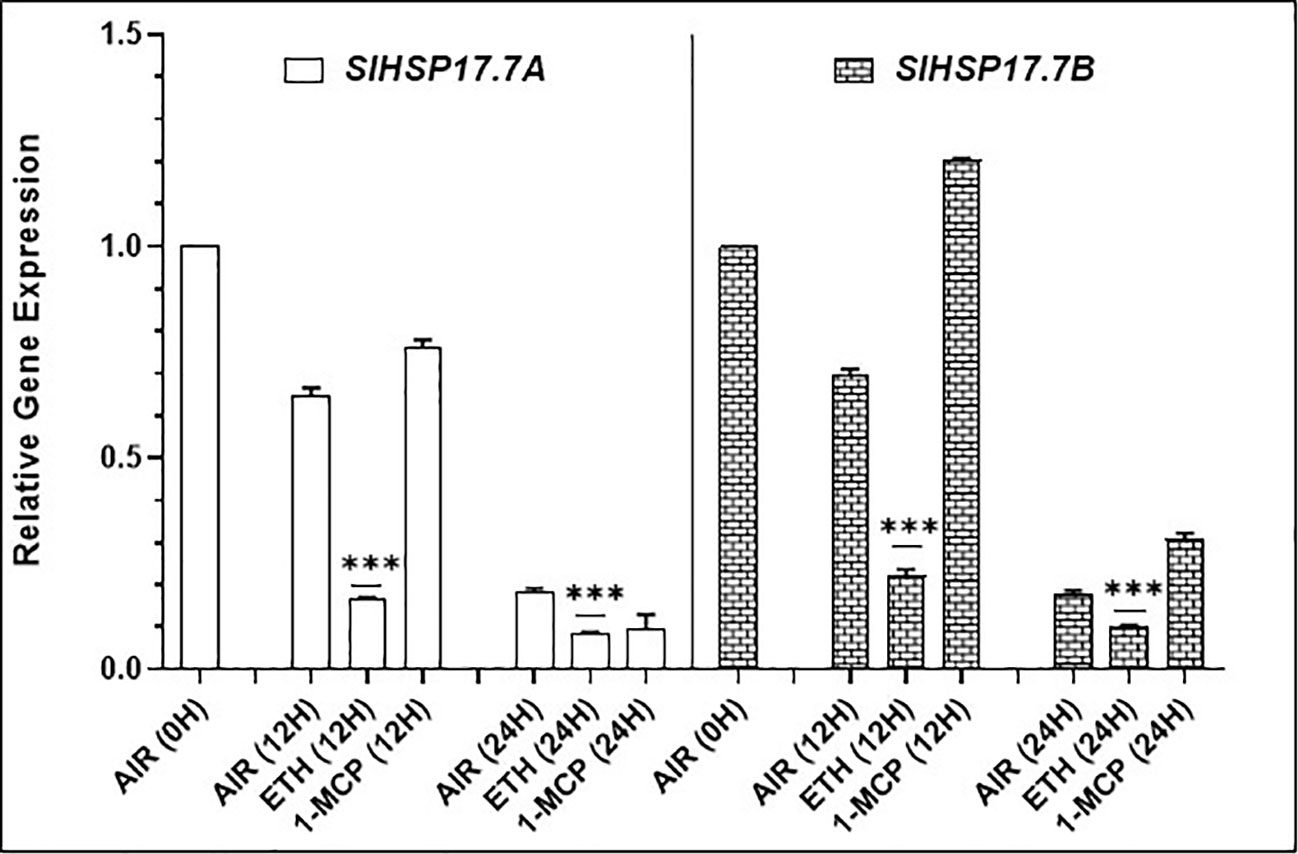
Figure 6 Effects of ethylene (ETH) and 1-methylcyclopropene (1-MCP) treatments on the expression of SlHSP17.7A and SlHSP17.7B genes. Wild-type (WT) (Ohio8245) fruits at (-)5BR/mature green stage were treated with either 25 ppm ethylene, 2 ppm 1-MCP, or left in air. Samples were then harvested at 0, 12 and 24 h, total RNA was isolated and expression of SlHSP17.7A and SlHSP17.7B genes was quantified. Error bars indicate standard deviation from a minimum of three replicates. SlTIP41 and SlUBI3 genes were used to normalize the expression of the target genes as described in the legend to Figure 3. Statistical differences [*P ≤ 0.05, **P ≤ 0.01, ***P ≤ 0.001, and ****P ≤ 0.0001] are indicated.
Ripening Mutants Are Compromised in SlHSP17.7A Expression but SlHSP17.7B Expression Is Differentially Elevated During Ripening as Compared to the Wild Type
Expression of SlHSP17.7A and SlHSP17.7B genes was also quantified in the near isogenic nonripening mutant lines - ripening-inhibitor (rin/rin), nonripening (nor/nor), and Never-ripe (Nr/Nr) at similar age/ripening as the WT-Ailsa Craig (Figure 7). SlHSP17.7A transcript abundance was significantly impacted in the rin/rin and nor/nor mutants during the progression of ripening as compared to the WT-Ailsa Craig (Figure 7A). Similar was the case with Nr/Nr mutant fruit except that SlHSP17.7A transcript abundance was significantly higher at BR and BR+3 than in nor/nor and rin/rin mutants vis a vis WT-Ailsa Craig fruit (Figure 7A). An opposite trend in the expression of SlHSP17.7B gene in the three mutant lines was apparent as compared to WT. SlHSP17.7B gene expression remained high in mutants as ripening progressed (in rin/rin: 2.5-fold higher at BR and Br+8; in nor/nor: 5 to 6-fold higher at BR and BR+3 but lower at BR+8 stage; in Nr/Nr: 2-fold higher at BR and BR+3 stages but lower at BR+8) in comparison to the same ripening period of the WT-Ailsa Craig fruits (Figure 7B).
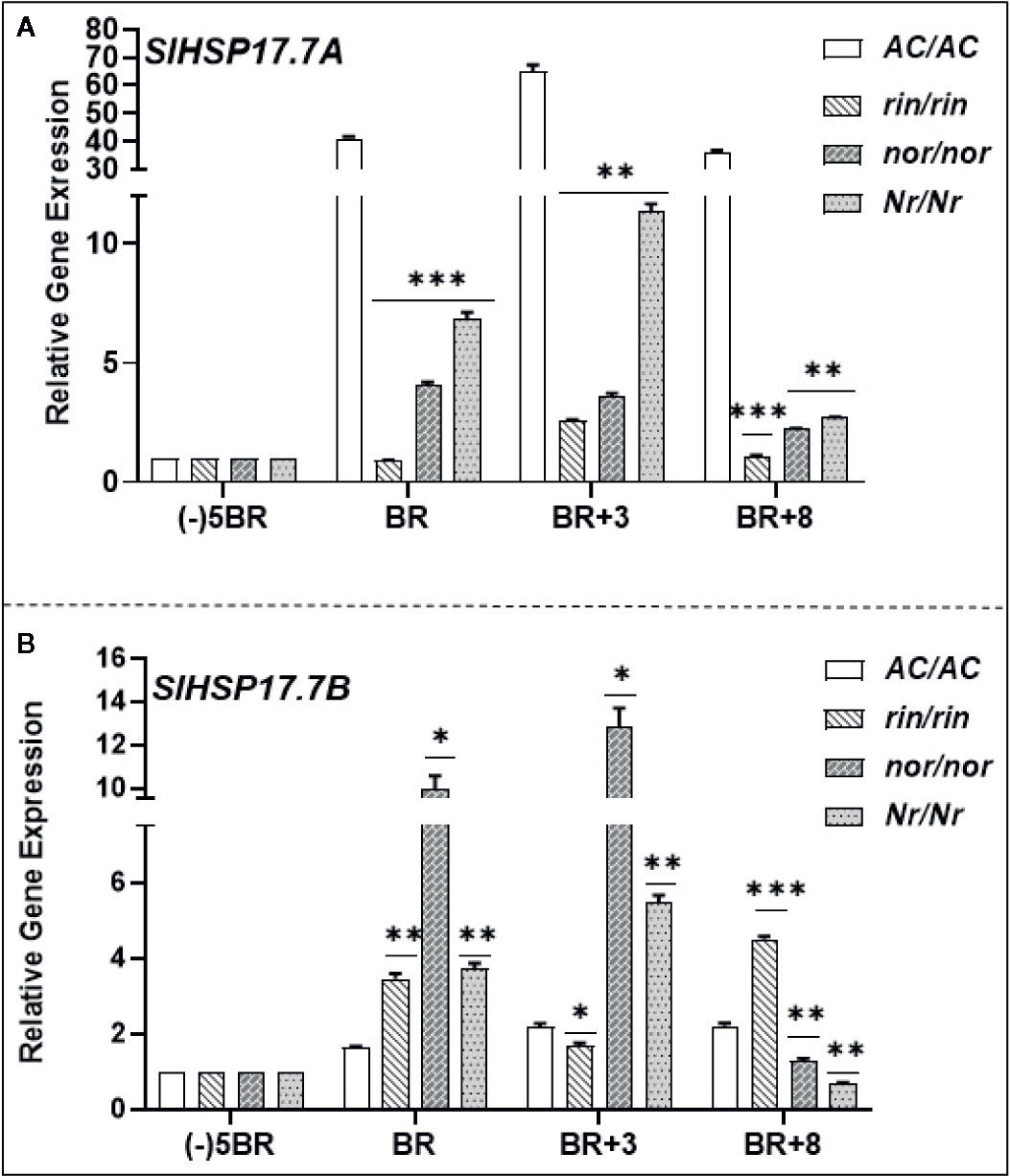
Figure 7 Expression patterns of (A) SlHSP17.7A and (B) SlHSP17.7B genes in nonripening tomato mutants. Fruits from wild-type tomato (S. lycopersicum) var. Ailsa Craig (AC/AC) and its ripening mutants (rin/rin, nor/nor, and Nr/Nr) were harvested as described in Materials and Methods section. quantitative real-time PCR (qRT-PCR) analysis, calibrator used for qRT-PCR calculations, and normalization of expression were the same as described in the legends to Figure 5. Statistical differences [*P ±0.05, **P ≤ 0.01, ***P ≤ 0.001, and ****P ≤ 0.0001] are indicated.
Since these tomato mutants are nonripening in nature and deficient in ethylene biosynthesis and perception, any deviation in their expression from wild type would be considered as ripening-specific and ethylene dependent. These results indicate that while SlHSP17.7A has ripening-specific expression, expression of SlHSP17.7B is not limited or regulated by ripening.
Differential Regulation of SlHSP17.7 and SlMADS-RIN Expression in ACS2 Gene-Silenced Transgenic Tomato
To differentiate ethylene regulation of the two sHSP genes and of SlMADS-RIN, we employed our previously characterized transgenic tomato line ACS2-AS silenced for ACS2 gene which produces 50% less ethylene than the wild type (Sobolev et al., 2014). Expression of both, SlHSP17.7A and SlHSP17.7B genes, was upregulated only at the breaker stage compared to the azygous control line; however, this upregulation was many-fold evident in the SlHSP17.7B gene right from breaker stage to BR+8 stage (Figures 8A, B). Interestingly, the ACS2-AS ethylene-deficient line was also found to be compromised in the expression of the ripening-regulator SlMADS-RIN gene (Figures 8C, D). These data invoke ethylene as a negative regulator of the two sHSPs and, likely, the SlMADS-RIN gene.
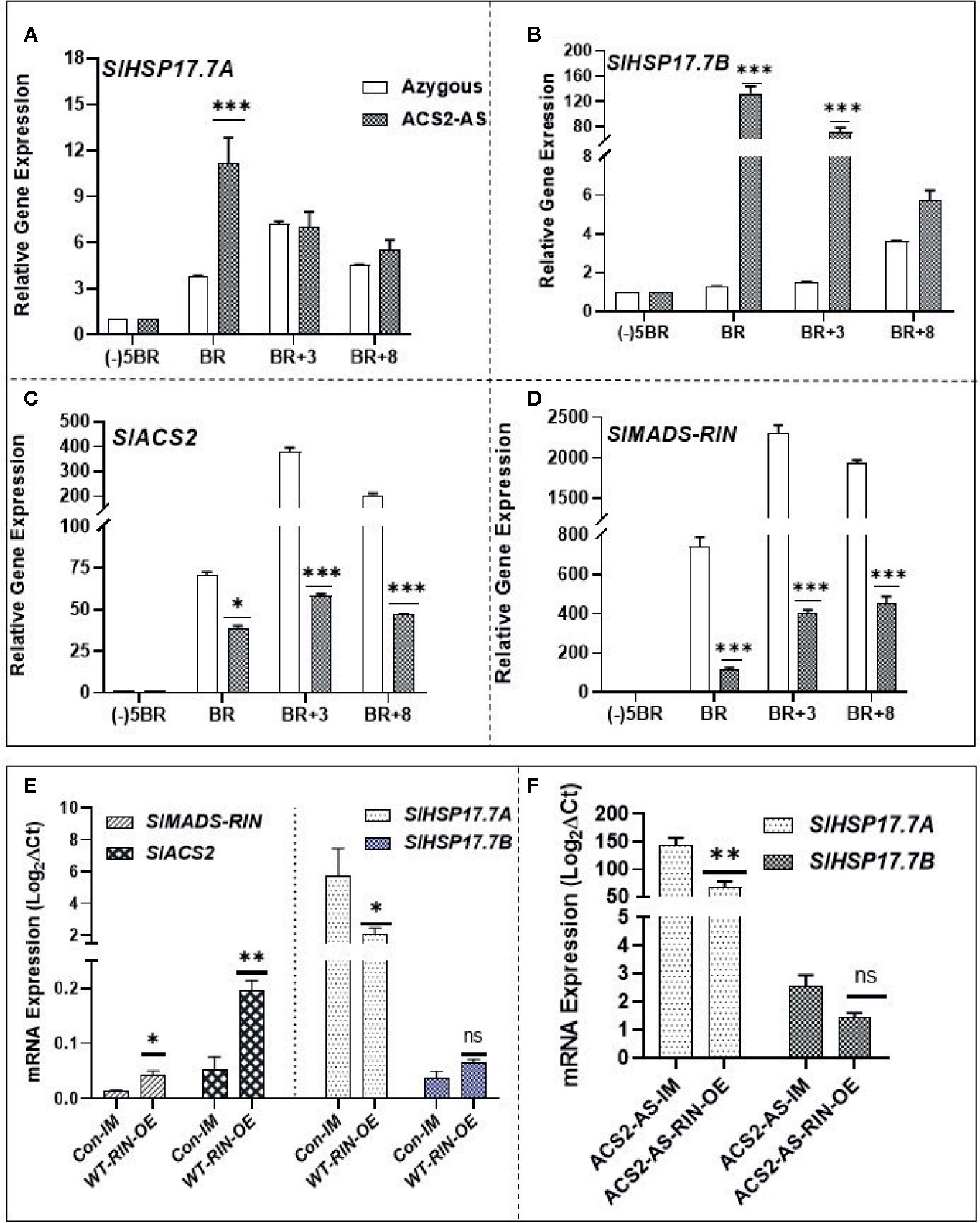
Figure 8 Expression dynamics of SlHSP17.7A, SlHSP17.7B, and SlMADS-RIN during fruit ripening of ACC synthase 2 (SlACS2)-silenced tomato transgenic line (ACS2-AS) and the azygous/wild-type control lines (WT-Ohio8245) and RIN agroinjection. Fruit at different ripening stages were harvested from ACS2-AS and azygous control plants. RNA was isolated and expression levels of (A) SlHSP17.7A; (B) SlHSP17.7B; (C) SlACS2; (D) SlRIN were determined by qRT-PCR analysis. (E) Wild-type mature green tomato fruits were infiltrated with agrobacterium culture carrying RIN overexpression construct as described in the Materials and Methods Section. Fruits injected with only the infiltration medium were taken as control. RNA was isolated from infiltrated fruits and cDNA was prepared. Expression studies were done for SlACS2, SlMADS-RIN, SlHSP17.7A, and SlHSP17.7B. (F) Similar methodology as in (A) was repeated for ACS2-AS tomato and expression of SlHSP17.7A and SlHSP17.7B was determined. Error bars indicate standard deviation from a minimum of three replicates for each data point and statistical differences [*P ≤ 0.05, **P ≤ 0.01, ***P ≤ 0.001 and ****P ≤ 0.0001] are indicated.
In Silico Analysis of the SlHSP17.7A, B Gene Promoters and Identification of Various cis-Elements
We also analyzed the 2 Kb upstream 5′ putative promoter regions of the two tomato SlHSPs genes for the presence of ethylene-related cis-elements ERE (ethylene response elements) and RIN binding ‘CArG’ box cis-elements using PLACE (Higo et al., 1999) and Plant Care (Lescot et al., 2002) databases. Several binding sites responsive to hormonal and environmental signals were found in the promoter of both the HSP genes (Supplementary Tables 3 and 4). Notably, two ‘CArG’ binding cis-elements in the promoters of each gene were found. The SlHSP17.7A promoter was decorated with one “atypical” [C(A/T)8G] motif type at -29 and other “possible” [C(C/T)(A/T)6(A/G)G] motif type at -1841 position (Supplementary Table 3). Similarly, SlHSP17.7B gene promoter was found decorated with both “atypical” [C(A/T)8G] motif types at -633 and at -1067 positions (Supplementary Table 4). Previously, CArG motifs have been shown to be target binding sites for SlMADS-RIN transcription factor to regulate fruit ripening genes (Martel et al., 2011; Fujisawa et al., 2013; Shukla et al., 2017). The presence of CArG motifs in SlHSP17.7A and SlHSP17.7B gene promoters suggests that SlMADS-RIN transcription factor may bind these cis-elements and regulate expression of HSPs during fruit ripening.
Transient Overexpression of SlMADS-RIN Transcription Factor Reveals Differential Expression of SlHSP17.7A and SlHSP17.7B Genes in WT Versus ACS2-AS Tomato Line
Tomato fruits were infiltrated with pK2GW7-SlMADS-RIN-OE construct and, after 72–96 h of incubation period, infiltrated fruits were analyzed for the abundance of SlMADS-RIN and SlACS2 transcripts as described in the methods section. Transcripts of both SlMADS-RIN and SlACS2 were found upregulated, which indicated successful activation of SlACS2 gene (3-fold; P < 0.01) by SlMADS-RIN transcription factor (Figure 8E). To check if SlMADS-RIN mediates suppression or activation of the two sHSPs, this cDNA was analyzed for the status of both the HSP genes. Interestingly, SlHSP17.7A expression was significantly suppressed (P=0.0023), and the SlHSP17.7B transcripts were activated but not to an extent to be statistically significant.
Finally, we also agro-injected the ACS2-AS transgenic tomato line (with low ethylene background) with the pK2GW7-SlMADS-RIN-OE construct. This experiment revealed a lower transcript abundance of SlHSP17.7A (P=0.0069); however, the SlHSP17.7B transcripts also were downregulated but not to a level to be statistically significant (Figure 8F). These results demonstrate that SlHSP17.7A transcripts are suppressed by SlMADS-RIN in different genetic backgrounds. These results are in tune with the previous findings where SlMADS-RIN was shown to regulate some genes in both ethylene-dependent and independent manner, reminiscent of the existence of negative as well as positive regulation of tomato genes during the transition of mature green fruit into ripening progression (Fujisawa et al., 2013).
Discussion
Two novel class I small HSP genes (SlHSP17.7A and SlHSP17.7B) were identified in tomato and their transcriptional regulation was found to be mediated by the plant hormone ethylene. Both the sHSP genes share sequence similarities with other tomato class I small HSPs sequences (Goyal et al., 2012; Paul et al., 2016; Yu et al., 2016; Shukla et al., 2017). However, they are localized in different chromosomes in tomato, SlHSP17.7A gene is localized to chr. 6 in a tandem repeated manner with a previously characterized cluster of three small HSP genes SlHSP17.6, SlHSP20.0 and SlHSP20.1 (Goyal et al., 2012; Shukla et al., 2017). However, the SlHSP17.7B gene is resident on chr. 9 but shares a close homology to SlHSP17.7A. Both the sHSP genes are ripening-specific with minimal expression in the vegetative tissues; moreover, the SlHSP17.7B transcripts were observed at the developmental stage 3 of tomato fruit, a stage earlier than the mature green fruit. These two sHSPs can be further differentiated by their expression patterns in the ethylene-deficient tomato mutants (rin/rin, nor/nor, and Nr/Nr), with SlHSP17.7A gene expression characteristically impaired in these mutants while SlHSP17.7B gene is abundantly expressed in them.
A mutation in the ripening-regulator MADS-RIN protein prevents the tomato ripening mutants, particularly rin, from ripening (Vrebalov, 2002) since MADS-RIN protein regulates the expression of ethylene biosynthesis genes ACS2 and ACS4 during fruit ripening (Cara and Giovannoni, 2008; Martel et al., 2011). This is also apparent from the data presented here showing that the SlMADS-RIN transcription is in congruence with the expression patterns of SlACS2. In this regard, our in silico analysis revealed that the promoters of both the SlHSP17.7A and SlHSP17.7B genes harbor at least two MADS-RIN binding cis-elements (Supplementary Tables 3 and 4), the CArG motif (Ito et al., 2008; Fujisawa et al., 2011; Zhong et al., 2013). Similar CArG motifs has been shown in promoters of other genes by previous workers. For example, CArG motif at position −1841 (CAAAAAAAAG) in gene promoter of SlHSP17.7A has been previously identified in Solyc04g082420 promoter as potential direct target of RIN transcription factor (Fujisawa et al., 2013). While, CArG motifs in SlHSP17.7B promoter at position −633 (CTTAAATATG) and −1067 (CATTAATTTG) has been previously shown as direct target of RIN in Solyc01g104050 and Solyc09g065030 gene promoters, respectively (Fujisawa et al., 2013). This further strengthen a possibility of RIN transcription factor binding with CArG motifs present in HSP gene promoters. Thus, we envisioned an interaction of RIN with promoter elements of the two sHSPs and tested this hypothesis via transient expression of RIN in WT and ethylene-deficient tomato line. Significant suppression was apparent for SlHSP17.7A gene but not the SlHSP17.7B. Thus, SlMADS-RIN may be a negative regulator of SlHSP17.7A gene.
Together, these data reinforce the view that some small HSPs regulate fruit ripening in tomato. Thus, ethylene and transcription of class-1 sHSP genes seem interlinked in tomato, particularly at the initial phase of fruit ripening. These findings also corroborate the suggestion put forward earlier that observation of ripening induced expression of some HSP ESTs (Fei et al., 2004) and another class-I sHSP21 gene may be crucial for progression of fruit ripening (Neta-Sharir et al., 2005). This indicates that additional factor(s) are involved in inducing these sHSP genes during fruit ripening. Our data showing that ethylene-treated tomato leaf is suppressed in the expression of SlHSP17.7 gene suggests that this regulation occurs in both tissues, fruit and leaf. tissue independent.
We opine that ethylene suppression of SlHSP genes at the onset of fruit ripening when ethylene synthesis is initiated may be an indigenous regulation slated to enable fruit ripening to proceed uninterruptedly. Interestingly, transient suppression of both SlHSP17.7A and SlHSP17.7B genes by exogenous ethylene was more robust in the ethylene-deficient ACS2-AS genotype. Since ACS2-AS transgenic line produces only 50% of ethylene relative to its control suggests that a certain threshold of ethylene is necessary to achieve robust suppression of SlHSP17.7 transcripts.
Our previous work on SlHSP17.6, 20.0, and 20.1 showed that transient suppression of these genes during fruit ripening transition is regulated by SlMADS-RIN-mediated and ethylene-dependent pathway (Shukla et al., 2017). Here, we demonstrated that SlHSP17.7A follows a similar kind of transcription regulation as the other members of this cluster (Goyal et al., 2012). However, SlHSP17.8B gene seems to be regulated by some other, as yet unknown transcriptional regulator different from SlMADS-RIN. However, in regard to regulation by ethylene both of these sHSPs are similarly suppressed by exogenous ethylene and their expression is highly upregulated at breaker stage in the ACS2-AS transgenic background tomato, a situation where SlMADS-RIN transcripts were found to be very low.
Notably, our results that demonstrated low SlMADS-RIN expression during fruit ripening in the ACS2-AS transgenic line deficient in ethylene by 50% suggest that (i) ethylene is required to induce SlMADS-RIN transcripts directly or/and (ii) an unknown ethylene-dependent regulator is necessary to induce SlMADS-RIN during fruit ripening transition. These results suggest the need for a reevaluation of the role of SlMADS-RIN as the master regulator of fruit ripening which is also recently studied by different groups (Ito et al., 2017; Wang et al., 2020). Although, this was not the primary focus of this study but our primary results indicate that still ethylene is upstream to RIN, albeit this hypothesis needs rigorous experimentation. Further functional genomics studies are needed to characterize in planta the promoter of the two SlHSP17.7 genes. Novel transgenic approaches can shed further light on specific role(s) of small HSPs in fruit physiology and ripening.
Differential gene expression in duplicated sHSP genes has been linked to homeostasis maintenance and their likely role(s) in responses to different stresses (Arce et al., 2018). Since HSP roles include protein folding, chaperone function and other, they are deemed essential for refolding proteins under abiotic stress situations (Murakami et al., 2004; Wang et al., 2004). Clearly, their committed role(s) in plant life and fruit ripening as presented here need to be followed further.
Data Availability Statement
The raw data supporting the conclusions of this article will be made available by the authors, without undue reservation.
Author Contributions
Conceived and designed the study: RU and AM. Performed the experiments: RU. Ethylene treatment of fruits and leaves: RU and MT. Analyzed the final data: RU and AM. Reagents availability: AM. Facilitated the research: AM. Wrote the paper: RU. Finalized the paper: AM.
Funding
This research was supported by an USDA-ARS intramural Project No. 8042-21000-143-00D (PI. AM). Mention of trade names or commercial products in this publication is solely for the purpose of providing specific information and does not imply recommendation or endorsement by the U.S. Department of Agriculture.
Conflict of Interest
The authors declare that the research was conducted in the absence of any commercial or financial relationships that could be construed as a potential conflict of interest.
Supplementary Material
The Supplementary Material for this article can be found online at: https://www.frontiersin.org/articles/10.3389/fpls.2020.00975/full#supplementary-material
References
Aevermann, B. D., Waters, E. R. (2008). A comparative genomic analysis of the small heat shock proteins in Caenorhabditis elegans and briggsae. Genetica 133, 307–319. doi: 10.1007/s10709-007-9215-9
Alexander, L., Grierson, D. (2002). Ethylene biosynthesis and action in tomato: a model for climacteric fruit ripening. J. Exp. Bot. 53, 2039–2055. doi: 10.1093/jxb/erf072
Arce, D., Spetale, F., Krsticevic, F., Cacchiarelli, P., Las-Rivas, J. D., Ponce, S., et al. (2018). Regulatory motifs found in the small heat shock protein (sHSP) gene family in tomato. BMC Genomics 19, 860. doi: 10.1186/s12864-018-5190-z
Barry, C. S., Giovannoni, J. J. (2007). Ethylene and fruit ripening. J. Plant Growth Regul. 26, 143–159. doi: 10.1007/s00344-007-9002-y
Becker, J., Craig, E. A. (1994). Heat-shock proteins as molecular chaperones. Eur. J. Biochem. 219, 11–23. doi: 10.1111/j.1432-1033.1994
Bustin, S. A., Benes, V., Garson, J. A., Hellemans, J., Huggett, J., Kubista, M., et al. (2009). The MIQE guidelines: minimum information for publication of quantitative real-time PCR experiments. Clin. Chem. 55, 611–622. doi: 10.1373/clinchem
Cara, B., Giovannoni, J. J. (2008). Molecular biology of ethylene during tomato fruit development and maturation. Plant Sci. 175, 106–113. doi: 10.1016/j
Expósito-Rodríguez, M., Borges, A. A., Borges-Pérez, A., Pérez, J. A. (2008). Selection of internal control genes for quantitative real-time RT-PCR studies during tomato development process. BMC Plant Biol. 8, 131. doi: 10.1186/1471-2229-8-131
Fei, Z. J., Tang, X., Alba, R. M., White, J. A., Ronning, C. M., Martin, G. B., et al. (2004). Comprehensive EST analysis of tomato and comparative genomics of fruit ripening. Plant J. 40, 47–59. doi: 10.1111/j.1365-313X.2004.02188.x
Fluhr, R., Mattoo, A. K. (1996). Ethylene — Biosynthesis and perception. CRC Crit. Rev. Plant Sci. 15, 479–523. doi: 10.1080/07352689609382368
Franck, E., Madsen, O., van Rheede, T., Ricard, G., Huynen, M. A., de Jong, W. W. (2004). Evolutionary diversity of vertebrate small heat shock proteins. J. Mol. Evol. 59, 792–805. doi: 10.1007/s00239-004-0013-z
Fray, R. G., Lycett, G. W., Grierson, D. (1990). Nucleotide sequence of a heat-shock and ripening-related cDNA from tomato. Nucleic Acids Res. 18, 7148. doi: 10.1093/nar/18.23.7148
Fu, X., Jiao, W., Chang, Z. (2006). Phylogenetic and biochemical studies reveal a potential evolutionary origin of small heat shock proteins of animals from bacterial class. A. J. Mol. Evol. 62, 257–266. doi: 10.1007/s00239-005-0076-5
Fujisawa, M., Nakano, T., Ito, Y. (2011). Identification of potential target genes for the tomato fruit-ripening regulator RIN by chromatin immunoprecipitation. BMC Plant Biol. 11, 26. doi: 10.1186/1471-2229-11-26
Fujisawa, M., Nakano, T., Shima, Y., Ito, Y. (2013). A large-scale identification of direct targets of the tomato MADS box transcription factor RIPENING INHIBITOR reveals the regulation of fruit ripening. Plant Cell 25, 371–386. doi: 10.1105/tpc.112.108118
Giorno, F., Wolters-Arts, M., Grillo, S., Scharf, K. D., Vriezen, W. H., Mariani, C. (2010). Developmental and heat stress-regulated expression of HsfA2 and small heat shock proteins in tomato anthers. J. Exp. Bot. 61, 453–462. doi: 10.1093/jxb/erp316
Giovannoni, J., Nguyen, C., Ampofo, B., Zhong, S., Fei, Z. (2017). The epigenome and transcriptional dynamics of fruit ripening. Ann. Rev. Plant Biol. 68, 61–84. doi: 10.1146/annurev-arplant-042916-040906
Giovannoni, J. J. (2007). Fruit ripening mutants yield insights into ripening control. Curr. Opin. Plant Biol. 10, 283–289. doi: 10.1016/j.pbi
Goyal, R. K., Kumar, V., Shukla, V., Mattoo, R., Liu, Y., Chung, S. H., et al. (2012). Features of a unique intron-less cluster of class I small heat shock protein genes in tandem with box C/D snoRNA genes on chromosome 6 in tomato (Solanum lycopersicum). Planta 235, 453–471. doi: 10.1007/s00425-011-1518-5
Gray, J. E., Picton, S., Giovannoni, J. J., Grierson, D. (1994). The use of transgenic and naturally occurring mutants to understand and manipulate tomato fruit ripening. Plant Cell Environ. 17, 557–571. doi: 10.1111/j.1365-3040
Hackett, R. M., Ho, C. W., Lin, Z., Foote, H. C., Fray, R. G., Grierson, D. (2000). Antisense inhibition of the Nr gene restores normal ripening to the tomato Never-ripe mutant, consistent with the ethylene receptor-inhibition model. Plant Physiol. 124, 1079–1086. doi: 10.1104/pp.124.3.1079
Hartl, F. U. (1996). Molecular chaperones in cellular protein folding. Nature 381, 571–579. doi: 10.1038/381571a0
Higo, K., Ugawa, Y., Iwamoto, M., Korenaga, T. (1999). Plant cis-acting regulatory DNA elements (PLACE) database: 1999. Nucleic Acids Res. 27, 297–300. doi: 10.1093/nar/27.1.297
Hileman, L. C., Sundstrom, J. F., Litt, A., Chen, M., Shumba, T., Irish, V. F. (2006). Molecular and phylogenetic analyses of the MADS-box gene family in tomato. Mol. Biol. Evol. 23, 2245–2258. doi: 10.1093/molbev/msl095
Ito, Y., Kitagawa, M., Ihashi, N., Yabe, K., Kimbara, J., Yasuda, J., et al. (2008). DNA-binding specificity, transcriptional activation potential, and the rin mutation effect for the tomato fruit-ripening regulator RIN. Plant J. 55, 212–223. doi: 10.1111/j.1365-313X
Ito, Y., Nishizawa-Yokoi, A., Endo, M., Mikami, M., Shima, Y., Nakamura, N., et al. (2017). Re-evaluation of the rin mutation and the role of RIN in the induction of tomato ripening. Nat. Plants 3, 866–874.
Kappé, G., Leunissen, J. A. M., de Jong, W. W. (2002). Evolution and diversity of prokaryotic small heat shock proteins. Small Stress Proteins 28, 1–17. doi: 10.1007/978-3-642-56348-5_1
Klee, H. J., Giovannoni, J. J. (2011). Genetics and control of tomato fruit ripening and quality attributes. Annu. Rev. Genet. 45, 41–59. doi: 10.1146/annurev-genet-110410-132507
Kumar, S., Stecher, G., Tamura, K. (2016). MEGA7: Molecular Evolutionary Genetics Analysis version 7.0 for bigger datasets. Mol. Biol. Evol. 33, 1870–1874. doi: 10.1093/molbev/msw054
Lescot, M., Déhais, P., Thijs, G., Marchal, K., Moreau, Y., Van de Peer, Y., et al. (2002). PlantCARE, a database of plant cis-acting regulatory elements and a portal to tools for in silico analysis of promoter sequences. Nucleic Acids Res. 30, 325–327. doi: 10.1093/nar/30.1.325
Liberek, K., Lewandowska, A., Zietkiewicz, S. (2008). Chaperones in control of protein disaggregation. EMBO J. 27, 328–335. doi: 10.1038/sj.emboj
Livak, K. J., Schmittgen, T. D. (2001). Analysis of relative gene expression data using real time quantitative PCR and the 2–ΔΔCT method. Methods 25, 402–408. doi: 10.1006/meth.2001.1262
Martel, C., Vrebalov, J., Tafelmeyer, P., Giovannoni, J. J. (2011). The tomato MADS-box transcription factor RIPENING INHIBITOR interacts with promoters involved in numerous ripening processes in a COLORLESS NONRIPENING-dependent manner. Plant Physiol. 157, 1568–1579. doi: 10.1104/pp.111.181107
Mascia, T., Santovito, E., Gallitelli, D., Cillo, F. (2010). Evaluation of reference genes for quantitative reverse-transcription polymerase chain reaction normalization in infected tomato plants. Mol. Plant Pathol. 11, 805–816. doi: 10.1111/j.1364-3703.2010
Mattoo, A. K., Upadhyay, R. K. (2019). “Plant hormones: Some glimpses on biosynthesis, signaling networks, and crosstalk,” in Sensory Biology of Plants. Ed. Sopory, S. (Singapore: Springer), 227–246. doi: 10.1007/978-981-13-8922-1_9
Mattoo, A. K., Vickery, R. S. (1977). Subcellular distributions of isoenzymes in fruits of a normal cultivar of tomato and of the rin mutant at two stages of development. Plant Physiol. 60, 496–498. doi: 10.1104/pp.60.4.496
Mehta, R. A., Cassol, T., Li, N., Ali, N., Handa, A. K., Mattoo, A. K. (2002). Engineered polyamine accumulation in tomato enhances phytonutrient content, juice quality, and vine life. Nat. Biotechnol. 20, 613–618. doi: 10.1038/nbt0602-613
Moore, S., Vrebalov, J., Payton, P., Giovannoni, J. (2002). Use of genomics tools to isolate key ripening genes and analyse fruit maturation in tomato. J. Exp. Bot. 53, 2023–2030. doi: 10.1093/jxb/erf057
Murakami, T., Matsuba, S., Funatsuki, H., Kawaguchi, K., Saruyama, H., Tanida, M., et al. (2004). Over-expression of a small heat shock protein, sHSP17.7, confers both heat tolerance and UV-B resistance to rice plants. Mol. Breed. 13, 165–175. doi: 10.1023/B:MOLB.0000018764.30795.c1
Neta-Sharir, I., Isaacson, T., Lurie, S., Weiss, D. (2005). Dual role for tomato heat shock protein 21: protecting photosystem II from oxidative stress and promoting color changes during fruit maturation. Plant Cell 17, 1829–1838. doi: 10.1105/tpc.105.031914
Ng, M., Yanofsky, M. F. (2001). Function and evolution of the plant MADS-box gene family. Nat. Rev. Genet. 2, 186–195. doi: 10.1038/35056041
Oeller, P. W., Lu, M. W., Taylor, L. P., Pike, D. A., Theologis, A. (1991). Reversible inhibition of tomato fruit senescence by antisense RNA. Science 254, 437–439. doi: 10.1126/science.1925603
Orzaez, D., Mirabel, S., Wieland, W. H., Granell, A. (2006). Agroinjection of Tomato Fruits. A tool for rapid functional analysis of transgenes directly in fruit. Plant Physiol. 140, 3–11. doi: 10.1104/pp.105.068221
Paul, A., Rao, S., Mathur, S. (2016). The α-Crystallin Domain Containing Genes: Identification, phylogeny and expression profiling in abiotic stress, phytohormone response and development in Tomato (Solanum lycopersicum). Front. Plant Sci. 7, 426. doi: 10.3389/fpls.2016.00426
Picton, S., Barton, S. L., Bouzayen, M., Hamilton, A. J., Grierson, D. (1993). Altered fruit ripening and leaf senescence in tomatoes expressing an antisense ethylene-forming enzyme transgene. Plant J. 3, 469–481. doi: 10.1111/j.1365-313X.1993.tb00167.x
Plesofsky-Vig, N., Vig, J., Brambl, R. (1992). Phylogeny of the α crystallin-related heat shock proteins. J. Mol. Evol. 35, 537–545. doi: 10.1007/BF00160214
Ré, M. D., Gonzalez, C., Escobar, M. R., Sossi, M. L., Valle, E. M., Boggio, S. B. (2016). Small heat shock proteins and the postharvest chilling tolerance of tomato fruit. Physiol. Plant 159, 148–160. doi: 10.1111/ppl.12491
Ramakrishna, W., Deng, Z., Ding, C., Handa, A. K., Ozminkowski, R. H. J. (2003). A novel small heat shock protein gene, vis1, contributes to pectin depolymerization and juice viscosity in tomato fruit. Plant Physiol. 131, 725–735. doi: 10.1104/pp.012401
Razdan, M. K., Mattoo, A. K. (2007). “Genetic improvement of Solanaceous crops,” in Tomato, vol. 2. (Enfield, UK: Science Publishers, Inc.). doi: 10.1017/S0014479707005443
Shukla, V., Upadhyay, R. K., Tucker, M. L., Giovannoni, J. J., Rudrabhatla, S. V., Mattoo, A. K. (2017). Transient regulation of three clustered tomato class-I small heat-shock chaperone genes by ethylene is mediated by SlMADS-RIN transcription factor. Sci. Rep. 7, 6474. doi: 10.1038/s41598-017-06622-0
Sobolev, A. P., Neelam, A., Fatima, T., Shukla, V., Handa, A. K., Mattoo, A. K. (2014). Genetic introgression of ethylene-suppressed transgenic tomatoes with higher-polyamines trait overcomes many unintended effects due to reduced ethylene on the primary metabolome. Front. Plant Sci. 5, 632. doi: 10.3389/fpls.2014.00632
Sun, W., Van Montagu, M., Verbruggen, N. (2002). Small heat shock proteins and stress tolerance in plants. Biochim. Biophys. Acta 1577, 1–9. doi: 10.1016/S0167-4781(02)00417-7
Tyedmers, J., Mogk, A., Bukau, B. (2010). Cellular strategies for controlling protein aggregation. Nat. Rev. Mol. Cell Biol. 11, 777–788. doi: 10.1038/nrm2993
Upadhyay, R. K., Mattoo, A. K. (2018). Genome-wide identification of tomato (Solanum lycopersicum L.) lipoxygenases coupled with expression profiles during plant development and in response to methyl-jasmonate and wounding. J. Plant Physiol. 231, 318–328. doi: 10.1016/j.jplph.2018.10.001.
Upadhyay, R. K., Gupta, A., Soni, D., Garg, R., Pathre, U. V., Nath, P., et al. (2017). Ectopic expression of a tomato DREB gene affects several ABA processes and influences plant growth and root architecture in an age-dependent manner. J. Plant Physiol. 214, 97–107. doi: 10.1016/j.jplph.2017.04.004
Vandesompele, J., De Preter, K., Pattyn, i., Poppe, B., Van Roy, N., De Paepe, A., et al. (2002). Accurate normalization of real-time quantitative RT-PCR data by geometric averaging of multiple internal control genes. Genome Biol. 3, 34–41. doi: 10.1186/gb-2002-3-7-research0034
Vrebalov, J. (2002). A MADS-Box gene necessary for fruit ripening at the tomato ripening-inhibitor (rin) locus. Science 296, 343–346. doi: 10.1126/science.1068181
Wang, W., Vinocur, B., Shoseyov, O., Altman, A. (2004). Role of plant heat-shock proteins and molecular chaperones in the abiotic stress response. Trends Plant Sci. 9, 244–252. doi: 10.1016/j.tplants.2004.03.006
Wang, R., Angenent, G. C., Seymour, G., de Maagd, R. A. (2020). Revisiting the role of master regulators in tomato ripening. Trends Plant Sci. 25, 291–301. doi: 10.1016/j.tplants.2019.11.005
Waters, E. R., Vierling, E. (1999). Chloroplast small heat shock proteins: evidence for atypical evolution of an organelle-localized protein. Proc. Natl. Acad. Sci. U.S.A. 96, 14394–14399. doi: 10.1073/pnas.96.25.14394
Waters, E. R. (2013). The evolution, function, structure, and expression of the plant sHSPs. J. Exp. Bot. 64, 391–403. doi: 10.1093/jxb/ers355
Wilkinson, J. Q., Lanahan, M. B., Yen, H., Giovannoni, J. J., Klee, H. J. (1995). An ethylene-inducible component of signal transduction encoded by never-ripe. Science 270, 14–16. doi: 10.1126/science.270.5243.1807
Yu, J., Cheng, Y., Feng, K., Ruan, M., Ye, Q., Wang, R., et al. (2016). Genome-wide identification and expression profiling of tomato Hsp20 Gene family in response to biotic and abiotic stresses. Front. Plant Sci. 7, 1215. doi: 10.3389/fpls.2016.01215
Zhang, J., Chen, H., Wang, H., Li, B., Yi, Y., Kong, F., et al. (2015). Constitutive expression of a tomato small heat shock protein gene LeHSP21 improves tolerance to high-temperature stress by enhancing antioxidation capacity in tobacco. Plant Mol. Biol. Rpt 34, 399–409. doi: 10.1007/s11105-015-0925-3
Keywords: gene expression, ethylene, 1-MCP, SlMADS-RIN, small heat shock protein genes, tomato, tomato ripening mutants
Citation: Upadhyay RK, Tucker ML and Mattoo AK (2020) Ethylene and RIPENING INHIBITOR Modulate Expression of SlHSP17.7A, B Class I Small Heat Shock Protein Genes During Tomato Fruit Ripening. Front. Plant Sci. 11:975. doi: 10.3389/fpls.2020.00975
Received: 15 April 2020; Accepted: 16 June 2020;
Published: 30 June 2020.
Edited by:
Carolina Andrea Torres, Washington State University, United StatesReviewed by:
Julia Vrebalov, Boyce Thompson Institute, United StatesNiranjan Chakraborty, National Institute of Plant Genome Research (NIPGR), India
Copyright © 2020 Upadhyay, Tucker and Mattoo. This is an open-access article distributed under the terms of the Creative Commons Attribution License (CC BY). The use, distribution or reproduction in other forums is permitted, provided the original author(s) and the copyright owner(s) are credited and that the original publication in this journal is cited, in accordance with accepted academic practice. No use, distribution or reproduction is permitted which does not comply with these terms.
*Correspondence: Autar K. Mattoo, YXV0YXIubWF0dG9vQHVzZGEuZ292